- 1Risk Assessment Laboratory of Feed Derived Factors to Animal Product Quality Safety of Ministry of Agriculture and Rural Affairs, National Engineering Research Center of Biological Feed, Institute of Feed Research, Chinese Academy of Agricultural Sciences, Beijing, China
- 2Animal Nutrition, Nutrition and Care, Evonik (China) Co., Ltd., Beijing, China
Bacillus subtilis (B. subtilis) as in-feed probiotics is a potential alternative for antibiotic growth promoters (AGP) in the poultry industry. The current study investigated the effects of B. subtilis on the performance, immunity, gut microbiota, and intestinal barrier function of broiler chickens. A 42-day feeding trial was conducted with a total of 600 1-day-old Arbor Acres broilers with similar initial body weight, which was randomly divided into one of five dietary treatments: the basal diet (Ctrl), Ctrl + virginiamycin (AGP), Ctrl + B. subtilis A (BSA), Ctrl + B. subtilis B (BSB), and Ctrl + B. subtilis A + B (1:1, BSAB). The results showed significantly increased average daily gain in a step-wise manner from the control, B. subtilis, and to the AGP groups. The mortality rate of the B. subtilis group was significantly lower than the AGP group. The concentrations of serum immunoglobulin (Ig) G (IgG), IgA, and IgM in the B. subtilis and AGP groups were higher than the control group, and the B. subtilis groups had the highest content of serum lysozyme and relative weight of thymus. Dietary B. subtilis increased the relative length of ileum and the relative weight of jejunum compared with the AGP group. The villus height (V), crypt depth (C), V/C, and intestinal wall thickness of the jejunum in the B. subtilis and AGP groups were increased relative to the control group. Dietary B. subtilis increased the messenger RNA (mRNA) expression of ZO-1, Occludin, and Claudin-1, the same as AGP. The contents of lactic acid, succinic acid, and butyric acid in the ileum and cecum were increased by dietary B. subtilis. Dietary B. subtilis significantly increased the lactobacillus and bifidobacteria in the ileum and cecum and decreased the coliforms and Clostridium perfringens in the cecum. The improved performance and decreased mortality rate observed in the feeding trial could be accrued to the positive effects of B. subtilis on the immune response capacity, gut health, and gut microflora balance, and the combination of two strains showed additional benefits on the intestinal morphology and tight junction protein expressions. Therefore, it can be concluded that dietary B. subtilis A and B could be used as alternatives to synthetic antibiotics in the promotion of gut health and productivity index in broiler production.
Introduction
Antibiotics have been widely used as a growth promoter and also to enhance the immunocompetence of birds against infectious diseases (1). Currently, the global trend in animal production is toward a reduction or ban on the use of feed antibiotics for growth [antibiotic growth promoter (AGP)] and an increase in the application of non-antibiotic approaches that can provide similar benefits. This is accrued to the fact that the widespread use of antibiotics over 50 years has led to the emergence of resistant bacteria and drug residues in animal products (2–4). In the context of growing consumer preference for antibiotic-free meat products, researchers in livestock production and poultry sectors have focused on finding alternatives to replace synthetic antibiotics used in most ongoing therapeutic regimes. There is an interest to characterize probiotics as a kind of viable alternatives that can promote the growth and health status of poultry through multiple ways/mechanisms(5–7).
Despite the large amount of microorganisms serving as probiotics in poultry production, the form of supplemental probiotics through the hostile environment such as low pH value and high concentration of bile salt within the gastrointestinal tract is a severe challenge for their survival (8). As a result, spore-forming bacteria such as Bacillus subtilis (B. subtilis) are gaining interest in animal health-related functional additive research due to their high tolerance and survivability under hostile environments in the gastrointestinal tract (9–11). Obviously, B. subtilis when applied in feed does not lose its viability due to its high stability and extended shelf life, hence a comparative advantage (10). Supplemental B. subtilis in poultry diets has many beneficial claims, including immune-modulation, enhanced nutrient digestibility, along with improvements in gut health, immunity, and growth performance in animals (12–14). However, many properties of probiotic bacteria vary as a function of strain (15). With respect to B. subtilis, its probiotic effects are highly strain-specific, and the underlying mechanisms of action remain largely elusive (16). It has been reported that the effects of dietary B. subtilis supplementation on growth performance and intestinal physiology in broilers were markedly strain-dependent (17, 18), hence the need for continuous studies on the various strains of B. subtilis to understand their mechanisms of action in these animals.
Bacillus subtilis A and B were selected as two potential probiotic strains through a multi-parameter selection process for their ability to remain viable in feed and through the harsh conditions of the upper gastrointestinal tract. However, far less is known about the effects of these two strains on broilers and their potential roles to be AGP substitutes. Therefore, this study aimed to evaluate the individual or combined effect of two strains of B. subtilis A and B on the growth performance, serum immunity, gut microbiota, and intestinal barrier function of broiler chickens.
Materials and Methods
Experimental Design and Bird Management
A total of 600 newly hatched male Arbor Acres (AA) broiler chicks with an average body weight (BW) of 40.09 g were obtained from a local hatchery and assigned into five dietary treatments in a randomized complete block design with 10 replicates per treatment. Each replicate contained 12 chicks (half male and half female) housed in two cages with male and female apart. The 42-day trial spanned three phases including the starter period (day 0–14), grower period (day 15–28), and finisher period (day 29–42). Three basal diets (cold pellet form) were formulated according to the nutrient requirements of AMINOChick®2.0 and the Chinese Feeding Standard of Chicken (NY/T, 33-2018), and their ingredient composition and nutrient levels are shown in Table 1. The control group was fed basal diets. The AGP group was fed basal diets supplemented with 15 mg/kg virginiamycin. Three probiotics groups were fed basal diets supplemented with 500 mg/kg B. subtilis A (BSA) (2E9 CFU/g), 500 mg/kg B. subtilis B (BSB) (2E9 CFU/g), or 500 mg/kg mixture of BSA and BSB (1:1, named as BSAB), respectively. Diet samples collected from all the treatments and phases were sent to Evonik Operations GmbH for the proximate and spore count analysis. The pre-products are both generally recognized as safe (GRAS) under the Association of American Feed Control Officials (AAFCO) definition 36.14 and contain a guaranteed minimum of 2 × 109 CFU/g.
