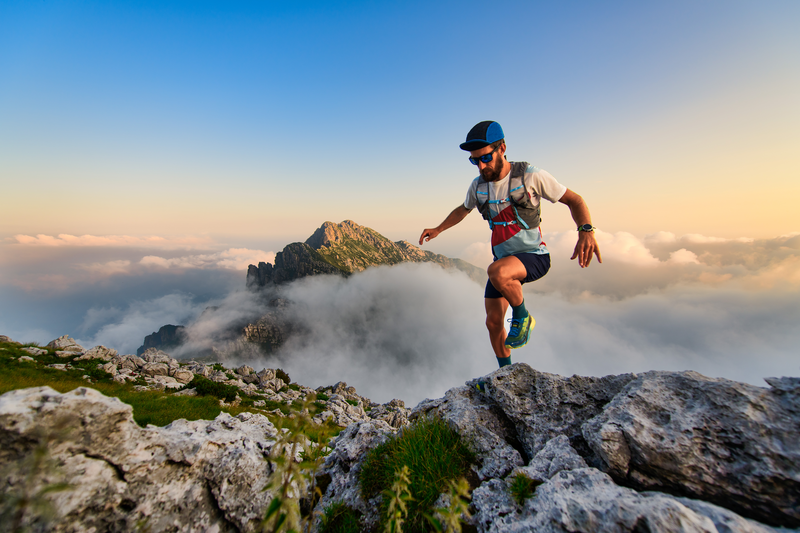
94% of researchers rate our articles as excellent or good
Learn more about the work of our research integrity team to safeguard the quality of each article we publish.
Find out more
REVIEW article
Front. Nutr. , 27 October 2021
Sec. Nutrition and Food Science Technology
Volume 8 - 2021 | https://doi.org/10.3389/fnut.2021.751686
This article is part of the Research Topic Risk-Benefit Assessment of Foods: Advances in Public Health View all 8 articles
Livestock production may improve nutritional outcomes of pregnant women and children by increasing household income, availability of nutrient-dense foods, and women's empowerment. Nevertheless, the relationship is complex, and the nutritional status of children may be impaired by presence of or proximity to livestock and their pathogens. In this paper, we review the benefits and risks of livestock production on child nutrition. Evidence supports the nutritional benefits of livestock farming through income, production, and women's empowerment. Increasing animal source food consumption requires a combination of efforts, including improved animal management so that herd size is adequate to meet household income needs and consumption and addressing sociocultural and gendered norms. Evidence supports the inclusion of behavior change communication strategies into livestock production interventions to facilitate the sustainability of nutritional benefits over time, particularly interventions that engage women and foster dimensions of women's empowerment. In evaluating the risks of livestock production, evidence indicates that a broad range of enteric pathogens may chronically infect the intestines of children and, in combination with dietary deficits, may cause environmental enteric dysfunction (EED), a chronic inflammation of the gut. Some of the most important pathogens associated with EED are zoonotic in nature with livestock as their main reservoir. Very few studies have aimed to understand which livestock species contribute most to colonization with these pathogens, or how to reduce transmission. Control at the point of exposure has been investigated in a few studies, but much less effort has been spent on improving animal husbandry practices, which may have additional benefits. There is an urgent need for dedicated and long-term research to understand which livestock species contribute most to exposure of young children to zoonotic enteric pathogens, to test the potential of a wide range of intervention methods, to assess their effectiveness in randomized trials, and to assure their broad adaptation and sustainability. This review highlights the benefits and risks of livestock production on child nutrition. In addition to identifying research gaps, findings support inclusion of poor gut health as an immediate determinant of child undernutrition, expanding the established UNICEF framework which includes only inadequate diet and disease.
Globally, undernutrition underlies nearly half of mortality of children under five (CU5) (1). Stunting and wasting, indicators of chronic and recent undernutrition, affected more than 149 and 45 million CU5, respectively, in 2020 (2). Stunted (defined as length/height (L/HAZ)-for-age Z scores < −2) children are more likely to encounter recurrent illness, experience lower vaccine effectiveness, and have poorer intellectual and emotional development, leading to lower financial attainment in the later stages of life and higher morbidity and mortality (3–5). Wasting (defined as weight-for-length/height (WL/H) Z scores < −2) is more acute and directly increases the risk of mortality in CU5 (2). Though debate remains about the reversibility of undernutrition, evidence indicates that children who are undernourished are more likely to become obese or overweight later in life (6–8), thus creating a dual lifetime burden from malnutrition.
Most current understanding of child nutrition is based on the well-established United Nations Children's Fund (UNICEF) framework, which identifies the determinants of undernutrition in children, consisting of immediate causes (inadequate dietary intake and diseases), underlying causes (household food security, inadequate care and feeding practices, and unhealthy household environment and inadequate health services), and basic causes (social, cultural, economic, livelihood, etc.) (9). In low- and middle-income countries (LMIC), agriculture has been linked to improved child nutrition outcomes through three primary pathways: food production, income, and women's empowerment (10). These pathways can be explored within the UNICEF framework to understand how small-scale (smallholder) livestock production, which promotes the potential to produce nutrient dense, high value animal source foods (ASF) and enhance socio-economic well-being (11, 12), can affect child nutritional outcomes. While livestock production is frequently mentioned in nutrition-sensitive agriculture as a mechanism to promote ASF consumption, literature examining the relationship between livestock production and ASF consumption is limited, complex, and the results are inconsistent (13). Most directly, livestock production may produce ASF that is consumed by the household, including children. The production pathway's influence on child nutrition varies widely, including by food item, market engagement, and other contextual factors (14). Socioeconomic status, cultural practices that limit or restrict ASF consumption, the capacity and/or opportunity for storage and preservation of ASF, community and household-level gender dynamics, and intra-household resource allocation are all factors that can affect individual ASF consumption (15). Many households engage in selling livestock or livestock products for income, which complicates an understanding of how livestock production may affect diets. Livestock production and productivity may require a certain threshold before households consume ASF produced on-farm—if at all. However, households that sell ASF produced on-farm may still benefit from improved diet composition and overall well-being given the increased purchasing power of the household (16–19). In addition to production and income, women's empowerment serves as a pathway from agriculture to improved child nutrition. Compared to other land or financial assets, women can access and control livestock as an agricultural asset (20). Women's ownership or co-ownership of livestock has been associated with improved nutritional outcomes compared to male ownership (21). Livestock production can help women build and secure their ownership over assets, providing a source of regular income that can contribute to a pathway out of poverty (22). Women who earn more, have greater control over income and other financial resources, and have more decision-making power are more likely to ensure better household health and nutrition (23–26).
Nevertheless, connections between livestock ownership and child nutrition are complex. Although small-scale livestock production presents an opportunity for increased ASF production/consumption to halt child undernutrition in LMIC (27, 28), a 2018 systematic review revealed no congruent relationship between ASF consumption and the alleviation of common undernutrition outcomes, including stunting (29).
Furthermore, although many observational studies significantly associate improved family-level water, sanitation, and hygiene (WaSH) with improved child linear growth and lessened burden of environmental enteric dysfunction (EED), a key mediator of growth faltering (30–32), these significant effects did not persist in landmark randomized controlled trials (WASH-Benefits Bangladesh & Kenya, SHINE) (33–36). Researchers suggested that unmeasured household risk factors could have confounded the beneficial effect of WaSH interventions, and called for a “transformative WaSH” approach to curtail fecal contamination at the household level in future research (33). Notwithstanding, a recent study applying this transformative approach found its intervention, without addressing environmental contaminations (substandard food hygiene, animal feces, soil), was associated with easing of intestinal epithelial damage but had no effect on gut inflammation, and the protective effect did not strengthen linear growth (37). Negative outcomes from these trials applying traditional and improved WaSH measures to improve child nutrition underscore the need to incorporate a more holistic view of WaSH, such that the role of livestock management and exposure to animal feces are considered in future rural intervention programs (38).
Why must livestock production be considered a risk? Livestock serve as reservoirs of many zoonotic pathogens, which affect both human and animal health globally (28). These zoonotic diseases not only have the potential to reduce production of labor and commodities by the farm animals, but they can also threaten food security, food safety, and the health and livelihoods of the farming households (39). More than 60% of emerging pathogens and over half of the identified human pathogen species are zoonotic; this pathogen group has been attributed to over $20 billion direct loss and more than $200 billion indirect loss globally (40–43). Though emerging zoonotic pathogens with pandemic capacity have received extensive research attention, considerably less attention has been given to endemic livestock-related zoonotic enteric pathogens sourced from their feces and typically transmitted through the fecal-oral pathways characterized by the well-known “F-diagram” (41, 44). A 2019 study estimated LMIC have a much higher burden of enteric pathogens of foodborne disease from ASF (45), which are commonly produced in low-resource non-intensive smallholding farming systems, typically with fewer cleaning, disinfection, and biosecurity practices (11, 28). Thus, smallholder households in LMIC may be more susceptible to the adverse effects of a high burden of zoonotic enteric pathogens driven directly or indirectly by exposure to animal excreta (46). Enteric pathogen infection may not only cause acute diseases such as diarrhea, but, more importantly, may cause subclinical enteric infection (47). These asymptomatic infections have been hypothesized to result in EED, a subclinical gastrointestinal disorder occurring in low-resource settings in LMIC (48). With the slow advancement of curtailing child undernutrition globally and the awareness that this crisis cannot be solely ascribed to poor dietary intake and diarrheal illnesses, EED has been suggested to be the primary mediator between exposure to enteric pathogens in environments and undernutrition (49–53).
In Figure 1, the agriculture to nutrition pathways previously described are mapped onto the UNICEF framework to demonstrate how smallholder livestock production (SLP) among rural households can affect child nutrition through benefits of established pathways. In addition, the risks of enteric pathogens' negative impact on child nutrition through inadequate feeding, care practices, and contamination of the household environment have been added, along with their potential, without control measures in place, to undermine child growth through impaired gut health. Therein emerges our hypothesis, that the nutritional benefits of ASF in smallholder families may be negated by children's exposure to zoonotic enteric pathogens from animal feces, in the absence of adequate sanitation and biosecurity protocols. Although discipline-based systematic reviews have been independently conducted on the relationships between ASF consumption and child growth indicators (29) and between exposure to animal feces and certain human health outcomes (46), no risk-benefit analysis has sought to jointly characterize the benefits and risks of ASF farming on child nutrition in LMIC. Here, using an integrated and multidisciplinary approach, we conduct a systematized review to address this knowledge gap. Specific research questions (see Table 1) address the associations depicted with dashed arrows in Figure 1. Established associations depicted by solid arrows in Figure 1 will be included in the synthesis based on existing reviews.
Figure 1. Conceptual framework of benefits and risks of smallholder livestock production on child nutrition in low- and middle-income countries.
Table 1. Research questions on benefits and risks of smallholder livestock production on child nutrition in low- and middle- income countries (LMIC).
As such, our work aims to synthesize the existing literature and evidence base on the role of livestock production on the WaSH landscape in relation to nutritional outcomes and pinpoint relevant research gaps and priorities through a systematized review.
Between March and June 2021 we performed systematized searches of the literature for the research questions shown in Table 1. We searched PubMed, Agricola, CABI, and Ebsco's Academic Search Premier, EconLit, CINAHL, and Women's Studies International databases for the benefit aspects and Web of Science Core Collection, PubMed, Embase, and CABI for the risk aspects using MeSH terms (PubMed) and truncated and phrase-searched keywords in the title or abstract. See Appendix for details on search strategies by research question, eligibility criteria, and the number of articles identified and screened. We included English-language full text materials published between January 2000 and June 2021. Duplicate results were removed, and we then screened title-abstract and then full-text with support of the Covidence software (54). We went on to include relevant articles from personal libraries, as well as references noted in full-text screening of articles generated. We extracted data from full text of included studies in spreadsheets then synthesized and summarized results. Given research team composition and expertise, cross fertilization of results and findings occurred at each stage of data extraction, synthesis and summary. Discussion section and final conclusions were developed collaboratively to ensure integration of findings.
This section reviews the benefits of SLP. It is structured around production, income, and women's empowerment (Supplementary Table 1). Women's empowerment is broken down into the major domains of women's empowerment identified in the literature.
Of the 145 records included, 24 reported results from interventions that investigated the impact of a livestock intervention on ASF consumption and/or dietary intake of children and/or households. Of the nine studies that measured child ASF consumption, a significant increase in child ASF consumption from baseline to endline was reported in one study (54); significant increases in child ASF consumption among intervention compared to control households were reported in five studies (55–59); two studies found no significant differences (60, 61); and one study did not report significance (62). Of the 4 studies that reported child dietary intake data, significant increases in child dietary diversity among intervention compared to control households were reported in two studies (57, 61), a marginally statistically significant greater increase in children's minimum dietary diversity among intervention compared to control households was reported in one study (63), and no significant difference in child intake of foods groups among intervention compared to control households was reported in one study (60).
Of the livestock intervention studies, 19 reported dietary data at the household level, 12 of which reported ASF consumption data at the household level. Of these 12 studies, significant increases in household ASF consumption from baseline to endline were reported in two studies (64, 65); significant increases in household ASF consumption among intervention compared to control households were reported in four studies (58, 59, 66, 67); no significant increases in household ASF consumption among intervention compared to control households were reported in three studies (68–70); and significance was not reported in three studies (61, 71, 72). Of the 19 studies that measured household dietary diversity, significant differences were reported in three studies (58, 61, 73); a marginally statistically significant greater increase among intervention compared to control households was reported in one study (63); and no significant differences were reported in three studies (67, 74, 75). Furthermore, of these 19 studies, one study reported a significantly lower percentage of food insecure households among intervention compared to control households (76); one study reported a significant improvement in food security among intervention compared to control households (77); one study reported a significant increase in household consumption of green, leafy vegetables and yellow/orange fruit among intervention compared to control households (61); and one study found no significant differences in household energy intake among intervention compared to control households (54).
Educational or behavior change communication (BCC) components that promote optimal care and feeding practices, health seeking behaviors, and/or knowledge were common in livestock production interventions, with 13 interventions including a BCC component. Of these 13 studies, five reported child ASF consumption data. Four of these five studies reported significantly greater increase in child ASF consumption among intervention households with the educational or BCC component compared to control households (55–58), while one study reported no significant difference in child ASF consumption among intervention compared to control households (61). Of these 13 studies, five reported household ASF consumption data. Two of five studies reported significant increases in household ASF consumption in intervention households from baseline to endline (64, 65); two studies reported significant increases in household ASF consumption among intervention households with the educational or BCC component compared to control households (58, 67); and one study did not report significance for the differences in household ASF consumption observed (72).
Of the 13 studies with BCC interventions, six reported household dietary diversity data. Of these six studies, three studies reported significant increases in household dietary diversity among intervention households with the educational or BCC component compared to control households (58, 61, 67); one study reported marginally statistically significant increase in household dietary diversity scores among intervention compared to control households (63); and two studies reported no significant differences (74, 75). Furthermore, results from one intervention that included a technical assistance component reported stronger program effects on income from livestock and higher consumption from own production for participants who received technical assistance compared to those who did not (77). The authors also reported an increase in program impacts with treatment exposure and length.
Of the 145 records included, 70 were observational studies investigating livestock production and dietary intake. Of these, five studies reported significant results for livestock production and increased child ASF intake (21, 78–81), five studies reported significant results for livestock production and increased household ASF intake (82–86), and two studies found no significance between livestock production and child ASF intake (87, 88). Regarding dietary intake, three studies reported significant results between livestock production and improved household dietary intake, including household food availability and household food calories (89), food consumption score (90), and household food consumption (91); remaining studies did not measure the association between livestock production and dietary intake. Among the observational studies, 40 described smallholder farmers' objectives for producing livestock. Of these, 23 reported ASF consumption from livestock production as a primary reason for livestock production, eight studies reported ASF consumption from production as not one of the primary reasons, and two studies did not assess ranking of objectives.
Of the 145 records included, 65 records investigated income and livestock production. Of these, 13 studies reported results from interventions that examined the effect of livestock production interventions on household income, nine of which reported that the interventions significantly increased household income from livestock sales (57–61, 64, 69, 73, 77), two of which reported no significant effects of the intervention on household income (67, 70), and two of which did not report significance values (62, 68). Furthermore, four of the 13 studies reported that increased income from livestock sales through program participation led to improved household nutritional outcomes through enhanced purchasing power for food, which can include ASF (58, 60, 73, 77).
Of the 65 records investigating income and livestock production, 52 were observational studies, four of which measured the association between livestock production and income. All four found positive results: one study reported a significant association between cow ownership and increased dairy income (86), one study reported significantly higher household incomes among households with livestock (92), one study reported that camel milk was a significant contributor to household income (93), and one study reported that livestock ownership was a determinant of household income (94). Three studies measured associations between income from livestock and nutritional outcomes. Of these, one study reported that income from livestock sales was significantly higher among households with adequate food consumption compared to households with poor and intermediate food consumption (91), one study reported that livestock income significantly increased food expenditure (95), and one study reported no effect of livestock income on household dietary diversity (96).
Of the 145 records included, 42 studies investigated livestock production and women's empowerment. Of these studies, eight studies were intervention studies and 33 were observational studies. The women's empowerment in livestock index (WELI), developed in 2015, is a quantitative assessment of the empowerment of women in agriculture, specifically focused on livestock production. It was developed in response to the women's empowerment in agriculture index (WEAI), which mainly focuses on the empowerment of women in agriculture in general, with less attention given specifically to livestock production (97). Given the gendered challenges and opportunities involved in livestock production, the WELI was developed to better address the measurement of women's empowerment in livestock production (20). The WELI identifies six dimensions of empowerment in livestock production: decisions about production, access to and control over resources, control and use of income, access to and control of opportunities, workload and control over time, and decisions related to nutrition (20). Though many studies investigated more than one dimension of the WELI, and most did not name or use the WELI, the results from observational studies are organized based on the WELI dimensions of empowerment. The search terms for this review reflect our overarching goal to understand the benefits of livestock production on child nutrition. As such, this section is not a complete literature review of the WELI domains, but rather a way of categorizing results of the search for this review.
Nine of the 33 observational studies investigated women's empowerment in the context of decisions about production. Four studies discussed women's decision-making and role in small-ruminant production and reported that women tend to be more responsible for small ruminant activities, mainly poultry production (98–103), while men tend to have more responsibility over large ruminant production, such as cattle or camel (99, 101, 104). One study pointed to the head of household's importance in decision-making ability over livestock production, discussing how women in women-headed households were more likely to retain control over livestock production compared to women in men-headed households (105). Though women engaged in SLP can contribute significantly economically to the household, their decision-making is often constrained, depending on the type of livestock, intensification of the production system, sociocultural context, and the type of decision being made (16, 22, 99, 102). For example, women may be able to make decisions about livestock inputs or care strategies but not about the sale or slaughter of livestock and/or the use of livestock assets (99).
Twenty-two observational studies discussed women's empowerment in livestock in the context of having access to and control over resources. Larger livestock and income from larger livestock tend to be more under men's control (106), while smaller-scale livestock activities tend to be more under the control of women (101, 104). Poultry production, in particular, was the most widely cited production system under the control of women (99, 100, 103, 107–109). Increasing women's control over production and resources can facilitate better access to ASF for women and children (110).
