- 1Laboratory of Food Function and Nutrigenomics, College of Food Science and Technology, Hunan Agricultural University, Changsha, China
- 2Department of Neurobiology and Human Anatomy, School of Basic Medical Science, Central South University, Changsha, China
- 3College of Bioscience and Biotechnology, Hunan Agricultural University, Changsha, Hunan, China
Glycolipid metabolic disorder is a serious threat to human health. Dark tea is a kind of traditional Chinese tea, which may regulate the glycolipid metabolic disorders. Dark tea extract (DTE) is the water extraction obtained from dark tea. Compared with traditional dark tea, DTE has the benefits of convenient consumption and greater potential for promoting health. However, the regulation of DTE on glycolipid metabolism and its molecular mechanism is rarely investigated. In our study, DTE was used as raw material to study the effect and molecular mechanism of its intervention on the glycolipid metabolic in db/db diabetic mice by using multiomics analysis and modern biological techniques. (1) DTE could significantly reduce fasting glucose in diabetic db/db mice, and the higher dose group has a better effect. Histopathological examination showed that DTE slightly improve the number of islets and decrease the number of islet β cells in the pancreatic tissue in db/db mice. (2) RNA-Seq was used to analyze the gene expression in liver tissue. In terms of biological processes, DTE mainly affected the inflammation and fatty acid metabolism. In terms of cell components, the lipoprotein and respiratory chain are mainly affected. In the aspect of molecular function, DTE mainly affected the redox related enzyme activity, iron ion binding and glutathione transferase. Arachidonic acid metabolism pathway, glutathione metabolism and PPAR signaling pathway were enriched by DTE with the results of KEGG pathway enrichment. In addition, real-time PCR results confirmed that DTE could significantly activate key genes of PPAR signaling pathway like Fabp1, Cyp4a1, Ehhadh, Cyp4a32, Aqp7 and Me1. (3) 16s rDNA showed that DTE could significantly decrease the ratio of Firmicutes/Bacteroidetes and the abundance of Proteobacteria, and increased the relative abundance of Verrucomicrobia at the phylum level. At the genus level, the relative abundance of Akkermansia, Prevotellaceae, Bacteroides and Alloprevotella was significantly increased after DTE treatment. This study provides multiomics molecular evidence for the intervention effect of DTE on abnormal glucose and lipid metabolism and the application of precise nutritional diet intervention of dark tea extract.
Introduction
Glucolipid metabolism disorder is a metabolic disorder that seriously threatens human health and affects the quality of life. Glucolipid metabolism disorders mainly take insulin resistance, oxidative stress, chronic inflammation, and intestinal flora imbalance as their core pathologies, resulting in systemic multi-tissue organ dysfunction and multi-system damage, which need to be comprehensively prevented and controlled from the whole body (1). Insulin-related signaling pathways such as IRS/PI3K/AKT/GLUT4, IRS/PI3K/GSK, MAPK/ERK, and PI3K/Akt/GSK play important roles in the development of metabolic syndrome associated with abnormal glucolipid metabolism. In addition, insulin resistance induces heterotopical lipid deposition and reduces mitochondrial function, which in turn leads to storms of inflammatory factors and induces oxidative imbalance in the body. Oxidative stress state destroys antioxidant enzymes, and ultimately leads to abnormal glucolipid metabolism (2, 3). The NF-κB signaling pathway is an important link between inflammation and glucolipid metabolism disorder (4). PPARγ can inhibit the activity of NF-κB DNA and reduce the expression of NF-κB mediated inflammatory mediators, finally exerting the anti-inflammatory effect (5). Concurrently, PPARγ can regulate the expression of various adipokines, inflammatory factors in adipocytes, insulin signal transduction, and regulate the regular balance of insulin and glucolipid metabolism. After investigating the intestinal flora of obese and normal people, it is found that the risk of metabolic diseases such as diabetes and nonalcoholic fatty liver disease will be increased in people with low microbial abundance (6). According to a study on the intestinal flora of 345 Chinese patients with T2DM, the abundance of Firmicutes and Clostridium in the intestinal flora of patients with diabetes mellitus tended to decrease (7).
Dark tea is one of the six major teas in China and belongs to post-fermented teas. Depending on its origin and different fermentation method, it includes ripe Pu'er Tea, Fuzhuan Brick Tea, Liupao Tea, Qing Brick Tea and Kang Brick Tea (8). Dark tea is rich in bioactive components, such as alkaloids, flavonoids, free amino acids, polyphenols, polysaccharides and volatile compounds, etc. A large number of research reports have confirmed that, dark tea and its extracts had significant effects in regulating abnormal glucolipid metabolism (9, 10). Many studies have shown that dark tea can significantly inhibit the absorption rate of glucose and enhance the insulin resistance (11). A study reported that Qingzhuan tea extracts exerted potent inhibitory effects against α-glucosidase (12). Instant dark tea with high levels of theabrownines showed antioxidant ability and inhibitory activities of α-glucosidase and pancreatic lipase (13). A series of in vivo studies also found that dark tea affected abnormal glucose metabolism by decreasing insulin resistance. Fuzhuan Brick Tea significantly antagonized HFD-induced insulin resistance with elevations in serum leptin, TC, TG, LDL-C, blood urea nitrogen, uric acid, and creatinine levels. Furthermore, Fuzhuan brick tea alleviated insulin resistance through down-regulation of SIRP-α expression and activation of the insulin signaling Akt/GLUT4, FoxO1, and mTOR/S6K1 pathways in skeletal muscle (14). Pu'er Tea extract improve the insulin sensitivity and glucose tolerance by increasing the expression of GLUT4 in adipose tissues, maintaining insulin signal transduction and reducing the expression of gluconeogenesis-related genes in the liver (15). Moreover, studies have shown that black tea can improve the oxidation and catabolism of fatty acids, while inhibiting the generation and accumulation of fat. Fuzhuan Brick Tea polysaccharides could up-regulate the expression of genes related to fatty acid decomposition in rats and down-regulate the expression of genes related to lipogenesis (16). A randomized, double-blind, placebo-controlled study showed that consumption of Pu'er Tea extract was associated with improvements to lipid profile, including a mild reduction in cholesterol and the cholesterol: high-density lipoprotein ratio after only 4 weeks, as well as a reduction in triglycerides and very small-density lipoproteins (17). Pu'er Tea also ameliorates hepatic lipid metabolism, inflammation, and insulin resistance in mice with HFD-induced nonalcoholic steatohepatitis, presumably by modulating hepatic IL-6/STAT3 signaling (18). In conclusion, dark tea or its extracts may affect abnormal glucolipid metabolism by reducing blood glucose levels, reducing insulin resistance, affecting the metabolism of fatty acid, and improving the related inflammation factors.
Multi-Omics combines two or more omics research methods, such as genomics, transcriptomics, microbiology, proteomics or metabolomics, to conduct systematic research on biological samples, and integrate and analyze the data of each omics to deeply mine the biological data. In recent years, a large number of studies have unveiled the molecular mechanisms of abnormal glucolipid metabolism using multi-omics technology, as well as precision nutritional intervention based on transcriptomics. Currently, relevant studies have explored the effects of black tea on gene expression regulators and intestinal microecological balance based on multiomic technology. Study on that utilization of microbiological technology, Fuzhuan Brick Tea-induced increase in abundances of beneficial bacteria Clostridiaceae, Bacteroidales, and Lachnospiraceae and decreases in harmful Ruminococcaceae, Peptococcaceae, Peptostreptococcaceae, and Erysipelotrichaceae were causal antecedents for Fuzhuan Brick Tea to reduce obesity and improve metabolic disorders (19).
In this study, the extract of dark tea was used to investigate the effects of blood glucose and lipid levels in diabetic mice. Then, RNA-Seq sequencing was performed on the liver of the mice to analyze the gene expression differences in the liver, screen the differentially expressed genes, perform Gene Ontology (GO) functional annotation and enrichment analysis on the differentially expressed genes, and further perform Kyoto Encyclopedia of Genes and Genomes (KEGG) signaling pathway annotation and enrichment analysis on the differential genes. Finally, 16s rDNA sequencing was conducted on mouse droppings to preliminary analyze structure, abundance, diversity, the differential species at the genus level and the significantly differential species of intestinal micro-organisms in mice. This study will provide new foundations and insights for precision nutritional intervention and the action mechanism of dark tea in metabolic syndrome.
Materials and methods
Materials and chemicals
Dark tea extract (DTE) was provided by Hunan Tea Industry Group Co. Ltd. (Changsha, China). Metformin hydrochloride (MET) was purchased from Beijing Jingfeng Pharmaceutical Group Co., Ltd. (Beijing, China).