All the birds were raised in wire floor cages (cage size, 110 × 100 × 55 cm) in a three-level battery under environmentally controlled room conditions in the Nankou CAAS experimental base (Beijing, China). All the management was in accordance with the AA broiler management guide. A continuous incandescent white light was provided for the first 3 days, and then a 23L:1D lighting regime was maintained throughout the rest feeding trial. The room temperature was maintained at 33°C for the first week and then reduced by 3°C per week until it reached 24°C. Fresh feed and water on a daily basis were available ad libitum through individual feeders and drinkers in each cage. The chicks were vaccinated with inactivated Newcastle disease vaccine on days 7 and 21 and inactivated infectious bursal disease vaccine on days 14 and 28. The vaccines were purchased from Shanghai Haili Biotechnology Co., LTD (Shanghai, China). During the trial, the mortality of birds was recorded daily, and the feed consumption of the corresponding replicate was adjusted with their body weight accordingly. The feed intake (FI), BW, and mortality of each replicate were recorded every 2 weeks. The average daily feed intake (ADFI), average daily gain (ADG), and feed conversion ratio (FCR) were calculated based on FI and BW.
Data and Sample Collection
On days 28 and 42, one chick weighing close to the average weight of the replicate was selected for sample collection after 12 h fasting. About 5 ml of blood was collected from the wing vein using a vacutainer tube, kept in a slanting position for 30 min, and then centrifuged at 3,000 rpm/min for 15 min at 4°C. The obtained serum samples were stored in 2 ml plastic vials at −20°C, pending for ELISA analysis.
Subsequently, the selected birds were euthanized and then dissected under aseptic conditions. Immune organs including the spleen, thymus, and bursa of Fabricius of the birds were weighed and their relative weight was calculated as the ratio of organ weight (g) to BW (kg). The whole duodenum, jejunum, ileum, and ceca were moved free of the mesentery and immediately placed on ice for sampling. About 4 g of digesta sample from the ileum and cecum were collected and immediately snap-frozen in liquid nitrogen followed by storage in −80°C until the short-chain fatty acids (SCFA) and microbiota analysis. Then, the relative index of the intestinal length (length/BW × 100%, cm/g) and weight (weight/BW × 100%, g/g) of the duodenum, jejunum, ileum, and cecum were calculated, respectively. About 3 cm of tissues from the duodenum (medial portion), jejunum (medial portion posterior to the bile ducts and anterior to Meckel's diverticulum), and ileum (medial portion posterior to Meckel's diverticulum and anterior to the ileocecal junction) were cut off gently in duplicate, one fixed in 10% formalin for histomorphology, the other immediately snap-frozen in liquid nitrogen and stored in −80°C until mRNA extraction.
Histology and Histomorphology Analysis of the Intestine
The fixed intestinal samples were dehydrated, embedded in paraffin wax, cut into serial 5 μm sections, and stained by hematoxylin and eosin. Histological sections were examined by a microscope coupled with a Microcomp integrated digital imaging analysis system (Nikon Eclipse 80i, Nikon Co., Tokyo, Japan). Three orientated sections cutting vertically from the villus enterocytes to the muscularis mucosa were selected from each sample and the measurements were carried out as follows (19). The vertical distance from the villus tip to villus–crypt junction level was taken as the intestinal villus height (VH), and the vertical distance from the villus-crypt junction to the lower limit of the crypt as the crypt depth (CD). Ten loci per section were selected for the measurement of the VH, CD, and intestinal wall thickness (IWT). The ratio of VH/CD was calculated as V/C.
RNA Extraction and Quantitative Real Time-PCR
The total DNA of the microbe from the intestinal digesta and the total RNA from the intestinal tissues were extracted using TRIZOL reagent (Invitrogen, Carlsbad, CA, USA) according to the instructions of the manufacturer. Samples of DNA and RNA were determined for integrity by 1% agarose gel electrophoresis and for concentration and purity using a NanoDrop ND-1000 spectrophotometer (Thermo Fisher Scientific, DE, USA). Then the RNA samples were treated with DNase I (Cwbio IT Group, Beijing, China) and converted into complementary DNA (cDNA) using a reverse transcription kit (Vazyme Biotech, Nanjing, China). An iCycler iQ5 multicolor RT-PCR detection system (Bio-Rad Laboratories, Hercules, CA, USA) and a RealMasterMix-SYBR Green kit [ChamQ SYBR Color qPCR Master Mix (2 ×), Vazyme, Nanjing] were used for the determination of gene expression according to the instructions of the manufacturer. The primers used in this study are listed in Table 2. The thermal cycling conditions of qRT-PCR were as follows: 95°C for 5 min; 40 cycles of 95°C for 10 s, 60°C for 30 s. The results of the absolute qRT-PCR of the microbe DNA were expressed as copies/g. The relative gene expression of the tissue samples was calculated using the 2−ΔΔCt method.
Chemical Analysis
The serum lysozyme activity was measured using Micrococcus lysodeikticus cells as a substrate. The serum immunoglobulin (Ig) A (IgA), IgG, and IgM were analyzed by colorimetric method using commercial kits (H108, H106, H109, Nanjing Jiancheng Bioengineering Institute, Nanjing, China) according to the instructions of the manufacturer. The concentrations of lactic acid, succinic acid, and SCFA (formic acid, acetic acid, propionic acid, butyric acid, iso-butyric acid, valeric acid, isovaleric acid) in the ileal and cecal digesta were measured as previously described (20) using the Dionex ICS-3000 Ion Chromatography System (ThermoFisher Scientific Inc., Waltham, MA, USA).
Statistical Analysis
The data of the B. subtilis group were generated from the data of the three groups, BSA, BAB, and BSAB, where six replicates in each treatment were averagely divided as two replicates of the B. subtilis group. All the data were subjected to a one-way ANOVA procedure for a completely randomized design using the General Linear Mode (GLM) procedures of SAS 9.2 (SAS Inst. Inc., Cary, NC, USA). The differences among the treatments were separated by Duncan's multiple range tests. P ≤ 0.05 was considered significant.
Results
Growth Performance
The growth performance of broiler chickens is shown in Table 3. On days 14 and 28, the BW of the birds fed with AGP was significantly higher (P ≤ 0.05) than those fed with the control and B. subtilis diets. At the end of the trial, the birds in the AGP group showed higher (P ≤ 0.05) BW than the B. subtilis groups whose BW was higher (P ≤ 0.05) than the control group. During the trial, the BW of the broiler chickens was similar among the three B. subtilis treatments on days 14, 28, and 42. During the starter phase (day 1–14), the broiler chickens in the AGP group showed increased (P ≤ 0.05) ADG, ADFI, and mortality rate and decreased FCR relative to the B. subtilis group which is not different from the control group, and all of these indexes were similar among the three B. subtilis groups. During the grower phase (day 15–28), the ADG and ADFI of the AGP and B. subtilis groups were significantly higher (P ≤ 0.05) than the control group. Among the B. subtilis groups, the BSB group showed higher (P ≤ 0.05) FCR than the BSAB group, and both of them did not vary with that of the BSA group. In the finisher phase (day 29–42), the ADG of the broiler chickens in the AGP group was higher (P ≤ 0.05) than those in the B. subtilis and control groups. The FCR of the AGP group was decreased (P ≤ 0.05) as compared with the control and B. subtilis groups. No differences in ADG, ADFI, FCR, and mortality rate were observed among the three B. subtilis groups. During the whole trial period, day 1–42, the ADG of the broiler chickens showed a significant increase (P ≤ 0.05) in a stepwise manner from the control, B. subtilis, to the AGP groups. The birds in the B. subtilis group had higher (P ≤ 0.05) ADFI than the control group. The FCR of the AGP group was improved (P ≤ 0.05) relative to the control and B. subtilis groups. The mortality rate of the B. subtilis group was significantly (P ≤ 0.05) decreased as compared with the AGP group. There was no variation in growth performance indices among the broilers fed with diets supplemented with the three B. subtilis groups during the entire feeding phase.
