- 1Department of Nutrition, Exercise and Sports, Faculty of Science, University of Copenhagen, Frederiksberg, Denmark
- 2Department of Veterinary and Animal Science, Faculty of Health and Medical Sciences, University of Copenhagen, Frederiksberg, Denmark
- 3Western Human Nutrition Research Center, Agricultural Research Service (USDA), Davis, CA, United States
Background: Appetite-regulating hormones (ARH) in human milk (HM) are suggested to affect infants’ milk intake and possibly infant growth. Maternal adiposity might contribute to higher levels of ARH in HM, either from the mammary gland or from raised circulating levels due to higher adiposity. Counterfactual-based mediation analysis can define indirect and direct effects between HM ARH and maternal and infant factors, and might be an important tool when investigating the mother-milk-infant triad.
Objective: We aim to investigate whether potential associations between (1) maternal adiposity and HM ARH and (2) HM ARH and infant milk intake and growth are mediated through maternal and infant plasma ARH, respectively.
Materials and methods: Maternal and infant anthropometry and body composition, HM and blood samples were collected from 223 mother-infant dyads participating in the Mother, Infant and Lactation Quality study at three postpartum visits from 1 to 8.49 months. Leptin, insulin and adiponectin were analyzed using immunoassays. Mediation analyses using linear mixed-effect models were applied to investigate the direct and indirect effects through maternal and infant plasma hormone concentrations.
Results: A positive association between maternal body-mass-index (BMI) and HM leptin was mediated by maternal plasma leptin by 29% when fixing BMI to < 25 kg/m2, and through 51% when fixing BMI to ≥ 25 kg/m2 (pinteraction < 0.01). There was no mediated effect through plasma insulin in the association between BMI and HM insulin (p = 0.068). We found negative and positive associations between HM insulin and total milk intake and infant weight, respectively, however, these diminished in mediation analyses with reduced sample sizes.
Conclusion: Our main results suggest that the association between maternal adiposity and HM leptin was mediated through circulating leptin to a stronger degree for mothers with overweight compared to mothers with normal-weight. This indicates that excess maternal adiposity, and the resulting rise of circulating leptin and possible concomitant low-grade inflammation, may be reflected in HM composition.
Clinical trials registry number: NCT03254329.
Introduction
The World Health Organization (WHO) recommends exclusive breastfeeding (EBF) for six months and continued breastfeeding up to 2 years to ensure optimal nutrition for the newborn infant (1). Current literature additionally posits that breastfeeding has beneficial short- and long-term effects on both mother and infant (2) including a reduced risk of overweight and obesity later in life (3, 4). The hormones leptin, insulin and adiponectin in human milk (HM) are suggested to affect infants growth perhaps through appetite-regulation (5). The adipokines leptin and adiponectin are secreted from the adipose tissue such that circulating levels reflect adiposity in adults as well as in children (6). Gastric leptin increases post-prandially and stimulates hypothalamic receptors in the brain, which further stimulates satiety and thereby promote self-regulation of energy intake (7). Circulating adiponectin has anti-inflammatory properties by promoting insulin sensitivity through decreased energy expenditure and increased glucose uptake (8). Circulating insulin is secreted from the pancreas as a response to postprandial glucose increase and stimulates glucose uptake in the liver and muscle (9).
Furthermore, circulating levels might also reflect obesity-related low-grade inflammation, which is characterized by increased levels of inflammation markers such as C-reactive protein (CRP) and adipokines, such as leptin, secreted from the adipose tissue. Through various mechanisms, low-grade inflammation with elevated levels of such markers contribute to increased risk of type 2 diabetes mellitus (DM), atherosclerosis and non-alcoholic fatty liver disease (10). A study by Savino and colleagues found maternal body mass index (BMI) positively correlated with both maternal serum leptin and HM leptin, which were furthermore correlated with each other (11). Most literature suggests that mammary epithelial cells and possibly adipose tissue secrete the appetite-regulating hormones (ARHs) leptin and adiponectin into the milk (12–14). However, other studies indicate that maternal circulating levels of ARH predict levels in HM (11, 15). The source of HM insulin is mainly hypothesized to be secretion from the epithelial cells of the mammary gland (16) or from maternal plasma (17), and not adipose tissue as such. However, adiposity contributes to insulin resistance increasing plasma insulin (18) and by extension might affect HM insulin. Thus, quantification of the pathways by which ARHs arrive into HM remain to be established.
All three hormones identified in HM have been independently associated with both maternal predictors (5, 19–21) and infant outcomes (11, 22–24). A recently published review concluded an inverse association between HM leptin and infant growth, namely weight and adiposity, based on the 18 included papers (25). Results were mainly seen within the first six months postpartum, where breastfeeding is also most prominent. Inverse associations have similarly been shown between HM adiponectin and infant weight and lean body mass during early breastfeeding (23). Furthermore, Young et al. found HM insulin negatively associated with weight-for-length (WLZ) trajectories, but only in infants of normal-weight mothers (26).
Animal studies have shown that labeled milk leptin is absorbed in the gut and can be subsequently detected in the neonatal circulation (27, 28), supporting that intestinal absorption of ARH from HM is a plausible mechanism for the associations seen between HM ARH and infant outcomes. Combined with results showing that maternal adiposity positively associates with HM ARH, these compounds appear to contribute to the complex signaling mechanisms within the mother-milk-infant triad, which covers the factors related to the mother, HM and the infant as well as the associations between these factors (29, 30). Furthermore, breastfeeding studies are limited in their observational nature with confounding making inferences problematic. Most studies rely on traditional approaches when trying to disentangle associations between maternal determinants and HM composition, e.g., adjusting for an intermediate variable. However, this approach may lead to false estimates of the direct and indirect effects. Novel approaches, such as counterfactual-based mediation analysis, identifies the indirect effect through resampling methods. Secondly, a direct effect is derived from the total and indirect effects within the association. This approach has been implemented in a small number of studies investigating associations between maternal predictors of HM composition and infant outcomes (31, 32) and the method improves validity and interpretation of the results (33). We hypothesize that a mediated effect is present through maternal circulation, and the remaining effect can be ascribed as the direct effect covering secretion of ARH from adipose tissue and mammary epithelial cells. Finally, the study exemplifies the use of counterfactual-based mediation analyses in the investigation of the mother-milk-infant triad within HM research.
With this study, we aim to investigate whether potential associations between (1) maternal body composition and HM ARH and (2) HM ARH and infant milk intake and infant growth are mediated through maternal and infant plasma levels of the respective ARHs. To our knowledge, this is the first paper using counterfactual-based mediation analyses to quantify the concentrations of HM ARH mediated through maternal circulation and to investigate mediated effects through infant plasma in the association between HM ARH and infant outcomes.
Materials and methods
Two-hundred-and-twenty-three Danish mother-infant dyads participating the Mothers, Infants and Lactation Quality (MILQ) study (H-17015174) were included in the present study. The MILQ study is an international multi-center cohort study with the overall aim of developing reference values for micro- and macronutrient concentration in human milk (HM), and is described in detail elsewhere (34).
Participants
Pregnant women were recruited from hospitals in the Copenhagen area and were included before entering the third trimester of pregnancy (<28th week of gestation) after written informed consent was obtained. Participants were screened according to maternal inclusion and exclusion criteria. Inclusion criteria included being 18–40 years old with a pre-pregnancy BMI of 18.5–29.9 kg/m2. They should be non-smokers, have a low intake of alcohol (<5 units per week) and fortified foods (<3 times per week), and should not follow a vegan or macrobiotic diet. Women were excluded if they developed gestational diabetes or preeclampsia during pregnancy, or had anemia, unless they were willing to take iron supplements. Women were informed that they should avoid taking multivitamin supplements and they were offered telephonic breastfeeding counseling by an International Board Certified Lactation Consultant to support WHO’s recommendation of EBF for six months.
Infants were screened telephonically between 2 and 3 weeks after birth. Inclusion criteria for the infants included being born at term (gestational week 37–42) with a birth weight between 2500 and 4200g and without having illnesses affecting growth, development or breastfeeding. Infants were excluded if they were not EBF at Visit 2 (V2) or had ceased breastfeeding completely at Visit 3 (V3). In EBF, formula was allowed only for the first week after birth and sugar water was allowed for analgesic purposes for blood sampling.
Study design
Participants collected a colostrum sample at birth (Visit 1 = V1) and attended three postpartum visits during the study period; 1.0–3.49 months (V2), 3.5–5.99 months (V3), and 6.0–8.5 months postpartum (Visit 4 = V4). All postpartum visits took place at the Department of Nutrition, Exercise and Sports, University of Copenhagen in Denmark. Visits 2–4 were divided into 3-week windows to which participants were evenly distributed such that data was collected at all ages from 1 to 8.49 months postpartum. Data collected at the three postpartum visits (V2–V4) included height, weight and body composition of mother and infant, maternal and infant blood samples and human milk samples. Daily infant milk intake at each of the three visits was estimated using 24-h test weighing. Information regarding birth, sociodemographic characteristics, maternal and infant morbidity and breastfeeding practices was obtained using questionnaires (34).
Data collection
Maternal and infant anthropometry and body composition
Pre-pregnancy weight and height were self-reported and pre-pregnancy BMI was calculated as weight divided by the squared height (kg/m2). Maternal weight and body composition were measured at V2–V4 using a digital scale with an inbuilt bioelectrical impedance function (Tanita-MC780MA; Tanita Corporation, Tokyo, Japan). The measurement was performed with an empty bladder with only light clothes worn, of which the weight was estimated and corrected for. Fat mass (FM) was estimated using the internal Tanita algorithm (not publically available) incorporating sex, age and height. Maternal height was measured at V2 to the nearest millimeter (mm) using an average of three measurements. FM and fat free mass (FFM) indexes (FMI and FFMI, respectively) were calculated by dividing FM and FFM (in kg) by the participant’s squared height (in m).