Animals and models
There were 40 male diabetic db/db mice and 10 C57BL/6 mice (8 weeks old, specific pathogen free grade) were obtained from Hunan Jingda Experimental Animal Co., Ltd. (SCXK (Xiang) 2019-0004) and raised in the barrier environment of Hunan Drug Safety Evaluation Research Center (SYXK (Xiang) 2015-0016). SPF male db/db mice were fasted for 12 h, and their fasting blood glucose was detected. Forty animals with fasting blood glucose higher than 11.1 mmol/L and body weight ranging from 28.9 to 36.0 g were randomly divided into control group, MET group (0.26 g/kg), low-dose DTE group (DTE-L, 0.78 g/kg) and high-dose DTE group (DTE-H, 1.56 g/kg), with 10 animals in each group. Another 10 male C57BL/6 mice of SPF were taken as the normal control group, with blood sugar values of 3.1–7.2 mmol/L and body weight of 19.3 23.1 g. Each dose group was given the corresponding drugs by gavage of 20 ml/kg once a day for 11 weeks, while the normal control group and the model control group were given the same volume by gavage. During the experiment, the weight and blood glucose of animals were monitored regularly every week. At the end of 11th week intervention, the mice were sacrificed by cervical vertebra after ether anesthesia. Then, serum was obtained from mouse eyes after centrifugation. Afterwards, liver and pancreas were collected and stored at-80 for further use.
Serum parameters analysis
The serum were used to evaluate triglyceride (TG), total cholesterol (CHO), high-density lipoprotein cholesterol (HDL), low-density lipoprotein cholesterol (LDL) (Wako Pure Chemical Industries, Ltd., Chuo-ku, Japan). Blood glucose level was measured using blood glucose test strip (Sinocare Inc Co. Ltd, Changsha, China). Insulin detection kit from Jiangsu MEIMIAN Biological Industry Co., Ltd. (Yancheng, China).
IRI and histopathology analysis
IRI = fasting blood glucose (mmol/L) × fasting insulin (mIU/L)/22.5. Histological Analysis and Morphometry Part of the liver tissue was fixed with 4% paraformaldehyde. After 24 h, the livers were embedded in paraffin wax. Then, the liver samples were processed using cryostat (CM1950, Leica, Germany) and stained with haematoxylin–eosin (H&E). Finally, these slices were observed using the Olympus light microscope.
Total RNA extraction and sequencing
The extraction and detection of total RNA of mice in each experimental group were completed by Beijing Novogene Technology Co., Ltd. (Beijing, China). RNA from the total sample was isolated and purified using Trizol (Invitrogen, USA). The quantity and purity of total RNA were then controlled by NanoDrop ND-1000, RNA integrity was tested by Bioanalyzer 2100, and RNA concentration was quantified by agarose electrophoresis. MRNAs with polyadenylation (PolyA) were purified by using oligo (dT) magnetic beads for specific capture, and the captured mRNA was fragmented by using a magnesium ion disruption kit at 94°C for 5–7 min under a high temperature condition, and the obtained fragmented RNA was reverse transcribed into cDNA by reverse transcriptase action. E. coli DNA polymerase I and RNase H were used to convert the compound double chains of these DNA and RNA into DNA double chains, and dUTP Solution was added into the two chains to fill the ends of the double-stranded DNA into flat ends and add an A base at each end to enable it to connect with the linker with T base at the end. Their fragment sizes were subsequently screened and purified using oligo(dT) magnetic beads. To be maintained at 95°Cfor 3 min by PCR-predenaturation, 98°C for a total of eight cycles of 15 s each, annealing to 60°C for 15 s, extension at 72°C for 30 s, and finally extension at 72°C for 5 min to form a library with a fragment size of 300 ± 50 bp. Finally, it was double-ended sequenced using Illumina NovaSeq™ 6,000 according to standard operations in sequencing mode PE150.
qRT-PCR analysis
To measure the expression of Fabp1, Cyp4a1, Ehhadh, Cyp4a32, Aqp7 and Me1 in the liver, total RNA of the liver was extracted using Omega total RNA Kit. RNA was reversely transcribed using PrimeScript RT Master Mix. QPCR primers were designed by Biological Engineering Co., Ltd. (Shanghai, China) based on the cDNA sequence. Real-time quantitative reverse transcription PCR (qRT PCR) was performed using SYBR GreenMaster Mix and QuantStudio 3 Flex according to the manufacturer's guidelines. The experiments were performed on a flat plate, three in each group in parallel, and using the 2−▵▵Ct method. The reaction procedure was as follows: 95°C for 10 min using a three-step cycle using the Rotor-Gene Q6200 real-time PCR system followed by 40 cycles of 95°C for 10 s, 60°C for 20 s and 72°C for 20 s. The reaction mixture (20 μl) consisted of 2 μl cDNA solution, 10 μl IQTM SYBRR Green Supermix, and 6 μl primers. Three for each group were parallel and the results were averaged. β-actin was used as a reference gene. The comparative cycle threshold method was used to evaluate the relative expression level of genes.
DNA extraction and 16S gene sequencing
DNA from different samples was extracted using the EZNA tool DNA Kit (D4015, Omega, Inc., USA) according to the manufacturer's instructions. The reagent that was designed to uncover DNA from trace amounts of the sample has been shown to be effective for the preparation of DNA of most bacteria. Nuclear-free water was used for blank. The total DNA was eluted in 50 μL of elution buffer and stored at −80°C until PCR measurement.
Statistical analysis
Results were expressed as mean ± SD. The significant differences between groups were analyzed by the One-way ANOVA followed Tukey's multiple comparison post-test, and by using the LSD procedure of SPSS 26, and different statistical significance were accepted at p < 0.05. The correlation between gut microbiota, serum index and metabolites were analyzed by SPSS statistical program. Statistical significance was set at p < 0.05.
Results
Effects of DTE on metabolic parameters including serum lipid and blood glucose levels in db/db mice
As shown in Figure 1, compared with the normal group, the fasting blood glucose of mice in the control group was significantly increased (p < 0.01). Compared with the control group, there is no significant hypoglycemic effect was observed in the DTE-L and DTE-H group at the levels of D0, D7, D14, D21, D28, D35, D42, D49, and D56. The fasting blood glucose of db/db diabetic mice in the DTE-L and DTE-H group was significantly reduced (p < 0.05 or p < 0.01) when D63, D70, and D77 were given. Besides, the reducing effects of the DTE-H group of fasting blood glucose in dB/dB diabetic mice were better than the DTE-L group in D49, D56, D63, D70, and D77. The fasting blood glucose of db/db mice in the MET was significantly reduced (p < 0.05 or p < 0.01) after administration of D21, D28, D35, D42, D49, D56, D63, D70, and D77.
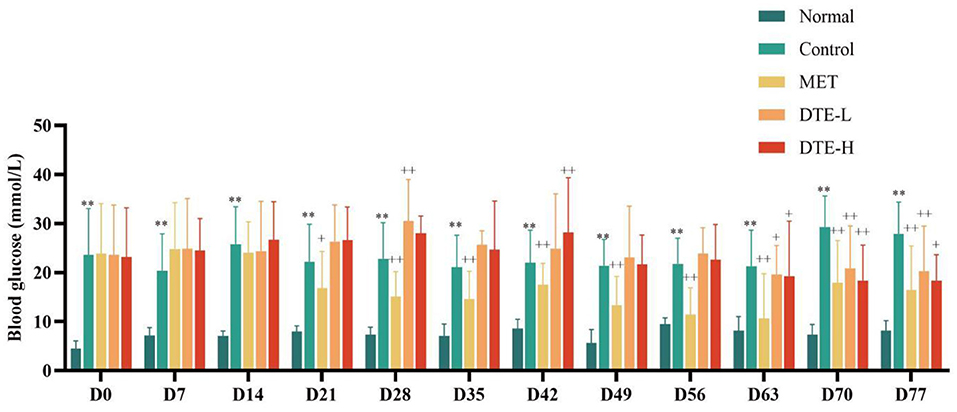
Figure 1. The effect of DTE on fasting blood glucose of db/db diabetic mice. Control, MET, DTE-L, DTE-H vs. Normal, **p < 0.01; MET, DTE-L, DTE-H vs. Control, +p < 0.05, ++p < 0.01.
HDL of the DTE-L and DTE-H groups showed an upward trend with no statistical difference with respect to the normal group. The content of TG of the control group, DTE-L group and DTE-H group tended to be decreased, but there was no statistical difference. The CHO level in the DTE-L group and DTE-H group was lower than that that of control group, but there was no statistical difference. Relative to the control group, the LDL level of the MET group increased significantly (p < 0.05) (Table 1).
Effect of DTE on serum insulin level and IRI of db/db mice
Compared with the normal group, serum insulin of db/db diabetic mice in the control group tended to decrease, and the IRI was significantly increased (p < 0.01). Compared with the control group, the serum insulin levels of db/db diabetic mice in the DTE-H group were significantly increased. The IRI of db/db diabetic mice in the MET group was decreased significantly (p < 0.01) (Table 2).
Effect of DTE on morphology of pancreas tissue in mice
As shown in Figure 2, the islets were spherical cell masses composed of endocrine cells, and each islet was composed of many cells, with a clear islet boundary in the normal control group. Compared to the normal group, the pathological score of pancreatic tissue hyperplasia in db/db diabetic mice was significantly increased (p < 0.01). In the control group, the islets of langerhans were seriously damaged, and the number of islets was significantly reduced, with the result that the islets became smaller, and the boundary was fuzzy. The number of β cells in the islets was significantly reduced, which was disorganized, with partial cell necrosis and unclear cell boundary. Compared to the control group, the pathological scores of pancreatic tissues in db/db diabetic mice in the MET group tended to decrease, but there was no statistical difference. The DTE-H group and MET group could slightly improve the damage of pancreatic tissue, vague islet boundary, and disordered arrangement of islet β cells in diabetic mice.
