- 1Pharmacognosy Group, Department of Pharmaceutical Biosciences, Biomedical Centre, Uppsala University, Uppsala, Sweden
- 2International Research Center for Food Nutrition and Safety, Jiangsu University, Zhenjiang, China
- 3International Joint Research Laboratory of Intelligent Agriculture and Agri-Products Processing, Jiangsu Education Department, Jiangsu University, Zhenjiang, China
- 4Department of Chemistry, Faculty of Science, Menoufia University, Shebeen El-Kom, Egypt
- 5Department of Bee Research, Plant Protection Research Institute, Agricultural Research Centre, Giza, Egypt
- 6School of Computing, Engineering & Physical Sciences, University of the West of Scotland, Paisley, United Kingdom
- 7Food Research Section, R&D Division, Abu Dhabi Agriculture and Food Safety Authority (ADAFSA), Abu Dhabi, United Arab Emirates
- 8Alnahalaljwal Foundation Saudi Arabia, Mecca, Saudi Arabia
- 9College of Food Science, Fujian Agriculture and Forestry University, Fuzhou, China
- 10General Zoology Group, Institute for Biology, Martin Luther University Halle-Wittenberg, Halle, Germany
- 11Zoology Department, Faculty of Science, Tanta University, Tanta, Egypt
- 12Biology Department, Faculty of Science, Taibah University, Medina, Saudi Arabia
- 13School of Food and Biological Engineering, Jiangsu University, Zhenjiang, China
- 14Programme of Food Science and Technology, BNU-HKBU United International College, Zhuhai, China
- 15Institute of Apicultural Research, Chinese Academy of Agricultural Sciences, Beijing, China
- 16Department of Molecular Biosciences, The Wenner-Gren Institute, Stockholm University, Stockholm, Sweden
Inflammation is a defense process triggered when the body faces assaults from pathogens, toxic substances, microbial infections, or when tissue is damaged. Immune and inflammatory disorders are common pathogenic pathways that lead to the progress of various chronic diseases, such as cancer and diabetes. The overproduction of cytokines, such as interleukin (IL)-1β, IL-6, and tumor necrosis factor-α, is an essential parameter in the clinical diagnosis of auto-inflammatory diseases. In this review, the effects of bee products have on inflammatory and autoimmune diseases are discussed with respect to the current literature. The databases of Google Scholar, PubMed, Science Direct, Sci-Finder and clinical trials were screened using different combinations of the following terms: “immunomodulatory”, “anti-inflammatory”, “bee products”, “honey”, “propolis”, “royal jelly”, “bee venom”, “bee pollen”, “bee bread”, “preclinical trials”, “clinical trials”, and “safety”. Honey bee products, including propolis, royal jelly, honey, bee venom, and bee pollen, or their bioactive chemical constituents like polyphenols, demonstrate interesting therapeutic potential in the regulation of inflammatory mediator production as per the increase of TNF-α, IL-1β, IL-6, Il-2, and Il-7, and the decrease of reactive oxygen species (ROS) production. Additionally, improvement in the immune response via activation of B and T lymphocyte cells, both in in vitro, in vivo and in clinical studies was reported. Thus, the biological properties of bee products as anti-inflammatory, immune protective, antioxidant, anti-apoptotic, and antimicrobial agents have prompted further clinical investigation.
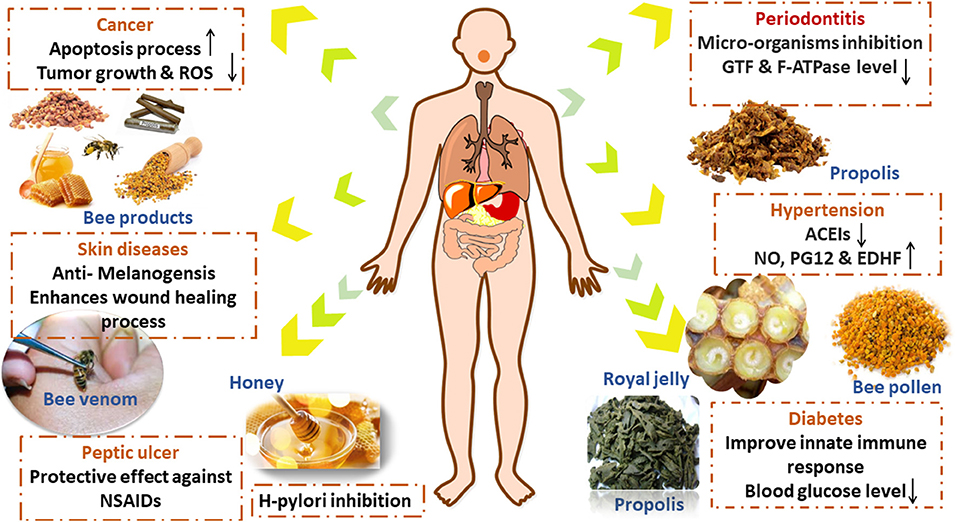
Graphical Abstract. The anti-inflammatory and immunomodulatory possible mechanisms of action of bee products suggested by preclinical and clinical studies.
Introduction
Inflammation is a defense mechanism that the immune system employs as a response to foreign stimuli, such as pathogens, toxic substances, bacterial infections, and irradiation, to repair tissue damage and return it to a state of hemostasis. Redness, swelling, heat, and pain are characteristic inflammatory processes involving various immune cells, such as B and T lymphocytes, macrophages, monocytes, basophils and neutrophils, mast and dendritic cells (1). The inflammatory response is mostly dependent on the nature of the stimuli; however, they share common mechanisms, such as the recognition of cell surface receptors, called pattern-recognition receptors, present on foreign pathogens or intracellular signaling that occurs because of damaged cells or tissues. The initiation of the inflammatory process activates different types of signaling, such as mitogen-activated protein kinase (MAPK), signal transducer, activator of transcription (STAT), nuclear factor kappa-B (NF-κB), and Janus kinase (JAK) pathways, leading to the release of inflammatory mediators, e.g., chemokines and cytokines, and the recruiting of inflammatory and immune cells. The NF-κB signaling pathway initiates the regulation of inflammatory cytokine production and the migration of inflammatory cells to injured tissues (2). Similarly, the MAPK pathway induces a cellular response to pathogens via protein kinase cascades that aid the regulation of the cell proliferation (3). Also, the JAK–STAT pathway promotes the release of various inflammatory cytokines, growth factors, and interferons (2). Cytokines are proteins with a molecular weight of <40 kDa that are released by immune cells, such as macrophages, monocytes, and lymphocytes, and are considered to be modulatory actors in acute and chronic inflammation (4). Uncontrolled acute inflammation, an overlap in the activation of intracellular signaling pathways, and the release of inflammatory mediators result in the onset of chronic inflammatory diseases, such as diabetes and cancer (2), peptic ulcers (5), cardiovascular, and periodontal diseases (6).
Honey, propolis, royal jelly, bee pollen, bee bread, venom, and wax are all products produced by bees. The health, nutritive, and medicinal values of bee products have been demonstrated by the ancient Egyptians, Greeks, and Chinese (7). Bee products have long been used as health-promoting nutritional additives (8). Bee products have diverse biological properties such as antimicrobial, anti-inflammatory, anticancer, and antioxidant activities (9, 10). Proteins, peptides, minerals, flavonoids, terpenes, fatty acids, and phenolic compounds are among the physiologically active components that exist in bee products (8, 11, 12). Propolis is a thick, resinous, lipophilic bee product known as “Bee glue & Russian Penicillin.” Propolis is a sticky substance that honeybees gather by mixing their own waxes with resinous sap obtained from the bark and leaf-buds of certain trees and other flowering plants, to use it in their nests. Chemically, it is composed of 50% plant resins, 30% waxes, 10% essential and aromatic oils, 5% pollens, and 5% other organic substances (1). It is known to have antibacterial, antiviral, anticancer, and anti-inflammatory effects. It is also reportedly the most potent antioxidant bee product (13–16). The propolis ethanolic extract (EEP) and its phenolic components have been shown to induce tumor necrosis factor-related apoptosis-inducing ligand (TRAIL)-mediated apoptosis in cancer cells (17). It is also worth mentioning that bee venom and melittin, a major component of bee venom, have demonstrated anti-inflammatory and immunomodulatory activities. Bee venom is a natural toxin that is secreted by the poison and accessory gland of honeybee workers, located in the abdominal cavity. It is then stored in the venom reservoir and plays a significant role in the defense strategy of bee colonies. Bee venom has anti-inflammatory, radio-protective, and potent antibacterial effects. It also has demonstrated effectiveness in treating certain pathological conditions, such as rheumatism, and cancer, as it can kill cancer cells (18, 19). Bee venom contains biologically active components such as melittin, apamin, adolapin, secapin, tertiapin, mast cell-degranulating phospholipase A2, and hyaluronidase. Histamine, epinephrine, and macromolecules (such as lipids, carbohydrates, and free amino acids) are also among the bioactive elements (2). Melittin combined with nanographene oxide showed anticancer activity toward MCF-7 and MDA-MB-231 breast cancer cells compared with melittin alone, and it also helped to reduce the unselective cytotoxicity toward normal cells (20). Honeybee pollen is a mixture of pollen and flower nectar that honeybees collect and mix as food supply for the hive. Proteins, amino acids, saccharides, vitamins, and minerals abound in it. As a result, it is commonly considered a “super food,” one of the most nutrient-dense natural items, and is commercially available as a food supplement (21). Honeybees primarily use royal jelly to feed the queen and young larvae. It is, nevertheless, one of the functional bee products, that has been manufactured for commercial usage in many nations, particularly in the fields of dietary products and cosmetics. Royal jelly is an acidic emulsion made up of fat, protein, and carbohydrates suspended in 60 to 70% water. The sugars glucose and fructose account for 90% of the total sugar content of royal jelly. The lipid content of royal jelly is unusual component, consisting primarily of short-chain fatty acids, mostly hydroxyl and carboxylic acids, which are distinct from other organic acids found in animals and plants (8–12). Royal jelly has antimicrobial, anti-inflammatory, immunomodulatory, and anti-diabetic properties, making it a commercially and medicinally valuable material (22, 23). The effectiveness of bee products, such as bee venom, royal jelly, propolis, bee pollen, and honey, in treating inflammatory and autoimmune diseases, have been documented in various reports (24–27). However, there are still few reports of clinical studies on bee products (Figure 1). This is due to the incidence of asthma and anaphylaxis, in some instances upon the consumption of royal jelly, bee venom, and bee pollen, which somewhat hinders their use for some individuals despite their medicinal and health benefits (28–30).
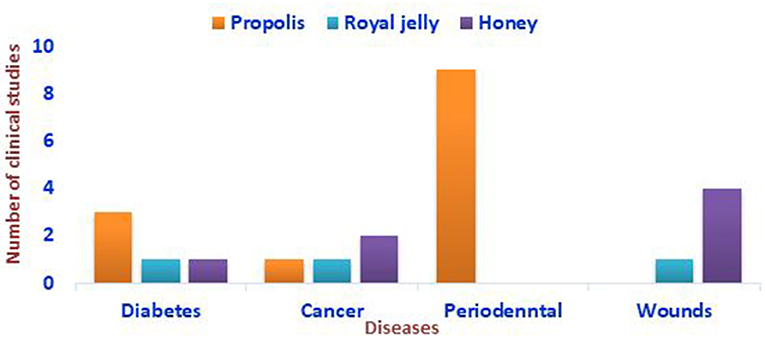
Figure 1. Representation of the clinical studies conducted on the impacts of bee products against some autoimmune diseases based on the literature screening.
