- CAS Engineering Laboratory for Nutrition, Shanghai Institute of Nutrition and Health, University of Chinese Academy of Sciences, Chinese Academy of Sciences, Shanghai, China
Selenium is recognized as an essential element for human health and enters human body mainly via diet. Selenium is a key constituent in selenoproteins, which exert essential biological functions, including antioxidant and anti-inflammatory effects. Several selenoproteins including glutathione peroxidases, selenoprotein P and selenoprotein S are known to play roles in the regulation of type 2 diabetes. Although there is a close association between certain selenoproteins with glucose metabolism or insulin resistance, the relationship between selenium and type 2 diabetes is complex and remains uncertain. Here we review recent advances in the field with an emphasis on roles of selenium on metabolism and type 2 diabetes. Understanding the association between selenium and type 2 diabetes is important for developing clinical practice guidelines, establishing and implementing effective public health policies, and ultimately combating relative health issues.
Introduction
Selenium is a natural chemical element existing in soil, water, and air. Selenium reaches the human body by food chain through incorporation into plants, animals, and aquatic organisms (1). From the public health perspective, selenium is treated as an essential micronutrient and is commonly used in dietary supplementation products widely consumed in western countries (2, 3). In human beings, the nutritional functions of selenium are achieved by 25 selenoproteins (4), with essential enzymatic functions, including hydroperoxide/phospholipid peroxide reduction (glutathione peroxidases, GPxs) (5, 6), thiol redox status regulation (thioredoxin reductases) (7), thyroid hormone activity regulation (iodothyronine deiodinases, Dios) (8), selenium transport (selenoprotein P, SelP) (9) and some are yet to be determined. Selenium is reported to play a role in maintaining redox balance, anti-cancer, and improving immunity, and is closely related to Keshan disease, diabetes, mental disorder, inflammation, and infections (10, 11).
Diabetes is a highly costly chronic disease (12), with an estimated worldwide prevalence of 463 million adults according to the 2019 report of the International Diabetes Federation Diabetes Atlas (IDF) (13). Type 2 diabetes accounts for 90% of all diabetes and is characterized by defective insulin secretion and/or insulin resistance (14). Although the mechanisms of insulin resistance and type 2 diabetes remain not fully understood, accumulating evidence suggests that oxidative stress plays an important role in both onset and progress (15). As several selenoproteins have the potential to protect the body from oxidative stress, selenium is expected to be protective against type 2 diabetes (10, 16). However, recent evidence raised concerns that a high level of selenium exposure may be associated with an increased risk of type 2 diabetes (17–20).
Even though how selenium affects the risk of type 2 diabetes is conflicting, evidence has confirmed that people with low status may benefit from additional selenium intake (21, 22). Although, selenium is assumed to be helpful in the prevention and therapy of type 2 diabetes (23, 24), selenium intake, including selenium supplementation, should be excluded for primary or secondary diabetes prevention in populations with adequate selenium status (25). This review focuses on the association of dietary selenium with type 2 diabetes epidemiology and discusses the major selenoproteins in the regulation of glucose and lipid metabolism and their implication in the development of type 2 diabetes.
Selenium in food
Diet is a major source of selenium for humans and the content of selenium in foods varies greatly (Figure 1). The main source of selenium in diet are cereals because of the large amounts consumed as well as meats and seafood with high protein contents (26). Also, plant-based sources of selenium, including wheat, pearl millet, and maize, are more effective in reversing the deleterious effects of selenium deficiency (27). Natural fruits generally contain low amounts of selenium, rarely exceeding 10 μg/kg and vegetables with a maximum concentration of 6 μg/kg (28). From the selected investigation, the main food sources in diet of selenium intake include cereals, meat products, milk and dairy products, beverages, fish and seafood (29–31). All these groups provide more than 85% of the selenium intake. In addition, ready-to-eat meals, vegetables, fruits, sweets, and beverages contribute to a small part of the dietary selenium intake. Processing technology could affect selenium content in food and bioaccessibility of selenium species, among which soaking, fermentation were reported to increase the bioaccessible selenium content and heating declined the bioaccessibility of SeMet and SeCys (32, 33). Another selenium source of exposure is well-known as selenium supplementation. Considering the low abundance of selenium in daily foods, consuming a diet with natural selenium concentrations is not abundant. Hence, scientific works have dealt with selenium-biofortification strategies to obtain selenium-enriched food or feed, via plant cultivation (soil fertilization with inorganic selenium), animal feeding (with selenium-enriched plants), microorganism transformation (fermentation) (34, 35). Asides from the classical methods, pharmacological products and nano-selenium applications can also increase the selenium concentration in the human body (36, 37).
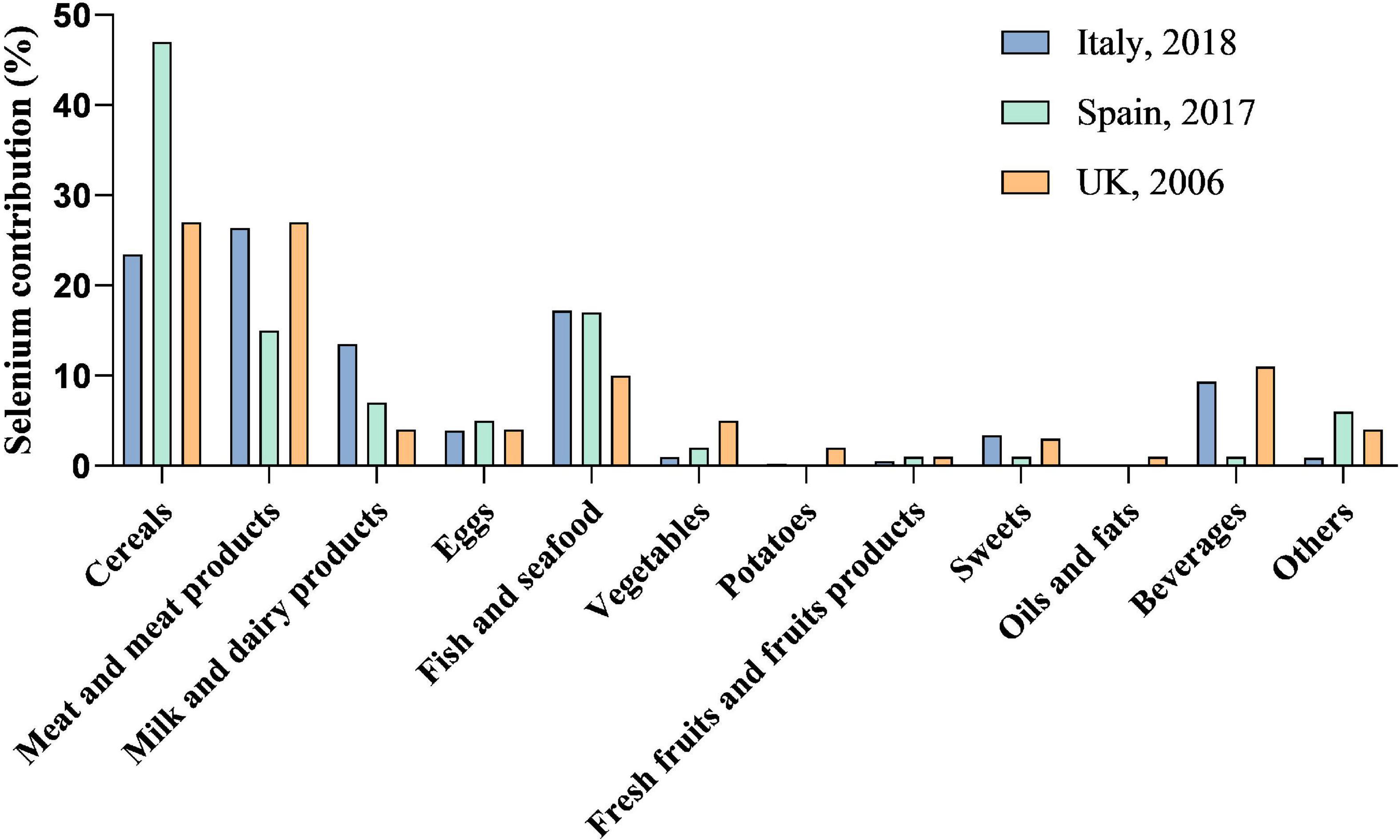
Figure 1. Selenium contribution to total dietary selenium intake. Data for selenium contribution to total dietary selenium intake are adapted from published studies. Diet is the main source of selenium for human beings. The species of food, selenium content in food, as well as the amount taken in diet affect the daily selenium intake and selenium status.