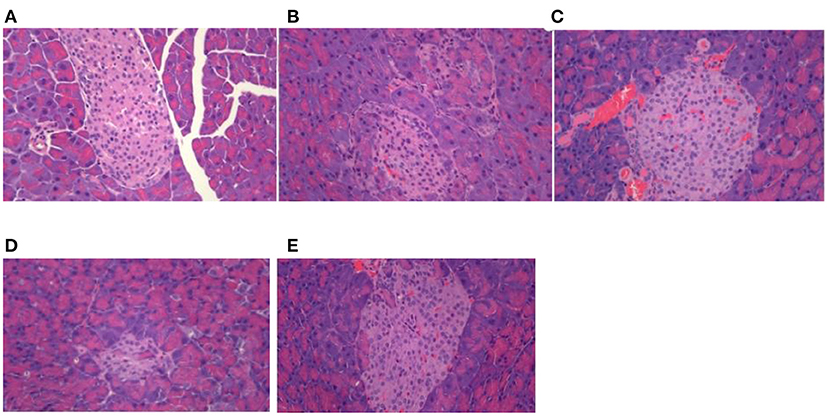
Figure 2. Effect of DTE on morphology of pancreas tissue in mice. (A) Normal group; (B) Control group; (C) MET group; (D) DTE-L group; (E) DTE-H group.
Effect of DTE on gene expression in liver of db/db mice
Data quality control required Q20> 90%, Q30> 85%, and GC content of 40–50% after filtration to indicate no abnormalities during sequencing. The minimum values of Q20 and Q30 for the samples were 96.75 and 91.30%, respectively, and the GC content was within the range of 46.49–49.86%, indicating that the sequencing quality was preferable. Principal component analysis (PCA) was also frequently used to assess inter-group differences and sample repetition within groups. Regarding the PCA data of the DTE group, we previously performed three sets of DTE, but only one set of experimental data was valid, and the experimental data showed significant differences between the DTE group, MET group, and control group. As shown in Figure 3, PC1 accounted for 41.55%, and it could significantly distinguish between the control group and other treatment groups, indicating that the other treatment groups had significant differences in liver transcriptional expression. PC2 accounted for 17.42%, significantly differentiating the MET group from the DTE group, indicating that DTE was able to regulate mouse gene expression at the transcriptome level.
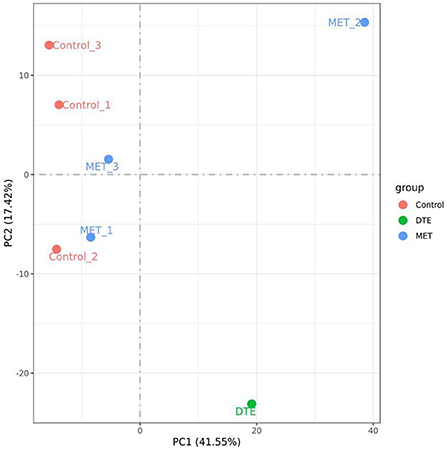
Figure 3. The PCA of the samples. The experimental data showed significant differences between the DTE group, MET group, and control group.
The data of gene transcriptional expression levels of the control, MET and DTE group obtained by RNA-Seq sequencing are shown in Figure 4. Compare to the Control group, DTE group had 1,506 differential genes, including 617 up-regulated genes and 889 down-regulated genes; Compare to the Control group, there were 777 differential genes in the MET group, including 441 down-regulated genes and 336 up-regulated genes; Compare to the DTE group, there were 336 differential genes in the MET group, including 233 down-regulated genes and 103 up-regulated genes.
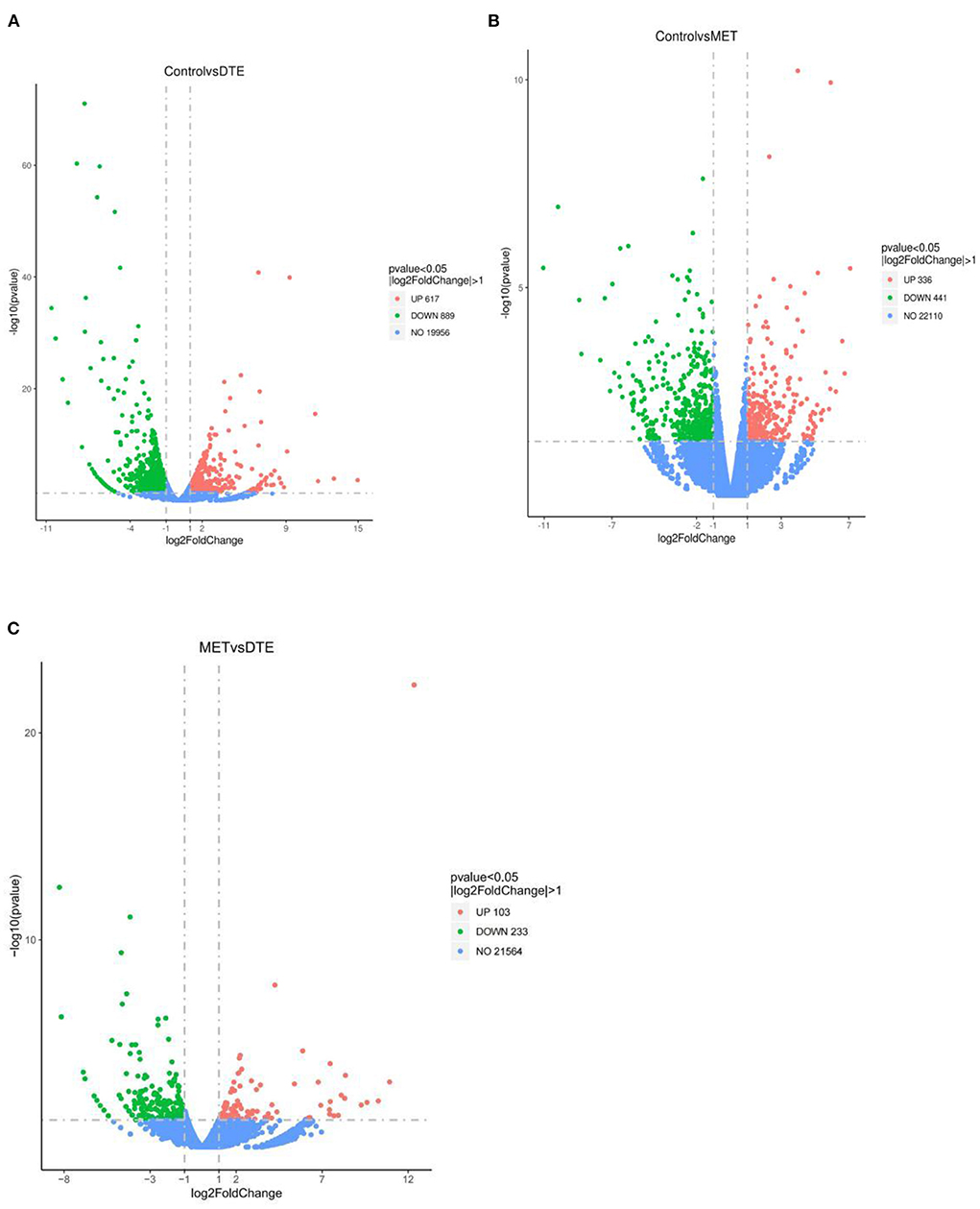
Figure 4. Volcanic map of difference gene expression between groups. (A) Control vs. DTE; (B) Control vs. MET; (C) MET vs. DTE.
Compare to the DTE group, the GO functional classification and enrichment analysis of differentially expressed genes in the control group were shown in Figure 5A. A total of 6,574 items were screened out, and a total of 423 items with significant statistical significance were screened out, including 294 items concerning biological processes (BP), 49 items concerning cellular components (CC) and 80 items concerning molecular functional processes (MF). In biological processes, the most significant 10 items were: response to stilbenoid, acute inflammatory response, response to bacterium, humoral immune response, fatty acid metabolic process, protein activation cascade, reactive oxygen species metabolic process, leukocyte migration involved in inflammatory response, positive regulation of response to external stimulu, and leukocyte migration. In cellular component, the most significant 10 items were: plasma lipoprotein particle, lipoprotein particle, extracellular matrix, protein-lipid complex, external side of plasma membrane, side of membrane, respiratory chain, collagen-containing extracellular matrix, respiratory chain complex, and high-density lipoprotein particle. In the molecular functional process, the most significant 10 items were: iron ion binding, heme binding, tetrapyrrole binding, monooxygenase activity, cofactor binding, glutathione transferase activity, arachidonic acid monooxygenase activity, oxidoreductase activity, peptidase regulator activity, and arachidonic acid epoxygenase activity. The biological effects of DTE are mainly concentrated on anti-inflammation, anti-oxidation, anti-bacteria, and fatty acid metabolism. In terms of cell composition, DTE mainly affects its lipoprotein and respiratory chain. In terms of molecular functional process, DTE mainly affects the activity of redox-related enzymes, iron ion binding and glutathione transferase, etc. Thus, DTE showed a regulatory effect on lipid metabolism, inflammation, and redox as compared with the control group.
