- 1Hunan Collaborative Innovation Center for Utilization of Botanical Functional Ingredients, College of Animal Science and Technology, Hunan Agricultural University, Changsha, China
- 2Animal Science and Technology College, Beijing University of Agriculture, Beijing, China
- 3Hunan Xinguang'an Agricultural Husbandry Co., Ltd., Changsha, China
Placental function is vital to the fetal growth of sows, and resveratrol (RES) can protect cells against oxidative stress, which is one of the major factors impairing placental function. This study aimed to investigate the effect of dietary resveratrol (RES) on placental function and reproductive performance during late pregnancy in a sow model from the aspects of oxidative stress, insulin resistance, and gut microbiota. A total of 26 hybrid pregnant sows (Landrace × Yorkshire) with similar parity were randomly allocated into two groups (n = 13) and fed with a basal diet or a diet containing 200 mg/kg of resveratrol from day 85 of gestation until parturition. The dietary supplementation of RES increased the litter weight at parturition by 12.53% (p = 0.145), with ameliorated insulin resistance (HOMA-IR), increased triglyceride (TG) levels, and decreased interleukin (IL)-1β and IL-6 levels in serum (p < 0.05). Moreover, resveratrol increased the placental vascular density (p < 0.05) with the enhanced expression of nutrient transporter genes (SLC2A1 and SLC2A3) and antioxidant genes, such as superoxide dismutase 2 (SOD2) and heme oxygenase-1 (HO-1) but declined the expression of inflammatory genes, such as IL-1β and IL-6 (p < 0.05). The characterization of the fecal microbiota revealed that resveratrol decreased the relative abundance of the Christensensllaceae R-7 group and Ruminococcaceae UCG-008 (p < 0.05), which had a positive linear correlation with the expression of IL-1β and IL-6 (p < 0.05), but had a negative linear correlation with the expression of SOD2, HO-1, SLC2A1, and SCL2A3 genes (p < 0.05). These data demonstrated that dietary supplementation with resveratrol can improve placental function with ameliorated insulin resistance, oxidative stress, and inflammation potentially by regulating Ruminococcaceae UCG-008 and the Christensensllaceae R-7 group in sows.
Introduction
Placental function is vital to the nutrition supply of a fetus and thus has a strong impact on the reproductive performance of humans and animals. Over the past 20 decades, genetic improvement has increased the productivity of the sow herd, and nowadays high-yielding sows achieve 35 pigs per sow per year (1). However, an increase in the litter size results in a significant decrease in piglet birth weight (2) and gives piglets a harder time accessing the mammary glands and consuming less colostrum, and leads to higher pre-weaning mortality rates (3). Piglets born at a low birth weight have fewer glycogen reserves right after birth, making them more vulnerable to hypothermia and hypoglycemia within the first 24 h of life (4, 5).
Late pregnancy is a crucial period for fetal growth, and the maternal body undergoes substantial metabolic changes (6, 7), which make sows adapt from anabolic to catabolic metabolism (8). Increased metabolic intensity may lead to low-level inflammation (9), progressive oxidative stress (10), and insulin resistance (11). Meanwhile, the gut microbiota undergoes dramatic remodeling during late pregnancy that can affect a sow's physiological state and metabolic process (12). Our previous studies have demonstrated that nutritional additives can improve antioxidant capacity and reduce inflammation by modulating the intestinal flora in late pregnancy (13, 14). Moreover, abnormal glucose metabolism can decrease glucose utilization and placental nutrient transport to cause a low-weight maternal fetus (15), and increased systemic oxidative stress in late gestation can result in vascular dysfunction in the placenta (16, 17). These studies suggest that alleviating inflammation, oxidative stress, and insulin resistance in late gestation might be a promising strategy for improving placental function and increasing the reproductive performance of sows.
The dietary supplementation of phytochemicals during pregnancy can positively affect reproductive performance in sows (18). Polyphenols are plant-derived natural bioactive compounds with a strong antioxidant ability (19), and our previous studies have shown that polyphenols possess biological functions, such as anti-inflammatory, antioxidant, and alleviation of insulin resistance (20, 21). Resveratrol (RES) is a polyphenol that belongs to the stilbene family of phytoalexins and has shown an effect against oxidative stress and apoptosis in embryos of diabetic dams (22). In addition, it has been reported that RES may increase uterine artery blood flow velocity and fetal weight in murine models (23), and the biological activity of RES appears to be closely linked with modulating gut microbiota (24). Therefore, this study aimed to investigate the effect of dietary RES on the placental function and reproductive performance of sows during late pregnancy from the aspects of oxidative stress, insulin resistance, and gut microbiota.
Materials and methods
The animal experiment protocol used for the present study was approved by the Hunan Agricultural University Institutional Animal Care and Use Committee.
Materials and reagents
Resveratrol (≥98%) was provided by Hunan Engineering and Technology Center for Natural Products. Ingredients for the basal diet were provided by Hunan Xinguang'an Agricultural Husbandry Co., Ltd. (Changsha, Hunan, China).
Experimental design and diets
This study was carried out on the farm of Hunan Xinguang'an Agricultural Husbandry Co., Ltd. A total of 26 pregnant sows (Landrace × Yorkshire) with similar body condition scores and parity from 2 to 5 were used in this study. Sows were randomly divided into two treatments (n = 13), housed individually in gestation stalls, and had free access to water under environmental temperature. The experiment started on the 90th day of gestation and continued until delivery. Based on our pilot experiment, sows in the control group (CTL) were fed a basal diet, while sows in the RES group were fed a basal diet containing 200 mg/kg of RES based on our pilot experiment (RES intake 640 mg/day/sow). Approximately 3.2 kg of feed/sow/day was fed at 6:00 a.m., 12:00 p.m., and 6:00 p.m. The experiment started on day 85 of gestation and lasted until delivery. The composition of the basal diet, which meets the nutritional requirements of pigs according to NRC (2012), as is shown in Supplementary Table S1.
Measurements of reproductive performance and sample collection
After delivery, the number of live births, litter weight at parturition, and average weight of piglets born alive were measured immediately. On the day of parturition, blood samples (5 ml) of sows were collected from the marginal auricular vein into anticoagulant-free vacuum tubes and centrifuged on 1,500 × g for 10 min after standing at room temperature for 30 min to obtain serum. The placentae were collected after being weighed and snap-frozen in liquid nitrogen (3–4 cm from the cord insertion point). Fecal samples (~2 g from each sow) were collected into sterile tubes after defecation in the morning, and snap-frozen in liquid nitrogen. Finally, these samples were stored at −80°C until further analysis. Another about 1 cm × 1 cm fresh placentas tissues were immediately fixed in 4% paraformaldehyde (G1119, Servicebio Technology Co., Ltd. Wuhan, Hubei, China) for at least 24 h for H&E staining.
