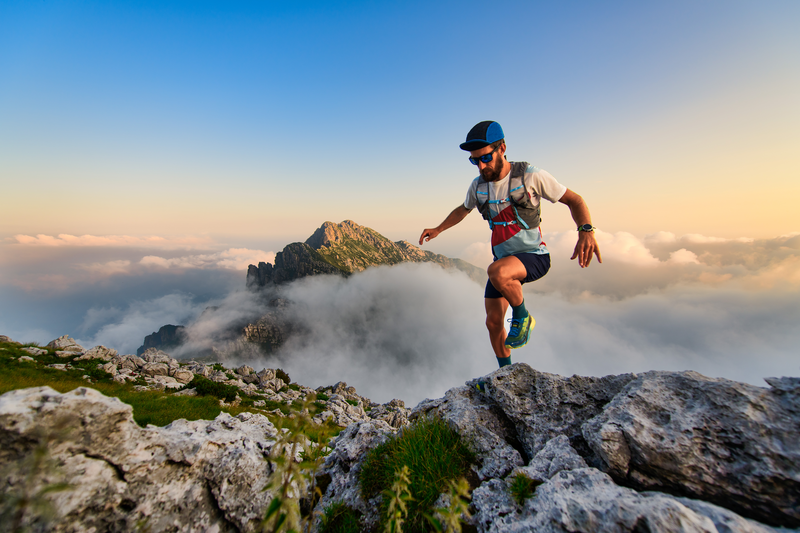
94% of researchers rate our articles as excellent or good
Learn more about the work of our research integrity team to safeguard the quality of each article we publish.
Find out more
REVIEW article
Front. Nutr. , 12 January 2022
Sec. Nutrition and Sustainable Diets
Volume 8 - 2021 | https://doi.org/10.3389/fnut.2021.793414
This article is part of the Research Topic Dietary Change Strategies for Sustainable Diets and their Impact on Human Health - Volume 1 View all 44 articles
Simple lifestyle changes can prevent or delay the onset of type 2 diabetes mellitus (T2DM). In addition to maintaining a physically active way of life, the diet has become one of the bases in managing TD2M. Due to many studies linking the ability of resistant starch (RS) to a substantial role in enhancing the nutritional quality of food and disease prevention, the challenge of incorporating RS into the diet and increasing its intake remains. Therefore, we conducted this review to assess the potential benefits of RS on metabolic biomarkers in pre-diabetes and diabetes adults based on available intervention studies over the last decade. Based on the conducted review, we observed that RS intake correlates directly to minimize possible effects through different mechanisms for better control of pre-diabetic and diabetic conditions. In most studies, significant changes were evident in the postprandial glucose and insulin incremental area under the curve (iAUC). Comparative evaluation of RS consumption and control groups also showed differences with inflammatory markers such as TNF-α, IL-1β, MCP-1, and E-selectin. Only RS2 and RS3 were extensively investigated and widely reported among the five reported RS types. However, a proper comparison and conclusion are deemed inappropriate considering the variations observed with the study duration, sample size, subjects and their metabolic conditions, intervention doses, and the intervention base products. In conclusion, this result provides interesting insights into the potential use of RS as part of a sustainable diet in diabetes management and should be further explored in terms of the mechanism involved.
Diabetes mellitus is a metabolic disorder characterized by hyperglycemia due to defective insulin secretion, action or both (1). The diagnostic criteria for diabetes include fasting plasma glucose (FPG) ≥7.0 mmol/L (2), glycated hemoglobin (HbA1c) ≥6.5% (2–4), 2-h plasma glucose (2hPG) in a 75 g oral glucose tolerance test (OGTT) ≥11.1 mmol/L (2) or random PG ≥11.1 mmol/L. Meanwhile, pre-diabetes refers to impaired fasting glucose (IFG) of 6.1–6.9 mmol/L (5), impaired glucose tolerance (IGT)/2hPG in a 75 g OGTT of 7.8–11.0 mmol/L (5) or HbA1c of 6.0% to 6.4% (6), each of which places individuals at high risk of developing diabetes and its complications (7).
A recent study by Khan and his team (8) presented the epidemiology of type 2 diabetes mellitus (T2DM) in terms of the global burden of disease and forecasted trends. The study showed that T2DM continues to be the leading cause of human suffering and deaths as it continues to increase in prevalence and incidence. This phenomenon continues, and there are no sights for reduction despite efforts in clinical care, research and public health interventions (8). Globally, it was recognized that diets low in whole grains, nuts, seeds and fruits were the leading dietary risks, especially in developing countries where globalization and emerging supermarkets increase access to processed, salt-laden, high-fat and sugar-added food products. Consequently, the attractiveness in line with low prices and higher accessibility led to decreased intake of whole grains, fruits and vegetables. In addition, poor dietary control and a sedentary lifestyle contribute to higher BMI that further elevates the associated risks (9).
The nutritional recommendation based on FAO/WHO states that the acceptable macronutrient distribution range should be within 55–75% of net energy for carbohydrates, 10% for sugar components, while dietary fiber intake requirements differ with 38 g and 25 g for men and women, respectively (10). Several published findings have highlighted the association of high fiber and grains intake toward lower risk of both obesity and diabetes. However, the usual dietary pattern does not meet the recommended quantity, and hence it was suggested to include an additional grain-fibrous food for a more enhanced approach in managing metabolic conditions, such as diabetes (11–13).
Starch is the predominant form of carbohydrate and is categorized as rapidly digestible starch (RDS), slowly digestible starch (SDS) and resistant starch (RS) determined based on their digestion rate. SDS seems to be slowly digested within the small intestines, whereas RDS tends to elevate glucose levels rapidly in the blood upon consumption. On the other hand, RS is undigested in the small intestines and usually fermented in the colon (14). Several studies have shown that RS is a linear molecule of α-1,4-D-glucan, derived from the retrograded amylose fraction and has a relatively low molecular weight (1.2 × 105 Da) (15, 16). The chemical structure of α-1,4-D-glucan is shown in Figure 1 (17).
Figure 1. Chemical structure of α-1,4-D-glucan [Obtained from: (17)].
RS is used widely as a functional ingredient, especially in foods containing high dietary fiber levels. These types of food are assumed to help prevent several pathologies such as colon cancer, diabetes, or obesity (18). It has been proposed that foods containing naturally occurring RS or foods modified to contain more RS may alter the postprandial glycemic response, prevent hypoglycemia, reduce hyperglycemia and explain differences in some foods' glycemic index (GI). Dietary supplementation of RS is one of the nutritional interventions practiced for metabolic disease (19). Bread, cooked cereals and pasta, vegetables, just-ripe bananas are several familiar food products that serve as natural as natural RS sources. Consumption of RS foods tends to resist digestion in the small intestines and reaches the large intestines for microbial fermentation (14, 20, 21).