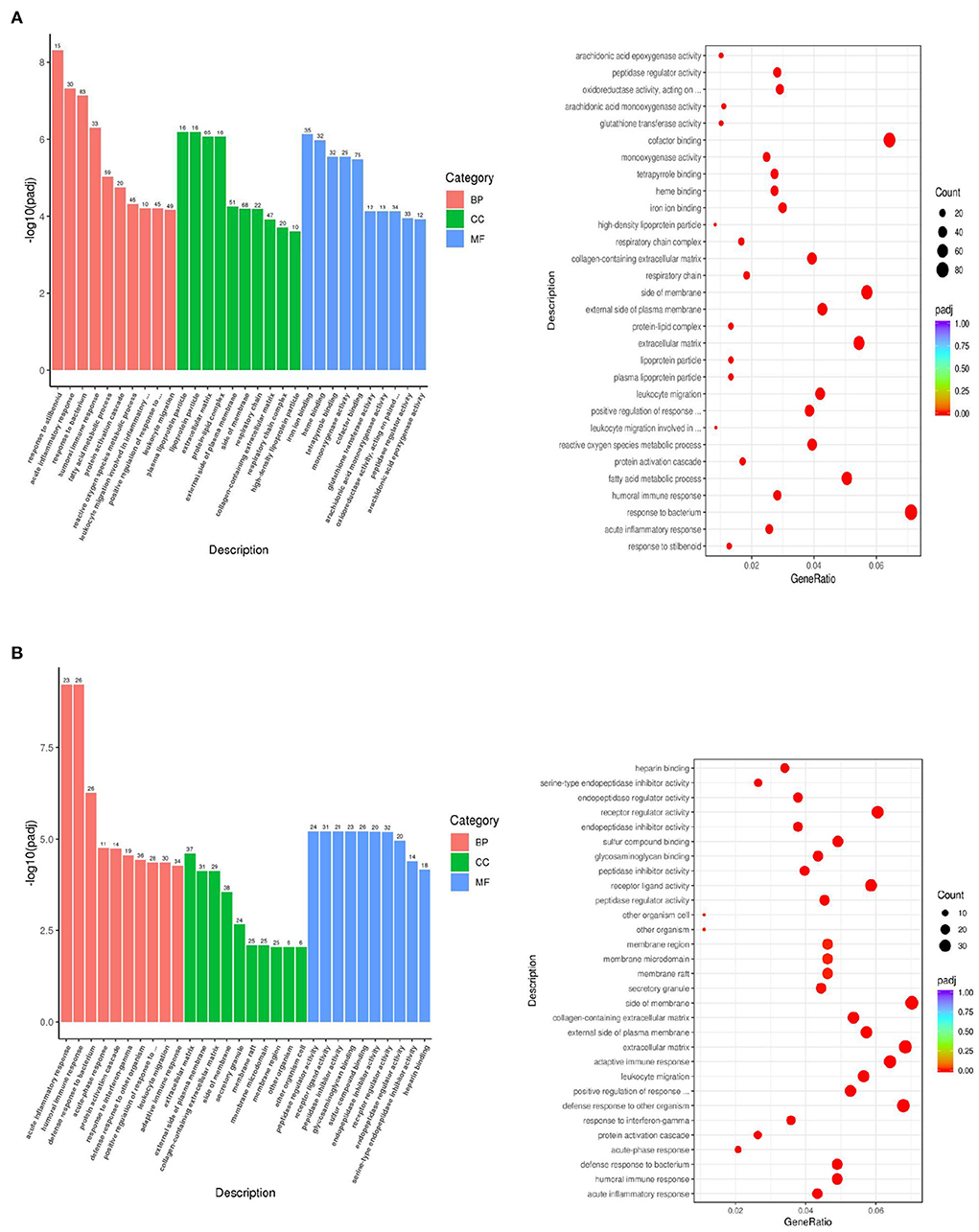
Figure 5. GO function analysis of difference gene expression. (A) Control vs. DTE; (B) Control vs. MET.
Compare to the MET group, the GO functional classification and enrichment analysis of differentially expressed genes in the control group are shown in Figure 5B. A total of 5,168 items were screened out, and a total of 248 items with significant statistical significance were screened out, including 191 items on biological process, 24 items on cellular component and 33 items on molecular functional process. In biological process, the most 10 significant items are: acute infectious response, humoral immune response, defense response to bacterium, acute-phase response, protein activation cascade, response to interferon-gamma, defense response to other organism, positive regulation of response to external stimulus, leukocyte migration, and adaptive immune response. In cellular component, the most 10 significant items are: extracellular matrix, externally side of plasma membrane, collagen-containing extracellular matrix, side of membrane, secretory grand, membrane raft, membrane microdomain, membrane region, other organism cell, and other organism cell. In items of molecular function, the most 10 significant entries were: peptidase regulator activity, receptor ligand activity, peptidase inhibitor activity, glycosaminoglycan binding, sulfur compound binding, endopeptidase inhibitor activity, receptor regulator activity, endopeptidase regulator activity, serine-type endopeptidase inhibitor activity, and heparin binding.
KEGG pathway analysis
As shown in Figure 6A, the KEGG biological pathways significantly enriched in the DTE group were as follows: Retinol metabolism, Chemical carcinogenesis, Arachidonic acid metabolism, PPAR signaling pathway, Drug metabolism enzymes, Staphylococcus aureus infection, Drug metabolism-cytochrome P450, Complement and coagulation cascades, Amoebiasis, atherosclerosis, Glutathione metabolism, and Oxidative phosphorylation. As shown in Figure 6B, the KEGG biological pathways significantly enriched in the MET group were as follows: Complement and coagulation cascades, Staphylococcus aureus infection, Cytokine-cytokine receptor interaction, Hematopoietic cell lineage, Systemic lupus erythematosus, Cell adhesion molecule, Amoebiasis, IL-17 signaling pathway, Chemokine signaling pathway, Glutathione metabolism, Primary immunodeficiency, Arachidonic acid metabolism.
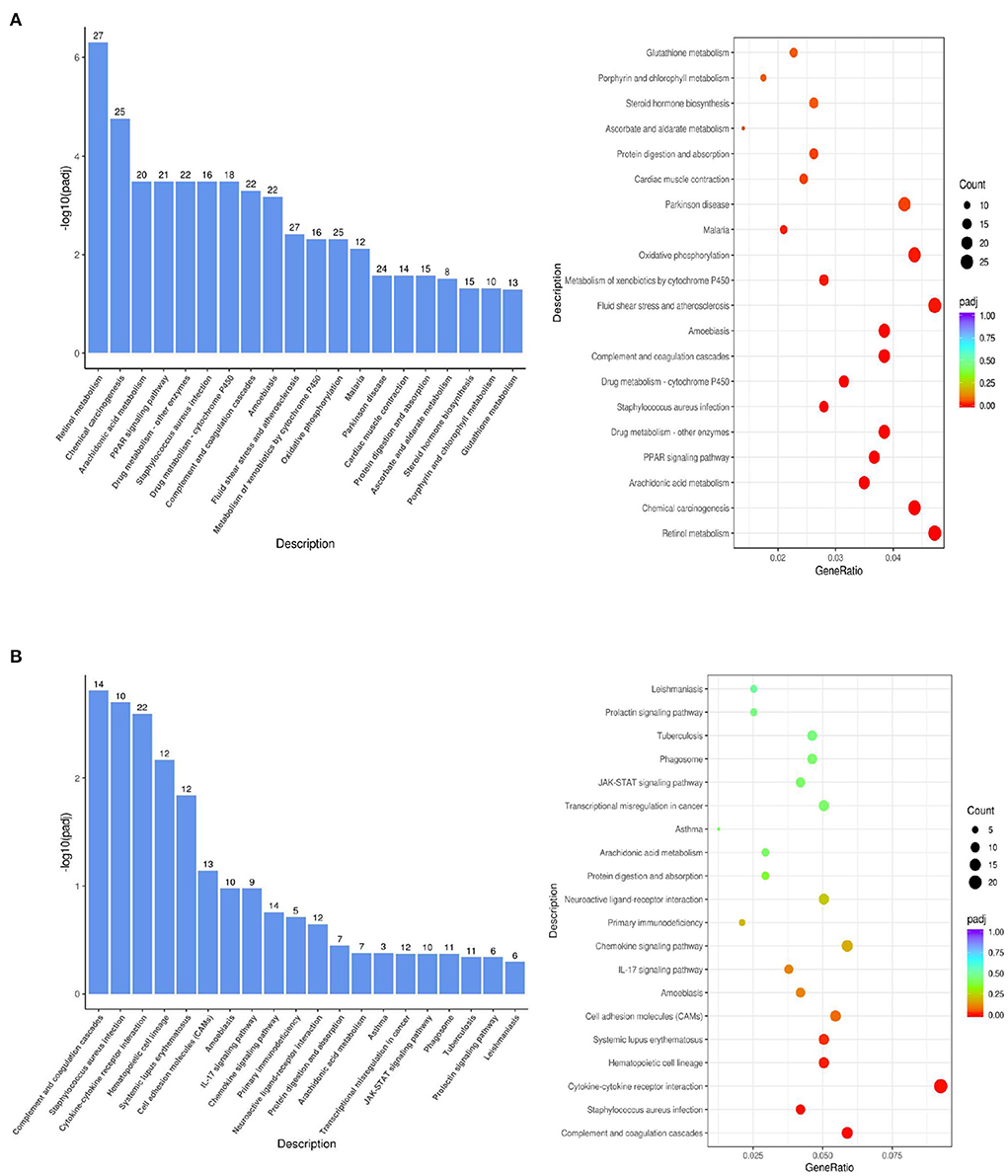
Figure 6. KEGG pathway analysis of the difference gene expression. (A) DTE vs. Control; (B) MET vs. Control.