Histomorphological analysis
Each sow's placentae were sectioned for histological analysis as in a previous study (14). Briefly, placenta tissues were fixed in 4% paraformaldehyde overnight and submitted to Wuhan Servicebio Technology Co., Ltd (Wuhan, Hubei, China) for paraffin embedding and sectioning, then put the slices into xylene for 20 min two times, 75% alcohol for 5 min, and washed with tap water. Then, the slices were stained with hematoxylin dye solution for 3–5 min, washed with tap water, differentiated with differentiation solution, washed with tap water, H&E stain returned to blue, and rinsed with tap water. The slices were dehydrated with 85 and 95% gradient alcohol for 5 min and stained with eosin for 5 min. Then, put in absolute ethanol I for 5 min, absolute ethanol II for 5 min, absolute ethanol III for 5 min, dimethyl I for 5 min, and xylene II for 5 min, transparent and sealed with neutral gum. For each slice, the total number of vessels in the placental stroma areas was determined, and then corrected with the total placental stroma areas measured (per unit area as mm2).
Measurement of serum biochemical indices
The activity of total superoxide dismutase (T-SOD) and serum levels of thiobarbituric acid reactive substances (TBARS), glucose (GLU), total cholesterol (TC), triglycerides (TGs), high-density lipoproteins (HDL-c), and low-density lipoprotein cholesterol (LDL-c) were determined by using respective assay kits (Nanjing Jiancheng Bioengineering Institute, Nanjing, China) according to the manufacturer's instructions as described previously.
Serum levels of hormones, such as progesterone (PRO), follicle-stimulating hormone (FSH), and prolactin (PRL), insulin, and serum cytokines, such as interleukin (IL)-1β, IL-6, and Monocyte Chemotactic Protein 1 (MCP1) were measured with respective ELISA kits (Aifang biological Co., Ltd, Changsha, Hunan, China) according to the manufacturer's manual.
Insulin resistance and sensitivity were evaluated by the homeostasis model assessment (HOMA) values using the following indirect methods.
Real-time PCR
The quantitative PCR assays were validated according to the MIQE guidelines (25). Total RNA from the placenta or PVECs was extracted with the Total RNA Kit (Steadypure universal RNA extraction kit, Accurate Biotechnology Co., Ltd., Changsha, Hunan, China) according to the manufacturer's instructions. The concentration of RNA was quantified using a NanoDrop® lite (Thermo Fisher, USA). Reverse transcription of 1 μg total RNA was performed by a reverse transcription kit (Evo M-MLV RT Premix, Accurate Biotechnology Co., Ltd., Changsha, Hunan, China). The PCR reactions were performed in a 20 μl total reaction volume, which included 10 μl of 2 × SybrGreen qPCR Master Mix (SYBR® Green Premix Pro Taq HS qPCR Kit), 0.4 μl of each of the forward and reverse primers (10 μmol/L), 2 μl of cDNA template, and 7.2 μl of sterilized water. The PCR was carried out on a LightCycler480 Real-Time PCR system (Rotkreuz, Switzerland). The thermal cycler parameters were as follows: 3 min at 95°C, 40 cycles for 5 s at 95°C, and 30 s at 60°C. The stability of the β-actin and GADPH genes was evaluated by measuring the fluctuation range of the Ct values. The 2−ΔΔCT method was used for data analysis. Primers used in this study are shown in Supplementary Table S2.
Characterization of the gut microbiota
The fecal microbiota was characterized by 16S rDNA gene sequencing as described previously (14). Briefly, total DNA was extracted from fecal samples (six random samples from each group) by using a DNA Isolation Kit (MoBio Laboratories, Carlsbad, CA, USA) following the manufacturer's manual. The V3-4 hypervariable region of the bacterial 16S rRNA gene was amplified with the primers 338F (5′-ACTCCTACGGGAGGCAGCA-3′) and 806R (5′-GGACTACHVGGGTWTCTAAT-3′). The PCR was carried out on a Mastercycler Gradient (Eppendorf, Germany) using 25 μl reaction volumes, containing 12.5 μl KAPA 2G Robust Hot Start Ready Mix, 1 μl Forward Primer (5 μmol/L), 1 μl Reverse Primer (5 μmol/L), 5 μl DNA (total template quantity is 30 ng), and 5.5 μl H2O. Cycling parameters were 95°C for 5 min, followed by 28 cycles of 95°C for 45 s, 55°C for 50 s, and 72°C for 45 s with a final extension at 72°C for 10 min. Three PCR products per sample were pooled to mitigate reaction-level PCR biases. The PCR products were purified using a QIAquick Gel Extraction Kit (QIAGEN, Germany), quantified using Real-Time PCR, and sequenced on the Miseq platform at Allwegene Technology Inc., Beijing, China. Qualified reads were separated using the sample-specific barcode sequences and trimmed with Illumina Analysis Pipeline Version 2.6. The dataset was analyzed using QIIME (Version 1.8.0). The sequences were clustered into operational taxonomic units (OTUs) at a similarity level of 97%, to generate rarefaction curves and to calculate the richness and diversity index. The Ribosomal Database Project (RDP) Classifier tool was used to classify all sequences into different taxonomic groups.
Statistical analysis
The significant differences between groups were analyzed by a t-test with the SPSS 23.0 program (SPSS 23, IBM Corp., Armonk, NY, USA). The Pearson correlation and linear regression methods were used for the correlation analysis between the abundance of the top 50 microbial genera and placental gene expression. The value of p < 0.05 was considered significant.
Results
The impact of RES on the reproductive performance of sows
As shown in Table 1, there was no significant difference in total born number, the number of live birth, weak piglets' number, litter weight at parturition, and the average weight of piglets born alive. However, dietary supplementation of RES increased the litter weight at parturition by 12.53% (p = 0.145).
The effect of RES on insulin resistance and lipid metabolism in perinatal sows
As shown in Figure 1, RES significantly decreased the serum glucose (Figure 1A) in perinatal sows (p < 0.01) but showed no influence on the insulin level (Figure 1B). HOMA-IR and HOMA-IS were used to reflect insulin resistance and insulin sensitivity, respectively. Supplementation of the diet with RES significantly decreased HOMA-IR and increased HOMA-IS (Figure 1C) (p < 0.01). Moreover, the TG (Figure 1D) in the RES group was significantly increased compared with the CTL group (p < 0.05), while there was no significant change in TC, HDL-C, and LDL-C.
