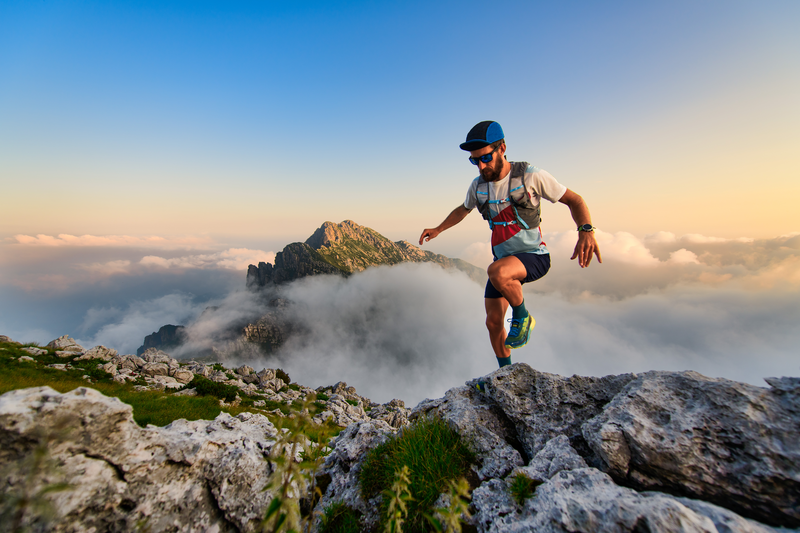
94% of researchers rate our articles as excellent or good
Learn more about the work of our research integrity team to safeguard the quality of each article we publish.
Find out more
SYSTEMATIC REVIEW article
Front. Nutr. , 01 February 2022
Sec. Nutrition and Metabolism
Volume 8 - 2021 | https://doi.org/10.3389/fnut.2021.810494
This article is part of the Research Topic Endocrine Regulation of Mineral Ions and their Relevance to Metabolic Diseases View all 14 articles
Background: Epidemiological studies have investigated the associations of dietary iron, copper, and selenium level with metabolic syndrome (MetS). However, their results are conflicting. This meta-analysis of observational study was, therefore, employed to investigate the associations above.
Methods: A comprehensive literature search was employed using PubMed, Web of Science, Embase, and Scopus database up to October 2021 (no restriction was set for the initiate time). The pooled relative risk (RR) of MetS for the highest vs. lowest dietary iron, copper, and selenium level was estimated, respectively.
Results: A total of 14 observational studies (55,131 participants) were identified as meeting the inclusion criteria. Specifically, 7 studies were related to the dietary iron level. The overall multivariable adjusted RR demonstrated that the dietary iron level was positively associated with MetS (RR = 1.27, 95% CI: 1.12–1.44; p < 0.001). With regard to the dietary copper level, 7 studies were included for meta-analysis. The overall multivariable adjusted RR showed that the dietary copper level was inversely associated with MetS (RR = 0.85, 95% CI: 0.78–0.93; p < 0.001). In addition, 4 studies were specified for the dietary selenium level. The overall multivariable adjusted RR indicated that the dietary selenium level was inversely associated with MetS (RR = 0.77, 95% CI: 0.63–0.95; p = 0.01) as well.
Conclusion: Our results suggest that the dietary iron level is positively associated with MetS, whereas a negative association between the dietary copper and selenium level and MetS is obtained. Further large well-designed prospective cohort studies are warranted to elaborate on the issues examined in this study.
Elevated waist circumference, blood pressure, fasting blood glucose, triglycerides, and decreased high-density lipoprotein cholesterol (at least three of the five above metabolic abnormalities) are considered as the presence of metabolic syndrome (MetS) (1). MetS is a well-known attributable risk to diabetes, stroke, and coronary heart disease epidemic (2–4). Moreover, MetS increases the incidence of atherosclerotic cardiovascular disorder and complication that decreases longevity (5). However, the subjects suffering from MetS are progressively growing worldwide and the current global prevalence of MetS is between 11.6 and 62.5% (6). Although the etiology of MetS is not well understood yet, dietary factors are considered to be involved in MetS (7–11).