Not only the total intake of dietary selenium but also the selenium species ingested is important to human health (38). Selenium exists in inorganic and organic forms and intakes of different foods are correlated with different types of selenium species. Organic selenium forms in foods mainly contain selenomethionine (SeMet), selenocysteine (SeCys), selenium-methylselenocysteine (SeMeCys), and gamma-glutamyl-Se-methylselenocysteine (GGMSC), and drinking water mainly contains inorganic selenium species such as selenate and selenite (36, 39). Efficient uptake and metabolism of dietary selenium primarily depend on its chemical forms (40). Organic selenium is more easily absorbed by the human body compared with inorganic selenium (41), and more than 90% of SeMet is absorbed in human body but only about 50% of selenium is from selenite. In addition, nano-selenium attracts growing attention due to its high chemical stability, biocompatibility, and low toxicity (42, 43). Nano-selenium has been applied as antioxidants, dietary supplements, antidiabetic agents (37, 44).
Selenium has long been termed “an essential poison” as selenium doses exceeding 400 μg/day may exert toxic actions according to the World Health Organization (WHO) (45). The recommended daily allowance (RDA) of selenium varies hugely depending on the geographical area, ranging from 25 μg/day for adult women in Japan up to 100 μg/day in the Netherlands and Macedonia, but most RDA levels are in the range of 50–60 μg/day. Both selenium deficiency and excess have been associated with adverse health effects, and the health effects of selenium are recognized as the inextricable U-shaped link with status (10). The selenium content in diabetes serum is commonly lower than normal ones, while additional concerns were raised about the diabetes risk associated with selenium intake above the RDA (55 μg/day) (46). Overall, selenium exposure adds to type 2 diabetes risk across a wide range, especially above dietary intake of 80 μg/day and blood selenium of 120 μg/L (17). Whereas people with low selenium status may benefit from additional selenium intake, those with adequate-to-high selenium status might be affected adversely and should not take selenium supplements.
Selenium in metabolism
Selenoproteins
The biological actions of selenium are mainly mediated by selenoproteins. Selenium is integrated into selenoproteins in the form of selenocysteine. To date, 25 selenoproteins have been identified in humans, among which glutathione peroxidase 1 (GPx1), selenoprotein P (SelP), selenoprotein S (SelS), selenoprotein V (SelV), and iodothyronine deiodinases (Dios) have been reported to associate with glucose and lipid homeostasis (Table 1) (47, 48).
GPx1 is localized in the cytosol and mitochondria. This enzyme can catalyze the reduction of hydrogen peroxide (H2O2) and lipid hydroperoxides using GSH as a reducing cofactor (59). GPx1 is highly sensitive to changes in both selenium status and oxidative stress conditions (60). GPx1 is involved in regulating insulin synthesis and secretion, insulin sensitivity, glucose and lipid homeostasis and the onset and progression of diabetes (61). SelP functions as a selenium-transport protein to deliver selenium from the liver to other tissues to maintain appropriate selenium levels in tissues. Selenium is necessary for the synthesis of antioxidative selenoproteins. Thus, SelP plays an important role in the cellular antioxidative system by maintaining these selenoproteins. Furthermore, SelP possesses multifunctional properties such as GPx-like antioxidant enzyme activity (62), peroxynitrite scavenging (63), and metal-binding activity (64). SelS is resident in the endoplasmic reticulum (ER). It is involved in regulating oxidative stress, ER stress, and inflammatory response (51, 52, 65). SelS plays roles in antioxidant protection and anti-ER stress in the pancreas and blood vessels, while it promotes insulin resistance in the liver, adipose tissue, and skeletal muscle (51, 66). SelV is highly expressed in the testis (mainly in seminiferous tubules). It is involved in regulating body selenium metabolism and lipid metabolism (56, 57). Dios, including Dio1, Dio2, and Dio3, are thyroid hormone-regulating iodothyronine deiodinase. Dios are expressed in multiple tissues. Dio1 and Dio3 are located in the plasma membrane and Dio2 is located in the endoplasmic reticulum membrane. Dios play an important role in thyroid hormone signaling involving many key reactions in energy homeostasis and individual growth and development (58, 67).
Glucose metabolism
Selenoproteins are involved in regulating glucose metabolism. Early studies found that inorganic selenium showed an insulin-like effect. Sodium selenate promoted glucose transport and glucose metabolism through the mitogen-activated protein/myelin basic protein kinases (MAPK) and ribosomal S6 protein kinases in rat adipocytes at very high doses (68–70).
Most selenoproteins are antioxidant enzymes and play roles in maintaining insulin secretion with their antioxidant activity. SelP is relatively highly expressed in pancreatic islets, which acts as an antioxidant to protect β cells (42). GPx1 can degrade intracellular H2O2 (59). In pancreatic islets, GPx1 reduces the damage of H2O2 on β cells and promotes the normal secretion of insulin.
SelP synthesis is regulated like a gluconeogenic enzyme in the liver. SelP gene expression is regulated by the interaction of the transcription factors FoxO1 and HNF-4α with the co-activator PGC-1α (71, 72). These transcription factors similarly control the expression of gluconeogenic enzymes: G6PC (glucose-6-phosphatase, G6Pase, catalytic subunit) and PCK1 (phosphoenolpyruvate carboxykinase, PEPCK), which are involved in hepatic glucose release to adapt to feeding and fasting (73, 74). It was found that liver SelP mRNA levels have been shown to increase during fasting and decrease after feeding in mice, which indicated that the liver could fine-tune SelP secretion according to the nutritional state (72). Furthermore, SelP transcription can be inhibited by insulin via the PI3K/Akt/FoxO1 pathway (72, 75). Insulin-induced phosphorylation of FoxO1 results in its nuclear exclusion and inhibition of FoxO1-dependent transcription of SelP in liver cells (71). Thus, insulin-mediated regulation of hepatic SelP production and secretion represents a physiological link between selenium homeostasis and carbohydrate metabolism (71).
Selenium deficient diet results in impaired islet function, low insulin secretion, and high blood glucose (76, 77). The deficiency of selenium decreases the expression of several selenoprotein genes and proteins in different tissues, which may dysregulate glucose homeostasis. It was reported that GPx1 deficiency induced type 1 diabetes-like phenotype (61). GPx1-knockout-mice developed islets β cell damage and insulin reduction (78, 79). Hepatic-specific deletion of SelS in mice caused obesity, hepatic steatosis, insulin resistance, and disturbed glucose homeostasis (66, 80). It was reported that reduced synthesis of selenoproteins, including GPx1 and MsrB1, caused by overexpression of an i(6)A(-) mutant selenocysteine tRNA promoted glucose intolerance and led to a diabetes-like phenotype (81). Selenocysteine lyase (Scly) is the enzyme that supplies selenium for selenoprotein biosynthesis via decomposition of the amino acid selenocysteine (82). Moreover, it was found that Scly knockout mice fed with low selenium dietary reduced GPx1 and SelS protein levels and affected hepatic glucose homeostasis (83). Taken together, selenium and selenoproteins play important roles in glucose metabolism, especially in maintaining a redox balance to promote the normal synthesis and secretion of insulin.
Lipid metabolism
Selenoproteins are also involved in regulating hepatic lipid accumulation. It was reported that SelS expression was down-regulated in the liver in high-fat diet (HFD)-fed mice and db/db mice, and SelS expression levels were reduced in the PA-induced primary hepatocytes (66).
Hepatic triglyceride synthesis consists of fatty acid uptake and de novo lipogenesis (84, 85). It was reported that serum free fatty acids level was elevated in hepatocyte-specific SelS knockout (SelSH-KO) mice, and the expression levels of cluster of differentiation 36 (CD36), fatty acid transport protein 2 (FATP2) and fatty acid transport protein 5 (FATP5), which are involved in fatty acid uptake, were markedly increased in the liver of SelSH-KO mice (66). On the other hand, the expression levels of peroxisome proliferator-activated receptor α (PPARα), carnitine palmitoyltransferase 2 (CPT2), and acyl-coenzyme A oxidase 1 (ACOX1), which are responsible for fatty acid oxidation, were down-regulated in SelSH-KO mice. These results suggested that hepatic SelS deletion increased hepatic triglyceride and diacylglycerol accumulation via promoting fatty acid uptake and reducing fatty acid oxidation (66).
FGF21 is an endocrine hepatokine produced predominantly in the liver (86, 87). Hepatic-specific deletion of SelS (SelS LKO) decreased the production of hepatokine FGF21 and adipokine adiponectin and increased adipose tissue size. These results indicated that the FGF21-adiponectin axis was inhibited in SelS LKO mice, which exacerbated hepatic metabolic disorders (66). Taken together, these studies show that selenoproteins participate in regulating lipid metabolism, especially in lipid intake and fatty acid oxidation, which indicates that selenoproteins may be a potential intervention target for lipid metabolic disorders.