Small animals, including poultry, are often the first animals sold to meet household needs, such as education or medical expenses (99, 111–113). However, one study reported that the decisions about the end use of high-value livestock (such as cattle) may involve a more joint-based decision-making approach due to such livestock's high economic and social value (99), but this remains to be further explored. While livestock production—particularly of small animals—is more readily accessible and controlled by women compared to other land or financial assets (20), the type of livestock owned and/or controlled by women can be culturally-and location-specific (22). The type of “rights” women may have over livestock production are heterogenous and can be divided into categories and sub-categories of rights such as resource access, right to withdraw or use products, and decision-making rights (22). “Ownership” of livestock does not necessarily entitle women to make decisions about it or about income generated from the livestock (99).
Eleven observational studies discussed women's empowerment in the context of control and use of income. Some evidence suggests that women may have more control over income from small ruminant, poultry, and dairy products (99, 101, 114, 115). Some evidence suggests that as a livestock production activity becomes more lucrative, the dynamics of control between men and women change, with more control shifting to men (107, 116, 117). Similarly, a transition toward commercialization, larger-scale production, or formalized markets may shift control and income toward men (104).
Only one observational study discussed the effect of group membership and women's empowerment in livestock production, noting that women in groups had more control over income from dairy products that those that were not in groups (118).
Eleven observational studies discussed livestock production and workload and control over time. The effect of livestock production on women's workload and control over time was mixed, with some evidence indicating a greater workload and labor demand among women engaged in livestock production (99, 102, 107, 119). Because women in smallholder farming households tend to be primarily responsible for domestic duties, such as caring for children and preparing food, livestock production activities are often performed in addition to their daily responsibilities (120). While some parts of production may be shared among household members, women are often more responsible for the day-to-day management of production (particularly for poultry production), including feeding, watering, and caring for livestock (103). However, three studies report differentiated impacts of livestock production on workload and time burden depending on the type of livestock (121), how intensive the production system is (120), and if the household is man vs. woman-headed (105). The implications of increasing livestock productivity on women's workload and well-being can be complex and unpredictable (99). Even when they invest time and labor into livestock production, women may not have control over the sale and/or resulting revenue (99, 122, 123).
Women's decision-making power related to the use of livestock derived ASF for household consumption varies. Even when women participate in livestock-related activities and generate ASF, they may not have control over the use of ASF from livestock production (123). Milk and poultry production are areas where women may be the main decision-makers and have more control over the use of ASF within the household (101, 114, 124, 125). Additionally, if a household generates a surplus of an ASF, then women may be given control over the surplus to use or sell (102).
The allocation of ASF within the household is not always equal, with individual access to ASF depending on myriad factors, including gender, age, and type of ASF (99). Dairy products, for example, may be more equally allocated within the household, with children often being a primary recipient, compared to meat (21, 99). Furthermore, co-owned or female-owned livestock has been found to be significantly associated with child ASF intake when compared to male ownership (21).
Having reviewed the literature from observational studies, the following section presents findings from intervention studies. The results of livestock production interventions on women's empowerment showed mixed effects, as some were significant (55, 75, 77, 126) and others non-significant (57, 59, 124, 127). Significant effects of livestock production interventions included increased women's decision-making about livestock products (55); improved overall empowerment score (75); increased women's empowerment as measured by the Women's Empowerment in Agriculture Index (WEAI) (77); and increased scores in social capital, asset access, financial empowerment, and agriculture empowerment domains (126). Regarding intervention effects on ASF consumption, significant increases in child ASF consumption were associated with interventions in three studies (55, 57, 59), while the remaining studies did not measure child ASF consumption. Significant increases in household milk consumption (59), child dietary diversity (57), and household food security (77) between intervention and control groups were reported. A non-significant difference in household dietary diversity between the intervention group and the control group was reported in one study (75). Incorporation of a BCC or technical assistance component as part of the livestock production interventions was common. A mix of significant increases (55, 75, 77, 126) and non-significant (57, 127) differences of livestock production interventions with BCC components on women's empowerment was reported.
Having mapped out the benefits of livestock production through the three primary pathways of production, income, and empowerment, it is important to acknowledge that in the absence of these protective elements, there is an increased risk of poor nutrition outcomes. Livestock production is a key resource for many smallholder farming households in LMIC, particularly for women. When these beneficial factors are not present, the pathway from livestock production to nutrition can counterfactually pose risks to child nutrition, in tandem with exposure to enteric pathogens.
In this section, we review evidence from studies on enteric pathogens associated with EED and/or undernutrition outcomes in CU5 and characterize their reservoirs and risk factors for infection. We identified 12 and 38 articles that found enteric pathogens statistically associated with EED and undernutrition, respectively. Despite being the gold standard of diagnosing EED (128), endoscopic biopsy is often not feasible in epidemiologic studies. Thus, in the reviewed studies, EED was characterized by biomarkers of different impairments to gut function as discussed in Tickell et al. (34), Rogawski and Guerrant (129).
Table 2 summarizes study results of pathogens significantly associated (P < 0.05) with increased levels of EED markers and/or undernutrition outcomes in CU5 and their reservoirs. Details regarding effect sizes of the associations between pathogen infections and the health endpoints are provided in Supplementary Tables 2, 3. Although this review is focused on zoonotic enteric pathogens, we include anthroponotic and sapronotic pathogens because of the limited level of speciation for some pathogens in the literature, and to offer a more comprehensive portrayal of enteric pathogens involved in the pathogenesis of EED and undernutrition (Table 2). Following WHO's definition, we classified the reservoir of infection as zoonotic (Z) if it is naturally transmissible from vertebrates to humans. We classified pathogens as anthroponotic (A) if the reservoir is exclusively human. Anthroponotic pathogens originating from a single zoonotic spillover event but lacking epidemiologic evidence on sustained zoonotic transmission (e.g., rotavirus, Cryptosporidium hominis subtype IfA12G2) were characterized as anthroponotic (180, 181). Pathogens mainly replicating in abiotic environments (water, soil, food) were categorized as sapronotic (S) (182). In the reviewed literature, the pathogens were characterized taxonomically at different levels of detail by their biological group, genus, species, and subtype. Lacking sufficient taxonomic data, we assigned pathogens to different reservoirs (e.g., A/Z) as appropriate.
Table 2. Enteric pathogens associated with increased risk of EED or undernutrition at the 95% confidence level.
Four studies associated enteroaggregative E. coli (EAEC) with the increased biomarkers of gut inflammation [lactoferrin, neopterin (NEO), myeloperoxidase (MPO)] and epithelial damage/repair [α-1-antitrypsin (AAT)] (131, 146–148), and two studies found increased levels of this pathogen to be associated with linear growth faltering (139, 147). Three studies found gut inflammation (measured by calprotectin (CAL) and MPO) was positively associated with enterotoxigenic E. coli (ETEC) infection (131, 137, 144). In contrast, a negative association was found in one study between ETEC and the inflammation marker NEO (131). Two studies demonstrated ETEC increased the risk of underweight and growth faltering (141, 145). Three studies reported a directionally heterogeneous association between Campylobacter spp. and markers of gut inflammation (131, 137, 138). All studies found a positive association with MPO. Again, a negative association was found in two studies with NEO (131, 138). As found by 2 and 1 studies respectively, Campylobacter spp. also elevated AAT, the marker of epithelial damage/repair and the lactulose and mannitol (L:M) ratio indicating increased intestinal permeability (131, 137, 138). Regarding undernutrition, 3 studies demonstrated Campylobacter infection increased the risk of reduced weight gain and growth faltering (138–140). Diagnostic methods in the MAL-ED study included an immunoassay targeting all (thermotolerant and non-thermotolerant) Campylobacter species (138) and a molecular assay targeting the (thermotolerant) species C. jejuni and C. coli (139). The effect size (measured as LAZ difference between children in the upper 10-percentile of Campylobacter burden vs. those in the lower 10-percentile) for Campylobacter spp. was approximately twice as high as for C. jejuni/coli (−0.33 vs. −0.17), suggesting one or more non-thermotolerant species are strongly associated with linear growth faltering. Shigella spp. were found to increase the markers of gut inflammation (MPO) and intestinal permeability (L:M) by one study and linear growth faltering and slow gain of length by two studies (131, 139, 154).
Kosek et al. found that enteroinvasive E. coli and Plesiomonas shigelloides were both associated with elevation of MPO, with the former also associated with increased L:M ratio (131). In contrast, decreased levels of biomarkers of epithelial damage or repair (AAT) were observed for enteropathogenic E. coli (EPEC) and the genera Salmonella and Aeromonas. Yersinia enterocolitica was associated with elevated MPO and decreased NEO, while both are markers of gut inflammation (131). Helicobacter pylori was found by one study in association with biomarkers of epithelial damage or repair (AAT, Reg1β) but with opposite directions of effects (150).
The enteric protozoa Cryptosporidium spp. and Giardia spp. were also associated with EED and undernutrition endpoints. At the genus level, Cryptosporidium was found in two studies to increase biomarkers of intestinal permeability (L:M) and epithelial damage/repair [intestinal fatty acid binding protein (I-FABP)] (131, 157), while 3 studies revealed the pathogen increased the risk of not only the common undernutrition endpoints of the (binarized) Z-scores of length and weight but slow velocity gains (measured by length and weight velocity Z-scores) of the two measures (145, 158, 159). Four studies associated Giardia spp. with markers of gut inflammation (MPO, NEO), epithelial damage/repair [AAT, regenerating protein 1β (Reg 1β)], intestinal permeability (L:M), and microbial translocation [anti-flic immunoglobulin A (Flic IgA)]. However, the effect(s) on the inflammation endpoint, when indicated by MPO were directionally reversed in two studies and were negative when solely measured by NEO in one study (131, 137, 148, 167). Other than the common undernutrition outcomes, at the species level, infection with G. lamblia also contributed to slow gain of length-for-age difference (-LAD) (159). Two studies associated Entamoeba histolytica with underweight and slow gain of weight-for-age Z score (WAZ) (145, 165).
Geohelminths consist of multiple anthroponotic parasites. When evaluated as a group, it was associated with linear growth faltering, stunting, and slow gain of LAD (159, 175), while hookworm was associated by one study with growth faltering (165). Trichuris spp., a genus of geohelminths, was found by one study to increase a composite EED score of MPO, NEO, and AAT (148). T. trichiura was positively associated with stunting (178).
Two studies associated adenovirus with elevated MPO and growth faltering (131, 132). Diarrheal illness by rotavirus and norovirus was found to increase the risk of growth faltering (132).
Several studies confirmed the strong associations between diarrheal viral and bacterial infections with reduced weight and height gain and growth faltering as suggested by the original UNICEF framework. More recently, studies have associated asymptomatic infections of Campylobacter spp. and EAEC and Giardia with similar undernutrition endpoints (139, 139, 140). Importantly, one study of Campylobacter infections in Peru found that asymptomatic infection had a larger effect size (−65.5 vs. −43.9 g) on weight gain vs. symptomatic infections (140).
More generally, extensive infection with enteric pathogens, including those that were not delineated in Table 2, might also be associated with EED or undernutrition (131). A 2021 study in Zambia associated cumulative burden of enteric pathogens (consisting of Shiga toxin producing E. coli (STEC) and others) in fecal samples with elevated concentrations of I-FABP, a marker of epithelial damage or repair (157). A 2019 study in an asymptomatic child cohort in Pakistan found, among the 40 enteric pathogens studied, presence of any enteric pathogen in a stool sample was positively correlated with flagellin IgA and Reg 1b, which are EED biomarkers of increased microbial translocation and epithelial damage/repair, respectively. Multiple infections of enteric pathogens, measured by pathogen counts, were negatively associated with growth faltering at 18 months of age (137). A 2020 study in Ethiopia suggested the group infected with at least one of seven detected intestinal parasites in stool specimens had significantly higher stunting prevalence than those that were not (183).
Furthermore, other than enteric pathogens, virtually any bacteria at high concentrations in the small bowel could cause EED (184). When normal composition of gut microbiota is disrupted, even commensal microorganisms, which are related to small intestinal bacterial overgrowth (SIBO) (185), are associated with EED and stunting. A Bangladesh study revealed fecal Reg1β and CAL were higher in SIBO-positive children (185). A study in sub-Saharan Africa associated overgrowth of bacteria belonging to the oropharyngeal taxa with stunting (186). A 2020 study suggested the elevating and reducing quantities of Proteobacteria and Prevotella spp. were associated with poor linear growth in Bangladesh, accordingly (187).
Given the observed epidemiological associations between (asymptomatic) infections with particular pathogens, including bacteria (e.g., E. coli pathotypes, Shigella spp., Campylobacter spp.) and intestinal protozoa (e.g., Giardia spp. and Cryptosporidium spp.), we investigated evidence from animal studies supportive of causal associations and found they have been reviewed previously (188, 189). One review that assessed mouse models of EED suggested that a combination of nutrient (protein, zinc) deficient diets and (a)symptomatic gut infections by enteric pathogens including Cryptosporidium parvum and Campylobacter jejuni could induce EED or EED-like conditions and/or growth restriction (188). Both reviews underlined that EED and growth restriction are complex and heterogeneous processes and advocated further advancements of animal studies through investigating the gut health and nutrition outcomes jointly (188, 189). A mouse model found that although undernutrition triggered by deficiencies of micronutrients and/or macronutrients could impede growth, the nutritional deficiencies alone were not sufficient to induce enteropathy. The combination of infections and nutrient deficient diets impaired the gut barrier and caused uncontrolled inflammatory response triggering growth failure (190). Peculiarly, a mouse model suggested respiratory infections by influenza viruses led to intestinal damage through a complicated immunological mechanism, indicating this respiratory pathogen might be involved in the etiology of EED (191, 192). Nonetheless, how these results can be extrapolated to humans remains uncertain (193).
Undernutrition is an established risk factor for diarrheal and other infectious diseases (194). We found 7 articles identifying undernutrition [i.e., growth faltering, (severe) stunting, wasting, underweight] as significant risk factors of enteric pathogen infections, as measured by a positive stool sample that could be either symptomatic (i.e., diarrheal) or asymptomatic (195–201). While wasting was a risk factor of more aggravated diarrheal symptoms related to rotavirus infections (195), stunting and severe stunting were significant risk factors of diarrheal/non-diarrheal Cryptosporidium and Aeromonas infections, respectively (196–199). A 2020 study suggested wasted infants had higher odds of having a stool sample positive for Campylobacter spp. (200).
We identified 27 eligible studies investigating risk factors associated with direct/indirect exposure to zoonotic enteric pathogens associated with EED and undernutrition in CU5 in smallholder settings (Table 2).
The level of pathogen specification was limited in many eligible studies, as in the previous section. Four studies defined the pathogens at the genus level (e.g., Salmonella, Cryptosporidium spp.) (202–205), and 8 studies characterized pathogens with general biological groups only [i.e., enteric protozoa infection (206, 207), intestinal parasite infection (208–212), enteric pathogen infection (213)]. We therefore defined pathogens as zoonotic (Z) and as zoonotic and/or anthroponotic (A/Z) as in section Enteric pathogens as determinants of EED or undernutrition in children under five.
Household risk factors directly/indirectly contributing to children's exposure to and/or infection of zoonotic enteric pathogens are summarized by different transmission pathways (i.e., animal contact, foodborne, waterborne, person-to-person, and environmental) used by the US Centers for Disease Control and Prevention (CDC) for source attribution (214). Socio-demographic factors were designated according to CDC's social-ecological model (215). We classified factors related to WaSH into categories using the ladders of WHO/UNICEF's joint monitoring program (216–218).
Risk factors classified as animal contact pathway are summarized in Table 3. One study found allowing random access to the farm was associated with increased level of Salmonella contamination on pen floors of livestock farm (202). Three studies positively correlated households' adjacency to livestock farms with fecal contamination (measured by E. coli) inside households and Salmonella on farm, as well as Giardia lamblia infection in CU5 (170, 202, 219). Four studies suggested owning livestock to be a risk factor for infection of CU5 or adults with enteric protozoa or Campylobacter spp. (200, 203, 206, 207). For families owning livestock, increasing livestock density in/around the households has been associated with visible indoor contamination of animal feces and infection by intestinal parasites (including Giardia lamblia) in household members (210, 220). A 2020 study associated presence of livestock feces (positive for Campylobacter) in/around households with infection of this pathogen in infants (200). Five studies found home slaughter or other direct contact with livestock were risk factors of zoonotic bacterial and parasitic pathogen infections in humans, in some cases accompanied by diarrhea (204, 211, 221–223). One of these five studies found that while animal contact was associated with Cryptosporidium infection at the genus level, further genotyping found C. parvum as the dominant species infecting livestock, whereas humans were mainly infected by C. hominis (222). Six studies suggested cohabitation with livestock was a risk factor of household contamination with livestock feces, intestinal parasite infection (containing Giardia lamblia), both symptomatic and asymptomatic infection with Cryptosporidium spp., Campylobacter spp., and other zoonotic enteric pathogens (e.g., STEC, G. lamblia, Yersinia spp.) in CU5 (200, 205, 209, 212, 213, 220). Other than exposure to livestock and their feces, a 2015 study associated frequent rodent sightings with the presence of non-typhoidal Salmonella in livestock, and Cryptosporidium infection in humans was positively correlated with presence of pet feces and scavengers in/around the households (221, 224, 225).
Table 3. Risk factors associated with the presence of zoonotic enteric pathogens in different endpoints (livestock, environment, human at all age range, and children under-five) through the animal contact pathway in smallholders in low- and middle- income countries (LMIC).
Seven studies reported risk factors through the waterborne and environmental pathways in smallholder settings (Table 4). One study found that using untreated water for cleaning was associated with higher level of fecal indicator E.coli in the household environment (219), and another study associated having poor access to safe drinking water with infection by G. lamblia and other intestinal parasites in humans (208). Two studies revealed that household crowding was associated with an elevated burden of infection with intestinal parasites, including G. lamblia (208, 212). Two studies found that improper disposal of garbage was significantly associated with increased risks of Campylobacter diarrhea in CU5 and Cryptosporidium infection in humans (205, 221). One study in Ethiopian households raising chickens associated floor samples contaminated by Campylobacter spp. with infection by these bacteria in infants (200).
Table 4. Risk factors associated with the presence of zoonotic enteric pathogens in different endpoints (environment, human at all age range, and children under-five) through the waterborne and environmental pathways in smallholders in LMIC.
Five studies reported other risk factors associated with infections of Cryptosporidium, Campylobacter and intestinal parasites (including G. lamblia) in CU5 and other household members (Table 5). One study in Tanzania identified residing with infected people as a risk factor of Cryptosporidium spp. infection (181). While chimpanzees and livestock were present in the study area, concurrent infections with C. hominis subtype IfA12G2 were found in chimpanzees and humans, while no common Cryptosporidium spp. infecting livestock and humans were identified, suggesting the person-to-person transmission might potentially be initiated by the zoonotic spillover from the chimpanzees (181). A study in Ethiopia suggested an increased Campylobacter burden in CU5 was associated with current breastfeeding and the intake of ASF (including raw milk) (32). Similarly, a study in Cambodia found raw meat consumption to be a risk factor of C. jejuni infection in humans (223).
Table 5. Risk factors associated with the presences of zoonotic enteric pathogens in different endpoints (human at all age range, and children under-five) fallen under the social-demographic category or through the foodborne and person-to-person pathways in smallholders in LMIC.