In the comparison between DTE group and MET group, we found that two common signal pathways: Arachidonic acid metabolism pathway and Glutathione metabolism were significantly enriched. As shown in Figure 7A, in the arachidonic acid metabolism signaling pathway, the cytochrome P450 (CYPs) such as CYP2, CYP4A, and CYP4A11 are significantly upregulated. As shown in Figure 7B, in the glutathione metabolic pathway, Cyp4f18, Cyp2b13, Ltc4s, Pla2g4f , Alox15, and Cyp2c40 genes were significantly upregulated. In the comparison between the DTE group and the control group, we found that the PPAR signaling pathway also exhibited significant enrichment. As shown in Figure 7C, in the PPAR signaling pathway, the Cyp4a12a, Cyp4a1, Acaa1b, Cyp4a10, Pltp, Plin4, Scd1, Cyp4a1, Apoa2, Pparg, Lpl, Cyp8b1, Fabp2, Adipoq, Cyp7a1, Me1, Cyp4a32, Ubc/Acsl1 genes were up-regulated, and the Ehhadh, Fabp1, FABP3, FABP4 and Aqp7 genes were down-regulated, all of which were closely related to glycolipid metabolism.
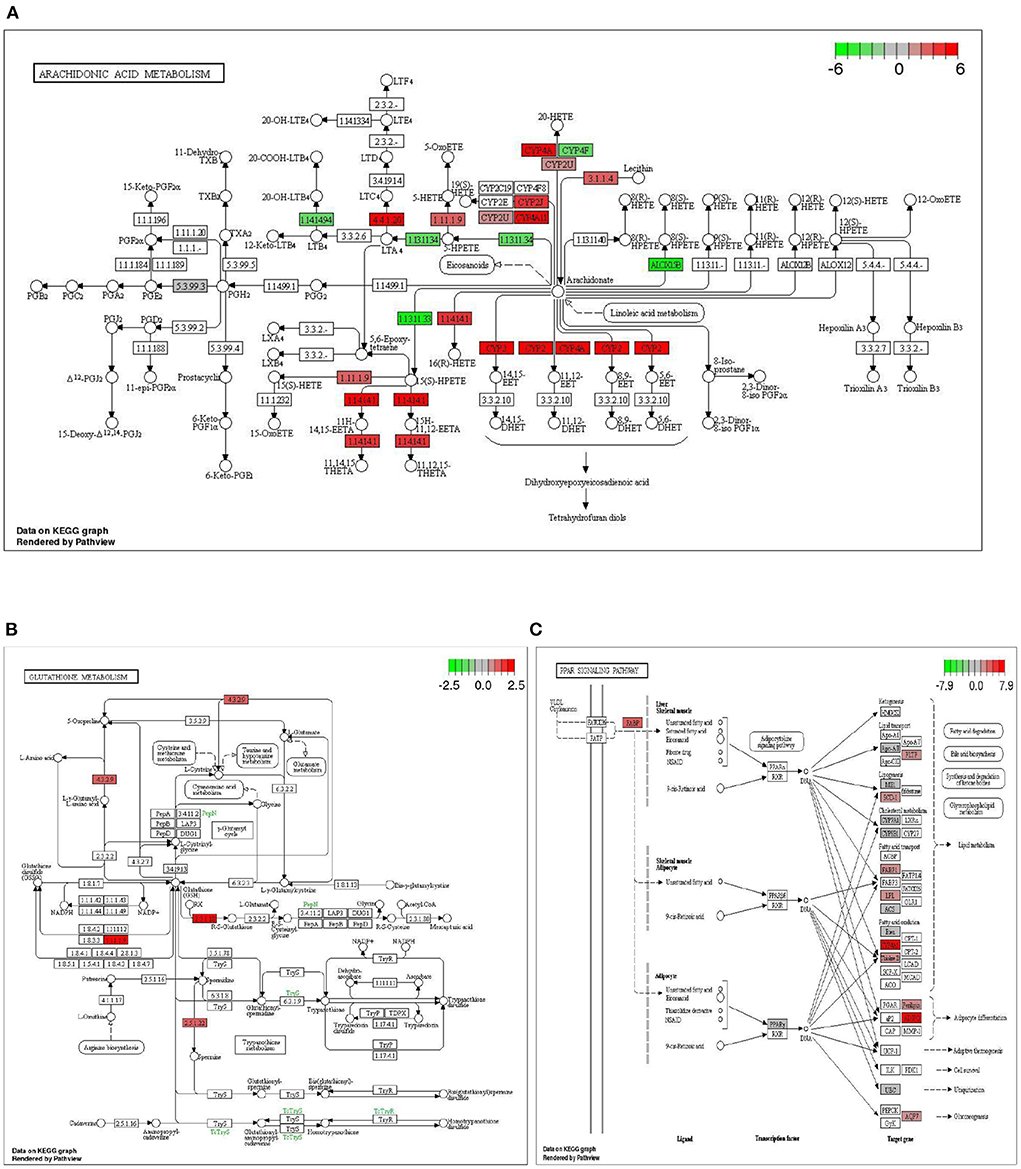
Figure 7. Significantly enriched signaling pathway by DTE. (A) Effect of DTE on Arachidonic acid metabolism pathway; (B) Effect of DTE on Glutathione metabolism pathway; (C) Effect of DTE on PPAR signaling pathway.
qRT-PCR analysis
To verify the effect of DTE on PPAR signaling pathway, qRT-PCR technology was used to analyze the genes related to glucolipid metabolism of Cyp4a1, Cyp4a32, Me1, Fabp1, Ehhadh, and Aqp7 in PPAR signaling pathway. The results of qRT-PCR were shown in Figure 8. Compared with the control group, the expressions of Cyp4a1, Cyp4a32, and Me1 genes involved in glycolipid metabolism were significantly up-regulated in DTE group, and the expressions of Fabp1, Ehhadh, and Aqp7 were significantly decreased (p < 0.05), suggesting that the extract of DTE could significantly activate key genes of PPAR signaling pathway, and the transcriptome sequencing result was credible.
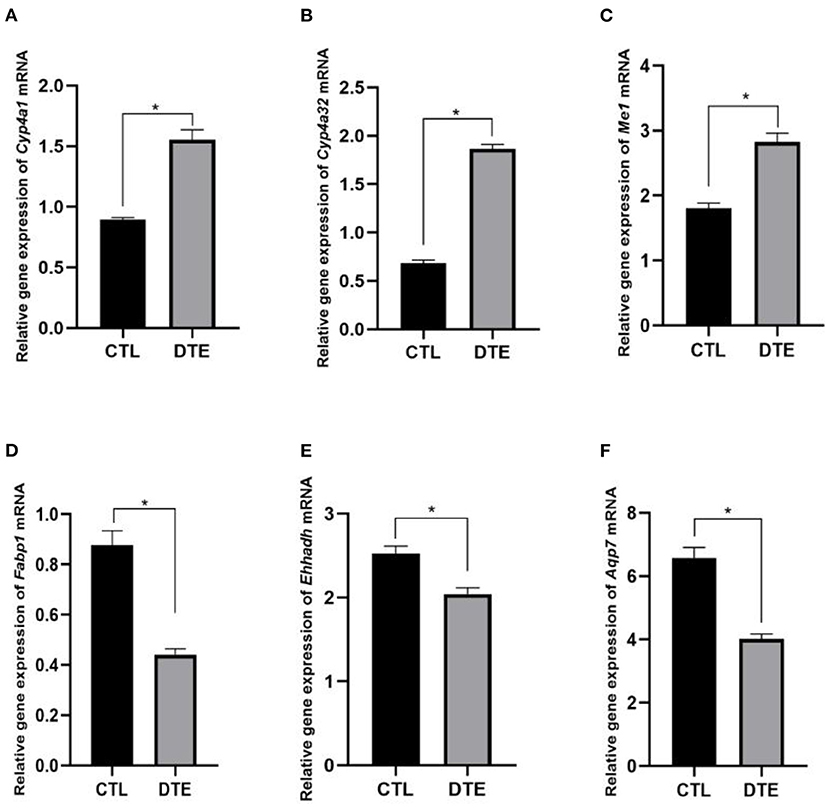
Figure 8. Effects of DTE on expression of PPAR signaling pathway related genes by qRT-PCR. (A) Cyp4a; (B) Cyp4a32; (C) Me1; (D) Fabp1; (E) Ehhadh; (F) Aqp7; DTE vs. Control, *p < 0.05; **p < 0.01.
Effects of DTE on composition of gut microbiota
As shown in Table 3, compare to the normal group, the community diversity, total number of species and abundance in the control, MET and DTE groups were decreased. Compare to the control group, the community diversity, total number of species and abundance in the MET group were decreased, while the community diversity in the DTE group was decreased, but the total number of species and abundance were increased without significant difference (p > 0.05). Compare to the MET group, the community diversity of the DTE group was decreased, and the total number and abundance of species were increased, but there was no significant difference (p > 0.05).