This review comprehensively outlines the preclinical and clinical studies that have been carried out to date on bee products and their bioactive ingredients, emphasizing their role in treating the immune and inflammatory disorders.
Immunomodulatory and Anti-Inflammatory Properties of Bee Products
Inflammation is a common underlying cause of serious diseases, as it initiates a pathogenic cascade of the failure of metabolic pathways, tissue damage, and even necrosis and apoptosis. Inflammatory diseases are diagnosed after an increase in interleukin (IL)-1β, IL-6, and tumor necrosis factor-α (TNF-α) cytokines (2). The in vitro and in vivo investigations have shown that bee products and bee products derived active constituents show potent anti-inflammatory and anti-autoimmune properties. The 8–12 C free fatty acids in royal jelly, such as 10-hydroxydecenoic acid (10-HDA), have modulated the immunological response in a concentration-dependent manner. In an in vitro study, 10-HDA at a dose of 500 mM inhibited the maturation of lipopolysaccharide (LPS)-stimulated human monocyte-derived dendritic cells (Mo-DCs) and the production of IL-8, IL-12, and TNF-cytokines, as well as down-regulating both Th1 and Th2 immune responses and decreasing the number of T helpers (Th1 and Th2) and Mo-DCs. A higher concentration of 10-HDA of more than 750–1,000 μM induced Mo-CD cell apoptosis (31). In vitro and in vivo studies, bee venom and melittin present a potential means to stop overactive immune responses and inflammatory disorders; however, the cytotoxic effect of melittin needs to be taken into account (32). Porphyromonas gingivalis and its lipopolysaccharides (PgLPS) are known pathogens that are involved in periodontal disease. Bee venom shows an inhibitory effect on the pro-inflammatory cytokines IFN-γ, IL-1β, IL-6, IL-8, and TNF-α by suppressing the NF-κB and AP1 pathways induced by PgLPS in the human keratinocyte cell line (HaCaT cells, in vitro) (33). Moreover, a polysaccharide of Chinese wolfberry bee pollen exhibited an immunomodulatory response toward RAW264.7 cells (in vitro) by decreasing the levels of IL-1, IL-6, TNF-α, and nitric oxide (NO) secretion (34). Topical application of propolis improved the wound healing process of a streptozotocin (STZ)-induced wound in a type I diabetes mellitus (DM) mouse model, which was attributed to a decrease in pro-inflammatory cytokines such as IL-6, IL-1B, and TNF-α, as well as an increase in collagen formation via the transforming growth factor-1 (Smad2 and Smad3) signaling pathways (35). Overall, the anti-inflammatory and immunomodulatory effects of bee products mainly venom, royal jelly, and propolis are linked to the secretion of pro-inflammatory cytokines such as IFN-, IL-1, IL-6, IL-8, and TNF-α, as well as the regulation of intra signaling pathways and modulation of B and T lymphocyte cell function.
Preclinical and Clinical Studies on Bee Products
Diabetes
Preclinical Studies
Diabetes (DM) is one of the chronic diseases that develop due to severe inflammation and manifests in an increase in pro-inflammatory cytokines and chemotaxis mediated by B and T lymphocytes, such as IL-1β and TNF-α, leading to insulin resistance (36). In mice model, the immunomodulatory activity of propolis was found to improve the innate immune response. It induces the up-regulation of Toll-like receptors 2 (TLR-2) and TLR-4, which leads to the activation of lymphocytes, and CCL21- and CXCL12-mediated chemotaxis by B and T lymphocytes. Also, propolis helps to restore the levels of IL-1β, IL-6, Il-2, Il-7, and TNF-α (37, 38). In diabetic male rats model, propolis decreases serum immunoglobulin (IgG, IgA, and IgE), that controls B and T lymphocyte proliferation (39). The peptic bee pollen polysaccharide (RBPP-P) of Rosa rugosa shows a regulatory effect toward certain pro-inflammatory cytokines that raise insulin resistance, e.g. TNF-α and IL-6. Treatment with RBPP-P decreases TNF-α and IL-6 levels, resulting in less insulin resistance observed in the tissues of mice fed with a high-fat diet (40).
Furthermore, DM is characterized by hyperglycemia, glycosuria, and dyslipidemia. Dyslipidemia and hyperglycemia induce the propagation of reactive oxygen species (ROS), which lead to cellular dysfunction and oxidative injuries, especially to the heart, kidney, and liver tissues (41). Propolis, royal jelly, and bee pollen act against the oxidative stress that arises in different diseases, e.g., DM (42). Propolis has anti-hyperglycemia, dyslipidemia, and antioxidant properties, which could be attributed to its polyphenolic content, e.g., α-amylase, α-glucosidase, and caffeic acid phenethyl ester (CAPE) (43, 44). Propolis improves lipid profile metabolism and decreases the ROS levels in blood, liver, and lymph nodes (45). Intraperitoneal administration of water-soluble-derivative of propolis (WSDP) or (–)-epigallocatechin gallate (EGCG) (50 mg/kg/day) in alloxan-induced diabetic mice for 7 days lead to reduce in lipid peroxidation in liver, kidney, brain tissues, and reduce DNA damage in peripheral lymphocytes of diabetic mice (46). WSDP and ethanolic propolis extract increased the animal body weight and the life span of treated mice vs. the untreated mice mediated by the improvement of the fatty acid metabolism impairment (47). In addition to this, propolis significantly controls body weight and bone homeostasis (48). An ethanolic extract of propolis has reduced hyperglycemia and protected the pancreatic and renal tissue of STZ-induced diabetic rats that received 30 or 15% propolis extract at a dosage of 50 ml/kg for 4 weeks. Blood sugar levels decreased from 393 ± 192.7 to 154 ± 28.0 mg/dl and from 386 ± 141.1 to 331.5 ± 123.74 mg/dl, was observed in the diabetic groups treated with 30 and 15% propolis, respectively. An improvement at the pancreatic, hepatic, and renal tissue levels was seen in both groups treated with propolis compared with the control group. The IC50 results for α-amylase (0.62 ± 0.00 μg/ml) and α-glucosidase (40.40 ± 0.09 μg/ml) whereas, CAPE and chrysin were the dominant phenolics (43). Bee pollen or date palm pollen suspension (100 mg/kg) administration for four weeks has shown to reduce body, testis, and pancreas weight and insulin resistance in STZ-induced diabetic Wistar male rats. The protective effect of bee pollen was found to be associated with a decrease in NO and LPx-biomarkers of oxidative stress and increase in glutathione (GSH), GSH-S-transferase, GSH peroxidase, and superoxide dismutase (SOD) antioxidant defense markers (49).
Clinical Studies
Propolis, royal jelly, and honey have been the subject of various clinical studies, as shown from the data presented in Table 1. Propolis administrated at different doses (300 and 400 mg) has shown to aid in the modulation of the glycemic mediators fasting serum glucose (FSG) and glycosylated hemoglobin A1C (HbA1C) in subjects with type 2 DM (T2DM) (51, 52). Meanwhile, the oral administration of encapsulated propolis has enhanced liver and kidney functions as well as lipid profiles by increasing high density lipoprotein (HDL) levels and glucose metabolism and decreasing the levels of HbA1C by 8%, 2-h postprandial (2 hpp), homeostasis model assessment of β-cell function (HOMA-β), and TNF-α as shown in Table 1 (55). The oral administration of encapsulated royal jelly (1,000 mg) three times per day for eight weeks has led to a decrease in glucose (−9.4 ± 13.5 mg/dL vs. 4 ± 8.2 mg/dL in the placebo group) and an increase of serum apolipoprotein A-I (ApoA-I) (34.4 ± 53.3 mg/dL vs. −1.08 ± 32.6 mg/dL in the placebo group) levels and thus diminish the risk of cardiovascular diseases (CVDs) in patients with T2DM (53). Figure 2 outlines the therapeutic potential of bee products in preclinical and clinical studies of diabetes, how different factors influence them, and how they are associated with the underlying pathogenesis.
Cancer
Preclinical Studies
In the 19th century, the detection of leukocytes in tumor cells was the first indication of the link between cancer and inflammation since 20% of cancers could be attributed to inflammation. Inflammation is involved in the different steps of tumor development, e.g., initiation, promotion, malignancy, invasion, and metastasis (58). The term chemoprevention describes the inhibition of the processes of mutagenesis, carcinogenesis, proliferation, and the progression of tumor cells using natural or synthetic compounds (59, 60).
Honey and its polyphenolic compounds, e.g. pinobanksin, pinocembrin, luteolin, galangin, chrysin, and quercetin (3,3′,4′,5,7-pentahydroxyflavanone), shown to have anti-inflammatory, immunomodulatory, anti-proliferative, and anti-metastatic properties. They were found to induce apoptosis and showed antioxidative activity toward several cancer types (61). Tyrosine-phosphorylated signal transducer and transcription three activator (p-STAT3) is a major player in lung and breast cancer (62). Manuka honey (MH) has shown to have an inhibitory effect on p-STAT3 in the triple-negative breast cancer cell line MDA-MB-231 and non-small cell lung cancer cell line A549. MH targets the IL-6 receptor α chain (IL-6Rα), preventing its binding to IL-6 by ~60%, thus decreasing the level of glycoprotein (gp130) and tyrosine-phosphorylated JAK2, which are important components of IL-6Rα. Galangin, chrysin, quercetin, and luteolin are the major flavonoid compounds in MH that can inhibit the binding of IL-6Rα and IL-6 by 34.3, 31.8, 29.2, and 22.4%, respectively, at a concentration of 50 μM (63).
Propolis containing mainly polyphenolic compounds has exhibited chemoprevention and tumor growth inhibition abilities, as well as antioxidant, pro-oxidant, and immunoprotective properties (64–66). These compounds have been reported to induce apoptosis, inhibit matrix metalloproteinase, regulate cell proliferation by promoting cytotoxicity, modulate gene methylation (67), and exhibit anti-inflammatory and anti-angiogenic activities toward urothelial and bladder cancer (68). WSDP and its polyphenolic compounds caffeic acid (CA) and CAPE show immunomodulatory properties and control the growth and spread of tumors. The administration of WSDP, CA, and CAPE at dose 50 or 150 mg/kg via intravenous injection into mice with mammary carcinoma was shown to decrease the number of colonies. In contrast, subcutaneous administration inhibited tumor growth and increased the survival of the mice. In the proposed mechanism, the phenolic compounds initiate the anti-tumor activity of macrophages, which help to block the metastasis process and increase hydrogen peroxide production, which modulates lymphocyte function. This is in agreement with the inhibition response of spleen cells toward the administration of polyclonal mitogens (phytohemagglutinin (PHA), concanavalin A (ConA), pokeweed mitogen (PWM), and LPS in mice treated with CA (65). WSDP inhibits IL-1, which is associated with B and T cell proliferation (65).