Micronutrients are important factors for cellular and biochemical functions (release of energy for synthesis and movement) (12). Iron, copper, and selenium are considered to be significant micronutrients and their dietary sources are meat, seeds, heme, tea, milk, nuts, cereals, eggs, fish, and so on (13–18). Iron is one of the most abundant elements, which plays a significant role in various cellular processes, such as iron-dependent signaling, cellular respiration, DNA replication and synthesis, nucleic acid repair, and energy metabolism (19–21). Iron consumption, uptake, transfer, and storage are involved to maintain iron homeostasis (22). However, excess iron leads to inflammation and tissue damage, produces hydroxyl radicals (Haber–Weiss–Fenton reactions), which cause oxidative damage to cellular components (lipids, proteins, and DNA) (23, 24). On the contrary, copper, a component of extracellular superoxide dismutase (25), is essential for iron uptake and signaling in eukaryotic organisms, energy metabolism, reactive oxygen species, and detoxification (26). In addition, copper plays an essential role in mitochondrial function and signaling involving mitophagy, bioenergetics, and dynamics, which affect cell fate by metabolic reprogramming (26). Selenium, also an essential micronutrient, is necessary to maintain the different cellular functions, such as signaling transduction pathways and immune-endocrine function (18). Moreover, selenium incorporates into selenoproteins and selenium-dependent enzymes (e.g., glutathione peroxidases), which are involved in intracellular redox regulation and modulation (27). Since oxidative stress and inflammation play a significant role in the pathophysiology of MetS (28), the dietary iron, copper, and selenium level is considered to be closely related to MetS.
A number of observational studies have been employed to investigate the associations of the dietary iron, copper, and selenium level with MetS (29–42). However, their results are still conflicting. Thus, this meta-analysis of observational studies is employed to further investigate the above associations. It is hypothesized that the dietary iron level is positively associated with MetS, whereas the dietary copper and selenium level is inversely associated with MetS.
Our meta-analysis was performed according to the Preferred Reporting Items for Systematic Reviews and Meta-analyses (PRISMA) guidelines (43). The PubMed, Web of Science, Embase, and Scopus electronic databases were searched up to October 2021 (no restriction was set for the initiate time) by using a combination of keywords that related to MetS (“metabolic syndrome”), iron (“iron,” “Fe”), copper (“copper,” “Cu”), and selenium (“selenium,” “Se”). No language restriction was set in the search strategy. We screened the titles and abstracts of all the articles and then read the full articles to identify the eligible studies.
The titles, abstracts, and full texts of all the retrieved studies were reviewed by two researchers independently. Disagreements were resolved by discussions. The included studies were required to meet the following criteria: (1) the study design is an observational study; (2) the outcomes include the associations of the dietary iron, copper, and selenium level with MetS; and (3) the relative risk (RR) or odds ratio (OR) with 95% CI was reported. The exclusion criteria were listed as follows: (1) duplicated or irrelevant articles; (2) reviews, letters, or case reports; (3) randomized controlled trials; and (4) non-human studies.
The data were extracted by two researchers independently and disagreements were resolved by discussions. The information about the first author and year of publication, location, age, gender, sample size, study design, adjustments, exposure, category of exposure, effect estimates, and diagnostic criteria of MetS was collected. The corresponding effect estimates with 95% CIs for the highest vs. lowest dietary iron, copper, and selenium level and MetS was extracted (adjusted for the maximum number of confounding variables).
The Newcastle–Ottawa Scale (NOS) criteria for non-randomized studies were employed to assess the quality of each included study. The NOS is based on three broad perspectives: (1) the selection process of the study cohorts; (2) the comparability among the different cohorts; and (3) the identification of exposure or outcome of the study cohorts. Disagreements with respect to the methodological quality were resolved by mutual consultation.
The RR for MetS was the outcome measure in this meta-analysis. The I2 statistic, which measures the percentage of total variation across studies due to heterogeneity, was examined (I2 > 50% was considered as heterogeneity). If significant heterogeneity was observed among the studies, a random-effects model was used; otherwise, a fixed-effects model was accepted. Begg's test was employed to assess the publication bias (44). A p-value of < 0.05 was considered statistically significant. Moreover, subgroup analysis for study design, diagnostic criteria of MetS, sample size, exposure assessment, type of iron, and the population was employed.
Figure 1 presents the detailed flow diagram of the study identification and selection. A total of 1,793 potentially relevant articles (PubMed: 236, Embase: 354, Web of Science: 477, and Scopus: 726) were retrieved during the initial literature search. After eliminating 749 duplicated articles, 1,044 articles were screened according to the titles and abstracts and 751 irrelevant studies were removed. Then, 182 reviews, case reports, or letters; 68 non-human studies; and 29 randomized controlled trial studies were excluded, respectively. Eventually, a total of 14 studies were identified for this meta-analysis.
Figure 1. The detailed flow diagram of the study identification and selection in this meta-analysis.