Epidemiology of selenium and type 2 diabetes
Selenium is expected to protect against type 2 diabetes because of the potential of several selenoproteins to protect against oxidative stress (19, 88, 89). Selenium intake varies greatly among countries due to the selenium differences in local soil and foods consumed (Figure 2). The relationship between selenium level and the prevalence of type 2 diabetes is possibly U-shaped, with possible adverse effects occurring both below and above the physiological range for optimal activity of some or all selenoproteins (90). Whereas dietary selenium supplement has been applied to improve glucose metabolism, accumulating evidence showed that exposure to a high level of selenium increased the risk of type 2 diabetes. In a multivariate logistic regression model, an increase of 10 μg/L in selenium induced to the prevalence of diabetes mellitus by 12% (91), showing a dose-dependent relationship between selenium level and diabetes. Higher serum selenium was discovered to be linked with increased plasma glucose levels and glycosylated hemoglobin levels (92). Intake of high-level selenium might affect the expression and(or) function of key regulators for glycolysis, gluconeogenesis, and lipogenesis (20). Furthermore, the association between selenium and type 2 diabetes was independent of insulin resistance at high serum selenium levels (19, 91). The prevalence of diabetes, as well as glucose and glycosylated hemoglobin levels, increased with increasing selenium concentrations up to 140 μg/L of selenium exposure (93), while several studies insisted on a risk serum selenium level of 160 μg/L (17, 94). In addition, the non-experimental studies reached agreement with the findings from randomized controlled trials, illustrated that selenium exposure from moderate to high levels is associated with increased risk for type 2 diabetes. Further research is required to clarify the optimal range of selenium intake and status for minimizing the potential adverse effects on glucose metabolism and preventing type 2 diabetes.
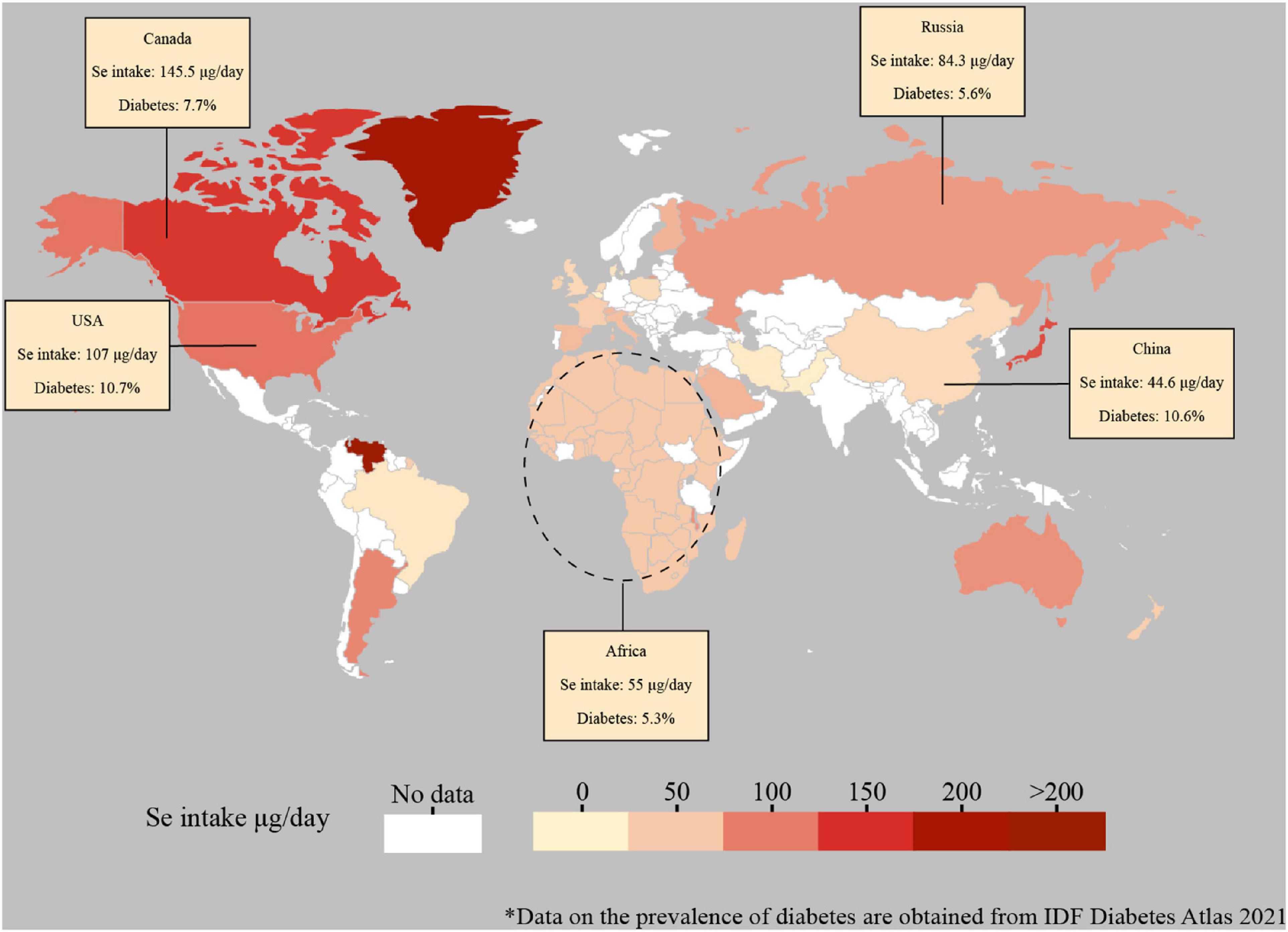
Figure 2. Global estimated daily intake of selenium and prevalence of diabetes. Data for the global estimated daily selenium intake are adapted from the published studies (95-102). The selenium data of mainland China was selected. The selenium data of Africa was adapted from the Africa selenium daily supplied amount. Data for the prevalence of diabetes (data included until 2021) are derived from the Diabetes Atlas of the International Diabetes Federation (https://diabetesatlas.org/data/en/indicators/2/). Dashed lines are not supposed to accurately represent regions.
Mechanisms of selenium in type 2 diabetes
Type 2 diabetes is characterized by defective insulin secretion and/or insulin resistance, and the potential molecular mechanisms include interference of oxidative stress, insulin signaling, gluconeogenesis, and endoplasmic reticulum (ER) stress (Figure 3). Abnormal selenium status affects the occurrence and development of diabetes through these mechanisms.
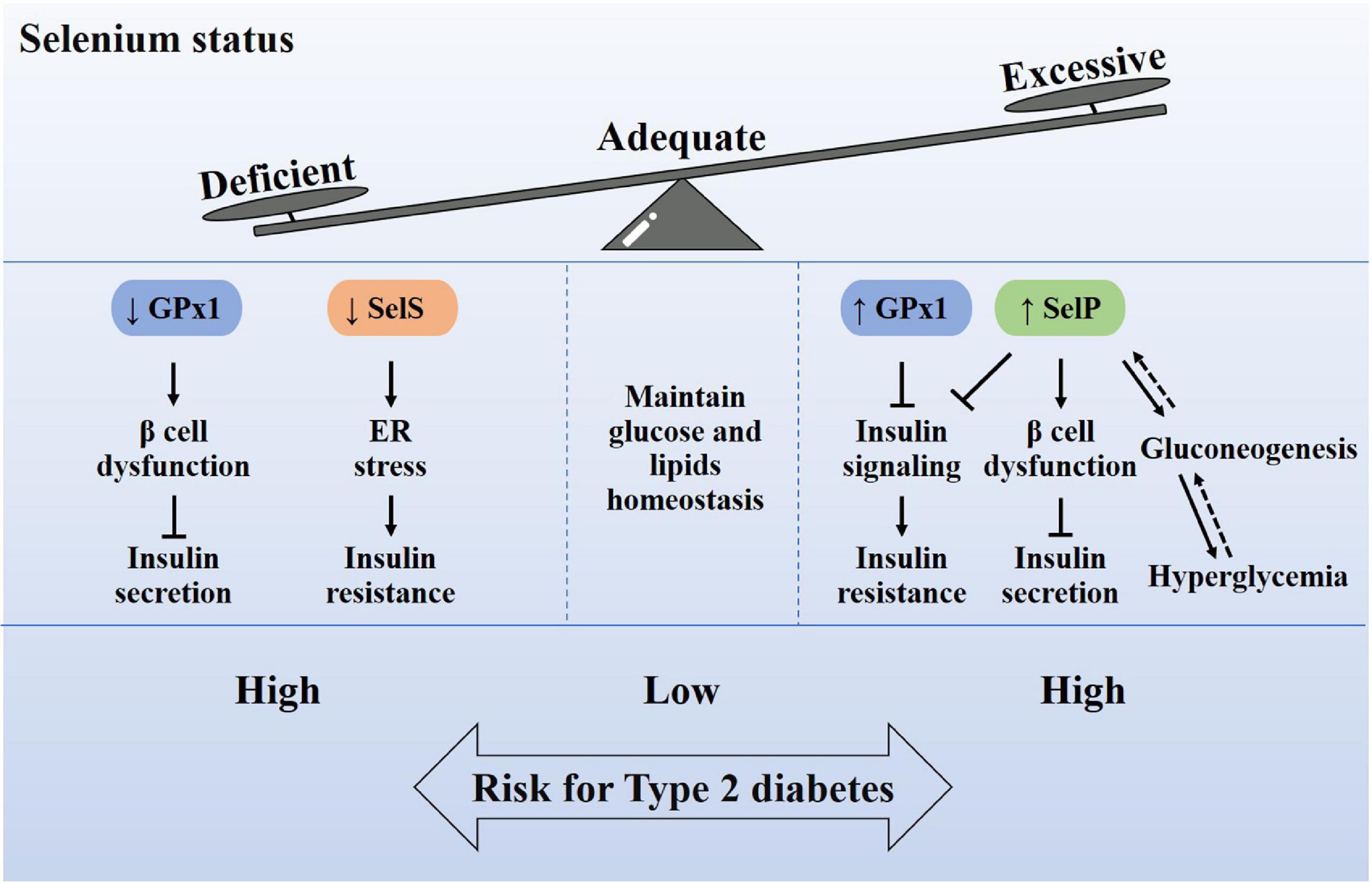
Figure 3. Relationship between selenium homeostasis and type 2 diabetes. Adequate selenium intake is very important for maintaining the homeostasis of glucose and lipid metabolism. Excessive or insufficient selenium intake will cause the increase or decrease of selenoproteins in the body, which in turn leads to a high risk of type 2 diabetes. The abnormal content of selenoproteins, including Gpx1, SelP, and SelS, may cause oxidative damage of β cells, insulin signaling impairment, endoplasmic reticulum stress and gluconeogenesis, which induce insulin secretion defects or insulin resistance. Therefore, it is recommended to supplement selenium according to the state of selenium.