Infant birth weight and length were self-reported from hospital records, and infant weight, length and body composition were measured at V2–V4. Infant weight was measured using a digital scale (Tanita-BD815MA; Tanita Corporation, Tokyo, Japan) and length was measured to the nearest mm with a portable measuring board (SECA 416; SECA, Hamburg, Germany) using an average of three measurements. Infant weight and length were measured without clothes or diaper. Infant body composition was estimated using bioelectrical impedance (Bodystat-500; Bodystat Ltd., Isle of Man). Two electrodes were placed on the right hand and two on the right ankle, and an average of three measurements was used. Age-appropriate equations generated by Lingwood et al. were used to convert whole-body impedance to FFM, which was subtracted from infant weight to give FM (35). Indexes of FM and FFM were calculated as mentioned above.
Maternal and infant blood sample collection
For the purpose of the entire MILQ study, non-fasting blood samples were collected from both mother (18 mL) and infant (6 mL) at V2–V4 and 200 μL was used for analysis of this present study. All infants had blood samples taken at V2, however, to reduce discomfort for the infants, only 50% had blood samples taken at V3 and the other 50% had blood samples taken at V4. Whole blood was collected by venipuncture into a vacutainer collection tube (K2EDTA 3 mL for infants and K2EDTA plasma mineral trace free for mothers), from which plasma was obtained after centrifugation (1500xg for 10 min. 4°C). Samples were stored in 2 mL amber screw cap tubes at –70 to –80°C until analysis. Local analgesics was offered for the infants prior to sample collection.
Human milk collection
Mothers collected a colostrum sample (1 mL) in a small, clean tube provided by study staff. The sample was collected by hand expression 24–72 h after birth, either at home or at the hospital. The sample was protected from sunlight using tinfoil and stored in the home freezer (–18°C) or at the hospital for a maximum of 3.49 months before being transferred to the university freezer (–80°C) until analysis.
Human milk was collected as full breast expressions using an electric breast pump and 250 mL collection bottles (Medela Symphony; Medela; Baar, Switzerland). Samples were collected around 09:00 or around 14:00 h from the breast not last used for feeding. The breast was cleaned with an alcohol-free wet wipe prior to sample collection and a sample of 30 mL was retained from the full breast expression in an amber 50 mL polypropylene tube. The whole milk sample was mixed and homogenized and aliquoted in 2 mL amber screw cap tubes immediately hereafter and stored in the freezer (–80°C) until analysis.
Analysis of human milk and plasma samples
Leptin and insulin in both whole milk and plasma were measured using MSD U-plex multiplex immunoassays (Meso Scale Diagnostics, Rockville, USA). Adiponectin in both whole milk and plasma was measured by sandwich enzyme-linked immunosorbent assay (sELISA) using a human adiponectin duoset (DY1065) from R&D (Biotechne, Minneapolis, MN, USA). Assays were performed according to manufacturer protocols. Plasma insulin and leptin were added undiluted to the wells while the respective HM samples were diluted 1:2 in in the provided diluent. For analysis of adiponectin, HM was diluted 1:10 and plasma samples 1:20,000 in PBS with 1% BSA, pH 7.2–7.4. A minor number of samples were out of the range and were re-analyzed at a higher or lower dilution.
Detection limits of insulin, leptin and adiponectin were 12, 11 pg/mL and 5 ng/mL, respectively. For non-detectable (NA) data, half of the lower cut-off concentration of the specific hormone was used for statistical analyses. Raw data prior to data imputation can be seen in Supplementary Table 1 including number of NA samples. An internal reference sample was prepared by pooling aliquots of 80 plasma samples and included in duplicates on each plate. The obtained values were used to determine assay variability. The intra assay coefficient of variability (CV) for insulin, leptin and adiponectin was 8.5, 9.5, and 11%, and inter assay CV-values were 18, 20, and 29%, respectively.
Energy in HM was calculated using macronutrients concentrations. Whole milk samples (500 μL) were analyzed using near infrared spectroscopy by measuring absorbance for fat, protein, and carbohydrates as per the manufacturer’s protocol (SpectraStar XT; Unity Scientific, Milford, MA, USA). Energy concentration was estimated using the macronutrients energy content per gram (4 calories/g for carbohydrate and protein; 9 calories/g for fat). The machine was originally calibrated with known human milk samples from a reference laboratory and subsequently tested with an in-house milk pool with every set of analysis. Samples were heated to 35°C and homogenized in dim light before analysis. The sonicator tip and measuring cup was cleaned firstly with deionized water and secondly with methanol in between scans, according to manufacturer’s protocol.
Infant milk intake
Human milk intake was measured by 24-h test weighing using a digital scale (ADE M101000-01; ADE GmbH & Co., Hamburg, Germany) with an accuracy of 5 g for weights below 10 kg and 10 g for weights above 10 kg. Participants received the scale after attending a visit and completed a weighing protocol within 7 days after the visit. The weighing took place from 08:00 to 07:59, plus one extra weighing to define the 24 h. The mother was informed to weigh the infant with the same clothes and diaper at the two weighings in order to get a correct estimate of milk intake defined by the weight difference between the two feeds. The mother logged the time and infant weight before and after the feed and which breast was used. Logs were double-entered electronically by study staff. Single feeds > 400g were regarded as being unrealistically high, and marked as missing. Logs with > 3 missing feedings were regarded insufficient and were discarded. For participants with ≤3 missing feedings, missing data was imputed using hot deck imputations based on neighboring weights from the same participant.
Statistical analysis
Continuous characteristics are given as mean ± standard deviation (SD) when normally distributed and as median ± inter quartile range (IQR) when non-normally distributed. Categorical characteristics are given as counts and percentages unless otherwise stated. Interactions, collinearity and assumptions of linearity, normality and equal variance of residuals were checked before reporting the final models. Model estimates of log-transformed variables were back-transformed and expressed as percent difference.
Concentrations of ARH in HM as well as maternal and infant plasma across lactation were described using linear mixed-effect models applying a robust unstructured covariance structure. Models were adjusted for infant sex and a centered age derived by subtracting infant age at a visit from the mean age of all infants at that visit.
Counterfactual-based mediation analyses using linear mixed-effect models were applied to investigate the following two research questions (RQ); (1) whether potential associations between maternal body composition (BMI and FMI) and HM ARH (leptin, insulin and adiponectin) are mediated through maternal plasma markers of the respective hormones, and (2) whether potential associations between the HM ARHs and either infant milk intake (total 24-h intake or intake per feed) and/or infant growth (weight, length, FMI and body fat %), respectively, are mediated through infant plasma levels of the respective hormones (Figure 1). Exposure-mediator interactions were tested in the initial models. If significant, the exposure was converted into a categorical variable, i.e., BMI < 25 or ≥ 25 kg/m2 and FMI ≤9 or > 9 corresponding to BMI groups (36), and the interaction term was included in the mediation analyses. Definitions of total, direct and indirect effects for categorical exposure variables can be found in Supplementary Table 2. Fixed effects further included infant sex, visit and mean-centered age for RQ1. For RQ2, separate mediation analyses investigated either milk intake as outcome, which included HM energy concentration, infant sex, weight and age as additional fixed effects, or infant growth as outcome, including HM energy concentration, total milk intake, birth weight, infant sex and age as additional fixed effects. Subjects were included as random effects in all models. Possible confounding variables mentioned as fixed effects were chosen a priori based on current literature and plausible biological associations.
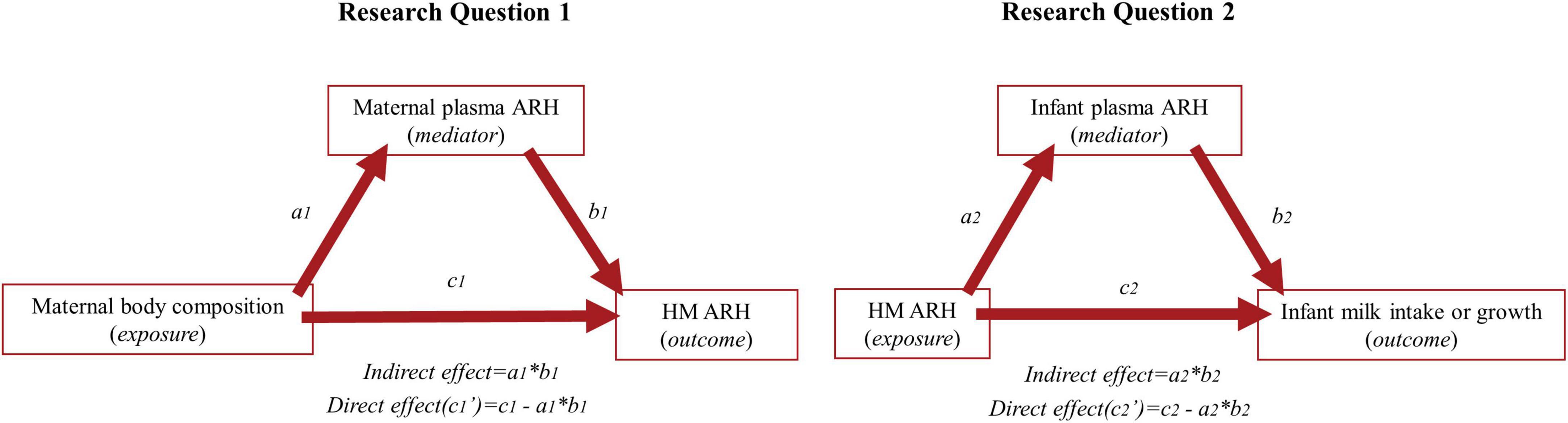
Figure 1. Conceptual diagram of the investigated mediation analyses of Research Question (RQ) 1 (left) and 2 (right). Maternal body composition (exposure) in RQ1 includes body mass index and fat mass index. Outcome in RQ1 and exposure in RQ2, HM ARH, includes human milk concentrations of leptin, insulin and adiponectin. Outcome in RQ2 includes infant milk intake (total 24-h milk intake and milk intake per feed), and infant growth (infant weight, length, fat mass index and body fat percentage). a1 describes the estimate for the influence of exposure on mediator, b1 indicates the estimate for the influence of the mediator on the outcome, and c1 describes the total effect (direct + indirect) of exposure on outcome. The indirect effect (a1b1) can be estimated by multiplying a1 and b1 and the direct effect can be estimated by c1’ = c1 - a1b1.