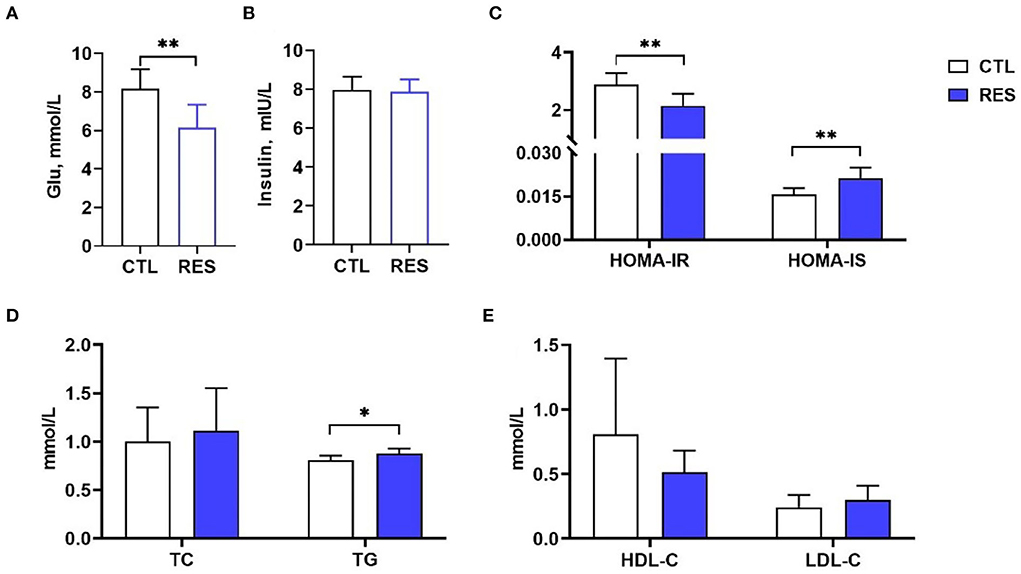
Figure 1. The effect of RES on glucose metabolism and lipid metabolism in perinatal sows. (A) Glu, (B) insulin, (C) HOMA-IR and HOMA-IS, (D) TC and TG, and (E) HDL-C and LDL-C. Glu, glucose; HOMA-IR, homeostasis model assessment-insulin resistance; HOMA-IS, homeostasis model assessment-insulin sensitivity; TC, total cholesterol; TG, triglycerides; HDL-C, high-density lipoprotein cholesterol; LDL-C, low-density lipoprotein cholesterol; CTL, a basal diet; and RES, a basal diet containing 200 mg/kg resveratrol. Data are expressed as mean ± SD (n = 10); *, p < 0.05; and **, p < 0.01.
The effect of RES on serum cytokines and hormones in perinatal sows
Serum cytokine and hormone levels in perinatal sows were measured to assess the inflammatory status in perinatal sows. Figure 2 shows that RES decreased serum levels of IL-1β (Figure 2A) and IL-6 (Figure 2B) (p < 0.05). However, there was no significant difference in the levels of MCP-1. Additionally, RES shows no significant effects on serum levels of FSH, prolactin, and progesterone (Figure 3).
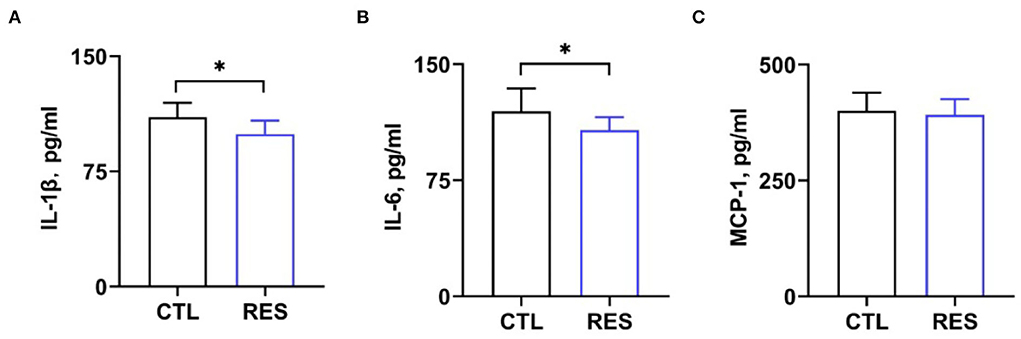
Figure 2. Effects of RES on blood inflammatory markers. Serum levels of (A) IL-1β, (B) IL-6, and (C) MCP-1 were measured by using ELISA kits. CTL, a basal diet; and RES, a basal diet containing 200 mg/kg resveratrol. Data are expressed as mean ± SD (n = 10); *, p < 0.05.
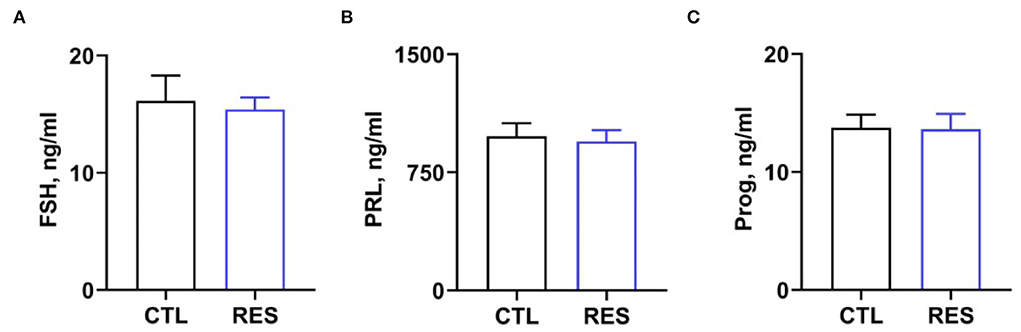
Figure 3. The effect of resveratrol on serum hormone levels in perinatal sows. (A) Follicle-stimulating hormone (FSH), (B) prolactin, and (C) progesterone. CTL, a basal diet; and RES, a basal diet containing 200 mg/kg resveratrol. Data are expressed as mean ± SD (n = 10); *, p < 0.05.
Effects of RES on vessel density and the expression of genes regulating nutrient transport, antioxidant defense, and inflammation in placenta
As shown in Figure 4, the placenta in the RES group showed significantly higher (p < 0.01) blood vessel density (Figures 4A,B) and the CD31 messenger RNA (mRNA) expression (a biomarker of the endothelial cell in small vessels) (Figure 4C). Meanwhile, RES significantly increased the expression of nutrient transporter genes of SLC2A1 and SCL2A3 (p < 0.01) but showed no effect on SLC7A1. Moreover, the expression of IL-1β and IL-6 were downregulated by RES, and the expression of SOD2 and HO-1 were upregulated by RES in the placenta (p < 0.05).