β cells and pancreas
Pancreas β cells have a fragile antioxidant system. The expression levels of antioxidant enzymes in pancreatic islets are substantially lower compared with various other tissues, which renders β cells sensitive toward oxidative and nitrosative stress (103). Severe antioxidant selenoproteins deficiency may result in oxidative damage of β cells and lower insulin secretion. GPx1-knockout-mice fed a high-fat diet for 12 weeks decreased plasma insulin and glucose-induced insulin secretion (78, 79). It was reported that GPx1-knockout-mice elevated islet superoxide and hydroperoxide production and up-regulated p53 phosphorylation. By contrast, after overexpressing GPx1 in pancreatic β cells, C57BLKS/J mice were protected from the β cell damage when stimulated with streptozotocin, and db/db mice exhibited reversed hypoinsulinemia and hyperglycemia (104). However, global overexpression of GPx1 induced obesity, hyperglycemia, insulin resistance in mice, and developed type 2 diabetes-like phenotypes (105–107), which infered adverse effects of excessive selenoprotein biosynthesis and the complexity of redox status. The results of SelP further prove this point. It has been shown that excess SelP impairs the function of pancreatic β cells and decreases insulin secretion (50, 108). The injection of purified human SelP protein in mice resulted in a decrease in insulin levels, a decline of β cells and α-cells in the pancreas, and also a rearrangement of the position of these cells in the pancreatic islets (50). Furthermore, the administration of SelP-neutralizing antibodies could improve insulin secretion and glucose intolerance in a mouse model of diabetes (50). Thus, selenium homeostasis and redox balance are extremely important for β cells and insulin secretion.
Insulin signaling
The antioxidant activity of selenoproteins can protect the islets from oxidative stress, but excessive antioxidant activity is not beneficial for insulin signaling. The binding of insulin to its receptor activates NADPH oxidase enzymes and results in the production of space H2O2 (109). These small amounts space of H2O2 can act as second messengers and are required to deactivate two insulin-signaling inhibitors: tyrosine phosphatase 1B (PTP-1B) and phosphatase and tensin homolog protein (PTEN). This H2O2-mediated deactivation is considered to enhance the insulin-induced PI3K/Akt signaling, which facilitates glucose uptake, inhibits glycogen synthesis, and suppresses gluconeogenesis (110). GPx1 can degrade intracellular H2O2 and regulate its concentration (59). However, when intracellular physiological H2O2 is eliminated by excessive activity of GPx1, insulin signaling may be impaired. In this regard, GPx1-knockout-mice were protected from insulin resistance induced by a high-fat diet due to increased H2O2 production and inactivation of PTEN (111). Conversely, mice over-expressing GPx1 exhibited insulin resistance and hyperinsulinemia (105–107). Therefore, the appropriate amount of selenium and selenoproteins will benefit insulin function. Based on the control of SelP transcription through PGC-1α/FoxO1/HNF-4α, it was found insulin could inhibit SelP transcription by the PI3K/Akt/FoxO1 pathway (75). High levels of SelP impaired insulin signaling and dysregulated glucose metabolism both in the liver and muscle via the inactivation of adenosine monophosphate-activated protein kinase (AMPK) (72, 112). So, SelP has been identified as a “hepatokine” that induces insulin resistance and excess SelP promotes type 2 diabetes (50, 108). Thus, a high level of selenoproteins may impair insulin sensitivity through the interference of the insulin signaling cascade.
Gluconeogenesis
Plasma SelP levels were reported elevated in patients with type 2 diabetes, and there was an association between high plasma selenium and fasting plasma glucose in type 2 diabetes patients (47, 113, 114). SelP and gluconeogenic enzyme gene expression are similarly regulated by methylation of the same transcription factors (71, 72). SelP, together with G6PC and PCK1 is transcribed through PGC-1α/FoxO1/HNF-4α. Under the normal metabolic condition, insulin inactivates the transcription of SelP and gluconeogenic enzymes. Under the condition of high glucose and insulin resistance, the dysregulated transcriptional activity of FoxO1 enhances the biosynthesis of SelP and gluconeogenic enzymes, which results in elevated plasma SelP and selenium levels and further elevated plasma glucose levels (72, 115, 116). Thus, from this point, elevated plasma SelP levels might be considered as the result rather than the cause of hyperglycemia and insulin resistance (117).
Endoplasmic reticulum stress
Chronic endoplasmic reticulum (ER) stress affects glucolipid metabolism, which is crucial to the occurrence and development of insulin resistance and nonalcoholic fatty liver disease (NAFLD) (118, 119). ER stress is induced by unfolded or misfolded proteins accumulated in the ER, which initiates unfolded protein response (UPR) to restore homeostasis in the ER. ER-associated protein degradation (ERAD) is activated to remove unfolded or misfolded proteins (120). Selenoproteins, such as SelS and SelK, are induced under ER stress, which play important roles in ERAD and ER stress. Mechanistically, SelS forms a multiprotein complex with degradation in endoplasmic reticulum protein 1 (Derlin1)-ubiquitin ligase E3-p97ATPase and SelK to participate in ERAD, which mediates misfolded proteins in the ER to be translocated back into the cytosol for degradation by the proteasome (121–123). Consistently, knockdown of SelS increased the expression of ER stress marker genes (124, 125), whereas its overexpression protected against ER stress injury in hepatocytes and cell lines (65, 66, 125, 126). Consistently, ER stress was increased in SelS-hepatic-knockout mice and SelS-knockdown hepatocytes, but suppressed in SelS-overexpress hepatocytes (66). It was likely that excessive misfolded or unfolded proteins were accumulated in SelS deficiency hepatocytes due to impaired ERAD capability, resulting in chronic ER stress (66). Collectively, these results show evidence supporting that SelS has the potential to reduce ER stress injury and may protect hepatocytes from the development of insulin resistance and hepatic steatosis.
Conclusion and future perspectives
In conclusion, we systemically review the role of selenium and selenoproteins in type 2 diabetes and indicate the therapeutic potential of selenium supplementation in the treatment of metabolic disorders. Even though the interaction of some other selenoproteins with type 2 diabetes has not been verified, their effective roles in the regulation of glucose and lipid metabolism are becoming increasingly clear. Although there have been some inconsistent results, extensive evidence has suggested that selenium supplementation is beneficial for preventing and treating several chronic diseases (127). Future studies are needed to explore the association between selenium exposure and metabolic effects in more details with selenium exposure, and the potential mechanisms.
Selenium supply is very important for maintaining glucose and lipid homeostasis in healthy adults and patients with type 2 diabetes. However, the epidemiology of observational and experimental studies of selenium in type 2 diabetes reveal that both selenium deficiency and severe excess lead to insulin resistance and β cell dysfunction, with potential molecular mechanisms including interference of oxidative stress, insulin signaling, gluconeogenesis, and ER stress. Thus, selenium should be supplemented according to the status of selenium, while excessive selenium supplement is not recommended.
Nutrigenetic research has identified several single nucleotide polymorphisms in selenoproteins, which may clarify the high variability of selenium nutritional status in different populations (34, 43) and influence metabolic parameters in response to selenium supplementation (44). Thus, more personalized nutritional recommendations are needed to consider not only the regional particularities but also the genetic characteristics of the population or individuals.