Among the community-level socio-demographic risk factors, residing in villages was associated with Cryptosporidium spp. infection vs. living in city and camps in two studies (181, 222). Lower social-economic status at the household level was described by one study as a risk factor of intestinal parasites (including G. lamblia) infection, and this endpoint was correlated with a lower level of adult education (211). At the individual level, one study found younger age (<16 year old) increased the risk of Campylobacter infection in humans (223) (Table 5).
In LMIC's smallholder settings, risks of ASF production to child health are driven by exposure to zoonotic enteric pathogens from livestock and other animal reservoirs through different transmission pathways. Source attribution of zoonotic diseases can be conducted at the animal reservoir and vehicle (i.e., point of human exposure) levels (226). Established approaches for source attribution include microbiological approaches (e.g., microbial subtyping and comparative exposure assessment) and epidemiologic approaches as reviewed in section Risks factors of exposure to or infection with zoonotic enteric pathogens associated with smallholder livestock production. Here, we reviewed the potential and implementation of microbiological approaches to provide such quantification in LMIC. Applications of these approaches in high-income settings have been reviewed previously (226, 227).
Microbial subtyping methods are based on phenotypic or genotypic subtyping of isolates of pathogenic organisms from humans and different putative sources. Probabilistic models are used to attribute infection in humans to different (animal) sources. Non-living sources such as foods, waters and fomites can also be included, e.g., the application of multi-locus sequence typing (MLST) to attribute Campylobacter jejuni infecting human to livestock and environmental surface water sources in Europe (228).
Microbial Source Tracking (MST), as applied in WaSH studies, can also be classified as a microbiological approach. MST tracks the source of fecal contamination of water or other non-living transmission vehicles such as food or fomites to specific animal hosts by molecular detection of genetic markers particular to a host species occurring in commensal Bacteroides spp. (229). This method cannot be used to attribute infections in humans.
Comparative exposure assessment aims to characterize the relative significance of pre-determined vehicles of transmission through inferring the risk of human exposure to a pathogen of interest per vehicle. The assessment is conducted by evaluating levels of contamination in sources of interest and other transmission pathways, and exposure frequencies under each route, using statistical models.
We identified 15 studies using microbiological approaches implemented to quantify the relative contribution of different sources of human exposure to zoonotic enteric pathogens in LMIC −5 implemented the microbial subtyping approach which were found based on citation tracking from a previous review (230), 8 applied MST host-specific markers, and 2 utilized comparative exposure assessment.
All five studies utilizing the microbial subtyping approach aimed to attribute sources of non-typhoidal Salmonella enterica (NTS) infections in human populations (children or adults) in Africa. Subtyping methods included MLST, phage typing, pulsed-field gel electrophoresis (PFGE), AMR profiles, and plasmid typing (Table 6). Three of the five studies found that NTS recovered from children and adults in some African settings were originated from human reservoirs despite NTS is commonly considered to be zoonotic and close contacts between humans and animals were observed (231–233). In contrast, two other studies found PFGE patterns of NTS isolates from wildlife and poultry were matched to or clustered with those isolated from children and adults, suggesting zoonotic transmission (234, 235). On study further supported transmission from poultry by phage typing (235).
Among the eight studies implementing the MST approach (Table 7), two quantified the level of fecal contamination by animal or human hosts species in environmental samples. A study in Bangladesh found human fecal contamination affected nearly 80% of ponds in the study community (236). Another Bangladeshi study revealed a ruminant fecal marker was present in more than 30% of sampled produce from markets (238). Six studies statistically compared the presence of a host-specific marker between vehicles or combined MST with epidemiologic data. Three of these found levels of human specific Bacteroides spp. were significantly higher in sanitary wastewater, home environment, and wet soil (closed to a public latrine) than in stored drinking water, ponds and tube wells, and dry soil (237, 241, 242). A study in India found human and animal fecal markers were more often detected on mothers' and children's hands than in household stored drinking water (240). Another study in Bangladesh positively associated the animal marker on mothers' hands with levels of common enteric pathogen genes (i.e., Giardia lamblia, pathogenic E. coli) on these hands (239). Utilizing markers of three reservoir types (i.e., human, avian, dog), a study in Peru found household floors were more contaminated by the feces from all three species than tables, and wooden tables were more contaminated by the feces from the non-human species (243). More importantly, an avian marker was associated with the presence of Campylobacter on environmental surfaces (243). Presence of Campylobacter in household surfaces was associated with fecal contamination from poultry, indicated by an avian host-specific marker (243). Increased levels of human and animal markers in household were associated with the occurrence of diarrhea in children (241).
Table 7. Applications of host-specific microbial source tracking (MST) markers of fecal contamination in low-resource settings.
Two eligible studies were belonged to the SaniPath analytical approach, based on collecting data from structured observations on child behavior, the concentration of generic E. coli in environmental samples and fitting the data into quantitative microbial risk assessment models to infer the relative risks of predetermined fecal exposure pathways (244, 245). Using parameters inferred from behavioral observation data, one study simulated daily behavioral sequences related to environmental fecal exposure of CU5 in an urban setting in Accra, Ghana. The simulation, based on the frequency of contact, indicated that younger children (<1 year) spent time predominantly off the ground, while the older children often entertained on the floor, suggesting that the floor could act as a key environmental source of exposure for the older CU5 group (246). The simulation also indicated that activities reducing fecal exposure, such as washing hands before eating, occurred infrequently (246). An exposure model combined the behavioral sequence model with a hierarchical model simulating E. coli concentrations in household environmental samples (245). The model suggested that food most substantially contributed (more than 99%) to fecal exposure, while hands likely acted as the key mediator between the mouthing of E. coli and environmental sources in the environments (245).
The classic “F-diagram” depicts multiple pathways through which fecal microbes can be transmitted from human feces through contaminated water, soil, arthropod vectors, food and direct contact with contaminated environment to ingestion by healthy people (44). In this review, we discuss intervention methods for reducing the exposure to animal feces based on the adapted F-diagram referring to Penakalapati et al. (46) which extended the classic F-diagram by including animal feces into the diagram. To select appropriate intervention methods, it is important to quantify the relative weights of different (animal) reservoirs and pathways in terms of contribution to the transmission of pathogens. As discussed in section Attribution of zoonotic enteric pathogen infections in LMIC, very few studies have aimed to attribute infections with zoonotic enteric pathogens to animal reservoirs and even fewer have aimed to quantify exposure pathways. A total of 29 records were included after screening (Table 8).
Proper disposal and management of animal waste would not only reduce children's exposure to animal feces in the domestic environment but would also prevent the contamination of water sources and soil/fields by animal waste, both of which are proximal pathways depicted in the F-diagram. It might also reduce fly density in and around the household. Of the 29 records included, four articles examined the effect of animal waste management measures (biogas digesters and composting) on pathogen loads in animal manure, prior to being applied to fields (247–250). Two articles used pig manure, and one experimental study used poultry feces. One study did not specify the type of manure.
The increased temperature and biological activity during anaerobic digestion creates a hostile environment for enteric microorganisms, which contributes to the reduction of pathogen loads in the animal waste. Three studies investigated the performance of biogas digesters in small-scale households or farms in LMIC. Overall, biogas digesters of various design reduced the concentration of fecal indicator organisms (FIO) including enterococci, E. coli, and coliforms by 1–2.5 log10 CFU/mL.
One study (249) suggested that installation of a biogas digester was associated with a significant reduction in the count of FIO inside and outside of households in Ethiopia. However, the FIO counts were significantly increased on door handles of households with biogas digesters, indicating an increased risk of exposure of hands to fecal microorganisms by handling animal manure. Composting pig manure in clay-covered heaps is a typical practice of animal waste management in Vietnamese small-scale pig farms (250). Results showed that the concentration of E. coli in composted pig manure with 2% urea added was reduced from 4 log10 CFU/g to below the detection limit within 2 weeks. The counts of total coliforms decreased from 5.11 to 0.72 log10 CFU/g at day 45. However, Enterococcus spp. were not reduced by composting.
Three studies from the same study group evaluated the effectiveness of corralling chicken in households on mitigating infection and diarrhea associated with C. jejuni in children in a Peruvian peri-urban setting. A 2-month household trial testing the cultural and socioeconomic feasibility of corralling chickens in local communities (251) indicated that corralling didn't fully achieve the goal of separating children from poultry, as children in the household persistently played with (chickens in) the corrals, even with child-proof door latches. In addition, young children were responsible for animal care in certain households (251), which continually put them in contact with animals/feces. Besides perceptions about the connection between poultry and disease, additional food and water costs would be potential obstacles for a sustainable intervention in this setting (251). A randomized controlled trial suggested that corralling significantly increased (by 2-fold) the incidence of Campylobacter-related diarrhea in children living in the corral group compared to the control group (275). There was a non-significant decrease in asymptomatic infections in the corral group than in the control group [2.68 episodes per person per year (epy) vs. 3.12 epy]. Households were revisited 10 years after the intervention to evaluate their attitudes and poultry-raising practices. A significant drop in poultry-raising was observed, and 81% of households no longer kept chickens in their homesteads. However, the majority of the participants (92.3%) would prefer to use corrals in poultry-raising, referencing the cleanliness of the home as the most common reason (253).
A recent study piloted the use of animal hutches to provide a separate space for small animals in households in the Democratic Republic of the Congo (254). The hutches were constructed in kitchens and living quarters with three levels for different species of small animals. Rabbits and guinea pigs were placed in the top two levels separately day and night. The bottom level was for chickens and other poultry, which were only contained during the night. Participants reported that the construction of the animal hutches prevented rabbits and guinea pigs from defecating anywhere in the house, thus preventing children from eating animal feces on the floor. No studies on infection outcomes were performed.
Of the 29 studies, four reported tailored WaSH interventions for infants and young children to help reduce their exposure to feces from both humans and free-range livestock in rural smallholder families (BabyWaSH) (256). One possible intervention is to provide a safe and clean play area and feeding environment, such as a play-yard or playpen for children. Plastic manufactured play-yards, combined with caregiver education, handwashing, and safe water practices have been included in the WaSH intervention package used in the Sanitation, Hygiene, Infant Nutrition Efficacy (SHINE) trial in rural Zimbabwe. The package failed to reduce the prevalence and levels of enteric pathogens and to prevent EED in children (36, 255). A pilot study in rural Zambia (256) suggested a community-built play-yard vs. a plastic play-yard may be a feasible and acceptable alternative to plastic play-yards made in the USA (as used in SHINE) for local caregivers in LMIC, and the community-built play-yard protected the young children from ingesting soil and livestock feces. The formative research of the Reducing Enteropathy, Diarrhea, Undernutrition, and Contamination in the Environment (REDUCE) program evaluated the feasibility and acceptability of BabyWaSH interventions in the Democratic Republic of the Congo (254). Locally sourced playmats, tested as one Care Group Module prevented children from mouthing dirt.
Milk and dairy products are critical, nutrient-dense components of a healthy diet, especially for children living in LMIC. However, the high nutrient concentrations also make them the ideal medium for the rapid growth of spoilage microorganisms and foodborne pathogens which is substantiated by storing products at ambient temperatures. Two review articles and two research articles reported on improving the microbiological safety of milk and dairy products.
Boiling or heating raw milk before consumption or further production of fermented dairy products is a workable practice to avoid the spoilage of milk (257, 258). Raw milk is heated up to around 65–80°C for about 30–50 min while producing fermented yogurt-like products in Ghana and Mali and can significantly reduce the levels pathogens in the raw milk. The application of pasteurization of raw milk (formally defined as 60–65°C for 30 m or 71–74°C for 15–40 s) can reduce pathogen levels including E. coli, Salmonella, Listeria monocytogenes, and C. jejuni/coli by a factor of 106 (276).
Natural fermentation of raw milk is considered the cheapest and most convenient measure to extend the shelf-life of milk among African smallholder dairy farmers (257). Lactic acid bacteria (LAB) and yeasts involved in the fermentation process can considerably decrease the pH from approximately 6.5 to 3.0 after 24 h of fermentation. The reduced pH along with the release of antimicrobial compounds by fermenting bacteria synergistically kill or inhibit the growth of pathogenic microbes in the milk (277).
Pastoralists traditionally smoke the inner surface of plastic or wooden milk vessels by burning wood chips of specific trees and shrubs to disinfect after cleaning. A study in Kenya (259) found a significant difference of ~1 log10 cfu/cm2 in median microbial load between smoked containers and negative controls (only washed by plain water). Indicators included total viable count (TVC), total coliform count (TCC), and LAB. A qualitative study in Ethiopia also identified the smoking of milk-handling containers as one of potential risk mitigation practices in the pastoral communities (260). The researchers further assessed the effect of using stainless-steel containers on the microbial load of Ethiopian yogurt compared to the traditional smoking method with wooden containers and found no significant difference in microbial load of traditional yogurt between the two container types. Moreover, the pastoralists showed strong preference for the smoked traditional wooden containers over stainless-steel containers (275).
Twelve studies investigated food hygiene interventions related to proper preparation, handling, and feeding of complementary food for children. Of these 12 articles, two studies discussed protocols/practices of cleaning and disinfecting baby feeding bottles. An experimental study artificially contaminated baby bottles with fecal bacteria including enteropathogenic Escherichia coli (EPEC) and Salmonella spp. and then disinfected using different approaches (261). Rinsing baby bottles with soapy water followed by tap water reduced pathogen loads by 3.1–3.7 log10 cfu/mL of formula. Another study examined caregiver preferability of two bottle-cleaning methods using the improved practices (TIP) approach (262). Mothers and caregivers preferred brushing the bottle with dish detergent for 30 s after every use compared to boiling the bottle for several minutes daily.
The remaining 10 records included 3 cluster randomized controlled trials (cRCTs) (263, 264, 272), 1 cluster-randomized before-after study (265), 1 experimental study (270), and 5 observational studies (266–269, 271). These studies primarily evaluated the uptake of improved complementary food hygiene behaviors among mothers and caregivers in LMIC. Critical control points and motivational drivers of behavior change were determined based on theoretical frameworks. These intervention programs usually developed measures targeting multiple food hygiene behaviors including: (1) handwashing before cooking; (2) handwashing before feeding children; (3) washing cooking utensils with safe water and soap and drying them on a clean and elevated surface; (4) proper and safe storage of cooked food and utensils; (5) thorough reheating food before feeding; (6) boiling children's drinking water. Seven of these studies only measured the adoption of recommended behaviors, and the results suggested a varying degree of increase in targeted complementary food hygiene behaviors. Two articles (270, 271) evaluated the effects of improved hygiene practices on reduction of microorganisms in foods and found a significant reduction in fecal contamination of the food samples 3 weeks after training of mothers. Only one RCT measured not only the intermediate outcome (adoption rate of improved behavior) but also a health-related outcome (272); children's diarrhea was significantly reduced by 60 and 30% at 6 months and 32 months post-intervention, respectively, suggesting noteworthy short and long-term effects of the community-level intervention.
Handwashing has been identified as one of the critical target behaviors that help interrupt fecal-oral transmission. One recent systematic review assessed the effects of handwashing promotion on preventing diarrhea in children and adults based on the results of 29 randomized controlled trials published before January 2020, of which 15 RCTs are community-based trials in LMIC with 29,347 participants (273). The handwashing promotion interventions consisted of various health education activities, along with provision of free soap in some trials. The major time points for handwashing targeted in the trials included after defecation or after disposal of children's feces and before eating, preparing, or handling foods. Handwashing prevented ~25% of diarrhea episodes.
One systematic review summarized studies evaluating interventions to improve the microbial quality of drinking water to prevent diarrhea (274). A total of 55 studies published before November 2014 were included in this systematic review; 50 were conducted in LMIC. The study types included cRCTs, quasi-RCTs, and controlled before-and-after studies. The primary outcome of interest in most trials was self-reported diarrhea. Interventions to improve the water quality were divided into two categories: source-based improvements and point-of-use (POU) interventions. In LMIC settings, the source-based interventions included providing protected ground water, communal tap stands, and improved community water supplies through chlorination or filtration. POU interventions consisted of chlorination, flocculation, installation of filtration systems, and solar disinfection. Current evidence was not sufficient to determine whether source-based interventions reliably reduced diarrhea, whereas POU water treatment measures demonstrated varying effects on reducing diarrhea episodes. Distributing disinfection products to households may reduce diarrhea by around 25%. POU filtration systems, specifically ceramic filters, biosand systems and LifeStraw filters, may lower diarrhea episodes by around a half. Proper application of SODIS (having filled bottles exposed to direct sunlight for at least 6 h before drinking) may reduce diarrhea by around 30%. No studies have evaluated the impact of POU water treatment on asymptomatic infections.
To understand the risks and benefits of ASF from livestock production, we started with a robust understanding of the literature on the benefits of livestock production. We reviewed the three primary pathways through which livestock production could benefit child nutritional outcomes: production, income, and empowerment.
This review found overall positive impacts of livestock production interventions on child and household dietary outcomes. Because improved livestock production can generate income and increase the availability of nutrient-rich ASF, livestock production interventions can have important implications for smallholder households compared to education-only or other non-production-focused interventions that aim to increase ASF consumption (73). Furthermore, the focus for livestock production interventions should not be on production or education alone, as increased production does not always lead to increased utilization or consumption of ASF (57, 116, 278–280); integrating an evidence-based educational or BCC strategy as part of the intervention may facilitate better nutritional and health outcomes, particularly for women and children (122). Benefits from livestock production interventions can occur rapidly but have the potential to strengthen over time, so coupling livestock production interventions with education, technical assistance, and/or BCC may also have implications for long-term sustainability. This may be particularly relevant to women and children, as the dynamics of intrahousehold ASF allocation can be complex (281). Translating production into optimal consumption of ASF requires a combination of efforts, including promoting optimal livestock keeping practices to maintain a healthy herd size that can meet both consumption and income needs and addressing sociocultural and gendered norms and practices (83).
The contribution of livestock production to household income may be limited for low-income households as a result of constraints in their access to markets, input and output services, hired labor, and high productivity animals (86). Evidence from three reviews points to the complexity of the allocation of income from livestock production, suggesting that livestock play multiple intersecting roles in smallholder farming households (116, 280, 282). There is some evidence that households engaged in livestock production may have to meet a certain income or livestock production threshold prior to livestock production significantly benefitting the household's nutrition (86, 98, 109, 283, 284).
Furthermore, the availability of and access to markets may cause a shift toward sale of livestock for income generation, as smallholder farmers are integrated into the cash economy and face competitive prices for livestock products (57, 285). Markets, however, also provide access to complementary resources that contribute to overall health and well-being, such education, health services, and other non-food items (89, 286). The presence of markets may also contribute to the sustainability and scalability of livestock production interventions, as livestock production may have positive implications for the local food environment when ASF are produced and sold locally; however, the results are mixed and suggest stronger support for livestock ownership at the household level and improved ASF consumption (73, 81). More research is needed to understand how to optimize dietary intake through integration into local markets.
Separating the effects of the livestock production and income pathways on dietary intake can be challenging, as the income pathway may be dependent on the production pathway (70). Their distinction, however, may be important for households that use livestock production primarily for their own consumption, as production for own-consumption can occur alongside production for sale as households adapt to changing agricultural and market conditions (287).
