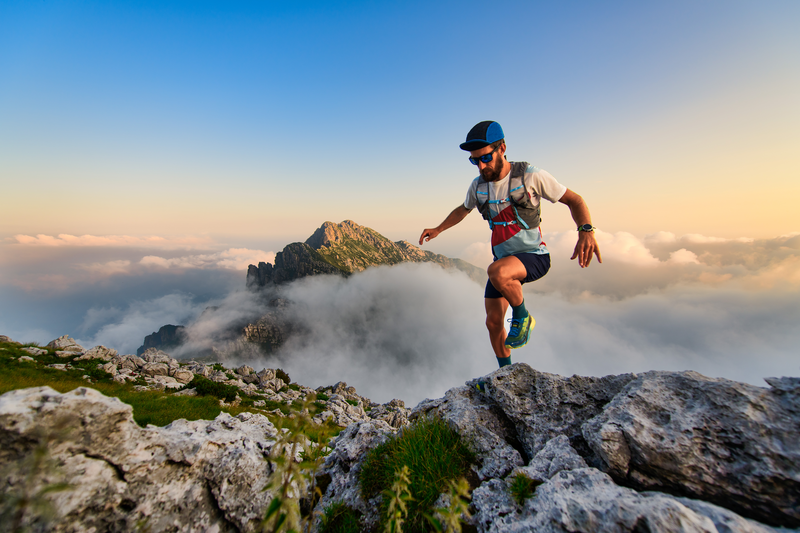
94% of researchers rate our articles as excellent or good
Learn more about the work of our research integrity team to safeguard the quality of each article we publish.
Find out more
ORIGINAL RESEARCH article
Front. Nutr. , 03 December 2021
Sec. Nutrition and Sustainable Diets
Volume 8 - 2021 | https://doi.org/10.3389/fnut.2021.792941
This article is part of the Research Topic Insects: a Source of Safe and Sustainable Food? View all 6 articles
Background: Undernutrition is a prevalent, serious, and growing concern, particularly in developing countries. Entomophagy—the human consumption of edible insects, is a historical and culturally established practice in many regions. Increasing consumption of nutritious insect meal is a possible combative strategy and can promote sustainable food security. However, the nutritional literature frequently lacks consensus, with interspecific differences in the nutrient content of edible insects generally being poorly resolved.
Aims and methods: Here we present full proximate and fatty acid profiles for five edible insect species of socio-economic importance in West Africa: Hermetia illucens (black soldier fly), Musca domestica (house fly), Rhynchophorus phoenicis (African palm weevil), Cirina butyrospermi (shea tree caterpillar), and Macrotermes bellicosus (African termite). These original profiles, which can be used in future research, are combined with literature-derived proximate, fatty acid, and amino acid profiles to analyse interspecific differences in nutrient content.
Results: Interspecific differences in ash (minerals), crude protein, and crude fat contents were substantial. Highest ash content was found in H. illucens and M. domestica (~10 and 7.5% of dry matter, respectively), highest crude protein was found in C. butyrospermi and M. domestica (~60% of dry matter), whilst highest crude fat was found in R. phoenicis (~55% of dry matter). The fatty acid profile of H. illucens was differentiated from the other four species, forming its own cluster in a principal component analysis characterized by high saturated fatty acid content. Cirina butyrospermi had by far the highest poly-unsaturated fatty acid content at around 35% of its total fatty acids, with α-linolenic acid particularly represented. Amino acid analyses revealed that all five species sufficiently met human essential amino acid requirements, although C. butyrospermi was slightly limited in leucine and methionine content.
Discussion: The nutritional profiles of these five edible insect species compare favorably to beef and can meet human requirements, promoting entomophagy's utility in combatting undernutrition. In particular, C. butyrospermi may provide a source of essential poly-unsaturated fatty acids, bringing many health benefits. This, along with its high protein content, indicates that this species is worthy of more attention in the nutritional literature, which has thus-far been lacking.
Two prominent issues facing global development are that of widespread undernutrition and poverty (1). A recent report by the Food and Agriculture Organization (2) estimated the number of people globally experiencing severe food insecurity at 750 million, a number which rises to two billion when moderate food insecurity is considered, with over 20% of children under five showing stunting. These issues particularly affect low-income countries, where nutritious diets are widely unaffordable and staple foods, often of low nutritional value, are relied upon (2). Food insecurity can have devastating health impacts, with undernutrition thought to be the leading cause of child mortality (3). Furthermore, early-life undernutrition has been linked to later-life issues, including hypertension, diabetes, and cardiovascular disease (3–5). Food insecurity has even been implicated in reducing immune function, increasing non-communicable disease burden, and altering gut microbiome (6, 7). The economic burden of diet-related mortality and non-communicable disease is expected to exceed 1.3 trillion dollars annually by 2030 (2).
Global levels of food insecurity are expected to increase further, a trend already seen since 2014, particularly in the Caribbean and Latin America, adding to already high levels in Africa and South Asia (2). The world population is predicted to rise by a quarter by 2050 (8), with the population of Sub-Saharan Africa expected to double in the same timeframe, exacerbating prevalent undernutrition there. As well as a larger population, per capita meat demand is rising in many parts of the world (9). This is particularly prominent in regions of highest economic growth, rather than in low-income countries, with the relationship between affluence and meat consumption well-documented (9, 10). The combination of rising populations and affluence are intensifying food insecurity issues in low-income countries (9); identifying alternative, more sustainable protein sources is vital in combatting rising undernutrition and achieving sustainable food security.
Entomophagy, or the consumption of insects by humans, has substantial potential in combatting these issues and is widely practiced globally, particularly within traditional communities (11). Despite common misconceptions, communities practicing entomophagy widely regard insects as a delicacy rather than a “hardship” food in times of starvation (12); for instance, locusts are thought to have been popular among the social elite of the ancient Near East (13). It is becoming increasingly clear that entomophagy has played a pivotal role in providing nutrition throughout hominin evolution, with insect species being identified in human coprolites and some researchers even suggesting a homology between insectivory in human and non-human primates (14). A huge diversity of edible insect species are known (15–17), with over 2,000 species formally listed (18). The prevalence of entomophagy, as well as the species eaten, is highly geographically variable. For example, at least 250 species have been identified in diets in Africa across a diverse array of insect orders, the most speciose of which are Lepidoptera, Orthoptera, and Coleoptera (17, 19, 20). Similarly, at least 55 species have been identified in Japan, where Orthoptera and Hymenoptera are particularly popular (21), whilst 164 species have been identified across Lao People's Democratic Republic, Myanmar, Thailand, and Vietnam (22).
The benefits of edible insects in achieving sustainable food security are many. They are widely regarded to constitute a highly nutritious foodstuff. Although variable between insect species (23, 24), the high protein and fat content of edible insects compares favorably to meat and fish (12, 24–27). Furthermore, the amino acid profiles of several species have been demonstrated to contain a high proportion of essential amino acids; this was shown in Imbrasia belina, Oryctes rhinoceros, Rhynchophorus phoenicis, and Macrotermes bellicosus, all of which are popular in Africa (26), as well as in Hermetia illucens and Musca domestica (25, 28, 29). Several species of popular edible insects, including R. phoenicis and M. bellicosus, have been shown to compensate for certain limiting amino acids in cereal- and staple-based diets (23, 26). Furthermore, the fatty acid profiles of many edible insects compare favorably with meat and fish (23, 24). For example, Hussein et al. (29) identified a high content of unsaturated, particularly monounsaturated, fatty acids in M. domestica larvae. Similarly, Womeni et al. (27) found a high proportion of polyunsaturated fatty acids in R. phoenicis, Homorocoryphus nitidulus, and Zonocerus variegates, with particular representation of linoleic and α-linolenic acid, omega-6 and omega-3 fatty acids essential in development (24). This could compensate for the lacking representations of these essential fatty acids in staple vegetable oils (27). Edible insects also present a promising source of micronutrients (12). Iron and zinc deficiencies are prevalent and dangerous, particularly in developing countries (30, 31); entomophagy could provide a combative strategy, with iron and zinc levels shown to exceed that of beef in several edible insect species (24).
Not only are they nutritious but edible insects also provide a more efficient, cheaper, and less environmentally damaging nutrient source than vertebrate livestock species. As insects are cold-blooded invertebrates, they are highly efficient in converting input feed to edible matter (12, 15, 24); estimates suggest that around 25 kilograms of feed are required per kilogram of edible beef, yet this reduces to around two kilograms for edible insects (23, 32), thus insect rearing exerts far lower land and environmental pressure. Insects also emit far lower levels of greenhouse gases and ammonia than cattle and swine livestock (12, 23, 24, 33) and can be raised on organic waste substrates, recycling these in the process reducing feedstock demand relative to other agro-species. Using organic waste substrates can reduce waste volume and convert this into nutritious insect meal and useful co-products such as fertilizers and soil conditioners (34). Diener et al. (35) documented a 70% reduction in organic waste matter by H. illucens larvae, whilst Sheppard et al. (36) showed over a 50% reduction in manure waste, again with H. illucens larvae. Similar outcomes have been seen with other species, including M. domestica (23, 24).
The extensive benefits of edible insects and entomophagy cannot all be described here. Briefly, insect farming is also thought to pose far lower risk of agro-zoonosis emergence due to high human-insect taxonomic distance relative to vertebrate species (12, 23, 24). For example, one theory for the emergence of SARS-Cov-2 in humans is a cross-species transmission event at a meat market (37), our susceptibility to which may have been reduced were a greater emphasis put on agro-insect species. Furthermore, post-raising, edible insects can be feedstock to a variety of processes rather than being limited to human consumption; this includes medicines (38–41), biodiesel (42, 43), and feed for other agro-species, including poultry and fish (44–49). Finally, due to the small capital investment and expertise requirements of insect farming, and the high profit potential (50), insect rearing can provide livelihoods to the poorest individuals and communities (12). As a result of these clear benefits, there has been recent interest in the semi-cultivation and domestication of edible insects into mini-livestock (24, 51). This interest is emphasized by predictions that the edible insect market will value over six billion dollars by 2030 (52).