Author contributions
JZ, HZ, and YL researched data for the manuscript, made substantial contributions to discussions of the content, and wrote the manuscript. YH and XW edited the manuscript with important intellectual content. All authors reviewed and edited the manuscript before submission.
Funding
This work was supported by grants from National Key R&D Program of China (2019YFA0802502), National Natural Science Foundation of China (81925008 and 32130047), Open Project Program of Metabolic Vascular Diseases Key Laboratory of Sichuan Province (2022MVDKL-K2) to YL.
Conflict of interest
The authors declare that the research was conducted in the absence of any commercial or financial relationships that could be construed as a potential conflict of interest.
Publisher’s note
All claims expressed in this article are solely those of the authors and do not necessarily represent those of their affiliated organizations, or those of the publisher, the editors and the reviewers. Any product that may be evaluated in this article, or claim that may be made by its manufacturer, is not guaranteed or endorsed by the publisher.
References
1. Galan-Chilet I, Tellez-Plaza M, Guallar E, De Marco G, Lopez-Izquierdo R, Gonzalez-Manzano I, et al. Plasma selenium levels and oxidative stress biomarkers: a gene-environment interaction Population-Based study. Free Radic Biol Med. (2014) 74:229–36. doi: 10.1016/j.freeradbiomed.2014.07.005
2. Yang H, Yang X, Ning Z, Kwon SY, Li ML, Tack FMG, et al. The beneficial and hazardous effects of selenium on the health of the soil-plant-human system: an overview. J Hazard Mater. (2022) 422:126876. doi: 10.1016/j.jhazmat.2021.126876
3. Wang N, Tan HY, Li S, Xu Y, Guo W, Feng Y. Supplementation of micronutrient selenium in metabolic diseases: its role as an antioxidant. Oxid Med Cell Longev. (2017) 2017:7478523. doi: 10.1155/2017/7478523
4. Kryukov GV, Castellano S, Novoselov SV, Lobanov AV, Zehtab O, Guigo R, et al. Characterization of mammalian selenoproteomes. Science. (2003) 300:1439–43. doi: 10.1126/science.1083516
5. Lubos E, Loscalzo J, Handy DE. Glutathione peroxidase-1 in health and disease: from molecular mechanisms to therapeutic opportunities. Antioxid Redox Sign. (2011) 15:1957–97. doi: 10.1089/ars.2010.3586
6. Ingold I, Berndt C, Schmitt S, Doll S, Poschmann G, Buday K, et al. Selenium utilization by GPX4 is required to prevent hydroperoxide-induced ferroptosis. Cell. (2018) 172:409–22.e21. doi: 10.1016/j.cell.2017.11.048
7. Arner ES. Focus on mammalian thioredoxin reductases–important selenoproteins with versatile functions. Biochim Biophys Acta. (2009) 1790:495–526. doi: 10.1016/j.bbagen.2009.01.014
8. Darras VM, Van Herck SL. Iodothyronine deiodinase structure and function: from ascidians to humans. J Endocrinol. (2012) 215:189–206. doi: 10.1530/JOE-12-0204
9. Burk RF, Hill KE. Selenoprotein P-expression, functions, and roles in mammals. Biochim Biophys Acta. (2009) 1790:1441–7. doi: 10.1016/j.bbagen.2009.03.026
10. Rayman MP. Selenium and human health. Lancet. (2012) 379:1256–68. doi: 10.1016/S0140-6736(11)61452-9
11. Roman M, Jitaru P, Barbante C. Selenium biochemistry and its role for human health. Metallomics. (2014) 6:25–54. doi: 10.1039/c3mt00185g
12. Peter P, Lipska K. The rising cost of diabetes care in the USA. Lancet Diabetes Endocrinol. (2016) 4:479–80. doi: 10.1016/s2213-8587(15)00519-7
13. International Diabetes Federation [IDF]. IDF Atlas. 9th ed. Brussels: International Diabetes Federation (2019).
14. Stumvoll M, Goldstein BJ, van Haeften TW. Type 2 diabetes: principles of pathogenesis and therapy. Lancet. (2005) 365:1333–46. doi: 10.1016/s0140-6736(05)61032-x
15. Houstis N, Rosen ED, Lander ES. Reactive oxygen species have a causal role in multiple forms of insulin resistance. Nature. (2006) 440:944–8. doi: 10.1038/nature04634
16. Weekley CM, Harris HH. Which form is that? The importance of selenium speciation and metabolism in the prevention and treatment of disease. Chem Soc Rev. (2013) 42:8870–94. doi: 10.1039/c3cs60272a
17. Vinceti M, Filippini T, Wise LA, Rothman KJ. A systematic review and dose-response meta-analysis of exposure to environmental selenium and the risk of type 2 diabetes in nonexperimental studies. Environ Res. (2021) 197:111210. doi: 10.1016/j.envres.2021.111210
18. Stranges S, Marshall JR, Natarajan R, Donahue RP, Trevisan M, Combs GF, et al. Effects of long-term selenium supplementation on the incidence of type 2 diabetes. Ann Intern Med. (2007) 147:217–23. doi: 10.7326/0003-4819-147-4-200708210-00175
19. Lu CW, Chang HH, Yang KC, Kuo CS, Lee LT, Huang KC. High serum selenium levels are associated with increased risk for diabetes mellitus independent of central obesity and insulin resistance. BMJ Open Diabetes Res Care. (2016) 4:e000253. doi: 10.1136/bmjdrc-2016000253
20. Zhou J, Huang K, Lei XG. Selenium and diabetes–evidence from animal studies. Free Radic Biol Med. (2013) 65:1548–56. doi: 10.1016/j.freeradbiomed.2013.07.012
21. Rayman MP, Blundell-Pound G, Pastor-Barriuso R, Guallar E, Steinbrenner H, Stranges S. A randomized trial of selenium supplementation and risk of type-2 diabetes, as assessed by plasma adiponectin. PLoS One. (2012) 7:e45269. doi: 10.1371/journal.pone.0045269
22. Wang XL, Yang TB, Wei J, Lei GH, Zeng C. Association between serum selenium level and type 2 diabetes mellitus: a non-linear dose-response meta-analysis of observational studies. Nutr J. (2016) 15:48. doi: 10.1186/s12937-016-0169-6
23. Faure P. Protective effects of antioxidant micronutrients (vitamin E, zinc and selenium) in type 2 diabetes mellitus. Clin Chem Lab Med. (2003) 41:995–8. doi: 10.1515/CCLM.2003.152
24. Faure P, Ramon O, Favier A, Halimi S. Selenium supplementation decreases nuclear factor-kappa B activity in peripheral blood mononuclear cells from type 2 diabetic patients. Eur J Clin Invest. (2004) 34:475–81. doi: 10.1111/j.1365-2362.2004.01362.x
25. Bleys J, Navas-Acien A, Guallar E. Serum selenium and diabetes in U.S. adults. Diabetes Care. (2007) 30:829–34. doi: 10.2337/dc06-1726
26. Hurst R, Collings R, Harvey LJ, King M, Hooper L, Bouwman J, et al. EURRECA-estimating selenium requirements for deriving dietary reference values. Crit Rev Food Sci Nutr. (2013) 53:1077–96. doi: 10.1080/10408398.2012.742861
27. Khanam A, Platel K. Bioavailability and bioactivity of selenium from wheat (Triticum aestivum), maize (Zea mays), and pearl millet (Pennisetum glaucum), in selenium-deficient rats. J Agric Food Chem. (2019) 67:6366–76. doi: 10.1021/acs.jafc.9b02614
28. Mehdi Y, Hornick JL, Istasse L, Dufrasne I. Selenium in the environment, metabolism and involvement in body functions. Molecules. (2013) 18:3292–311. doi: 10.3390/molecules18033292
29. Rose M, Baxter M, Brereton N, Baskaran C. Dietary exposure to metals and other elements in the 2006 UK Total Diet Study and some trends over the last 30 years. Food Addit Contam Part A Chem Anal Control Expo Risk Assess. (2010) 27:1380–404. doi: 10.1080/19440049.2010.496794
30. Filippini T, Cilloni S, Malavolti M, Violi F, Malagoli C, Tesauro M, et al. Dietary intake of cadmium, chromium, copper, manganese, selenium and zinc in a Northern Italy community. J Trace Elem Med Biol. (2018) 50:508–17. doi: 10.1016/j.jtemb.2018.03.001
31. Olza J, Aranceta-Bartrina J, Gonzalez-Gross M, Ortega RM, Serra-Majem L, Varela-Moreiras G, et al. Reported dietary intake and food sources of zinc, selenium, and vitamins A, E and C in the Spanish population: findings from the ANIBES study. Nutrients. (2017) 9:697. doi: 10.3390/nu9070697