There are five types of RS based on their indigestible properties (RS1, RS2, RS3, RS4, and RS5) (16, 17). RS1 refers to physically entrapped starch within whole plant cells and food matrices (e.g., partly milled grains and seeds) where there is a physical barrier to amylolysis. The presence of intact cell walls contributes to the RS content of legumes. Extensive milling (and chewing) allows these starches to be more accessible and less resistant. RS2 comprises the poorly gelatinized and hydrolyzed granules by α-amylases from certain plants (e.g., raw potato and green banana, HAM). Retrograded starches constitute RS3, which includes cooked and cooled rice or potatoes. Meanwhile, RS4 is a group of chemically modified starches that can improve the functional characteristics of the starch. Amylose-lipid complex has been proposed as RS5 because high-amylose starch tends to be more resistant to enzyme hydrolysis than low-amylose starch. The amylose-lipid complex in starch granules increases their enzyme resistance by restricting the granule swelling during cooking. Although these modified starches are found widely in processed foods, neither their contribution to RS intakes nor their physiological effects have been extensively studied (22).
RS has the advantage of having a less negative influence on the sensory properties of final products than traditional fibers, such as whole grains, bran or fruit fibers, which is very positive for consumer acceptability (23). Consumption of RS has resulted in changes in insulin sensitivity, IGT and satiety in healthy humans (24) and therefore has been hypothesized to have implications for glycemic control in individuals at risk of or with T2DM. Among the five types, RS2 and RS3 were the most common form utilized for interventions, especially in evaluating their effects on blood lipids, GI and colon cancer (24, 25). RS-based diets are one of the nutritional interventions practiced for metabolic disease control, and these include common food sources such as bread, cooked cereals and pasta, vegetables and just-ripe bananas. Despite the numerous interventions on evaluating RS effects on diabetic control, the findings seem to be limited to short-term studies and lack longer-term studies to prove the benefits of RS. Thus, in this review, we intend to explore the reported studies on RS diet interventions to further understand its importance as well as to investigate the possible effects exerted by various RS-based intervention studies among pre-diabetes and diabetes adults.
Original articles were searched in three databases (PubMed, Scopus and ScienceDirect) from the year 2011 to 2021 using the Medical subject heading (MeSH) terms “diabetes,” crossed with the term “resistant starch.” Publications with available abstracts were reviewed and limited to studies published in English. Papers on human and clinical trials related to diabetes were included. However, review articles, proceedings, letters to the editor, and in vitro and in vivo studies were excluded. Duplicate articles were eliminated. The study identification process and reasons for exclusion are illustrated in Figure 2.
We included published intervention studies (defined as a randomized controlled trial, crossover study and quasi-experimental study) comparing markers of glucose metabolism for RS consumption. We included only human studies with adult participants aged at least 18 years old from both genders. Studies were included if they analyzed at least one of the biomarkers as mentioned earlier.
A pair of authors independently assessed the titles and abstracts during the initial screening. The difference in the initial assessment was resolved by a discussion leading to a consensus, with a third party serving as arbitrator if necessary. Each study was recorded as include, exclude or unclear. Full articles were retrieved for further assessment if recorded as include or unclear. Any disagreement was re-evaluated and re-assessed among the reviewers.
The search resulted in 50 articles produced with a refined search based on the availability of full text, peer-reviewed articles and library collection access. Upon further assessment, only 24 full-text articles were relevant and included for final review (Table 1). All the related articles were printed out for further assessment of evidence-based to explore the effectiveness of RS as a potential antidiabetic agent.
Table 1 was constructed based on available intervention studies on RS over the last decade. Although there are five types of RS, only three types have been clinically studied based on our search strategies from the three selected databases. HAM-RS2, an ingredient available to both food producers and consumers, was the usual form of RS2 used. HAM-RS2 is a bland, white substance with a small particle size comprised of 60% RS and 40% SDS (50). A randomized crossover study done by Bodinham and her colleagues on 12 overweight individuals reported significantly higher plasma insulin and C-peptide concentrations in individuals who consumed 40 g/d RS2 for 4 wks compared with the placebo (27). The process of insulin synthesis involves cleaving stages of C-peptide from proinsulin and is stored within secretory granules before its release in equimolar amounts with insulin into the bloodstream. C-peptide plays a vital role during this process by linking the A and B chains leading to precise folding and formation of interchain disulphide bonds (51). The presence of a higher level of both insulin and C-peptide assists the cells for better glucose absorption, blood sugar reduction and channeling glucose to the cells for energy synthesis. It is important to note that the circulating venous (or arterial) fasting insulin concentrations are about 18–90 pmol/L in healthy LN individuals (52). However, no changes were reported to either bodyweight or habitual food intake of the subjects in the study by Bodinham and team (26).
In another crossover study done by the same group of researchers on individuals with well-controlled T2DM, RS2 consumption resulted in significantly lower postprandial glucose concentrations (p < 0.05) without any effect on hepatic, peripheral insulin sensitivity or HbA1c levels (27). Several biochemical abnormalities were observed in insulin and glucagon secretion, uptake and suppression of hepatic glucose production, and uptake of peripheral glucose among diabetic individuals that led to higher and prolonged postprandial glucose (PPG) excursions when compared with non-diabetic individuals (53). Generally, PPG accounted for ~80% of HbA1c when HbA1c was <6.2% and only about 40% when HbA1c was above 9.0% (54). Decreases in PPG accounted for nearly twice as much for the reduction in HbA1c as did the decline in FPG. HbA1c values reflect overall glycemic exposure over the past 2-3 mths integrating both FPG and PPG levels.