The following steps comprised the mediation analysis: (1) testing if the exposure was significantly associated with the outcome (total effect = c1), (2) testing if the exposure was significantly associated with the mediating variable (a1) in a “mediator model,” (3) testing if the mediating variable was significantly associated with the outcome (b1) in an “outcome model,” and (4) testing if the mediated (indirect) effect (a1b1) was significant. The indirect effect can also be defined as the product of a1 and b1 and the total effect is equal to the sum of the direct and indirect effect (Figure 1). The significance of the indirect effect was tested using The Monte Carlo Method for Assessing Mediation (37) where a 95% confidence interval (CI) was estimated by resampling of the estimates and distributions of a1 and b1. Sample size in the initial model, testing the total effect (c1), was restricted to include complete cases of included covariates of this model only, whereas sample size in the mediation analyses was restricted to include complete cases of included covariates in all steps of the analyses.
All statistical analyses were conducted using R software (version 4.1.3; R Foundation for Statistical Computing) (38). The LMMstar package (39) was used to define linear mixed-effect models with robust unstructured covariance structures to compare HM ARH in colostrum and mature milk. The lme4 and mediation packages (40, 41) were used to define linear mixed-effect models to be used in mediation analyses, and to conduct the mediation analyses, respectively. Sex differences in infant characteristics were tested using a parametric t-test for normally distributed data and a Mann-Whitney U-test for non-normally distributed data. A (two-sided) P-value < 0.05 was considered statistically significant.
Results
Three-hundred-and-eighty-three women gave informed consent to participation of which n = 146 collected a colostrum sample and n = 227, n = 211 and n = 204 completed V2, V3, and V4, respectively. The majority dropped out or were excluded either (a) at birth (n = 72) due to exclusion criteria regarding birth weight or gestational age at birth, or (b) before V2 (n = 71) because infants were not EBF or parents considered participation too time consuming. For the present analyses, mother-infant dyads were further excluded if they did not breastfeed at any time point (n = 4) resulting in n = 223 being included in the analyses of which n = 204 completed all three postpartum visits (Figure 2). Excluded participants did not differ significantly from the completers with regards to relevant outcomes and/or baseline data (data not shown).
Mothers were 31.5 ± 3.4 years old with a pre-pregnancy BMI of 22.5 ± 2.5 kg/m2 and 14% being overweight (BMI ≥ 25 kg/m2) before pregnancy. Two thirds had a long education and more than 20% had a household income more than 1 million DKK/year corresponding to about 135,000 euro/year (Table 1). Mothers gained 13.4 ± 4.4 kg during pregnancy and had lost their pregnancy weight gain by the end of the study. Maternal BMI decreased slightly from V2 to V4 (Table 2A) and 31, 5, and 20% were overweight, obese and had excessive fat mass, respectively, at any visit.
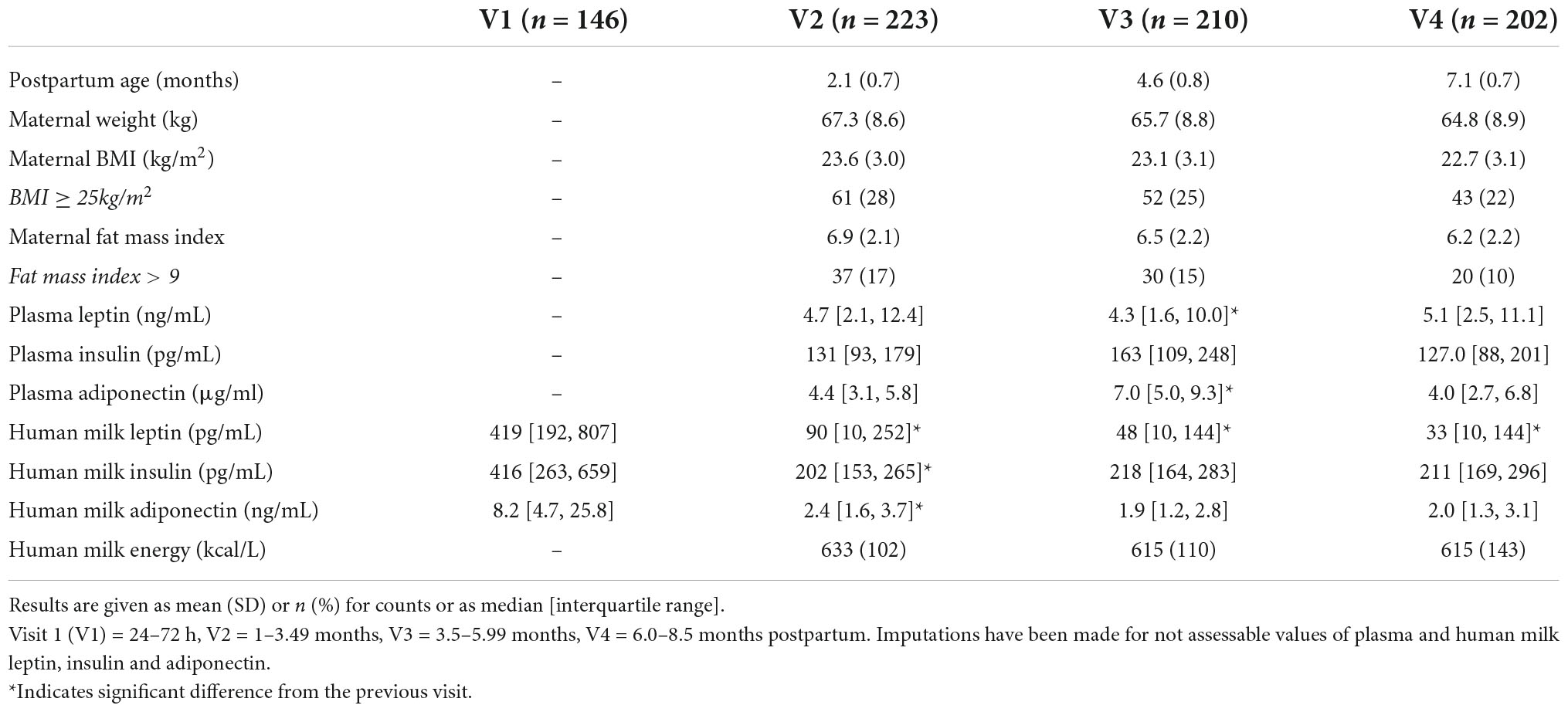
Table 2A. Maternal anthropometry, body composition and plasma and human milk hormone concentrations at the three postpartum visits.
Infants were born at 40.3 ± 1.1 weeks of gestation with a mean birth weight of 3510 ± 364 g and birth length of 51.8 ± 1.9 cm (Table 1). Infants were exclusively breastfed for 4.6 ± 0.9 months and 94 and 53% were exclusively breastfed at V2 and V3, respectively. At V2, 6% had received minimal amounts (≤1 tablespoon; ≤5times) of water or sugar water. Infants had a mean age of 2.1 ± 0.7, 4.6 ± 0.7 and 7.1 ± 0.7 months when attending V2, V3, and V4, respectively.
We had complete milk volume records for n = 204, n = 172 and n = 148 at V2, V3, and V4, respectively, following imputations as described. Total milk intake was significantly higher for males at V2 only (β = 52.2 mL, p = 0.020), whereas milk intake per kg bodyweight was higher for females at V3 (β = 11.6 mL, p = 0.0035; Table 2B).
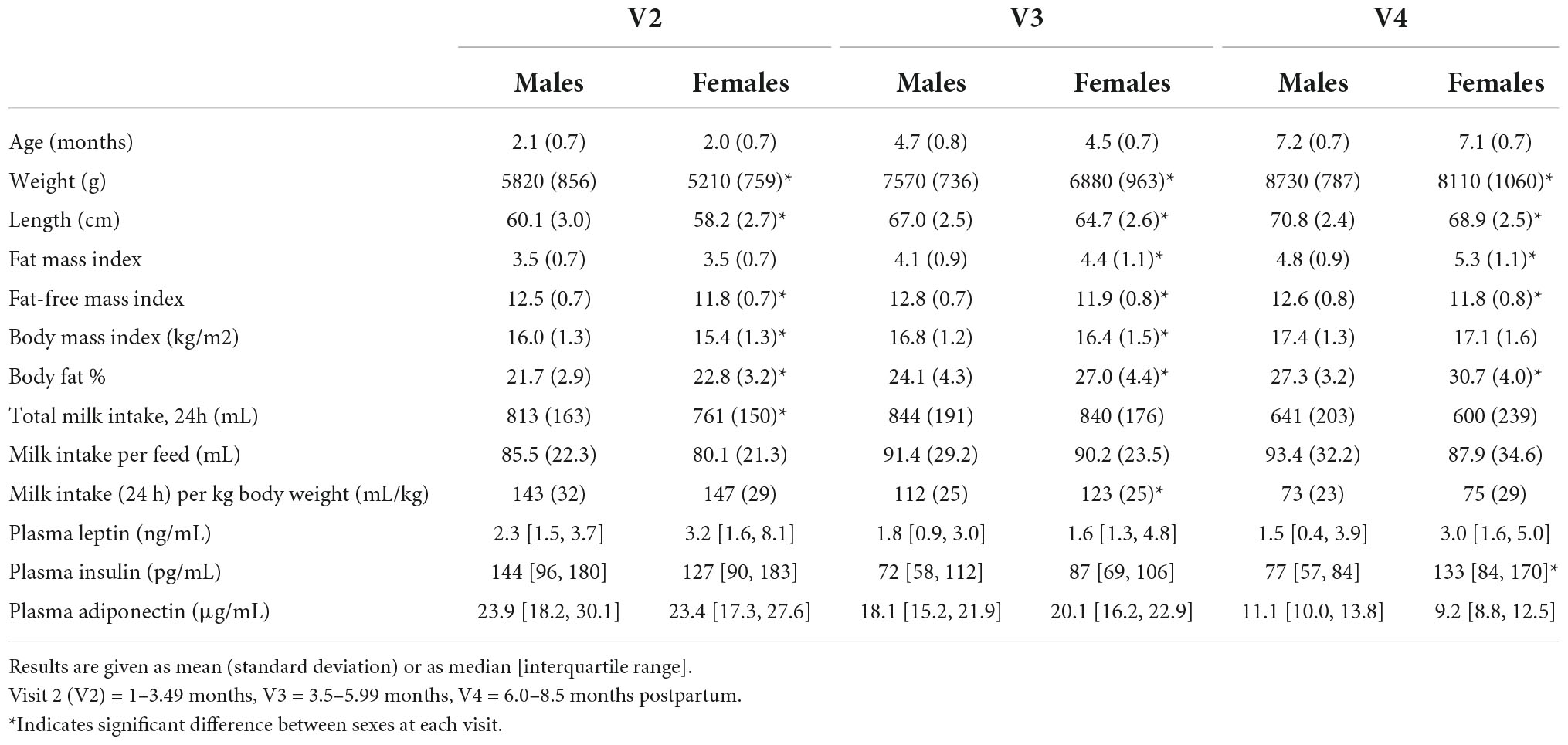
Table 2B. Infant anthropometry, body composition, milk intake and plasma hormone concentrations at the three postpartum visits.