There is some evidence that livestock production interventions with women as the primary recipients have beneficial effects on women's empowerment. Livestock represent a store of value and wealth for rural smallholder households, and women tend to be more engaged in poultry production than in other livestock production activities (282, 288). Women are crucial actors in food systems, generally playing a major role in caring for and managing livestock, and are often the primary decision-makers about child diet and healthcare (288). Thus, addressing women's empowerment in livestock interventions has important potential for improving their ability to care for their children through facilitated earning potential, control over resources, and autonomy over decisions (75, 77, 288, 289). Increased women's empowerment, such as increasing control over production or ensuring ownership of livestock, may also have important implications for child nutrition (127, 290).
Evidence suggests that interventions incorporating BCC strategies into livestock production interventions may be more effective at changing aspects of women's empowerment than those that do not. The benefits of livestock production may not accrue to the most vulnerable within the household (which are often women and children). Furthermore, supporting livestock production by women is not an automatic route to empowerment, so livestock production interventions coupled with evidence-based BCC strategies have the potential to improve children's health outcomes through improving human and social capital, enhancing women's knowledge about production, improving production outcomes, reducing risk of diseases, and facilitating sustainability of intervention impacts over time (21, 77, 122, 290).
However, the use of different measures of dietary outcomes and women's empowerment have limited the comparability of interventions (75). Moreover, it has been argued that the use of quantitative women's empowerment measures in development research, such as the WEAI, often excludes local meanings, values, and features of empowerment, producing results that may not reflect local conceptualizations of empowerment (291). As such, livestock production interventions that aim to empower women may not be structured in relevant or sustainable ways for communities (292). Rigorous evaluations of intervention design and consistent measurements of outcomes coupled with impact pathway analyses are needed to continue to build a robust evidence base on how livestock production interventions can be designed and implemented to best contribute to women's empowerment, gender equity, and sustained improved child health and nutrition outcomes (126).
Findings from the MAL-ED study suggested consumption of foods with insufficient energy and proteins, combined with asymptomatic enteric pathogen infection were key drivers of undernutrition. In seeking to understand stunting, researchers have suggested that EED, as an outcome of asymptomatic gut infection, may be the “missing piece” (47, 49). Following this body of thought, we explicitly incorporate impaired gut health into the long-established UNICEF framework as earlier recommended (47). Conversely, both acute (wasting) and chronic (stunting) undernutrition are risk factors for diarrhea and other infectious diseases, amplifying the “vicious cycle of diseases of poverty” (293). We found that many enteric pathogens have been associated with EED and/or undernutrition outcomes. These epidemiological associations are supported by evidence from experimental studies in laboratory animals, suggesting that the combination of enteric pathogen infections and deficient diets may lead to EED and undernutrition (188). The relative risks of enteric pathogen infection differ between species or genera. Many species or genera of enteric pathogens, alone or in combination, have been associated with increased EED markers and/or growth impairment. At the population level, risks are mainly associated with those pathogens that occur at high prevalence in infants and young children. These include zoonotic pathogens, notably Campylobacter species and to a lesser extent Giardia and Cryptosporidium species. Among Campylobacter species, the impact of poorly studied non-thermotolerant (“emerging”) species such as the C. hyointestinalis and C. fetus groups have been associated with higher risks than the established thermotolerant species C. jejuni and C. coli. Thus, the full benefits of improved nutrition through livestock production can only be realized by concurrently averting CU5 infection with zoonotic enteric pathogens.
Utilizing studies that have evaluated the association between animal ownership and (a)symptomatic health outcomes without measuring the pathogens themselves (294–296), we reviewed risk factors in/around the smallholder household and their association with zoonotic pathogens in the household/farm environment, livestock, or humans. Results indicate risk of exposure through the animal contact pathway, but also point to direct/indirect exposure of CU5 from other pathways related to WaSH, infant and young child feeding, general household hygiene, or food safety. Our review found that exposure assessments embedded in these studies and the identification of putative exposure sources were based on assumptions regarding dominant exposure routes that were not quantified. Future epidemiological or interventional studies should incorporate microbiological attribution technologies to strengthen the measurement or control of risks.
There are numerous mitigation strategies to prevent transmission of zoonotic pathogens from livestock to infants and young children, yet very few of them have been tested in LMIC and even fewer are based on quantified contribution of different reservoirs and pathways to transmission. Among the possible options, many have additional benefits, including animal health, reusing waste products, and energy generation.
Reducing the transmission of zoonotic pathogens at the source by minimizing the dissemination of animal feces to broader environments is a powerful option. Biogas digesters are a low-cost and promising measure in LMIC settings, as they can effectively reduce the pathogen loads in animal waste while simultaneously generating energy for cooking and lighting. Biogas may replace the use of dung patties as cooking fuel, a practice that negatively affects indoor air quality and women and children's health (249, 297–299). Other methods of processing animal manure for safety, e.g., before applying as a fertilizer, have not yet been studied in the context of LMIC.
Measures to improve the microbial safety and quality of milk and milk products, such as boiling, natural fermentation, and smoking milk-handling containers, should continue to be encouraged in the local communities. Milking hygiene and udder health also play important roles, and interventions on milking hygiene to control mastitis should be emphasized and further studied to help reduce pathogens in the milk (300). More broadly, improved animal health management may help reduce the exposure of livestock to animal and zoonotic pathogens. For example, providing a hygienic and comfortable environment for livestock and reducing pathogen levels in feed bunks and communal water supplies can interrupt the horizontal and vertical transmission of pathogens among livestock (301, 302).
Although corralling chicken failed to reduce the Campylobacter-associated diarrhea in children in one study site, this type of intervention should not be dismissed, as contact between children and chickens was not fully controlled in these studies. Furthermore, dynamic models have suggested that when taking acquired immunity to Campylobacter into consideration, a moderate decrease in the force of infection may lead to an increase in diarrhea incidence (303, 304). As illustrated in this review, current evidence indicates that most Campylobacter infections are asymptomatic without overt diarrhea but cannot be neglected given the considerable long-term impacts on children. We suggest that the effectiveness of interventions in preventing asymptomatic colonization of enteric pathogens should also be included in the design of future interventions among children under five in LMIC.
The results of behavior-focused studies suggest that significant improvements in both individual and community level food hygiene behaviors can be achieved through theory-driven BCC approaches. However, few interventions have targeted intermediate endpoints (e.g., microbial loads) or child health outcomes; current studies are more formative in nature. Additional research is needed to evaluate the downstream effects of behavior change, to ensure that changes in caregivers' behaviors translate to improvements in child health.
Animal ownership presents competing risks and benefits to smallholder households, through animal feces exposure and nutrition supply (46). By including authors from the fields of social science, microbiology, epidemiology, and library science, we were able to review a broad array of literature to synthesize the empirical risk-benefit evidence on this topic. Though data synthesis as seen in meta-analyses was not feasible given the diversity of study designs, our novel approach had team members collectively synthesize narrative results, putting into dialogue findings across disciplines to understand the full scope of benefits and risks of SLP on human nutrition. To our knowledge, this is the first system-level risk-benefit analysis of SLP and child nutrition.
Our study had several limitations. The etiology of EED and stunting is highly complex, and focusing on specific risk factors, as in this review, may lead to oversimplification. Also, the association between enteric pathogens and undernutrition may be mediated through other disease pathways, such as anemia, whose association with livestock husbandry has been investigated in a previous review (305). Study designs and population characteristics of included studies were highly heterogeneous, limiting our ability to infer the strength of evidence of the associations reviewed in these studies and to draw direct comparisons across some results and intervention designs. Some empirical evidence included in the review comes from a reduced modeling approach, which may limit the effect of endogenous factors on outcomes such as decision-making, thereby biasing results and complicating interpretation. We also omitted non-English full texts in this review due to language capacity of the authors and time constraints, making the results vulnerable to publication bias.
In this review, we aimed to conduct a risk-benefit analysis. We acknowledge that our system-level analysis, from smallholder livestock production to child nutrition, is a sub-set of relations within a broader system, as depicted within the UNICEF framework's basic, underlying conditions. While the interdisciplinary approach to include benefits and risks expands the boundaries of dynamics examined, this, like any effort to understand a bounded part of a system, may be biased and/or inconsistent.
The level of pathogen speciation in many of the epidemiological studies in section Risks factors of exposure to or infection with zoonotic enteric pathogens associated with smallholder livestock production was limited, as they defined endpoints of human infection by pathogen groups rather than at the genus, species, or subtype level. This complicates inference about putative reservoirs of these pathogens as anthroponotic, zoonotic, or sapronotic. Further source attribution studies, particularly for pathogens with both anthroponotic and zoonotic reservoirs, are recommended. Furthermore, such studies should aim to quantify the relative importance of sources and transmission pathways so that interventions can be appropriately directed. The SaniPath analytical approach, one of the few examples of quantification, relied on E. coli as an indicator of fecal contamination, but could not differentiate sources at the reservoir level. We concur with the authors who suggested addressing this limitation by combining the tool with other attribution approaches (244). There is also a need to adapt the tool, originally developed for urban settings with a focus on human waste, to rural settings focusing on animal waste.
Very few studies address specific interventions to reduce exposure to zoonotic pathogens in LMIC, particularly in smallholder environments. Lacking quantitative assessment of the relative weights of pathogen reservoirs and pathways, available studies on control measures focus on selected transmission pathways without evidence that the targeted pathways are the main contributors to exposure. Future intervention studies should be guided by source attribution estimates and quantitative exposure assessment to select the most promising sites for intervention.
There has recently been a call for a One Health approach to strengthen efforts to improve child nutrition by taking into account enteric pathogens from animal feces, which remains a neglected element in mainstream WaSH interventions (306). The literature in this review extends this call to fully evaluate the benefits and risks of livestock production on child nutrition and supports the inclusion of poor gut health, manifested as EED, into the UNICEF framework of child undernutrition (see Figure 2). The attribution of zoonotic enteric infection in children to certain sources and implementation of corresponding control measures could forestall the risks, resulting in symptomatic diseases and poor gut health, and maximize the benefits, conduce to adequate diet intake, brought by the SLP.
SM, AH, DC, XL, and KM conceptualized the topic. With significant methodological support from NS, DC, XL, and KM specified keywords and search strategies, and conducted literature search, screenings, and data extraction. DC, XL, and KM synthesized the evidence and drafted portions of the manuscript, with substantial conceptual guidance from AH and SM. All authors contributed to the drafting of the final manuscript.
This work was funded by the United States Agency for International Development (USAID) Bureau for Food Security under Agreement #AID-OAA-L-15-00003 as part of Feed the Future Innovation Lab for Livestock Systems, and by the Bill & Melinda Gates Foundation OPP#1175487.
The use of Covidence for this publication was supported by the University of Florida Clinical and Translational Science Institute, which was supported in part by the NIH National Center for Advancing Translational Sciences under award number UL1TR001427.
Any opinions, findings, conclusions, or recommendations expressed here are those of the authors alone. The content is solely the responsibility of the authors and does not necessarily represent the official views of the funders.
The authors declare that the research was conducted in the absence of any commercial or financial relationships that could be construed as a potential conflict of interest.
All claims expressed in this article are solely those of the authors and do not necessarily represent those of their affiliated organizations, or those of the publisher, the editors and the reviewers. Any product that may be evaluated in this article, or claim that may be made by its manufacturer, is not guaranteed or endorsed by the publisher.
We thank Daniel Acosta and Isa Arrazola for aesthetically strengthening the figures in this publication, and Lela Scully for copy-editing.
The Supplementary Material for this article can be found online at: https://www.frontiersin.org/articles/10.3389/fnut.2021.751686/full#supplementary-material
1. World Health Organization. Fact sheets—Malnutrition. Available online at: https://www.who.int/news-room/fact-sheets/detail/malnutrition (accessed June 20, 2021).
3. Alderman H. The economic cost of a poor start to life. J Dev Orig Health Dis. (2010) 1:19–25. doi: 10.1017/S2040174409990158
4. Murray-Kolb LE, Acosta AM, De Burga RR, Chavez CB, Flores JT, Olotegui MP, et al. Early childhood cognitive development is affected by interactions among illness, diet, enteropathogens and the home environment: findings from the MAL-ED birth cohort study. BMJ Glob Health. (2018) 3:e000752. doi: 10.1136/bmjgh-2018-000752
5. Nahar B, Hossain M, Mahfuz M, Islam MM, Hossain MI, Murray-Kolb LE, et al. Early childhood development and stunting: findings from the MAL-ED birth cohort study in Bangladesh. Matern Child Nutr. (2020) 16:e12864. doi: 10.1111/mcn.12864
6. Hoffman DJ, Sawaya AL, Verreschi I, Tucker KL, Roberts SB. Why are nutritionally stunted children at increased risk of obesity? Studies of metabolic rate and fat oxidation in shantytown children from São Paulo, Brazil. Am J Clin Nutr. (2000) 72:702–7. doi: 10.1093/ajcn/72.3.702
7. Matrins VJB, Toledo Florêncio TMM, Grillo LP, Franco M do CP, Martins PA, Clemente APG, et al. Long-lasting effects of undernutrition. Int J Environ Res Public Health. (2011) 8:1817–46. doi: 10.3390/ijerph8061817
8. Wells JC, Sawaya AL, Wibaek R, Mwangome M, Poullas MS, Yajnik CS, et al. The double burden of malnutrition: aetiological pathways and consequences for health. Lancet. (2020) 395:75–88. doi: 10.1016/S0140-6736(19)32472-9
9. United Nations Children's Fund. UNICEF's Approach to Scaling Up Nutrition for Mothers and Their Children. Discussion Paper. New York, NY: United Nations Children's Fund (2015).
10. Herforth A, Harris J. Linking Agriculture & Nutrition Understanding and Applying Primary Pathways and Principles. (2014). Available online at: www.spring-nutrition.org (accessed June 20, 2021)
11. Adesogan AT, Havelaar AH, McKune SL, Eilittä M, Dahl GE. Animal source foods: Sustainability problem or malnutrition and sustainability solution? Perspective matters. Glob Food Sec. (2020) 25:100325. doi: 10.1016/j.gfs.2019.100325
12. WHO. World Health Assembly Global Nutrition Targets 2025: Stunting Policy Brief. Geneva: WHO (2014).
13. Carletto G, Ruel M, Winters P, Zezza A. Farm-Level pathways to improved nutritional status: introduction to the special issue. J Dev Stud. (2015) 51:945–57. doi: 10.1080/00220388.2015.1018908
14. Sharma IK, Di Prima S, Essink D, Broerse JEW. Nutrition-Sensitive agriculture: a systematic review of impact pathways to nutrition outcomes. Adv Nutr. (2021) 12:251–75. doi: 10.1093/advances/nmaa103
15. Dominguez-Salas P, Kauffmann D, Breyne C, Alarcon P. Leveraging human nutrition through livestock interventions: perceptions, knowledge, barriers and opportunities in the Sahel. Food Secur. (2019) 11:777–96. doi: 10.1007/s12571-019-00957-4
16. Njuki J, Wyatt A, Baltenweck I, Yount K, Null C, Ramakrishnan U, et al. An exploratory study of dairying intensification, women's decision making, and time use and implications for child nutrition in Kenya. Eur J Dev Res. (2016) 28:722–40. doi: 10.1057/ejdr.2015.22
17. Hossain ME, Hoque MA, Giorgi E, Fournié G, Das GB, Henning J. Impact of improved small-scale livestock farming on human nutrition. Sci Rep. (2021) 11:191. doi: 10.1038/s41598-020-80387-x
18. Jones AD, Shrinivas A, Bezner-Kerr R. Farm production diversity is associated with greater household dietary diversity in Malawi: findings from nationally representative data. Food Policy. (2014) 46:1–12. doi: 10.1016/j.foodpol.2014.02.001
19. Muchenje A, Mushunje V. Determinants of rural household dietary diversity: the case of Amatole and Nyandeni districts, South Africa. J Dev Sust. (2013) 2:2233–47. Available online at: www.isdsnet.com/ijds (accessed June 22, 2021).
20. Galie A, Teufel N, Korir L, Baltenweck I, Girard AW, Dominguez-Salas P, et al. The women's empowerment in livestock index. Soc Indic Res. (2019) 142:799–825. doi: 10.1007/s11205-018-1934-z
21. Jin M, Iannotti LL. Livestock production, animal source food intake, and young child growth: the role of gender for ensuring nutrition impacts. Soc Sci Med. (2014) 105:16–21. doi: 10.1016/j.socscimed.2014.01.001
22. Kristjanson P, Waters-Bayer A, Johnson N, Tipilda A, Njuki J, Baltenweck I, et al. Livestock and women's livelihoods. In: Quisumbing AR, Meinzen-Dick R, Raney TL, Croppenstedt A, Behrman JA, Peterman A, editors. Gender in Agriculture: Closing the Knowledge Gap. Rome: Food and Agriculture Organization of the United Nations (2014).
23. Cunningham K, Ruel M, Ferguson E, Uauy R. Women's empowerment and child nutritional status in South Asia: a synthesis of the literature. Matern Child Nutr. (2015) 11:1–19. doi: 10.1111/mcn.12125
24. Santoso MV, Kerr RB, Hoddinott J, Garigipati P, Olmos S, Young SL. Role of women's empowerment in child nutrition outcomes: a systematic review. Adv Nutr. (2019) 10:1138–51. doi: 10.1093/advances/nmz056
25. Jones R, Haardörfer R, Ramakrishnan U, Yount KM, Miedema S, Girard AW. Women's empowerment and child nutrition: the role of intrinsic agency. SSM - Popul Heal. (2019) 9:1000475. doi: 10.1016/j.ssmph.2019.100475
26. IFPRI. Women's Empowerment and Nutrition: An Evidence Review. International Food Policy Research Institute. Available onlne at: https://www.ifpri.org/publication/women's-empowerment-and-nutrition-evidence-review (accessed June 22, 2021).
27. Dominguez-Salas P, Omore A, Omosa E, Ouma E. Agrifood systems in low- and middle-income countries: Status and opportunities for smallholder dairy in LMIC. In: Encyclopedia of Food Security and Sustainability. Elsevier (2018) 326–39.