The Relative Weight of Immune Organ and Serum Immunity
As shown in Figure 1, at day 28, all the three B. subtilis groups had higher (P ≤ 0.05) relative weight of thymus compared with the control and AGP groups. On day 42, the relative weights of the immune organs including the thymus, spleen, and bursa of fabricius were not influenced by the dietary treatments. The effects of the dietary treatments on the concentration of IgA, IgG, IgM, and lysozyme in the serum are shown in Table 4. On day 28, the level of serum IgG and IgA was higher (P ≤ 0.05) in the AGP and B. subtilis groups as compared with the control group, while no differences were observed among the B. subtilis groups. On day 42, the concentration of serum IgA in the B. subtilis and AGP groups was higher (P ≤ 0.05) compared with the control group. The B. subtilis group showed a higher (P ≤ 0.05) content of serum lysozyme than the control and AGP groups. The contents of serum IgA, IgG, and IgM did not differ among the three B. subtilis groups at day 42, but the concentration of serum lysozyme was higher (P ≤ 0.05) in the BSAB group than in the BSB group.
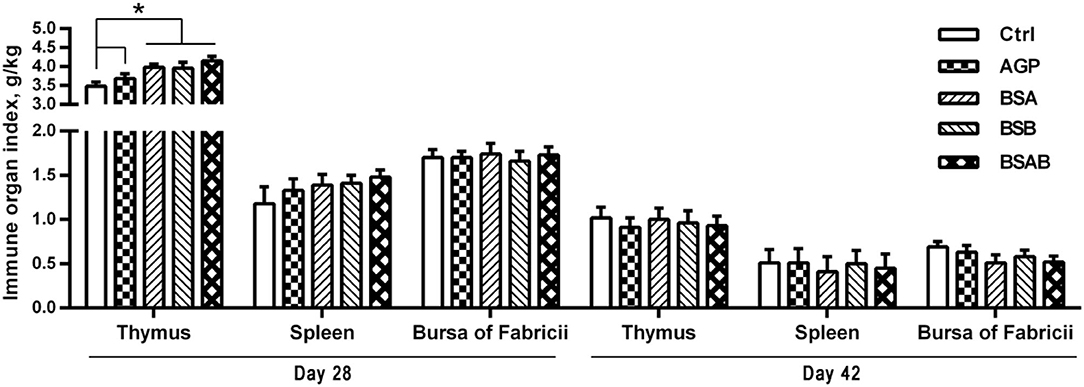
Figure 1. Effects of dietary Bacillus subtilis on the immune organ index of broiler chickens (n = 10, g/kg). ADD, additives; Ctrl, the control group; AGP, antibiotic growth promoter; BS, B. subtilis; BSA, B. subtilis A; BSB, B. subtilis B; BSAB, the mixture of BSA and BSB (1:1). *Means significant different (P < 0.05).
Intestinal Development
The relative length and weight of the duodenum, jejunum, ileum, and cecum are presented in Table 5. On day 28, the relative length of the duodenum and ileum of the B. subtilis group was longer (P ≤ 0.05) than those of the AGP group, while similar to the control group. The B. subtilis group showed increased (P ≤ 0.05) relative length of the cecum as compared with the control group. On day 42, the relative length of the ileum was significantly longer (P ≤ 0.05) in the B. subtilis and control groups than in the AGP group. As for the relative weight of the duodenum, jejunum, ileum, and cecum at days 28 and 42, only the relative weight of the jejunum in the B. subtilis group was greater (P ≤ 0.05) than the AGP group at day 28. The relative length and weight of the duodenum, jejunum, ileum, and cecum at days 28 and 42 did not differ between the three B. subtilis groups.
The results of the intestinal morphology are shown in Table 6. With respect to the duodenum, at day 28, the VH, V/C, and IWT were higher (P ≤ 0.05) in the B. subtilis group than in the control group. The CD of the AGP group increased (P ≤ 0.05) relative to the control group, while both of them were similar to the B. subtilis group. The CD of the BSB group was significantly deeper (P ≤ 0.05) than the BSA and BSAB groups. On day 42, the IWT of the AGP group was thicker (P ≤ 0.05) than the control group, while both of them were not different from the B. subtilis group. The VH, CD, V/C, and IWT did not differ between the three B. subtilis groups. As for jejunum, at day 28, the B. subtilis group showed increased (P ≤ 0.05) VH, V/C, and IWT relative to the control group, while it was similar with the AGP group. No differences in intestinal morphology were found between the three B. subtilis groups. On day 42, the CD and IWT of the B. subtilis and AGP groups were increased (P ≤ 0.05) relative to the control group. The VH and IWT of the BSAB group were higher (P ≤ 0.05) than the BSA and BSB groups. The CD of the BSB group was deeper (P ≤ 0.05) than the control group, but not different from the BSAB group. The V/C of the BSAB and control groups was bigger (P ≤ 0.05) than the BSB group. Regarding the ileum, no differences between the control, AGP, and B. subtilis groups were found for VH, CD, V/C, and IWT at days 28 and 42. The V/C of the BSAB group was greater (P ≤ 0.05) than the BSA group on day 28, and both of them were not significantly different from the BSB group. On day 42, the BSA and BSAB groups showed bigger (P ≤ 0.05) VH and V/C than the BSB group. The IWT of the BSAB group was thicker (P ≤ 0.05) than the BSB group.
The mRNA expressions of the genes encoding intestinal tight junction proteins in the jejunum including ZO1, ZO2, Occludin, Claudin 1, and Claudin 5 are shown in Figure 2. There were no differences between the three B. subtilis groups for these gene expressions. The B. subtilis and AGP groups showed higher (P ≤ 0.05) mRNA expression of ZO-1, Occludin, Claudin-1 than the control group.