Table 1 presents the main characteristics of the included studies. These studies were published between 2010 and 2021. Eight studies were performed in Asian countries [Korea (31), China (32, 35, 37, 39, 41), and Iran (33, 40)]. The other 6 studies were conducted in Brazil (29, 38, 42), USA (30), Spain (36), and Columbia (34), respectively. Except for the study by Bruscato (only female) (29), both the male and female participants were considered. The sample size ranged from 284 to 15,051 for a total of 55,131. The dietary iron, copper, and selenium level were assessed by a food-frequency questionnaire (FFQ) in 3 studies (35, 37, 40) and 24 h or 3-day recall method in 11 studies (29–34, 36, 38, 39, 41, 42). The criteria for MetS were the National Cholesterol Education Program-Adult Treatment Panel III (NCEP ATP III) (31, 32, 38–41), the International Diabetes Federation (IDF) (29, 33, 37), and the American Heart Association (AHA) (30, 35, 36) in 6, 3, and 3 studies, respectively. Moreover, some other criteria (34, 42) were also employed for adolescents.
The overall multivariable adjusted RR showed that the dietary iron level was positively associated with MetS (RR = 1.27, 95% CI: 1.12–1.44; p < 0.001) (Figure 2). No substantial level of heterogeneity was obtained among various studies (p = 0.097, I2 = 44.1%). No evidence of publication bias existed according to the Begg's rank correlation test (p = 1.000). Table 2 presents the results of subgroup analysis. The above findings were confirmed in cross-sectional (RR = 1.31, 95% CI: 1.13–1.52; p < 0.001), Asians (RR = 1.44, 95% CI: 1.13–1.83; p = 0.003), the NCEP ATP III (RR = 1.59, 95% CI: 1.30–1.93; p < 0.001), >1,000 sample sized (RR = 1.34, 95% CI: 1.09–1.65; p = 0.006), 24 h or 3-day recall method (RR = 1.25, 95% CI: 1.10–1.42; p < 0.001), and non-heme iron (RR = 1.25, 95% CI: 1.08–1.46; p = 0.004) studies, respectively.
Figure 2. Forest plot of meta-analysis: Overall multi-variable adjusted RR of MetS for the highest vs. lowest dietary iron level.
Table 2. Subgroup analysis of metabolic syndrome (MetS) for the highest vs. lowest dietary iron level category.
The overall multivariable adjusted RR showed that the dietary copper level was negatively associated with MetS (RR = 0.85, 95% CI: 0.78–0.93; p < 0.001) (Figure 3). No substantial level of heterogeneity was obtained among various studies (p = 0.391, I2 = 5.3%). No evidence of publication bias existed according to the Begg's rank correlation test (p = 0.754). Table 3 presents the results of subgroup analysis. The above findings were confirmed in > 1,000 sample sized (RR = 0.86, 95% CI: 0.78–0.94; p = 0.001) and adults (RR = 0.85, 95% CI: 0.78–0.93; p < 0.001) studies, respectively.
Figure 3. Forest plot of meta-analysis: Overall multi-variable adjusted RR of MetS for the highest vs. lowest dietary copper level.
The overall multivariable adjusted RR showed that the dietary selenium level was negatively associated with MetS (RR = 0.77, 95% CI: 0.63–0.95; p = 0.01) (Figure 4). No substantial level of heterogeneity was obtained among various studies (p = 0.985, I2 = 0.0%). No evidence of publication bias existed according to the Begg's rank correlation test (p = 1.000). Table 4 presents the results of subgroup analysis. The above findings were confirmed in Asians (RR = 0.77, 95% CI: 0.63–0.95; p = 0.02), the NCEP ATP III/IDF (RR = 0.79, 95% CI: 0.62–1.00; p = 0.05), >1,000 sample sized (RR = 0.76, 95% CI: 0.61–0.96; p = 0.02), 24 h or 3-day recall (RR = 0.79, 95% CI: 0.62–0.99; p = 0.04), and adults (RR = 0.77, 95% CI: 0.63–0.95; p = 0.02) studies, respectively.
Figure 4. Forest plot of meta-analysis: Overall multi-variable adjusted RR of MetS for the highest vs. lowest dietary selenium level.
A total of 14 observational studies were identified in the present meta-analysis. The pooled results showed that the dietary iron level was positively associated with MetS, whereas a negative association between the dietary copper and selenium level and MetS was obtained.