To study how the intestinal microflora of mice changed, we compared different groups of OTUs (Figure 9). There are 131 differences in normal group, 70 differences in control group, 45 differences in MET group and 72 differences in DTE group, which indicates that the abundance of intestinal microorganisms in each group is different. In addition, there are 252 identical OTUs in the four comparison groups, and the OTUs in the control group are more abundant than those in other groups.
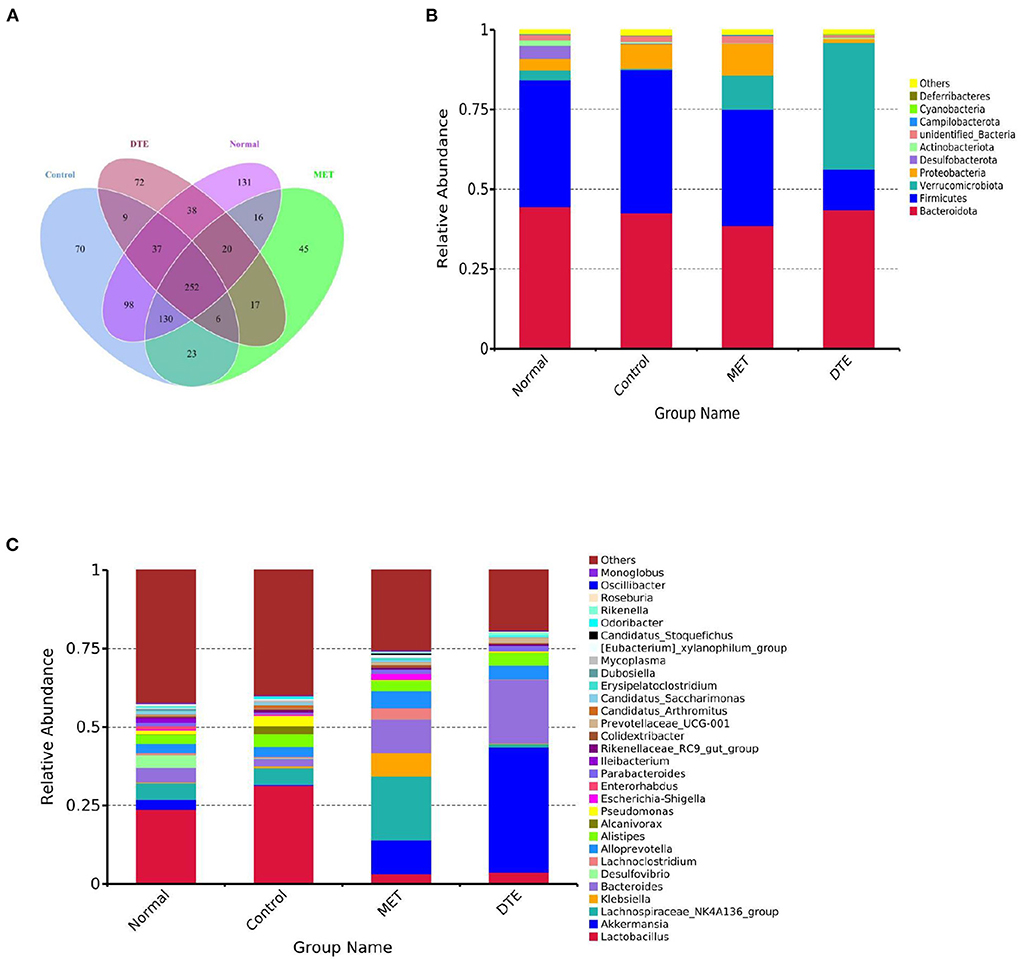
Figure 9. Effect of DTE on intestinal microbial community of diabetic mice. (A) Venn diagram based on OTU; (B) Effect of DTE on intestinal microbial community of diabetic mice at phylum species level; (C) Effect of DTE on intestinal microbial community of diabetic mice at genus level.
At the phylum level, as shown in Figure 9B, the relative abundance of Bacteroidetes and Firmicutes is higher in MET group and DTE group. Among the four groups, the relatively abundant ones are: Bacteroidetes, Firmicutes, Verrucomicrobia, Desulfobacterota, Proteobacteria, Actinobacteriota, Campilobacterota, Cyanobacteria, and Deferribacter. In normal group, control group, MET group and DTE group, the ratio of F/B was 0.89, 1.05, 0.94, 0.48, and 0.29, respectively. Compare to the normal group, the relative abundance of Proteobacteria in control group increased. In addition, compared with control group, DTE group significantly increased the relative abundance of Verrucomicrobia.
At the genus level, as shown in Figure 9C, the relative abundance of beneficial bacteria such as Bacteroide, Akkermansia, and Alloprevotella was decreased in the control group. The MET group significantly increased the relative abundance of Lachnospiraceae NK4A136, Alloprevotella, and Escherichia shigella. The DTE group significantly increased the relative abundance of Akkermansia, Prevotella, Bacteroides, and Alloprevotella.
Discussion
With the changes in diet structure, lifestyle and the increasingly serious aging trend, the incidence of metabolic syndrome, with glycolipid metabolic disorders as the core, is showing a rapidly rising trend worldwide (20). How to efficiently restore mitochondrial function, regulate oxidative stress and inflammatory signaling pathways is the goal of improving disorders of glucolipidic metabolism (21). The natural food active ingredient has remarkable effect on regulate glycolipid metabolism disorder. Cellular, animal, and clinical studies have shown that dark tea has a variety of physiological functions and health benefits, including anti-oxidant (22), anti-inflammatory (23), anti-cancer (24), anti-diabetes (25), prevent cardiovascular disease (26), and regulation of intestinal microbiota (27). However, effects of DTE on glucolipid metabolism by multi-omic strategy have been rarely studied. Thus, in this study, 16S rDNA and metabonomic approach were applied to comprehensively investigate the effects of DTE on glucolipid metabolism. Serum parameters indicated that DTE could regulate blood glucose and lipid levels. 16S rDNA sequencing and metabolomics showed significant effects on the composition of the intestinal microbiota and liver metabolites.
Our study showed that DTE may ameliorate metabolic disorders in db/db diabetic mice by significantly lowering blood glucose, serum insulin level, TC, TG, LDL, and lightly improve the islet damage. Dark tea has been reported to play an important role in reducing blood lipid and blood glucose. Fuzhuan brick tea water extracts could significantly suppress the increase of body weight and accumulation of adipose tissue, and reduced the level of serum triacylglycerol, total cholesterol and low-density lipoprotein (LDL) cholesterol in obese rats fed a high-fat diet (28). Qing Brick Tea exerted remarkable functions on decreasing the level of serum triglyceride and preventing hepatic fat accumulation (29). Pu'er Tea was found to have anti-hyperglycemic effects via inhibition on alpha-amylase and alpha-glucosidase (30). The above studies are consistent with our experimental results that DTE can regulate blood glucose and blood lipid.
RNA-Seq technique was used to preliminarily analyze the gene expression of mouse liver, and the potential differential metabolites and related metabolic pathways were analyzed from the perspective of metabonomics, which could reveal the effect of DTE on metabolic reaction of life system from the overall and dynamic perspective. Through GO functional classification and enrichment analysis, we found that DTE effects on biological processes mainly focused on anti-inflammatory, antioxidant, antibacterial and fatty acid metabolism. In terms of cell components, DTE mainly affects lipoprotein and respiratory chain. In terms of molecular functional process, DTE mainly affects its redox-related enzyme activity, iron binding and glutathione transferase. MET has been recognized as an effective drug to regulate glycolipid metabolism and reduce blood glucose (31). Compared with MET group, DTE group has some similarities in BP, CC, and MF. We speculate that DTE may have potential biological functions such as regulating glucose and lipid metabolism and improving inflammatory response.
By analyzing the enrichment results of KEGG pathway, we found that three typical signal pathways: Arachidonic acid metabolism pathway, Glutathione metabolism and PPAR pathway were significantly enriched. Arachidonic acid plays an important role in blood, liver, muscle and other organ systems as phospholipid-bound structural lipid. Cytochrome P450 (CYP) is a kind of protein with iron porphyrin as the auxiliary group, also known as monooxygenase, which mainly exists in the endoplasmic reticulum of liver cells. This enzyme can promote the metabolism of drugs, so it is also known as liver drug enzyme. Arachidonic acid is a bioactive substance of many circulating eicosanoic acid derivatives, such as prostaglandin E2 (PGE2), prostacyclin (PGI2), thromboxane A2 (TXA2), leukotrienes and C4 (LTC4), which plays an important role in regulating lipid protein metabolism, vascular elasticity, leukocyte function and platelet activation (32). In the signal pathway of arachidonic acid metabolism, CYP such as CYP2, CYP4A, CYP4A11 are significantly up-regulated. It was found that the extract of Chenopodium album can reduce the activity of cytochrome P4502E1 (CYP2E1), enhance the activity of catalase and improve the histological structure of rat liver to reduce the oxidative stress (33). We speculate that DTE may intervene the abnormal metabolism of glucose and lipid in the liver of diabetic mice through arachidonic acid metabolism.
Glutathione (GSH) is the most abundant antioxidant and a major detoxification agent in cells. It is synthesized through two-enzyme reaction catalyzed by glutamate cysteine ligase and glutathione synthetase, and its level is well regulated in response to redox change. Accumulating evidence suggests that GSH may play important roles in cell signaling (34). As shown in the effect of DTE on the glutathione metabolic pathway, Cyp4f18, Cyp2b13, Ltc4s, Pla2g4f , Alox15, and Cyp2c40 genes were significantly up-regulated. We speculated that DTE might intervene in glucolipid metabolism by regulating oxidative stress through the glutathione metabolic pathway.