28. Balehegn M, Duncan A, Tolera A, Ayantunde AA, Issa S, Karimou M, et al. Improving adoption of technologies and interventions for increasing supply of quality livestock feed in low- and middle-income countries. Glob Food Sec. (2020) 26:100372. doi: 10.1016/j.gfs.2020.100372
29. Shapiro MJ, Downs SM, Swartz HJ, Parker M, Quelhas D, Kreis K, et al. A systematic review investigating the relation between animal-source food consumption and stunting in children aged 6-60 months in low and middle-income Countries. Adv Nutr. (2019) 10:827–47. doi: 10.1093/advances/nmz018
30. Lauer JM, Duggan CP, Ausman LM, Griffiths JK, Webb P, Bashaasha B, et al. Unsafe drinking water is associated with environmental enteric dysfunction and poor growth outcomes in young children in rural southwestern Uganda. Am J Trop Med Hyg. (2018) 99:1606–12. doi: 10.4269/ajtmh.18-0143
31. Exum NG, Lee GO, Olórtegui MP, Yori PP, Salas MS, Trigoso DR, et al. A longitudinal study of household water, sanitation, and hygiene characteristics and environmental enteropathy markers in children less than 24 months in Iquitos, Peru. Am J Trop Med Hyg. (2018) 98:995–1004. doi: 10.4269/ajtmh.17-0464
32. Chen D, Mckune SL, Singh N, Hassen JY, Gebreyes W, Manary M, et al. Campylobacter colonization, environmental enteric dysfunction, stunting, and associated risk factors among young children in rural ethiopia: a cross-sectional study from the campylobacter genomics and environmental enteric dysfunction (CAGED) project. Front Public Heal. (2020) 8:1043. doi: 10.3389/fpubh.2020.615793
33. Pickering AJ, Null C, Winch PJ, Mangwadu G, Arnold BF, Prendergast AJ, et al. The WASH benefits and SHINE trials: interpretation of WASH intervention effects on linear growth and diarrhoea. Lancet Glob Heal. (2019) 7:e1139–46. doi: 10.1016/S2214-109X(19)30268-2
34. Tickell KD, Atlas HE, Walson JL. Environmental enteric dysfunction: a review of potential mechanisms, consequences and management strategies. BMC Med. (2019) 17:181. doi: 10.1186/s12916-019-1417-3
35. Lin A, Ali S, Arnold BF, Ziaur Rahman M, Alauddin M, Grembi J, et al. Effects of water, sanitation, handwashing, and nutritional interventions on environmental enteric dysfunction in young children: a cluster-randomized, controlled trial in rural Bangladesh. Clin Infect Dis. (2020) 70:738–47. doi: 10.1093/cid/ciz291
36. Gough EK, Moulton LH, Mutasa K, Ntozini R, Stoltzfus RJ, Majo FD, et al. Effects of improved water, sanitation, and hygiene and improved complementary feeding on environmental enteric dysfunction in children in rural Zimbabwe: a cluster-randomized controlled trial. PLoS Negl Trop Dis. (2020) 14:e0007963. doi: 10.1371/journal.pntd.0007963
37. Sinharoy SS, Reese HE, Praharaj I, Chang HH, Clasen T. Effects of a combined water and sanitation intervention on biomarkers of child environmental enteric dysfunction and associations with height-for-age Z-score: a matched cohort study in rural Odisha, India. PLoS Negl Trop Dis. (2021) 15:e0009198. doi: 10.1371/journal.pntd.0009198
38. Prendergast AJ, Gharpure R, Mor S, Viney M, Dube K, Lello J, et al. Putting the “A” into WaSH: a call for integrated management of water, animals, sanitation, and hygiene. Lancet Planet Heal. (2019) 3:e336–7. doi: 10.1016/S2542-5196(19)30129-9
39. Gebreyes WA, Jackwood D, de Oliveira CJB, Lee C-W, Hoet AE, Thakur S. Molecular epidemiology of infectious zoonotic and livestock diseases. Microbiol Spectr. (2020) 8. doi: 10.1128/microbiolspec.AME-0011-2019
40. Taylor LH, Latham SM, Woolhouse MEJ. Risk factors for human disease emergence. Philos Trans R Soc B Biol Sci. (2001) 356:983–9. doi: 10.1098/rstb.2001.0888
41. Jones KE, Patel NG, Levy MA, Storeygard A, Balk D, Gittleman JL, et al. Global trends in emerging infectious diseases. Nature. (2008) 451:990–3. doi: 10.1038/nature06536
42. Woolhouse MEJ, Gowtage-Sequeria S. Host range and emerging and reemerging pathogens. Emerg Infect Dis. (2005) 11:1842–7. doi: 10.3201/eid1112.050997
43. The World Bank. People, Pathogens and Our Planet. (2010). Available online at: https://openknowledge.worldbank.org/bitstream/handle/10986/2844/508330ESW0whit1410B01PUBLIC1PPP1Web.pdf?sequence=1&isAllowed=y
44. Wagner EG, Lanoix JN. Excreta disposal for rural areas and small communities. In: Monograph Series. Geneva: World Health Organization (1958).
45. Li M, Havelaar AH, Hoffmann S, Hald T, Kirk MD, Torgerson PR, et al. Global disease burden of pathogens in animal source foods, 2010. PLoS ONE. (2019) 14:e0216545. doi: 10.1371/journal.pone.0216545
46. Penakalapati G, Swarthout J, Delahoy MJ, McAliley L, Wodnik B, Levy K, et al. Exposure to animal feces and human health: a systematic review and proposed research priorities. Environ Sci Technol. (2017) 51:11537–52. doi: 10.1021/acs.est.7b02811
47. Acosta AM, De Burga RR, Chavez CB, Flores JT, Olotegui MP, Pinedo SR, et al. Relationship between growth and illness, enteropathogens and dietary intakes in the first 2 years of life: findings from the MAL-ED birth cohort study. BMJ Glob Heal. (2017) 2:e000370. doi: 10.1136/bmjgh-2017-000370
48. Syed S, Ali A, Duggan C. Environmental enteric dysfunction in children: a review hhs public access. J Pediatr Gastroenterol Nutr. (2016) 63:6. doi: 10.1097/MPG.0000000000001147
49. Budge S, Parker AH, Hutchings PT, Garbutt C. Environmental enteric dysfunction and child stunting. Nutr Rev. (2019) 77:240–53. doi: 10.1093/nutrit/nuy068
50. Morais MB, Silva GAP. Environmental enteric dysfunction and growth. J Pediatr. (2019) 95:S85–94. doi: 10.1016/j.jped.2018.11.004
51. Owino V, Ahmed T, Freemark M, Kelly P, Loy A, Manary M, et al. Environmental enteric dysfunction and growth failure/stunting in global child health. Pediatrics. (2016) 138:e20160641. doi: 10.1542/peds.2016-0641
52. Harper KM, Mutasa M, Prendergast AJ, Humphrey J, Manges AR. Environmental enteric dysfunction pathways and child stunting: a systematic review. PLoS Negl Trop Dis. (2018) 12:e0006205. doi: 10.1371/journal.pntd.0006205
53. Humphrey JH. Child undernutrition, tropical enteropathy, toilets, and handwashing. Lancet. (2009) 374:1032–5. doi: 10.1016/S0140-6736(09)60950-8
54. Berti PR, Araujo Cossio H. Raising chickens for increased egg consumption in a rural highland bolivian population. Food Secur. (2017) 9:1329–41. doi: 10.1007/s12571-017-0728-5
55. McKune SL, Stark H, Sapp AC, Yang Y, Slanzi CM, Moore EV, et al. Behavior change, egg consumption, and child nutrition: a cluster randomized controlled trial. Pediatrics. (2020) 146:e2020007930. doi: 10.1542/peds.2020-007930
56. Omer A, Mulualem D, Classen H, Vatanparast H, Whiting SJ. A community poultry intervention to promote egg and eggshell powder consumption by young children in halaba special woreda, SNNPR, Ethiopia. J Agric Sci. (2018) 10. doi: 10.5539/jas.v10n5p1
57. Passarelli S, Ambikapathi R, Gunaratna NS, Madzorera I, Canavan CR, Noor AR, et al. A chicken production intervention and additional nutrition behavior change component increased child growth in ethiopia: a cluster-randomized trial. J Nutr. (2020) 150:2806–17. doi: 10.1093/jn/nxaa181
58. Talukder A, Haselow NJ, Osei AK, Villate E, Reario D, Kroeun H, et al. Homestead food production model contributes to improved household food security and nutrition status of young children and women in poor populations: lessons learned from scaling-up programs in Asia (Bangladesh, Cambodia, Nepal and Philippines). J F Actions. (2010). Available online at: http://journals.openedition.org/factsreports/404
59. Walingo MK. Role of livestock projects in empowering women smallholder farmers for sustainable food security in rural Kenya. Afric J Food Agric Nutr Dev. (2009) 9:1468–83. doi: 10.4314/ajfand.v9i7.47678
60. Nielsen H, Roos N, Thilsted SH. The impact of semi-scavenging poultry production on the consumption of animal source foods by women and girls in Bangladesh. J Nutr. (2003) 133:4027S−30S. doi: 10.1093/jn/133.11.4027S
61. Olney DK, Talukder A, Iannotti LL, Ruel MT, Quinn V. Assessing impact and impact pathways of a homestead food production program on household and child nutrition in Cambodia. Food Nutr Bull. (2009) 30:355–69. doi: 10.1177/156482650903000407
62. Ayele Z, Peacock C. Improving access to and consumption of animal source foods in rural households: the experiences of a women-focused goat development program in the highlands of Ethiopia. J Nutr. (2003) 133:3981S−6S. doi: 10.1093/jn/133.11.3981S
63. Olney DK, Pedehombga A, Ruel MT, Dillon A. A 2-year integrated agriculture and nutrition and health behavior change communication program targeted to women in burkina faso reduces anemia, wasting, and diarrhea in children 3–12.9 months of age at baseline: a cluster-randomized controlled trial. J Nutr. (2015) 145:1317–24. doi: 10.3945/jn.114.203539
64. Alabi OO, Ajayi FO, Bamidele O, Yakubu A, Ogundu EU, Sonaiya EB, et al. Impact assessment of improved chicken genetics on livelihoods and food security of smallholder poultry farmers in Nigeria. Livest Res Rural Dev. (2020) 32:77. Available online at: http://www.lrrd.org/lrrd32/5/alabi32077.html
65. Murty PVVS, Rao MV, Bamji MS. Impact of enriching the diet of women and children through health and nutrition education, introduction of homestead gardens and backyard poultry in rural India. Agric Res. (2016) 5:210–7. doi: 10.1007/s40003-016-0206-x
66. Rawlins R, Pimkina S, Barrett CB, Pedersen S, Wydick B. Got milk? The impact of heifer International's livestock donation programs in Rwanda on nutritional outcomes. Food Policy. (2014) 44:202–13. doi: 10.1016/j.foodpol.2013.12.003
67. Rosenberg AM, Maluccio JA, Harris J, Mwanamwenge M, Nguyen PH, Tembo G, et al. Nutrition-sensitive agricultural interventions, agricultural diversity, food access and child dietary diversity: evidence from rural Zambia. Food Policy. (2018) 80:10–23. doi: 10.1016/j.foodpol.2018.07.008
68. Dumas S, Lungu L, Mulambya N, Daka W, McDonald E, Steubing E, et al. Sustainable smallholder poultry interventions to promote food security and social, agricultural, and ecological resilience in the luangwa valley, Zambia. Food Secur. (2016) 8:507–20. doi: 10.1007/s12571-016-0579-5
69. Henning J, Morton J, Pym R, Hla T, Meers J. Evaluation of strategies to improve village chicken production: controlled field trials to assess effects of newcastle disease vaccination and altered chick rearing in Myanmar. Prev Vet Med. (2009) 90:17–30. doi: 10.1016/j.prevetmed.2009.04.007
70. Olney DK, Vicheka S, Kro M, Chakriya C, Kroeun H, Hoing LS, et al. Using program impact pathways to understand and improve program delivery, utilization, and potential for impact of helen keller international's homestead food production program in Cambodia. Food Nutr Bull. (2013) 34:169–84. doi: 10.1177/156482651303400206
71. Habtemariam K, Ayalew W, Habte GZ, Meske G. Enhancing the role of livestock production in improving nutritional status of farming families: lessons from a dairy goat development project in Eastern Ethiopa. Livest Res Rural Dev. (2003) 15:6. Available online at: http://www.lrrd.org/lrrd15/6/kass156.htm
72. Iannotti L, Cunningham K, Ruel M. Diversifying into healthy diets: homestead food production in Bangladesh. In: Millions Fed: Proven Successes in Agricultural Development. Washington, DC: International Food Policy Research Institute (2009). p. 145–51.
73. Jodlowski M, Winter-Nelson A, Baylis K, Goldsmith PD. Milk in the data: food security impacts from a livestock field experiment in Zambia. World Dev. (2016) 77:99–114. doi: 10.1016/j.worlddev.2015.08.009
74. Langworthy M, Caldwell R. Save the Children USA in Bangladesh: Jibon O Jibika Program: Endline Survey Report. TANGO International (2009).
75. Olney DK, Bliznashka L, Pedehombga A, Dillon A, Ruel MT, Heckert J. A 2-year integrated agriculture and nutrition program targeted to mothers of young children in burkina faso reduces underweight among mothers and increases their empowerment: a cluster-randomized controlled trial. J Nutr. (2016) 146:1109–17. doi: 10.3945/jn.115.224261
76. Osei A, Pandey P, Nielsen J, Pries A, Spiro D, Davis D, et al. Combining home garden, poultry, and nutrition education program targeted to families with young children improved anemia among children and anemia and underweight among nonpregnant women in Nepal. Food Nutr Bull. (2016) 38:49–64. doi: 10.1177/0379572116676427
77. Salazar L, Fahsbender J, Kim N. Livestock Transfers, Food Security and Women's Empowerment: Evidence From a Randomized Phased-in Program in Nicaragua. IDB Working Paper Series. Washington, DC: International American Development Bank (2018). doi: 10.18235/0001447
78. Broaddus-Shea ET, Manohar S, Thorne-Lyman AL, Bhandari S, Nonyane BAS, Winch PJ, et al. KP. Small-Scale livestock production in nepal is directly associated with children's increased intakes of eggs and dairy, but not meat. Nutrients. (2020) 12:252. doi: 10.3390/nu12010252
79. Choudhury S, Headey DD. Household dairy production and child growth: evidence from Bangladesh. Econ Hum Biol. (2018) 30:150–61. doi: 10.1016/j.ehb.2018.07.001
80. Mosites E, Aol G, Otiang E, Bigogo G, Munyua P, Montgomery JM, et al. Child height gain is associated with consumption of animal-source foods in livestock-owning households in Western Kenya. Public Health Nutr. (2017) 20:336–45. doi: 10.1017/S136898001600210X
81. Hoddinott J, Headey D, Dereje M. Cows, missing milk markets, and nutrition in rural Ethiopia. J Dev Stud. (2015) 51:958–75. doi: 10.1080/00220388.2015.1018903
82. Azzarri C, Zezza A, Haile B, Cross E. Does livestock ownership affect animal source foods consumption and child nutritional status? Evidence from rural Uganda. J Dev Stud. (2015) 51:1034–59. doi: 10.1080/00220388.2015.1018905
83. Bundala N, Kinabo J, Jumbe T, Rybak C, Sieber S. Does homestead livestock production and ownership contribute to consumption of animal source foods? A pre-intervention assessment of rural farming communities in Tanzania. Sci African. (2020) 7:e00252. doi: 10.1016/j.sciaf.2019.e00252
84. de Zoysa M. Transformation of traditional silvo-pastoral home-gardens: a case study in Southern Sri Lanka. Sustain Agric Res. (2018) 7:98–108. doi: 10.5539/sar.v7n1p98
85. Hetherington JB, Wiethoelter AK, Negin J, Mor SM. Livestock ownership, animal source foods and child nutritional outcomes in seven rural village clusters in Sub-Saharan Africa. Agric Food Secur. (2017) 6:9. doi: 10.1186/s40066-016-0079-z
86. Kidoido M, Korir L. Do low-income households in Tanzania derive income and nutrition benefits from dairy innovation and dairy production? Food Secur. (2015) 7:681–92. doi: 10.1007/s12571-015-0419-z
87. Enahoro D, Lannerstad M, Pfeifer C, Dominguez-Salas P. Contributions of livestock-derived foods to nutrient supply under changing demand in low- and middle-income countries. Glob Food Sec. (2018) 19:1–10. doi: 10.1016/j.gfs.2018.08.002
88. Dumas S, Kassa L, Young S, Travis A. Examining the association between livestock ownership typologies and child nutrition in the Luangwa Valley, Zambia. PLoS ONE. (2018) 13:e0191339. doi: 10.1371/journal.pone.0191339
89. Mekuria W, Mekonnen K, Melese T. Food production and consumption in the highlands of Ethiopia: the missing link in food systems. Rev Agric Appl Econ. (2019) 22:71–80. doi: 10.15414/raae.2019.22.02.71-80
90. Mango N, Makate C, Mapemba L, Sopo M. The role of crop diversification in improving household food security in central Malawi. Agric Food Secur. (2018) 7:1–10. doi: 10.1186/s40066-018-0160-x
91. Aweke CS, Lahiff E, Hassen JY. The contribution of agriculture to household dietary diversity: evidence from smallholders in East Hararghe, Ethiopia. Food Secur. (2020) 12:625–36. doi: 10.1007/s12571-020-01027-w
92. Nicholson CF, Thornton PK, Muinga RW. Household-level impacts of dairy cow ownership in coastal Kenya. J Agric Econ. (2004) 55:175–95. doi: 10.1111/j.1477-9552.2004.tb00092.x
93. Elhadi YA, Nyariki DM, Wasonga O V. Role of camel milk in pastoral livelihoods in Kenya: contribution to household diet and income. Pastor Res Policy Pract. (2015) 5:1–8. doi: 10.1186/s13570-015-0028-7
94. Ahmed MM, Jabbar M, Ehui S. Household-level economic and nutritional impacts of market-oriented dairy production in the ethiopian highlands. Food Nutr Bull. (2000) 21:460–5. doi: 10.1177/156482650002100423
95. Rubhara TT, Oduniyi OS, Mudhara M, Akwasi AM. Analysis of household food expenditure patterns. A case of Shamva district Zimbabwe. Futur Food J Food Agric Soc. (2020) 8:20–8. doi: 10.17170/kobra-202003241099
96. Romeo A, Meerman J, Demeke M, Scognamillo A, Asfaw S. Linking farm diversification to household diet diversification: evidence from a sample of Kenyan ultra-poor farmers. Food Secur. (2016) 8:1069–85. doi: 10.1007/s12571-016-0617-3
97. Alkire S, Meinzen-Dick R, Peterman A, Quisumbing A, Seymour G, Vaz A. Women's empowerment in agriculture index. World Dev. (2013) 52:71–91. doi: 10.1016/j.worlddev.2013.06.007
98. Aklilu HA, Udo HMJ, Almekinders CJM, Van der Zijpp AJ. How resource poor households value and access poultry: village poultry keeping in Tigray, Ethiopia. Agric Syst. (2008) 96:175–83. doi: 10.1016/j.agsy.2007.07.008
99. Dumas S, Maranga A, Mbullo P, Collins S, Wekesa P, Onono M, et al. “Men are in front at eating time, but not when it comes to rearing the chicken”: unpacking the gendered benefits and costs of livestock ownership in Kenya. Food Nutr Bull. (2018) 39:3–27. doi: 10.1177/0379572117737428
100. Mapiye C, Sibanda S. Constraints and opportunities of village chicken production systems in the smallholder sector of Rushinga district of Zimbabwe. Livest Res Rural Dev. (2005) 17:2005. Available online at: http://www.lrrd.org/lrrd17/10/mapi17115.htm
101. Nori M. Along the milky way: marketing camel milk in puntland, Somalia. Eur J Dev Res. (2010) 22:696–714. doi: 10.1057/ejdr.2010.40