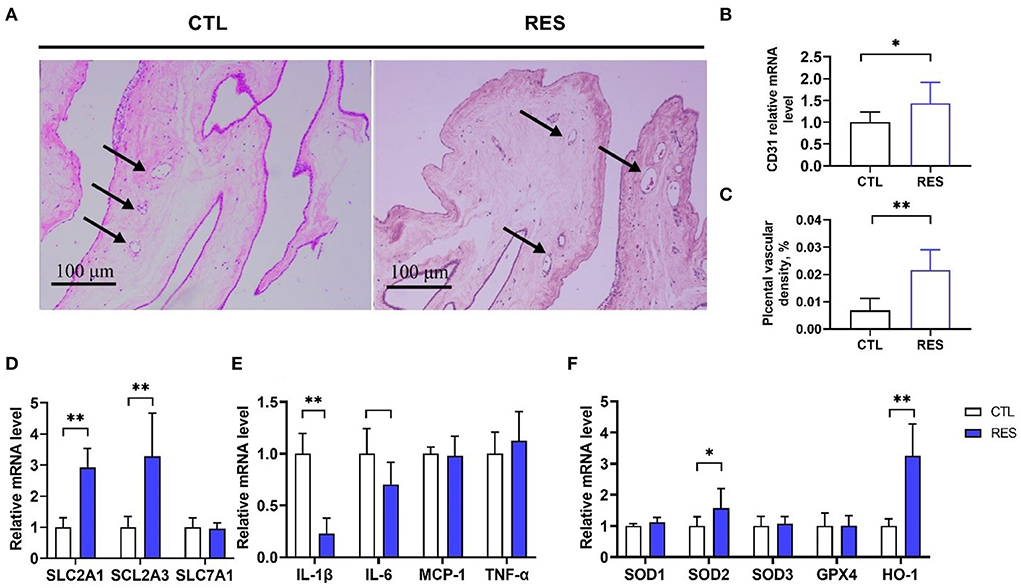
Figure 4. The effect of RES on vessel density, nutrient transport, antioxidant, and inflammation mRNA expression in the placental. (A,B) The hematoxylin and eosin method was used to examine blood vessel density in placental tissues, and the black arrows indicate placental blood vessels (bar = 100 μm, n = 12); (C) CD31 mRNA expression; (D) Nutrient transporters SLC2A1, SCL2A3, and SLC7A1 genes expression; (E) Inflammatory factors IL-1β, IL-6, MCP1, and TNF-α genes expression; (F) Antioxidant genes SOD1, SOD2, SOD3, GPX4, and HO-1 expression. CTL, a basal diet; and RES, a basal diet containing 200 mg/kg resveratrol. Data are expressed as mean ± SD (n = 8); *, p < 0.05; and **, p < 0.01.
The effect of RES on the gut microbiota
As shown in Figure 5, principal component analysis (PCA) indicated that the CTL and RES groups had a similarity cluster (Figure 5A), and RES showed limited effects on the Chao 1 index (Figure 5B) and the Shannon index (Figure 5C). Firmicutes, Bacteroidetes, Proteobacteria, and Euryarchaeota accounted for 90% of the total microbes at the phylum level (Figure 5D), and RES significantly downregulated the relative abundances of the Christensenellaceae R-7 group (Figure 5E), and Ruminococcaceae UCG-005 (Figure 5F) at the genus level (p < 0.05).
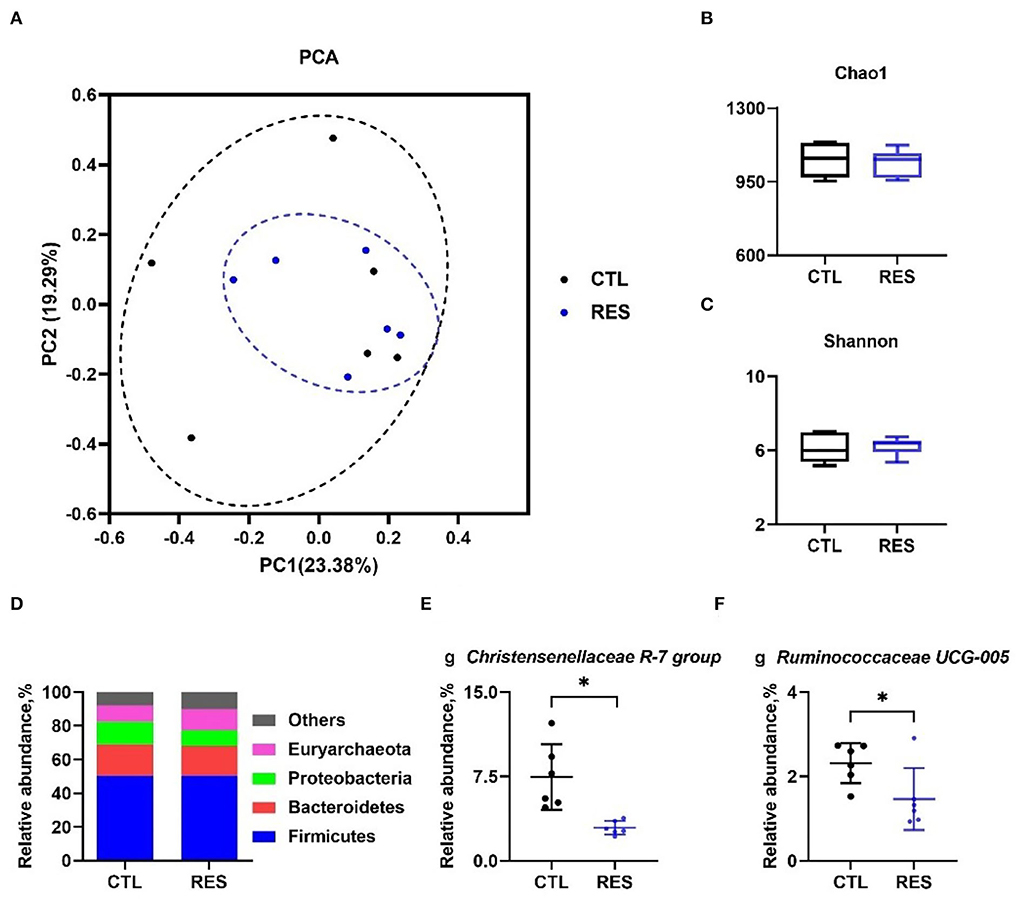
Figure 5. Modulation of the gut microbiota by RES. The effect of RES on genus-based (n = 6) principal component analysis (PCA) (A), the Chao 1 index (B), the Shannon index (C), the effect of RES on the relative abundance of main microbes at the phylum level (D) and the genera Christensenellaceae R-7 group (E), and Ruminococcaceae UCG-005 (F). CTL, a basal diet; and RES, a basal diet containing 200 mg/kg resveratrol. Data are expressed as mean ± SD (n = 6); and *, p < 0.05.