Also, 10-HDA in royal jelly exhibits anti-inflammatory properties toward human colon adenocarcinoma cells. 10-HDA inhibits the production of pro-inflammatory cytokines, TNF-α, IL-1β, and IL-8 in WiDR human adenocarcinoma cell (BCRC 60157). Receptor antagonist cytokine (LI-1ra) production induced by 10-HDA at doses of 0.1–3 mM helps to reduce tumor growth and stop the production of IL-8 and IL-1, which are causative factors in the development of some cancers, including pancreatic and colon carcinoma (69). CAPE causes distinctive changes to breast cancer stem cells (bCSCs) as it halts the generation of malignant cells by suppressing their self-generation and CD44 clonal expansion. Additionally, the results suggest that MDA-231-derived bCSCs treated with CAPE are less susceptible to malignant infections (70). TRAIL is an effective endogenous anticancer agent that promotes apoptosis, especially of tumor cells. An ethanolic extract of green propolis promote TRAIL-mediated apoptosis in LNCaP prostate cancer cells via the modulation of the intrinsic and extrinsic apoptotic pathways and alteration of the NF-κB activity. Brazilian green propolis able to enhance TRAIL-mediated apoptosis, which could be one of the mechanisms responsible for its cancer-preventive properties. The major components of Brazilian green propolis determined by high-performance liquid chromatography (HPLC) including; quercetin, artepillin C, kaempferol (KAE), and p-coumaric acid. Brazilian EEP at 50 μg/mL and its components exhibit better free radical scavenging than the other compounds in the propolis extract at 50 μg/mL. Quercetin was the most bioactive compound that induces cell death and apoptosis toward LNCaP (71). Collectively, galangin, chrysin, CA, CAPE, and 10-HDA are the common bioactive components of bee products that show anticancer activity (Figure 3).
Despite its potential anticancer activity, clinical studies/trials on propolis are limited due to its poor physicochemical properties and oral bioavailability. Thus, the encapsulation of propolis extract in nanoparticle systems has been suggested. Through a well-designed polymeric architecture, propolis encapsulation in nano-in-microparticles (NIMs) enhances bioavailability, therapeutic efficiency, and controlled drug release. Nano spray drying is a method for producing polymeric nanoparticles, microparticles, and NIMs that contain a variety of bioactive compounds and drugs. The prepared spray-dried NIMs of propolis extract have been supplemented orally for the treatment of colon and liver cancer and were found to promote the cytotoxicity of human liver (HepG2) and colon (HCT-116) carcinoma cell lines (67, 72).
The application of propolis and lycopene extract nanoemulsions is safe and has been shown to have a highly protective effect on skin cells against UV irradiation, which induces different skin cancers via erythema and pigmentation. Nanoemulsions have been widely applied in the delivery of poorly soluble drugs. Nanoemulsions have been shown to increase solubility, kinetic stability, and decrease collagenase activity (73). Also, the trapping of bee venom components in nanoparticles may help to reduce its unselective toxicity and improve its prospects for application as a therapeutic anticancer agent (74).
Clinical Studies
Anti-inflammatory and antioxidant properties of honey, propolis, royal jelly, and bee pollen can explain the impact of bee products against different types of cancer. As demonstrated in Table 2, honey and propolis are used to treat oral mucositis, lymphoblastic leukemia, and head and neck malignancies, whereas bee pollen is used to treat renal cell carcinoma (75, 77–81). Additionally, propolis and honey reduced the risk of oral mucositis induced by undergoing radiotherapy treatment, as shown in Table 2. The anti-oxidant and anti-inflammatory activities of encapsulated royal jelly (400 mg) were studied and proven protective against the fatigue and anorexia induced by tyrosine kinase inhibitors (TKIs) in patients with renal cell carcinoma (RCC) (76).
Hypertension
Preclinical Studies
Inflammation is a common predisposing factor of atherosclerosis and hypertension, and consequently, CVDs. Hypertension is a global problem and one of the leading causes of CVDs e.g., cerebral stroke, acute myocardial infarction, and heart failure. The renin–angiotensin system (RAAS) is essential in regulating blood pressure and maintaining inflammatory processes in CVDs. The use of angiotensin-converting enzyme inhibitors (ACEIs), angiotensin receptor blockers, and direct renin inhibitors to inhibit RAAS pharmacological action is effective in the treatment of hypertension (82).
The antioxidant properties of bee pollen polyphenols have been shown to play an important role in inhibiting the angiotensin-converting enzyme. The supplementation of bee pollen extract (0.1 and 1 g/kg for 16 weeks) decrease the high levels of ACEIs and angiotensin II-induced by high-fat diets in C57BL6 mice, leading to an enhancement in endothelial function due to the modulatory effect it has on RAAS (Figure 4) (83). It has also been noted that protease N-treated royal jelly (Pro-RJ) with Ile–Tyr (IY), Val–Tyr (VY), and Ile–Val–Tyr (IVY) shows high inhibitory activity toward ACEIs. The oral administration of Pro-RJ peptide (IY, VY, or IVY) or a peptide mixture (IY, VY, and IVY) for 28 days was shown to yield anti-hypertensive activity. The long-lasting hypotensive activity of royal jelly protein (RJPH) has been attributed to its small molecular weight peptide content and its fast digestion time (84, 85). Major RJPH 1 (MRJP1) is another bioactive component of royal jelly that has shown anti-hypertensive activity. MRJP1 exhibits a high regulatory effect on blood pressure via its action on vascular smooth muscle cells (VSMCs). The MRJP1 shows a protective effect against the contraction, abnormal migration, and proliferation of VSMCs by reducing their movement and energy supply. VSMCs regulate blood pressure as they play the main role in blood vessel contraction and relaxation. Also, the proliferation of VSMCs and the increase in its numbers leads to a reduction in the blood vessel diameter, which in turn affects blood flow (86).
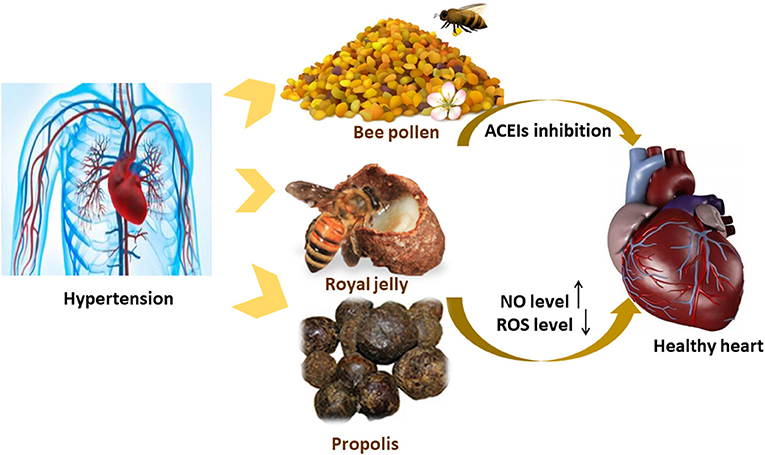
Figure 4. Possible mechanism of actions explaining the anti-hypertensive effect of the bee products.
The pathophysiological condition of hypertension is manifested by the decrease in NO production, prostacyclin, and endothelium-derived hyperpolarizing factor, which are endothelium-derived relaxing factors of vascular endothelial cells. Royal jelly has a muscarinic receptor agonist effect that is similar to acetylcholine, which increases NO formation and vasorelaxation via the NO/cGMP pathway and calcium channels. An increase in NO formation promotes vasodilation and hypotension (84). A total of 200 mg/kg of propolis supplementation has reduced oxidative stress in hypertensive rats treated with NO-nitro-L-arginine methyl ester NO synthase inhibitor (Figure 4). ROS causes NO loss by its conversion into a peroxynitrite oxidant. The antioxidative effect of propolis helps in reducing ROS, increasing the levels of NO synthesis, and enhancing the endothelial function (87).
Clinical Studies
In clinical studies, it was reported that the utilization of mouthwash containing propolis causes a reduction in the harmful effect of oral bacteria, hence disrupting NO levels and leading to a decrease in blood pressure (88).
Periodontitis
Preclinical Studies
Periodontal disease is a phenomenon where the periodontal tissue accumulates bacterial plaque followed by a secondary immune and inflammatory response (89). The anti-inflammatory, antioxidant, antibacterial, anti-caries, and antiplaque properties of propolis support its use in preventing and treating a variety of oral disorders, including dental plaque, gingiva, caries, and oral cancer (90, 91). The formation of dental caries is a chronic process in which a chemical interaction occurs between acid-producing tooth-adherent bacteria and fermented carbohydrate, resulting in dental decay. The most popular pathogenic factor is the high consumption of sugars, as a high number of microorganisms, especially S. mutans, which is the most predominant carcinogenic factor in caries (92). The anti-caries and antiplaque activities of crude extracts or hexane fractions of Brazilian propolis have been tested in vitro and in vivo. The suggested mechanism for the anti-caries activity of propolis is related to the antibacterial action against S. sobrinus and S. mutans and the inhibition of glucosyltransferase (GTF) enzyme (90, 93). Propolis extracts' cariostatic effects are linked to GTF inhibition rather than antibacterial activity (90). For five weeks, the rats were given hexane fraction of propolis topically twice a day. In in vivo study, the fraction greatly reduced the incidence of smooth surface caries while maintaining the percentage of S. sobriuns biofilms in the animals' plaque. The high fatty acid content of Brazilian propolis comprising palmitic, oleic, and stearic acids, enables it to exhibit highly potent anti-caries activity when incorporated in a rat diet (93).
The other components in propolis, e.g., CAPE and quercetin exhibit anti-caries properties. CAPE has an antibacterial effect on the most prevalent oral bacteria (S. mutans, S. sobrinus, Actinomyces viscosus, and Lactobacillus acidophilus) and contributes toward a decrease in the formation of biofilms, as well as reducing their thickness. The antibacterial effect of CAPE is mostly observed toward S. mutans. It has been suggested that CAPE penetrates the thick extracellular matrix biofilm and damages the cells, increasing the number of dead bacteria. When administered at half of its minimum inhibitory concentration (MIC), CAPE has been shown to reduce the acidity of S. mutans at pH 5, resulting in cell death (94). Quercetin in the Ardabil EEP with a MIC value in the range of 3.12–100 μg/mL was shown to inhibit the growth of oral streptococci and reduce biofilm formation (95). Topical treatment of red propolis (800 μg/mL) in the form of neovestitol–vestitol has been shown to prevent the formation of biofilm and reduce the bacteria-derived exopolysaccharide (EPS)-rich matrix building of biofilm. The proposed mechanism of action for this is via the inhibition of the GTF-derived EPS enzyme activity, which contributes toward the development of EPS by producing soluble glucan or suppressing certain genes linked with the stress and survival of S. mutans (96). Topical application of an EEP varnish (15% propolis) or its bioactive fraction twice a day for 4–5 weeks on rat teeth has been shown to exhibit anti-caries activity comparable to that of the drug conventionally used to treat caries, GS/Duraphat®. Also, no toxic effects on fibroblast or osteoblast cells were observed (90).
Clinical Studies
The protective and treatment effects of propolis as anti-caries, anti-dental plaques, and anti-gingivitis in periodontal diseases have been detected in various clinical studies (Table 3). Propolis or extract reduces dental biofilm formation, raises salivary pH, reduces plaque formation, and protects the oral cavity from bacterial infection (97, 98, 103).
Skin Diseases
Preclinical Studies
Melanogenesis
Melanin pigmentation determines the color of animals' and humans' skin. Melanin protects the skin from UV rays, excessive accumulation of melanin can lead to major skin problems such discoloration, nevus, melasma, and skin aging (107). In a mouse melanocyte cell line (B16F1), royal jelly has exhibited anti-melanogenesis activity, as it decreases mRNA transcription and thus the expression of the tyrosinase enzyme, leading to a decrease in melanin content (108). 10-HDA has shown anti-pigmentation activity in vivo using the animal model. 10-HDA decreases tyrosinase, tyrosinase-related protein (TRP)-1, and TRP-2 mRNA transcription by suppressing the microphthalmia-associated transcription factor in B16F10 melanoma cells (109).