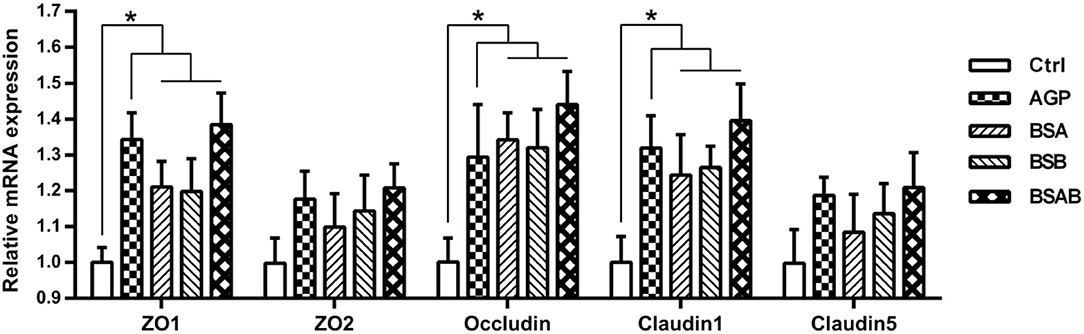
Figure 2. Effects of dietary B. subtilis on intestinal tight junction protein genes expressions in the jejunum of broiler chickens (n = 10). ADD, additives; Ctrl, the control group; AGP, antibiotic growth promoter; BS, B. subtilis; BSA, B. subtilis A; BSB, B. subtilis B; BSAB, the mixture of BSA and BSB (1:1). *Means significant difference between the groups (P < 0.05).
Composition of Organic Acid and Microbiota in Digesta
As shown in Table 7, the concentration of lactic acid and succinic acid in the ileal digesta of the B. subtilis group was higher (P ≤ 0.05) than the control and AGP groups. The B. subtilis group was found to have higher (P ≤ 0.05) content of propionic acid and butyric acid in the ileal digesta than the AGP group, while it is not different from the control group. The content of formic acid, acetic acid, and total SCFA did not differ between the control, AGP, and B. subtilis groups. Except that the BSB group showed higher (P ≤ 0.05) content of total SCFA than the BSA group, the concentration of other organic acids in the ileal digesta was similar between the three B. subtilis groups. With regard to the cecum, the concentration of lactic acid, succinic acid, formic acid, butyric acid, and isovaleric acid in the digesta of the B. subtilis group was higher (P ≤ 0.05) than the control and AGP groups. The B. subtilis group showed higher (P ≤ 0.05) content of isobutyric acid in the digesta than the AGP group, but similar with the control group. There are no differences in organic acids content in the digesta among the three B. subtilis groups.
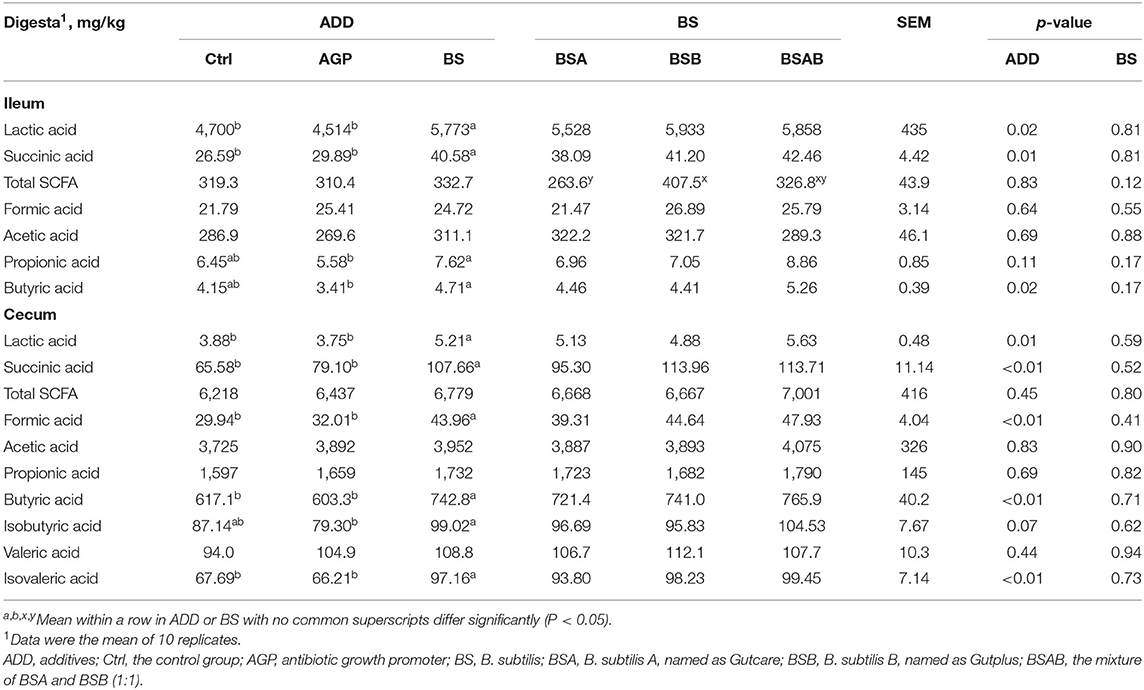
Table 7. Effects of dietary B. subtilis on short-chain fatty acid content in the ileum and cecum digesta of broiler chickens.
The effects of experimental treatments on intestinal microflora including Lactobacillus, Bifidobacteria, Coliforms, and Clostridium perfringens are presented in Table 8. For the ileum microflora, the amount of Lactobacillus in the B. subtilis group was higher (P ≤ 0.05) than the control and AGP groups, and the amount of Bifidobacteria in the B. subtilis group was higher (P ≤ 0.05) than the AGP group, and similar with the control group. No differences existed between the three B. subtilis groups about the content of microflora. For the cecum microflora, the amount of Lactobacillus and Bifidobacteria in the B. subtilis group was higher (P ≤ 0.05) than the AGP group, but no difference was found in the control group. The amounts of coliforms and Clostridium perfringens in the AGP and B. subtilis group were increased (P ≤ 0.05) than the control group. The number of coliforms in the BSAB group was higher (P ≤ 0.05) than the BSA group, and did not differ from the BSB group.