Correlation analysis of the gut microbiota and placental gene expression
To further understand the role of whole gut microbiota in regulating oxidative and inflammatory status, the abundance of the top 50 genera was chosen to process correlation analysis with placental gene expression to better understand the role of the whole gut microbiota in regulating oxidative and inflammatory status. As shown in Figure 6A, a total number of 14 microbial genera were significantly correlated (p < 0.05) with the placental nutrient transport, antioxidant defense, and inflammation-related mRNA expression. Based on the results in Figure 5, the Christensenellaceae R-7 group and Ruminococcaceae UCG-005 were selected to perform linear regression analyses. As shown in Figure 6B, the Christensenellaceae R-7 group and Ruminococcaceae UCG-005 had a positive linear correlation with the expression of IL-1β and IL-6 (p < 0.05). Moreover, the Christensenellaceae R-7 group had a negative linear correlation with SOD2 and HO-1 (p < 0.05) (Figure 6C). In addition, the Christensenellaceae R-7 group and Ruminococcaceae UCG-005 had a negative linear correlation with SLC2A1 and SCL2A3 (p < 0.05) (Figure 6D).
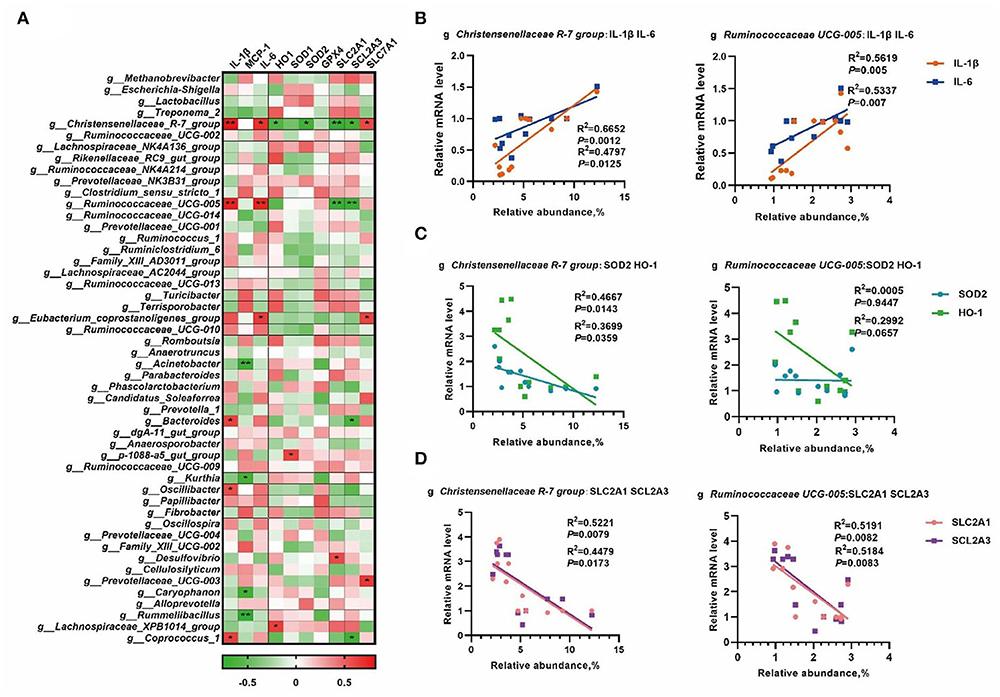
Figure 6. The correlation analysis of the gut microbiota and placental gene expression. The correlation analysis between the abundance of the top 50 microbial genera and placental gene expression by Spearman's correlation analysis (A), linear regression analyses between genera and placental mRNA expression of inflammatory cytokines (B), mRNA expression of antioxidative genes (C), and expression of genes involved in the regulation of nutrient transport in placenta (D). *, p < 0.05; and **, p < 0.01.
Discussion
The latter part of pregnancy is critical for fetal development and the critical period of litter weight at parturition. About 60% of fetal body weight gain occurs in late gestation (6). During pregnancy, sows have a complex and dynamic metabolism, which includes nutrient intake, digestion, and absorption, and as well as complex transport processes, such as nutrients transported from maternal tissues to fetal and breast tissues (8). As a result, the metabolic intensity in the sow is significantly increased during late pregnancy due to the rapid growth of the fetus. Studies have shown that sows suffered increased oxidative stress during delivery of late gestation, as indicated by their increased reactive oxygen (ROS), 8-hydroxy-deoxyguanosine, and thiobarbituric acid reactive substance levels (16). A series of metabolic changes characterize the metabolic syndrome in late gestation, especially insulin resistance. Yang et al. (15) have reported that starch inclusion in the maternal diet can improve the sows' insulin resistance during late gestation, increase piglet weight at birth and weaning, and decrease the stillbirth rate.
In the present study, supplementation of 200 mg/kg RES in late gestation can be increased, (although not significantly, p > 0.05). An increase in insulin resistance during gestation has been considered as a fundamental cause of the reduced reproductive performance of sows (26). Previous studies have demonstrated that RES may attenuate insulin resistance to improve glucose homeostasis and metabolic disorders (27, 28). Our results showed that RES improved insulin resistance, which suggests that RES may improve metabolic disorders and maternal metabolism during late gestation. The development of insulin resistance during pregnancy is considered to be induced by some placental hormones (29). For insulin-secreting beta-cells that reside in the pancreas, maternal prolactin can directly promote their proliferation, enhance their sensitivity, and increase insulin secretion from this group of cells after glucose stimulation (29). Progesterone could induce insulin resistance by inhibiting GLUT-4 translocation, decreasing the expression of insulin receptor substrate-1 (IRS-1), and blocking the glucose absorption by adipocytes (30). Moreover, FSH has been reported to have a negative association with insulin resistance (31). However, RES showed no significant effect on these hormones in the present study, indicating that RES may improve insulin resistance through other mechanisms, rather than regulating hormones level.