Wound Healing
Skin is the main physical barrier of the body, which protects it from harmful environmental agents. Bee products have long been used in skincare and are present in many dermatological preparations for purposes, such as skin refreshing, skin whitening, the treatment of burns, and wound healing (110). Royal jelly has antimicrobial activity and enhances the healing of damaged tissues (111). Water-soluble RJPHs, such as MRJP2, MRJP3, and MRJP7, induce the proliferation, differentiation, and migration of human epidermal keratinocyte cells to promote wound closure (112). Royal jelly increases collagen formation and shows anti-inflammatory properties (113). 10-HDA, defensin-1, and MRJP3, which are components of royal jelly, have an inhibitory effect on pro-inflammatory cytokines, such as IL-1 and IL-6, without harming the macrophages themselves. MRJP induces the proliferation and migration of specific cells, such as fibroblasts.
Propolis' anti-inflammatory, antioxidant, and antibacterial properties may have contributed to its efficacy in wound healing (114, 115). Propolis reduces the concentration of pro-inflammatory proteins, such as cyclooxygenase (COX-1 and COX-2), proteinase, and prostaglandins (prostaglandin E) owing to the presence of flavonoid and phenolic compounds, such as CAPE (116, 117). In addition, the topical application of propolis on diabetic wounds has reduced/declined IL-1β, IL-6, TNF-α, and MMP9 levels in STZ-induced type I diabetic mice (35). Propolis has also enhanced wound healing in rats and reduced the closure time by stimulating collagen formation and keratinocyte migration. The antibacterial effect of a mixture of two different types of propolis collected from different regions in Iraq has been tested on Escherichia coli, Staphylococcus aureus, and Candida albicans. A combination of the two types of propolis was found to significantly improve the anti-inflammatory, antioxidant, and re-epithelization properties of the materials compared with their use alone, leading to an acceleration in the wound healing process (118).
Tissue engineering is a scientific technique that focuses on remolding tissue damage and organ substitutes (119). In tissue engineering, different functional polymer membranes, such polyvinyl alcohol (PVA), and cellulose acetate are used to fabricate nanostructure-based scaffolds for use in wound dressings. In vivo and in vitro studies have shown that the combination of propolis with the polymer wound dressings helps to promote their usability in the wound healing process (120–122). The loading of propolis in a PVA nanofiber wound dressing has promoted wound closure of up to 68% after seven days of treatment in a diabetic wound healing model (121). The treatment of male rats suffering from second-degree burns with propolis associated in natural rubber membrane has resulted in regeneration, re-epithelization, collagen deposition, and a decrease in the number of inflammatory cells in the animals (123). Moreover, bilayer wound dressing was prepared using a polycaprolactone/gelatin scaffold (sub-layer) that was electrospun on a dense membrane consisting of an EEP membrane (top layer). The bilayer was then shown to induce wound healing and reduce the closure time. In general, the bilayer technique improves the biocompatibility, biodegradability, and mechanical proprieties of dressings in wound healing. The presence of propolis in the first layer provides protection for wounds against infections due to its antibacterial and antifungal properties (124).
Clinical Studies of Bee Products Against Wounds
Honey's antibacterial activity, immunological modulatory capabilities, and biocompatibility all contribute to its usefulness as a wound-healing agent (125). Honey in combination with other therapies has superior antibacterial action, suggesting that it may be useful for malignant wounds, diabetic foot ulcers, wounds, leg ulcers, and burn wounds (Table 4) (126, 127, 133, 137, 139). PedyPhar® Ointment consists of royal jelly and panthenol enhanced the healing rate in patients with diabetic foot ulcers. As well, a daily application of Leptospermum honey gel on ulcers or wounds promoted tissue proliferation and decreased the accumulation of necrotic tissue in wounds (Table 4) (128).
Peptic Ulcers
Preclinical Studies
Vomiting, nausea, postprandial abdominal pain, and weight loss are typical symptoms associated with peptic ulcer. The term “peptic ulcer” refers to the irritation of the digestive tract, mostly of the stomach or proximal duodenum wall because of the hyper secretion of peptic acid. The use of non-steroidal anti-inflammatory drugs (NSAIDs) or aspirin, and Helicobacter pylori infections could all lead to peptic ulcers (5). H. pylori are Gram-negative bacteria that infect the human mucosa. H. pylori as a causative factor in gastric inflammatory, ulcers, and cancers stimulate the expression of the outer membrane proteins related to epithelial gastric cells (142). Bacterial honey, Bacillus subtilis, is a source of different types of levans. Bacillus sp. levan (200 mg/kg) has been shown to act as a probiotic agent against gastric ulcers induced by ethanol in adult male Sprague–Dawley rats. The probiotic activity of levan enables it to cover the stomach epithelial cells and prevent the adhesion of H. pylori (143). The suggested mechanism of the gastro-protective effects of propolis and its isolated metabolites drupanin, artepillin C, kaempferide, and aromadendrin-4-O-methyl-ether are mostly associated with their anti-inflammatory, antioxidant, and inhibitory properties toward gastric secretion (144).
The administration of hydroalcoholic extracts of red propolis (HERP, 250 and 500 mg/kg) or its formononetin isoflavonoid (10 mg/kg) has provided protection against ulcer formation, decreased the volume of gastric secretions, and led to healing the damaged areas of the mucosa in a dose-dependent manner. HERP has been shown to inhibit H. pylori infection and decrease the diameter of the affected zone by 13.0 ± 2.0 mm at 100 mg/mL (145). The MAPK and NF-κB pathways promote the pathogenic action of H. pylori. An ethanolic extract of Korean propolis has reduced levels of pro-inflammatory interleukins, such as IL-12, IL-8, IL-1β, and COX-2 via the inhibition of the MAPK and NF-κB pathways (146). KAE (160 mg/kg) causes a decrease of TNF-α by 48% and IL-1β by 37% in the gastric ulcer. It also increases the level of NO vasodilator factor and increasing the blood flow to the gastric mucosa (147).
Bee venom has been shown to exhibit anti-inflammatory and anti-apoptotic properties and have a protective effect against NSAID-induced gastric ulcers. An intraperitoneal injection of 2 mg/kg of bee venom into Sprague–Dawley rats with ASA-induced peptic ulcers has been shown to reduce the levels of pro-inflammatory cytokines, such as TNF-α and maintain normal levels of antioxidant enzymes, such as SOD and GSH. In addition, it decreases the expression of caspase-3 and B-cell lymphoma associated X, and enhances the recovery of mucosal damage and inhibiting apoptosis in gastric cells (148). Propolis, honey, and bee venom mostly demonstrate protective and healing effects on peptic ulcers because of their anti-inflammatory, antioxidant, and antimicrobial properties (Figure 5).
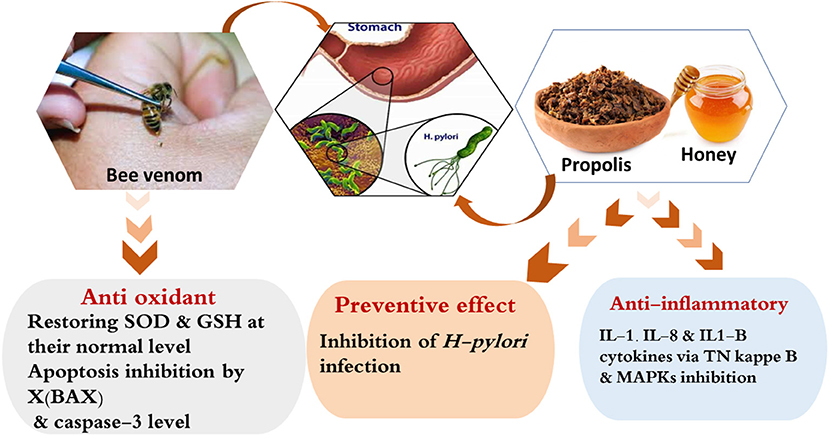
Figure 5. Possible mechanism of actions explaining the anti-peptic ulcers effect of the bee products.
The Safety of Bee Products
An allergic reaction could occur following the use of bee products but it is rare, hypersensitive reactions to honey vary from a cough to anaphylaxis (28, 149, 150). This allergic reaction could be attributed to various causes, such as the bee pollen content, mold spores, algae, and other bee-derived proteins (151). The consumption of royal jelly could be associated with serious adverse reactions, such as contact dermatitis, acute asthma, anaphylaxis, and even death for some individuals (152, 153). In countries with a high utilization of royal jelly, positive skin prick tests are used to diagnose atopic reactions (154). Clinical studies have shown that serum IgE antibodies against a number of royal jelly components are found in subjects with clinical sensitivity. These IgE anti-binding proteins are mostly derived from major RJPHs, especially MRJP1 and MRJP2. Antibody-binding proteins with a molecular weight of 25–55 kDa have been identified in a study in the sera of subjects that showed an adverse reaction to royal jelly, where two proteins with molecular weights of 47 and 55 kDa may be the most diagnostic biomarkers discovered so far (155, 156). IgE-binding proteins are not specific to royal jelly and can be present in other cases of allergies. This can be explained by the contamination and cross-reactivity of RJPHs with inhalant allergens, such as pollen.
Airborne pollen is a known cause of respiratory allergy, which might later progress to a life-threatening allergic reaction. Although bee pollen is mainly made up of the pollen of entomophilous plants that attract insects for their pollination, it also contains a significant amount of pollen from wind-pollinated trees or weeds (anemophilous plants), which may induce hypersensitive reactions after the ingestion of bee pollen (28, 156). In addition, bee pollen can be contaminated by different types of fungi, such as Aspergillus, Cladosporium, and Alternaria, which can induce allergic reactions (28).
Because the Gram-positive Bacterium Clostridium botulinum, which is considered a potential risk factor for baby botulism, and can be found in both honey and bee pollen, the World Health Organization prohibits the usage of honey for newborns under the age of one. C. botulinum's can produce eight distinct toxins (A, B, C1, C2, D, E, F, G) (157–159). Also, allergic reactions to bee venom have been observed. As a result of this hypersensitivity, anaphylaxis may arise and develop into fatal shock (160). Compared with airborne allergens, such as mites and pollen (161), venom allergens are directly injected into the skin and easily reach the bloodstream. This promotes an immune response that manifests in the form of edema, erythema, and pruritus at 10 cm in diameter or greater. The skin inflammation that occurs in response to venom allergens takes 1–2 days to appear and 3–10 days to be resolved.
Therefore, the accurate diagnosis of an allergy to insect venom is important and is based on history, skin tests, and the determination of specific IgE antibodies for bee venom (162, 163). The allergen crosslinking of IgE bound to mast cells leads to the release of histamine, lipid mediators, enzymes, cytokines, and chemokines responsible for the acute allergic response. Cross-reactivity can occur due to similarities between the composition of venom and the structures of single allergens (164). Similarities can be found between different bee species, and even between bees and wasps (165). Cross-reactive carbohydrate determinants were found to act as antigens against IgE in the sera of patients with bee venom allergies, so this may be the cause of honeybee venom allergies (165).
The main therapeutic options for bee venom allergy are in the form of emergency medications, including antihistamines, epinephrine (adrenaline) injection, and corticosteroids. Moreover, venom-specific immunotherapy has been developed, whereby repeated injections of increasing venom doses are given to a patient over a period of years to reduce allergic symptoms (160, 166, 167).
Conclusion
Despite the vital role of inflammation as a potential defense mechanism of the body, its uncontrolled regulation can result in various autoimmune diseases, which cause serious health problems. Bee products are apitherapeutic agents, rich in hundreds of bioactive chemical compounds that account for their various biological activities, such as their antioxidant, anti-inflammatory, immunomodulatory, neuroprotective, and antimicrobial properties. Different bee products and their bioactive ingredients have been shown to be successful in the protection and treatment of various autoimmune diseases in both preclinical and clinical studies.