The pathophysiology of MetS is involved in oxidative stress and inflammation. As a strong pro-oxidant, iron causes oxidative stress and damage to pancreatic beta cells, which decreases the synthesis and secretion of insulin, impairs insulin signaling, and then alters the glucose metabolism (45, 46). Recently, iron-mediated cell death (ferroptosis) has also been reported to induce cardiomyocyte damage and plays an important role in cardiovascular disorders-related pathology (47). On the contrary, copper and selenium are important antioxidants that act against oxidative stress (25, 27). Copper is served as a cofactor of the copper/zinc superoxide dismutase, a protein located in both the cytosol and the mitochondrial inner membrane space to relieve the electron transport chain-generated reactive oxygen species (26). Differently from other metals, selenium works by incorporation into proteins by a cotranslational mechanism (as part of the amino acid selenocysteine) (48). Most selenium proteins participate in antioxidant defense and redox state regulation, particularly the families of glutathione peroxidases and thioredoxin reductases (48). Copper deficiency is associated with the increased high-density lipoprotein (HDL) cholesterol level in rats (49) and blood cholesterol levels in humans (50). Similarly, selenium is also considered to prevent high-fat diet-induced hyperglycemia and liver damage in rats (51) and type 2 diabetes mellitus and cardiovascular disease in humans (52). The above may significantly account for the major findings of this study.
Ferritin, a ubiquitous intracellular protein, is important in the regulation of iron homeostasis (53). Similarly, a meta-analysis of the observational study suggested that the increased ferritin level is positively associated with MetS (54). Moreover, iron chelation therapy (reduce serum ferritin level) was associated with improved serum glucose and HDL levels (55). The phlebotomy with a reduction of body iron could significantly improve cardiovascular risk and glycemic control in patients with MetS (56). The above findings are decent supplements to ours.
Heme iron exists in most animal foods, whereas the rest in animal or plant food is non-heme iron (57). Non-heme iron is absorbed less efficiently than heme iron (~5% non-heme iron and 25% heme iron are absorbed from diet) (41). The epidemiological data indicate that the effect of heme and non-heme iron on MetS may vary from the regional variety (39, 40) and heme iron alone cannot reflect the iron status (58, 59). Indeed, our results with regard to heme and non-heme iron are quite different. The specification of the iron subtype should be considered in further study.
Interestingly, our findings are only confirmed in the NCEP ATP III/IDF diagnostic criteria and > 1,000 sample-sized studies. It is speculated that MetS diagnostic criteria or sample size may influence the results of this study. Moreover, the negative relationship between the dietary iron and selenium and MetS is only specified in the 24 h or 3-day recall method and adult population. Although the number of studies for FFQ and adolescents is limited, the recall method might be precise to reflect the issues and some age-related differences with the dietary pathology of MetS cannot be fully excluded. In addition, the issue of race should also be noted. The corresponding findings only exist in Asians, but not in Americans (with regard to the dietary iron and selenium). Our results suggest that the potential effect of racial variation should not be ignored either.
Our findings can be incorporated into the daily lives of subjects suffering from MetS. The programs to build awareness with collaboration between physicians and nutritionists should be encouraged in the future. For example, reinforce the dietary education in MetS subjects: avoid the dietary iron overdose or copper/selenium deficiency. Nevertheless, the toxicity of excess copper intake should also be emphasized. Excess copper intake is reported to induce oxidative stress, damage to the mitochondrial, contributes to apoptosis, DNA damage, and inflammatory responses (60, 61). Therefore, careful validation by randomized controlled trial/prospective cohort study is still needed before its clinical application.
This study has several strengthens. To begin with, this is the first meta-analysis of observational studies on the associations of the dietary iron, copper, and selenium level with MetS. In addition, the included studies are analyzed based on the adjusted results and large samples. Moreover, the limited heterogeneity level may reflect the decent reliability of our results. Finally, our findings might provide significant information to better consider the dietary effects on MetS.
The limitations of this study should also be acknowledged. First, only 2 prospective cohort studies were identified totally due to the limited relevant literature (causal relationships could not be obtained). Second, the classification of exposure varies greatly among individuals. Third, the adjusted factors and definition of MetS were not uniform. Forth, one included study has combined the data for the dietary iron and red meat as a whole (40). These limitations may weaken the significance of this study.
Our results suggest that the dietary iron level is positively associated with MetS, whereas a negative association between the dietary copper and selenium level and MetS is obtained. Further large well-designed prospective cohort studies are warranted to elaborate on the issues examined in this study.
The raw data supporting the conclusions of this article will be made available by the authors, without undue reservation.
YZ and JD conceived the idea and drafted this manuscript and selected and retrieved relevant papers. ZL and QL performed the statistical analysis. JL and HG assessed each study. YZ was the guarantor of the overall content. All the authors revised and approved the final version of the manuscript.