Despite no improvement effect of HAM-RS2 on tissue insulin sensitivity in well-controlled T2DM, it demonstrated beneficial effects on meal handling, possibly due to higher postprandial glucagon-like peptide-1 (GLP1). GLP-1 delays gastric emptying and gut motility in healthy LN and obese subjects and patients with T2DM (55, 56). GLP-1 also contributes to the change in gastric volume that occurs in anticipation of food ingestion (57). In contrast, a double-blind crossover study done by Dainty and her colleagues in adults at an increased risk of T2DM characterized by a Canadian diabetes risk assessment questionnaire (CANRISK) score ≥21 (58) found that consumption of one bagel containing 25 g/d HAM-RS2 for 8 wks did not show any differences in FPG and PPG between the RS-intervention and control. However, the HAM-RS2 bagel recorded significantly lower fasting insulin iAUC and fasting IR (28). These findings are consistent with the results of another study done by Galarregui and co-workers that found positive associations of both fasting insulin and HOMA-IR with the insulin iAUC for foods with varied macronutrient composition consumed by senior participants (59). Interestingly, an investigation by Peterson and co-investigators (29) also recorded no improvement in glycemic control, cardiovascular disease risk factors and energy metabolism relative to baseline when pre-diabetic adults consumed HAM-RS2 for 12 wks. The sole exception was a decrease in circulating concentrations of TNF-α.
A possible explanation for the inconsistent findings of RS2 is partly because of the underlying dietary variability among individuals who participated in the studies. As mentioned earlier, RS2 supplementation did not improve cardiometabolic health in adults with pre-diabetes, although it does reduce TNF-α concentrations (29). Thus, it may be necessary to search for any relevant articles that correlate various factors in terms of gut microbiota, diet composition, biological and environmental factors that respond better to RS2 supplementation than others. For example, the possible mechanism in the management of T2DM via activation of protein phosphatase-4, TNF-α and plasminogen-activator inhibitors were reported in several studies (60, 61). These articles could lead to a better understanding of the potential beneficial effects of RS supplementation on metabolic health and whether such effects are modulated by diet compositions or existing microbial populations in the gut.
In addition, increasing evidence relates the roles of gut microbiota with T2DM development (62). Diabetic developments are also affected by chemical and diet-related factors (63). Recently, RS2 in the form of green-banana biomass studied in 39 T2DM patients of both genders for 6 mths showed significantly lower TC, non-HDL-cholesterol, glucose, and HbA1c levels (p < 0.05), as well as improved the LDL particles protection against oxidation which indirectly assists in lowering cardiovascular disease risk among T2DM patients (31). Gargari and co-investigators (32) have conducted a study to determine if RS can react as an alimentary prebiotic for patients with T2DM. They have found that RS2 decreased HbA1c (−0.3%, −3.6%) and TNF-α (−3.4 pg/mL, −18.9%) significantly compared with the placebo group (p < 0.05). However, changes in FBS, hs-CRP and IL-6 were not significant in the RS2 group compared with the control group. In contrast to the previous finding by Heianza and co-workers (6), both studies done by Lotfollahi and Gargari recorded decreased HbA1c levels after the intervention (31, 32).
Although RS2 can improve the glycemic status and inflammatory markers in some individuals with T2DM, more studies are needed to confirm the efficacy of RS2 as an adjunct therapy in diabetes, as reported by Gargari et al. (32). Similarly, Alfa and colleagues conducted another study to evaluate the tolerability, glucose, and insulin modulating ability in healthy MID and ELD adults upon consumption of MSPrebiotic® digestion resistant starch (DRS) (33). MSPrebiotic® is a product made from unmodified natural DRS from potatoes and contains an active ingredient, Solanum tuberosum extract. The subjects were randomized and required to consume 30 g/d of either MSPrebiotic® or placebo for 12 wks. Several parameters including blood glucose, lipid profile, CRP, TNF-α, IL-10, and insulin resistance (IR) were measured over the whole study period. Baseline data from this study indicated that the ELD population had a significantly higher elevated glucose and TNF-α compared to MID adults. Interestingly, a significant difference was observed in blood glucose, insulin levels and HOMA-IR in ELD subjects who consumed MSPrebiotic® compared to placebo. Unfortunately, there were no significant changes found in MID subjects. A newly published systematic review to evaluate the effect of prebiotics on metabolic and inflammatory biomarkers in T2DM patients found that ~70% of the study reported improvements in glycemia including HBA1c, HOMA-IR and inflammatory markers (64).
Besides its action on diabetic parameters and gut microbiota, RS2 was also found to affect macronutrient oxidation based on a study conducted in healthy adults aged 25–45 yrs in 2019. The subjects who consumed RS and RS high fiber (RSF) demonstrated a 32% increase in fat oxidation (p < 0.05) with a concomitant 18% decrease in CHO oxidation (p < 0.05). Furthermore, insulin responses were unaltered after breakfast but lower in RS and RSF after lunch, at equivalent glucose concentrations. Based on this finding, RS and RSF were expected to improve insulin sensitivity at later meals, though there has been no earlier expression of insulin response (34). Nevertheless, this study was carried out on healthy adults and dissimilar findings can be documented if the trial was performed on patients with T2DM.
Based on our search, we also noticed that RS3-based interventions had been extensively investigated. In 2019, Belobrajdic and his colleagues (35) had conducted a crossover-controlled study on 20 healthy non-diabetic men who consumed bread made from HAW and LAW. They determined that flour processing did not affect the glycemic, insulinemic or increatin response. However, HAW bread had lower insulinemic and increatin (24–30% less) than LAW (35). In other words, replacing LAW with HAW flour is more practical as an effective strategy for lowering glycemic and insulinemic responses in healthy men. Figure 3 represents the schematic action of gut nutrients on insulin release (65, 66). From a mechanistic perspective, vast data suggest that acetate has an important regulatory role in body weight control and insulin sensitivity via lipid metabolism and glucose homeostasis.
In another study, healthy subjects who consumed EAW bread showed significantly lower PPG response than those who consumed the reference white wheat bread during the first 120 min (p < 0.05), although there were no differences in insulin responses (36). This novel wheat with 38% EAW was field-grown under standard cultural practices in the experimental station of the University of Tuscia (Viterbo, Central Italy). Interestingly, higher RS content in bread significantly reduced the GI (p < 0.001). Despite promising results, this study was limited in terms of sample size that does not guarantee effectiveness in a larger population. Hence, further investigations are warranted for a more effective outcome on the roles of EAW in the improvement of postprandial glycemic response (36).