In preclinical studies, bee products improve the immune response via the modulation of B and T lymphocytes function and chemotaxis. Also, they inhibit the production of inflammatory cytokines and regulate intracellular signaling pathways. Taken together, these results supported the clinical studies of bee products. For instance, in diabetes, propolis helped increasing TLR-2 and TLR-4, thus activating the B and T function, improving the lipid profile, reducing the ROS and modulating the HbA1C and FSG glycemic factors. Meanwhile, honey, propolis and their phenolic compounds can act as chemoprevention and tumor growth inhibitors in cancer cells. Antibacterial, anti-caries, and antiplaque properties of propolis play important role in the treatment of periodontal diseases such as gingival and caries. The gastroprotective effect of honey and propolis is related to the inhibition of H-pylori infection and the reduction of the NSAIDs-related inflammation.
However, to date, there have been a few applicable clinical studies on the roles that bee products play in the treatment of inflammatory and autoimmune diseases. Also, adverse allergic reactions and unselective toxicity still present challenges in the development of bee products if they are to be used to achieve the greatest scientific and commercial outcomes in drug discovery development. Therefore, it is important to carry out more clinical studies to explore the benefits of the use of bee products in future drug discovery. It is also critical to take care of honeybees as a source of bee products, especially in the aftermath of colony collapse disorder.
Author Contributions
HE-S and SK: conceptualization and supervision. NE, SK, and AAA: writing of the original draft. SK, HE-S, MR, HA, AFA, CZ, YA, SA, HT, BX, and KW: revision and editing of the final version. All authors have read and agreed to the published version of the manuscript.
Funding
This work was supported by Swedish Research links (Grant VR 2016–05885), National Natural Science Foundation of China (Grant No. 32172791), and State Key Laboratory of Animal Nutrition (2004DA125184F1904).
Conflict of Interest
The authors declare that the research was conducted in the absence of any commercial or financial relationships that could be construed as a potential conflict of interest.
Publisher's Note
All claims expressed in this article are solely those of the authors and do not necessarily represent those of their affiliated organizations, or those of the publisher, the editors and the reviewers. Any product that may be evaluated in this article, or claim that may be made by its manufacturer, is not guaranteed or endorsed by the publisher.
Acknowledgments
Authors are very grateful to the Swedish Research links Grant VR 2016–05885 and the Department of Molecular Biosciences, Wenner-Grens Institute, Stockholm University, Sweden, for the financial support. Authors also gratefully acknowledged the financial support from the National Natural Science Foundation of China and open project foundation from State Key Laboratory of Animal Nutrition, China.
References
1. Turner MD, Nedjai B, Hurst T, Pennington DJ. Cytokines and chemokines: at the crossroads of cell signalling and inflammatory disease. Biochim Biophys Acta. (2014) 1843:2563–82. doi: 10.1016/j.bbamcr.2014.05.014
2. Chen L, Deng H, Cui H, Fang J, Zuo Z, Deng J, et al. Inflammatory responses and inflammation-associated diseases in organs. Oncotarget. (2018) 9:7204–18. doi: 10.18632/oncotarget.23208
3. Wei Z, Liu HT. MAPK signal pathways in the regulation of cell proliferation in mammalian cells. Cell Res. (2002) 12:9–18. doi: 10.1038/sj.cr.7290105
4. Kany S, Vollrath JT, Relja B. Cytokines in inflammatory disease. Int J Mol Sci. (2019) 20:6008–37. doi: 10.3390/ijms20236008
5. Lanas A, Chan FKL. Peptic ulcer disease. Lancet. (2017) 390:613–24. doi: 10.1016/S0140-6736(16)32404-7
6. Paquette DW, Brodala N, Nichols TC. Cardiovascular disease, inflammation, and periodontal infection. Periodontol 2000. (2007) 44:113–26. doi: 10.1111/j.1600-0757.2006.00196.x
7. El-Seedi HR, Khalifa SAM, Abd El-Wahed AA, Gao R, Guo Z, Tahir HE, et al. Honeybee products: an updated review of neurological actions. Trends Food Sci Technol. (2020) 101:17–27. doi: 10.1016/j.tifs.2020.04.026
8. Thakur M, Nanda V. Composition and functionality of bee pollen: a review. Trends Food Sci Technol. (2020) 98:82–106. doi: 10.1016/j.tifs.2020.02.001
9. Nainu F, Masyita A, Bahar MA, Raihan M, Prova SR, Mitra S, et al. Pharmaceutical prospects of bee products: special focus on anticancer, antibacterial, antiviral, and antiparasitic properties. Antibiotics. (2021) 10:822–58. doi: 10.3390/antibiotics10070822
10. Ranneh Y, Akim AM, Hamid HA, Khazaai H, Fadel A, Zakaria ZA, et al. Honey and its nutritional and antiinflammatory value. BMC Complement Med Ther. (2021) 21:30–46. doi: 10.1186/s12906-020-03170-5
11. Huang S, Zhang CP, Wang K, Li GQ, Hu FL. Recent advances in the chemical composition of propolis. Molecules. (2014) 19:19610–32. doi: 10.3390/molecules191219610
12. Carpena M, Nuñez-Estevez B, Soria-Lopez A, Simal-Gandara J. Bee venom: an updating review of its bioactive molecules and its health applications. Nutrients. (2020) 12:3360–86. doi: 10.3390/nu12113360
13. Campoccia D, Ravaioli S, Santi S, Mariani V, Santarcangelo C, De Filippis A, et al. Exploring the anticancer effects of standardized extracts of poplar-type propolis: In vitro cytotoxicity toward cancer and normal cell lines. Biomed Pharmacother. (2021) 141:111895. doi: 10.1016/j.biopha.2021.111895
14. Soleimani D, Miryan M, Tutunchi H, Navashenaq JG, Sadeghi E, Ghayour-Mobarhan M, et al. A systematic review of preclinical studies on the efficacy of propolis for the treatment of inflammatory bowel disease. Phyther Res. (2021) 35:701–10. doi: 10.1002/ptr.6856
15. Ibrahim MEE-D, Alqurashi RM. Anti-fungal and antioxidant properties of propolis (bee glue) extracts. Int J Food Microbiol. (2021) 361:109463. doi: 10.1016/j.ijfoodmicro.2021.109463
16. Yosri N, Abd El-Wahed AA, Ghonaim R, Khattab OM, Sabry A, Ibrahim MAA, et al. Anti-viral and immunomodulatory properties of propolis: chemical diversity, pharmacological properties, preclinical and clinical applications, and in silico potential against sars-cov-2. Foods. (2021) 10:1776–822. doi: 10.3390/foods10081776
17. Szliszka E, Czuba ZP, Domino M, Mazur B, Zydowicz G, Krol W. Ethanolic extract of propolis (EEP) enhances the apoptosis- inducing potential of trail in cancer cells. Molecules. (2009) 14:738–54. doi: 10.3390/molecules14020738
18. Aufschnaiter A, Kohler V, Khalifa S, El-Wahed A, Du M, El-Seedi H, et al. Apitoxin and its components against cancer, neurodegeneration and rheumatoid arthritis: limitations and possibilities. Toxins (Basel). (2020) 12:66–80. doi: 10.3390/toxins12020066
19. El-Seedi H, El-Wahed AA, Yosri N, Musharraf SG, Chen L, Moustafa M, et al. Antimicrobial properties of Apis mellifera's bee venom. Toxins (Basel). (2020) 12:451–67. doi: 10.3390/toxins12070451
20. Miao W, Shim G, Lee S, Lee S, Choe YS, Oh YK. Safety and tumor tissue accumulation of pegylated graphene oxide nanosheets for co-delivery of anticancer drug and photosensitizer. Biomaterials. (2013) 34:3402–10. doi: 10.1016/j.biomaterials.2013.01.010
21. Khalifa SAM, Elashal MH, Yosri N, Du M, Musharraf SG, Nahar L, et al. Bee pollen: current status and therapeutic potential. Nutrients. (2021) 13:1876–90. doi: 10.3390/nu13061876
22. Ahmad S, Campos MG, Fratini F, Altaye SZ, Li J. New insights into the biological and pharmaceutical properties of royal jelly. Int J Mol Sci. (2020) 21:382–407. doi: 10.3390/ijms21020382
23. Ramanathan ANKG, Nair AJ, Sugunan VS. A review on royal jelly proteins and peptides. J Funct Foods. (2018) 44:255–64. doi: 10.1016/j.jff.2018.03.008
24. Machado JL, Assunçao AK, da Silva MC, Reis AS, Costa GC, Arruda DD, et al. Brazilian green propolis: anti-inflammatory property by an immunomodulatory activity. Evid Based Complement Altern Med. (2012) 2012:157652. doi: 10.1155/2012/157652
25. McLoone P, Warnock M, Fyfe L. Honey: an immunomodulatory agent for disorders of the skin. Food Agric Immunol. (2016) 27:338–49. doi: 10.1080/09540105.2015.1104653
26. Kocyigit A, Guler EM, Kaleli S. Anti-inflammatory and antioxidative properties of honey bee venom on freund's complete adjuvant-induced arthritis model in rats. Toxicon. (2019) 161:4–11. doi: 10.1016/j.toxicon.2019.02.016
27. Maruyama H, Sakamoto T, Araki Y, Hara H. Anti-inflammatory effect of bee pollen ethanol extract from Cistus sp. of Spanish on carrageenan-induced rat hind paw edema. BMC Complement Altern Med. (2010) 10:30–40. doi: 10.1186/1472-6882-10-30
28. Choi JH, Jang YS, Oh JW, Kim CH, Hyun IG. Bee pollen-induced anaphylaxis: a case report and literature review. Allergy Asthma Immunol Res. (2015) 7:513–7. doi: 10.4168/aair.2015.7.5.513
29. Roh J, Jo N. Systemic immediate hypersensitive reactions after treatment with sweet bee venom: a casereport. J Pharmacopuncture. (2015) 18:59–62. doi: 10.3831/KPI.2015.18.039
30. Paola F, Pantalea DD, Gianfranco C, Antonio F, Angelo V, Eustachio N, et al. Oral allergy syndrome in a child provoked by royal jelly. Case Rep Med. (2014) 2014:941248. doi: 10.1155/2014/941248
31. Mihajlovic D, Rajkovic I, Chinou I, Colic M. Dose-dependent immunomodulatory effects of 10-hydroxy-2-decenoic acid on human monocyte-derived dendritic cells. J Funct Foods. (2013) 5:838–46. doi: 10.1016/j.jff.2013.01.031
32. Effects A. Anti-inflammatory applications of melittin, a major component of bee venom: detailed mechanism of action and adverse effects. Molecules. (2016) 21:616–25. doi: 10.3390/molecules21050616