Despite these huge benefits in combatting undernutrition and promoting sustainable development, there has been a recent decrease in the prevalence of ento-ethno practices across traditional communities (53), where the benefits could be highest. This has generally been attributed to three main factors. Firstly, many communities are thought to be abandoning their traditions and are becoming increasingly westernized in their customs (53). DeFoliart (54) condemns western aversion to entomophagy and argues that it is a detriment to the prevalence of traditional uses of edible insects. Secondly, many argue that historical and recent edible insect over-exploitation has led to reduced supply for entomophagy (53). For example, Ramos-Elorduy (55) reports a decrease in the populations of several edible insect species in Mexico through over-exploitation. Finally, continued use of agricultural pesticides has been widely implicated in reducing edible insect populations and harvests (56, 57). Therefore, we must act quickly to conserve traditional knowledge of the prevalence, benefits, and potential of edible insect usage before it is lost. This includes documenting the traditional uses of edible insects in communities globally (53), assessing their nutritional and chemical value (12, 24, 53), and quantifying the extent to which they can meet the desired nutrition and sustainable development goals (53). There has also been a recent emphasis on research for promoting large-scale edible insect farming, including the innovation of mass-production technologies, development of international ento-ethno legislature, and education of new consumers (12, 24).
Recent strides have been made to nutritionally profile a variety of edible insect species and the number of entomophagy-related publications annually has increased from <10 pre-2012 to 114 in 2020. The general consensus is that edible insects are of high nutritional value and can potentially constitute an effective alternative feedstuff to meat and fish (12, 24, 58, 59). However, it is also widely accepted that edible insect nutrient profiles have major interspecific differences. For example, Rumpold and Schluter (59) identified a protein content range of 8.85–71.1% on a dry matter basis within Coleoptera. Similarly, high differences in protein, lipid, and ash, an indicator of mineral content (60), as well as in amino acid profiles, have been reported between R. phoenicis, Z. variegatus, and M. bellicosus (27, 61). Not only does interspecific variation exist but there is also within-species variation, including between different life stages, populations, and diets (23, 59, 62). H. illucens larvae reared on different substrates have been shown to diverge in nutrient profile, in particular in fatty acids, introducing the potential of modulating edible insect nutritional provision through substrate modification (63–65). More data is required to resolve this nutritional variation and fully elucidate the nutritional potential of insects (59). There are also growing concerns that some nutritional research is methodologically inconsistent and of low quality and yet is used to justify entomophagy's health benefits (66). Furthermore, the nutritional literature can often be taxonomically restricted and biased, with much emphasis on species of commercial or economic importance to major rearing companies rather than those of socio-economic or health benefit to traditional communities. For example, 25 times as many papers are found featuring “Tenebrio nutrition” than for “Cirina nutrition.” Tenebrio is a genus containing the yellow mealworm which is commercially raised for the pet food industry whereas Cirina is a genus of moth popular for consumption in Africa. Therefore, the nutritional literature must expand in order to maximize the health, social, livelihood, and sustainability benefits of entomophagy.
Here we analyzed proximate and fatty acid profiles of five species of edible insect of socio-economic importance in West Africa, a region with an emerging profile in ento-ethno research. These species, and the abbreviation used to refer to them in tables and figures, include Hermetia illucens (black soldier fly; BSF), Musca domestica (house fly; HSF), Rhynchophorus phoenicis (African palm weevil; PL), Cirina butyrospermi (shea tree caterpillar; STC), and Macrotermes bellicosus (African termite; TM). These nutritional profiles, which can be used in future research, are here combined with proximate, fatty acid, and also amino acid data present in the literature for these five species in order to rigorously assess interspecific nutrient differences. Through combining original and literature data, we provide an improved consensus of the nutritional offering of these five important species which have highest potential in combatting undernutrition and promoting sustainable development in Africa.
Larvae of Hermetia illucens, Musca domestica, Rhynchophorus phoenicis, Cirina butyrospermi and adults of Macrotermes bellicosus were obtained from the field in Ghana through sampling.
Samples were inactivated by putting them in 70% alcohol and dried by freezing in liquid nitrogen before being milled to flour.
The five insect meal flours were analyzed for proximate and fatty acid content at the Chemical Laboratory of the International Center for Insect Physiology and Ecology (icipe) in Nairobi, Kenya.
Dried and milled H. illucens, M. domestica, R. phoenicis, and C. butyrospermi samples were assessed for proximate content using analytical procedures outlined by the Association of Official Analytical Chemists (67). Briefly, dry matter was assessed by oven drying method at 105°C for 24 h (67). Ash content, generally used as an indicator of the mineral content of foods (60), was determined by overnight incineration at 550°C, whilst organic matter was evaluated by subtracting this ash content from 100. Total nitrogen in the samples was assessed using the Kjeldahl method, with the crude protein content determined by multiplying total nitrogen by a conversion factor of 6.25. Crude fat content was evaluated using a Velp solvent extractor (SER 148/6; Velp Scientifica, Italy) with an ethyl ether extractant. Finally, neutral detergent fiber and acid detergent fiber were analyzed using a Velp fiber analyzer (FIWE 6; Velp Scientifica, Italy) and reagents described by Van Soest et al. (68).
All proximate measures, other than dry matter, were evaluated on a % dry matter basis; dry matter, organic matter, and ash content were determined in triplicate whilst all other proximate nutrients were in duplicate, except for M. domestica in which all proximate measures were evaluated in duplicate. All replication analyses were carried out using different batches of insect samples.
Fatty acid profiles were produced for each of the five species of edible insect according to the methods of Cheseto et al. (69) and Christie (70). Briefly, fatty acids were analyzed following conversion to their corresponding methyl esters; esterification was conducted by adding a solution of sodium methoxide in dry ethanol to each sample followed by extraction with GC-grade hexane. Fatty acid methyl esters were then analyzed by GC-MS using a 7950A gas chromatograph system with a 5975C mass selective detector (Agilent Technologies Inc., CA, USA) and HP-5 ms capillary column (length: 30 m, internal diameter: 0.25 mm, film thickness: 0.25 μm; Agilent Technologies Inc., CA, USA). An oven temperature programme of 35°C (5 min) increasing at 10°C per min to 285°C (20.4 min) was used, whilst a constant detector temperature of 230°C and 180°C for the ion source and quadrupole was conserved. The carrier gas was helium (1.25 ml per min flow rate). An acceleration energy of 70 eV was used to obtain electron impact mass spectra, with a 40–550 m/z mass range used for fragment ion analysis, having set a 3.3-min delay time for the filament. Fatty acid methyl ester identification was done by comparing fragmentation patterns and retention times to known fatty acid methyl ester standards where available and library-MS database reference spectra. Standard methyl octadecanoate of known concentrations (0.2–125 ng/μL) was subsequently analyzed under the same GC-MS conditions to obtain a linear calibration curve [y = 7E + 06x – 4E + 07 (R2 = 0.9757)] that was used for external quantification of fatty acid methyl esters.
Fatty acid profiles for each species were evaluated in triplicate, except for M. domestica and M. bellicosus which were both performed in duplicate, and are presented on a % total fatty acid basis.
In order to evaluate interspecific differences in proximate and fatty acid profiles the nutritional literature was rigorously searched and published nutritional profiles were combined with the profiles produced in this study. Although not evaluated originally for each species here, amino acid profiles were also extracted from the literature to assess interspecific differences. The following Google Scholar search string was employed to identify papers, published between 2000 and 2021, concerning the nutritive content of the five species analyzed here:
“Nutrition AND Edible AND (Entomophagy OR Proximate OR “Fatty Acid” OR “Amino Acid”) (“Species Binomial Name”)—(“Literature Review”)—(“Meta-analysis”)”
The resultant papers were then briefly read and subjected to the following inclusion criteria to identify relevant publications before being read in detail:
• The paper is an original article and has been through a peer review process, rather than being a review/meta-analysis, which uses external data, or a thesis/preprint.
• The paper concerns one or more of the relevant species (H. illucens, M. domestica, R. phoenicis, C. butyrospermi, or M. bellicosus).
• The paper specifically measures species nutritive content rather than addressing some other aspect of insect physiology, ecology, or evolution.
Due to the literature search being sorted by relevance to the search string and the high number of returned papers for certain species, with the BSF and HSF searches returning 1,380 and 1,060 papers, respectively, the search was terminated if 50 papers had elapsed since the last relevant one was identified. Relevant papers were then read in detail and subjected to the following further inclusion criteria before the data was extracted:
• The paper nutritionally profiles the desired life-stage of each species. For the species analyzed, the desired life-stages were as follows: larvae for H. illucens, M. domestica, R. phoenicis, and C. butyrospermi; adult for M. bellicosus.
• The paper nutritionally profiles the unadulterated insect sample, including no mixing with external ingredients, no cooking, and no defatting.
• The paper follows standard methods where possible, with no inappropriate sample preparation.
• The paper reports nutritional results in a form consistent with the wider literature and the nutritional analyses presented here. This included units of % dry matter, % total fatty acids, and g/100 g crude protein for proximate, fatty acid, and amino acid profiles, respectively.
For each paper satisfying all of the above criteria, proximate, fatty acid, and amino acid data was extracted for each species analyzed. The number of repeats used by each paper in producing their nutritional profiles was not accounted for during data extraction. Consequently, each paper, including the original profiles produced here, contributed a maximum of one datapoint to the final dataset for each of the proximate, fatty acid, and amino acid analyses conducted for each species analyzed. All papers used are given in Supplementary Table 1.
There is high heterogeneity in the nutritional literature in terms of the fatty acids identified and the fatty acid naming system used, with some papers opting for the trivial names whilst others prefer the systematic names or lipid numbers. Therefore, certain steps were taken to increase the comparability of the fatty acid literature.
Firstly, it was ensured that every paper contained the same fatty acids under a consistent naming system; where a paper did not identify a particular fatty acid, it was introduced at a content of 0%. Secondly, papers with a high proportion (>2.5%) of ambiguously named fatty acids, meaning that the specific isomer cannot be discerned, were removed. Where ambiguously named fatty acids accounted for <2.5% of a particular paper's fatty acid profile then the paper was retained but without the ambiguous fatty acid. Where a particular fatty acid was not reported in any form other than the ambiguous form across the utilized literature then the ambiguous name was retained; this was the case for pentadecenoic acid, docosadienoic acid, and decenoic acid. Furthermore, singleton fatty acids, those only identified in a single publication, were removed to reduce the impact of experimental variability and because their inclusion would increase the fatty acid diversity of more represented species. The sum of fatty acid profile in each paper was fixed to 100% by introducing an “Others” category when the profile was below 100%, for instance due to the removal of ambiguous fatty acids. Finally, where a paper reported M. domestica, R. phoenicis, C. butyrospermi, or M. bellicosus fatty acid profiles in differing units, they were converted to the standard units of % total fatty acids due to H. illucens being over-represented in the literature.