32. Khanam A, Platel K. Bioaccessibility of selenium, selenomethionine and selenocysteine from foods and influence of heat processing on the same. Food Chem. (2016) 194:1293–9. doi: 10.1016/j.foodchem.2015.09.005
33. Khanam A, Platel K. Influence of domestic processing on the bioaccessibility of selenium from selected food grains and composite meals. J Food Sci Technol. (2016) 53:1634–9. doi: 10.1007/s13197-015-2075-x
34. Donadio JLS, Rogero MM, Guerra-Shinohara EM, Barbosa F Jr., Desmarchelier C, Borel P, et al. Genetic variants in selenoprotein genes modulate biomarkers of selenium status in response to Brazil nut supplementation (the SU.BRA.NUT study). Clin Nutr. (2019) 38:539–48. doi: 10.1016/j.clnu.2018.03.011
35. Wan J, Zhang M, Adhikari B. Advances in selenium-enriched foods: from the farm to the fork. Trends Food Sci Tech. (2018) 76:1–5. doi: 10.1016/j.tifs.2018.03.021
36. Navarro-Alarcon M, Cabrera-Vique C. Selenium in food and the human body: a review. Sci Total Environ. (2008) 400:115–41. doi: 10.1016/j.scitotenv.2008.06.024
37. Chen J, Feng T, Wang B, He R, Xu Y, Gao P, et al. Enhancing organic selenium content and antioxidant activities of soy sauce using nano-selenium during soybean soaking. Front Nutr. (2022) 9:970206. doi: 10.3389/fnut.2022.970206
38. Rayman MP, Infante HG, Sargent M. Food-chain selenium and human health: spotlight on speciation. Br J Nutr. (2008) 100:238–53. doi: 10.1017/S0007114508922522
39. Rayman MP. Food-chain selenium and human health: emphasis on intake. Br J Nutr. (2008) 100:254–68. doi: 10.1017/S0007114508939830
40. Fra̧czek A, Pasternak K. Selenium in medicine and treatment. J Elementol. (2013) 18:145–63. doi: 10.5601/jelem.2013.18.1.13
41. Kieliszek M. Selenium-fascinating microelement, properties and sources in food. Molecules. (2019) 24:1298. doi: 10.3390/molecules24071298
42. Steinbrenner H, Hotze AL, Speckmann B, Pinto A, Sies H, Schott M, et al. Localization and regulation of pancreatic selenoprotein P. J Mol Endocrinol. (2013) 50:31–42. doi: 10.1530/JME-12-0105
43. Donadio JLS, Duarte GBS, Borel P, Cozzolino SMF, Rogero MM. The influence of nutrigenetics on biomarkers of selenium nutritional status. Nutr Rev. (2021) 79:1259–73. doi: 10.1093/nutrit/nuaa136
44. Moriguchi Watanabe L, Bueno AC, de Lima LF, Ferraz-Bannitz R, Dessordi R, Guimaraes MP, et al. Genetically determined variations of selenoprotein P are associated with antioxidant, muscular, and lipid biomarkers in response to Brazil nut consumption by patients using statins. Br J Nutr. (2022) 127:679–86. doi: 10.1017/S000711452100146X
45. Schiavon M, Nardi S, Dalla Vecchia F, Ertani A. Selenium biofortification in the 21(st) century: status and challenges for healthy human nutrition. Plant Soil. (2020) 453:245–70. doi: 10.1007/s11104-020-04635-9
46. Tanguy Y, Falluel-Morel A, Arthaud S, Boukhzar L, Manecka DL, Chagraoui A, et al. The PACAP-regulated gene selenoprotein T is highly induced in nervous, endocrine, and metabolic tissues during ontogenetic and regenerative processes. Endocrinology. (2011) 152:4322–35. doi: 10.1210/en.2011-1246
47. Steinbrenner H. Interference of selenium and selenoproteins with the insulin-regulated carbohydrate and lipid metabolism. Free Radic Biol Med. (2013) 65:1538–47. doi: 10.1016/j.freeradbiomed.2013.07.016
48. Labunskyy VM, Hatfield DL, Gladyshev VN. Selenoproteins: molecular pathways and physiological roles. Physiol Rev. (2014) 94:739–77. doi: 10.1152/physrev.00039.2013
49. Lei XG, Cheng WH, McClung JP. Metabolic regulation and function of glutathione peroxidase-1. Annu Rev Nutr. (2007) 27:41–61. doi: 10.1146/annurev.nutr.27.061406.093716
50. Mita Y, Nakayama K, Inari S, Nishito Y, Yoshioka Y, Sakai N, et al. Selenoprotein P-neutralizing antibodies improve insulin secretion and glucose sensitivity in type 2 diabetes mouse models. Nat Commun. (2017) 8:1658. doi: 10.1038/s41467-017-01863-z
51. Yu SS, Du JL. Selenoprotein S: a therapeutic target for diabetes and macroangiopathy? Cardiovasc Diabetol. (2017) 16:101. doi: 10.1186/s12933-017-0585-8
52. Walder K, Kantham L, McMillan JS, Trevaskis J, Kerr L, De Silva A, et al. Tanis a link between type 2 diabetes and inflammation? Diabetes. (2002) 51:1859–66. doi: 10.2337/diabetes.51.6.1859
53. Marzi C, Huth C, Herder C, Baumert J, Thorand B, Rathmann W, et al. Acute-phase serum amyloid A protein and its implication in the development of type 2 diabetes in the KORA S4/F4 Study. Diabetes Care. (2013) 35:1321–6. doi: 10.2337/dc12-1514
54. Karlsson HK, Tsuchida H, Lake S, Koistinen HA, Krook A. Relationship between serum amyloid A level and Tanis/SelS mRNA expression in skeletal muscle and adipose tissue from healthy and type 2 diabetic subjects. Diabetes. (2004) 53:1424–8. doi: 10.2337/diabetes.53.6.1424
55. Yu SS, Men LL, Wu JL, Huang LW, Xing Q, Yao JJ, et al. The source of circulating selenoprotein S and its association with type 2 diabetes mellitus and atherosclerosis: a preliminary study. Cardiovasc Diabetol. (2016) 15:70. doi: 10.1186/s12933-016-0388-3
56. Chen LL, Huang JQ, Wu YY, Chen LB, Li SP, Zhang X, et al. Loss of Selenov predisposes mice to extra fat accumulation and attenuated energy expenditure. Redox Biol. (2021) 45:102048. doi: 10.1016/j.redox.2021.102048
57. Zhang X, Xiong W, Chen LL, Huang JQ, Lei XG. Selenoprotein V protects against endoplasmic reticulum stress and oxidative injury induced by pro-oxidants. Free Radic Biol Med. (2020) 160:670–9. doi: 10.1016/j.freeradbiomed.2020.08.011
58. Köhrle J. Thyroid hormone transporters in health and disease: advances in thyroid hormone deiodination. Best Pract Res Clin Endocrinol Metab. (2007) 21:173–91. doi: 10.1016/j.beem.2007.04.001
59. Brigelius-Flohé R. Tissue-specific functions of individual glutathione peroxidases. Free Radic Biol Med. (1999) 27:951–65. doi: 10.1016/S0891-5849(99)00173-2
60. Sunde RA, Raines AM, Barnes KM, Evenson JK. Selenium status highly regulates selenoprotein mRNA levels for only a subset of the selenoproteins in the selenoproteome. Biosci Rep. (2009) 29:329–38. doi: 10.1042/bsr20080146
61. Huang JQ, Zhou JC, Wu YY, Ren FZ, Lei XG. Role of glutathione peroxidase 1 in glucose and lipid metabolism-related diseases. Free Radic Biol Med. (2018) 127:108–15. doi: 10.1016/j.freeradbiomed.2018.05.077
62. Takebe G, Yarimizu J, Saito Y, Hayashi T, Nakamura H, Yodoi J, et al. A comparative study on the hydroperoxide and thiol specificity of the glutathione peroxidase family and selenoprotein P. J Biol Chem. (2002) 277:41254–8. doi: 10.1074/jbc.M202773200
63. Sies H, Arteel GE. Interaction of peroxynitrite with selenoproteins and glutathione peroxidase mimics. Free Radic Biol Med. (2000) 28:1451–5. doi: 10.1016/s0891-5849(00)00253-7
64. Yoneda S, Suzuki KT. Equimolar Hg-Se complex binds to selenoprotein P. Biochem Biophys Res Commun. (1997) 231:7–11. doi: 10.1006/bbrc.1996.6036
65. Fradejas N, Serrano-Pérez Mdel C, Tranque P, Calvo S. Selenoprotein S expression in reactive astrocytes following brain injury. Glia. (2011) 59:959–72. doi: 10.1002/glia.21168
66. Qiao L, Men L, Yu S, Yao J, Li Y, Wang M, et al. Hepatic deficiency of selenoprotein S exacerbates hepatic steatosis and insulin resistance. Cell Death Dis. (2022) 13:275. doi: 10.1038/s41419-022-04716-w
67. Liu YY, Milanesi A, Brent GA. Chapter 21 - Thyroid hormones. In: Litwack G, editor. Hormonal Signaling in Biology and Medicine. London: Academic Press (2020). 487–506. doi: 10.1016/B978-0-12-813814-4.00021-3