The placenta is a highly vascularized tissue and is essential for the growth and development of the fetus. The development of the epitheliochorial placenta of pigs begins at the embryonic stage (29). It has been reported that the piglet birth weight has a significant positive correlation with placental vascular density (17), and RES may increase uterine artery blood flow and fetal weight (32). In this study, RES increased the placental vessel density with upregulated mRNA expression of CD31, which is a biomarker of endothelial cells in blood vessels (33). Meanwhile, RES increased the mRNA levels of SLC2A3 and SLC2A1, which code glucose transporter GLUT-3 and GLUT-1, respectively. However, RES showed no significant effect on SLC7A1, which encodes a cationic amino acid transporter (34). Thus, RES might benefit fetal growth and development by improving placental glucose transport, which is the primary energy source for fetal development (35). Oxidative stress and inflammation have been considered important factors that affect the growth and function of the placenta. The activation of the NF-κB pathway in the placental has been reported to have a close relationship with the downregulation of angiogenesis-related genes, such as HIF1α, VEGFA, and ANGPTL6 (36). Oxidative stress can also induce vascular endothelial injury and abnormal development of the placenta vascular (17). RES is a known antioxidant and anti-inflammatory agent and has been proved to promote the nuclear accumulation, DNA binding, and transcriptional activity of Nrf2 (37) in sows' placenta. Moreover, RES can suppress Cd-induced apoptosis in the placenta and JEG-3 cells and decrease Cd-induced expression of TNF-α, IFN-γ, MCP-1, MIP-2, and KC in the placenta (32). In this study, RES alleviated placenta oxidative stress and inflammation via improving SOD2 and HO-1 expression, but inhibiting IL-1β and IL-6 production.
Increasing pieces of evidence have suggested that the gut microbiota plays a crucial role in pregnancy, and maternal gut microbiota undergoes dramatic changes throughout the gestation period. The abundance and diversity of the gut microbiota increase as the pregnancy continues and they are closely related with the metabolism and reproduction of the host (38). Our previous studies have shown that the gut microbiota is associated with low-grade inflammation and oxidative stress in normal pregnancy, and β-carotene can inhibit the production of inflammatory cytokines and improve antioxidant capacity by modulating the gut microbiota (13, 14). RES is a kind of polyphenol with low in vivo bioavailability (39), and it can interact with the gut microbiota in the hindgut (40). When passing the small intestinal lumen, RES can be absorbed into the enterocyte and undergoes sulfation and glucuronidation. The RES and conjugated metabolites that exit from the apical membrane of the small intestine will move to the large intestine and further be metabolized by the gut microbiota to generate dihydroresveratrol, lunularin, and 3,4'-dihydroxy-trans-stilbene (41, 42). Previous studies have revealed that the biological functions of RES may be attributed to the regulation of gut microbiota (43, 44). In the present study, dietary supplementation of RES mainly downregulated the relative abundance of Ruminococcaceae UCG-008 and the Christensensllaceae R-7 group at the genus level. Ruminococcaceae UCG-008 belongs to the family of Ruminococcaceae and has a close correlation with butyrate production (45), and negatively correlated with inflammatory cytokines in our previous study (46). The Christensensllaceae R-7 group belongs to the family of Christensensllaceae, which has a negative correlation with lipid biosynthesis and energy metabolism pathways in humans (47). In the present study, the Christensensllaceae R-7 group and Ruminococcaceae UCG-008 had a positive linear correlation with IL-1β and IL-6 but had a negative linear correlation with SLC2A1 and SCL2A3. However, only the Christensensllaceae R-7 group had a negative linear correlation with SOD2 and HO-1. These results suggest that the Christensensllaceae R-7 group and Ruminococcaceae UCG-008 may play an important role in the beneficial effects of RES on placental function.
Conclusion
Dietary supplementation of resveratrol during late pregnancy showed a positive effect on the placental function of sows, with ameliorated insulin resistance (HOMA-IR), increased triglyceride (TG) levels, and reduced levels of inflammatory cytokines, such as IL-1β and IL-6. Resveratrol could enhance the placental vascular density by upregulating the expression of nutrient transporters genes (SLC2A1 and SLC2A3), attenuate placental oxidative stress and inflammation by promoting the expression of antioxidant genes (SOD2 and HO-1), and suppressing inflammatory genes (IL-1β and IL-6). An analysis of the gut microbiota revealed that resveratrol can reduce the relative abundance of the Christensensllaceae R-7 group and Ruminococcaceae UCG-008, which have a positive linear correlation with IL-1β, IL-6, SLC2A1, and SCL2A3.
Data availability statement
The original contributions presented in the study are publicly available. This data can be found here https://www.ncbi.nlm.nih.gov/bioproject/PRJNA885401.
Ethics statement
The animal study was reviewed and approved by Hunan Agricultural University Institutional Animal Care and Use Committee.
Author contributions
RH is the primary investigator in this study. JT and ZL participated in the animal experiments. LW and MS performed statistical data analysis. BL, ML, and XY participated in the sample analysis. JH and XW designed this study and wrote the manuscript as the corresponding author. All authors contributed to the article and approved the submitted version.
Funding
The authors gratefully acknowledge the support from the National Natural Science Foundation of China (U22A20515; 31772819) and the earmarked fund for CARS (CARS-35).
Conflict of interest
Author XY was employed by Hunan Xinguang'an Agricultural Husbandry Co., Ltd.
The remaining authors declare that the research was conducted in the absence of any commercial or financial relationships that could be construed as a potential conflict of interest.
Publisher's note
All claims expressed in this article are solely those of the authors and do not necessarily represent those of their affiliated organizations, or those of the publisher, the editors and the reviewers. Any product that may be evaluated in this article, or claim that may be made by its manufacturer, is not guaranteed or endorsed by the publisher.