Appetite-regulating hormone concentration across lactation
Colostrum samples were collected 2.1 days ± 0.07 postpartum, and median and IQR for leptin, insulin and adiponectin concentrations were 419 pg/mL [192, 807], 416 pg/mL [263, 659] and 8.2 ng/mL [4.7, 25.8], respectively (Table 2A). Concentrations of all three ARH decreased significantly from V1 to V2 (colostrum to mature milk) by 78, 54, and 80% (β = -1.54; β = -0.78; β = -1.64, pall < 0.01) and with no difference by infant sex (pinteractions > 0.05) (data not shown).
In mature milk, median and IQR for leptin concentrations were 90 pg/mL [10, 252] at V2, which halved at each subsequent visit (βV2–V3 = β0.71; βV3–V4 = -0.69, p = 0.04). Insulin concentration was 202 pg/mL [153, 265] at V2 and remained constant to V4 (β = 0.13, p = 0.41). Adiponectin concentration was 2.4 ng/mL [1.6, 3.7] at V2 and decreased by 27% from V2 to V3 only (β = -0.33, p = 0.02; Table 2A).
Maternal body composition and appetite-regulating hormones in human milk (RQ1)
We found a significant exposure-mediator interaction between maternal BMI and maternal plasma leptin in the initial model associating maternal BMI with log-HM leptin (Table 3). Specifically, the strength of the positive association between maternal BMI and log-HM leptin increased with higher plasma leptin. Consequently, maternal BMI was categorized into mothers with normal-weight (BMI < 25kg/m2) or with overweight (BMI ≥ 25kg/m2) and the interaction term (BMIcategorical*plasma leptinlog) was included in the mediation analyses as described. As such, we found 400% higher leptin in milk of mothers with compared to without overweight (βc1 = 1.6, p < 0.01). The effect was mediated through maternal plasma leptin by 29% when BMI was fixed to normal-weight (βa1b1 = 0.48; p < 0.01) and by 51% when BMI was fixed to overweight (βa1b1 = 0.81; p < 0.01; Figure 3 and Supplementary Table 3). Furthermore, we found an interaction between maternal BMI and infant sex in the initial association between maternal BMI and log-HM leptin. This resulted in mothers with female offspring had a 30% higher HM leptin concentrations compared to mothers of males in the initial model (β = 0.28; p = 0.057; Table 3). An exposure-mediator interaction in the association between maternal FMI and log-HM leptin (β = 0.080; p = 0.002) (data not shown) resulted in a categorization of mothers into having normal (FMI ≤ 9) or excessive (FMI > 9) fat mass as described. The association was only mediated through maternal plasma leptin when FMI was fixed to normal fat mass by 26% (βa1b1 = 0.41; p = 0.002), but not when fixed to excessive fat mass (βa1b1 = 0.20; p = 0.19; Figure 3 and Supplementary Table 3). We did not find an interaction between maternal FMI and infant sex in the initial association between maternal FMI and log-HM leptin.
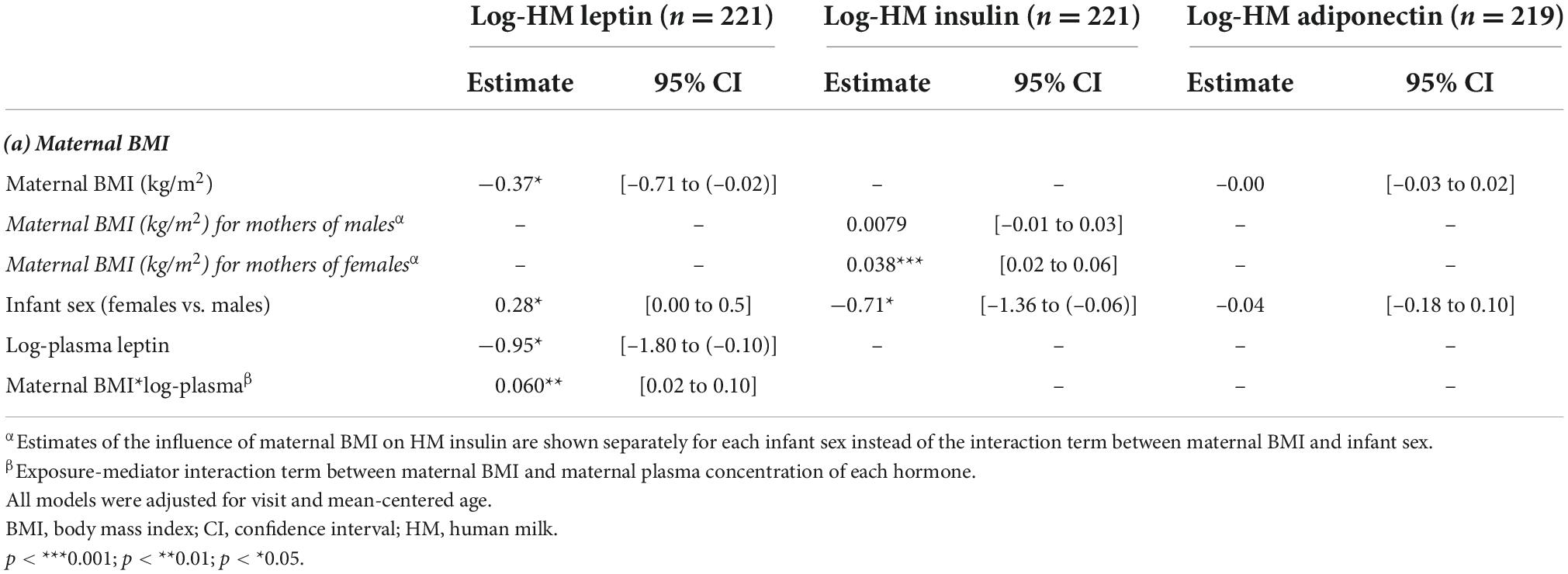
Table 3. Model estimates of the initial testing of the total effect (c1) of maternal BMI on human milk leptin, insulin and adiponectin.
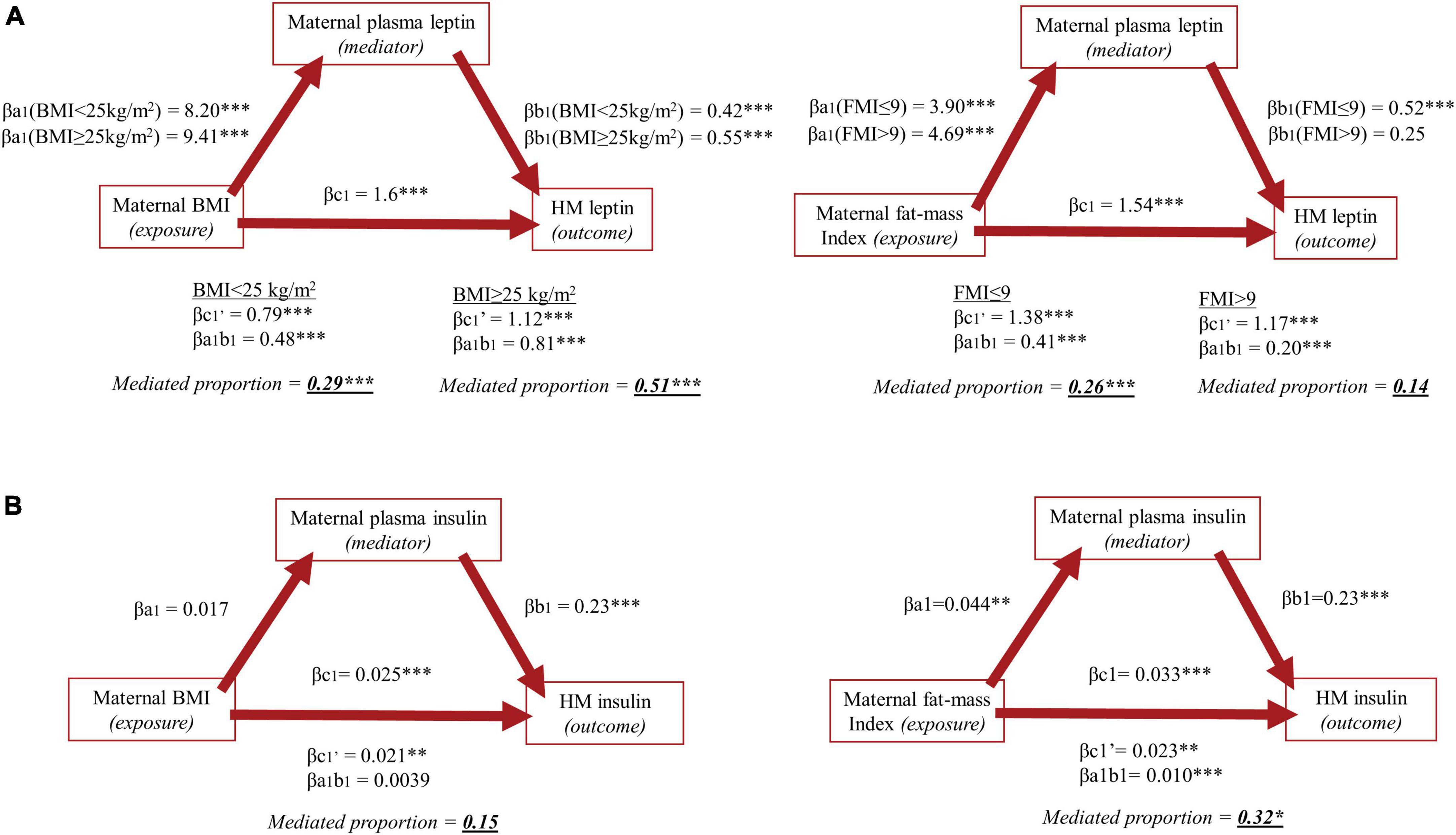
Figure 3. Mediation analyses of Research Question 1 investigating the association between maternal body mass index (left) and fat-mass index (right) as exposure variables and human milk concentrations of (A) leptin and (B) insulin as outcome variables, with maternal plasma concentrations of the respective hormone as mediating variables. In mediation analyses including HM leptin, an exposure-mediator interaction resulted in categorization of maternal BMI into normal- or overweight (BMI < 25 or ≥ 25kg/m2) and FMI into having normal or excessive fat mass (FMI ≤ 9 or > 9) and estimates are given for each group. No exposure-mediator interaction was present in mediation analyses including HM insulin, and the exposure variables were therefore not categorized and estimates are given accordingly.