Besides using wheat bread, the effect of grain sorghum flour was also investigated in both healthy and pre-diabetic subjects, respectively (37, 38). Grain sorghum flour contained 22.7 ± 0.8, 43.8 ± 0.8, and 33.5 ± 0.1, whereas wheat flour contained 37.5 ± 0.3, 47.4 ± 0.4, and 15.1 ± 0.1 of RDS, SDS, and RS; respectively. In healthy subjects, incremental changes in plasma glucose and plasma insulin concentrations were recorded with the grain sorghum muffin treatment. Furthermore, the glucose responses were significantly lower, particularly at 45–120 min intervals (p < 0.05) and mean insulin responses reduced at 15–90 min intervals compared to control (p < 0.05). This study demonstrated a significant reduction at about 26% in the mean glucose iAUC responses with sorghum at 2,871 ± 163 mg compared to wheat at 3,863 ± 443 mg (~3 h)/dL. Similarly, insulin responses also significantly decreased from 3,029 ± 965 μU (~3 h)/L for wheat to 1,357 ± 204 with sorghum (p < 0.05), respectively. Furthermore, significant reduction on postprandial blood glucose and insulin responses within 45–120 min intervals (p < 0.05) along with reduction in glucose iAUC by 35.0%, from 5457.5 ± 645.4 to 3550.0 ± 428.9 mg (~3 h)/ dL (p < 0.05) were recorded in pre-diabetic men. Additionally, the mean insulin iAUC was also significantly lowered by 36.7%, from 7254.6 ± 1228.9 to 4589.3 ± 737.2 mU (~3 h)/L (p < 0.05). In short, both studies documented interesting results that grain sorghum is an excellent functional ingredient to assist in managing glucose and insulin levels among healthy individuals and controlling blood glucose and insulin levels among the pre-diabetic population (37, 38).
A group of researchers has also embarked on a study to evaluate the effect of a new formula, PPB-R-203 (a retrograded starch product manufactured by Pharma Power Biotec Co., Ltd.) vs. commercially available food on blood glucose regulation and safety in both healthy and T2DM individuals aged 20–65 yrs old (39). The findings demonstrated that mean blood glucose concentration and glucose iAUC were lower with the PPB-R-203 regimen than control among T2DM patients. In brief, PPB-R-203-based food managed to improve postprandial hyperglycemia in T2DM patients without giving a risk of hypoglycemia compared with commercially available food (39).
Recently, a comparison study has been done by Sanders and his friends to assess the metabolic effects in subjects that consumed 300 g of cooked then chilled potatoes containing ~18 g of RS compared to a control diet matched for calories and macronutrients but with <1 g of RS over a 24 h period (40). By introducing RS-enriched potatoes, the values for muscle insulin sensitivity index (MISI), HOMA2 insulin sensitivity (%S), and HOMA2 β-cell function (%B) increased, although the differences did not approach statistical significance compared with the control group. However, a significant reduction of the FPG (p < 0.05) was recorded with a slight insignificant decrease of fasting plasma insulin (p = 0.077). In addition, breath hydrogen at the end of 300 min was significantly higher (p < 0.05), and plasma FFA was significantly lower (p < 0.05) in the potato condition compared to the control. Even though RS-enriched potatoes may have a favorable impact on CHO metabolism, additional research with a large study sample is necessary.
Newly developed high fiber white rice (HFWR) has also been investigated for its GI and effect on glycemic parameters among healthy volunteers. The subjects were given HFWR and commercial white rice (WR) diets containing 50 g of available CHO as the test foods. HFWR was documented with a 23% lower GI value than WR, in which HFWR was found to be of medium GI (61.3) whereas WR was of higher GI (79.2). The dietary fiber, RS, and amylose content were also significantly higher in HFWR than WR. These results showed that the newly developed HFWR had a lower GI and other favorable characteristics than WR. Hence, the HFWR could help reduce the overall dietary GI and the glycemic load (41). However, it may be necessary to conduct an additional study to identify the HFWR capacity on its impact on blood glucose parameters, particularly for those with disabilities in glucose management.
Another valuable product that has been recognized as one of the ancient cultivated cereal grains globally is barley (Hordeum vulgare L.). Barley is an enriched source of high fiber contents, at about 15.6 g/100 g (67). Nomura and his colleagues conducted a study to determine the GI, blood glucose, postprandial blood glucose and iAUC after BARLEYmax® consumption in healthy Japanese subjects (42). BARLEYmax® is a unique, non-genetically modified barley developed by Australia's Commonwealth Scientific and Industrial Research Organization (CSIRO) (68). They found that the GI of the BARLEYmax® was 24.3, and glucose iAUC was statistically lower than those who took the ordinary glucose drink. They presumed that the presence of fructans in BARLEYmax® at 11% could contribute to glycemic response reduction, which is higher than other components comprised of RS, β-glucan and arabinoxylan at 3, 6 and 7%, respectively (42).
Meanwhile, Zhu and his colleagues have investigated the potential benefits of a combination of several non-cereal starchy (LS, AD and dried LB) compared with (MI, BR and AB) into a glycemic management diet while taking the pre-soaking and cooking duration into account (43). Unfortunately, despite being whole grains, the waxy and pre-soaked cereals can be very high-GI food. Moreover, pulses such as the AB can maintain a low GI even after a long-time pre-soaking and prolonged cooking and the LS is a valuable low-GI food ingredient in staple food which behaved more like pulses in terms of GR, while the AD and LB were more like millet and strongly dependent on cooking practice for GI values. Thus, they concluded that careful choice of whole-grain materials, minimized pre-soaking, and moderate cooking may be critical for successful glycemic management for people with impaired glucose metabolism. On the contrary, Yulianto and his friends evaluated the content of RS and GI of Cr-PR coated with herbal extracts (cinnamon bark powder, pandan leaf and bay leaf) with concentrations of Cr-PR at 1, 2, and 3%, respectively (44). In their study, 18 non-diabetic individuals were required to consume one type of cooked rice sample equivalent to 50 g of glucose. They recorded that the RS content of Cr-PR coated with herbal extracts ranged between 8.27 and 8.84% and the highest RS was gained from coating rice with 3% cinnamon extract. On the whole, it was classified into the low GI category as it ranged from 29 to 40 and the lowest GI was observed in Cr-PR coated with 6–9% of cinnamon extract (29–30) (44).
So far, no specific high-fiber or CHO-rich foods are arguably superior to the others. Sandberg and his colleagues have conducted a randomized, controlled, crossover overnight study to investigate the effect of WG-based products on glucose and appetite regulation (45). In this noteworthy study, four rye-based evening test meals were given to 21 healthy subjects consisting of either whole-grain RFB or a 1:1 ratio of RFB/RKB, with or without added RS, while WWB was used as the reference meal. Interestingly, the findings showed that the evening test meal of RFB/RKB + RS resulted in decreased postprandial glucose and insulin responses and increased the gut hormone in plasma the following morning after the standardized breakfast. Apart from that, consumption of RFB and RFB/RKB also resulted in a decreased feeling of hunger in the test subjects (AUC 0–210 min), while all the rye-based evening meals resulted in reduced fasting FFA and increased breath hydrogen concentration. This finding suggests that WG bread had the potential to improve cardiometabolic variables at 11 to 14.5 h perspective in healthy humans (45).