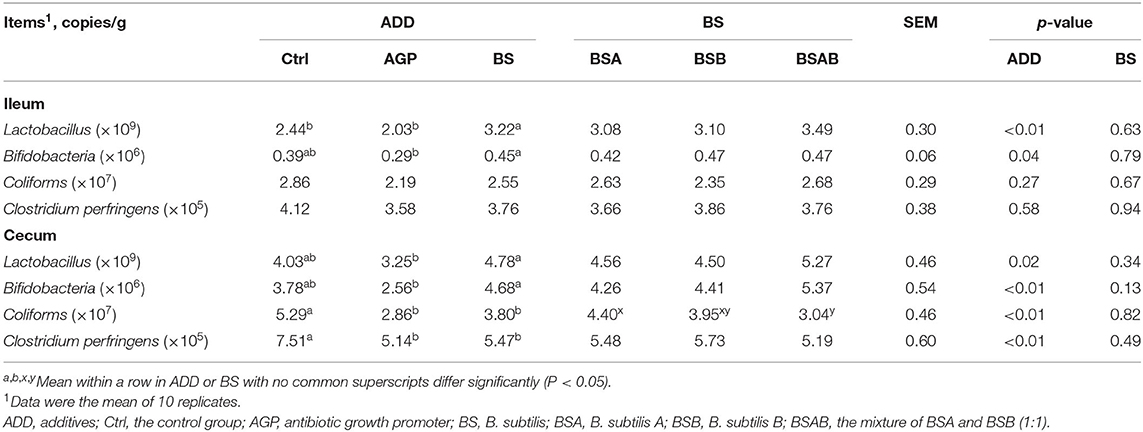
Table 8. Effects of dietary B. subtilis on ileum and cecum microbial populations of broiler chickens.
Discussion
Over the past decades, therapeutic regimes in animal production globally entail about 70% use of synthetic antibiotics, probably due to their ability to improve gut health, hence health status and production index. However, the wide use of antibiotics as growth promoters in food animals has raised a lot of public health concerns (21). Along with the increasing consumer awareness of antimicrobial resistance and food safety, the use of AGP in animal agriculture was successively banned or restricted by the European Union, South Korea, the United States, and China. Such a scenario may reduce the livestock productivity index and the health status of animals due to impaired gut health, but natural alternatives such as supplemental B. subtilis in diets could ameliorate these effects (14). The probiotic effects of B. subtilis for different animals are highly strain-specific because of the different characteristics of the gastrointestinal environment (16). Therefore, it becomes imperative to discover which strains of B. subtilis are effective for broiler production concerning growth performance and health response.
Virginiamycin, a well-established AGP, has been widely used in the poultry industry and its growth-promoting effects have already been demonstrated in various studies (22, 23). In the present study, virginiamycin was taken as a positive control to evaluate the effects of two strains of B. subtilis A and B, and their combination (1:1) on the growth performance, serum immunity, and intestinal health of broiler chickens. The significant growth-promoting effects of virginiamycin in broiler chickens were validated again in this study. Numerous reports showed that broiler chickens direct-fed with B. subtilis showed enhanced growth performance than those fed a basal diet and comparably with the AGP group (14, 17, 18, 24, 25). However, in this study, although dietary B. subtilis significantly increased the ADG of broiler chickens, the improvement on both ADG and FCR was not comparable to AGP. The group fed the combination of BSA and BSB showed similar improvement on the ADG of broiler chickens relative to the AGP group. It may be due to the different strains and doses of B. subtilis used (18, 26). Remarkably, it is worthy to note that dietary B. subtilis in our study significantly decreased the mortality rate of broiler chickens relative to the AGP group. It is consistent with previous reports that the strain of B. subtilis reduced the pathology and improved the performance of broilers with necrotic enteritis induced by Clostridium perfringens challenge (27–29), and BSB alleviated diarrhea severity and systemic inflammation and improved gut health and growth performance of weaned pigs infected with enterotoxigenic Escherichia coli F18 (30). Therefore, it can be deduced that B. subtilis A and B probably decreased mortality and enhanced performance via enhanced gut health.
The immunoglobulin levels (IgG, IgA, and IgM) and lysozyme activity in the serum are important indicators to evaluate the non-specific immunity status of the animal (31, 32). Probiotics have been demonstrated to be beneficial immunomodulators of mammals at both phenotypic and molecular levels (33, 34). In the present study, dietary B. subtilis A and B significantly increased the contents of immunoglobulins IgG, IgA, and IgM in the serum to be comparable to AGP, and increased the lysozyme activity relatively higher than AGP. Also, for immune organ development, dietary B. subtilis A and B increased the weight of the thymus. The positive effects of these two strains of B. subtilis on immunity indices are consistent with previous reports that B. subtilis A and B significantly reduced the enteritis index of broiler chickens and the pathogenic bacteria-induced systemic inflammation of weaned pigs (30, 35). Other strains of B. subtilis were also reported to have auxo-actions on the immunity of broiler chickens, such as B. subtilis 1781, 747, DSM 29784, CPB 011, CPB 029, HP 1.6, and D 014 (14, 17, 36, 37). Therefore, the results of this study reveal that dietary B. subtilis could enhance the immunity of broiler chickens.
Intestinal barrier integrity is a prerequisite for the homeostasis of mucosal function to maximize the absorptive capacity and defense against chemical and microbial challenges (38). Gut commensals, referred to as probiotics, were discovered to reinforce intestinal health by impacting the intestinal barrier function (39, 40). Supplemental probiotics in diets can positively alter the intestinal micro-environment and promote early intestinal development (41, 42). In the present study, the dietary addition of B. subtilis significantly increased the relative weight and length of the small intestine relative to AGP, and the effects of dietary B. subtilis and AGP on intestinal morphology and barrier integrity were similar, both better than that of the control group. The results are consistent with the previous reports that birds fed BSA showed a high-efficient intestine with shallower crypt depth and higher villus height to crypt depth ratio, and pigs fed with BSB had enhanced gut health (11, 30, 35). Besides, the effects of the improvement on the epithelial barrier integrity and gut health were also reported with C. butyricum, E. faecalis, and other strains of B. subtilis (14, 17, 36, 37, 43). Therefore, we deduced that dietary B. subtilis A and B improved the intestinal barrier and enhanced the gut health of broiler chickens for better nutrient digestibility and utilization.
Gut microbes play vital roles in many aspects of animal health including immune, metabolic, and developmental traits (44, 45). Microbial fermentation results in the generation of SCFA, such as acetate, propionate, and butyrate, which can indirectly affect various physiological processes and may contribute to enhancing health or create a diseased state in the animal (46–48). SCFA can stimulate specific membrane-bound receptors to regulate aspects of intestinal motility, hormone secretion, maintenance of the epithelial barrier, and immune cell function (49). In the present study, dietary B. subtilis significantly increased the contents of lactic acids, succinic acid, and butyric acid in the ileum and cecum, and the contents of formic acid, isobutyric acid, and isovaleric acid in the cecum relative to the control and AGP groups. Butyrate is the main energy source for intestinal epithelial cells, propionate transferred to the liver regulates gluconeogenesis and satiety signaling, and other fatty acids produced also have been implicated directly in animal health outcomes (44). Therefore, it indicated that dietary B. subtilis A and B improved gut health of broiler chickens probably through increasing intestinal fatty acids production.