102. Veena B. Status of women in transhumant societies. J Sociol Soc Anthropol. (2011) 2:1–22. doi: 10.1080/09766634.2011.11885543
103. Okitoi LO, Ondwasy HO, Obali MP, Murekefu F. Gender issues in poultry production in rural households of Western Kenya. Livest Res Rural Dev. (2007) 19:2007. Available online at: http://www.lrrd.org/lrrd19/2/okit19017.htm
104. Tavenner K, Crane TA. Gender power in Kenyan dairy: cows, commodities, and commercialization. Agric Human Values. (2018) 35:701–15. doi: 10.1007/s10460-018-9867-3
105. Carter NA, Humphries S, Grace D, Ouma EA, Dewey CE. Men and women farmers' perceptions of adopting improved diets for pigs in Uganda: decision-making, income allocation, and intra-household strategies that mitigate relative disadvantage. Agric Food Secur. (2017) 6:1–24. doi: 10.1186/s40066-017-0095-7
106. Bikila A. Revisiting the status of pastoral women's access to and control over livelihood assets; evidences from fafan zone, somali region, Ethiopia. J Agric Ext Rural Dev. (2019) 11:114–27. doi: 10.5897/JAERD2019.1058
107. Karmeback VN, Wairore JN, Jirstrom M, Nyberg G. Assessing gender roles in a changing landscape: diversified agro-pastoralism in drylands of West Pokot, Kenya. Pastor Res Policy Pract. (2015) 5:21. doi: 10.1186/s13570-015-0039-4
108. Meseret M, Solomon D, Tadelle D. Marketing system, socio economic role and intra household dynamics of indigenous chicken in gomma wereda, jimma zone, Ethiopia. Livest Res Rural Dev. (2011) 23. Available online at: http://www.lrrd.org/lrrd23/6/mese23131.htm
109. Muchadeyi FC, Sibanda S, Kusina NT, Kusina J, Makuza S. The village chicken production system in rushinga District of Zimbabwe. Livest Res Rural Dev. (2004) 16:2004. Available online at: http://www.lrrd.org/lrrd16/6/much16040.htm
110. Valdivia C. Gender, livestock assets, resource management, and food security: lessons from the SR-CRSP. Agric Human Values. (2001) 18:27–39. doi: 10.1023/A:1007613031102
111. Sultana R, Nahar N, Rimi NA, Azad S, Islam MS, Gurley ES, et al. Backyard poultry raising in Bangladesh: a valued resource for the villagers and a setting for zoonotic transmission of avian influenza. A qualitative study. Rural Remote Heal. (2012) 12:1–14. doi: 10.22605/RRH1927
112. Guliye AY Noor IM Bebe BO Kosgey IS. Role of camels (Camelus dromedarius) in the traditional lifestyle of Somali pastoralists in northern Kenya. Outlook Agric. (2007) 36:29–34. doi: 10.5367/000000007780223669
113. Tsegaye B, Adugna T, Berg T. Livestock production and feed resource constraints in Akaki and Lume districts, central Ethiopia. Outlook Agric. (2008) 37:15–21. doi: 10.5367/000000008783883582
114. Abebe W, Galmessa U. Gender role in peri urban dairy production system of Ambo town, Ethiopia. J Agric Ext Rural Dev. (2011) 3:224–8. doi: 10.5897/JAERD11.030
115. Badejo AF, Majekodunmi AO, Kingsley P, Smith J, Welburn SC. The impact of self-help groups on pastoral women's empowerment and agency: a study in Nigeria. Pastoralism. (2017) 7:28. doi: 10.1186/s13570-017-0101-5
116. Alders R, Dumas S, Rukambile E, Magoke G, Maulaga W, Jong J, et al. Family poultry: multiple roles, systems, challenges, and options for sustainable contributions to household nutrition security through a planetary health lens. Matern Child Nutr. (2018) 14:e12668. doi: 10.1111/mcn.12668
117. Salmon G, Teufel N, Baltenweck I, Wijk M van, Claessens L, Marshall K. Trade-offs in livestock development at farm level: different actors with different objectives. Glob Food Sec. (2018) 17:103–12. doi: 10.1016/j.gfs.2018.04.002
118. Kimaro EG, Lyimo-Macha JG, Jeckoniah JN. Gender roles in small holder dairy farming: pertinent issues on access and control over dairy farming resources in Arumeru district, Tanzania. Livest Res Rural Dev. (2013) 25. Available online at: http://www.lrrd.org/lrrd25/5/kima25082.htm
119. Jothilakshmi M, Thirunavukkarasu D, Sudeepkumar NK. Exit of youths and feminization of smallholder livestock production - a field study in India. Renew Agric Food Syst. (2014) 29:146–50. doi: 10.1017/S174217051300001X
120. Didanna H, Wossen A, Shano B. Future prospects and challenges of intensifying dairy production systems in Ethiopia. African J Sci Technol Innov Dev. (2019) 11:653–61. doi: 10.1080/20421338.2019.1571149
121. Namgail T, Veer Bhatnagar Y, Mishra C, Bagchi S. Pastoral nomads of the Indian Changthang: production system, landuse and socioeconomic changes. Hum Ecol Interdiscip J. (2007) 35:497–504. doi: 10.1007/s10745-006-9107-0
122. Nordhagen S, Klemm R. Implementing small-scale poultry-for-nutrition projects: successes and lessons learned. Matern Child Nutr. (2018) 14:e12676. doi: 10.1111/mcn.12676
123. Yisehak K. Gender responsibility in smallholder mixed crop-livestock production systems of jimma zone, South West Ethiopia. Livest Res Rural Dev. (2008) 20:12–9. Available online at: http://www.lrrd.org/lrrd20/1/yise20011.htm
124. Quisumbing AR, Roy S, Njuki J, Kakuly T, Waithanji E. Can dairy value-chain projects change gender norms in rural Bangladesh? Impacts on assets, gender norms, and time use. Discussion Paper. Washington, DC: IFPRI (2013). doi: 10.2139/ssrn.2373264
125. Walugembe M, Tebug SF, Tapio M, Missohou A, Juga J, Marshall K, et al. Gendered intra-household contributions to low-input dairy in Senegal. Iowa State Univ Anim Ind Rep. (2016) 13. doi: 10.31274/ans_air-180814-208
126. Kumar N, Nguyen PH, Harris J, Harvey D, Rawat R, Ruel MT. What it takes: evidence from a nutrition- and gender-sensitive agriculture intervention in rural Zambia. J Dev Eff. (2018) 10:341–72. doi: 10.1080/19439342.2018.1478874
127. Heckert J, Olney DK, Ruel MT. Is women's empowerment a pathway to improving child nutrition outcomes in a nutrition-sensitive agriculture program? Evidence from a randomized controlled trial in Burkina Faso. Soc Sci Med. (2019) 233:93–102. doi: 10.1016/j.socscimed.2019.05.016
128. Trehan I, Kelly P, Shaikh N, Manary MJ. New insights into environmental enteric dysfunction. Arch Dis Child. (2016) 101:741–4. doi: 10.1136/archdischild-2015-309534
129. Rogawski ET, Guerrant RL. The burden of enteropathy and “subclinical” infections. Pediatr Clin North Am. (2017) 64:815–36. doi: 10.1016/j.pcl.2017.03.003
130. Lynch JP, Kajon AE. Adenovirus: epidemiology, global spread of novel serotypes, and advances in treatment and prevention. Semin Respir Crit Care Med. (2016) 37:586–602. doi: 10.1055/s-0036-1584923
131. Kosek MN, Ahmed T, Bhutta ZA, Caulfield L, Guerrant RL, Houpt E, et al. Causal pathways from enteropathogens to environmental enteropathy: findings from the MAL-ED birth cohort study. EBioMedicine. (2017) 18:109–17. doi: 10.1016/j.ebiom.2017.02.024
132. Bray AE, Ahmed S, Das SK, Khan SH, Chisti MJ, Ahmed T, et al. Viral pathogen-specific clinical and demographic characteristics of children with moderate-to-severe diarrhea in Rural Bangladesh. Am J Trop Med Hyg. (2019) 101:304–9. doi: 10.4269/ajtmh.19-0152
133. Koopmans M. Progress in understanding norovirus epidemiology. Curr Opin Infect Dis. (2008) 21:544–52. doi: 10.1097/QCO.0b013e3283108965
134. Haffejee IE. The epidemiology of rotavirus infections: a global perspective. J Pediatr Gastroenterol Nutr. (1995) 20:275–86. doi: 10.1097/00005176-199504000-00003
135. Fernández-Bravo A, Figueras MJ. An update on the genus aeromonas: taxonomy, epidemiology, and pathogenicity. Microorganisms. (2020) 8:129. doi: 10.3390/microorganisms8010129
136. Kaakoush NO, Castaño-Rodríguez N, Mitchell HM, Man SM. Global epidemiology of campylobacter infection. Clin Microbiol Rev. (2015) 28:687–720. doi: 10.1128/CMR.00006-15
137. Iqbal NT, Syed S, Kabir F, Jamil Z, Akhund T, Qureshi S, et al. Pathobiome driven gut inflammation in pakistani children with environmental enteric dysfunction. PLoS ONE. (2019) 14:e0221095. doi: 10.1371/journal.pone.0221095
138. Amour C, Gratz J, Mduma ER, Svensen E, Rogawski ET, McGrath M, et al. Epidemiology and impact of campylobacter infection in children in 8 low-resource settings: results from the MAL-ED Study. Clin Infect Dis. (2016) 63:11171–9. doi: 10.1093/cid/ciw542
139. Rogawski ET, Liu J, Platts-Mills JA, Kabir F, Lertsethtakarn P, Siguas M, et al. Use of quantitative molecular diagnostic methods to investigate the effect of enteropathogen infections on linear growth in children in low-resource settings: longitudinal analysis of results from the MAL-ED cohort study. Lancet Glob Heal. (2018) 6:e1319–28. doi: 10.1016/S2214-109X(18)30351-6
140. Lee G, Pan W, Peñataro Yori P, Paredes Olortegui M, Tilley D, Gregory M, et al. Symptomatic and asymptomatic campylobacter infections associated with reduced growth in peruvian children. PLoS Negl Trop Dis. (2013) 7:e2036. doi: 10.1371/journal.pntd.0002036
141. Platts-Mills JA, Gratz J, Mduma E, Svensen E, Amour C, Liu J, et al. Association between stool enteropathogen quantity and disease in Tanzanian children using TaqMan array cards: a nested case-control study. Am J Trop Med Hyg. (2014) 90:133–8. doi: 10.4269/ajtmh.13-0439
142. Schnee AE, Haque R, Taniuchi M, Uddin MJ, Alam MM, Liu J, et al. Identification of Etiology-Specific Diarrhea Associated with Linear Growth Faltering in Bangladeshi Infants. Am J Epidemiol. (2018) 187:2210–8. doi: 10.1093/aje/kwy106
143. Wasteson Y. Zoonotic Escherichia coli. Acta Vet Scand Suppl. (2001) 95:79–84. doi: 10.1186/1751-0147-43-S1-S79
144. George CM, Burrowes V, Perin J, Oldja L, Biswas S, Sack D, et al. Enteric infections in young children are associated with environmental enteropathy and impaired growth. Trop Med Int Heal. (2018) 23:26–33. doi: 10.1111/tmi.13002
145. Mondal D, Haque R, Sack RB, Kirkpatrick BD, Petri WA Jr. Attribution of malnutrition to cause-specific diarrheal illness: evidence from a prospective study of preschool children. Am J Trop Med Hyg. (2009) 80:824–6. doi: 10.4269/ajtmh.2009.80.824
146. Opintan JA, Newman MJ, Ayeh-Kumi PF, Affrim R, Gepi-Attee R, Sevilleja JEAD, et al. Pediatric diarrhea in Southern Ghana: etiology and association with intestinal inflammation and malnutrition. Am J Trop Med Hyg. (2010) 83:936–43. doi: 10.4269/ajtmh.2010.09-0792
147. Rogawski ET, Guerrant RL, Havt A, Lima IFN, Medeiros PHQS, Seidman JC, et al. Epidemiology of enteroaggregative Escherichia coli infections and associated outcomes in the MAL-ED birth cohort. PLoS Negl Trop Dis. (2017) 11:e0005798. doi: 10.1371/journal.pntd.0005798
148. Fahim SM, Das S, Gazi MA, Mahfuz M, Ahmed T. Association of intestinal pathogens with faecal markers of environmental enteric dysfunction among slum-dwelling children in the first 2 years of life in Bangladesh. Trop Med Int Heal. (2018) 26:943–52. doi: 10.1111/tmi.13141
149. Brown LM. Helicobacter pylori: epidemiology and routes of transmission. Epidemiol Rev. (2000) 22:283–97. doi: 10.1093/oxfordjournals.epirev.a018040
150. Fahim SM, Das S, Gazi MA, Alam MA, Hasan MM, Hossain MS, et al. Helicobacter pylori infection is associated with fecal biomarkers of environmental enteric dysfunction but not with the nutritional status of children living in Bangladesh. PLoS Negl Trop Dis. (2020) 14:e0008243. doi: 10.1371/journal.pntd.0008243
151. Michael Janda J, Abbott SL, McIver CJ. Plesiomonas shigelloides revisited. Clin Microbiol Rev. (2016) 29:349–74. doi: 10.1128/CMR.00103-15
152. Giannella RA. Salmonella. In: Baron S, editor. Medical Microbiology. Galveston, TX: University of Texas Medical Branch at Galveston (1996).
153. Faherty CS, Lampel KA. Shigella. in Food Microbiology: Fundamentals and Frontiers. Wiley (2019). p. 317–45. doi: 10.1128/9781555819972.ch12
154. Lee G, Olortegui MP, Yori PP, Black RE, Caulfield L, Chavez CB, et al. Effects of shigella-, campylobacter- and ETEC-associated diarrhea on childhood growth. Pediatr Infect Dis J. (2014) 33:1004–9. doi: 10.1097/INF.0000000000000351
155. Bottone EJ. Yersinia enterocolitica: overview and epidemiologic correlates. Microbes Infect. (1999) 1:323–33. doi: 10.1016/S1286-4579(99)80028-8
156. Leitch GJ, He Q. Cryptosporidiosis-an overview. J Biomed Res. (2011) 25:1–16. doi: 10.1016/S1674-8301(11)60001-8
157. Amadi B, Zyambo K, Chandwe K, Besa E, Mulenga C, Mwakamui S, et al. Adaptation of the small intestine to microbial enteropathogens in Zambian children with stunting. Nat Microbiol. (2021) 6:445–54. doi: 10.1038/s41564-020-00849-w
158. Korpe PS, Valencia C, Haque R, Mahfuz M, McGrath M, Houpt E, et al. Epidemiology and risk factors for cryptosporidiosis in children from 8 low-income sites: results from the MAL-ED Study. Clin Infect Dis. (2018) 67:1660–9. doi: 10.1093/cid/ciy355
159. Garzón M, Pereira-da-Silva L, Seixas J, Papoila AL, Alves M. Subclinical enteric parasitic infections and growth faltering in infants in São Tomé, Africa: a birth cohort study. Int J Environ Res Public Health. (2018) 15:688. doi: 10.3390/ijerph15040688
160. Korpe PS, Haque R, Gilchrist C, Valencia C, Niu F, Lu M, et al. Natural history of cryptosporidiosis in a longitudinal study of slum-dwelling Bangladeshi children: association with severe malnutrition. PLoS Negl Trop Dis. (2016) 10:e0004564. doi: 10.1371/journal.pntd.0004564
161. Delahoy MJ, Omore R, Ayers TL, Schilling KA, Blackstock AJ, Ochieng JB, et al. Clinical, environmental, and behavioral characteristics associated with cryptosporidium infection among children with moderate-to-severe diarrhea in rural western Kenya, 2008–2012: the global enteric multicenter study (GEMS). PLoS Negl Trop Dis. (2018) 12:e0006640. doi: 10.1371/journal.pntd.0006640
162. Dillingham RA, Lima AA, Guerrant RL. Cryptosporidiosis: epidemiology and impact. Microbes Infect. (2002) 4:1059–66. doi: 10.1016/S1286-4579(02)01630-1
163. Tumwine JK, Kekitiinwa A, Nabukeera N, Akiyoshi DE, Rich SM, Widmer G, et al. Cryptosporidium parvum in children with diarrhea in mulago hospital, kampala, Uganda. Am J Trop Med Hyg. (2003) 68:710–5. doi: 10.4269/ajtmh.2003.68.710
164. Ackers JP, Mirelman D. Progress in research on entamoeba histolytica pathogenesis. Curr Opin Microbiol. (2006) 9:367–73. doi: 10.1016/j.mib.2006.06.014
165. LaBeaud AD, Nayakwadi Singer M, McKibben M, Mungai P, Muchiri EM, McKibben E, et al. Parasitism in children aged three years and under: relationship between infection and growth in rural coastal Kenya. PLoS Negl Trop Dis. (2015) 9:e0003721. doi: 10.1371/journal.pntd.0003721
166. Fantinatti M, Bello AR, Fernandes O, Da-Cruz AM. Identification of giardia lamblia assemblage e in humans points to a new anthropozoonotic cycle. J Infect Dis. (2016) 214:1256–9. doi: 10.1093/infdis/jiw361
167. Rogawski ET, Bartelt LA, Platts-Mills JA, Seidman JC, Samie A, Havt A, et al. Determinants and impact of Giardia infection in the first 2 years of life in the MAL-ED birth cohort. J Pediatric Infect Dis Soc. (2017) 6:153–60. doi: 10.1093/jpids/piw082
168. Donowitz JR, Alam M, Kabir M, Ma JZ, Nazib F, Platts-Mills JA, et al. A prospective longitudinal cohort to investigate the effects of early life giardiasis on growth and all cause diarrhea. Clin Infect Dis. (2016) 63:792–7. doi: 10.1093/cid/ciw391
169. Goto R, Panter-Brick C, Northrop-Clewes CA, Manahdhar R, Tuladhar NR. Poor intestinal permeability in mildly stunted Nepali children: associations with weaning practices and giardia lamblia infection. Br J Nutr. (2002) 88:141–9. doi: 10.1079/BJN2002599
170. Caron Y, Hong R, Gauthier L, Laillou A, Wieringa FT, Berger J, et al. Stunting, beyond acute diarrhoea: giardia duodenalis, in Cambodia. Nutrients. (2018) 10:1420. doi: 10.3390/nu10101420
171. Lehto KM, Fan YM, Oikarinen S, Nurminen N, Hallamaa L, Juuti R, et al. Presence of Giardia lamblia in stools of six- to 18-month old asymptomatic malawians is associated with children's growth failure. Acta Paediatr. (2019) 108:1833–40. doi: 10.1111/apa.14832
172. Aiemjoy K, Gebresillasie S, Stoller NE, Shiferaw A, Tadesse Z, Chanyalew M, et al. Epidemiology of soil-transmitted helminth and intestinal protozoan infections in preschool-aged children in the Amhara region of Ethiopia. Am J Trop Med Hyg. (2017) 96:866. doi: 10.4269/ajtmh.16-0800
173. Osman KA, Zinsstag J, Tschopp R, Schelling E, Hattendorf J, Umer A, et al. Nutritional status and intestinal parasites among young children from pastoralist communities of the Ethiopian Somali region. Matern Child Nutr. (2020) 16:e12955. doi: 10.1111/mcn.12955
174. Botero-Garcés JH, García-Montoya GM, Grisales-Patiño D, Aguirre-Acevedo DC, Álvarez-Uribe MC. Giardia intestinalis and nutritional status in children participating in the complementary nutrition program, Antioquia, Colombia, May to October 2006. Rev Inst Med Trop São Paulo. (2009) 51:155–62. doi: 10.1590/S0036-46652009000300006
175. Gyorkos TW, Maheu-Giroux M, Casapía M, Joseph SA, Creed-Kanashiro H. Stunting and helminth infection in early preschool-age children in a resource-poor community in the Amazon lowlands of Peru. Trans R Soc Trop Med Hyg. (2011) 105:204–8. doi: 10.1016/j.trstmh.2010.12.003
176. Mehlhorn H editor. Anthroponoses. In: Encyclopedia of Parasitology. Berlin: Springer (2016). p. 89.
177. Hotez PJ, Brooker S, Bethony JM, Bottazzi ME, Loukas A, Xiao S. Hookworm infection. N Engl J Med. (2004) 351:799–807. doi: 10.1056/NEJMra032492
178. Rabaoarisoa CR, Rakotoarison R, Rakotonirainy NH, Mangahasimbola RT, Randrianarisoa AB, Jambou R, et al. The importance of public health, poverty reduction programs and women's empowerment in the reduction of child stunting in rural areas of Moramanga and Morondava, Madagascar. PLoS ONE. (2017) 12:e0186493. doi: 10.1371/journal.pone.0186493
179. World Health Organization. WHO Child Growth Standards: Growth Velocity Based on Weight, Length and Head Circumference: Methods and Development. Geneva: World Health Organization (2009).