PPAR is a core factor in the anti-inflammatory response pathway and participates in the formation of inflammatory and metabolic signal regulatory networks by different pathways, thus significantly affecting the regulation of glucolipid metabolism. PPARγ also can reduce the expression of NF-κB-mediated inflammatory mediators and exert its anti-inflammatory effect (35). PPARγ regulate the expression of many fat and inflammatory factors such as TNFα and regulate the glucolipid metabolism process (36). In the PPAR signaling pathway, Cyp4a12a, Cyp4a1, Acaa1b, Cyp4a10, Pltp, Plin4, Scd1, Cyp4a1, Apoa2, Pparg, Lpl, Cyp8b1, Fabp2, Adipoq, Cyp7a1, Me1, Cyp4a32, and Ubc/Acsl1 genes were up-regulated, and Ehhadh, Fabp1, FABP3, FABP4, and Aqp7 genes were down-regulated, all of which were closely related to glycolipid metabolism. The increased expression of FABP1 is mainly related to the occurrence of insulin resistance and the promotion of fatty acid transport (37). AQP7 also showed a significant correlation with glucolipid metabolism indicators (38). Since DTE may significantly up-regulate the genes expression of PPAR signaling pathway, we speculate that DTE may have the potential to regulate inflammatory factors, glycolipid metabolism, and promote fatty acid transport.
Intestinal microflora plays an important role in host health, the change of intestinal microflora may induce intestinal oxidative stress, and lead to glycolipid metabolism disorder (39). The ratio of Firmicutes/Bacteroidetes is widely believed to be important for maintaining the homeostasis of the intestinal environment. Reduction of the F/B value leads to T2D (40). After treatment with DTE, the value of F/B was decreased, indicating DTE positively regulated the gut microbiota. Bacteroidetes, Firmicutes, Proteobacteria and Verrucomicrobia can regulate glycolipid metabolism and improve insulin resistance (40). After treatment with DTE, the relative abundance of Proteobacteria was decreased, and the relative abundance of Verrucomicrobia was increased. These results indicated that DTE could regulate the structural composition of the intestinal flora to improve the intestinal environment and reduce the risks of diabetes and obesity.
At the genus level, treatment with DTE significantly increased the relative abundance of Akkermansia, Prevotellaceae, Bacteroides, and Alloprevotella. Akkermansia can regulate immune response, lipid metabolism, inhibit inflammation, and improve the disorder of intestinal flora. The decrease in abundance is closely related to the development of inflammatory intestinal diseases, T2D and cardiovascular diseases (41). As shown in Figure 9C, the proportion of Akkermansia in control group is 0.34%, and that in DTE group is 35.76%. The results showed that DTE could significantly increase the relative abundance of Akkermansia, improve the intestinal microenvironment, and then regulate the abnormal of glucose and lipid metabolism.
Conclusion
In the present study, multi-omics precision nutrition method was applied to carry out multi-dimensional health big data detection and analysis at the animal level from the aspects of the microbiome and RNA sequencing. We found that DTE could significantly reduce the blood glucose in db/db diabetic mice, improve the blood lipid to a certain extent, slightly repair the pancreatic injury, and promote the insulin secretion. According to GO analysis, DTE mainly affected the inflammation, fatty acid metabolism, and redox-related enzyme activities. 16S rDNA sequencing showed that DTE significantly reduced the ratio of F/B and increased the relative abundances of Akkermansia, Prevotellaceae, Bacteroides, and Alloprevotella. Besides, real-time qPCR was performed to verify the regulatory effect of DTE on PPAR signaling pathway, which provides us with direct and comprehensive molecular evidence for the beneficial effect of DTE on metabolic disorders.
Data availability statement
The data presented in the study are deposited in the GSA repository: https://ngdc.cncb.ac.cn/gsub/, accession number CRA007923.
Ethics statement
The animal study was reviewed and approved by Hunan Drug Safety Evaluation Research Center.
Author contributions
CL and MH wrote the original draft. CL, MH, and CW performed the experiments and collected the samples. CL, YY, and XW contributed to methodology and data analysis. MS and CZ participated in supervision. CL, MS, and CZ contributed to project administration and conceptualization, writing, reviewing, and editing. All authors listed have made a substantial, direct, and intellectual contribution to the work and approved it for publication.
Funding
This work was partially supported by the National Key Research and Development Program of China (2019YFC1604903) and the Hunan Province Innovative Postdoctoral Project (2021RC2080).
Conflict of interest
The authors declare that the research was conducted in the absence of any commercial or financial relationships that could be construed as a potential conflict of interest.
Publisher's note
All claims expressed in this article are solely those of the authors and do not necessarily represent those of their affiliated organizations, or those of the publisher, the editors and the reviewers. Any product that may be evaluated in this article, or claim that may be made by its manufacturer, is not guaranteed or endorsed by the publisher.
References
1. Guo J. Research progress on prevention and treatment of glucolipid metabolic disease with integrated traditional Chinese and western medicine. Chin J Integr Med. (2017) 23:403–9. doi: 10.1007/s11655-017-2811-3
2. You M, Matsumoto M, Pacold CM, Cho WK, Crabb DW. The role of Amp-activated protein kinase in the action of ethanol in the liver. Gastroenterology. (2004) 127:1798–808. doi: 10.1053/j.gastro.2004.09.049
3. Hou L, Jiang F, Huang B, Zheng W, Jiang Y, Cai G, et al. Dihydromyricetin ameliorates inflammation-induced insulin resistance via phospholipase C-Camkk-Ampk signal pathway. Oxid Med Cell Longev. (2021) 2021:8542809. doi: 10.1155/2021/8542809
4. Barazzoni R, Gortan Cappellari G, Ragni M, Nisoli E. Insulin resistance in obesity: an overview of fundamental alterations. Eat Weight Disord. (2018) 23:149–57. doi: 10.1007/s40519-018-0481-6
5. Zhao Y, Li Z, Lu E, Sheng Q, Zhao Y. Berberine exerts neuroprotective activities against cerebral ischemia/reperfusion injury through up-regulating ppar-Γ to suppress Nf-Kb-mediated pyroptosis. Brain Res Bull. (2021) 177:22–30. doi: 10.1016/j.brainresbull.2021.09.005
6. Le Chatelier E, Nielsen T, Qin J, Prifti E, Hildebrand F, Falony G, et al. Richness of human gut microbiome correlates with metabolic markers. Nature. (2013) 500:541–6. doi: 10.1038/nature12506
7. Qin J, Li Y, Cai Z, Li S, Zhu J, Zhang F, et al. A metagenome-wide association study of gut microbiota in type 2 diabetes. Nature. (2012) 490:55–60. doi: 10.1038/nature11450
8. Zhu M-z, Li N, Zhou F, Ouyang J, Lu D-m, Xu W, et al. Microbial bioconversion of the chemical components in dark tea. Food Chemistry. (2020) 312:126043. doi: 10.1016/j.foodchem.2019.126043
9. Zhu J, Yu C, Zhou H, Wei X, Wang Y. Comparative evaluation for phytochemical composition and regulation of blood glucose, hepatic oxidative stress and insulin resistance in mice and Hepg2 models of four typical Chinese dark teas. J Sci Food Agric. (2021) 101:6563–77. doi: 10.1002/jsfa.11328
10. Wu Y, Sun H, Yi R, Tan F, Zhao X. Anti-obesity effect of liupao tea extract by modulating lipid metabolism and oxidative stress in high-fat-diet-induced obese mice. J Food Sci. (2021) 86:215–27. doi: 10.1111/1750-3841.15551
11. Wang PC, Zhao S, Yang BY, Wang QH, Kuang HX. Anti-diabetic polysaccharides from natural sources: a review. Carbohydr Polym. (2016) 148:86–97. doi: 10.1016/j.carbpol.2016.02.060
12. Liu S, Yu Z, Zhu H, Zhang W, Chen Y. In vitro alpha-glucosidase inhibitory activity of isolated fractions from water extract of qingzhuan dark tea. BMC Complement Altern Med. (2016) 16:378. doi: 10.1186/s12906-016-1361-0