Research on cardiometabolic risk markers that consumed RB has also been evaluated in 38 healthy individuals aged 52–70 yrs. Insulin sensitivity index, PYY levels and plasma acetate fasting concentrations, butyrate, and total SCFA were recorded to increase with RB + RS2 bread product consumption. In addition, the insulin sensitivity index was found to correlate positively with working memory test performance (Figure 3). Intriguingly, IL−1β fasting levels were found to decrease after the intervention (46).
Besides RS2- and RS3-based diets studied by numerous researchers, wheat-based RS4-enriched flour (30% v/v) has also been investigated in 86 subjects above 18 yrs old (47). Except for TG, the RS4-enriched flour significantly reduced cholesterol levels (TC, HDL and non-HDL, including LDL). Although mean blood glucose levels were lowered with RS4 consumption, the differences did not approach statistical significance compared to the control. Mesa Garcia and her colleagues have taken a step ahead of research to study a new fructose-free DSF in fragile elderly T2DM patients for 6 wks and evaluate the influence of total enteral feeding on the glycemic control and diabetes-derived cardiovascular risk biomarkers (48). These new fructose-free DSF and RS4-enriched DSF formulations effectively controlled glycemic by decreasing blood HbA1c, and some improvements were documented in MCP-1, sE-selectin, and sVCAM-1 and PAI-1 plasma levels. While this formulation managed to improve glycemic control and cardiovascular risk biomarkers, lipid metabolism has not changed, which is helpful for the nutritional treatment of frail elderly T2DM patients.
Apart from RS4- enriched flour and DSF formulations, two fermentable-fibers were also investigated on postprandial SCFA and second-meal glycemic response among healthy OWO vs. LN participants (NCT02562014) (49). Intriguingly, no significant differences were observed in postprandial SCFA responses between LN and OWO participants after acute fiber consumption. They reported that any effect of the colonic fermentation of dietary fiber on glycemic responses was not attributed to SCFA, as IN increased SCFA serum without reducing the second-meal glycemic response. The low glucose response after RS consumption at 4–6 h is consistent with other studies that find the RS therapy increases insulin susceptibility and reduces insulin and C-peptide responses (49). The decreased glycemic reaction is probably not related to SCFA but may be related to the decrease in FFA recovery. A higher-than-expected amount of available but SDS in the RS ingredient used would have explained the acute reduction of FFA, as both prolonged and enhanced absorption of CHO managed to reduce postprandial FFA rebound. Based on these findings, they predicted that IN is fermented promptly in the colon and that breath-hydrogen and serum SCFA concentrations are rapidly rising, while RS is fermented gradually for 6 h after consumption with far smaller increases of breath hydrogen and serum SCFA. Moreover, the results did not demonstrate the impact on glucose metabolism of the acute increments in SCFA. In addition, the results did not support the hypothesis that colonial SCFA was increased due to obesity/overweight conditions (49).
In conclusion, our review highlighted the benefits of RS that are loaded with antidiabetic properties. A considerable amount of RS consumption acts differently through several pathways to reduce the severity and exert better control of the diabetic condition. Although a specific RS type could not be highlighted for a better action mechanism, we observed that both RS2 and RS3 were among the most extensively explored form of RS over the last decade. The search results demonstrated that most of the studies were focused on insulin secretion and plasma glucose. Nevertheless, the RS effectiveness on HbA1c, inflammatory markers and lipid profile were also investigated in several studies. However, a proper comparison and conclusion are deemed inappropriate in view that the study parameters varied in terms of study duration, sample size, subjects and their metabolic conditions, intervention doses, and the intervention base products. Furthermore, the underlying mechanisms involved in the action of RS for diabetes control should be explored in more detailed perspectives for a better understanding.
All authors listed have made a substantial, direct, and intellectual contribution to the work and approved it for publication.
The authors declare that the research was conducted in the absence of any commercial or financial relationships that could be construed as a potential conflict of interest.
All claims expressed in this article are solely those of the authors and do not necessarily represent those of their affiliated organizations, or those of the publisher, the editors and the reviewers. Any product that may be evaluated in this article, or claim that may be made by its manufacturer, is not guaranteed or endorsed by the publisher.
The authors thank the Director General of Health Malaysia and the Director of Institute for Medical Research (IMR), Malaysia, for giving the permission to publish this article. We also thank the staff of Nutrition, Metabolism and Cardiovascular Center, Institute for Medical Research, NIH for their continuous support.
Aβ, Amyloid-beta; AD, Adzuki bean; ACh, Acetylcholine; AChE, Acetylcholinesterase; AD, Alzheimer's disease; AFM, Atomic Force Microscope; BMI, Body mass index; BR, Waxy black rice; CHO, Carbohydrate; CRP, C-reactive protein; CSIRO, Commonwealth Scientific and Industrial Research Organization; ELD, Elderly; FFA, Free Fatty acid; FPG, Fasting Plasma Glucose; FSIVGTT, Frequently sampled intravenous glucose tolerance test; GI, Glycemic index; GLP1, Glucagon-like peptide-1; IFG, Impaired fasting glucose; IGT, Impaired glucose tolerance; iAUC, Incremental area under the curve; IR, Insulin resistance; IL, Interleukin; IN, Inulin; HbA1c, Glycated hemoglobin; HAM, High-amylose maize; HAW, High-amylose wheat; HDL, High-density lipoprotein; HFWR, High fiber white rice; hs, High-sensitive; LAW, Low-amylose wheat; LB, Lily bulb; LDL, Low-density lipoprotein; LN, Lean; LS, Lotus seed; MCP-1, Monocyte chemotactic protein-1; MI, Millet; MID, Mid-age; MISI, Muscle insulin sensitivity index; MTT, Meal tolerance test; NEFA, Non-esterified fatty acids; OGTT, Oral glucose tolerance test; OWO, Overweight or obese; PAI-1, Plasminogen activator inhibitor-1; PYY, Peptide YY; RB, Rye-based bread; RDS, Rapidly digestible starch; RFB, Rye flour bread; RKB, Rye kernels bread; RS, Resistant starch; SCFA, Serum short-chain FA; SDS, slowly digestible starch; TC, Total cholesterol; TEE, Total energy expenditure; TG, Triglycerides; T2DM, Type 2 diabetes; sVCAM-1, Soluble vascular cell adhesion molecule −1; WG, Whole grain rye; WR, White rice; WWB, Wheat flour bread; 2hPG, 2-h plasma glucose.