This study was supported by the National Natural Science Foundation of China (82102581), the National Postdoctoral Science Foundation of China (2021M693562), the Provincial Outstanding Postdoctoral Innovative Talents Program of Hunan (2021RC2020), the Provincial Natural Science Foundation of Hunan (2019JJ40517), the Young Investigator Grant of Xiangya Hospital, Central South University (2020Q14), and the Fuqing Postdoc Program of Xiangya Hospital, Central South University (176).
The authors declare that the research was conducted in the absence of any commercial or financial relationships that could be construed as a potential conflict of interest.
All claims expressed in this article are solely those of the authors and do not necessarily represent those of their affiliated organizations, or those of the publisher, the editors and the reviewers. Any product that may be evaluated in this article, or claim that may be made by its manufacturer, is not guaranteed or endorsed by the publisher.
1. Zhang Y, Zhang D-Z. Relationship between serum zinc level and metabolic syndrome: a meta-analysis of observational studies. J Am Coll Nutr. (2018) 37:708–15. doi: 10.1080/07315724.2018.1463876
2. Gurka MJ, Guo Y, Filipp SL, DeBoer MD. Metabolic syndrome severity is significantly associated with future coronary heart disease in Type 2 diabetes. Cardiovascular Diabetol. (2018) 17:17. doi: 10.1186/s12933-017-0647-y
3. DeBoer MD, Gurka MJ, Golden SH, Musani SK, Sims M, Vishnu A, et al. Independent associations between metabolic syndrome severity and future coronary heart disease by sex and race. J Am Coll Cardiol. (2017) 69:1204–5. doi: 10.1016/j.jacc.2016.10.088
4. Decker JJ, Norby FL, Rooney MR, Soliman EZ, Lutsey PL, Pankow JS, et al. Metabolic syndrome and risk of ischemic stroke in atrial fibrillation: ARIC. Study Stroke. (2019) 50:3045–50. doi: 10.1161/STROKEAHA.119.025376
5. Rochlani Y, Pothineni NV, Kovelamudi S, Mehta JL. Metabolic syndrome: pathophysiology, management, and modulation by natural compounds. Ther Adv Cardiovasc Dis. (2017) 11:215–25. doi: 10.1177/1753944717711379
6. Ranasinghe P, Mathangasinghe Y, Jayawardena R, Hills AP, Misra A. Prevalence and trends of metabolic syndrome among adults in the Asia-pacific region: a systematic review. BMC Public Health. (2017) 17:101. doi: 10.1186/s12889-017-4041-1
7. Zhang Y, Zhang D-Z. Relationship between nut consumption and metabolic syndrome: a meta-analysis of observational studies. J Am Coll Nutr. (2019) 38:499–505. doi: 10.1080/07315724.2018.1561341
8. Zhang Y, Zhang D-Z. Associations of vegetable and fruit consumption with metabolic syndrome. A meta-analysis of observational studies. Public Health Nutr. (2018) 21:1693–703. doi: 10.1017/S1368980018000381
9. Guo H, Ding J, Liang J, Zhang Y. Associations of whole grain and refined grain consumption with metabolic syndrome. A meta-analysis of observational studies. Front Nutr. (2021) 8:695620. doi: 10.3389/fnut.2021.695620
10. Guo H, Ding J, Liang J, Zhang Y. Association of red meat and poultry consumption with the risk of metabolic syndrome: a meta-analysis of prospective cohort studies. Front Nutr. (2021) 8:691848. doi: 10.3389/fnut.2021.691848
11. Guo H, Ding J, Liu Q, Li Y, Liang J, Zhang Y. Vitamin C and metabolic syndrome: a meta-analysis of observational studies. Front Nutr. (2021). 8:728880. doi: 10.3389/fnut.2021.728880
12. Mahan LK, Escott Stump S, Reymond JL. Krause's Food and the Nutrition Care Process. 13th ed. St Louis, MO: Elsevier (2012).