There was no exposure-mediator interaction in the association between maternal BMI and neither log-HM insulin nor log-HM adiponectin (pboth > 0.05; Figure 3 and Supplementary Table 3). The association between maternal BMI and log-HM insulin was mainly influenced by a direct effect of BMI of 84% (βc1’ = 0.021; p < 0.01) and with an indirect effect of 15% (βa1b1 = 0.0039; p = 0.068). Thirty-two percent of the association between maternal FMI and log-HM insulin (βc1 = 0.033, p < 0.01) was mediated through maternal plasma insulin (βa1b1 = 0.010, p < 0.01). Lastly, we found a significant interaction between maternal BMI and infant sex in the initial model including insulin (β = 0.030; p = 0.03; Table 4), showing a significant increase in HM insulin of 4% per unit increase in BMI for mothers of female offspring (β = 0.04; p = 0.0001), but not for mothers of male (β = 0.008; p = 0.5).
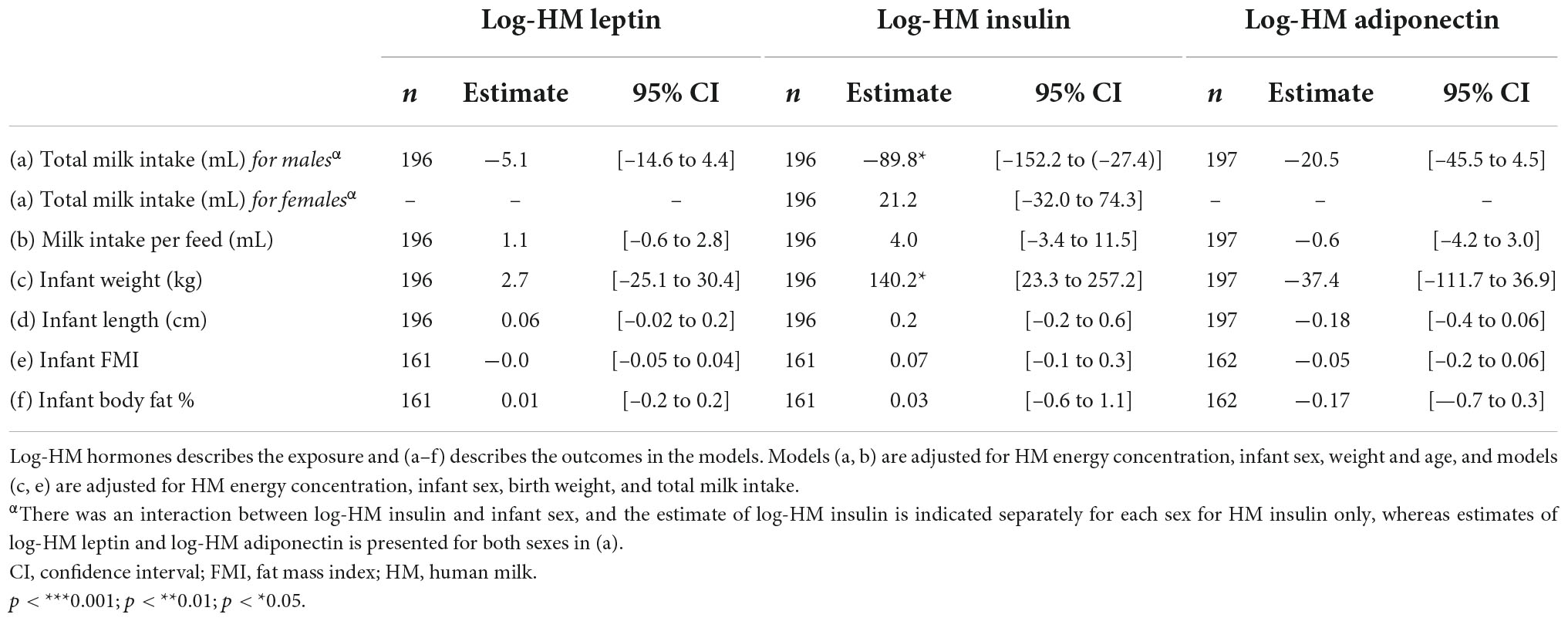
Table 4. Initial testing of the total effect (c1) of leptin, insulin and adiponectin concentrations on (a) total milk intake, (b) milk intake per feed, (c) infant weight, (d) length, (e) fat mass index and (f) body fat percentage, respectively.
Human milk adiponectin was not influenced by either maternal BMI (β = -0.0; p = 0.4; Table 3) or FMI (β = 0.0, p = 0.7) (data not shown).
Appetite-regulating hormones in human milk and infant outcomes (RQ2)
We found a negative association between log-HM insulin and total milk intake in male offspring (β = -89.8, p = 0.005) resulting in 8.5 mL lower milk intake per 10% increase in HM insulin, but not in female (β = 21.2, p = 0.43). Furthermore, a positive association was seen between log-HM insulin and infant weight (β = 140.2, p = 0.02; Table 4) resulting in a 13g increase in weight per 10% increase in HM insulin. However, the associations disappeared in mediation analyses when sample size was reduced from n = 197 to n = 123–135 due to restrictions to number of complete cases in the mediation analyses (data not shown).
There was no association between log-HM leptin and either total milk intake or milk intake per feed (pboth > 0.05). Infant weight and mean-centered age, i.e., being older at the visit, were associated with either total milk intake or milk intake per feed for all three ARH (pall < 0.05) (data not shown). No associations were found between either log-HM leptin or adiponectin and either of the infant growth outcomes, respectively (pall > 0.05; Table 4).
Discussion
We found concentrations of all three ARH decreasing from colostrum to mature milk, which is in accordance with previous literature (6). A large gap between tight junctions in the mammary epithelial cells due to inflammation when the first milk “comes in” creates space for larger compounds to enter the milk (12, 42). Furthermore, we found leptin in mature milk decreasing throughout lactation, whereas adiponectin decreased until 3.5–6 months, and remained constant to 6–8.5 months, as did insulin in mature milk throughout lactation.
A study by Bronsky et al. found median HM leptin falling from 0.30 to 0.05 ng/mL from 0 to 12 months (43), which support our findings of declining leptin concentrations across lactation. This is further supported by a study by Dadres and colleagues reporting declining HM leptin concentrations from 1 to 3 months (19). However, two separate studies find HM leptin nearly constant (44, 45). The study by Dadres and colleagues furthermore found HM adiponectin declining from 1 to 3 months, but constant levels of HM insulin from 1 to 3 months, which is in accordance with our findings. In the present study, neither time of sample collection nor time since last feed affected any of the HM ARH concentrations.
Maternal body composition and appetite-regulating hormones in human milk (RQ1)
We found maternal BMI positively associated with both HM leptin and insulin, but not with HM adiponectin. The association with HM leptin was mediated through plasma leptin concentrations to a stronger degree when BMI was set to ≥ 25kg/m2 compared to < 25kg/m2. There was no mediated effect through plasma insulin in the association between maternal BMI and HM insulin, but a mediated effect was present when using FMI as exposure.
Studies have suggested that leptin is secreted from secretory cells in the membrane of the mammary glands (46), from the adipose tissue or that it traverses the basolateral membrane of the epithelial cells from the blood stream to the milk (47). Our results suggest that the route through maternal circulation is more prominent in mothers with overweight compared to mothers with normal-weight. The percentage at which the association between maternal BMI and HM leptin can be explained by the circulating levels is about 50% for mothers with overweight and about 30% for mothers with normal-weight. Accordingly, a direct effect is more prominent in mothers with normal-weight compared to those overweight. The estimated direct effect of maternal adiposity covers other mechanisms than through maternal circulation, which to our knowledge include secretion from the mammary gland and the adipose tissue. However, the latter two mechanisms are not distinguished in the present analysis. A greater mediated effect seen in mothers with overweight support the influence of adiposity on low-grade inflammation in these mothers, in whom enlarged adipocytes are suggested to produce more leptin. The adipocytes are known to further secrete inflammatory cytokines such as tumor-necrosis factor-α (TNF-α) and interleukins, which upregulate mRNA expression of leptin in the adipocytes, creating a loop of compounding signaling components maintaining a chronic inflammatory state (10, 48). However, inflammation markers such as TNF-α and CRP were not measured in the present study, which could be relevant to include for assessment of the inflammatory condition and justifying the use of leptin as a marker of low-grade inflammation. In mediation analyses associating FMI with HM leptin, a mediated effect was only present when FMI was fixed to ≤9 compared to >9. More mothers categorized as overweight (≤28%) than categorized as having excessive fat mass (≤17%) reduces power and could explain the absence of a mediated effect.