13. Wang Y, Zhang M, Zhang Z, Lu H, Gao X, Yue P. High-theabrownins instant dark tea product by aspergillus niger via submerged fermentation: A-glucosidase and pancreatic lipase inhibition and antioxidant activity. J Sci Food Agric. (2017) 97:5100–6. doi: 10.1002/jsfa.8387
14. Du H, Wang Q, Yang X. Fu Brick tea alleviates chronic kidney disease of rats with high fat diet consumption through attenuating insulin resistance in skeletal muscle. J Agric Food Chem. (2019) 67:2839–47. doi: 10.1021/acs.jafc.8b06927
15. Cai X, Hayashi S, Fang C, Hao S, Wang X, Nishiguchi S, et al. Pu'erh tea extract-mediated protection against hepatosteatosis and insulin resistance in mice with diet-induced obesity is associated with the induction of de novo lipogenesis in visceral adipose tissue. J Gastroenterol. (2017) 52:1240–51. doi: 10.1007/s00535-017-1332-3
16. Chen G, Xie M, Wan P, Chen D, Dai Z, Ye H, et al. Fuzhuan brick tea polysaccharides attenuate metabolic syndrome in high-fat diet induced mice in association with modulation in the gut microbiota. J Agric Food Chem. (2018) 66:2783–95. doi: 10.1021/acs.jafc.8b00296
17. Jensen GS, Beaman JL, He Y, Guo Z, Sun H. Reduction of body fat and improved lipid profile associated with daily consumption of a puer tea extract in a hyperlipidemic population: a randomized placebo-controlled trial. Clin Interv Aging. (2016) 11:367–76. doi: 10.2147/CIA.S94881
18. Cai X, Fang C, Hayashi S, Hao S, Zhao M, Tsutsui H, et al. Pu-Erh tea extract ameliorates high-fat diet-induced nonalcoholic steatohepatitis and insulin resistance by modulating hepatic Il-6/Stat3 signaling in mice. J Gastroenterol. (2016) 51:819–29. doi: 10.1007/s00535-015-1154-0
19. Liu D, Huang J, Luo Y, Wen B, Wu W, Zeng H, et al. Fuzhuan brick tea attenuates high-fat diet-induced obesity and associated metabolic disorders by shaping gut microbiota. J Agric Food Chem. (2019) 67:13589–604. doi: 10.1021/acs.jafc.9b05833
20. Zhou Y-J, Xu N, Zhang X-C, Zhu Y-Y, Liu S-W, Chang Y-N. Chrysin improves glucose and lipid metabolism disorders by regulating the Ampk/Pi3k/Akt signaling pathway in insulin-resistant Hepg2 cells and Hfd/Stz-induced C57bl/6j mice. J Agric Food Chem. (2021) 69:5618–27. doi: 10.1021/acs.jafc.1c01109
21. Arnold SE, Arvanitakis Z, Macauley-Rambach SL, Koenig AM, Wang H-Y, Ahima RS, et al. Brain insulin resistance in type 2 diabetes and alzheimer disease: concepts and conundrums. Nature Reviews Neurology. (2018) 14:168–81. doi: 10.1038/nrneurol.2017.185
22. Zhao P, Alam MB, Lee S-H. Protection of Uvb-induced photoaging by fuzhuan-brick tea aqueous extract via Mapks/Nrf2-mediated down-regulation of Mmp-1. Nutrients. (2019) 11:60. doi: 10.3390/nu11010060
23. Zhang X, Wu Q, Zhao Y, Aimy A, Yang X. Consumption of post-fermented Jing-Wei Fuzhuan brick tea alleviates liver dysfunction and intestinal microbiota dysbiosis in high fructose diet-fed mice. RSC Adv. (2019) 9:17501–13. doi: 10.1039/C9RA02473E
24. Zheng K, Zhao Q, Chen Q, Xiao W, Jiang Y, Jiang Y. The synergic inhibitory effects of dark tea (Camellia sinensis) extract and p38 inhibition on the growth of pancreatic cancer cells. J Cancer. (2019) 10:6557–69. doi: 10.7150/jca.34637
25. Gao W, Xiao C, Hu J, Chen B, Wang C, Cui B, et al. Qing Brick Tea (Qbt) aqueous extract protects monosodium glutamate-induced obese mice against metabolic syndrome and involves up-regulation transcription factor nuclear factor-erythroid 2-related factor 2 (Nrf2) antioxidant pathway. Biomed Pharmacother. (2018) 103:637–44. doi: 10.1016/j.biopha.2018.04.043
26. Liu Y, Luo L, Luo Y, Zhang J, Wang X, Sun K, et al. Prebiotic properties of green and dark tea contribute to protective effects in chemical-induced colitis in mice: a fecal microbiota transplantation study. J Agric Food Chem. (2020) 68:6368–80. doi: 10.1021/acs.jafc.0c02336
27. Chen G, Xie M, Wan P, Chen D, Ye H, Chen L, et al. Digestion under Saliva, simulated gastric and small intestinal conditions and fermentation in vitro by human intestinal microbiota of polysaccharides from Fuzhuan brick tea. Food Chem. (2018) 244:331–9. doi: 10.1016/j.foodchem.2017.10.074
28. Li Q, Liu Z, Huang J, Luo G, Liang Q, Wang D, et al. Anti-obesity and hypolipidemic effects of Fuzhuan brick tea water extract in high-fat diet-induced obese rats. J Sci Food Agric. (2013) 93:1310–6. doi: 10.1002/jsfa.5887
29. Mao QQ, Li BY, Meng JM, Gan RY, Xu XY, Gu YY, et al. Effects of several tea extracts on nonalcoholic fatty liver disease in mice fed with a high-fat diet. Food Sci Nutr. (2021) 9:2954–67. doi: 10.1002/fsn3.2255
30. Yang C-Y, Yen Y-Y, Hung K-C, Hsu S-W, Lan S-J, Lin H-C. Inhibitory effects of Pu-Erh tea on alpha glucosidase and alpha amylase: a systemic review. Nutr Diabetes. (2019) 9:23. doi: 10.1038/s41387-019-0092-y
31. Sanchez-Rangel E, Inzucchi SE. Metformin: clinical use in type 2 diabetes. Diabetologia. (2017) 60:1586–93. doi: 10.1007/s00125-017-4336-x
32. Wang B, Wu L, Chen J, Dong L, Chen C, Wen Z, et al. Metabolism pathways of arachidonic acids: mechanisms and potential therapeutic targets. Signal Transduct Target Ther. (2021) 6:94. doi: 10.1038/s41392-020-00443-w
33. Duh PD, Chen SY, Chu CC, Chyau CC, Fu ZH. Effect of Water Extract of Djulis (Chenopodium Formosaneum) and Its Bioactive Compounds on Alcohol-Induced Liver Damage in Rats. International J of Food and Nutritional Sci. (2018) 5:55–63. doi: 10.15436/2377-0619.18.1816
34. Zhang H, Forman HJ. Glutathione synthesis and its role in redox signaling. Semin Cell Dev Biol. (2012) 23:722–8. doi: 10.1016/j.semcdb.2012.03.017
35. Kong L, Chen J, Ji X, Qin Q, Yang H, Liu D, et al. Alcoholic fatty liver disease inhibited the co-expression of Fmo5 and Pparα to activate the Nf-Kb signaling pathway, thereby reducing liver injury via inducing gut microbiota disturbance. J Exp Clin Cancer Res. (2021) 40:18. doi: 10.1186/s13046-020-01782-w
36. Guilherme A, Virbasius JV, Puri V, Czech MP. Adipocyte dysfunctions linking obesity to insulin resistance and type 2 diabetes. Nat Rev Mol Cell Biol. (2008) 9:367–77. doi: 10.1038/nrm2391
37. Tsai IT, Wu C-C, Hung W-C, Lee T-L, Hsuan C-F, Wei C-T, et al. Fabp1 and Fabp2 as markers of diabetic nephropathy. Int J Med Sci. (2020) 17:2338–45. doi: 10.7150/ijms.49078
38. Shen FX, Gu X, Pan W, Li WP, Li W, Ye J, et al. Over-expression of Aqp7 contributes to improve insulin resistance in adipocytes. Exp Cell Res. (2012) 318:2377–84. doi: 10.1016/j.yexcr.2012.07.016
39. Cani PD, Amar J, Iglesias MA, Poggi M, Knauf C, Bastelica D, et al. Metabolic endotoxemia initiates obesity and insulin resistance. Diabetes. (2007) 56:1761–72. doi: 10.2337/db06-1491
40. Abenavoli L, Scarpellini E, Colica C, Boccuto L, Salehi B, Sharifi-Rad J, et al. Gut microbiota and obesity: a role for probiotics. Nutrients. (2019) 11:2690. doi: 10.3390/nu11112690
Keywords: dark tea, glycolipid metabolism, gene expression, gut microbiota, multiple omics
Citation: Wang C, Hu M, Yi Y, Wen X, Lv C, Shi M and Zeng C (2022) Multiomic analysis of dark tea extract on glycolipid metabolic disorders in db/db mice. Front. Nutr. 9:1006517. doi: 10.3389/fnut.2022.1006517
Received: 29 July 2022; Accepted: 17 August 2022;
Published: 08 September 2022.
Edited by:
Yong Su, Nanjing Agricultural University, ChinaReviewed by:
Yongsheng Chen, Jinan University, ChinaWuquan Deng, Chongqing Emergency Medical Center, China
Copyright © 2022 Wang, Hu, Yi, Wen, Lv, Shi and Zeng. This is an open-access article distributed under the terms of the Creative Commons Attribution License (CC BY). The use, distribution or reproduction in other forums is permitted, provided the original author(s) and the copyright owner(s) are credited and that the original publication in this journal is cited, in accordance with accepted academic practice. No use, distribution or reproduction is permitted which does not comply with these terms.
*Correspondence: Meng Shi, c2hpbWVuZyYjeDAwMDQwO2h1bmF1LmVkdS5jbg==; Chaoxi Zeng, Y2hhb3hpemVuZyYjeDAwMDQwO2h1bmF1LmVkdS5jbg==; Chenghao Lv, MTc1Njc5MDE4NyYjeDAwMDQwO3FxLmNvbQ==
†These authors have contributed equally to this work and share first authorship