68. Ezaki O. The insulin-like effects of selenate in rat adipocytes. J Biol Chem. (1990) 265:1124–8.
69. Hei YJ, Farahbakhshian S, Chen X, Battell ML, McNeill JH. Stimulation of MAP kinase and S6 kinase by vanadium and selenium in rat adipocytes. Mol Cell Biochem. (1998) 178:367–75. doi: 10.1023/a:1006819906820
70. Stapleton SR. Selenium: an insulin-mimetic. Cell Mol Life Sci. (2000) 57:1874–9. doi: 10.1007/pl00000669
71. Speckmann B, Walter PL, Alili L, Reinehr R, Sies H, Klotz LO, et al. Selenoprotein P expression is controlled through interaction of the coactivator PGC-1alpha with FoxO1a and hepatocyte nuclear factor 4alpha transcription factors. Hepatology. (2008) 48:1998–2006. doi: 10.1002/hep.22526
72. Misu H, Takamura T, Takayama H, Hayashi H, Matsuzawa-Nagata N, Kurita S, et al. A liver-derived secretory protein, selenoprotein P, causes insulin resistance. Cell Metab. (2010) 12:483–95. doi: 10.1016/j.cmet.2010.09.015
73. Schmoll D, Walker KS, Alessi DR, Grempler R, Burchell A, Guo S, et al. Regulation of glucose-6-phosphatase gene expression by protein kinase Balpha and the forkhead transcription factor FKHR. Evidence for insulin response unit-dependent and -independent effects of insulin on promoter activity. J Biol Chem. (2000) 275:36324–33. doi: 10.1074/jbc.M003616200
74. Yoon JC, Puigserver P, Chen G, Donovan J, Wu Z, Rhee J, et al. Control of hepatic gluconeogenesis through the transcriptional coactivator PGC-1. Nature. (2001) 413:131–8. doi: 10.1038/35093050
75. Walter PL, Steinbrenner H, Barthel A, Klotz LO. Stimulation of selenoprotein P promoter activity in hepatoma cells by FoxO1a transcription factor. Biochem Biophys Res Commun. (2008) 365:316–21. doi: 10.1016/j.bbrc.2007.10.171
76. Asayama K, Kooy NW, Burr IM. Effect of vitamin E deficiency and selenium deficiency on insulin secretory reserve and free radical scavenging systems in islets: decrease of islet manganosuperoxide dismutase. J Lab Clin Med. (1986) 107:459–64.
77. Reddi AS, Bollineni JS. Selenium-deficient diet induces renal oxidative stress and injury via TGF-beta1 in normal and diabetic rats. Kidney Int. (2001) 59:1342–53. doi: 10.1046/j.1523-1755.2001.0590041342.x
78. Wang X, Vatamaniuk MZ, Roneker CA, Pepper MP, Hu LG, Simmons RA, et al. Knockouts of SOD1 and GPX1 exert different impacts on murine islet function and pancreatic integrity. Antioxid Redox Signal. (2011) 14:391–401. doi: 10.1089/ars.2010.3302
79. Merry TL, Tran M, Stathopoulos M, Wiede F, Fam BC, Dodd GT, et al. High-fat-fed obese glutathione peroxidase 1-deficient mice exhibit defective insulin secretion but protection from hepatic steatosis and liver damage. Antioxid Redox Signal. (2014) 20:2114–29. doi: 10.1089/ars.2013.5428
80. Gao Y, Feng HC, Walder K, Bolton K, Sunderland T, Bishara N, et al. Regulation of the selenoprotein SelS by glucose deprivation and endoplasmic reticulum stress - SelS is a novel glucose-regulated protein. FEBS Lett. (2004) 563:185–90. doi: 10.1016/s0014-5793(04)00296-0
81. Labunskyy VM, Lee BC, Handy DE, Loscalzo J, Hatfield DL, Gladyshev VN. Both maximal expression of selenoproteins and selenoprotein deficiency can promote development of type 2 diabetes-like phenotype in mice. Antioxid Redox Signal. (2011) 14:2327–36. doi: 10.1089/ars.2010.3526
82. Mihara H, Kurihara T, Watanabe T, Yoshimura T, Esaki N. cDNA cloning, purification, and characterization of mouse liver selenocysteine lyase. Candidate for selenium delivery protein in selenoprotein synthesis. J Biol Chem. (2000) 275:6195–200. doi: 10.1074/jbc.275.9.6195
83. Seale LA, Hashimoto AC, Kurokawa S, Gilman CL, Seyedali A, Bellinger FP, et al. Disruption of the selenocysteine lyase-mediated selenium recycling pathway leads to metabolic syndrome in mice. Mol Cell Biol. (2012) 32:4141–54. doi: 10.1128/mcb.00293-12
84. Cui A, Ding D, Li Y. Regulation of hepatic metabolism and cell growth by the ATF/CREB family of transcription factors. Diabetes. (2021) 70:653–64. doi: 10.2337/dbi20-0006
85. Ress C, Kaser S. Mechanisms of intrahepatic triglyceride accumulation. World J Gastroenterol. (2016) 2:1664–73. doi: 10.3748/wjg.v22.i4.1664
86. Gong Q, Hu Z, Zhang F, Cui A, Chen X, Jiang H, et al. Fibroblast growth factor 21 improves hepatic insulin sensitivity by inhibiting mammalian target of rapamycin complex 1 in mice. Hepatology. (2016) 64:425–38. doi: 10.1002/hep.28523
87. Sun Y, Xia M, Yan H, Han Y, Zhang F, Hu Z, et al. Berberine attenuates hepatic steatosis and enhances energy expenditure in mice by inducing autophagy and fibroblast growth factor 21. Br J Pharmacol. (2018) 175:374–87. doi: 10.1111/bph.14079
88. Rayman MP. The importance of selenium to human health. Lancet. (2000) 356:233–41. doi: 10.1016/s0140-6736(00)02490-9
89. Stoffaneller R, Morse NL. A review of dietary selenium intake and selenium status in Europe and the Middle East. Nutrients. (2015) 7:1494–537. doi: 10.3390/nu7031494
90. Rayman MP, Stranges S. Epidemiology of selenium and type 2 diabetes: can we make sense of it? Free Radic Biol Med. (2013) 65:1557–64. doi: 10.1016/j.freeradbiomed.2013.04.003
91. Kim J, Chung HS, Choi MK, Roh YK, Yoo HJ, Park JH, et al. Association between serum selenium level and the presence of diabetes mellitus: a meta-analysis of observational studies. Diabetes Metab J. (2019) 43:447–60. doi: 10.4093/dmj.2018.0123
92. Lin J, Shen T. Association of dietary and serum selenium concentrations with glucose level and risk of diabetes mellitus: a cross sectional study of national health and nutrition examination survey, 1999-2006. J Trace Elem Med Biol. (2021) 63:126660. doi: 10.1016/j.jtemb.2020.126660
93. Vinceti M, Filippini T, Rothman KJ. Selenium exposure and the risk of type 2 diabetes: a systematic review and meta-analysis. Eur J Epidemiol. (2018) 33:789–810. doi: 10.1007/s10654-018-0422-8
94. Laclaustra M, Navas-Acien A, Stranges S, Ordovas JM, Guallar E. Serum selenium concentrations and diabetes in U.S. adults: national health and nutrition examination survey (NHANES) 2003-2004. Environ Health Perspect. (2009) 117:1409–13. doi: 10.1289/ehp.0900704
95. Winther KH, Rayman MP, Bonnema SJ, Hegedus L. Selenium in thyroid disorders - essential knowledge for clinicians. Nat Rev Endocrinol. (2020) 16:165–76. doi: 10.1038/s41574-019-0311-6
96. Zhang L, Song H, Guo Y, Fan B, Huang Y, Mao X, et al. Benefit-risk assessment of dietary selenium and its associated metals intake in China (2017-2019): is current selenium-rich agro-food safe enough? J Hazard Mater. (2020) 398:123224. doi: 10.1016/j.jhazmat.2020.123224
97. Dinh QT, Cui Z, Huang J, Tran TAT, Wang D, Yang W, et al. Selenium distribution in the Chinese environment and its relationship with human health: a review. Environ Int. (2018) 112:294–309. doi: 10.1016/j.envint.2017.12.035
98. Skalny AV, Burtseva TI, Salnikova EV, Ajsuvakova OP, Skalnaya MG, Kirichuk AA, et al. Geographic variation of environmental, food, and human hair selenium content in an industrial region of Russia. Environ Res. (2019) 171:293–301. doi: 10.1016/j.envres.2019.01.038
99. Dos Santos M, Penteado JO, Baisch PRM, Soares BM, Muccillo-Baisch AL, da Silva Júnior FMR. Selenium dietary intake, urinary excretion, and toxicity symptoms among children from a coal mining area in Brazil. Environ Geochem Health. (2021) 43:65–75. doi: 10.1007/s10653-020-00672-6