180. Cook N, Bridger J, Kendall K, Gomara MI, El-Attar L, Gray J. The zoonotic potential of rotavirus. J Infect. (2004) 48:289–302. doi: 10.1016/j.jinf.2004.01.018
181. Parsons MB, Travis D, Lonsdorf EV, Lipende I, Roellig DMA, Kamenya S, et al. Epidemiology and Molecular Characterization of Cryptosporidium spp. in Humans, Wild Primates, and Domesticated Animals in the Greater Gombe Ecosystem, Tanzania. PLoS Negl Trop Dis. (2015) 9:e0003529. doi: 10.1371/journal.pntd.0003529
182. Hubálek Z. Emerging human infectious diseases: anthroponoses, zoonoses, and sapronoses - volume 9, number 3—march 2003 - emerging infectious diseases journal - CDC. Emerg Infect Dis. (2003) 9:403–4. doi: 10.3201/eid0903.020208
183. Yoseph A, Beyene H. The high prevalence of intestinal parasitic infections is associated with stunting among children aged 6-59 months in boricha woreda, southern Ethiopia: a cross-sectional study. BMC Public Health. (2020) 20:1270. doi: 10.1186/s12889-020-09377-y
184. Mbuya MNN, Humphrey JH. Preventing environmental enteric dysfunction through improved water, sanitation and hygiene: an opportunity for stunting reduction in developing countries. Matern Child Nutr. (2016) 12 (uppl. 1):106–20. doi: 10.1111/mcn.12220
185. Donowitz JR, Haque R, Kirkpatrick BD, Alam M, Lu M, Kabir M, et al. Small intestine bacterial overgrowth and environmental enteropathy in Bangladeshi children. MBio. (2016) 7:e02102–15. doi: 10.1128/mBio.02102-15
186. Vonaesch P, Morien E, Andrianonimiadana L, Sanke H, Mbecko JR, Huus KE, et al. Stunted childhood growth is associated with decompartmentalization of the gastrointestinal tract and overgrowth of oropharyngeal taxa. Proc Natl Acad Sci USA. (2018) 115:E8489–98. doi: 10.1073/pnas.1806573115
187. Perin J, Burrowes V, Almeida M, Ahmed S, Haque R, Parvin T, et al. A retrospective case-control study of the relationship between the gut microbiota, enteropathy, and child growth. Am J Trop Med Hyg. (2020) 103:520–7. doi: 10.4269/ajtmh.19-0761
188. Bartelt LA, Bolick DT, Guerrant RL. Disentangling microbial mediators of malnutrition: modeling environmental enteric dysfunction. CMGH. (2019) 7:692–707. doi: 10.1016/j.jcmgh.2018.12.006
189. Salameh E, Morel FB, Zeilani M, Déchelotte P, Marion-Letellier R. Animal models of undernutrition and enteropathy as tools for assessment of nutritional intervention. Nutrients. (2019) 11:2233. doi: 10.3390/nu11092233
190. Giallourou N, Medlock GL, Bolick DT, Medeiros PH, Ledwaba SE, Kolling GL, et al. A novel mouse model of Campylobacter jejuni enteropathy and diarrhea. PLOS Pathog. (2018) 14:e1007083. doi: 10.1371/journal.ppat.1007083
191. Prendergast AJ, Kelly P. Interactions between intestinal pathogens, enteropathy and malnutrition in developing countries. Curr Opin Infect Dis. (2016) 29:229–36. doi: 10.1097/QCO.0000000000000261
192. Wang J, Li F, Wei H, Lian Z-X, Sun R, Tian Z. Respiratory influenza virus infection induces intestinal immune injury via microbiota-mediated Th17 cell–dependent inflammation. J Exp Med. (2014) 18:e20201776. doi: 10.1084/jem.2014062511242014c
193. Louis-Auguste J, Kelly P. Tropical enteropathies. Curr Gastroenterol Rep. (2017) 19:29. doi: 10.1007/s11894-017-0570-0
194. Gakidou E, Afshin A, Abajobir AA, Abate KH, Abbafati C, Abbas KM, et al. Global, regional, and national comparative risk assessment of 84 behavioural, environmental and occupational, and metabolic risks or clusters of risks, 1990–2016: a systematic analysis for the global burden of disease study 2016. Lancet. (2017) 390:1345–422. doi: 10.1016/S0140-6736(17)32366-8
195. Nitiema LW, Nordgren J, Ouermi D, Dianou D, Traore AS, Svensson L, et al. Burden of rotavirus and other enteropathogens among children with diarrhea in Burkina Faso. Int J Infect Dis. (2011) 15:e646–52. doi: 10.1016/j.ijid.2011.05.009
196. Qamar FN, Nisar MI, Quadri F, Shakoor S, Sow SO, Nasrin D, et al. Aeromonas-associated diarrhea in children under 5 years: the gems experience. Am J Trop Med Hyg. (2016) 95:774–80. doi: 10.4269/ajtmh.16-0321
197. Sarkar R, Kattula D, Francis MR, Ajjampur SSR, Prabakaran AD, Jayavelu N, et al. Risk factors for cryptosporidiosis among children in a semi urban slum in southern India: a nested case-control study. Am J Trop Med Hyg. (2014) 91:1128–37. doi: 10.4269/ajtmh.14-0304
198. Sarkar R, Ajjampur SSR, Prabakaran AD, Geetha JC, Sowmyanarayanan TV, Kane A, et al. Cryptosporidiosis among children in an endemic semiurban community in southern India: does a protected drinking water source decrease infection? Clin Infect Dis. (2013) 57:398–406. doi: 10.1093/cid/cit288
199. Tellevik MG, Moyo SJ, Blomberg B, Hjøllo T, Maselle SY, Langeland N, et al. Prevalence of cryptosporidium parvum/hominis, entamoeba histolytica and giardia lamblia among young children with and without diarrhea in dar es salaam, Tanzania. PLoS Negl Trop Dis. (2015) 9:e0004125. doi: 10.1371/journal.pntd.0004125
200. Budge S, Barnett M, Hutchings P, Parker A, Tyrrel S, Hassard F, et al. Risk factors and transmission pathways associated with infant Campylobacter spp. Prevalence and malnutrition: A formative study in rural Ethiopia. PLoS ONE. (2020) 15:e0232541. doi: 10.1371/journal.pone.0232541
201. Koyuncu A, Simuyandi M, Bosomprah S, Chilengi R. Nutritional status, environmental enteric dysfunction, and prevalence of rotavirus diarrhoea among children in Zambia. PLoS ONE. (2020) 15:e0240258. doi: 10.1371/journal.pone.0240258
202. Dang-Xuan S, Nguyen-Viet H, Pham-Duc P, Unger F, Tran-Thi N, Grace D, et al. Risk factors associated with Salmonella spp. prevalence along smallholder pig value chains in Vietnam. Int J Food Microbiol. (2019) 290:105–15. doi: 10.1016/j.ijfoodmicro.2018.09.030
203. Yang Y, Zhou YB, Xiao PL, Shi Y, Chen Y, Liang S, et al. Prevalence of and risk factors associated with cryptosporidium infection in an underdeveloped rural community of Southwest China. Infect Dis Poverty. (2017) 6:2. doi: 10.1186/s40249-016-0223-9
204. Zambrano LD, Levy K, Menezes NP, Freeman MC. Human diarrhea infections associated with domestic animal husbandry: a systematic review and meta-analysis. Trans R Soc Trop Med Hyg. (2014) 108:313–25. doi: 10.1093/trstmh/tru056
205. Hassan KE, Mansour A, Shaheen H, Amine M, Riddle MS, Young SYN, et al. The impact of household hygiene on the risk of bacterial diarrhea among egyptian children in rural areas, 2004-2007. J Infect Dev Ctries. (2014) 8:1541–51. doi: 10.3855/jidc.4539
206. Chard AN, Levy K, Baker KK, Tsai K, Chang HH, Thongpaseuth V, et al. Environmental and spatial determinants of enteric pathogen infection in rural lao people's democratic republic: a cross-sectional study. PLoS Negl Trop Dis. (2020) 14:e0008180. doi: 10.1371/journal.pntd.0008180
207. Li XX, Chen JX, Wang LX, Tian LG, Zhang YP, Dong SP, et al. Prevalence and risk factors of intestinal protozoan and helminth infections among pulmonary tuberculosis patients without HIV infection in a rural county in P. R. China. Acta Trop. (2015) 149:19–26. doi: 10.1016/j.actatropica.2015.05.001
208. Feleke BE, Beyene MB, Feleke TE, Jember TH, Abera B. Intestinal parasitic infection among household contacts of primary cases, a comparative cross-sectional study. PLoS ONE. (2019) 14:e0221190. doi: 10.1101/723494
209. Forson AO, Arthur I, Olu-Taiwo M, Glover KK, Pappoe-Ashong PJ, Ayeh-Kumi PF. Intestinal parasitic infections and risk factors: a cross-sectional survey of some school children in a suburb in Accra, Ghana. BMC Res Notes. (2017) 10:485. doi: 10.1186/s13104-017-2802-7
210. Sackey ME, Weigel MM, Armijos RX. Predictors and nutritional consequences of intestinal parasitic infections in rural ecuadorian children. J Trop Pediatr. (2003) 49:17–23. doi: 10.1093/tropej/49.1.17
211. Periago MV, García R, Astudillo OG, Cabrera M, Abril MC. Prevalence of intestinal parasites and the absence of soil-transmitted helminths in Añatuya, Santiago del Estero, Argentina. Parasit Vect. (2018) 11:638. doi: 10.1186/s13071-018-3232-7
212. Macchioni F, Segundo H, Totino V, Gabrielli S, Rojas P, Roselli M, et al. Intestinal parasitic infections and associated epidemiological drivers in two rural communities of the Bolivian Chaco. J Infect Dev Ctries. (2016) 10:1012–19. doi: 10.3855/jidc.7657
213. Lowenstein C, Vasco K, Sarzosa S, Salinas L, Torres A, Perry MJ, et al. Determinants of childhood zoonotic enteric infections in a semirural community of Quito, Ecuador. Am J Trop Med Hyg. (2020) 102:1269–78. doi: 10.4269/ajtmh.19-0690
214. Beshearse E, Bruce BB, Nane GF, Cooke RM, Aspinall W, Hald T, et al. Attribution of illnesses transmitted by food and water to comprehensive transmission pathways using structured expert judgment, United States. Emerg Infect Dis. (2021) 27:182–95. doi: 10.3201/eid2701.200316
215. US Centers for Disease Control Prevention. The Social-Ecological Model: A Framework for Prevention Violence Prevention Injury Center. CDC. Available online at: https://www.cdc.gov/violenceprevention/about/social-ecologicalmodel.html (accessed July 7, 2021).
216. WHO UNICEF. Drinking Water, 2019. WHO (2017). Available online at: https://washdata.org/monitoring/drinking-water
217. WHO UNICEF. Sanitation, 2019. WHO (2017). Available online at: https://washdata.org/monitoring/sanitation
218. WHO UNICEF. Hygiene, 2019. WHO (2017). Available online at: https://washdata.org/monitoring/hygiene
219. Benwic A, Kim E, Khema C, Phanna C, Sophary P, Cantwell RE. Factors associated with post-treatment E. coli contamination in households practising water treatment: a study of rural Cambodia. Int J Environ Health Res. (2018) 28:178–91. doi: 10.1080/09603123.2018.1453055
220. Gelli A, Headey D, Becquey E, Ganaba R, Huybregts L, Pedehombga A, et al. Poultry husbandry, water, sanitation, and hygiene practices, and child anthropometry in rural Burkina Faso. Matern Child Nutr. (2019) 15:e12818. doi: 10.1111/mcn.12818
221. Gharieb RMA, Merwad AMA, Saleh AA, Abd El-Ghany AM. Molecular screening and genotyping of cryptosporidium species in household dogs and in-contact children in Egypt: Risk factor analysis and zoonotic importance. Vector Borne Zoo Dis. (2018) 18:424–32. doi: 10.1089/vbz.2017.2254
222. Helmy YA, von Samson-Himmelstjerna G, Nöckler K, Zessin K-H. Frequencies and spatial distributions of cryptosporidium in livestock animals and children in the ismailia province of Egypt. Epidemiol Infect. (2015) 143:1208–18. doi: 10.1017/S0950268814001824
223. Osbjer K, Boqvist S, Sokerya S, Chheng K, San S, Davun H, et al. Risk factors associated with campylobacter detected by PCR in humans and animals in rural Cambodia. Epidemiol Infect. (2016) 144:2979–88. doi: 10.1017/S095026881600114X
224. Tu LTP, Hoang NVM, Cuong NV, Campbell J, Bryant JE, Hoa NT, et al. High levels of contamination and antimicrobial-resistant non-typhoidal Salmonella serovars on pig and poultry farms in the Mekong Delta of Vietnam. Epidemiol Infect. (2015) 143:3074–86. doi: 10.1017/S0950268815000102
225. Yvonne Domingo CJ, Ador Dionision RD, Lanzanida GC, Mae Corales RI. Human and Caprine Cryptosporidiosis Among Smallhold Farms in Aurora Province, Philippines. (2014). Available online at: https://www.pjvas.org/index.php/pjvas/article/view/53 (accessed June 11, 2021).