This manipulation left a final fatty acid dataset comprised of 16 H. illucens, six M. domestica, nine R. phoenicis, three C. butyrospermi, and five M. bellicosus papers.
There is high heterogeneity in the units used to report amino acid profiles in the literature, with amino acid content in g/100 g crude protein, % dry matter, and % total amino acids all being present. Units of g/100 g crude protein were chosen due to being the most prevalent. As a result of H. illucens being very heavily represented in the edible insect literature relative to the other species, where a paper reported M. domestica, R. phoenicis, C. butyrospermi, or M. bellicosus amino acid profiles on a % dry matter basis, this was converted to the selected units of g/100 g crude protein using the paper's reported crude protein value. This process left a final amino acid dataset comprised of six H. illucens, two M. domestica, eight R. phoenicis, two C. butyrospermi, and four M. bellicosus papers. Unlike for proximate and fatty acid analyses, this dataset was comprised entirely of profiles derived from the literature as original amino acid profiles were not produced here.
All statistical analyses were conducted in R (v4.1.0) (71) with RStudio (v1.4.1106) (72).
Proximate content differences between the five analyzed species were assessed by a series of non-parametric Kruskal-Wallis tests, with post-hoc differences evaluated using the package, dunn.test (73). Visualizations were produced with the package ggplot2 (74). This was performed for the three macronutrients most widely reported in the literature, which included ash, crude protein, and crude fat; other proximate nutrients were not reported frequently and consistently enough to allow statistical evaluation of interspecific differences.
Fatty acid profile structuring between species was assessed by principle component analysis. The PCA output was visualized using the package ggbiplot and interspecific differences in the distributions of the two primary principle components were evaluated using non-parametric Kruskal-Wallis tests, with post-hoc differences evaluated using the package, dunn.test (73). Furthermore, reported fatty acids were classified based on saturation status and, for each paper, the sum of saturated fatty acids, mono-unsaturated fatty acids, and poly-unsaturated fatty acids was determined. Interspecific differences in the degree of saturation were assessed by a series of non-parametric Kruskal-Wallis tests, with post-hoc differences again being evaluated with the package, dunn.test (73). The association of each saturation class with each of the two primary principle components in the fatty acid analysis was also assessed by a correlation test using the Pearson method.
There is high variation in the literature in terms of which amino acids are profiled, with some papers reporting only essential amino acids, others reporting all amino acids, whilst others report some other subset of amino acids. Consequently, it was not possible to statistically evaluate how amino acid profiles structure across species. Instead, the mean content of each essential amino acid was determined for each species and these, along with the essential amino acid requirements for adults (75), were visualized in ggplot2 (74) in order to assess to what extent these species' amino acid profiles meet human requirements.
The insect species generally contained high protein levels, ranging from 31% in R. phoenicis to 64% in C. butyrospermi, demonstrating their potential as a protein source, with C. butyrospermi and M. domestica even exceeding beef in their protein content. The highest fat content was found in R. phoenicis (65%), greatly exceeding that of the other insect species and of beef (41%). Besides R. phoenicis, the insect species were generally characterized by low fat levels (18.03%, 11.49%, and 12.18% in H. illucens, M. domestica, and C. butyrospermi, respectively). Ash content was highest in the dipteran species (17.71% and 9.84% in H. illucens and M. domestica, respectively), whilst levels of neutral and acid detergent fiber were similar in C. butyrospermi and M. domestica but lower in H. illucens (Table 1).
Table 1. Original proximate profiles produced in this study for the four analyzed species of edible insects alongside a beef control, obtained from van Huis et al. (24).
The black soldier fly, H. illucens, contained the highest proportion of saturated fatty acids (61.36%), whilst the other dipteran, M. domestica, had the lowest (44.74%) (Table 2). In H. illucens, these saturated fatty acids were primarily composed of dodecanoic acid (8.37%), which was absent or at low level in the other species, tetradecanoic acid (5.01%), hexadecanoic acid (9.44%), and octadecanoic acid (6.61%), which were also prevalent in the other species, with M. bellicosus containing 24.26% hexadecanoic acid.
The palm weevil, R. phoenicis, had the highest mono-unsaturated fatty acid content (41.78%) and C. butyrospermi the lowest (14.02%). In R. phoenicis, this portion was primarily composed of 11-octadecenoic acid (29.38%), whilst oleic acid (9-octadecenoic acid) was absent; similarly, oleic acid was not detected in C. butyrospermi but was present in the other three species.
Contrary to mono-unsaturated content, the highest proportion of poly-unsaturated fatty acids was found in the caterpillar, C. butyrospermi, and the lowest in R. phoenicis (28.94 and 2.79%, respectively). Across the species, this portion was primarily comprised of n-6 fatty acids, with n-3 fatty acids being absent completely in H. illucens, M. domestica, and M. bellicosus. However, the opposite was found in C. butyrospermi, with n-3 fatty acids being more prevalent due to the very high α-linolenic acid content, which was not detected in the other species.
Species differences in their ash, crude protein, and crude fat contents are shown in Figure 1, as well as how they compare with beef in the latter two nutrients. These three macronutrients were the only proximate measures of high enough sample size to allow rigorous inspection of interspecific differences after combining the proximate analyses conducted in this paper with the nutritional literature.
Figure 1. Proximate content, on a % dry matter basis, using both data analyzed in this paper and literature-sourced data. From top to bottom: ash, crude protein, and crude fat content. From left to right: Hermetia illucens (black soldier fly, BSF; in gray), Musca domestica (house fly, HSF; in purple), Rhynchophorus phoenicis (palm weevil, PL; in orange), Cirina butyrospermi (shea tree caterpillar, STC; in green), and Macrotermes bellicosus (termite, TM; in blue). The horizontal hashed lines in the bottom two panels represent the crude protein and crude fat content of beef, respectively, obtained from van Huis et al. (24).
Highly significant interspecies differences were found in ash, crude protein, and crude fat content (Table 3). As was the case for the proximate profiles produced in this paper (Table 1), ash content was highest in H. illucens and M. domestica, which did not differ from each other but both differed significantly from the other three species, except when comparing M. domestica and C. butyrospermi. Ash accounted for around 5% of dry matter in the remaining three species, with no differences between them.
Table 3. Statistical output of a series of non-parametric tests: firstly, Kruskal-Wallis output, in the form χ2 (p-value), assessing whether species differ in their content of ash, crude protein, and crude fat, and below the post-hoc significance output determining whether individual species pairs differ in their levels of these proximate nutrients.
Crude protein was found to be most prevalent in C. butyrospermi and M. domestica at around 60%, corroborating the patterns detected previously (Table 1). Although these species' protein content did not differ from each other, they were both significantly greater than the levels found in the remaining three insect species and both exceeded that found in beef (55%).
Crude fat was most prevalent in R. phoenicis and M. bellicosus and was lowest in C. butyrospermi and M. domestica, with significant differences existing between but not within these species-pairs. Furthermore, R. phoenicis was found to be highly variable in crude fat content, ranging from around 20 to over 70% of dry matter; this variable distribution can be seen to fit into two distinct clusters of datapoints, one at around 60–70% and the other below 40%.
The principal component analysis assessing between-species structuring in fatty acid profiles indicates clear, major differences between species in their position relative to PC1 and PC2, particularly in the case of PC2 (Figure 2, Table 4). Figure 2 also illustrates the relative position of the fatty acid profile of beef (24). In particular, the H. illucens data was found to diverge, forming a distinct cluster in the PCA while differences between the other species, and beef, were minimal.
Figure 2. Principal component analysis of the fatty acid profiles of the five analyzed species of edible insect. The relationship between the two primary principal components and individual datapoints, corresponding to a fatty acid profile of an individual species presented either in the literature or analyzed in this study, is shown. Points are colored based on the species to which they belong, as are the ellipses surrounding each species' datapoints. Superimposed onto the plot is the position of the fatty acid profile of beef, obtained from van Huis et al. (24), relative to the two primary principle components.
Table 4. Statistical summary of non-parametric tests assessing how these species of edible insect differ in their fatty acid profiles.
PC2 was shown to share a strong positive correlation with saturated fatty acid content and a strong negative correlation with mono-unsaturated fatty acid content (Table 4), suggesting these parameters may show the strongest interspecific structuring (Figure 3). Hermetia illucens showed the highest content of saturated fatty acids (~65%), corroborating the PCA output, higher than all compared species other than C. butyrospermi and exceeding that of beef. The lowest proportion of saturated fatty acids was found in M. domestica. Furthermore, mono-unsaturated fatty acid content was lowest in H. illucens and C. butyrospermi and highest in the other three species, with strongly significant differences existing between but not within these high and low groups.
Figure 3. Variation in fatty acid profile saturation as a function of insect species. Shown are each species' content, as a % of total fatty acids, of, from top to bottom, saturated fatty acids, mono-unsaturated fatty acids, poly-unsaturated fatty acids, n-6 poly-unsaturated fatty acids, and n-3 poly-unsaturated fatty acids. Shown, from left to right, in each panel: Hermetia illucens (black soldier fly, BSF; in gray), Musca domestica (house fly, HSF; in purple), Rhynchophorus phoenicis (palm weevil, PL; in orange), Cirina butyrospermi (shea tree caterpillar, STC; in green), and Macrotermes bellicosus (termite, TM; in blue). The hashed horizontal lines in each panel represent the degree of saturation of the fatty acid profile of beef, obtained from van Huis et al. (24).
Poly-unsaturated fatty acid content was highest in C. butyrospermi, exceeding that of the other four insect species and beef. This can primarily be attributed to n-3 fatty acids, of which C. butyrospermi contained around 30%, differing from the basal levels of the other species. Conversely, little interspecies variation was found in the content of n-6 fatty acids.
For each species, each essential amino acid is reported as the mean of data reported in the literature and in g/100 g of crude protein. No literature was found reporting the tryptophan content of C. butyrospermi, indicating the underrepresentation of this species in the nutritional literature, so the total sum of essential amino acids was evaluated in the absence of tryptophan. Also shown in Figure 4 are the concentrations of each amino acid required by the human adult (75).