33. Kim WH, An HJ, Kim JY, Gwon MG, Gu H, Park JB, et al. Bee venom inhibits Porphyromonas gingivalis lipopolysaccharides-induced pro-inflammatory cytokines through suppression of NF-κB and AP-1 signaling pathways. Molecules. (2016) 21:1508–22. doi: 10.3390/molecules21111508
34. Zhou W, Zhao Y, Yan Y, Mi J, Lu L, Luo Q, et al. Antioxidant and immunomodulatory activities in vitro of polysaccharides from bee collected pollen of Chinese wolfberry. Int J Biol Macromol. (2020) 163:190–9. doi: 10.1016/j.ijbiomac.2020.06.244
35. Hozzein WN, Badr G, Al Ghamdi AA, Sayed A, Al-Waili NS, Garraud O. Topical application of propolis enhances cutaneous wound healing by promoting TGF-beta/smad-mediated collagen production in a streptozotocin-induced type I diabetic mouse model. Cell Physiol Biochem. (2015) 37:940–54. doi: 10.1159/000430221
36. Odegaard JI, Chawla A. Connecting type 1 and type 2 diabetes through innate immunity. Cold Spring Harb Perspect Med. (2012) 2:a007724. doi: 10.1101/cshperspect.a007724
37. Al Ghamdi AA, Badr G, Hozzein WN, Allam A, Al-Waili NS, Al-Wadaan MA, et al. Oral supplementation of diabetic mice with propolis restores the proliferation capacity and chemotaxis of B and T lymphocytes towards CCL21 and CXCL12 by modulating the lipid profile, the pro-inflammatory cytokine levels and oxidative stress. BMC Immunol. (2015) 16:54–67. doi: 10.1186/s12865-015-0117-9
38. Orsatti CL, Missima F, Pagliarone AC, Bachiega TF, Búfalo MC, Araújo JPSJ Jr. Propolis immunomodulatory action in vivo on Toll-Like receptors 2 and 4 expression and on Pro-Infl ammatory cytokines production in mice. Phyther Res. (2010) 22:1141–6. doi: 10.1002/ptr.3086
39. El Rabey HA, Al-Seeni MN, Bakhashwain AS. The antidiabetic activity of nigella sativa and propolis on streptozotocin-induced diabetes and diabetic nephropathy in male rats. Evid Based Complement Altern Med. (2017) 2017:5439645. doi: 10.1155/2017/5439645
40. Li X, Gong H, Yang S, Yang L, Fan Y, Zhou Y. Pectic bee pollen polysaccharide from Rosa rugosa alleviates diet-induced hepatic steatosis and insulin resistance via induction of AMPK/mTOR-mediated autophagy. Molecules. (2017) 22:699–711. doi: 10.3390/molecules22050699
41. Kröger J, Zietemann V, Enzenbach C, Weikert C, Jansen EHJM, Döring F, et al. Erythrocyte membrane phospholipid fatty acids, desaturase activity, and dietary fatty acids in relation to risk of type 2 diabetes in the European prospective investigation into cancer and nutrition (EPIC)-potsdam study. Am J Clin Nutr. (2011) 93:127–42. doi: 10.3945/ajcn.110.005447
42. Kocot J, Kiełczykowska M, Luchowska-Kocot D, Kurzepa J, Musik I. Antioxidant potential of propolis, bee pollen, and royal jelly: Possible medical application. Oxid Med Cell Longev. (2018) 2018:7074209. doi: 10.1155/2018/7074209
43. El Adaouia Taleb R, Djebli N, Chenini H, Sahin H, Kolayli S. In vivo and in vitro anti-diabetic activity of ethanolic propolis extract. J Food Biochem. (2020) 44:e13267. doi: 10.1111/jfbc.13267
44. Pittalà V, Salerno L, Romeo G, Acquaviva R, Di Giacomo C, Sorrenti V. Therapeutic potential of caffeic acid phenethyl ester (CAPE) in diabetes. Curr Med Chem. (2018) 25:4827–36. doi: 10.2174/0929867324666161118120908
45. Fuliang HU, Hepburn HR, Xuan H, Chen M, Daya S, Radloff SE. Effects of propolis on blood glucose, blood lipid and free radicals in rats with diabetes mellitus. Pharmacol Res. (2005) 51:147–52. doi: 10.1016/j.phrs.2004.06.011
46. Oršolić N, Sirovina D, Gajski G, Garaj-Vrhovac V, Jembrek MJ, Kosalec I. Assessment of DNA damage and lipid peroxidation in diabetic mice: effects of propolis and epigallocatechin gallate (EGCG). Mutat Res. (2013) 757:36–44. doi: 10.1016/j.mrgentox.2013.04.022
47. Oršolić N, Sirovina D, Končić MZ, Lacković G, Gregorović G. Effect of croatian propolis on diabetic nephropathy and liver toxicity in mice. BMC Complement Altern Med. (2012) 12:117–31. doi: 10.1186/1472-6882-12-117
48. Aral CA, Kesim S, Greenwell H, Kara M, Çetin A, Yakan B. Alveolar bone protective and hypoglycemic effects of systemic propolis treatment in experimental periodontitis and diabetes mellitus. J Med Food. (2015) 18:195–201. doi: 10.1089/jmf.2013.3137
49. Mohamed NA, Ahmed OM, Hozayen WG, Ahmed MA. Ameliorative effects of bee pollen and date palm pollen on the glycemic state and male sexual dysfunctions in streptozotocin-Induced diabetic wistar rats. Biomed Pharmacother. (2018) 97:9–18. doi: 10.1016/j.biopha.2017.10.117
50. Clinicaltrials. Available online at: https://clinicaltrials.gov/ct2/show/NCT02794506 (accessed June 12, 2021).
51. Samadi N, Mozaffari-Khosravi H, Rahmanian M, Askarishahi M. Effects of bee propolis supplementation on glycemic control, lipid profile and insulin resistance indices in patients with type 2 diabetes: a randomized, double-blind clinical trial. J Integr Med. (2017) 15:124–34. doi: 10.1016/S2095-4964(17)60315-7
52. Clinicaltrials. Available online at: https://clinicaltrials.gov/ct2/show/NCT03416127 (accessed November 12, 2021).
53. Khoshpey B, Djazayeri S, Amiri F, Malek M, Hosseini AF, Hosseini S, et al. Effect of royal ielly intake on serum glucose, apolipoprotein A-I (ApoA-I), apolipoprotein B (ApoB) and ApoB/ApoA-I Ratios in patients with Type 2 diabetes: a randomized, double-blind clinical trial study. Can J Diabetes. (2016) 40:324–8. doi: 10.1016/j.jcjd.2016.01.003
54. Clinicaltrials. Available online at: https://clinicaltrials.gov/ct2/show/NCT01554566 (accessed June 12, 2021).
55. Zakerkish M, Jenabi M, Zaeemzadeh N, Hemmati AA, Neisi N. The effect of iranian propolis on glucose metabolism, lipid profile, insulin resistance, renal function and inflammatory biomarkers in patients with type 2 diabetes mellitus: a randomized double-blind clinical trial. Sci Rep. (2019) 9:7289–99. doi: 10.1038/s41598-019-43838-8
56. IRCT. Available online at: https://en.irct.ir/trial/23981 (accessed June 12, 2021).
57. Abdulrhman MM, El-Hefnawy MH, Aly RH, Shatla RH, Mamdouh RM, Mahmoud DM, et al. Metabolic effects of honey in type 1 diabetes mellitus: a randomized crossover pilot study. J Med Food. (2013) 16:66–72. doi: 10.1089/jmf.2012.0108
58. Grivennikov SI, Greten FR, Karin M. Immunity, inflammation, and cancer. Cell. (2010) 140:883–99. doi: 10.1016/j.cell.2010.01.025
59. Sova M, Saso L. Design and development of Nrf2 modulators for cancer chemoprevention and therapy: a review. Drug Des Devel Ther. (2018) 12:3181–97. doi: 10.2147/DDDT.S172612
60. Rather RA, Bhagat M. Cancer chemoprevention and piperine: molecular mechanisms and therapeutic opportunities. Front Cell Dev Biol. (2018) 6:10. doi: 10.3389/fcell.2018.00010
61. Afrin S, Haneefa SM, Fernandez-Cabezudo MJ, Giampieri F, Al-Ramadi BK, Battino M. Therapeutic and preventive properties of honey and its bioactive compounds in cancer: an evidence-based review. Nutr Res Rev. (2020) 33:50–76. doi: 10.1017/S0954422419000192
62. Berishaj M, Gao SP, Ahmed S, Leslie K, Al-Ahmadie H, Gerald WL, et al. Stat3 is tyrosine-phosphorylated through the interleukin-6/glycoprotein 130/Janus kinase pathway in breast cancer. Breast Cancer Res. (2007) 9:1–8. doi: 10.1186/bcr1680
63. Aryappalli P, Shabbiri K, Masad RJ, Al-Marri RH, Haneefa SM, Mohamed YA, et al. Inhibition of tyrosine-phosphorylated STAT3 in human breast and lung cancer cells by manuka honey is mediated by selective antagonism of the IL-6 receptor. Int J Mol Sci. (2019) 20:4340–60. doi: 10.3390/ijms20184340
64. Chiu HF, Han YC, Shen YC, Golovinskaia O, Venkatakrishnan K, Wang CK. Chemopreventive and chemotherapeutic effect of propolis and its constituents: a mini-review. J Cancer Prev. (2020) 25:70–8. doi: 10.15430/JCP.2020.25.2.70
65. Oršolić N, Šver L, Terzić S, Tadić Z, Bašić I. Inhibitory effect of water-soluble derivative of propolis and its polyphenolic compounds on tumor growth and metastasizing ability: a possible mode of antitumor action. Nutr Cancer. (2003) 47:156–63. doi: 10.1207/s15327914nc4702_8
66. Heo MY, Sohn SJ, Au WW. Anti-genotoxicity of galangin as a cancer chemopreventive agent candidate. Mutat Res. (2001) 488:135–50. doi: 10.1016/S1383-5742(01)00054-0
67. Elbaz NM, Khalil IA, Abd-Rabou AA, El-Sherbiny IM. Chitosan-based nano-in-microparticle carriers for enhanced oral delivery and anticancer activity of propolis. Int J Biol Macromol. (2016) 92:254–69. doi: 10.1016/j.ijbiomac.2016.07.024
68. Watanabe MAE, Amarante MK, Conti BJ, Sforcin JM. Cytotoxic constituents of propolis inducing anticancer effects: a review. J Pharm Pharmacol. (2011) 63:1378–86. doi: 10.1111/j.2042-7158.2011.01331.x
69. Yang YC, Chou WM, Widowati DA, Lin IP, Peng CC. 10-Hydroxy-2-decenoic acid of royal jelly exhibits bactericide and anti-inflammatory activity in human colon cancer cells. BMC Complement Altern Med. (2018) 18:202–8. doi: 10.1186/s12906-018-2267-9
70. Omene CO, Wu J, Frenkel K. Caffeic acid phenethyl ester (CAPE) derived from propolis, a honeybee product, inhibits growth of breast cancer stem cells. Invest New Drugs. (2012) 30:1279–88. doi: 10.1007/s10637-011-9667-8
71. Szliszka E, Zydowicz G, Janoszka B, Dobosz C, Kowalczyk-Ziomek G, Krol W. Ethanolic extract of Brazilian green propolis sensitizes prostate cancer cells to TRAIL-induced apoptosis. Int J Oncol. (2011) 38:941–53. doi: 10.3892/ijo.2011.930
72. Busch VM, Pereyra-Gonzalez A, Šegatin N, Santagapita PR, Poklar Ulrih N, Buera MP. Propolis encapsulation by spray drying: characterization and stability. LWT. (2017) 75:227–35. doi: 10.1016/j.lwt.2016.08.055
73. Butnariu MV, Giuchici CV. The use of some nanoemulsions based on aqueous propolis and lycopene extract in the skin's protective mechanisms against UVA radiation. J Nanobiotechnol. (2011) 9:3–11. doi: 10.1186/1477-3155-9-3
74. Soman NR, Baldwin SL, Hu G, Marsh JN, Lanza GM, Heuser JE, et al. Molecularly targeted nanocarriers deliver the cytolytic peptide melittin specifically to tumor cells in mice, reducing tumor growth. J Clin Invest. (2009) 119:2830–42. doi: 10.1172/JCI38842