Lastly, the direct and total effects of maternal BMI and FMI were considerable higher for leptin compared to insulin, which shows a distinctly stronger influence of maternal adiposity on HM leptin compared to HM insulin. This can possibly be explained by leptin secretion directly from the adipose tissue, possibly also locally in the breast.
The origin of HM insulin is less investigated, but a study by Whitmore et al. suggests that HM insulin is transported from the circulation as they found insulin in milk of mothers with type 1 DM originating from exogenous insulin administration (17). On the contrary, HM insulin is also suggested to originate from the epithelial cells of the mammary gland (16). As we found no prominent indirect effect through maternal plasma insulin, our results support the latter findings. In addition, insulin is the only hormone of the three investigated, which we found in higher concentrations in HM compared to plasma. This has been shown previously (49) and supports that insulin is secreted into the milk through other mechanisms than through circulating insulin, possibly from the epithelial cells. Our results showed a mediated effect through plasma insulin when using FMI as the exposure. Although the adipose tissue does not secrete insulin, adiposity contributes to insulin resistance and might explain the mediated effect through plasma. All biological samples were non-fasting due to ethical concerns for lactating mothers, however, adjusting for time since last feed did not change the results.
We found no association between maternal body composition and HM adiponectin. Aside from one study which reported positive associations between maternal body composition and HM adiponectin (50), most recent studies found positive correlations with maternal circulating adiponectin and not body composition (23). This could indicate that HM adiponectin to some degree originates from the circulation, however, this was not investigated in the present study.
Leptin in HM of mothers with female compared to male offspring was 30% higher in the initial model, however, only borderline significant. The results support the findings by Weyermann and colleagues, who reported lower HM leptin levels from women with male compared to female offspring (51). Two separate studies found higher circulating leptin concentrations in breastfed females compared to breastfed males (52, 53), which could be explained by increased ingested leptin. Whether higher circulating leptin for females is caused by increased ingestion from HM, or if the milk leptin is “tailored” to the sex of the infant is not yet established. Other possible explanations could be either a larger fat mass for females compared to males (54) or by testosterone in males suppressing leptin secretion (55). A study by Fields et al. found higher HM insulin in mothers with compared to without obesity, but only in mothers with female offspring (56). A similar interaction between BMI and infant sex for HM insulin was also found in the present study. Fields and colleagues suggest that hormone secretion by the placenta during pregnancy differing by infant sex might contribute to these results. Infant-driven factors could similarly affect the association, e.g., lower milk intake for female offspring increasing insulin concentration in the milk if total insulin was constant across individuals. In the present study, mothers with female compared to male offspring had a 4% greater increase in HM insulin per increase in BMI unit. Furthermore, HM insulin across individuals was constant in mature milk, so a lower milk intake for female offspring could have affected the association. Estimates in the study by Fields et al. were considerably larger with a 179% higher HM insulin in mothers with obesity with female offspring compared to male offspring. Compared to our results, this might indicate that the interaction with infant sex has a greater effect the higher the BMI.
Appetite-regulating hormones in human milk and infant outcomes (RQ2)
Insulin concentrations in HM was negatively associated with total milk intake, but only in male offspring, and not female, suggesting that HM insulin influence appetite-regulation in male and female offspring differently. Insulin in HM was furthermore positively associated with infant weight with 13g increase in body weight per 10% increase in HM insulin. The study by Young et al. report inverse associations between HM insulin and WLZ z-scores in infants of mothers with normal-weight (26), which indicate that insulin either affected infant length positively or infant weight negatively. The latter being opposite to our findings and we did not find positive associations with length. However, lack of associations seen in infants of mothers with obesity might be explained by a greater infant weight, which could mask the association. A study by Chan et al. found that intermediate HM insulin concentrations were associated with lower WLZ at four months (21) in a U-shaped association, but Fields et al. found no associations between HM insulin and infant body composition during the first six months after birth (56). These studies all have sample sizes ≤52 and results need to be confirmed in other studies. However, both associations with HM insulin disappeared in mediation analyses, where sample size was reduced.
We found no associations between HM leptin concentrations and either total milk intake or milk intake per feed, but as expected, infant weight and mean-centered age were independently associated with the two intake variables. However, it is likely that milk intake is the driver of infant weight and not the other way around. We did not find any associations between neither HM leptin nor adiponectin and any of the growth outcomes, and the literature states conflicting results within this research area (25). Galante and colleagues found leptin concentrations in HM 5 days postpartum positively associated with infant fat mass at discharge for moderate-late preterm infants, however HM leptin was negatively associated with fat mass at 4 months’ corrected age (57). A considerable unknown factor is the plausible mechanism assumed when posing hypotheses regarding HM composition and associations with infant outcomes. Although gastrointestinal uptake of ingested leptin and adiponectin has been shown in animal studies (14, 27, 28), the mechanism has not been confirmed in humans. Studies often lack infant plasma concentrations, which could explain associations with growth, and even with infant plasma available, concentrations might reflect endogenous produced hormones rather than orally ingested. Finally, the hormones might correlate with other nutrients in HM, which may be the true driver of associations seen.
Materials and methods
Colostrum samples were collected between 24 and 72 h after birth, which was chosen for flexibility allowing most mothers to collect the sample. The variation in HM concentration of each hormone was largest for colostrum compared to any of the other visit’s samples, indicating either a physiologically greater variation in colostrum compared to mature milk, or that some samples might have been transition milk with lower concentrations compared to colostrum.
The use of mediation analyses holds the assumptions that no unmeasured confounding should influence (a) the exposure-outcome association, (b) the mediator-outcome association and/or (c) the exposure-mediator association. Counterfactual-based mediation analyses further assumes that (d) no unmeasured mediator-outcome effect are affected by the exposure, which is necessary in defining direct and indirect effects (33). In HM research, and especially observational studies using biomarkers in blood and/or HM, the assumptions are a concern as confounding can rarely be completely removed. Contrary to randomized controlled trials, the exposure in the present study (BMI/FMI groups) was not randomized, which increases the risk of unmeasured confounding. However, infant age and mean-centered age were assumed to be confounders in step a-c above and appropriately adjusted for. Furthermore, the risk of exposure-induced mediator-outcome confounding (d) is markedly reduced, when the mediator appears shortly after the exposure (58), which is the case in the present study.
Strengths and limitations
The main strength of the present study is the longitudinal design combined with the comprehensive data systematically collected from both mother and infant. Compared to similar studies, sample size is comparable, especially when using multilevel modelling which is robust to missing data points.
A main limitation in the present study is the use of assays yet to be validated for hormone analyses in human milk. Our results of HM leptin are in the low range of the wide range of concentrations presented in the review by Suwaydi et al., whereas insulin concentrations are within the range and adiponectin is lower than the presented range (59). For plasma concentrations of the ARHs, our results of leptin and insulin are comparable to the literature, while adiponectin is lower than reported elsewhere (60). However, the ratio between plasma and HM adiponectin is similar to previously reported (59–61), indicating that it might be the standard of the assay rather than the matrix that causes the low concentrations. As our results show a systematic underestimation compared to current literature, the underestimation is less likely to affect our results of associations between maternal adiposity and HM ARH as well as the mediated proportions found in mediation analyses. Suwaydi and colleagues further mention that factors such as time of collection (e.g., morning or afternoon), sample type (e.g., colostrum or mature milk), sampling (pre- and post-feed or complete breast expression) influence the concentrations measured and challenge comparison between studies. These considerations supplement the need for validated assays for analysis of ARH in HM.
Furthermore, it is a limitation that we generally had a reduced sample size in the group of mothers with overweight, and for infants with blood samples, which likely reduced power to detect significant associations. Therefore, studies are highly encouraged to include both maternal and infant blood samples as well as milk samples in order to more comprehensively investigate the signaling mechanisms through human milk. Using BMI for classification of overweight may overestimate adiposity in certain populations (62), which could be relevant in the present study as we find more women categorized as overweight compared to having excessive fat mass estimated by BIA. This is a limitation, and our results show discrepancy in estimates of these two exposures. This could reflect true differences in group sizes or potentially an overestimation of adiposity when using BMI. The discrepancy could hypothetically be explained by an underestimation of FM caused by overestimation of FFM due to breastmilk and hydration status effecting bioelectrical impedance assessment. However, fat mass has been shown to be overestimated in lactating women using the BIA method compared to the golden standard of deuterium oxide method (63), which emphasizes the relevance of studies determining body composition in lactating women using more precise techniques.
In conclusion, our results illuminate the influence of maternal adiposity on HM ARH composition possibly through obesity related low-grade inflammation and further describe the influence on infant outcomes. The present study is the first to use counterfactual-based mediation analyses, and thereby provides a novel statistical approach in the investigation of associations between maternal predictors of ARH in HM and infant growth. Future studies are needed to confirm the findings in large observational studies and by using same methodological approaches.
Data availability statement
The datasets for this article are not publicly available due to concerns regarding participant/patient anonymity. Requests to access the datasets should be directed to the corresponding author.
Ethics statement
This study involved human subjects including under-aged subjects (<18 years old). Written informed consent was obtained from all subjects, and all custody holders of the under-aged subjects gave written informed consent for participation. The study was approved by the National Committee on Health Research Ethics on 1st August 2017 (Journal number: H-17015174).
Author contributions
LA, KM, SC, AL, and CM participated in designing and conducting the study. HF analyzed the biological samples. SC and JL analyzed the data statistically. SC wrote the manuscript and had primary responsibility for the final content. All authors have read and approved the final manuscript.