Figure 4. The mean content of each essential amino acid, in g/100 g of crude protein, in each species of edible insect, alongside the total sum of essential amino acids. As neither of the two published papers on C. butyrospermi's amino acid content reported tryptophan, the mean total essential amino acid contents have here been calculated in the absence of tryptophan. The horizontal lines superimposed onto each panel refer to current estimates for adult requirements of each amino acid, obtained from WHO/FAO/UNU (75). Within each amino acid panel, reported is, from left to right: Hermetia illucens (black soldier fly, BSF; in gray), Musca domestica (house fly, HSF; in purple), Rhynchophorus phoenicis (palm weevil, PL; in orange), Cirina butyrospermi (shea tree caterpillar, STC; in green), and Macrotermes bellicosus (termite, TM; in blue).
For many amino acids, relatively little interspecies variation was detected (Figure 4). This was the case for histidine, lysine, phenylalanine, threonine, and valine, with all species showing concentrations of these amino acids exceeding that of human requirements.
Greater variation was detected in leucine and methionine, with the highest levels of these found in M. bellicosus and M. domestica, respectively. In both cases, levels in C. butyrospermi fell slightly short of human requirements. However, the small sample size of the C. butyrospermi amino acid data should also be noted, with only two papers contributing profiles.
For all other amino acids, all species exceeded human requirements, as did the total essential amino acid content of all species, demonstrating their nutritive potential.
Here, original nutritional profiles, including proximate and fatty acid data, have been produced for five edible insect species of socio-economic importance in West Africa, a region where entomophagy is highly popular (76) but has been relatively neglected in ento-ethno research. These profiles can be used in future research, adding to the available data, which has thus-far been lacking for some species; for example, the shea tree caterpillar, C. butyrospermi, evaluated in this study, has been neglected in the nutritional literature despite being popularly consumed. These original profiles have been combined here with those proximate, fatty acid, and also amino acid profiles previously produced in the literature in order to highlight interspecific variation in nutrient content. This has allowed rigorous assessment not only of whether these species have potential in combatting widespread undernutrition but also which of the five species show nutrient profiles most favorable for consumption.
These results promote the general suitability and substantial potential of edible insects as a nutrient source. The ash and crude protein content values fit well within the range previously identified for edible insects, with ash previously reported at <1%-25.95% and protein at 4.9–77.13% (59) and 20–70% (77). Similarly, crude fat content, which here ranged from around 15% of dry matter in C. butyrospermi to around 55% in R. phoenicis, was broadly corroborated by the nutritional literature; Rumpold and Schlüter (59) reported a range of <1–77% across edible insects whilst this narrowed to 10–50% according to Xiaoming et al. (77). The insects analyzed here compare well with the protein and fat content of around 55 and 40%, respectively, previously reported for beef (24).
The composition of the crude fat portion, described in part by the fatty acid profile, was also shown here to be generally favorable in the insects. In particular, the insects showed a higher proportion of poly-unsaturated fatty acids than beef, which are recognized as essential for development in children and are often limited in landlocked countries with limited fish access (24). The amino acid profiles of these edible insect species generally exceeded the essential amino acid requirements of adult humans, with minor exceptions, such as the limited leucine and methionine content of the caterpillar.
These data confirm previous work that edible insects generally represent a viable and thus-far underutilized nutrient source, comparing extremely well to human requirements and traditional meat sources, such as beef (12, 24, 58, 59). Not only are they nutritionally comparable and often more desirable than beef but edible insects also provide numerous other benefits for sustainable food security, including the efficiency, low cost, and environmental benefits of raising them (23). However, the results presented here, as well as highlighting the potential of entomophagy, do promote certain insect species over others.
Much interspecific nutritional variation was found between the five species analyzed here, with implications for their utility in assuring sustainable food security. The protein content and quality of ingredients has been described as the most important consideration in food and feed production (29). Proteins have a wide variety of bodily functions, including providing the structural components of many tissues (26), promoting growth and recovery (78), being involved in biochemical processes (79), and maintaining immune function (80). Consequently, protein deficiency, which is prevalent globally (2, 81), threatens these processes. Therefore, our results indicate that the caterpillar, C. butyrospermi, and house fly, M. domestica, may be most suitable for human consumption, both showing the highest crude protein content and greatly exceeding that of the other three species.
Furthermore, ash is generally used as an indicator of the mineral content of foods (60), suggesting that M. domestica and H. illucens, with the highest ash content found here, may show potential in combatting mineral and micronutrient deficiency, which are also prevalent and dangerous (30, 31). Also, phosphorus is a mineral sometimes added to aquaculture feed (82), potentially causing water pollution (83). The high mineral content of M. domestica emphasizes its suitability as an aquaculture feedstock as well as for human consumption, potentially alleviating phosphorus pollution. The considerably higher levels of neutral and acid detergent fiber identified in C. butyrospermi and M. domestica compared to H. illucens further their consumption potential. The neutral and acid detergent fiber portions of edible insects are thought to contain chitin (84, 85), a molecule which can act as an anticoagulant and can promote wound healing (86).
Musca domestica and especially C. butyrospermi were also found to possess fatty acid profiles perhaps more suited for human consumption than the other three species. Cirina butyrospermi contained by far the highest proportion of poly-unsaturated fatty acids, particularly omega-3 fatty acids, with the original profile produced here being especially rich in α-linolenic acid (ALA). Poly-unsaturated fatty acids have been shown to lower low-density lipoprotein cholesterol levels in men and women (87), protecting against atherosclerotic issues (26) and are also thought essential in child development (24). Dietary deficiencies of poly-unsaturated fatty acids can cause a range of symptoms, including growth inhibition and reduced efficacy of wound healing (88). Conversely, saturated fatty acids, which were highest in H. illucens, the species which formed its own fatty acid profile cluster characterized by high saturation, are a risk factor of increased blood pressure, low-density lipoprotein levels, and atherosclerosis (26, 27, 88, 89). Indeed, a food's ratio of poly-unsaturated to saturated fatty acids is often used to indicate its ability to lower cholesterol and reduce the risk of cardiovascular diseases (27). Poly-unsaturated fatty acids are also essential for aquaculture production (25, 29, 90), suggesting that a C. butyrospermi-based fish feed may provide the necessary fatty acid profile without the need for dietary supplementation. Meanwhile, M. domestica contained the joint highest proportion of mono-unsaturated fatty acids, alongside R. phoenicis and M. bellicosus, with palmitoleic, elaidic, and oleic acid particularly represented. Mono-unsaturated fatty acids have also been shown to have cholesterol lowering abilities (27, 87) and oleic acid even has anti-carcinogenic properties, suppressing the expression of breast cancer oncogene, Her-2/neu (91). Therefore, a diet rich in the shea tree caterpillar, C. butyrospermi, and house fly, M. domestica, would likely provide a high content of unsaturated fatty acids, bringing multiple associated health benefits. However, an issue with such a diet is that both of these species were found to have the lowest crude fat content whilst the highest was found in R. phoenicis.
Fat is the most energy dense nutrient source, producing 9 kcal per gram (92), meaning that low-fat diets may result in calorie deficiency, a leading issue in malnutrition (26, 27). Fat deficiency can also exacerbate protein deficiency due to protein being redirected toward energy metabolism in low fat conditions (26). Furthermore, fats are vital in the structural and biological functioning of cells (60), as well as increasing food palatability through retaining flavors (26, 27, 60). Therefore, supplementation of a C. butyrospermi- and M. domestica-based diet with high-fat R. phoenicis may be ideal. Rhynchophorus phoenicis are also seen as a delicacy in many areas and, through breaking down waste in palm agro-systems, can contribute to circularizing palm agronomies in Africa (93), aiding sustainable development.
Furthermore, although not the case for M. domestica, C. butyrospermi was found here to be marginally limited in its leucine and methionine content. These essential amino acids are important in energy production by facilitating carnitine synthesis for the transport of fatty acids into the mitochondria for β-oxidation (94); leucine also enhances growth in infants (26). However, the low leucine and methionine in C. butyrospermi may be inconsequential because here amino acids are reported relative to crude protein content which was high in this species. Therefore, levels of essential amino acids per unit of C. butyrospermi-based food may be expected to more than meet human requirements. All insect species were also shown to contain sufficient lysine, an essential amino acid particularly lacking in staple cereal-based diets (26), which has been shown to have antiviral properties (95).
Therefore, overall these results, although in certain cases with limited sample sizes, suggest that consumption of C. butyrospermi and M. domestica, with supplementation by the high-fat R. phoenicis, may optimize the health benefits of entomophagy. This further supports redressing the current neglect of C. butyrospermi in the nutritional literature and promoting investigation of its farming potential as co-production with a growing, and high value, shea-related industry.
In addition to the between-species nutritional variation discussed above, we also identified substantial within-species variation. For example, the crude protein content of R. phoenicis ranged from 10.5 to 66.3% of dry matter and their crude fat from 17.3 to 71.6%, with this fat distribution segregating into two distinct clusters. There are several reasons why such within-species variation may arise.
A first source of variation may stem from differences in the experimental procedures used by different studies as some studies may use non-standard methodology or a differing number of repeats. Here, only studies which nutritionally profiled using standard AOAC (67) methods were incorporated into the dataset in an attempt to reduce experimental variation. However, even within standard procedures there is disagreement in the best way to nutritionally profile edible insects, perhaps due to entomophagy research being relatively novel. For example, many methods for assessing sample crude protein content, including the Kjeldahl method used here, rely on converting nitrogen content to crude protein with a conversion factor (96). A conversion factor of 6.25 has popularly and traditionally been used, stemming from the idea that protein is made up of 16% nitrogen (97). However, this has faced much scrutiny, especially regarding edible insect profiling. This is firstly due to the nitrogen content of protein depending strongly on the amino acid composition (97). Furthermore, the presence of non-protein nitrogen in insects, including in chitin, can cause overestimation of insect crude protein levels (96, 98). Therefore, many have suggested that such conversion factors must be re-evaluated in insect food science. Janssen and Lambertus (96), through comparing the total nitrogen and protein nitrogen of three edible insect species, suggested a new conversion factor of 4.76 for insects. However, Finke (85) argues the opposite, that chitin accounts for a relatively small portion of an insect's nitrogen and is therefore not impacting calculated crude protein values. Indeed, some researchers still favor the conversion factor of 6.25 (29, 99–103) whilst others use 4.76 (104–106). This discrepancy can introduce high protein variation in the literature; for example, Basto, Matos, and Valente (107) calculated H. illucens crude protein content with both a conversion factor of 4.76 and 6.25 and obtain values of 32.2 and 46.1%, respectively. Therefore, a consensus must be reached to increase the comparability of the nutritional literature. However, what is there to suggest that a single consensus conversion factor would be appropriate for insects, the most speciose animal group? A different strategy may be for researchers to calculate sample chitin content alongside total nitrogen and scale the nitrogen value accordingly (98). Alternatively, researchers could calculate protein content from amino acid analyses, although this suffers from some methods destroying certain amino acids, such as tryptophan and cysteine being destroyed during acid hydrolysis (108). Regardless, this discussion of contentious conversion factors emphasizes the need to resolve experimental variation so that nutritional consensus can emerge.