Probiotics and prebiotics are microbiota-management tools for improving host health (50). Probiotics have been used to prevent a wide range of diseases for decades, and studies have suggested positive effects of certain probiotics on gut microbiota balance (51). In the current study, dietary B. subtilis significantly increased the amount of Lactobacillus and Bifidobacteria in ileum and cecum and decreased the amount of coliforms and Clostridium perfringens in the cecum. Lactic acid bacteria and Bifidobacteria are well-known probiotics and are widely introduced into the food chain (52). Birds infected with pathogenic strains of E. coli show low performance because of high diarrhea and mortality rates (53). Acute necrotic enteritis caused by Clostridium perfringens infections results in severe losses in broiler production (37). The increased Lactic acid bacteria and Bifidobacteria and the reduced Coliforms and Clostridium perfringens induced by the dietary B. subtilis in the current study imply an improved intestinal micro-ecological balance. Therefore, these results showed that dietary B. subtilis A and B increased intestinal fatty acids production and gut health, probably through improving the balance of the intestinal microflora.
Conclusion
Supplementation of BSA and BSB in broiler diets decreased mortality and enhanced growth performance. These positive effects could be accrued to efficient immune response capacity, intestinal microbial flora balance, the abundance of Lactobacillus and Bifidobacteria, and decreased coliforms and Clostridium perfringens, enhanced production of intestinal SCFA, and enhanced gut health. Also, the combination of two strains, BSA and BSB, synergistically enhanced intestinal morphology and integrity functions. Conspicuously, the results of the present study have provided strong evidence that supports supplementation of BSA and BSB in diets of broiler chickens as alternatives to synthetic antibiotics in the promotion of gut health and productivity index.
Data Availability Statement
The original contributions presented in the study are included in the article/supplementary material, further inquiries can be directed to the corresponding authors.
Ethics Statement
All experimental protocols were approved (AEC-CAAS-20191101) by the Animal Care and Use Committee of the Institute of Feed Research of the Chinese Academy of Agricultural Sciences.
Author Contributions
Conceptualization, methodology, and project administration were performed by H-jZ and G-hQ. Animal experiment, chemical analysis, and data collection were conducted by KQ and C-lL. Original draft was written by KQ, C-lL, and H-jZ. Writing-review was finished by S-gW, JW, and JG. All authors have agreed to the final manuscript.
Funding
This study was funded by the Shandong Key Science and Technology Innovation Program (2019JZZY010704), Beijing Innovation Consortium of Agriculture Research System (BAIC04-2021), Agricultural Science and Technology Innovation Program (ASTIP) of the Chinese Academy of Agricultural Sciences, and Evonik Degussa (China) Co., Ltd.
Conflict of Interest
JG was employed by company Evonik (China) Co., Ltd. The authors declare that this study received funding from Evonik (China) Co., Ltd. The funder was not involved in the study design, collection, analysis, interpretation of data, the writing of this article or the decision to submit it for publication.
The remaining authors declare that the research was conducted in the absence of any commercial or financial relationships that could be construed as a potential conflict of interest.
Publisher's Note
All claims expressed in this article are solely those of the authors and do not necessarily represent those of their affiliated organizations, or those of the publisher, the editors and the reviewers. Any product that may be evaluated in this article, or claim that may be made by its manufacturer, is not guaranteed or endorsed by the publisher.
References
1. Gadde U, Kim WH, Oh ST, Lillehoj HS. Alternatives to antibiotics for maximizing growth performance and feed efficiency in poultry: a review. Anim Health Res Rev. (2017) 18:26–45. doi: 10.1017/S1466252316000207
2. Sweeney MT, Lubbers BV, Schwarz S, Watts JL. Applying definitions for multidrug resistance, extensive drug resistance and pandrug resistance to clinically significant livestock and companion animal bacterial pathogens. J Antimicrob Chemother. (2018) 73:1460–3. doi: 10.1093/jac/dky043
3. Al-Khalaifa H, Al-Nasser A, Al-Surayee T, Al-Kandari S, Al-Enzi N, Al-Sharrah T, et al. Effect of dietary probiotics and prebiotics on the performance of broiler chickens. Poult Sci. (2019) 98:4465–79. doi: 10.3382/ps/pez282
4. Andrew Selaledi L, Mohammed Hassan Z, Manyelo TG, Mabelebele M. The current status of the alternative use to antibiotics in poultry production: an african perspective. Antibiotics. (2020) 9:594. doi: 10.3390/antibiotics9090594
5. Alagawany M, Abd El-Hack ME, Farag MR, Sachan S, Karthik K, Dhama K. The use of probiotics as eco-friendly alternatives for antibiotics in poultry nutrition. Environ Sci Pollut Res Int. (2018) 25:10611–8. doi: 10.1007/s11356-018-1687-x
6. Abd El-Hack ME, El-Saadony MT, Shafi ME, Qattan SYA, Batiha GE, Khafaga AF, et al. Probiotics in poultry feed: a comprehensive review. J Anim Physiol Anim Nutr. (2020) 104:1835–50. doi: 10.1111/jpn.13454
7. Tarradas J, Tous N, Esteve-Garcia E, Brufau J. The control of intestinal inflammation: a major objective in the research of probiotic strains as alternatives to antibiotic growth promoters in poultry. Microorganisms. (2020) 8:148. doi: 10.3390/microorganisms8020148
8. Sarao LK, Arora M. Probiotics, prebiotics, and microencapsulation: a review. Crit Rev Food Sci Nutr. (2017) 57:344–71. doi: 10.1080/10408398.2014.887055
9. Hong HA, Duc LH, Cutting SM. The use of bacterial spore formers as probiotics. FEMS Microbiol Rev. (2005) 29:813–35. doi: 10.1016/j.femsre.2004.12.001