13. Szudzik M, Lipiński P, Jończy A, Mazgaj R, Pieszka M, Kamyczek M, et al. Long-term effect of split iron dextran/hemoglobin supplementation on erythrocyte and iron status, growth performance, carcass parameters, and meat quality of polish large white and 990 line pigs. Biol Trace Elem Res. (2020) 196:472–80. doi: 10.1007/s12011-019-01950-w
14. Mari S, Bailly C, Thomine S. Handing off iron to the next generation: how does it get into seeds and what for? Biochem J. (2020) 477:259–74. doi: 10.1042/BCJ20190188
15. Staroń R, Lipiński P, Lenartowicz M, Bednarz A, Gajowiak A, Smuda E, et al. Dietary hemoglobin rescues young piglets from severe iron deficiency anemia: duodenal expression profile of genes involved in heme iron absorption. PLoS ONE. (2017) 12:e0181117. doi: 10.1371/journal.pone.0181117
16. Podwika W, Kleszcz K, Krośniak M, Zagrodzki P. Copper, manganese, zinc, and cadmium in tea leaves of different types and origin. Biol Trace Elem Res. (2018) 183:389–95. doi: 10.1007/s12011-017-1140-x
17. Puchkova LV, Babich PS, Zatulovskaia YA, Ilyechova EY, Di Sole F. Copper metabolism of newborns is adapted to milk ceruloplasmin as a nutritive source of copper: overview of the current. Data Nutr. (2018) 10:1591. doi: 10.3390/nu10111591
18. Gorini F, Sabatino L, Pingitore A, Vassalle C. Selenium: an element of life essential for thyroid function. Molecules. (2021) 26:7084. doi: 10.3390/molecules26237084
19. Torti SV, Torti FM. Iron and cancer: more ore to be mined. Nat Rev Cancer. (2013) 13:342–55. doi: 10.1038/nrc3495
20. Jung M, Mertens C, Tomat E, Brüne B. Iron as a central player and promising target in cancer progression. Int J Mol Sci. (2019) 20:273. doi: 10.3390/ijms20020273
21. Munoz M, Garcia-Erce JA, Remacha AF. Disorders of iron metabolism. Part 1: Molecular basis of iron homoeostasis. J Clin Pathol. (2011) 64:281–6. doi: 10.1136/jcp.2010.079046
22. Abbaspour N, Hurrell R, Kelishadi R. Review on iron and its importance for human health. J Res Med Sci. (2014) 19:164–74.
23. Galaris D, Barbouti A, Pantopoulos K. Iron homeostasis and oxidative stress: an intimate relationship. Biochim Biophys Acta Mol Cell Res. (2019) 1866:118535. doi: 10.1016/j.bbamcr.2019.118535
24. Hansen JB, Moen IW, Mandrup-Poulsen T. Iron: the hard player in diabetes pathophysiology. Acta Physiol. (2014) 210:717–32. doi: 10.1111/apha.12256
25. Ma X, Jiang S, Yan S, Li M, Wang C, Pan Y, et al. Association between copper, zinc, iron, and selenium intakes and TC/HDL-C ratio in US adults. Biol Trace Elem Res. (2020) 197:43–51. doi: 10.1007/s12011-019-01979-x
26. Ruiz LM, Libedinsky A, Elorza AA. Role of copper on mitochondrial function and metabolism. Front Mol Biosci. (2021) 8:711227. doi: 10.3389/fmolb.2021.711227
27. Ding J, Zhang Y. Relationship between the circulating selenium level and stroke: a meta-analysis of observational studies. J Am Coll Nutr. (2021) 1–9. doi: 10.1080/07315724.2021.1902880
28. Birben E, Sahiner UM, Sackesen C, Erzurum S, Kalayci O. Oxidative stress and antioxidant defense. World Allergy Organ J. (2012) 5:9–19. doi: 10.1097/WOX.0b013e3182439613
29. Bruscato NM, da Costa Vieira JL, do Nascimento NM, Canto ME, Stobbe JC, Gottlieb MG, et al. Dietary intake is not associated to the metabolic syndrome in elderly women. N Am J Med Sci. (2010) 2:182–8.