As well as between-study analytical variation, the husbandry and rearing conditions or whether insects were farmed or wild-caught, could inflate nutritional variation. Larval diets can greatly affect the insect's nutritional profile, meaning that different substrates in the studies used here could cause marked within-species nutritional divergence. Nutritional divergence between H. illucens individuals raised on different diets has been widely reported (63–65). This has implications for the use of edible insects as waste valorisers as well as a source of nutrients as the type of organic waste substrate used can impact the utility of the resultant insects. For example, Scala et al. (109) showed that H. illucens raised on apple waste accumulated 50% more fat than those on a mixed diet of fruit and grain waste. Therefore, organic waste of high enough quality may be required for edible insect nutrient profiles suitable for consumption. If heavy supplementation of waste substrates is required, such as with fish offal (65), then the sustainability benefits of circularizing food production systems by raising insects on organic waste side streams may be reduced. Furthermore, differences in the age of harvesting may be inflating the nutritional variation we found. Although only specific life stages were analyzed here, within-life stage nutritional variation has been documented and could be influential. For example, Do et al. (110) showed crude protein differences of around 5% between H. illucens larvae with a 3 day age gap, which increased to over 10% with a seven day age gap. Insect farmers and harvesters can thus plan optimal harvesting age for desired nutrient profiles. Finally, rearing conditions may have a large impact on nutrient accumulation, further inflating nutritional variation. Temperature increases can reduce livestock feed intake and conversion efficiency (111, 112), with marked impacts on resultant nutrient profiles. For instance, Dobermann, Field, and Michaelson (113) showed reduced protein content in cricket meal under heat stress. Therefore, with much husbandry variation potentially impacting nutrient profiles, perhaps it is not surprising that any definitive consensus on interspecific nutrient differences is yet to emerge. Quantifying these effects should be a major goal of research going forwards.
Finally, a large contributor to this high within-species variation could be natural or biological variation in individual or population nutrient content. The papers used here isolated insects from a wide range of localities; for example, the original profiles in this paper were produced from individuals from Ghana, whereas Akullo et al. (114) isolated M. bellicosus from Uganda. Therefore, nutritional results may differ depending on the specific population being sampled, perhaps due to ancestry or genetic differences. For example, in this study, the R. phoenicis crude fat distribution was found to split into two discrete clusters, a high- and low-fat group, potentially reflecting the sampling of two distinct populations or meta-populations. Stark genetic differences have indeed been found between edible insect populations globally. For example, high mitochondrial and microsatellite diversity and structure has been identified in H. illucens (115, 116) and M. domestica (117, 118). This genetic divergence could potentially translate into nutritional differences. Therefore, perhaps future research, rather than focusing on specific localities, could investigate the geographic and population structuring of nutrient content in order to assess the extent of this biological variation. Beyond that, research could start to use genetic and nutritional variation to allow selective breeding for nutrient content in order to further domesticate and optimize mini-livestock for combatting undernutrition. This has long been practiced in more traditional agro-species. For example, work on cows has identified genetic polymorphisms associated with altered fatty acid profiles, with the potential to select loci in order to reduce saturation (119, 120). There is even interest in the genetic-assisted selective breeding for reduced methane emissions in cows, aiding the cattle industry's sustainability (121). Similar interest in selective breeding has been seen more recently in aquatic agro-species (122, 123). Therefore, research should aim to implement similar methods in edible insect species. Depending on the extent of genotypic and phenotypic variation, the edible insect industry may not be restricted to nutritional levels found naturally, identified in this and other studies. Instead, selective breeding of optimal profiles may be possible, particularly if industrial interest in the commercial rearing of edible insects continues. Many edible insects may be especially suitable from a high-throughput, selective breeding standpoint due to them being manipulable, mass rearable, and with short generation times. However, in order to use genetic techniques for improving nutrient profiles, such as marker-assisted selection (124), the available genetic resources for edible insect species must be increased. For example, although genomes are available for H. illucens (125) and M. domestica (126), they have not yet been produced for the other three species analyzed here. Therefore, research could do well to make increasing available genetic and nutritional resources a priority.
Insects can clearly play an important role in future sustainable development and food security, and it is also becoming increasingly clear that this is particularly the case for certain species. Research should continue to characterize the nutrient profiles of as many tractable species as possible to direct interest into promising species in an informed way. For example, here we showed that the shea tree caterpillar, C. butyrospermi, alongside the house fly, M. domestica, appears particularly nutritionally suitable for human consumption. This is surprising as it is a species that has received little to no research interest. Therefore, continuing to profile this species, including identifying any safety concerns surrounding its consumption, may substantiate the promising results presented here. This is not to say that the other three species analyzed here should not be considered a useful aspect of sustainable food security. As discussed, they may be particularly useful in dietary supplementation, such as the high fat content of R. phoenicis. Furthermore, H. illucens and R. phoenicis show promise in circularizing agro-processes, with H. illucens especially popular in the animal feed industry. Furthermore, research could continue to assess the factors dictating individual nutrient content, including husbandry effects such as diet, rearing conditions, sex, and stage of the insect. This would allow insect farmers to optimize nutrient accumulation through husbandry modifications. It may also mean that insect farming moves away from selecting the most nutritious species and toward modifying and optimizing the nutrient content of species which are easily reared or more beneficial for sustainable development. Finally, linked to that is the idea that research could increasingly resolve the extent to which there is natural within-species nutritional variation, as well as any genetic variation underlying this. This could allow the targeted selective breeding of edible insects to create “founder stocks,” which optimize their nutrient profiles for combatting undernutrition and assuring sustainable food security.
The original contributions presented in the study are included in the article/Supplementary Material, further inquiries can be directed to the corresponding author/s.
JA designed the research and carried out fieldwork. XC and IO conducted the biochemical analyses. JA and XC analyzed the data. BR conducted the statistical and meta-analyses. JA and BR wrote the initial manuscript, with contributions from VS and CC. All authors contributed to the article and approved the submitted version.
This study received funding contributions from the University of Michigan PARTNER II, AnePaare Farms, Aspire Food Group, the Association of African Universities (AAU), Research England's Global Challenges Research Fund (GCRF), and the Royal Society (UK).
The authors declare that the research was conducted in the absence of any commercial or financial relationships that could be construed as a potential conflict of interest.
All claims expressed in this article are solely those of the authors and do not necessarily represent those of their affiliated organizations, or those of the publisher, the editors and the reviewers. Any product that may be evaluated in this article, or claim that may be made by its manufacturer, is not guaranteed or endorsed by the publisher.
Authors are thankful to the staff of the International Center for Insect Physiology and Ecology (icipe) especially Prof. Baldwyn Torto for the analytical support provided to JA. We are grateful to Dr. Herman Lutterodt of the Food Science department of the Kwame Nkrumah University of Science and Technology (KNUST) who has encouraged and reviewed the manuscript.
The Supplementary Material for this article can be found online at: https://www.frontiersin.org/articles/10.3389/fnut.2021.792941/full#supplementary-material
1. Griggs D, Stafford-Smith M, Gaffney O, Rockström J, Öhman MC, Shyamsundar P, et al. Sustainable development goals for people and planet. Nature. (2013) 495:305–7. doi: 10.1038/495305a
2. FAO IFAD UNICEF WFP WHO. The State of Food Security and Nutrition in the World 2020. Transforming Food Systems for Affordable Healthy Diets. Rome: FAO (2020).
3. Martins VJB, Toledo Florêncio TMM, Grillo LP, Franco M do CP, Martins PA, Clemente APG, et al. Long-lasting effects of undernutrition. Int J Environ Res Public Health. (2011) 8:1817–46. doi: 10.3390/ijerph8061817
4. Sawaya AL, Martins P, Hoffman D, Roberts SB. The link between childhood undernutrition and risk of chronic diseases in adulthood: a case study of Brazil. Nutr Rev. (2003) 61:168–75. doi: 10.1301/nr.2003.may.168-175
5. Sawaya AL, Sesso R, De Menezes TM, Florêncio T, Fernandes MTB, Martins Msc PA. Association between chronic undernutrition and hypertension. Matern Child Nutr. (2005) 1:155–63. doi: 10.1111/j.1740-8709.2005.00033.x
6. Jonkers DMAE. Microbial perturbations and modulation in conditions associated with malnutrition and malabsorption. Best Pract Res Clin Gastroenterol. (2016) 30:161–72. doi: 10.1016/j.bpg.2016.02.006
7. Rodríguez L, Cervantes E, Ortiz R. Malnutrition and gastrointestinal and respiratory infections in children: a public health problem. Int J Environ Res Public Health. (2011) 8:1174–205. doi: 10.3390/ijerph8041174
8. UN. World Population Prospects 2019: Ten Key Findings. (2019). Available online at: https://population.un.org/wpp (accessed June 17, 2021).
9. Msangi S, Rosegrant MW. Feeding the Future's Changing Diets: Implications for Agriculture Markets, Nutrition, and Policy. New Delhi: IFPRI (2020).
10. Asian DB. Food Security Poverty in Asia the Pacific: Key Challenges Policy Issues. (2012). Available online at: http://hdl.handle.net/11540/904 (accessed June 17, 2021).
11. Dickie F, Miyamoto M, Collins CMT. The potential of insect farming to increase food security. In: Edible Insects. (2019). p. 1–10. doi: 10.5772/intechopen.88106
12. Halloran A, Vantomme P. The Contribution of Insects to Food Security, Livelihoods the Environment. (2013). Available online at: www.fao.org/forestry/edibleinsects/en/ (accessed June 17, 2021).