1. Goldenberg R, Punthakee Z. Definition, classification and diagnosis of diabetes, prediabetes and metabolic syndrome. Can J Diab. (2013) 37:S8–11. doi: 10.1016/j.jcjd.2013.01.011
2. Colagiuri S, Lee CM, Wong TY, Balkau B, Shaw JE, Borch-Johnsen K, et al. Glycemic thresholds for diabetes-specific retinopathy: implications for diagnostic criteria for diabetes. Diab Care. (2011) 34:145–50. doi: 10.2337/dc10-1206
3. Ito C. Evidence for diabetes mellitus criteria in 2010 using HbA1c. Diabetol Int. (2013) 4:9–15. doi: 10.1007/s13340-012-0086-7
4. Kowall B, Rathmann W. HbA1c for diagnosis of type 2 diabetes. Is there an optimal cut point to assess high risk of diabetes complications, and how well does the 6.5% cutoff perform? Diab Metab Syndrome Obes. (2013) 6:477. doi: 10.2147/DMSO.S39093
5. Huang Y, Cai X, Mai W, Li M, Hu Y. Association between prediabetes and risk of cardiovascular disease and all cause mortality: systematic review and meta-analysis. BMJ. (2016) 355. doi: 10.1136/bmj.i5953
6. Heianza Y, Arase Y, Fujihara K, Tsuji H, Saito K, Hsieh S, et al. Screening for pre-diabetes to predict future diabetes using various cut-off points for HbA1c and impaired fasting glucose: the Toranomon Hospital Health Management Center Study 4 (TOPICS 4). Diab Med. (2012) 29:e279–85. doi: 10.1111/j.1464-5491.2012.03686.x
7. Association AD. Diagnosis and classification of diabetes mellitus. Diab Care. (2013) 36(Suppl. 1):S67. doi: 10.2337/dc13-S067
8. Khan MAB, Hashim MJ, King JK, Govender RD, Mustafa H, Al Kaabi J. Epidemiology of type 2 diabetes–global burden of disease and forecasted trends. J Epidemiol Glob Health. (2020) 10:107. doi: 10.2991/jegh.k.191028.001
9. Lin X, Xu Y, Pan X, Xu J, Ding Y, Sun X, et al. Global, regional, and national burden and trend of diabetes in 195 countries and territories: an analysis from 1990 to 2025. Sci Rep. (2020) 10:1–11. doi: 10.1038/s41598-020-71908-9
10. WHO. Diet, Nutrition and Prevention of Chronic Diseases. Geneva: World Health Organ Tech Rep Ser (2002).
11. Gentile CL, Ward E, Holst JJ, Astrup A, Ormsbee MJ, Connelly S, et al. Resistant starch and protein intake enhances fat oxidation and feelings of fullness in lean and overweight/obese women. Nutr J. (2015) 14:1–10. doi: 10.1186/s12937-015-0104-2
12. Priebe M, van Binsbergen J, de Vos R, Vonk RJ. Whole grain foods for the prevention of type 2 diabetes mellitus. Cochrane Database Syst Rev. (2008) CD006061. doi: 10.1002/14651858.CD006061.pub2
13. Meenu M, Xu B. A critical review on anti-diabetic and anti-obesity effects of dietary resistant starch. Crit Rev Food Sci Nutr. (2019) 59:3019–31. doi: 10.1080/10408398.2018.1481360
14. Englyst HN, Kingman SM, Cummings J. Classification and measurement of nutritionally important starch fractions. Eur J Clin Nutr. (1992) 46:S33–50.
15. Tharanathan RN. Food-derived carbohydrates—structural complexity and functional diversity. Crit Rev Biotechnol. (2002) 22:65–84. doi: 10.1080/07388550290789469
16. Sánchez-Zapata E, Viuda-Martos M, Fernández-López J, Pérez-Alvarez J. Resistant starch as functional ingredient. Polysacch Bioactivity Biotechnol. (2015) 43:1911–31. doi: 10.1007/978-3-319-16298-0_34
17. Information NCfB. PubChem Compound Summary for CID 53477911, 1,4-beta-D-Glucan. (2021). Available online at: https://pubchem.ncbi.nlm.nih.gov/compound/1_4-beta-D-Glucan (accessed June 4, 2021).
18. Mikulíková D, Masár Š, Kraic J. Biodiversity of legume health-promoting starch. Starch Stärke. (2008) 60:426–432. doi: 10.1002/star.200700693
19. Gao C, Rao M, Huang W, Wan Q, Yan P, Long Y, et al. Resistant starch ameliorated insulin resistant in patients of type 2 diabetes with obesity: a systematic review and meta-analysis. Lipids Health Dis. (2019) 18:205. doi: 10.1186/s12944-019-1127-z
20. Maziarz MP. Role of fructans and resistant starch in diabetes care. Diab Spectrum. (2013) 26:35–9. doi: 10.2337/diaspect.26.1.35
21. Nugent AP. Health properties of resistant starch. Nutr Bull. (2005) 30:27–54. doi: 10.1111/j.1467-3010.2005.00481.x
22. Hasjim J, Ai Y, Jane J-l. Novel applications of amylose-lipid complex as resistant starch type 5. In: Shi Y-C, Maningat CC, . Resistant Starch. (2013) 79–94. doi: 10.1002/9781118528723.ch04
23. Perez-Alvarez J, López J. Overview of meat products as functional foods. Technol Strat Funct Meat Prod Dev. (2008) 1–17.