30. Otto MCO, Alonso A, Lee D-H, Delclos GL, Bertoni AG, Jiang R, et al. Dietary intakes of zinc and heme iron from red meat, but not from other sources, are associated with greater risk of metabolic syndrome and cardiovascular disease. J Nutr. (2012) 142:526–33. doi: 10.3945/jn.111.149781
31. Choi M-K, Bae Y-J. Relationship between dietary magnesium, manganese, and copper and metabolic syndrome risk in Korean adults: the Korea National Health and Nutrition Examination Survey (2007-2008). Biol Trace Elem Res. (2013) 156:56–66. doi: 10.1007/s12011-013-9852-z
32. Li Y, Guo H, Wu M, Liu M. Serum and dietary antioxidant status is associated with lower prevalence of the metabolic syndrome in a study in Shanghai, China. Asia Pac J Clin Nutr. (2013) 22:60–8. doi: 10.6133/apjcn.2013.22.1.06
33. Motamed S, Ebrahimi M, Safarian M, Ghayour-Mobarhan M, Mouhebati M, Azarpazhouh M, et al. Micronutrient intake and the presence of the metabolic syndrome. N Am J Med Sci. (2013) 5:377–85. doi: 10.4103/1947-2714.114171
34. Suarez-Ortegón MF, Ordoñez-Betancourth JE, Aguilar-de Plata C. Dietary zinc intake is inversely associated to metabolic syndrome in male but not in female urban adolescents. Am J Hum Biol. (2013) 25:550–4. doi: 10.1002/ajhb.22408
35. Wei J, Zeng C, Gong Q-y, Li X-x, Lei G-h, Yang T-b. Associations between dietary antioxidant intake and metabolic syndrome. PLoS ONE. (2015) 10:e0130876. doi: 10.1371/journal.pone.0130876
36. Bulka CM, Daviglus ML, Sotres-Alvarez D, Durazo-Arvizu RA, Mossavar-Rahmani Y, Persky VW, et al. Dietary minerals and metabolic syndrome: results from the hispanic community health study/study of latinos (HCHS/SOL). Circulation. (2018) 137:AP246. doi: 10.1161/circ.137.suppl_1.p246
37. Qu R, Jia Y, Liu J, Jin S, Han T, Na L. Dietary flavonoids, copper intake, and risk of metabolic syndrome in Chinese adults. Nutrients. (2018) 10:991. doi: 10.3390/nu10080991
38. Vieira DAS, Sales CH, Cesar CLG, Marchioni DM, Fisberg RM. Influence of haem, non-haem, and total iron intake on metabolic syndrome and its components: a population-based study. Nutrients. (2018) 10:314. doi: 10.3390/nu10030314
39. Zhu Z, Wu F, Lu Y, Wu C, Wang Z, Zang J, et al. Total and nonheme dietary iron intake is associated with metabolic syndrome and its components in Chinese men and women. Nutrients. (2018) 10:1663. doi: 10.3390/nu10111663
40. Esfandiar Z, Hosseini-Esfahani F, Mirmiran P, Habibi-Moeini A-L, Azizi F. Red meat and dietary iron intakes are associated with some components of metabolic syndrome: Tehran Lipid and Glucose Study. J Transl Med. (2019) 17:313. doi: 10.1186/s12967-019-2059-0
41. Zhu Z, He Y, Wu F, Zhao L, Wu C, Lu Y, et al. The associations of dietary iron, zinc and magnesium with metabolic syndrome in China's mega cities. Nutrients. (2020) 12:659. doi: 10.3390/nu12030659
42. Batista CC, Nascimento LM, de Sá Lustosa LCR, Rodrigues BGM, Campelo V, de Macêdo Gonçalves Frota K. Metabolic syndrome in adolescents and antioxidant nutrient intake: a cross-sectional study. Rev Assoc Med Bras. (2021) 67:918–25. doi: 10.1590/1806-9282.20200733
43. Liberati A, Altman DG, Tetzlaff J, Mulrow C, Gøtzsche PC, Ioannidis JP, et al. The PRISMA statement for reporting systematic reviews and meta-analyses of studies that evaluate healthcare interventions: explanation and elaboration. BMJ. (2009) 339:b2700. doi: 10.1136/bmj.b2700
44. Begg CB, Mazumdar M. Operating characteristics of a rank correlation test for publication bias. Biometrics. (1994) 50:1088–101. doi: 10.2307/2533446
45. Rajpathak SN, Crandall JP, Wylie-Rosett J, Kabat GC, Rohan TE, Hu FB. The role of iron in type 2 diabetes in humans. Biochim Biophys Acta. (2009) 1790:671–81. doi: 10.1016/j.bbagen.2008.04.005
46. Fernandez-Real JM, McClain D, Manco M. Mechanisms linking glucose homeostasis and iron metabolism toward the onset and progression of type 2 diabetes. Diabetes Care. (2015) 38:2169–76. doi: 10.2337/dc14-3082
47. Wu X, Li Y, Zhang S, Zhou X. Ferroptosis as a novel therapeutic target for cardiovascular disease. Theranostics. (2021) 11:3052–9. doi: 10.7150/thno.54113
48. Roman M, Jitaru P, Barbante C. Selenium biochemistry and its role for human health. Metallomics. (2014) 6:25–54. doi: 10.1039/C3MT00185G