100. Joy EJ, Ander EL, Young SD, Black CR, Watts MJ, Chilimba AD, et al. Dietary mineral supplies in Africa. Physiol Plant. (2014) 151:208–29. doi: 10.1111/ppl.12144
101. Ahmad S, Bailey EH, Arshad M, Ahmed S, Watts MJ, Stewart AG, et al. Environmental and human iodine and selenium status: lessons from Gilgit-Baltistan, North-East Pakistan. Environ Geochem Health. (2021) 43:4665–86. doi: 10.1007/s10653-021-00943-w
102. Jenny-Burri J, Haldimann M, Dudler V. Estimation of selenium intake in Switzerland in relation to selected food groups. Food Addit Contam Part A Chem Anal Control Expo Risk Assess. (2010) 27:1516–31. doi: 10.1080/19440049.2010.506603
103. Lenzen S, Drinkgern J, Tiedge M. Low antioxidant enzyme gene expression in pancreatic islets compared with various other mouse tissues. Free Radic Biol Med. (1996) 20:463–6. doi: 10.1016/0891-5849(96)02051-5
104. Harmon JS, Bogdani M, Parazzoli SD, Mak SSM, Oseid EA, Berghmans M, et al. beta-Cell-specific overexpression of glutathione peroxidase preserves intranuclear MafA and reverses diabetes in db/db mice. Endocrinology. (2009) 150:4855–62. doi: 10.1210/en.2009-0708
105. McClung JP, Roneker CA, Mu W, Lisk DJ, Langlais P, Liu F, et al. Development of insulin resistance and obesity in mice overexpressing cellular glutathione peroxidase. Proc Natl Acad Sci USA. (2004) 101:8852–7. doi: 10.1073/pnas.0308096101
106. Wang XD, Vatamaniuk MZ, Wang SK, Roneker CA, Simmons RA, Lei XG. Molecular mechanisms for hyperinsulinaemia induced by overproduction of selenium-dependent glutathione peroxidase-1 in mice. Diabetologia. (2008) 51:1515–24. doi: 10.1007/s00125-008-1055-3
107. Pepper MP, Vatamaniuk MZ, Yan X, Roneker CA, Lei XG. Impacts of dietary selenium deficiency on metabolic phenotypes of diet-restricted GPX1-overexpressing mice. Antioxid Redox Signal. (2011) 14:383–90. doi: 10.1089/ars.2010.3295
108. Saito Y. Selenoprotein P as a significant regulator of pancreatic β cell function. J Biochem. (2020) 167:119–24. doi: 10.1093/jb/mvz061
109. Lennicke C, Cochemé HM. Redox regulation of the insulin signalling pathway. Redox Biol. (2021) 42:101964. doi: 10.1016/j.redox.2021.101964
110. Steinbrenner H, Speckmann B, Pinto A, Sies H. High selenium intake and increased diabetes risk: experimental evidence for interplay between selenium and carbohydrate metabolism. J Clin Biochem Nutr. (2011) 48:40–5. doi: 10.3164/jcbn.11-002FR
111. Loh K, Deng H, Fukushima A, Cai X, Boivin B, Galic S, et al. Reactive oxygen species enhance insulin sensitivity. Cell Metab. (2009) 10:260–72. doi: 10.1016/j.cmet.2009.08.009
112. Jung TW, Choi HY, Lee SY, Hong HC, Yang SJ, Yoo HJ, et al. Salsalate and adiponectin improve palmitate-induced insulin resistance via inhibition of selenoprotein P through the AMPK-FOXO1α pathway. PLoS One. (2013) 8:e66529. doi: 10.1371/journal.pone.0066529
113. Yang SJ, Hwang SY, Choi HY, Yoo HJ, Seo JA, Kim SG, et al. Serum selenoprotein P levels in patients with type 2 diabetes and prediabetes: implications for insulin resistance, inflammation, and atherosclerosis. J Clin Endocrinol Metab. (2011) 96:E1325–9. doi: 10.1210/jc.2011-0620
114. Misu H, Ishikura K, Kurita S, Takeshita Y, Ota T, Saito Y, et al. Inverse correlation between serum levels of selenoprotein P and adiponectin in patients with type 2 diabetes. PLoS One. (2012) 7:e34952. doi: 10.1371/journal.pone.0034952
115. Takayama H, Misu H, Iwama H, Chikamoto K, Saito Y, Murao K, et al. Metformin suppresses expression of the selenoprotein P gene via an AMP-activated kinase (AMPK)/FoxO3a pathway in H4IIEC3 hepatocytes. J Biol Chem. (2014) 289:335–45. doi: 10.1074/jbc.M113.479386
116. Speckmann B, Sies H, Steinbrenner H. Attenuation of hepatic expression and secretion of selenoprotein P by metformin. Biochem Biophys Res Commun. (2009) 387:158–63. doi: 10.1016/j.bbrc.2009.06.143
117. Mao J, Teng W. The relationship between selenoprotein P and glucose metabolism in experimental studies. Nutrients. (2013) 5:1937–48. doi: 10.3390/nu5061937
118. Lebeaupin C, Vallée D, Hazari Y, Hetz C, Chevet E, Bailly-Maitre B. Endoplasmic reticulum stress signalling and the pathogenesis of non-alcoholic fatty liver disease. J Hepatol. (2018) 69:927–47. doi: 10.1016/j.jhep.2018.06.008
119. Wu J, Wu D, Zhang L, Lin C, Liao J, Xie R, et al. NK cells induce hepatic ER stress to promote insulin resistance in obesity through osteopontin production. J Leukoc Biol. (2020) 107:589–96. doi: 10.1002/jlb.3ma1119-173r
120. Meusser B, Hirsch C, Jarosch E, Sommer T. ERAD: the long road to destruction. Nat Cell Biol. (2005) 7:766–72. doi: 10.1038/ncb0805-766
121. Ye Y, Shibata Y, Kikkert M, van Voorden S, Wiertz E, Rapoport TA. Recruitment of the p97 ATPase and ubiquitin ligases to the site of retrotranslocation at the endoplasmic reticulum membrane. Proc Natl Acad Sci USA. (2005) 102:14132–8. doi: 10.1073/pnas.0505006102
122. Lee JH, Kwon JH, Jeon YH, Ko KY, Lee SR, Kim IY. Pro178 and Pro183 of selenoprotein S are essential residues for interaction with p97(VCP) during endoplasmic reticulum-associated degradation. J Biol Chem. (2014) 289:13758–68. doi: 10.1074/jbc.M113.534529
123. Lee JH, Park KJ, Jang JK, Jeon YH, Ko KY, Kwon JH, et al. Selenoprotein S-dependent selenoprotein K binding to p97(VCP) protein is essential for endoplasmic reticulum-associated degradation. J Biol Chem. (2015) 290:29941–52. doi: 10.1074/jbc.M115.680215
124. Du S, Liu H, Huang K. Influence of SelS gene silence on beta-Mercaptoethanol-mediated endoplasmic reticulum stress and cell apoptosis in HepG2 cells. Biochim Biophys Acta. (2010) 1800:511–7. doi: 10.1016/j.bbagen.2010.01.005
125. Ye Y, Fu F, Li X, Yang J, Liu H. Selenoprotein S is highly expressed in the blood vessels and prevents vascular smooth muscle cells from apoptosis. J Cell Biochem. (2016) 117:106–17. doi: 10.1002/jcb.25254
126. Kim KH, Gao Y, Walder K, Collier GR, Skelton J, Kissebah AH. SEPS1 protects RAW264.7 cells from pharmacological ER stress agent-induced apoptosis. Biochem Biophys Res Commun. (2007) 354:127–32 doi: 10.1016/j.bbrc.2006.12.183
Keywords: selenium, dietary intake, glucose and lipid metabolism, type 2 diabetes, selenoproteins
Citation: Zhao J, Zou H, Huo Y, Wei X and Li Y (2022) Emerging roles of selenium on metabolism and type 2 diabetes. Front. Nutr. 9:1027629. doi: 10.3389/fnut.2022.1027629
Received: 25 August 2022; Accepted: 10 October 2022;
Published: 10 November 2022.
Edited by:
Xianli Gao, Jiangsu University, ChinaReviewed by:
Yang Yuan, Guangzhou University, ChinaSusmita Barman, University of Nebraska Medical Center, United States
Copyright © 2022 Zhao, Zou, Huo, Wei and Li. This is an open-access article distributed under the terms of the Creative Commons Attribution License (CC BY). The use, distribution or reproduction in other forums is permitted, provided the original author(s) and the copyright owner(s) are credited and that the original publication in this journal is cited, in accordance with accepted academic practice. No use, distribution or reproduction is permitted which does not comply with these terms.
*Correspondence: Yu Li, liyu@sinh.ac.cn
†These authors have contributed equally to this work and share first authorship