24. Carlson JJ, Eisenmann JC, Norman GJ, Ortiz KA, Young PC. Dietary fiber and nutrient density are inversely associated with the metabolic syndrome in US adolescents. J Am Dietetic Assoc. (2011) 111:1688–95. doi: 10.1016/j.jada.2011.08.008
25. Heijnen M, Van Amelsvoort J, Deurenberg P, Beynen AC. Limited effect of consumption of uncooked (RS2) or retrograded (RS3) resistant starch on putative risk factors for colon cancer in healthy men. Am J Clin Nutr. (1998) 67:322–31. doi: 10.1093/ajcn/67.2.322
26. Bodinham CL, Smith L, Wright J, Frost GS, Robertson MD. Dietary fibre improves first-phase insulin secretion in overweight individuals. PLoS ONE. (2012) 7:e40834. doi: 10.1371/journal.pone.0040834
27. Bodinham C, Smith L, Thomas EL, Bell JD, Swann JR, Costabile A, et al. Efficacy of increased resistant starch consumption in human type 2 diabetes. Endocr Connect. (2014) 3:75–84. doi: 10.1530/EC-14-0036
28. Dainty SA, Klingel SL, Pilkey SE, McDonald E, McKeown B, Emes MJ, et al. Resistant starch bagels reduce fasting and postprandial insulin in adults at risk of type 2 diabetes. J Nutr. (2016) 146:2252–9. doi: 10.3945/jn.116.239418
29. Peterson CM, Beyl RA, Marlatt KL, Martin CK, Aryana KJ, Marco ML, et al. Effect of 12 wk of resistant starch supplementation on cardiometabolic risk factors in adults with prediabetes: a randomized controlled trial. Am J Clin Nutr. (2018) 108:492–501. doi: 10.1093/ajcn/nqy121
30. Kwak JH, Paik JK, Kim HI, Kim OY, Shin DY, Kim H-J, et al. Dietary treatment with rice containing resistant starch improves markers of endothelial function with reduction of postprandial blood glucose and oxidative stress in patients with prediabetes or newly diagnosed type 2 diabetes. Atherosclerosis. (2012) 224:457–64. doi: 10.1016/j.atherosclerosis.2012.08.003
31. Lotfollahi Z, Mello APQ, Costa ES, Oliveira CLP, Damasceno NRT, Izar MC, et al. Green-banana biomass consumption by diabetic patients improves plasma low-density lipoprotein particle functionality. Sci Rep. (2020) 10:12269. doi: 10.1038/s41598-020-69288-1
32. Gargari BP, Namazi N, Khalili M, Sarmadi B, Jafarabadi MA, Dehghan P. Is there any place for resistant starch, as alimentary prebiotic, for patients with type 2 diabetes? Compl Ther Med. (2015) 23:810–5. doi: 10.1016/j.ctim.2015.09.005
33. Alfa MJ, Strang D, Tappia PS, Olson N, DeGagne P, Bray D, et al. A randomized placebo controlled clinical trial to determine the impact of digestion resistant starch MSPrebiotic® on glucose, insulin, and insulin resistance in elderly and mid-age adults. Front Med. (2018) 4:260. doi: 10.3389/fmed.2017.00260
34. Giles ED, Brown IL, MacLean PS, Pan Z, Melanson EL, Heard KJ, et al. The in vivo net energy content of resistant starch and its effect on macronutrient oxidation in healthy adults. Nutrients. (2019) 11:2484. doi: 10.3390/nu11102484
35. Belobrajdic DP, Regina A, Klingner B, Zajac I, Chapron S, Berbezy P, et al. High-amylose wheat lowers the postprandial glycemic response to bread in healthy adults: a randomized controlled crossover trial. J Nutr. (2019) 149:1335–45. doi: 10.1093/jn/nxz067
36. Hallström E, Sestili F, Lafiandra D, Björck I, Östman E. A novel wheat variety with elevated content of amylose increases resistant starch formation and may beneficially influence glycaemia in healthy subjects. Food Nutr Res. (2011) 55:7074. doi: 10.3402/fnr.v55i0.7074
37. Poquette NM, Gu X, Lee S-O. Grain sorghum muffin reduces glucose and insulin responses in men. Food Funct. (2014) 5:894–899. doi: 10.1039/C3FO60432B
38. Gu X. Effects of Grain Sorghum Muffin on Blood Glucose and Insulin Responses in Prediabetic Men. Fayetteville: University of Arkansas (2014).
39. Lin C-H, Chang D-M, Wu D-J, Peng H-Y, Chuang L-M. Assessment of blood glucose regulation and safety of resistant starch formula-based diet in healthy normal and subjects with type 2 diabetes. Medicine. (2015) 94:e1332. doi: 10.1097/MD.0000000000001332
40. Sanders L, Dicklin M, Palacios O, Maki C, Wilcox M, Maki K. Effects of potato resistant starch intake on insulin sensitivity, related metabolic markers and appetite ratings in men and women at risk for type 2 diabetes: a pilot cross-over randomised controlled trial. J Hum Nutr Dietetics. (2021) 34:94–105. doi: 10.1111/jhn.12822
41. Mohan V, Anjana RM, Gayathri R, Ramya Bai M, Lakshmipriya N, Ruchi V, et al. Glycemic index of a novel high-fiber white rice variety developed in India—A randomized control trial study. Diab Technol Ther. (2016) 18:164–70. doi: 10.1089/dia.2015.0313
42. Nomura N, Miyoshi T, Hamada Y, Kitazono E. Glycemic index of boiled BARLEYmax® in healthy Japanese subjects. J Cereal Sci. (2020) 93:102959. doi: 10.1016/j.jcs.2020.102959
43. Zhu R, Fan Z, Han Y, Li S, Li G, Wang L, et al. Acute effects of three cooked non-cereal starchy foods on postprandial glycemic responses and in vitro carbohydrate digestion in comparison with whole grains: a randomized trial. Nutrients. (2019) 11:634. doi: 10.3390/nu11030634
44. Yulianto W, Suryani C, Susiati A, Luwihana S. Evaluation of chromium fortified-parboiled rice coated with herbal extracts: resistant starch and glycemic index. Int Food Res J. (2018) 25:2608–13.