10. Cutting SM. Bacillus probiotics. Food Microbiol. (2011) 28:214–20. doi: 10.1016/j.fm.2010.03.007
11. Zhang S, Zhong G, Shao D, Wang Q, Hu Y, Wu T, et al. Dietary supplementation with bacillus subtilis promotes growth performance of broilers by altering the dominant microbial community. Poult Sci. (2021) 100:100935. doi: 10.1016/j.psj.2020.12.032
12. Gadde UD, Oh S, Lee Y, Davis E, Zimmerman N, Rehberger T, et al. Dietary bacillus subtilis-based direct-fed microbials alleviate lps-induced intestinal immunological stress and improve intestinal barrier gene expression in commercial broiler chickens. Res Vet Sci. (2017) 114:236–43. doi: 10.1016/j.rvsc.2017.05.004
13. Al-Fataftah AR, Abdelqader A. Effects of dietary bacillus subtilis on heat-stressed broilers performance, intestinal morphology and microflora composition. Anim Feed Sci Technol. (2014) 198:279–85. doi: 10.1016/j.anifeedsci.2014.10.012
14. Park I, Lee Y, Goo D, Zimmerman NP, Smith AH, Rehberger T, et al. The effects of dietary bacillus subtilis supplementation, as an alternative to antibiotics, on growth performance, intestinal immunity, and epithelial barrier integrity in broiler chickens infected with eimeria maxima. Poult Sci. (2020) 99:725–33. doi: 10.1016/j.psj.2019.12.002
15. Dowarah R, Verma AK, Agarwal N, Singh P, Singh BR. Selection and characterization of probiotic lactic acid bacteria and its impact on growth, nutrient digestibility, health and antioxidant status in weaned piglets. PLoS ONE. (2018) 13:e0192978. doi: 10.1371/journal.pone.0192978
16. Larsen N, Thorsen L, Kpikpi EN, Stuer-Lauridsen B, Cantor MD, Nielsen B, et al. Characterization of bacillus spp. strains for use as probiotic additives in pig feed. Appl Microbiol Biotechnol. (2014) 98:1105–18. doi: 10.1007/s00253-013-5343-6
17. Gadde U, Oh ST, Lee YS, Davis E, Zimmerman N, Rehberger T, et al. The effects of direct-fed microbial supplementation, as an alternative to antibiotics, on growth performance, intestinal immune status, and epithelial barrier gene expression in broiler chickens. Probiotics Antimicro. (2017) 9:397–405. doi: 10.1007/s12602-017-9275-9
18. Rhayat L, Jacquier V, Brinch KS, Nielsen P, Nelson A, Geraert PA, et al. Bacillus subtilis strain specificity affects performance improvement in broilers. Poult Sci. (2017) 96:2274–80. doi: 10.3382/ps/pex018
19. Qiu K, Qin CF, Luo M, Zhang X, Sun WJ, Jiao N, et al. Protein restriction with amino acid-balanced diets shrinks circulating pool size of amino acid by decreasing expression of specific transporters in the small intestine. PLoS ONE. (2016) 11:e0162475. doi: 10.1371/journal.pone.0162475
20. Qiu K, Zhang X, Jiao N, Xu D, Huang C, Wang Y, et al. Dietary protein level affects nutrient digestibility and ileal microbiota structure in growing pigs. Anim Sci J. (2018) 89:537–46. doi: 10.1111/asj.12946
21. Aidara-Kane A, Angulo FJ, Conly JM, Minato Y, Silbergeld EK, McEwen SA, et al. World health organization (who) guidelines on use of medically important antimicrobials in food-producing animals. Antimicrob Resist in. (2018) 7:7. doi: 10.1186/s13756-017-0294-9
22. Manafi M, Hedayati M, Pirany N, Omede AA. Comparison of performance and feed digestibility of the non-antibiotic feed supplement (novacid) and an antibiotic growth promoter in broiler chickens. Poult Sci. (2019) 98:904–11. doi: 10.3382/ps/pey437
23. Miles RD, Butcher GD, Henry PR, Littell RC. Effect of antibiotic growth promoters on broiler performance, intestinal growth parameters, and quantitative morphology. Poult Sci. (2006) 85:476–85. doi: 10.1093/ps/85.3.476
24. Jacquier V, Nelson A, Jlali M, Rhayat L, Brinch KS, Devillard E. Bacillus subtilis 29784 induces a shift in broiler gut microbiome toward butyrate-producing bacteria and improves intestinal histomorphology and animal performance. Poult Sci. (2019) 98:2548–54. doi: 10.3382/ps/pey602
25. Bai K, Huang Q, Zhang J, He J, Zhang L, Wang T. Supplemental effects of probiotic bacillus subtilis fmbj on growth performance, antioxidant capacity, and meat quality of broiler chickens. Poult Sci. (2017) 96:74–82. doi: 10.3382/ps/pew246
26. Ouwehand AC. A review of dose-responses of probiotics in human studies. Benef Microbes. (2017) 8:143–51. doi: 10.3920/BM2016.0140
27. Whelan RA, Doranalli K, Rinttilä T, Vienola K, Jurgens G, Apajalahti J. The impact of bacillus subtilis dsm 32315 on the pathology, performance, and intestinal microbiome of broiler chickens in a necrotic enteritis challenge. Poult Sci. (2019) 98:3450–63. doi: 10.3382/ps/pey500
28. Bortoluzzi C, Serpa Vieira B, de Paula Dorigam JC, Menconi A, Sokale A, Doranalli K, et al. Dsm 32315 supplementation attenuates the effects of challenge on the growth performance and intestinal microbiota of broiler chickens. Microorganisms. (2019) 7:71. doi: 10.3390/microorganisms7030071
29. Ma Y, Wang W, Zhang H, Wang J, Zhang W, Gao J, et al. Supplemental bacillus subtilis dsm 32315 manipulates intestinal structure and microbial composition in broiler chickens. Sci Rep. (2018) 8:15358. doi: 10.1038/s41598-018-33762-8
30. He Y, Jinno C, Kim K, Wu Z, Tan B, Li X, et al. Dietary spp enhanced growth and disease resistance of weaned pigs by modulating intestinal microbiota and systemic immunity. J Anim Sci Biotechnol. (2020) 11:101. doi: 10.1186/s40104-020-00498-3
31. Long LN, Kang BJ, Jiang Q, Chen JS. Effects of dietary lycium barbarum polysaccharides on growth performance, digestive enzyme activities, antioxidant status, and immunity of broiler chickens. Poult Sci. (2020) 99:744–51. doi: 10.1016/j.psj.2019.10.043
32. Wu Y, Zhen W, Geng Y, Wang Z, Guo Y. Effects of dietary enterococcus faecium ncimb 11181 supplementation on growth performance and cellular and humoral immune responses in broiler chickens. Poult Sci. (2019) 98:150–63. doi: 10.3382/ps/pey368
33. Sánchez B, Delgado S, Blanco-Míguez A, Lourenço A, Gueimonde M, Margolles A. Probiotics, gut microbiota, and their influence on host health and disease. Mol Nutr Food Res. (2017) 61:240. doi: 10.1002/mnfr.201600240