13. Lanfranchi GB. Minilivestock consumption in the ancient near East: the case of locusts. In: Ecological Implications of Minilivestock: Potential of Insects, Rodents, Frogs and Snails. Enfield: Science Publisher Inc. (2005). p. 163–74.
14. Tommaseo-Ponzetta M. Insects: food for human evolution. In: Ecological Implications of Minilivestock: Potential of Insects, Rodents, Frogs and Snails. Enfield: Science Publisher Inc. (2005). p. 141–61.
15. Anankware JP, Fening KO, Osekre E, Obeng-Ofori D. Insects as food and feed. Int J Agric Res. (2015) 3:143–151. doi: 10.1002/fsat.3201_7.x
16. Paoletti MG. Ecological Implications of Minilivestock. Enfield: Science Publisher Inc. (2005). doi: 10.1201/9781482294439
17. van Huis A. Insects as food in Sub-Saharan Africa. Insect Sci its Appl. (2003) 23:163–85. doi: 10.1017/S1742758400023572
18. Jongema Y. List of Edible Insects of the World (April 1, 2017) - WUR. (2017). Available online at: https://www.wur.nl/en/Research-Results/Chair-groups/Plant-Sciences/Laboratory-of-Entomology/Edible-insects/Worldwide-species-list.htm (accessed June 17, 2021).
19. Malaise F. Human consumption of lepidoptera, termites, orthoptera, and ants in Africa. In: Ecological Implications of Minilivestock: Potential of Insects, Rodents, Frogs and Snails. Enfield: Science Publisher Inc. (2005). p. 175–230.
20. van Huis A. Insects eaten in Africa (Coleoptera, Hymenoptera, Diptera, Heteroptera, Homoptera). In: Ecological Implications of Minilivestock: Potential of Insects, Rodents, Frogs and Snails. Enfield: Science Publisher Inc. (2005). p. 231–44.
21. Mitsuhashi J. Edible insects in Japan. In: Ecological Implications of Minilivestock: Potential of Insects, Rodents, Frogs and Snails. Enfield: Science Publisher Inc. (2005). p. 251–62.
22. Yhoung-Aree J, Viwatpanich K. Edible insects in the Laos PDR, Myanmar, Thailand, and Vietnam. In: Ecological Implications of Minilivestock: Potential of Insects, Rodents, Frogs and Snails. Enfield: Science Publisher Inc. (2005).
23. van Huis A. Potential of insects as food and feed in assuring food security. Annu Rev Entomol. (2013) 58:563–83. doi: 10.1146/annurev-ento-120811-153704
24. van Huis A, van Itterbeeck J, Klunder H, Mertens E, Halloran A, Muir G, et al. Edible Insects: Future Prospects for Food and Feed Security. Rome: FAO. (2013).
25. Barroso FG, de Haro C, Sánchez-Muros MJ, Venegas E, Martínez-Sánchez A, Pérez-Bañón C. The potential of various insect species for use as food for fish. Aquaculture. (2014) 3422–:193–201. doi: 10.1016/j.aquaculture.2013.12.024
26. Ekpo KE. Effect of processing on the protein quality of four popular insects consumed in Southern Nigeria. Arch Appl Sci Res. (2011) 3:307–26. Available online at: http://scholarsresearchlibrary.com/archive.html
27. Womeni HM, Linder M, Tiencheu B, Mbiapo FT, Villeneuve P, Fanni J, et al. Oils of insects and larvae consumed in Africa: potential sources of polyunsaturated fatty acids. OCL Ol Corps Gras Lipides. (2009) 16:230–5. doi: 10.1051/ocl.2009.0279
28. Bosch G, Zhang S, Oonincx DGAB, Hendriks WH. Protein quality of insects as potential ingredients for dog and cat foods. J Nutr Sci. (2014) 3:e29. doi: 10.1017/jns.2014.23
29. Hussein M, Pillai VV, Goddard JM, Park HG, Kothapalli KS, Ross DA, et al. Sustainable production of housefly (Musca domestica) larvae as a protein-rich feed ingredient by utilizing cattle manure. PLoS ONE. (2017) 12:e0171708. doi: 10.1371/journal.pone.0171708
30. FAO WHO. Human Vitamin and Mineral Requirements: Report of a Joint FAO/WHO expert consultation. Bangkok, Thailand (2001).
31. Muller O, Krawinkel M. Malnutrition and health in developing countries. Can Med Assoc J. (2005) 173:279–86. doi: 10.1503/cmaj.050342
32. Smil V. Worldwide transformation of diets, burdens of meat production and opportunities for novel food proteins. Enzyme Microb Technol. (2002) 30:305–11. doi: 10.1016/S0141-0229(01)00504-X
33. Oonincx DGAB, van Itterbeeck J, Heetkamp MJW, van den Brand H, van Loon JJA, van Huis A. An exploration on greenhouse gas and ammonia production by insect species suitable for animal or human consumption. PLoS ONE. (2010) 5:e14445. doi: 10.1371/journal.pone.0014445
34. Lalander CH, Fidjeland J, Diener S, Eriksson S, Vinnerås B. High waste-to-biomass conversion and efficient Salmonella spp. reduction using black soldier fly for waste recycling. Agron Sustain Dev. (2015) 35:261–71. doi: 10.1007/s13593-014-0235-4
35. Diener S, Solano NMS, Gutiérrez FR, Zurbrügg C, Tockner K. Biological treatment of municipal organic waste using black soldier fly larvae. Waste Biomass Valoriz. (2011) 2:357–63. doi: 10.1007/s12649-011-9079-1
36. Sheppard DC, Newton GL, Thompson SA, Savage S. A value added manure management system using the black soldier fly. Bioresour Technol. (1994) 50:275–9. doi: 10.1016/0960-8524(94)90102-3
37. Mallapaty S. Invesitgation into covid origin begins but faces challenges. Nature. (2020)341–2. doi: 10.1038/d41586-020-03165-9
38. Meyer-Rochow VB. Therapeutic arthropods and other, largely terrestrial, folk-medicinally important invertebrates: a comparative survey and review. J Ethnobiol Ethnomed. (2017) 13:1–31. doi: 10.1186/s13002-017-0136-0
39. Pemberton R. Contemporary use of insects and other arthropods in traditional Korean medicine (Hanbang) in South Korea and elsewhere. In: Ecological Implications of Minilivestock: Potential of Insects, Rodents, Frogs and Snails. Enfield: Science Publisher Inc. (2005). p. 459–73.
40. Rastogi N. Provisioning services from ants: food and pharmaceuticals. Asian Myrmecol. (2011) 4:103–20. Available online at: http://www.asian-myrmecology.org/publications/am04_103-120_ragosti_2011.pdf
41. Zimian D, Yonghua Z, Xiwu G. Medicinal terrestrial arthropods in China. in Ecological Implications of Minilivestock: Potential of Insects, Rodents, Frogs and Snails. Enfield: Science Publisher Inc. (2005). p. 481–9.
42. Li Q, Zheng L, Qiu N, Cai H, Tomberlin JK, Yu Z. Bioconversion of dairy manure by black soldier fly (Diptera: Stratiomyidae) for biodiesel and sugar production. Waste Manag. (2011) 31:1316–20. doi: 10.1016/j.wasman.2011.01.005
43. Zheng L, Hou Y, Li W, Yang S, Li Q, Yu Z. Biodiesel production from rice straw and restaurant waste employing black soldier fly assisted by microbes. Energy. (2012) 47:225–9. doi: 10.1016/j.energy.2012.09.006
44. Belghit I, Liland NS, Gjesdal P, Biancarosa I, Menchetti E, Li Y, et al. Black soldier fly larvae meal can replace fish meal in diets of sea-water phase Atlantic salmon (Salmo salar). Aquaculture. (2019) 503:609–19. doi: 10.1016/j.aquaculture.2018.12.032
45. Ido A, Iwai T, Ito K, Ohta T, Mizushige T, Kishida T, et al. Dietary effects of housefly (Musca domestica) (Diptera: Muscidae) pupae on the growth performance and the resistance against bacterial pathogen in red sea bream (Pagrus major) (Perciformes: Sparidae). Appl Entomol Zool. (2015) 50:213–21. doi: 10.1007/s13355-015-0325-z
46. Kim B, Kim HR, Lee S, Baek Y-C, Jeong JY, Tae Bang H, et al. Effects of dietary inclusion level of microwave-dried and press-defatted black soldier fly (Hermetia illucens) larvae meal on carcass traits and meat quality in broilers. Animals. (2021) 11:665. doi: 10.3390/ani11030665
47. Reyes M, Rodríguez M, Montes J, Barroso FG, Fabrikov D, Morote E, et al. Nutritional and growth effect of insect meal inclusion on seabass (Dicentrarchuss labrax) feeds. Fishes. (2020) 5:16. doi: 10.3390/fishes5020016
48. Saleh HHE. Effect of feeding on fresh (wet) housefly maggots (Musca domestica) with or without artificial diet on water quality and growth rates of African catfish (Clarias gariepinus Burchell, 1822) fry under laboratory conditions. J Zool Res. (2020) 2:15–22. doi: 10.30564/jzr.v2i2.2053
49. Schiavone A, De Marco M, Martínez S, Dabbou S, Renna M, Madrid J, et al. Nutritional value of a partially defatted and a highly defatted black soldier fly larvae (Hermetia illucens L.) meal for broiler chickens: apparent nutrient digestibility, apparent metabolizable energy and apparent ileal amino acid digestibility. J Anim Sci Biotechnol. (2017) 8:1–9. doi: 10.1186/s40104-017-0181-5
50. Commander T, Anankware JP, Royal OO, Obeng-Ofori D. Econometrics of domestication of the African Palm Weevil (Rhynchophorus phoenicis F.) production as small-scale business in Ghana. In: Edible Insects. (2019).
51. Ngoka BM, Kioko EN, Raina SK, Mueke JM, Kimbu DM. Semi-captive rearing of the African wild silkmoth Gonometa postica (Lepidoptera: Lasiocampidae) on an indigenous and a non-indigenous host plant in Kenya. Int J Trop Insect Sci. (2008) 27:183–90. doi: 10.1017/S1742758407883160
52. Godwin R. If We Want to Save the Planet, the Future of Food is Insects. The Observer Food (2021). Available online at: https://www.theguardian.com/food/2021/may/08/if-we-want-to-save-the-planet-the-future-of-food-is-insects (accessed June 20, 2021).