75. Clinicaltrials. Available online at: https://clinicaltrials.gov/ct2/show/NCT01375088 (accessed June 12, 2021).
76. Araki K, Miyata Y, Ohba K, Nakamura Y, Matsuo T, Mochizuki Y, et al. Oral intake of royal jelly has protective effects against tyrosine kinase inhibitor-induced toxicity in patients with renal cell carcinoma: A randomized, double-blinded, placebo-controlled trial. Medicines. (2018) 6:2–12. doi: 10.3390/medicines6010002
77. Rao S, Hegde S, Rao P, Dinkar C, Thilakchand K, George T, et al. Honey mitigates radiation-induced oral mucositis in head and neck cancer patients without affecting the tumor response. Foods. (2017) 6:77–84. doi: 10.3390/foods6090077
78. Clinicaltrials. Available online at: https://clinicaltrials.gov/show/NCT03399331 (accessed January 19, 2021).
79. Al Jaouni SK, Al Muhayawi MS, Hussein A, Elfiki I, Al-Raddadi R, Al Muhayawi SM, et al. Effects of honey on oral mucositis among pediatric cancer patients undergoing chemo/radiotherapy treatment at king abdulaziz university hospital in jeddah, kingdom of saudi arabia. Evid Based Complement Altern Med. (2017) 2017:5861024. doi: 10.1155/2017/5861024
80. Clinicaltrials. Available online at: https://clinicaltrials.gov/ct2/show/NCT04382079 (accessed November 12, 2021).
81. Clinicaltrials. Available online at: https://clinicaltrials.gov/ct2/show/NCT03684096 (accessed November 13, 2021).
82. Pacurari M, Kafoury R, Tchounwou PB, Ndebele K. The renin-angiotensin-aldosterone system in vascular inflammation and remodeling. Int J Inflam. (2014) 2014:689360. doi: 10.1155/2014/689360
83. Rzepecka-Stojko A, Kabała-Dzik A, Kubina R, Jasik K, Kajor M. Wrze' sniok D, Stojko J. Protective effect of polyphenol-rich extract from bee pollen in a high-fat diet. Molecules. (2018) 23:805–22. doi: 10.3390/molecules23040805
84. Pan Y, Rong Y, You M, Ma Q, Chen M, Hu F. Royal jelly causes hypotension and vasodilation induced by increasing nitric oxide production. Food Sci Nutr. (2019) 7:1361–70. doi: 10.1002/fsn3.970
85. Takaki-Doi S, Hashimoto K, Yamamura M, Kamei C. Antihypertensive activities of royal jelly protein hydrolysate and its fractions in spontaneously hypertensive rats. Acta Med Okayama. (2009) 63:57–64. doi: 10.18926/AMO/31859
86. Fan P, Han B, Feng M, Fang Y, Zhang L, Hu H, et al. Functional and proteomic investigations reveal major royal jelly protein 1 associated with anti-hypertension activity in mouse vascular smooth muscle cells. Sci Rep. (2016) 6:30230–41. doi: 10.1038/srep30230
87. Selamoglu Talas Z. Propolis reduces oxidative stress in l-NAME-induced hypertension rats. Cell Biochem Funct. (2014) 32:150–4. doi: 10.1002/cbf.2986
88. Clinicaltrials. Available online at: https://clinicaltrials.gov/ct2/show/NCT04117451 (accessed June 12, 2021).
89. Kinane DF, Stathopoulou PG, Papapanou PN. Periodontal diseases. Nat Rev Dis Prim. (2017) 3:1–14. doi: 10.1038/nrdp.2017.38
90. Hayacibara MF, Koo H, Rosalen PL, Duarte S, Franco EM, Bowen WH, et al. In vitro and in vivo effects of isolated fractions of Brazilian propolis on caries development. J Ethnopharmacol. (2005) 101:110–5. doi: 10.1016/j.jep.2005.04.001
91. Vagish Kumar LS. Propolis in dentistry and oral cancer management. N Am J Med Sci. (2014) 6:250–9. doi: 10.4103/1947-2714.134369
92. Giacaman RA. Sugars and beyond. The role of sugars and the other nutrients and their potential impact on caries. Oral Dis. (2018) 24:1185–97. doi: 10.1111/odi.12778
93. Duarte S, Rosalen PL, Hayacibara MF, Cury JA, Bowen WH, Marquis RE, et al. The influence of a novel propolis on mutans streptococci biofilms and caries development in rats. Arch Oral Biol. (2006) 51:15–22. doi: 10.1016/j.archoralbio.2005.06.002
94. Niu Y, Wang K, Zheng S, Wang Y, Ren Q, Li H, et al. Antibacterial effect of caffeic acid phenethyl ester on cariogenic bacteria and Streptococcus mutans biofilms. Antimicrob Agents Chemother. (2020) 64:e00251–20. doi: 10.1128/AAC.00251-20
95. Asgharpour F, Moghadamnia AA, Zabihi E, Kazemi S, Ebrahimzadeh Namvar A, Gholinia H, et al. Iranian propolis efficiently inhibits growth of oral streptococci and cancer cell lines. BMC Complement Altern Med. (2019) 19:266–73. doi: 10.1186/s12906-019-2677-3
96. Bueno-Silva B, Koo H, Falsetta ML, Alencar SM, Ikegaki M, Rosalen PL. Effect of neovestitol-vestitol containing Brazilian red propolis on accumulation of biofilm in vitro and development of dental caries in vivo. Biofouling. (2013) 29:1233–42. doi: 10.1080/08927014.2013.834050
97. Clinicaltrials. Available online at: https://clinicaltrials.gov/ct2/show/NCT03394729 (accessed June 12, 2021).
98. Clinicaltrials. Available online at: https://clinicaltrials.gov/ct2/show/NCT01142843 (accessed June 12, 2021).
99. Clinicaltrials. Available online at: https://clinicaltrials.gov/ct2/show/NCT03533231 (accessed June 12, 2021).
100. Clinicaltrials. Available online at: https://clinicaltrials.gov/ct2/show/NCT03553628 (accessed June 12, 2021).
101. Clinicaltrials. Available online at: https://clinicaltrials.gov/ct2/show/NCT03222960 (accessed June 12, 2021).
102. Clinicaltrials. Available online at: https://clinicaltrials.gov/ct2/show/NCT03812315 (accessed June 12, 2021).
103. Clinicaltrials. Available online at: https://clinicaltrials.gov/ct2/show/NCT02052973 (accessed June 12, 2021).
104. Clinicaltrials. Available online at: https://clinicaltrials.gov/ct2/show/NCT04145102 (accessed January 19, 2021).
105. Clinicaltrials. Available online at: https://clinicaltrials.gov/ct2/show/NCT01943877 (accessed November 12, 2021).
106. Clinicaltrials. Available online: https://clinicaltrials.gov/ct2/show/NCT01750801 (accessed November 12, 2021).
107. DE Luca MP, Freires IA, Gala-García A, Santos VR, Vale MP, Alencar SM de, et al. The anti-caries activity and toxicity of an experimental propolis-containing varnish. Braz Oral Res. (2017) 31:e45. doi: 10.1590/1807-3107bor-2017.vol31.0045
108. Han SM, Yeo JH, Cho YH, Pak SC. Royal Jelly reduces melanin synthesis through down-regulation of tyrosinase expression. Am J Chin Med. (2011) 39:1253–60. doi: 10.1142/S0192415X11009536
109. Peng CC, Sun HT, Lin IP, Kuo PC Li JC. The functional property of royal jelly 10-hydroxy-2-decenoic acid as a melanogenesis inhibitor. BMC Complement Altern Med. (2017) 17:4–11. doi: 10.1186/s12906-017-1888-8
110. Kurek-Górecka A, Górecki M, Rzepecka-Stojko A, Balwierz R, Stojko J. Bee products in dermatology and skin care. Molecules. (2020) 25:556–72. doi: 10.3390/molecules25030556
111. Sofiabadi M, Samiee-Rad F. Royal jelly accelerates healing of acetate induced gastric ulcers in male rats. Gastroenterol Hepatol Bed Bench. (2020) 13:18–22.
112. Kunugi H, Ali AM. Royal jelly and its components promote healthy aging and longevity: from animal models to humans. Int J Mol Sci. (2019) 20:4662–87. doi: 10.3390/ijms20194662
113. Park HM, Cho MH, Cho Y, Kim SY. Royal jelly increases collagen production in rat skin after ovariectomy. J Med Food. (2012) 15:568–75. doi: 10.1089/jmf.2011.1888
114. Oryan A, Alemzadeh E, Moshiri A. Potential role of propolis in wound healing: biological properties and therapeutic activities. Biomed Pharmacother. (2018) 98:469–83. doi: 10.1016/j.biopha.2017.12.069
115. Corrêa FRS, Schanuel FS, Moura-Nunes N, Monte-Alto-Costa A, Daleprane JB. Brazilian red propolis improves cutaneous wound healing suppressing inflammation-associated transcription factor NFκB. Biomed Pharmacother. (2017) 86:162–71. doi: 10.1016/j.biopha.2016.12.018
116. Rossi A, Longo R, Russo A, Borrelli F, Sautebin L. The role of the phenethyl ester of caffeic acid (CAPE) in the inhibition of rat lung cyclooxygenase activity by propolis. Fitoterapia. (2002) 73:30–7. doi: 10.1016/S0367-326X(02)00188-0
117. Hämäläinen M, Nieminen R, Asmawi MZ, Vuorela P, Vapaatalo H, Moilanen E. Effects of flavonoids on prostaglandin E2 production and on COX-2 and mPGES-1 expressions in activated macrophages. Planta Med. (2011) 77:1504–11. doi: 10.1055/s-0030-1270762
118. Al-waili N. Mixing two different propolis samples potentiates their antimicrobial activity and wound healing property: a novel approach in wound healing and infection. Vet World. (2018) 11:1188–95. doi: 10.14202/vetworld.2018.1188-1195
119. Zhou Y, Chyu J, Zumwalt M. Recent progress of fabrication of cell scaffold by electrospinning technique for articular cartilage tissue engineering. Int J Biomater. (2018) 2018:1953636. doi: 10.1155/2018/1953636
120. Jatoi AW, Ogasawara H, Kim IS Ni QQ. Polyvinyl alcohol nanofiber based three phase wound dressings for sustained wound healing applications. Mater Lett. (2019) 241:168–71. doi: 10.1016/j.matlet.2019.01.084
121. Alberti TB, Coelho DS, de Prá M, Maraschin M, Veleirinho B. Electrospun PVA nanoscaffolds associated with propolis nanoparticles with wound healing activity. J Mater Sci. (2020) 55:9712–27. doi: 10.1007/s10853-020-04502-z
122. Khoshnevisan K, Maleki H, Samadian H, Doostan M, Khorramizadeh MR. Antibacterial and antioxidant assessment of cellulose acetate/polycaprolactone nanofibrous mats impregnated with propolis. Int J Biol Macromol. (2019) 140:1260–8. doi: 10.1016/j.ijbiomac.2019.08.207
123. Krupp T, dos Santos BD, Gama LA, Silva JR, Arrais-Silva WW, de Souza NC, et al. Natural rubber -propolis membrane improves wound healing in second-degree burning model. Int J Biol Macromol. (2019) 131:980–8. doi: 10.1016/j.ijbiomac.2019.03.147
124. Eskandarinia A, Kefayat A, Agheb M, Rafienia M, Amini Baghbadorani M, Navid S, et al. A novel bilayer wound dressing composed of a dense polyurethane/propolis membrane and a biodegradable polycaprolactone/gelatin nanofibrous scaffold. Sci Rep. (2020) 10:3063–30674. doi: 10.1038/s41598-020-59931-2
125. McLoone P, Tabys D, Fyfe L. Honey combination therapies for skin and wound infections: a systematic review of the literature. Clin Cosmet Investig Dermatol. (2020) 13:875–88. doi: 10.2147/CCID.S282143