Supplementary material
The Supplementary Material for this article can be found online at: https://www.frontiersin.org/articles/10.3389/fnut.2022.1001031/full#supplementary-material
References
1. Kraeling RR, Webel SK. Current strategies for reproductive management of gilts and sows in North America. J Anim Sci Biotechnol. (2015) 6:3. doi: 10.1186/2049-1891-6-3
2. McPherson RL, Ji F, Wu G, Blanton JR Jr, Kim SW. Growth and compositional changes of fetal tissues in pigs. J Anim Sci. (2004) 82:2534–40. doi: 10.2527/2004.8292534x
3. Declerck I, Sarrazin S, Dewulf J, Maes D. Sow and piglet factors determining variation of colostrum intake between and within litters. Animal. (2017) 11:1336–43. doi: 10.1017/S1751731117000131
4. Vanden Hole C, Ayuso M, Aerts P, Prims S, Van Cruchten S, Van Ginneken C. Glucose and glycogen levels in piglets that differ in birth weight and vitality. Heliyon. (2019) 5:e02510. doi: 10.1016/j.heliyon.2019.e02510
5. Muns R, Manzanilla EG, Sol C, Manteca X, Gasa J. Piglet behavior as a measure of vitality and its influence on piglet survival and growth during lactation. J Anim Sci. (2013) 91:1838–43. doi: 10.2527/jas.2012-5501
6. Hedemann MS, Flummer C, Kristensen NB, Theil PK. Metabolic profiling of plasma from sows before parturition and during lactation using a liquid chromatography-mass spectrometry-based approach. J Anim Sci. (2012) 90:200–2. doi: 10.2527/jas.53911
7. Newbern D, Freemark M. Placental hormones and the control of maternal metabolism and fetal growth. Curr Opin Endocrinol Diabetes Obes. (2011) 18:409–16. doi: 10.1097/MED.0b013e32834c800d
8. Hansen AV, Lauridsen C, Sorensen MT, Knudsen KEB, Theil PK. Effects of nutrient supply, plasma metabolites, and nutritional status of sows during transition on performance in the next lactation. J Anim Sci. (2012) 90:466–80. doi: 10.2527/jas.2011-3984
9. Palm M, Axelsson O, Wernroth L, Larsson A, Basu S. Involvement of inflammation in normal pregnancy. Acta Obstet Gynecol Scand. (2013) 92:601–5. doi: 10.1111/aogs.12093
10. Pereira RD, De Long NE, Wang RC, Yazdi FT, Holloway AC, Raha S. Angiogenesis in the placenta: the role of reactive oxygen species signaling. Biomed Res Int. (2015) 2015:814543. doi: 10.1155/2015/814543
11. Tan CQ, Wei HK, Ao JT, Long G, Peng J. Inclusion of konjac flour in the gestation diet changes the gut microbiota, alleviates oxidative stress, and improves insulin sensitivity in sows. Appl Environ Microbiol. (2016) 82:5899–909. doi: 10.1128/AEM.01374-16
12. Huang XC, Gao J, Zhao YZ, He MZ, Ke SL, Wu JY, et al. Dramatic remodeling of the gut microbiome around parturition and its relationship with host serum metabolic changes in sows. Front Microbiol. (2019) 10:2123. doi: 10.3389/fmicb.2019.02123
13. Yuan X, Yan J, Hu R, Li Y, Wang Y, Chen H, et al. Modulation of gut microbiota and oxidative status by β-carotene in late pregnant sows. Front Nutr. (2020) 7:612875. doi: 10.3389/fnut.2020.612875
14. Yang X, He Z, Hu R, Yan J, Zhang Q, Li B, et al. Dietary β-carotene on post-partum uterine recovery in mice: crosstalk between gut microbiota and inflammation. Front Immunol. (2021) 12:744425. doi: 10.3389/fimmu.2021.744425
15. Yang Y, Deng M, Chen J, Zhao X, Xiao K, He W, et al. Starch supplementation improves the reproductive performance of sows in different glucose tolerance status. Anim Nutr. (2021) 7:1231–41. doi: 10.1016/j.aninu.2021.03.010
16. Tan CQ, Wei HK, Sun HQ, Ao JT, Long G, Jiang SW, et al. Effects of dietary supplementation of oregano essential oil to sows on oxidative stress status, lactation feed intake of sows, and piglet performance. Biomed Res Int. (2015) 2015:525218. doi: 10.1155/2015/525218
17. Huang SB, Wu ZF, Huang ZH, Hao XY, Zhang LM, Hu CJ, et al. Maternal supply of cysteamine alleviates oxidative stress and enhances angiogenesis in porcine placenta. J Anim Sci Biotechnol. (2021) 12:91. doi: 10.1186/s40104-021-00609-8
18. Reyes-Camacho D, Vinyeta E, Perez JF, Aumiller T, Criado L, Palade LM, et al. Phytogenic actives supplemented in hyperprolific sows: effects on maternal transfer of phytogenic compounds, colostrum and milk features, performance and antioxidant status of sows and their offspring, and piglet intestinal gene expression. J Anim Sci. (2020) 98:1–13. doi: 10.1093/jas/skz390
19. Hu RZ, He YJ, Arowolo MA, Wu SS, He J. Polyphenols as potential attenuators of heat stress in poultry production. Antioxidants. (2019) 8:1–11. doi: 10.3390/antiox8030067
20. Wu S, Yano S, Hisanaga A, He X, He J, Sakao K, et al. Polyphenols from Lonicera caerulea L. berry attenuate experimental non-alcoholic steatohepatitis by inhibiting proinflammatory cytokines productions and lipid peroxidation. Mol Nutr Food Res. (2017) 61:8. doi: 10.1002/mnfr.201600858
21. Wu S, He X, Wu X, Qin S, He J, Zhang S, et al. Inhibitory effects of blue honeysuckle (Lonicera caerulea L) on adjuvant-induced arthritis in rats: crosstalk of anti-inflammatory and antioxidant effects. J Funct Foods. (2015) 17:514–23. doi: 10.1016/j.jff.2015.06.007
22. Singh CK, Kumar A, Hitchcock DB, Fan DP, Goodwin R, LaVoie HA, et al. Resveratrol prevents embryonic oxidative stress and apoptosis associated with diabetic embryopathy and improves glucose and lipid profile of diabetic dam. Mol Nutr Food Res. (2011) 55:1186–96. doi: 10.1002/mnfr.201000457
23. Poudel R, Stanley JL, Rueda-Clausen CF, Andersson IJ, Sibley CP, Davidge ST, et al. Effects of resveratrol in pregnancy using murine models with reduced blood supply to the uterus. PLoS ONE. (2013) 8:e64401. doi: 10.1371/journal.pone.0064401
24. Briskey D, Rao A. Trans-resveratrol oral bioavailability in humans using lipiSperse (TM) dispersion technology. Pharmaceutics. (2020) 12:1190. doi: 10.3390/pharmaceutics12121190
25. Bustin SA, Benes V, Garson JA, Hellemans J, Huggett J, Kubista M, et al. The MIQE guidelines: minimum information for publication of quantitative real-time PCR experiments. Clin Chem. (2009) 55:611–22. doi: 10.1373/clinchem.2008.112797
26. Mosnier E, Le Floc'h N, Etienne M, Ramaekers P, Seve B, Pere MC. Reduced feed intake of lactating primiparous sows is associated with increased insulin resistance during the peripartum period and is not modified through supplementation with dietary tryptophan. J Anim Sci. (2010) 88:612–25. doi: 10.2527/jas.2008-1768