53. Meyer-Rochow VB, Chakravorty J. Notes on entomophagy and entomotherapy generally and information on the situation in India in particular. Appl Entomol Zool. (2013) 48:105–12. doi: 10.1007/s13355-013-0171-9
54. Defoliart GR. INSECTS AS FOOD: why the western attitude is important. Annu Rev Entomol. (1999) 44:21–50. doi: 10.1146/annurev.ento.44.1.21
55. Ramos-Elorduy J. Threatened edible insects in Hidalgo, Mexico and some measures to preserve them. J Ethnobiol Ethnomed. (2006) 2:1–10. doi: 10.1186/1746-4269-2-51
56. Payne CLR. Wild harvesting declines as pesticides and imports rise: the collection and consumption of insects in contemporary rural Japan. J Insects as Food Feed. (2014) 1:57–65. doi: 10.3920/JIFF2014.0004
57. van Huis A, Oonincx DGAB. The environmental sustainability of insects as food and feed. A review. Agron Sustain Dev. (2017) 37:43. doi: 10.1007/s13593-017-0452-8
58. Hlongwane ZT, Slotow R, Munyai TC. Nutritional composition of edible insects consumed in africa: a systematic review. Nutrients. (2020) 12:1–28. doi: 10.3390/nu12092786
59. Rumpold BA, Schlüter OK. Nutritional composition and safety aspects of edible insects. Mol Nutr Food Res. (2013) 57:802–23. doi: 10.1002/mnfr.201200735
60. Omotoso OT, Adedire CO. Nutrient composition, mineral content and the solubility of the proteins of palm weevil, Rhynchophorus phoenicis F. (Coleoptera: Curculionidae). J Zhejiang Univ Sci B. (2007) 8:318–22. doi: 10.1631/jzus.2007.B0318
61. Awobusuyi TD, Siwela M, Pillay K. Nutritional composition of insect types most commonly consumed by the Olugboja Community of Ondo State, Nigeria. Int J Trop Insect Sci. (2021) 41:2975–82. doi: 10.1007/s42690-021-00483-x
62. Smets R, Verbinnen B, Van De Voorde I, Aerts G, Claes J, Van Der Borght M. Sequential extraction and characterisation of lipids, proteins, and chitin from black soldier fly (Hermetia illucens) larvae, prepupae, and pupae. Waste Biomass Valoriz. (2020) 11:6455–66. doi: 10.1007/s12649-019-00924-2
63. Danieli PP, Lussiana C, Gasco L, Amici A, Ronchi B. the effects of diet formulation on the yield, proximate composition, and fatty acid profile of the black soldier fly (Hermetia illucens L.) prepupae intended for animal feed. Animals. (2019) 9:178. doi: 10.3390/ani9040178
64. Hoc B, Francis F, Carpentier J, Mostade L, Blecker C, Purcaro G, et al. ω3-enrichment of Hermetia illucens (L. 1758) prepupae from oilseed byproducts. J Saudi Soc Agric Sci. (2021) 20:155–63. doi: 10.1016/j.jssas.2021.01.001
65. St-Hilaire S, Cranfill K, McGuire MA, Mosley EE, Tomberlin JK, Newton L, et al. Fish offal recycling by the black soldier fly produces a foodstuff high in omega-3 fatty acids. J World Aquac Soc. (2007) 38:309–13. doi: 10.1111/j.1749-7345.2007.00101.x
66. Payne CLR, Scarborough P, Rayner M, Nonaka K. A systematic review of nutrient composition data available for twelve commercially available edible insects, and comparison with reference values. Trends Food Sci Technol. (2016) 47:69–77. doi: 10.1016/j.tifs.2015.10.012
67. AOAC. Official Methods of Analysis: Association of Official Analytical Chemists. 15th ed. Arlington: AOAC (1990).
68. Van Soest PJ, Robertson JB, Lewis BA. Methods for dietary fiber, neutral detergent fiber, and nonstarch polysaccharides in relation to animal nutrition. J Dairy Sci. (1991) 74:3583–97. doi: 10.3168/jds.S0022-0302(91)78551-2
69. Cheseto X, Baleba SBS, Tanga CM, Kelemu S, Torto B. Chemistry and sensory characterization of a bakery product prepared with oils from African edible insects. Foods. (2020) 9:800. doi: 10.3390/foods9060800
70. Christie WW. Preparation of ester derivatives of fatty acids for chromatographic analysis. in Advances in Lipid Methodology. Dundee: Oily Press. (1993). p. 69–111.
71. R Core Team. R: A Language Environment for Statistical Computing. Vienna: R Foundation for Statistical Computing (2021). Available online at: https://www.r-project.org/. (accessed June 01, 2021).
72. RStudio Team. RStudio: Integrated Development Environment for R. Boston, MA: RStudio, PBC (2021).
73. Dinno A. Package ‘dunn.test’. CRAN Repos (2017). p. 1–7. Available online at: https://cran.r-project.org/web/packages/dunn.test/dunn.test.pdf (accessed January 18, 2020).
74. Wickham H,. ggplot2: Elegant Graphics for Data Analysis. (2016). Available online at: https://ggplot2-book.org/ (accessed January 18, 2020). doi: 10.1007/978-3-319-24277-4
75. WHO/FAO/UNU. Protein and Amino Acid Requirements in Human Nutrition: Report of a Joint FAO/WHO/UNU Expert Consultation. Geneva: WHO/FAO/UNU (2007).
76. Anankware JP, Osekre EA, Obeng-Ofori D, Khamala CM. Identification and classification of common edible insects in Ghana. Int J Entomol Res. (2016) 1:33–9. doi: 10.3920/JIFF2016.0007
77. Xiaoming C, Ying F, Hong Z, Zhiyong C. Review of the nutritive value of edible insects. In: Edible Forest Insects: Humans Bite Back. Bangkok: FAO. (2010). p. 85–92.
78. Yeung SE, Hilkewich L, Gillis C, Heine JA, Fenton TR. Protein intakes are associated with reduced length of stay: a comparison between Enhanced Recovery After Surgery (ERAS) and conventional care after elective colorectal surgery. Am J Clin Nutr. (2017) 106:44–51. doi: 10.3945/ajcn.116
79. Cuesta SM, Rahman SA, Furnham N, Thornton JM. The classification and evolution of enzyme function. Biophys J. (2015) 109:1082–6. doi: 10.1016/j.bpj.2015.04.020
80. Li P, Yin Y-L, Li D, Kim SW, Wu G. Amino acids and immune function. Br J Nutr. (2007) 98:237–52. doi: 10.1017/S000711450769936X
81. Semba RD. The rise and fall of protein malnutrition in global health. Ann Nutr Metab. (2016) 69:79–88. doi: 10.1159/000449175
82. Hepher B, Sandbank S. The effect of phosphorus supplementation to common carp diets on fish growth. Aquaculture. (1984) 36:323–32. doi: 10.1016/0044-8486(84)90325-9
83. Wiesmann D, Scheid H, Pfeffer E. Water pollution with phosphorus of dietary origin by intensively fed rainbow trout (Salmo gairdneri Rich.). Aquaculture. (1988) 69:263–70. doi: 10.1016/0044-8486(88)90334-1
84. Barker D, Fitzpatrick MP, Dierenfeld ES. Nutrient composition of selected whole invertebrates. Zoo Biol. (1998) 17:123–34.3.
85. Finke MD. Estimate of chitin in raw whole insects. Zoo Biol. (2007) 26:105–15. doi: 10.1002/zoo.20123
86. Chen X, Feng Y, Chen Z. Common edible insects and their utilization in China. Entomol Res. (2009) 39:299–303. doi: 10.1111/j.1748-5967.2009.00237.x
87. Mensink R, Katan M. Effect of a diet enriched with monounsaturated or polyunsaturated fatty acids on levels of low-density and high-density lipoprotein cholesterol in healthy women and men. N Engl J Med. (1989) 321:436–41. doi: 10.1056/NEJM198908173210705
88. Whetsell MS, Division WVU, Rayburn EB, Lozier JD, Service WVUE. Human Health Effects of Fatty Acids in Beef. (2003). Available online at: https://extension.wvu.edu/files/d/56abd724-9154-409a-8090-80ae37ff5b22/human-health-effects-of-fattty-acids-in-beef.pdf (accessed July 27, 2021).