Funding
This work was supported by the Bill & Melinda Gates Foundation (grant numbers: OPP1148405 and INV-002300), intramural USDA-Agricultural Research Service project 5306-51000-004-00D, and the University of Copenhagen. USDA is an equal opportunity employer and provider.
Acknowledgments
We thank all the mothers and infants participating in the MILQ study as well as all employers at the MILQ study in both the Danish and the International MILQ team. Lastly, this work was supported, in whole or in part, by the Bill & Melinda Gates Foundation (OPP1148405). Under the grant conditions of the Foundation, a Creative Commons Attribution 4.0 Generic License has already been assigned to the author accepted manuscript version that might arise from this submission.
Conflict of interest
The authors declare that the research was conducted in the absence of any commercial or financial relationships that could be construed as a potential conflict of interest.
Publisher’s note
All claims expressed in this article are solely those of the authors and do not necessarily represent those of their affiliated organizations, or those of the publisher, the editors and the reviewers. Any product that may be evaluated in this article, or claim that may be made by its manufacturer, is not guaranteed or endorsed by the publisher.
Supplementary material
The Supplementary Material for this article can be found online at: https://www.frontiersin.org/articles/10.3389/fnut.2022.1025439/full#supplementary-material
Abbreviations
ARH, appetite-regulating hormones; BMI, body mass index; CV, coefficient of variance; CI, confidence interval; CRP, C-reactive protein; DM, diabetes mellitus; EBF, exclusive breastfeeding/exclusively breastfed; FFM, fat-free mass; FFMI, fat-free mass index; FM, fat mass; FMI, fat mass index; HM, human milk; IQR, Interquartile range; MILQ, “Mothers Infants and Lactation Quality” study; MP, mediated proportion; NA, non-detectable; RQ, research question; SD, standard deviation; TNF-α, tumor-necrosis factor-α; V2, V3, V4, Visit 2, Visit 3, Visit 4; WHO, World Health Organization; WLZ, Weight-for-length Z-scores.
References
1. Butte NF, Lopez-Alarcon MG, Garza C. World health organization, nutrition for health and development. Geneva: World Health Organization (2002).
2. Geneva S. The optimal duration of exclusive breastfeeding. (2001). Available online at: http://www.who.int/entity/nutrition/publications/infantfeeding/optimal_duration_of_exc_bfeeding_report_eng.pdf (accessed February 3, 2017).
3. Horta BL, Loret de Mola C, Victora CG. Long-term consequences of breastfeeding on cholesterol, obesity, systolic blood pressure and type 2 diabetes: a systematic review and meta-analysis. Acta Paediatr. (2015) 104:30–7. doi: 10.1111/apa.13133
4. Horta BL, Rollins N, Dias MS, Garcez V, Pérez-Escamilla R. Systematic review and meta-analysis of breastfeeding and later overweight or obesity expands on previous study for world health organization. Acta Paediatr. (2022) 2022:16460. doi: 10.1111/apa.16460
5. Fields DA, Schneider CR, Pavela G. A narrative review of the associations between six bioactive components in breast milk and infant adiposity: heterogeneity of breast milk. Obesity. (2016) 24:1213–21. doi: 10.1002/oby.21519
6. Kratzsch J, Bae YJ, Kiess W. Adipokines in human breast milk. Best Pract Res Clin Endocrinol Metab. (2018) 32:27–38. doi: 10.1016/j.beem.2018.02.001
7. Obradovic M, Sudar-Milovanovic E, Soskic S, Essack M, Arya S, Stewart AJ, et al. Leptin and obesity: role and clinical implication. Front Endocrinol. (2021) 12:582887. doi: 10.3389/fendo.2021.585887
8. Robinson K, Prins J, Venkatesh B. Clinical review: adiponectin biology and its role in inflammation and critical illness. Crit Care. (2011) 15:221. doi: 10.1186/cc10021
9. Rahman MS, Hossain KS, Das S, Kundu S, Adegoke EO, Rahman MA, et al. Role of insulin in health and disease: an update. Int J Mol Sci. (2021) 22:6403. doi: 10.3390/ijms22126403
10. Iikuni N, Lam QLK, Lu L, Matarese G, La Cava A. Leptin and inflammation. Curr Immunol Rev. (2008) 4:70–9. doi: 10.2174/157339508784325046
11. Savino F, Sardo A, Rossi L, Benetti S, Savino A, Silvestro L. Mother and Infant body mass index, breast milk leptin and their serum leptin values. Nutrients. (2016) 8:383. doi: 10.3390/nu8060383
12. Catherine G, Karen WW. Anatomy and physiology of lactation. In: K Wambach, B Spencer editors. Breastfeeding and human lactation. Jones & Barlett Learning (2021).
13. Panuganti PL, Bazzano LA, Ley SH. Chapter 9 – hormones in human milk: a summary of the quantity, determinants, and health outcomes of milk hormones. In: MK Mcguire, D O’connor editors. Human Milk. Academic Press (2021). doi: 10.1016/B978-0-12-815350-5.00009-7
14. Newburg DS, Woo JG, Morrow AL. Characteristics and potential functions of human milk adiponectin. J Pediatr. (2010) 156:S41–6. doi: 10.1016/j.jpeds.2009.11.020
15. Schneider-Worthington CR, Bahorski JS, Fields DA, Gower BA, Fernández JR, Chandler-Laney PC. Associations among maternal adiposity, insulin, and adipokines in circulation and human milk. J Hum Lact Off J Int Lact Consult Assoc. (2021) 37:714–22. doi: 10.1177/0890334420962711
16. Hassiotou F, Lai C, Filgueira L, Stuebe A, Hartmann P, Geddes D. Mammary cells synthesize insulin during lactation. FASEB J. (2014) 28:622.3. doi: 10.1096/fasebj.28.1_supplement.622.3
17. Whitmore TJ, Trengove NJ, Graham DF, Hartmann PE. Analysis of insulin in human breast milk in mothers with type 1 and type 2 diabetes mellitus. Int J Endocrinol. (2012) 2012:e296368. doi: 10.1155/2012/296368
18. Cefalu WT. Insulin resistance: cellular and clinical concepts. Exp Biol Med Maywood NJ. (2001) 226:13–26. doi: 10.1177/153537020122600103
19. Dadres GS, Whitaker K, Haapala JL, Foster L, Smith KD, Teague AM, et al. Relationship of maternal weight status before, during, and after pregnancy with breast milk hormone concentrations. Obes Silver Spring Md. (2019) 27:621–8. doi: 10.1002/oby.22409
20. Whitaker KM, Marino RC, Haapala JL, Foster L, Smith KD, Teague AM, et al. Associations of maternal weight status before, during, and after pregnancy with inflammatory markers in breast milk. Obes Silver Spring Md. (2017) 25:2092–9. doi: 10.1002/oby.22025
21. Chan D, Goruk S, Becker AB, Subbarao P, Mandhane PJ, Turvey SE, et al. Adiponectin, leptin and insulin in breast milk: associations with maternal characteristics and infant body composition in the first year of life. Int J Obes. (2018) 42:36–43. doi: 10.1038/ijo.2017.189
22. de Fluiter KS, Kerkhof GF, van Beijsterveldt IALP, Breij LM, van Vark-van der Zee LC, Mulder MT, et al. Appetite-regulating hormone trajectories and relationships with fat mass development in term-born infants during the first 6 months of life. Eur J Nutr. (2021) 60:3717–25. doi: 10.1007/s00394-021-02533-z
23. Brunner S, Schmid D, Zang K, Much D, Knoeferl B, Kratzsch J, et al. Breast milk leptin and adiponectin in relation to infant body composition up to 2 years. Pediatr Obes. (2015) 10:67–73. doi: 10.1111/j.2047-6310.2014.222.x
24. Woo JG, Guerrero ML, Altaye M, Ruiz-Palacios GM, Martin LJ, Dubert-Ferrandon A, et al. Human milk adiponectin is associated with infant growth in two independent cohorts. Breastfeed Med. (2009) 4:101–9. doi: 10.1089/bfm.2008.0137
25. Juan Castell MF, Peraita-Costa I, Soriano JM, Llopis-Morales A, Morales-Suarez-Varela M. A review of the relationship between the appetite-regulating hormone leptin present in human milk and infant growth. Breastfeed Med. (2022) 17:98–111. doi: 10.1089/bfm.2021.0149
26. Young BE, Levek C, Reynolds RM, Rudolph MC, MacLean P, Hernandez TL, et al. Bioactive components in human milk are differentially associated with rates of lean and fat mass deposition in infants of mothers with normal vs. elevated BMI. Pediatr Obes. (2018) 13:598–606. doi: 10.1111/ijpo.12394
27. Casabiell X, Piñeiro V, Tomé MA, Peinó R, Diéguez C, Casanueva FF. Presence of leptin in colostrum and/or breast milk from lactating mothers: a potential role in the regulation of neonatal food intake. J Clin Endocrinol Metab. (1997) 82:4270–3. doi: 10.1210/jcem.82.12.4590
28. Sánchez J, Oliver P, Miralles O, Ceresi E, Picó C, Palou A. Leptin orally supplied to neonate rats is directly uptaken by the immature stomach and may regulate short-term feeding. Endocrinology. (2005) 146:2575–82. doi: 10.1210/en.2005-0112
29. Bode L, Raman AS, Murch SH, Rollins NC, Gordon JI. Understanding the mother-breastmilk-infant “triad.” Science. (2020) 367:1070–2. doi: 10.1126/science.aaw6147
30. Christian P, Smith ER, Lee SE, Vargas AJ, Bremer AA, Raiten DJ. The need to study human milk as a biological system. Am J Clin Nutr. (2021) 113:1063–72. doi: 10.1093/ajcn/nqab075
31. Berger PK, Plows JF, Jones RB, Pollock NK, Alderete TL, Ryoo JH, et al. Maternal blood pressure mediates the association between maternal obesity and infant weight gain in early postpartum. Pediatr Obes. (2019) 14:e12560. doi: 10.1111/ijpo.12560
32. Berger PK, Plows JF, Jones RB, Alderete TL, Yonemitsu C, Poulsen M, et al. Human milk oligosaccharide 2′-fucosyllactose links feedings at 1 month to cognitive development at 24 months in infants of normal and overweight mothers. PLoS One. (2020) 15:e0228323. doi: 10.1371/journal.pone.0228323
33. Richiardi L, Bellocco R, Zugna D. Mediation analysis in epidemiology: methods, interpretation and bias. Int J Epidemiol. (2013) 42:1511–9. doi: 10.1093/ije/dyt127
34. Allen LH, Hampel D, Shahab-Ferdows S, Andersson M, Barros E, Doel AM, et al. The mothers, infants, and lactation quality (milq) study: a multi-center collaboration. Curr Dev Nutr. (2021) 5:nzab116. doi: 10.1093/cdn/nzab116
35. Lingwood BE, Leeuwen AMS, Carberry AE, Fitzgerald EC, Callaway LK, Colditz PB, et al. Prediction of fat-free mass and percentage of body fat in neonates using bioelectrical impedance analysis and anthropometric measures: validation against the PEA POD. Br J Nutr. (2012) 107:1545–52. doi: 10.1017/S0007114511004624
36. Kelly TL, Wilson KE, Heymsfield SB. Dual energy X-ray absorptiometry body composition reference values from NHANES. PLoS One. (2009) 4:e7038. doi: 10.1371/journal.pone.0007038
37. MacKinnon DP, Lockwood CM, Williams J. Confidence limits for the indirect effect: distribution of the product and resampling methods. Multivar Behav Res. (2004) 39:99–128. doi: 10.1207/s15327906mbr3901_4
38. CRAN. R: the R project for statistical computing. (2022). Available online at: https://www.r-project.org/ (accessed May 2, 2022).