49. Lefevre M, Keen CL, Lönnerdal B, Hurley LS, Schneeman BO. Copper deficiency-induced hypercholesterolemia: effects on HDL subfractions and hepatic lipoprotein receptor activity in the rat. J Nutr. (1986) 116:1735–46. doi: 10.1093/jn/116.9.1735
50. Salonen JT, Salonen R, Korpela H, Suntionen S, Tuomilehto J. Serum copper and the risk of acute myocardial infarction: a prospective population study in men in Estern Finland. Am J Epidemiol. (1991) 134:268–76. doi: 10.1093/oxfordjournals.aje.a116080
51. Gao Y, Xu Y, Ruan J, Yin J. Selenium affects the activity of black tea in preventing metabolic syndrome in high-fat diet-fed Sprague-Dawley rats. J Sci Food Agric. (2020) 100:225–34. doi: 10.1002/jsfa.10027
52. Wang X-L, Yang T-B, Wei J, Lei G-H, Zeng C. Association between serum selenium level and type 2 diabetes mellitus: a non-linear dose-response meta-analysis of observational studies. Nutr J. (2015) 15:48. doi: 10.1186/s12937-016-0169-6
53. Cook JD, Lipschitz DA, Miles LE, Finch CA. Serum ferritin as a measure of iron stores in normal subjects. Am J Clin Nutr. (1974) 27:681–7. doi: 10.1093/ajcn/27.7.681
54. Cooksey RC, Jones D, Gabrielsen S, Huang J, Simcox JA, Luo B, et al. Dietary iron restriction or iron chelation protects from diabetes and loss of beta-cell function in the obese (ob/ob lep-/-) mouse. Am J Physiol Endocrinol Metab. (2010) 298:E1236–43. doi: 10.1152/ajpendo.00022.2010
55. Houschyar KS, Ludtke R, Dobos GJ, Kalus U, Broecker-Preuss M, Rampp T, et al. Effects of phlebotomy-induced reduction of body iron stores on metabolic syndrome: results from a randomized clinical trial. BMC Med. (2012) 10:54. doi: 10.1186/1741-7015-10-54
57. Monsen ER, Hallberg L, Layrisse M, Hegsted DM, Cook JD, Mertz W, et al. Estimation of available dietary iron. Am J Clin Nutr. (1978) 31:134. doi: 10.1093/ajcn/31.1.134
58. He J, Shen X, Fang A, Song J, Li H, Guo M, et al. Association between predominantly plant-based diets and iron status in Chinese adults: a cross-sectional analysis. Br J Nutr. (2016) 116:1621–32. doi: 10.1017/S0007114516003639
59. Asakura K, Sasaki S, Murakami K, Takahashi Y, Uenishi K, Yamakawa M, et al. Iron intake does not significantly correlate with iron deficiency among young Japanese women: a cross-sectional study. Public Health Nutr. (2009) 12:1373–83. doi: 10.1017/S1368980008004072
60. Huo H, Wang S, Bai Y, Liao J, Li X, Zhang H, et al. Copper exposure induces mitochondrial dynamic disorder and oxidative stress via mitochondrial unfolded protein response in pig fundic gland. Ecotoxicol Environ Saf . (2021) 223:112587. doi: 10.1016/j.ecoenv.2021.112587
Keywords: dietary iron level, dietary copper level, dietary selenium level, metabolic syndrome, meta-analysis, observational studies
Citation: Ding J, Liu Q, Liu Z, Guo H, Liang J and Zhang Y (2022) Associations of the Dietary Iron, Copper, and Selenium Level With Metabolic Syndrome: A Meta-Analysis of Observational Studies. Front. Nutr. 8:810494. doi: 10.3389/fnut.2021.810494
Received: 07 November 2021; Accepted: 21 December 2021;
Published: 01 February 2022.
Edited by:
Mohammed S. Razzaque, Lake Erie College of Osteopathic Medicine, United StatesReviewed by:
Farhana Akter, Chittagong Medical College, BangladeshCopyright © 2022 Ding, Liu, Liu, Guo, Liang and Zhang. This is an open-access article distributed under the terms of the Creative Commons Attribution License (CC BY). The use, distribution or reproduction in other forums is permitted, provided the original author(s) and the copyright owner(s) are credited and that the original publication in this journal is cited, in accordance with accepted academic practice. No use, distribution or reproduction is permitted which does not comply with these terms.
*Correspondence: Yi Zhang, emhhbmd5aTAyMDVAY3N1LmVkdS5jbg==
Disclaimer: All claims expressed in this article are solely those of the authors and do not necessarily represent those of their affiliated organizations, or those of the publisher, the editors and the reviewers. Any product that may be evaluated in this article or claim that may be made by its manufacturer is not guaranteed or endorsed by the publisher.
Research integrity at Frontiers
Learn more about the work of our research integrity team to safeguard the quality of each article we publish.