89. Te Morenga L, Montez JM. Health effects of saturated and trans-fatty acid intake in children and adolescents: systematic review and meta-analysis. PLoS ONE. (2017) 12:e0186672. doi: 10.1371/journal.pone.0186672
90. Tocher DR. Fatty acid requirements in ontogeny of marine and freshwater fish. Aquac Res. (2010) 41:717–32. doi: 10.1111/j.1365-2109.2008.02150.x
91. Menendez JA, Vellon L, Colomer R, Lupu R. Oleic acid, the main monounsaturated fatty acid of olive oil, suppresses Her-2/neu (erb B-2) expression and synergistically enhances the growth inhibitory effects of trastuzumab (HerceptinTM) in breast cancer cells with Her-2/neu oncogene amplification. Ann Oncol. (2005) 16:359–71. doi: 10.1093/annonc/mdi090
92. National National Center for Chronic Disease Prevention Health Promotion: Division of Nutrition Physical Activity Obesity. Low-Energy-Dense Foods and Weight Management: Cutting Calories While Controlling Hunger (2011). Available online at: https://www.cdc.gov/nccdphp/dnpa/nutrition/pdf/r2p_energy_density.pdf
93. Savolainen V, Clottey VA, Doubi BTS, Konan JL, Quain M, Bezeng BS, et al. Systems thinking creates opportunities for a circular economy and sustainable palm agriculture in Africa. Curr Res Environ Sustain. (2020) 1:31–4. doi: 10.1016/j.crsust.2020.05.001
94. Vaz FM, Wanders RJA. Carnitine biosynthesis in mammals. Biochem J. (2002) 361:417–29. doi: 10.1042/bj3610417
95. Griffith RS, Walsh DE, Myrmel KH, Thompson RW, Behforooz A. Success of L-lysine therapy in frequently recurrent herpes simplex infection. Dermatology. (1987) 175:183–90. doi: 10.1159/000248823
96. Janssen RH, Lambertus AM. Nitrogen-to-protein conversion factors for three edible insects: tenebrio molitor, alphitobius diaperinus, and hermetia illucens. Agric Food Chem. (2017) 65:2275–8. doi: 10.1021/acs.jafc.7b00471
97. Mariotti F, Tomé D, Patureau Mirand P, Ois Mariotti F, Tométom D. Converting nitrogen into protein-beyond 6.25 and Jones' factors. Crit Rev Food Sci Nutr. (2008) 48:177–84. doi: 10.1080/10408390701279749
98. Wong C-Y, Rosli S-S, Uemura Y, Ho YC, Leejeerajumnean A, Kiatkittipong W, et al. Potential protein and biodiesel sources from black soldier fly larvae: insights of larval harvesting instar and fermented feeding medium. Energies. (2019) 12:1570. doi: 10.3390/en12081570
99. Barroso FG, Sánchez-Muros MJ, Rincón MÁ, Rodriguez-Rodriguez M, Fabrikov D, Morote E, et al. Production of n-3-rich insects by bioaccumulation of fishery waste. J Food Compos Anal. (2019) 82:103237. doi: 10.1016/j.jfca.2019.103237
100. Campbell M, Ortuño J, Stratakos AC, Linton M, Corcionivoschi N, Elliott T, et al. Impact of thermal and high-pressure treatments on the microbiological quality and in vitro digestibility of black soldier fly (Hermetia illucens) larvae. Animals. (2020) 10:682. doi: 10.3390/ani10040682
101. González CM, Garzón R, Rosell CM. Insects as ingredients for bakery goods. A comparison study of H. illucens, A. domestica and T. molitor flours. Innov Food Sci Emerg Technol. (2019) 51:205–10. doi: 10.1016/j.ifset.2018.03.021
102. Liu X, Liu X, Yao Y, Qu X, Chen J, Xie K, et al. Effects of different levels of Hermetia illucens larvae meal on performance, egg quality, yolk fatty acid composition and oxidative status of laying hens. Ital J Anim Sci. (2021) 20:256–66. doi: 10.1080/1828051X.2021.1878946
103. Mba ARF, Kansci G, Viau M, Hafnaoui N, Meynier A, Demmano G, et al. Lipid and amino acid profiles support the potential of Rhynchophorus phoenicis larvae for human nutrition. J Food Compos Anal. (2017) 60:64–73. doi: 10.1016/j.jfca.2017.03.016
104. Beniers JJA, Graham RI. Effect of protein and carbohydrate feed concentrations on the growth and composition of black soldier fly (Hermetia illucens) larvae. J Insects as Food Feed. (2019) 5:193–9. doi: 10.3920/JIFF2018.0001
105. Chia SY, Tanga CM, Osuga IM, Cheseto X, Ekesi S, Dicke M, et al. Nutritional composition of black soldier fly larvae feeding on agro-industrial by-products. Entomol Exp Appl. (2020) 168:472–81. doi: 10.1111/eea.12940
106. Matthäus B, Piofczyk T, Katz H, Pudel F. Renewable resources from insects: exploitation, properties, and refining of fat obtained by cold-pressing from Hermetia illucens (Black Soldier Fly) larvae. Eur J Lipid Sci Technol. (2018) 121:1800376. doi: 10.1002/ejlt.201800376
107. Basto A, Matos E, Valente LMP. Nutritional value of different insect larvae meals as protein sources for European sea bass (Dicentrarchus labrax) juveniles. Aquaculture. (2020) 521:735085. doi: 10.1016/j.aquaculture.2020.735085
108. Liland NS, Biancarosa I, Araujo P, Biemans D, Bruckner CG, Waagbø R, et al. Modulation of nutrient composition of black soldier fly (Hermetia illucens) larvae by feeding seaweed-enriched media. PLoS ONE. (2017) 12:e0183188. doi: 10.1371/journal.pone.0183188
109. Scala A, Cammack JA, Salvia R, Scieuzo C, Franco A, Bufo SA, et al. Rearing substrate impacts growth and macronutrient composition of Hermetia illucens (L.) (Diptera: Stratiomyidae) larvae produced at an industrial scale. Sci Rep. (2020) 10:19448. doi: 10.1038/s41598-020-76571-8
110. Do S, Koutsos L, Utterback PL, Parsons CM, de Godoy MRC, Swanson KS. Nutrient and AA digestibility of black soldier fly larvae differing in age using the precision-fed cecectomized rooster assay. J Anim Sci. (2020) 98:1–10. doi: 10.1093/jas/skz363
111. Moyo M, Nsahlai I. Consequences of increases in ambient temperature and effect of climate type on digestibility of forages by ruminants: a meta-analysis in relation to global warming. Animals. (2021) 11:1–17. doi: 10.3390/ani11010172
112. Rojas-Downing MM, Nejadhashemi AP, Harrigan T, Woznicki SA. Climate change and livestock: impacts, adaptation, and mitigation. Clim Risk Manag. (2017) 16:145–63. doi: 10.1016/j.crm.2017.02.001
113. Dobermann D, Field LM, Michaelson L V. Impact of heat processing on the nutritional content of Gryllus bimaculatus (black cricket). Nutr Bull. (2019) 44:116–22. doi: 10.1111/nbu.12374
114. Akullo J, Nakimbugwe D, Obaa BB, Okwee-Acai J, Agea JG. Development and quality evaluation of crackers enriched with edible insects. Int Food Res J. (2018) 25:1592–9. Available online at: http://www.ifrj.upm.edu.my/25%20(04)%202018/(36).pdf
115. Kaya C, Generalovic TN, Ståhls G, Hauser M, Samayoa AC, Nunes-Silva CG, et al. Global population genetic structure and demographic trajectories of the black soldier fly, Hermetia illucens. BMC Biol. (2021) 19:94. doi: 10.1186/s12915-021-01029-w
116. Ståhls G, Meier R, Sandrock C, Hauser M, Šašić Zorić L, Laiho E, et al. The puzzling mitochondrial phylogeography of the black soldier fly (Hermetia illucens), the commercially most important insect protein species. BMC Evol Biol. (2020) 20:60. doi: 10.1186/s12862-020-01627-2
117. Krafsur ES, Bryant NL, Marquez JG, Griffiths NT. Genetic distances among North American, British, and West African house fly populations (Musca domestica L.). Biochem Genet. (2000) 38:275–84. doi: 10.1023/a:1002010632647
118. Marquez JG, Krafsur ES. Gene flow among geographically diverse housefly populations (Musca domestica L.): a worldwide survey of mitochondrial diversity. Am Genet Assoc. (2002) 93:254–9. doi: 10.1093/jhered/93.4.254
119. Palombo V, Milanesi M, Sgorlon S, Capomaccio S, Mele M, Nicolazzi E, et al. Genome-wide association study of milk fatty acid composition in Italian Simmental and Italian Holstein cows using single nucleotide polymorphism arrays. J Dairy Sc. (2018) 101:11004–19. doi: 10.3168/jds.2018-14413
120. Schennink A, Stoop WM, Visker MHPW, Heck JML, Bovenhuis H, Van Der Poel JJ, et al. DGAT1 underlies large genetic variation in milk-fat composition of dairy cows. Anim Genet. (2007) 38:467–73. doi: 10.1111/j.1365-2052.2007.01635.x
121. de Haas Y, Veerkamp RF, de Jong G, Aldridge MN. Selective breeding as a mitigation tool for methane emissions from dairy cattle. Animal. (2021). doi: 10.1016/j.animal.2021.100294. [Epub ahead of print].
122. Gjedrem T, Robinson N, Rye M. The importance of selective breeding in aquaculture to meet future demands for animal protein: a review. Aquaculture. (2012) 350–3:117–29. doi: 10.1016/j.aquaculture.2012.04.008
123. De Verdal H, Komen H, Quillet E, Benzie JAH, Vandeputte M. Improving feed efficiency in fish using selective breeding: a review. Rev Aquac. (2018) 10:833–51. doi: 10.1111/raq.12202
124. Williams JL. The use of marker-assisted selection in animal breeding and biotechnology. OIE Rev Sci Tech. (2005) 24:379–91. doi: 10.20506/rst.24.1.1571
125. Generalovic TN, Mccarthy SA, Warren IA, Wood JMD, Torrance J, Sims Y, et al. A high-quality, chromosome-level genome assembly of the Black Soldier Fly (Hermetia illucens L.). G3. (2021) 11:jkab085. doi: 10.1093/g3journal/jkab085
Keywords: entomophagy, nutrition, food security, Hermetia illucens, Musca domestica, Rhynchophorus phoenicis, Cirina butyrospermi, Macrotermes bellicosus
Citation: Anankware JP, Roberts BJ, Cheseto X, Osuga I, Savolainen V and Collins CM (2021) The Nutritional Profiles of Five Important Edible Insect Species From West Africa—An Analytical and Literature Synthesis. Front. Nutr. 8:792941. doi: 10.3389/fnut.2021.792941
Received: 11 October 2021; Accepted: 09 November 2021;
Published: 03 December 2021.
Edited by:
Simone Belluco, Istituto Zooprofilattico Sperimentale delle Venezie (IZSVe), ItalyReviewed by:
Kehinde Ademolu, Federal University of Agriculture, Abeokuta, NigeriaCopyright © 2021 Anankware, Roberts, Cheseto, Osuga, Savolainen and Collins. This is an open-access article distributed under the terms of the Creative Commons Attribution License (CC BY). The use, distribution or reproduction in other forums is permitted, provided the original author(s) and the copyright owner(s) are credited and that the original publication in this journal is cited, in accordance with accepted academic practice. No use, distribution or reproduction is permitted which does not comply with these terms.
*Correspondence: Jacob P. Anankware, YW5hbmt3YXJlQHlhaG9vLmNvbQ==
†These authors have contributed equally to this work and share first authorship
‡ORCID:Jacob P. Anankware orcid.org/0000-0002-9245-6501
Benjamin J. Roberts orcid.org/0000-0002-7550-3388
Xavier Cheseto orcid.org/0000-0002-9779-0357
Vincent Savolainen orcid.org/0000-0001-5350-9984
C. M. Collins orcid.org/0000-0003-0966-5343
Disclaimer: All claims expressed in this article are solely those of the authors and do not necessarily represent those of their affiliated organizations, or those of the publisher, the editors and the reviewers. Any product that may be evaluated in this article or claim that may be made by its manufacturer is not guaranteed or endorsed by the publisher.
Research integrity at Frontiers
Learn more about the work of our research integrity team to safeguard the quality of each article we publish.