126. Clinicaltrials. Available online at: https://clinicaltrials.gov/ct2/show/NCT00435474 (accessed June 12, 2021).
127. Clinicaltrials. Available online at: https://clinicaltrials.gov/ct2/show/NCT02577900 (accessed June 12, 2021).
128. Clinicaltrials. Available online at: https://clinicaltrials.gov/ct2/show/NCT01531517 (accessed June 12, 2021).
129. Clinicaltrials. Available online at: https://clinicaltrials.gov/ct2/show/NCT02482948 (accessed June 12, 2021).
130. Clinicaltrials. Available online at: https://clinicaltrials.gov/ct2/show/NCT03934281 (accessed June 12, 2021).
131. Clinicaltrials. Available online at: https://clinicaltrials.gov/ct2/show/NCT03641053 (accessed November 12, 2021).
132. Clinicaltrials. Available online at: https://clinicaltrials.gov/ct2/show/NCT04849143 (accessed November 12, 2021).
133. Clinicaltrials. Available online at: https://clinicaltrials.gov/ct2/show/NCT01868412 (accessed November 12, 2021).
134. Clinicaltrials. Available online at: https://clinicaltrials.gov/ct2/show/NCT04831112 (accessed November 12, 2021).
135. Clinicaltrials. Available online at: https://clinicaltrials.gov/ct2/show/NCT05025306 (accessed November 12, 2021).
136. Clinicaltrials. Available online at: https://clinicaltrials.gov/ct2/show/NCT04942470 (accessed November 12, 2021).
137. Clinicaltrials. Available online at: https://clinicaltrials.gov/ct2/show/NCT04277182 (accessed November 13, 2021).
138. Clinicaltrials. Available online at: https://clinicaltrials.gov/ct2/show/NCT01748318 (accessed November 12, 2021).
139. Clinicaltrials. Available online at: https://clinicaltrials.gov/ct2/show/NCT01111695 (accessed November 12, 2021).
140. Clinicaltrials. Available online at: https://clinicaltrials.gov/ct2/show/NCT04545476 (accessed November 12, 2021).
141. Clinicaltrials. Available online at: https://clinicaltrials.gov/ct2/show/NCT0367415 (accessed November 12, 2021).
142. Haddioui-hbabi L, Treilhou M, Sauvain M. Anti-Helicobacter pylori properties of the ant-venom peptide bicarinalin. Toxins (Basel). (2018) 10:21–30. doi: 10.3390/toxins10010021
143. Ragab TIM, Shalaby ASG, Awdan SAE, El-Bassyouni GT, Salama BM, Helmy WA, et al. Role of levan extracted from bacterial honey isolates in curing peptic ulcer: in vivo. Int J Biol Macromol. (2020) 142:564–73. doi: 10.1016/j.ijbiomac.2019.09.131
144. Costa P, Almeida MO, Lemos M, Arruda C, Casoti R, Somensi LB, et al. Artepillin C, drupanin, aromadendrin-4′-O-methyl-ether and kaempferide from Brazilian green propolis promote gastroprotective action by diversified mode of action. J Ethnopharmacol. (2018) 226:82–9. doi: 10.1016/j.jep.2018.08.006
145. de Mendonça MAA, Ribeiro ARS, de Lima AK, Bezerra GB, Pinheiro MS, de Albuquerque-Júnior RLC, et al. Red propolis and its dyslipidemic regulator formononetin: evaluation of antioxidant activity and gastroprotective effects in rat model of gastric ulcer. Nutrients. (2020) 12:1–17. doi: 10.3390/nu12102951
146. Song MY, Lee DY, Kim EH. Anti-inflammatory and antioxidative effect of Korean propolis on Helicobacter pylori-induced gastric damage in vitro. J Microbiol. (2020) 58:878–85. doi: 10.1007/s12275-020-0277-z
147. Li Q, Hu X, Xuan Y, Ying J, Fei Y, Rong J, et al. Kaempferol protects ethanol-induced gastric ulcers in mice via pro-inflammatory cytokines and NO. Acta Biochim Biophys Sin (Shanghai). (2018) 50:246–53. doi: 10.1093/abbs/gmy002
148. Mohamed WA, Abd-Elhakim YM, Ismail SAA. Involvement of the anti-inflammatory, anti-apoptotic, and anti-secretory activity of bee venom in its therapeutic effects on acetylsalicylic acid-induced gastric ulceration in rats. Toxicology. (2019) 419:11–23. doi: 10.1016/j.tox.2019.03.003
149. Cifuentes L. Allergy to honeybee. Not only stings. Curr Opin Allergy Clin Immunol. (2015) 15:364–8. doi: 10.1097/ACI.0000000000000191
150. Kiistala R, Hannuksela M, Mäkinen-Kiljunen S, Niinimäki A, Haahtela T. Honey allergy is rare in patients sensitive to pollens. Allergy. (1995) 50:844–7. doi: 10.1111/j.1398-9995.1995.tb05061.x
151. Bauer L, Kohlich A, Hirschwehr R, Siemann U, Ebner H, Scheiner O, et al. Food allergy to honey: pollen or bee products? Characterization of allergenic proteins in honey by means of immunoblotting. J Allergy Clin Immunol. (1996) 97:65–73. doi: 10.1016/S0091-6749(96)70284-1
152. Leung R, Ho A, Chan J, Choy D, Lai CKW. Royal jelly consumption and hypersensitivity in the community. Clin Exp Allergy. (1997) 27:333–6. doi: 10.1111/j.1365-2222.1997.tb00712.x
153. Takahama H, Shimazu T. Food-induced anaphylaxis caused by ingestion of royal jelly. J Dermatol. (2006) 33:424–6. doi: 10.1111/j.1346-8138.2006.00100.x
154. Hata T, Furusawa-Horie T, Arai Y, Takahashi T, Seishima M, Ichihara K. Studies of royal jelly and associated cross-reactive allergens in atopic dermatitis patients. PLoS One. (2020) 15:e0233707. doi: 10.1371/journal.pone.0233707
155. Thien FCK, Leung R, Baldo BA, Weiner JA, Plomley R, Czarny D. Asthma and anaphylaxis induced by royal jelly. Clin Exp Allergy. (1996) 26:216–22. doi: 10.1111/j.1365-2222.1996.tb00082.x
156. Katayama M, Aoki M, Kawana S. Case of anaphylaxis caused by ingestion of royal jelly. J Dermatol. (2008) 35:222–4. doi: 10.1111/j.1346-8138.2008.00448.x
157. Maikanov B, Mustafina R, Auteleyeva L, Wiśniewski J, Anusz K, Grenda T, et al. Clostridium botulinum and Clostridium perfringens occurrence in Kazakh honey samples. Toxins (Basel). (2019) 11:472. doi: 10.3390/toxins11080472
158. Melling J, Hambleton P, Shone CC. Clostridium botulinum toxins: nature and preparation for clinical use. Eye. (1988) 2:16–23. doi: 10.1038/eye.1988.5
159. Gücükoglu A, Saka E, Uyanik T, Kanat S, Çadirci Ö, Akpinar R. Determination of C. botulinum presence in pollen samples collected from different regions of Turkey by Real-Time PCR. Lwt. (2020) 134:11072. doi: 10.1016/j.lwt.2020.110172
160. Schiener M, Graessel A, Ollert M, Schmidt-Weber CB, Blank S. Allergen-specific immunotherapy of Hymenoptera venom allergy–also a matter of diagnosis. Hum Vaccines Immunother. (2017) 13:2467–81. doi: 10.1080/21645515.2017.1334745
161. Lâm HT, Ekerljung L, Bjerg A, Văn Tuòng N, Lundbäck B, Rönmark E. Sensitization to airborne allergens among adults and its impact on allergic symptoms: a population survey in northern Vietnam. Clin Transl Allergy. (2014) 4:6. doi: 10.1186/2045-7022-4-6
162. Eberlein B, Krischan L, Darsow U, Ollert M, Ring J. Double positivity to bee and wasp venom: improved diagnostic procedure by recombinant allergen-based IgE testing and basophil activation test including data about cross-reactive carbohydrate determinants. J Allergy Clin Immunol. (2012) 130:155–61. doi: 10.1016/j.jaci.2012.02.008
163. Gattinger P, Lupinek C, Kalogiros L, Silar M, Zidarn M, Korosec P, et al. The culprit insect but not severity of allergic reactions to bee and wasp venom can be determined by molecular diagnosis. PLoS ONE. (2018) 13:e0199250. doi: 10.1371/journal.pone.0199250
164. Eržen R, Korošec P, Šilar M, Mušič E, Košnik M. Carbohydrate epitopes as a cause of cross-reactivity in patients allergic to Hymenoptera venom. Wien Klin Wochenschr. (2009) 121:349–52. doi: 10.1007/s00508-009-1171-1
165. Jappe U, Hoffmann M, Burow G, Enk A. In vitro hymenoptera venom allergy diagnosis : improved by screening for cross-reactive carbohydrate determinants and reciprocal inhibition. Alleragy. (2006) 61:1220–9. doi: 10.1111/j.1398-9995.2006.01232.x
166. Müller U, Hari Y, Berchtold E. Premedication with antihistamines may enhance efficacy of specific-allergen immunotherapy. J Allergy Clin Immunol. (2001) 107:81–6. doi: 10.1067/mai.2001.111852
Keywords: bee products, inflammation, diabetes, hypertension, cancer, preclinical and clinical studies, safety
Citation: El-Seedi HR, Eid N, Abd El-Wahed AA, Rateb ME, Afifi HS, Algethami AF, Zhao C, Al Naggar Y, Alsharif SM, Tahir HE, Xu B, Wang K and Khalifa SAM (2022) Honey Bee Products: Preclinical and Clinical Studies of Their Anti-inflammatory and Immunomodulatory Properties. Front. Nutr. 8:761267. doi: 10.3389/fnut.2021.761267
Received: 08 September 2021; Accepted: 23 November 2021;
Published: 03 January 2022.
Edited by:
Julio Villena, CONICET Centro de Referencia para Lactobacilos (CERELA), ArgentinaReviewed by:
Stefania Del Fabbro, University of Southampton, United KingdomSandra Quilodrán-Vega, University of Concepción, Chile
Copyright © 2022 El-Seedi, Eid, Abd El-Wahed, Rateb, Afifi, Algethami, Zhao, Al Naggar, Alsharif, Tahir, Xu, Wang and Khalifa. This is an open-access article distributed under the terms of the Creative Commons Attribution License (CC BY). The use, distribution or reproduction in other forums is permitted, provided the original author(s) and the copyright owner(s) are credited and that the original publication in this journal is cited, in accordance with accepted academic practice. No use, distribution or reproduction is permitted which does not comply with these terms.
*Correspondence: Hesham R. El-Seedi, aGVzaGFtLmVsLXNlZWRpJiN4MDAwNDA7ZmFybWJpby51dS5zZQ==; Kai Wang, d2FuZ2thaSYjeDAwMDQwO2NhYXMuY24=