34. Delgado S, Sánchez B, Margolles A, Ruas-Madiedo P, Ruiz L. Molecules produced by probiotics and intestinal microorganisms with immunomodulatory activity. Nutrients. (2020) 12:391. doi: 10.3390/nu12020391
35. Sokale AO, Menconi A, Mathis GF, Lumpkins B, Sims MD, Whelan RA, et al. Effect of bacillus subtilis dsm 32315 on the intestinal structural integrity and growth performance of broiler chickens under necrotic enteritis challenge. Poult Sci. (2019) 98:5392–400. doi: 10.3382/ps/pez368
36. Wang Y, Heng C, Zhou X, Cao G, Jiang L, Wang J, et al. Supplemental dsm 29784 and enzymes, alone or in combination, as alternatives for antibiotics to improve growth performance, digestive enzyme activity, anti-oxidative status, immune response and the intestinal barrier of broiler chickens. Br J Nutr. (2021) 125:494–507. doi: 10.1017/S0007114520002755
37. Ramlucken U, Ramchuran SO, Moonsamy G, Lalloo R, Thantsha MS. Jansen van Rensburg C. A novel bacillus based multi-strain probiotic improves growth performance and intestinal properties of clostridium perfringens challenged broilers. Poult Sci. (2020) 99:331–41. doi: 10.3382/ps/pez496
38. Hermiston ML, Gordon JI. Inflammatory bowel disease and adenomas in mice expressing a dominant negative n-cadherin. Science. (1995) 270:1203–7. doi: 10.1126/science.270.5239.1203
39. Bron PA, Kleerebezem M, Brummer R-J, Cani PD, Mercenier A, MacDonald TT, et al. Can probiotics modulate human disease by impacting intestinal barrier function? Br J Nutr. (2017) 117:93–107. doi: 10.1017/S0007114516004037
40. Hiippala K, Jouhten H, Ronkainen A, Hartikainen A, Kainulainen V, Jalanka J, et al. The potential of gut commensals in reinforcing intestinal barrier function and alleviating inflammation. Nutrients. (2018) 10:988. doi: 10.3390/nu10080988
41. Yu Y, Lu J, Oliphant K, Gupta N, Claud K, Lu L. Maternal administration of probiotics promotes gut development in mouse offsprings. PLoS ONE. (2020) 15:e0237182. doi: 10.1371/journal.pone.0237182
42. Hutsko SL, Meizlisch K, Wick M, Lilburn MS. Early intestinal development and mucin transcription in the young poult with probiotic and mannan oligosaccharide prebiotic supplementation. Poult Sci. (2016) 95:1173–8. doi: 10.3382/ps/pew019
43. Wang K, Cao G, Zhang H, Li Q, Yang C. Effects of clostridium butyricum and enterococcus faecalis on growth performance, immune function, intestinal morphology, volatile fatty acids, and intestinal flora in a piglet model. Food Funct. (2019) 10:7844–54. doi: 10.1039/C9FO01650C
44. Valdes AM, Walter J, Segal E, Spector TD. Role of the gut microbiota in nutrition and health. BMJ. (2018) 361:k2179. doi: 10.1136/bmj.k2179
45. Wu S, Shen Y, Zhang S, Xiao Y, Shi S. Interacts with autophagy to offense or defense. Front Microbiol. (2020) 11:721. doi: 10.3389/fmicb.2020.00721
46. Koh A, De Vadder F, Kovatcheva-Datchary P, Bäckhed F. From dietary fiber to host physiology: short-chain fatty acids as key bacterial metabolites. Cell. (2016) 165:1332–45. doi: 10.1016/j.cell.2016.05.041
47. Hu Y, Wang L, Shao D, Wang Q, Wu Y, Han Y, et al. Selectived and reshaped early dominant microbial community in the cecum with similar proportions and better homogenization and species diversity due to organic acids as agp alternatives mediate their effects on broilers growth. Front Microbiol. (2019) 10:2948. doi: 10.3389/fmicb.2019.02948
48. Zhang S, Shen YR, Wu S, Xiao YQ, He Q, Shi SR. The dietary combination of essential oils and organic acids reduces salmonella enteritidis in challenged chicks. Poult Sci. (2019) 98:6349–55. doi: 10.3382/ps/pez457
49. Priyadarshini M, Kotlo KU, Dudeja PK, Layden BT. Role of short chain fatty acid receptors in intestinal physiology and pathophysiology. Compr Physiol. (2018) 8:1091–115. doi: 10.1002/cphy.c170050
50. Sanders ME, Merenstein DJ, Reid G, Gibson GR, Rastall RA. Probiotics and prebiotics in intestinal health and disease: from biology to the clinic. Nat Rev Gastroenterol Hepatol. (2019) 16:605–16. doi: 10.1038/s41575-019-0173-3
51. Wieërs G, Belkhir L, Enaud R, Leclercq S, Philippart de Foy J-M, Dequenne I, et al. How probiotics affect the microbiota. Front Cell Infect Mi. (2019) 9:454. doi: 10.3389/fcimb.2019.00454
52. FAO/WHO. Probiotics in Food - Health and Nutritional Properties and Guidelines for Evaluation. Rome: Food and Agriculture Organization of the United Nations (2006).
53. Glombowsky P, Campigotto G, Galli GM, Griss LG, da Rosa G, Lopes MT, et al. Experimental infection with escherichia coli in broilers: impacts of the disease and benefits of preventive consumption of a stimulator of homeopathic immunity. Microb Pathog. (2020) 149:104570. doi: 10.1016/j.micpath.2020.104570
Keywords: Bacillus subtilis, broiler chickens, gut microbiota, immunity, probiotic
Citation: Qiu K, Li C-l, Wang J, Qi G-h, Gao J, Zhang H-j and Wu S-g (2021) Effects of Dietary Supplementation With Bacillus subtilis, as an Alternative to Antibiotics, on Growth Performance, Serum Immunity, and Intestinal Health in Broiler Chickens. Front. Nutr. 8:786878. doi: 10.3389/fnut.2021.786878
Received: 30 September 2021; Accepted: 25 October 2021;
Published: 29 November 2021.
Edited by:
Shourong Shi, Poultry Institute, Chinese Academy of Agricultural Sciences (CAAS), ChinaReviewed by:
Xiao Xu, Wuhan Polytechnic University, ChinaMd. Nazim Uddin, Sylhet Agricultural University, Bangladesh
Copyright © 2021 Qiu, Li, Wang, Qi, Gao, Zhang and Wu. This is an open-access article distributed under the terms of the Creative Commons Attribution License (CC BY). The use, distribution or reproduction in other forums is permitted, provided the original author(s) and the copyright owner(s) are credited and that the original publication in this journal is cited, in accordance with accepted academic practice. No use, distribution or reproduction is permitted which does not comply with these terms.
*Correspondence: Hai-jun Zhang, zhanghaijun@caas.cn; Shu-geng Wu, wushugeng@caas.cn
†These authors have contributed equally to this work