39. Ozenne B, Forman J. LMMstar: repeated measurement models for discrete times. (2022). Available online at: https://CRAN.R-project.org/package=LMMstar (accessed May 2, 2022).
40. Bates D, Maechler M, Walker S, Christensen RHB. lme4: linear mixed-effects models using “eigen” and S4. (2022). Available online at: https://CRAN.R-project.org/package=lme4 (accessed May 2, 2022).
41. Tingley D, Yamamoto T, Hirose K, Keele L, Imai K. mediation : R package for causal mediation analysis. J Stat Softw. (2014) 59:i05. doi: 10.18637/jss.v059.i05
42. Nguyen DAD, Neville MC. Tight junction regulation in the mammary gland. J Mammary Gland Biol Neoplasia. (1998) 3:233–46. doi: 10.1023/A:1018707309361
43. Bronsky J, Mitrova K, Karpisek M, Mazoch J, Durilova M, Fisarkova B, et al. Adiponectin, AFABP, and leptin in human breast milk during 12 months of lactation. J Pediatr Gastroenterol Nutr. (2011) 52:474–7. doi: 10.1097/MPG.0b013e3182062fcc
44. Schuster S, Hechler C, Gebauer C, Kiess W, Kratzsch J. Leptin in maternal serum and breast milk: association with infants’ body weight gain in a longitudinal study over 6 months of lactation. Pediatr Res. (2011) 70:633–7. doi: 10.1203/PDR.0b013e31823214ea
45. Andreas NJ, Hyde MJ, Herbert BR, Jeffries S, Santhakumaran S, Mandalia S, et al. Impact of maternal BMI and sampling strategy on the concentration of leptin, insulin, ghrelin and resistin in breast milk across a single feed: a longitudinal cohort study. BMJ Open. (2016) 6:e010778. doi: 10.1136/bmjopen-2015-010778
46. Smith-Kirwin SM, O’Connor DM, De Johnston J, Lancey ED, Hassink SG, Funanage VL. Leptin expression in human mammary epithelial cells and breast milk. J Clin Endocrinol Metab. (1998) 83:1810–3. doi: 10.1210/jcem.83.5.4952
47. Bonnet M, Delavaud C, Laud K, Gourdou I, Leroux C, Djiane J, et al. Mammary leptin synthesis, milk leptin and their putative physiological roles. Reprod Nutr Dev. (2002) 42:399–413. doi: 10.1051/rnd:2002034
48. Xu H, Uysal KT, Becherer JD, Arner P, Hotamisligil GS. Altered tumor necrosis factor-α (TNF-α) processing in adipocytes and increased expression of transmembrane TNF-α in obesity. Diabetes. (2002) 51:1876–83. doi: 10.2337/diabetes.51.6.1876
49. Young BE, Patinkin Z, Palmer C, de la Houssaye B, Barbour LA, Hernandez T, et al. Human milk insulin is related to maternal plasma insulin and BMI: but other components of human milk do not differ by BMI. Eur J Clin Nutr. (2017) 2017:75. doi: 10.1038/ejcn.2017.75
50. Martin LJ, Woo JG, Geraghty SR, Altaye M, Davidson BS, Banach W, et al. Adiponectin is present in human milk and is associated with maternal factors. Am J Clin Nutr. (2006) 83:1106–11. doi: 10.1093/ajcn/83.5.1106
51. Weyermann M, Brenner H, Rothenbacher D. Adipokines in human milk and risk of overweight in early childhood: a prospective cohort study. Epidemiology. (2007) 18:722–9. doi: 10.1097/EDE.0b013e3181567ed4
52. Socha P, Hellmuth C, Gruszfeld D, Demmelmair H, Rzehak P, Grote V, et al. Endocrine and metabolic biomarkers predicting early childhood obesity risk. Preventive aspects of early nutrition. Karger Publishers (2016). p. 81–8. Available online at: http://www.karger.com/Article/FullText/439489 (accessed Jun 21, 2017) doi: 10.1159/000439489
53. Hamann TV, Mørk FCB, Jensen AK, Jørgensen NR, Heidemann MS, Schou AJ, et al. Reference serum percentile values of adiponectin, leptin, and adiponectin/leptin ratio in healthy danish children and adolescents. Scand J Clin Lab Invest. (2022) 0:1–10.
54. Taylor RW, Gold E, Manning P, Goulding A. Gender differences in body fat content are present well before puberty. Int J Obes Relat Metab Disord J Int Assoc Study Obes. (1997) 21:1082–4. doi: 10.1038/sj.ijo.0800522
55. Luukkaa V, Pesonen U, Huhtaniemi I, Lehtonen A, Tilvis R, Tuomilehto J, et al. Inverse correlation between serum testosterone and leptin in men1. J Clin Endocrinol Metab. (1998) 83:3243–6. doi: 10.1210/jcem.83.9.5134
56. Fields DA, George B, Williams M, Whitaker K, Allison DB, Teague A, et al. Associations between human breast milk hormones and adipocytokines and infant growth and body composition in the first 6 months of life. Pediatr Obes. (2017) 12:78–85. doi: 10.1111/ijpo.12182
57. Galante L, Reynolds CM, Milan AM, Alexander T, Bloomfield FH, Jiang Y, et al. Metabolic hormone profiles in breast milk from mothers of moderate-late preterm infants are associated with growth from birth to 4 months in a sex-specific manner. Front Nutr. (2021) 8:641227. doi: 10.3389/fnut.2021.641227
58. VanderWeele TJ, Vansteelandt S, Robins JM. Effect decomposition in the presence of an exposure-induced mediator-outcome confounder. Epidemiol Camb Mass. (2014) 25:300–6. doi: 10.1097/EDE.0000000000000034
59. Suwaydi MA, Gridneva Z, Perrella SL, Wlodek ME, Lai CT, Geddes DT. Human milk metabolic hormones: analytical methods and current understanding. Int J Mol Sci. (2021) 22:8708. doi: 10.3390/ijms22168708
60. Nayak BS, Ramsingh D, Gooding S, Legall G, Bissram S, Mohammed A, et al. Plasma adiponectin levels are related to obesity, inflammation, blood lipids and insulin in type 2 diabetic and non-diabetic Trinidadians. Prim Care Diabetes. (2010) 4:187–92. doi: 10.1016/j.pcd.2010.05.006
61. Savino F, Lupica M, Benetti S, Petrucci E, Liguori S, Cordero Di Montezemolo L. Adiponectin in breast milk: relation to serum adiponectin concentration in lactating mothers and their infants. Acta Paediatr. (2012) 101:1058–62. doi: 10.1111/j.1651-2227.2012.02744.x
62. Kaluski DN, Keinan-Boker L, Stern F, Green MS, Leventhal A, Goldsmith R, et al. BMI may overestimate the prevalence of obesity among women of lower socioeconomic status. Obesity. (2007) 15:1808–15. doi: 10.1038/oby.2007.215
Keywords: human milk, infant growth, body composition, maternal adiposity, appetite-regulating hormones, leptin, insulin, adiponectin
Citation: Christensen SH, Lewis JI, Larnkjær A, Frøkiær H, Allen LH, Mølgaard C and Michaelsen KF (2022) Associations between maternal adiposity and appetite-regulating hormones in human milk are mediated through maternal circulating concentrations and might affect infant outcomes. Front. Nutr. 9:1025439. doi: 10.3389/fnut.2022.1025439
Received: 22 August 2022; Accepted: 13 October 2022;
Published: 04 November 2022.
Edited by:
Aifric O’Sullivan, University College Dublin, IrelandReviewed by:
Sophie Blat, INRAE, Nutrition Metabolisms Cancer (NUMECAN), FranceMariana Muelbert, The University of Auckland, New Zealand
Copyright © 2022 Christensen, Lewis, Larnkjær, Frøkiær, Allen, Mølgaard and Michaelsen. This is an open-access article distributed under the terms of the Creative Commons Attribution License (CC BY). The use, distribution or reproduction in other forums is permitted, provided the original author(s) and the copyright owner(s) are credited and that the original publication in this journal is cited, in accordance with accepted academic practice. No use, distribution or reproduction is permitted which does not comply with these terms.
*Correspondence: Sophie Hilario Christensen, c2NoQG5leHMua3UuZGs=