27. Sun C, Zhang F, Ge XJ, Yan TT, Chen XM, Shi XL, et al. SIRT1 improves insulin sensitivity under insulin-resistant conditions by repressing PTP1B. Cell Metab. (2007) 6:307–19. doi: 10.1016/j.cmet.2007.08.014
28. Liu K, Zhou R, Wang B, Mi MT. Effect of resveratrol on glucose control and insulin sensitivity: a meta-analysis:of 11 randomized controlled trials. Am J Clin Nutr. (2014) 99:1510–9. doi: 10.3945/ajcn.113.082024
29. Barbour LA, McCurdy CE, Hernandez TL, Kirwan JP, Catalano PM, Friedman JE. Cellular mechanisms for insulin resistance in normal pregnancy and gestational diabetes. Diabetes Care. (2007) 30:S112–9. doi: 10.2337/dc07-s202
30. Wada T, Hori S, Sugiyama M, Fujisawa E, Nakano T, Tsuneki H, et al. Progesterone inhibits glucose uptake by affecting diverse steps of insulin signaling in 3T3-L1 adipocytes. Am J Physiol Endocrinol Metab. (2010) 298:E881–8. doi: 10.1152/ajpendo.00649.2009
31. Yeung EH, Zhang CL, Mumford SL, Ye AJ, Trevisan M, Chen LW, et al. Longitudinal study of insulin resistance and sex hormones over the menstrual cycle: the biocycle study. J Clin Endocrinol Metab. (2010) 95:5435–42. doi: 10.1210/jc.2010-0702
32. Wang W, Liu G, Jiang X, Wu G. Resveratrol ameliorates toxic effects of cadmium on placental development in mouse placenta and human trophoblast cells. Birth Defects Res. (2021) 113:1470–83. doi: 10.1002/bdr2.1962
33. Hu CJ, Yang YY, Deng M, Yang LF, Shu G, Jiang QY, et al. Placentae for low birth weight piglets are vulnerable to oxidative stress, mitochondrial dysfunction, and impaired angiogenesis. Oxid Med Cell Longev. (2020) 2020:8715412. doi: 10.1155/2020/8715412
34. Zhao WC, Liu F, Bell AW, Le HH, Cottrell JJ, Leury BJ, et al. Controlled elevated temperatures during early-mid gestation cause placental insufficiency and implications for fetal growth in pregnant pigs. Sci Rep. (2020) 10:20677. doi: 10.1038/s41598-020-77647-1
35. Vallet JL, McNeel AK, Miles JR, Freking BA. Placental accommodations for transport and metabolism during intra-uterine crowding in pigs. J Anim Sci Biotechnol. (2014) 5:55. doi: 10.1186/2049-1891-5-55
36. Saben J, Lindsey F, Zhong Y, Thakali K, Badger TM, Andres A, et al. Maternal obesity is associated with a lipotoxic placental environment. Placenta. (2014) 35:171–7. doi: 10.1016/j.placenta.2014.01.003
37. Meng QW, Guo T, Li GQ, Sun SS, He SQ, Cheng BJ, et al. Dietary resveratrol improves antioxidant status of sows and piglets and regulates antioxidant gene expression in placenta by Keap1-Nrf2 pathway and Sirt1. J Anim Sci Biotechnol. (2018) 9:34. doi: 10.1186/s40104-018-0248-y
38. Koren O, Goodrich JK, Cullender TC, Spor A, Laitinen K, Backhed HK, et al. Host remodeling of the gut microbiome and metabolic changes during pregnancy. Cell. (2012) 150:470–80. doi: 10.1016/j.cell.2012.07.008
39. Cote CD, Rasmussen BA, Duca FA, Zadeh-Tahmasebi M, Baur JA, Daljeet M, et al. Resveratrol activates duodenal Sirt1 to reverse insulin resistance in rats through a neuronal network. Nat Med. (2015) 21:498–505. doi: 10.1038/nm.3821
40. Chaplin A, Carpene C, Mercader J. Resveratrol, metabolic syndrome, and gut microbiota. Nutrients. (2018) 10:1651. doi: 10.3390/nu10111651
41. Bode LM, Bunzel D, Huch M, Cho GS, Ruhland D, Bunzel M, et al. In vivo and in vitro metabolism of trans-resveratrol by human gut microbiota. Am J Clin Nutr. (2013) 97:295–309. doi: 10.3945/ajcn.112.049379
42. Timmers S, de Ligt M, Phielix E, van de Weijer T, Hansen J, Moonen-Kornips E, et al. Resveratrol as add-on therapy in subjects with well-controlled type 2 diabetes: a randomized controlled trial. Diabetes Care. (2016) 39:2211–7. doi: 10.2337/dc16-0499
43. Sung MM, Kim TT, Denou E, Soltys CLM, Hamza SM, Byrne NJ, et al. Improved glucose homeostasis in obese mice treated with resveratrol is associated with alterations in the gut microbiome. Diabetes. (2017) 66:418–25. doi: 10.2337/db16-0680
44. Li F, Han YH, Cai XK, Gu M, Sun J, Qi C, et al. Dietary resveratrol attenuated colitis and modulated gut microbiota in dextran sulfate sodium-treated mice. Food Funct. (2020) 11:1063–73. doi: 10.1039/C9FO01519A
45. Chen RZ, Wu P, Cai Z, Fang YY, Zhou H, Lasanajak Y, et al. Puerariae lobatae radix with chuanxiong rhizoma for treatment of cerebral ischemic stroke by remodeling gut microbiota to regulate the brain-gut barriers. J Nutr Biochem. (2019) 65:101–14. doi: 10.1016/j.jnutbio.2018.12.004
46. Hu RZ, He ZY, Liu M, Tan JJ, Zhang HF, Hou DX, et al. Dietary protocatechuic acid ameliorates inflammation and up-regulates intestinal tight junction proteins by modulating gut microbiota in LPS-challenged piglets. J Anim Sci Biotechnol. (2020) 11:12. doi: 10.1186/s40104-020-00492-9
Keywords: resveratrol, sow, reproductive performance, placental function, gut microbiota
Citation: Hu R, Tan J, Li Z, Wang L, Shi M, Li B, Liu M, Yuan X, He J and Wu X (2022) Effect of dietary resveratrol on placental function and reproductive performance of late pregnancy sows. Front. Nutr. 9:1001031. doi: 10.3389/fnut.2022.1001031
Received: 22 July 2022; Accepted: 20 September 2022;
Published: 27 October 2022.
Edited by:
Kai Wang, Chinese Academy of Agricultural Sciences, ChinaReviewed by:
Irena Krga, University of Belgrade, SerbiaGuangtian Cao, China Jiliang University, China
Shengyu Xu, Sichuan Agricultural University, China
Yong Zhao, Chinese Academy of Agricultural Sciences, China
Copyright © 2022 Hu, Tan, Li, Wang, Shi, Li, Liu, Yuan, He and Wu. This is an open-access article distributed under the terms of the Creative Commons Attribution License (CC BY). The use, distribution or reproduction in other forums is permitted, provided the original author(s) and the copyright owner(s) are credited and that the original publication in this journal is cited, in accordance with accepted academic practice. No use, distribution or reproduction is permitted which does not comply with these terms.
*Correspondence: Jianhua He, amlhbmh1YWh5JiN4MDAwNDA7aHVuYXUubmV0; Xiaosong Wu, d3V4aWFvc29uZzUyOSYjeDAwMDQwOzEyNi5jb20=