45. Sandberg JC, Björck IM, Nilsson AC. Effects of whole grain rye, with and without resistant starch type 2 supplementation, on glucose tolerance, gut hormones, inflammation and appetite regulation in an 11–14.5 hour perspective; a randomized controlled study in healthy subjects. Nutr J. (2017) 16:1–11. doi: 10.1186/s12937-017-0246-5
46. Sandberg JC, Björck IME, Nilsson AC. Impact of rye-based evening meals on cognitive functions, mood and cardiometabolic risk factors: a randomized controlled study in healthy middle-aged subjects. Nutr J. (2018) 17:102. doi: 10.1186/s12937-018-0412-4
47. Nichenametla SN, Weidauer LA, Wey HE, Beare TM, Specker BL, Dey M. Resistant starch type 4-enriched diet lowered blood cholesterols and improved body composition in a double blind controlled cross-over intervention. Mol Nutr Food Res. (2014) 58:1365–9. doi: 10.1002/mnfr.201300829
48. García MDM, García-Rodríguez CE, de la Cruz Rico M, Aguilera CM, Pérez-Rodríguez M, Pérez-de-la-Cruz AJ, et al. A new fructose-free, resistant-starch type IV-enriched enteral formula improves glycaemic control and cardiovascular risk biomarkers when administered for six weeks to elderly diabetic patients. Nutricion Hospital. (2017) 34:73–80. doi: 10.20960/nh.978
49. Rahat-Rozenbloom S, Fernandes J, Cheng J, Gloor GB, Wolever TM. The acute effects of inulin and resistant starch on postprandial serum short-chain fatty acids and second-meal glycemic response in lean and overweight humans. Eur J Clin Nutr. (2017) 71:227–33. doi: 10.1038/ejcn.2016.248
50. Murphy MM, Douglass JS, Birkett A. Resistant starch intakes in the United States. J= Am Dietetic Assoc. (2008) 108:67–78. doi: 10.1016/j.jada.2007.10.012
51. Wahren J, Ekberg K, Johansson J, Henriksson M, Pramanik A, Johansson B-L, et al. Role of C-peptide in human physiology. Am J Physiol Endocrinol Metab. (2000) 278:E759–68. doi: 10.1152/ajpendo.2000.278.5.E759
52. Kahn SE, Porte D Insulin secretion in the normal and diabetic human. In: Alberti KGMM ZP, Defronzo RA, Keen H, editor. International Textbook of Diabetes Mellitus. New York, NY: John Wiley & Sons (1997). p. 337–54.
53. Association AD. Postprandial blood glucose. Diabetes Care. (2001) 24:775–8. doi: 10.2337/diacare.24.4.775
54. Woerle HJ, Neumann C, Zschau S, Tenner S, Irsigler A, Schirra J, et al. Impact of fasting and postprandial glycemia on overall glycemic control in type 2 diabetes: importance of postprandial glycemia to achieve target HbA1c levels. Diab Res Clin Pract. (2007) 77:280–5. doi: 10.1016/j.diabres.2006.11.011
55. Schirra J, Wank U, Arnold R, Göke B, Katschinski M. Effects of glucagon-like peptide-1 (7–36) amide on motility and sensation of the proximal stomach in humans. Gut. (2002) 50:341–8. doi: 10.1136/gut.50.3.341
56. Meier JJ, Gallwitz B, Salmen S, Goetze O, Holst JJ, Schmidt WE, et al. Normalization of glucose concentrations and deceleration of gastric emptying after solid meals during intravenous glucagon-like peptide 1 in patients with type 2 diabetes. J Clin Endocrinol Metab. (2003) 88:2719–25. doi: 10.1210/jc.2003-030049
57. Shah M, Vella A. Effects of GLP-1 on appetite and weight. Rev Endocr Metab Disord. (2014) 15:181–7. doi: 10.1007/s11154-014-9289-5
59. Galarregui C, Navas-Carretero S, Gonzalez-Navarro CJ, Martinez JA, Zulet MA, Abete I. Both macronutrient food composition and fasting insulin resistance affect postprandial glycemic responses in senior subjects. Food Funct. (2021) 12:6540–8. doi: 10.1039/D1FO00731A
60. Zhao H, Huang X, Jiao J, Zhang H, Liu J, Qin W, et al. Protein phosphatase 4 (PP4) functions as a critical regulator in tumor necrosis factor (TNF)-α-induced hepatic insulin resistance. Sci Rep. (2015) 5:18093. doi: 10.1038/srep18093
61. Correia ML, Haynes WG. A role for plasminogen activator inhibitor-1 in obesity: from pie to PAI? Arterioscler Thromb Vasc Biol. (2006) 26:2183–5. doi: 10.1161/01.ATV.0000244018.24120.70
62. Palau-Rodriguez M, Tulipani S, Isabel Queipo-Ortuño M, Urpi-Sarda M, Tinahones FJ, Andres-Lacueva C. Metabolomic insights into the intricate gut microbial–host interaction in the development of obesity and type 2 diabetes. Front Microbiol. (2015) 6:1151. doi: 10.3389/fmicb.2015.01151
63. Sami W, Ansari T, Butt NS, Ab Hamid MR. Effect of diet on type 2 diabetes mellitus: a review. Int J Health Sci. (2017) 11:65.
64. Colantonio AG, Werner SL, Brown M. The effects of prebiotics and substances with prebiotic properties on metabolic and inflammatory biomarkers in individuals with type 2 diabetes mellitus: a systematic review. J Acad Nutr Dietetics. (2020) 120:587–607.e2. doi: 10.1016/j.jand.2018.12.013
65. Holst JJ, Deacon CF. Is there a place for incretin therapies in obesity and prediabetes? Trends Endocrinol Metab. (2013) 24:145–52. doi: 10.1016/j.tem.2013.01.004
66. González Hernández MA, Canfora EE, Jocken JW, Blaak EE. The short-chain fatty acid acetate in body weight control and insulin sensitivity. Nutrients. (2019) 11:1943. doi: 10.3390/nu11081943
67. Azam A, Itrat N, Ahmed U. Hypoglycemic effect of barley (Hordeum vulgare) in diabetics. Group. (2019) 37:165.8–3.67.
Keywords: resistant starch, type 2 diabetes, biomarkers, glucose, insulin
Citation: Rashed AA, Saparuddin F, Rathi D-NG, Nasir NNM and Lokman EF (2022) Effects of Resistant Starch Interventions on Metabolic Biomarkers in Pre-Diabetes and Diabetes Adults. Front. Nutr. 8:793414. doi: 10.3389/fnut.2021.793414
Received: 12 October 2021; Accepted: 15 December 2021;
Published: 12 January 2022.
Edited by:
Alexandru Rusu, Biozoon Food Innovations GmbH, GermanyReviewed by:
Shaun Sabico, King Saud University, Saudi ArabiaCopyright © 2022 Rashed, Saparuddin, Rathi, Nasir and Lokman. This is an open-access article distributed under the terms of the Creative Commons Attribution License (CC BY). The use, distribution or reproduction in other forums is permitted, provided the original author(s) and the copyright owner(s) are credited and that the original publication in this journal is cited, in accordance with accepted academic practice. No use, distribution or reproduction is permitted which does not comply with these terms.
*Correspondence: Aswir Abd Rashed, YXN3aXJAbW9oLmdvdi5teQ==
Disclaimer: All claims expressed in this article are solely those of the authors and do not necessarily represent those of their affiliated organizations, or those of the publisher, the editors and the reviewers. Any product that may be evaluated in this article or claim that may be made by its manufacturer is not guaranteed or endorsed by the publisher.
Research integrity at Frontiers
Learn more about the work of our research integrity team to safeguard the quality of each article we publish.