226. Pires SM, Evers EG, Van Pelt W, Ayers T, Scallan E, Angulo FJ, et al. Attributing the human disease burden of foodborne infections to specific sources. Foodborne Pathog Dis. (2009) 6:417–24. doi: 10.1089/fpd.2008.0208
227. Harwood VJ, Staley C, Badgley BD, Borges K, Korajkic A. Microbial source tracking markers for detection of fecal contamination in environmental waters: relationships between pathogens and human health outcomes. FEMS Microbiol Rev. (2014) 38:1–40. doi: 10.1111/1574-6976.12031
228. Kovac J, Stessl B, CadeŽ N, Gruntar I, Cimerman M, Stingl K, Lušicky M, et al. Population structure and attribution of human clinical Campylobacter jejuni isolates from central Europe to livestock and environmental sources. Zoonoses Public Health. (2018) 65:51–8. doi: 10.1111/zph.12366
229. Scott TM, Rose JB, Jenkins TM, Farrah SR, Lukasik J. Microbial source tracking: current methodology and future directions. Appl Environ Microbiol. (2002) 68:5796–803. doi: 10.1128/AEM.68.12.5796-5803.2002
230. Mather AE, Vaughan TG, French NP. Molecular approaches to understanding transmission and source attribution in nontyphoidal salmonella and their application in Africa. Clin Infect Dis. (2015) 61:S259–65. doi: 10.1093/cid/civ727
231. Dione MM, Ikumapayi UN, Saha D, Mohammed NI, Geerts S, Ieven M, et al. Clonal differences between non-typhoidal salmonella (NTS) recovered from children and animals living in close contact in the Gambia. PLoS Negl Trop Dis. (2011) 5:e0001148. doi: 10.1371/journal.pntd.0001148
232. Kariuki S, Revathi G, Gakuya F, Yamo V, Muyodi J, Hart CA. Lack of clonal relationship between non-typhi salmonella strain types from humans and those isolated from animals living in close contact. FEMS Immunol Med Microbiol. (2002) 33:165–71. doi: 10.1111/j.1574-695X.2002.tb00587.x
233. Kariuki S, Revathi G, Kariuki N, Kiiru J, Mwituria J, Muyodi J, et al. Invasive multidrug-resistant non-typhoidal salmonella infections in Africa: zoonotic or anthroponotic transmission? J Med Microbiol. (2006) 55:585–91. doi: 10.1099/jmm.0.46375-0
234. Smith AM, Ismail H, Henton MM, Keddy KH. Similarities between salmonella enteritidis isolated from humans and captive wild animals in South Africa. J Infect Dev Ctries. (2014) 8:1615–9. doi: 10.3855/jidc.5393
235. Kagambèga A, Lienemann T, Aulu L, Traoré AS, Barro N, Siitonen A, et al. Prevalence and characterization of Salmonella enterica from the feces of cattle, poultry, swine and hedgehogs in burkina faso and their comparison to human salmonella isolates. BMC Microbiol. (2013) 13:253. doi: 10.1186/1471-2180-13-253
236. Knappett PSK, Escamilla V, Layton A, McKay LD, Emch M, Williams DE, et al. Impact of population and latrines on fecal contamination of ponds in rural Bangladesh. Sci Total Environ. (2011) 409:3174–82. doi: 10.1016/j.scitotenv.2011.04.043
237. Harada H, Fujimori Y, Gomi R, Ahsan MN, Fujii S, Sakai A, et al. Pathotyping of Escherichia coli isolated from community toilet wastewater and stored drinking water in a slum in Bangladesh. Lett Appl Microbiol. (2018) 66:542–8. doi: 10.1111/lam.12878
238. Harris AR, Islam MA, Unicomb L, Boehm AB, Luby S, Davis J, et al. Fecal contamination on produce from wholesale and retail food markets in Dhaka, Bangladesh. Am J Trop Med Hyg. (2018) 98:287–94. doi: 10.4269/ajtmh.17-0255
239. Fuhrmeister ER, Ercumen A, Pickering AJ, Jeanis KM, Ahmed M, Brown S, et al. Predictors of enteric pathogens in the domestic environment from human and animal sources in rural Bangladesh. Environ Sci Technol. (2019) 53:10023–33. doi: 10.1021/acs.est.8b07192
240. Schriewer A, Odagiri M, Wuertz S, Misra PR, Panigrahi P, Clasen T, et al. Human and animal fecal contamination of community water sources, stored drinking water and hands in rural India measured with validated microbial source tracking assays. Am J Trop Med Hyg. (2015) 93:509–16. doi: 10.4269/ajtmh.14-0824
241. Odagiri M, Schriewer A, Daniels ME, Wuertz S, Smith WA, Clasen T, et al. Human fecal and pathogen exposure pathways in rural Indian villages and the effect of increased latrine coverage. Water Res. (2016) 100:232–44. doi: 10.1016/j.watres.2016.05.015
242. Holcomb DA, Knee J, Sumner T, Adriano Z, de Bruijn E, Nalá R, et al. Human fecal contamination of water, soil, and surfaces in households sharing poor-quality sanitation facilities in Maputo, Mozambique. Int J Hyg Environ Health. (2020) 226:113496. doi: 10.1016/j.ijheh.2020.113496
243. Schiaffino F, Trigoso DR, Colston JM, Olortegui MP, Shapiama Lopez WV, Garcia Bardales PF, et al. Associations among household animal ownership, infrastructure, and hygiene characteristics with source attribution of household fecal contamination in peri-urban communities of Iquitos, Peru. Am J Trop Med Hyg. (2021) 104:372–81. doi: 10.4269/ajtmh.20-0810
244. Raj SJ, Wang Y, Yakubu H, Robb K, Siesel C, Green J, et al. The sanipath exposure assessment tool: a quantitative approach for assessing exposure to fecal contamination through multiple pathways in low resource urban settlements. PLoS ONE. (2020) 15:e0234364. doi: 10.1371/journal.pone.0234364
245. Wang Y, Moe CL, Null C, Raj SJ, Baker KK, Robb KA, et al. Multipathway quantitative assessment of exposure to fecal contamination for young children in low-income urban environments in Accra, Ghana: the Sanipath analytical approach. Am J Trop Med Hyg. (2017) 97:1009–19. doi: 10.4269/ajtmh.16-0408
246. Teunis PFM, Reese HE, Null C, Yakubu H, Moe CL. Quantifying contact with the environment: behaviors of young children in Accra, Ghana. Am J Trop Med Hyg. (2016) 94:920–31. doi: 10.4269/ajtmh.15-0417
247. Yongabi KA, Harris PL, Lewis DM. Poultry faeces management with a simple low cost plastic digester. Afri J Biotechnol. (2009) 8:1560–6. Available online at: https://academicjournals.org/article/article1380011068_Yongabi%20et%20al.pdf
248. Huong LQ, Forslund A, Madsen H, Dalsgaard A. Survival of Salmonella spp. and fecal indicator bacteria in Vietnamese biogas digesters receiving pig slurry. Int J Hyg Environ Health. (2014) 217:785–95. doi: 10.1016/j.ijheh.2014.04.004
249. Nakamya J, Tumuhairwe JB, Sabiiti EN, Strachan NJC, Avery LM, Smith J. Influence of biogas digesters on faecal indicator organisms in digestate and around homesteads in Ethiopia. Biomass Bioenergy. (2020) 142:105746. doi: 10.1016/j.biombioe.2020.105746
250. Dang STT, Van Truong D, Madsen H, Dalsgaard A. Survival of faecal indicator bacteria in treated pig manure stored in clay-covered heaps in Vietnam. Vet Microbiol. (2011) 152:374–8. doi: 10.1016/j.vetmic.2011.05.004
251. Harvey SA, Winch PJ, Leontsini E, Torres Gayoso C, López Romero S, Gilman RH, et al. Domestic poultry-raising practices in a peruvian shantytown: implications for control of Campylobacter jejuni-associated diarrhea. Acta Trop. (2003) 86:41–54. doi: 10.1016/S0001-706X(03)00006-8
252. Oberhelman RA, Gilman RH, Sheen P, Cordova J, Zimic M, Cabrera L, et al. An intervention-control study of corralling of free-ranging chickens to control campylobacter infections among children in a peruvian periurban shantytown. Am J Trop Med Hyg. (2006) 74:1054–9. doi: 10.4269/ajtmh.2006.74.1054
253. Martinez L, Collazo G, Cabrera L, Bernabe-Ortiz A, Ramos-Peña Y, Oberhelman R. Short report: free-ranging chickens in households in a periurban shantytown in peru - attitudes and practices 10 years after a community-based intervention project. Am J Trop Med Hyg. (2013) 89:229–31. doi: 10.4269/ajtmh.12-0760
254. Kuhl J, Bisimwa L, Thomas ED, Williams C, Ntakirutimana J, Coglianese N, et al. Formative research for the development of baby water, sanitation, and hygiene interventions for young children in the democratic republic of the congo (REDUCE program). BMC Public Health. (2021) 21:427. doi: 10.1186/s12889-021-10246-5
255. McQuade ETR, Platts-Mills JA, Gratz J, Zhang J, Moulton LH, Mutasa K, et al. Impact of water quality, sanitation, handwashing, and nutritional interventions on enteric infections in rural zimbabwe: the sanitation hygiene infant nutrition efficacy (SHINE) trial. J Infect Dis. (2020) 221:1379–86. doi: 10.1093/infdis/jiz179
256. Reid B, Seu R, Orgle J, Roy K, Pongolani C, Chileshe M, et al. A community-designed play-yard intervention to prevent microbial ingestion: a baby water, sanitation, and hygiene pilot study in rural Zambia. Am J Trop Med Hyg. (2018) 99:513–25. doi: 10.4269/ajtmh.17-0780
257. Owusu-Kwarteng J, Akabanda F, Agyei D, Jespersen L. Microbial safety of milk production and fermented dairy products in africa. Microorganisms. (2020) 8:752. doi: 10.3390/microorganisms8050752
258. Bereda A, Yesuf Kurtu M, Yilma Z. Handling, processing and utilization of milk and milk products in Ethiopia: a review. World J Dairy Food Sci. (2014) 9:105–12. doi: 10.5829/idosi.wjdfs.2014.9.2.8522
259. Wanjala NW, Matofari JW, Nduko JM. Antimicrobial effect of smoking milk handling containers' inner surfaces as a preservation method in pastoral systems in Kenya. Pastoralism. (2016) 6:17. doi: 10.1186/s13570-016-0064-y
260. Amenu K, Wieland B, Szonyi B, Grace D. Milk handling practices and consumption behavior among Borana pastoralists in southern Ethiopia. J Health Popul Nutr. (2019) 38:6. doi: 10.1186/s41043-019-0163-7
261. Ma L, Zhang G, Swaminathan B, Doyle M, Bowen A. Efficacy of protocols for cleaning and disinfecting infant feeding bottles in less developed communities. Am J Trop Med Hyg. (2009) 81:132–9. doi: 10.4269/ajtmh.2009.81.132
262. Rothstein JD, Mendoza AL, Cabrera LZ, Pachas J, Calderón M, Pajuelo MJ, et al. Household contamination of baby bottles and opportunities to improve bottle hygiene in Peri-Urban Lima, Peru. Am J Trop Med Hyg. (2019) 100:988–97. doi: 10.4269/ajtmh.18-0301
263. Freeman MC, Ellis AS, Ogutu EA, Caruso BA, Linabarger M, Micek K, et al. Impact of a demand-side integrated WASH and nutrition community-based care group intervention on behavioural change: a randomised controlled trial in western Kenya. BMJ Glob Heal. (2020) 5:e002806. doi: 10.1136/bmjgh-2020-002806
264. Manjang B, Hemming K, Bradley C, Ensink J, Martin JT, Sowe J, et al. Promoting hygienic weaning food handling practices through a community-based programme: intervention implementation and baseline characteristics for a cluster randomised controlled trial in rural Gambia. BMJ Open. (2018) 8:e017573. doi: 10.1136/bmjopen-2017-017573
265. Gautam OP, Schmidt WP, Cairncross S, Cavill S, Curtis V. Trial of a novel intervention to improve multiple food hygiene behaviors in Nepal. Am J Trop Med Hyg. (2017) 96:1415–26. doi: 10.4269/ajtmh.16-0526
266. Geresomo NC, Mbuthia EK, Matofari JW, Mwangwela AM. Targeting caregivers with context specific behavior change training increased uptake of recommended hygiene practices during food preparation and complementary feeding in Dedza district of central Malawi. Ecol Food Nutr. (2018) 57:301–13. doi: 10.1080/03670244.2018.1492379
267. Chidziwisano K, Slekiene J, Mosler HJ, Morse T. Improving complementary food hygiene behaviors using the risk, attitude, norms, ability, and self-regulation approach in rural Malawi. Am J Trop Med Hyg. (2020) 102:1104–15. doi: 10.4269/ajtmh.19-0528
268. Wodnik BK, Freeman MC, Ellis AS, Ogutu EA, Girard AW, Caruso BA. Development and application of novel caregiver hygiene behavior measures relating to food preparation, handwashing, and play environments in rural Kenya. Int J Environ Res Public Health. (2018) 15:1994. doi: 10.3390/ijerph15091994
269. Rahman MJ, Nizame FA, Nuruzzaman M, Akand F, Islam MA, Parvez SM, et al. Toward a scalable and sustainable intervention for complementary food safety. Food Nutr Bull. (2016) 37:186–201. doi: 10.1177/0379572116631641
270. Touré O, Coulibaly S, Arby A, Maiga F, Cairncross S. Improving microbiological food safety in peri-urban Mali; an experimental study. Food Control. (2011) 22:1565–72. doi: 10.1016/j.foodcont.2011.03.012
271. Touré O, Coulibaly S, Arby A, Maiga F, Cairncross S. Piloting an intervention to improve microbiological food safety in Peri-Urban Mali. Int J Hyg Environ Health. (2013) 216:138–45. doi: 10.1016/j.ijheh.2012.02.003
272. Manaseki-Holland S, Manjang B, Hemming K, Martin JT, Bradley C, Jackson L, et al. Effects on childhood infections of promoting safe and hygienic complementary-food handling practices through a community-based programme: a cluster randomised controlled trial in a rural area of the Gambia. PLoS Med. (2021) 18:e1003260. doi: 10.1371/journal.pmed.1003260
273. Ejemot-Nwadiaro RI, Ehiri JE, Arikpo D, Meremikwu MM, Critchley JA. Hand-washing promotion for preventing diarrhoea. Cochrane Database Syst Rev. (2021) 12:CD004265. doi: 10.1002/14651858.CD004265.pub4
274. Clasen TF, Alexander KT, Sinclair D, Boisson S, Peletz R, Chang HH, et al. Interventions to improve water quality for preventing diarrhoea. Cochrane Database Syst Rev. (2015) 2015:CD004794. doi: 10.1002/14651858.CD004794.pub3
275. Amenu K, Tiki W, Amdhun K, Desta H, Agga G, Wieland B, et al. Interventions towards improving the microbiological quality of traditional yogurt in Borana pastoral communities, Ethiopia. In: American Dairy Science Association Annual Meeting (Cincinnati, OH). Available online at: https://m.adsa.org/2019/abs/t/77836
276. Claeys WL, Cardoen S, Daube G, De Block J, Dewettinck K, Dierick K, et al. Raw or heated cow milk consumption: review of risks and benefits. Food Control. (2013) 31:251–62. doi: 10.1016/j.foodcont.2012.09.035
277. Tadesse G, Ephraim E, Ashenafi M. Assessment of the antimicrobial activity of lactic acid bacteria isolated from borde and shamita, traditional Ethiopian fermented beverages, on some food- borne pathogens and effect of growth medium on the inhibitory activity. Internet J Food Saf. (2005) 13–20. Available online at: http://www.foodhaccp.com/internetjournal/ijfsv5-3.pdf
278. Ampaire A, Rothschild MF. Pigs, goats and chickens for rural development: small holder farmer's experience in Uganda. Livest Res Rural Dev. (2010) 22:102. Available online at: http://www.lrrd.org/lrrd22/6/ampa22102.htm
279. Randolph TF, Schelling E, Grace D, Nicholson CF, Leroy JL, Cole DC, et al. Invited review: role of livestock in human nutrition and health for poverty reduction in developing countries. J Anim Sci. (2007) 85:2788–800. doi: 10.2527/jas.2007-0467
280. Turk JM. Poverty, livestock and food security in developing countries. CAB Rev. (2013) 8:1–8. doi: 10.1079/PAVSNNR20138033
281. Nyantakyi-Frimpong H, Colecraft EK, Awuah RB, Adjorlolo LK, Wilson ML, Jones AD. Leveraging smallholder livestock production to reduce anemia: a qualitative study of three agroecological zones in Ghana. Soc Sci Med. (2018) 212:191–202. doi: 10.1016/j.socscimed.2018.07.028
282. Wong JT, de Bruyn J, Bagnol B, Grieve H, Li M, Pym R, et al. Small-scale poultry and food security in resource-poor settings: A review. Glob Food Sec. (2017) 15:43–52. doi: 10.1016/j.gfs.2017.04.003
283. Majekodunmi AO, Fajinmi A, Dongkum C, Shaw APM, Welburn SC. Pastoral livelihoods of the fulani on the jos plateau of Nigeria. Pastor Res Policy Pract. (2014) 4:20. doi: 10.1186/s13570-014-0020-7
284. Smith J, Sones K, Grace D, MacMillan S, Tarawali S, Herrero M. Beyond milk, meat, and eggs: role of livestock in food and nutrition security. Anim Front. (2013) 3:6–13. doi: 10.2527/af.2013-0002
285. Chenyambuga SW, Nalaila SM, Mbaga SH. Assessment of uses, special qualities and management aspects of iringa red zebu cattle in Tanzania. Livest Res Rural Dev. (2008) 20:20017. Available online at: http://www.lrrd.org/lrrd20/2/chen20017.htm
286. Bekele M, Abebe H, Kitron U. Energy and nutrient intake and associated factors among pastoral children in southern Ethiopia. Food Nutr Bull. (2020) 41:446–458. doi: 10.1177/0379572120953042
287. Hawkes C, Ruel MT. From Agriculture to Nutrition: Pathways, Synergies and Outcomes. Washington, DC: World Bank Group (2008).
288. Herrero M, Grace D, Njuki J, Johnson N, Enahoro D, Silvestri S, et al. The roles of livestock in developing countries. Animal. (2013) 7:3–18. doi: 10.1017/S1751731112001954
289. Smith L, Ramakrishnan U, Ndiaye A, Haddad L, Martorell R. The Importance of Women's Status for Child Nutrition in Developing Countries. Baltimore:, MD John Hopkins University Press (2003). doi: 10.1177/156482650302400309
290. Price M, Galiè A, Marshall J, Agu N. Elucidating linkages between women's empowerment in livestock and nutrition: a qualitative study. Dev Pract. (2018) 28:510–24. doi: 10.1080/09614524.2018.1451491
291. McOmber C, McNamara K, McKune S. Community concept drawing: a participatory visual method for incorporating local knowledge into conceptualization. Field Methods. (2021) 1–18. doi: 10.1177/1525822X211014736
292. Clement F, Buisson M-C, Leder S, Balasubramanya S, Saikia P, Bastakoti R, et al. From women's empowerment to food security: revisiting global discourses through a cross-country analysis. Glob Food Sec. (2019) 23:160–72. doi: 10.1016/j.gfs.2019.05.003
293. Guerrant RL, DeBoer MD, Moore SR, Scharf RJ, Lima AA. The impoverished gut–a triple burden of diarrhoea, stunting and chronic disease. Nat Rev Gastroenterol Hepatol. (2013) 10:220–9. doi: 10.1038/nrgastro.2012.239
294. Headey D, Nguyen P, Kim S, Rawat R, Ruel M, Menon P. Is exposure to animal feces harmful to child nutrition and health outcomes? A multicountry observational analysis. Am J Trop Med Hyg. (2017) 96:961–9. doi: 10.4269/ajtmh.16-0270
295. Headey D, Hirvonen K. Is exposure to poultry harmful to child nutrition? An observational analysis for rural Ethiopia. PLoS ONE. (2016) 11:e0160590. doi: 10.1371/journal.pone.0160590
296. Conan A, O'Reilly CE, Ogola E, Ochieng JB, Blackstock AJ, Omore R, et al. Animal-related factors associated with moderate-to-severe diarrhea in children younger than five years in western Kenya: a matched case-control study. PLoS Negl Trop Dis. (2017) 11:e0005795. doi: 10.1371/journal.pntd.0005795
297. James BS, Shetty RS, Kamath A, Shetty A. Household cooking fuel use and its health effects among rural women in southern India-A cross-sectional study. PLoS ONE. (2020) 15:e0231757. doi: 10.1371/journal.pone.0231757
298. Smith KR, Samet JM, Romieu I, Bruce N. Indoor air pollution in developing countries and acute lower respiratory infections in children. Thorax. (2000) 55:518–32. doi: 10.1136/thorax.55.6.518
299. Ezzati M, Kammen DM. The health impacts of exposure to indoor air pollution from solid fuels in developing countries: knowledge, gaps, and data needs. Environ Health Perspect. (2002) 110:1057–68. doi: 10.1289/ehp.021101057
300. Ndahetuye JB, Twambazimana J, Nyman AK, Karege C, Tukei M, Ongol MP, et al. A cross sectional study of prevalence and risk factors associated with subclinical mastitis and intramammary infections, in dairy herds linked to milk collection centers in Rwanda. Prev Vet Med. (2020) 179:105007. doi: 10.1016/j.prevetmed.2020.105007
301. Pell AN. Manure and microbes: public and animal health problem? J Dairy Sci. (1997) 80:2673–81. doi: 10.3168/jds.S0022-0302(97)76227-1
302. Callaway TR, Callaway TR, Anderson RC, Edrington TS, Genovese KJ, Harvey RB, et al. Recent pre-harvest supplementation strategies to reduce carriage and shedding of zoonotic enteric bacterial pathogens in food animals. Anim Heal Res Rev. (2004) 5:35–47. doi: 10.1079/AHR200462
303. Swart AN, Tomasi M, Kretzschmar M, Havelaar AH, Diekmann O. The protective effects of temporary immunity under imposed infection pressure. Epidemics. (2012) 4:43–7. doi: 10.1016/j.epidem.2011.12.002
304. Havelaar AH, Van Pelt W, Ang CW, Wagenaar JA, Van Putten JPM, Gross U, et al. Immunity to campylobacter: Its role in risk assessment and epidemiology campylobacter immunity in epidemiology and risk assessment A. H. Havelaar et al. Crit Rev Microbiol. (2009) 35:1–22. doi: 10.1080/10408410802636017
305. Lambrecht NJ, Wilson ML, Jones AD. Assessing the impact of animal husbandry and capture on anemia among women and children in low- and middle-income countries: a systematic review. Adv Nutr. (2019) 10:331–44. doi: 10.1093/advances/nmy080
Keywords: child nutrition, livestock, gut health, enteric pathogens, risk factors, animal feces, WaSH (water sanitation and hygiene), environmental enteric dysfunction (EED)
Citation: Chen D, Mechlowitz K, Li X, Schaefer N, Havelaar AH and McKune SL (2021) Benefits and Risks of Smallholder Livestock Production on Child Nutrition in Low- and Middle-Income Countries. Front. Nutr. 8:751686. doi: 10.3389/fnut.2021.751686
Received: 01 August 2021; Accepted: 29 September 2021;
Published: 27 October 2021.
Edited by:
Sara Monteiro Pires, Technical University of Denmark, DenmarkReviewed by:
Luis Paulo Vidaletti, International Center for Equity in Health, BrazilCopyright © 2021 Chen, Mechlowitz, Li, Schaefer, Havelaar and McKune. This is an open-access article distributed under the terms of the Creative Commons Attribution License (CC BY). The use, distribution or reproduction in other forums is permitted, provided the original author(s) and the copyright owner(s) are credited and that the original publication in this journal is cited, in accordance with accepted academic practice. No use, distribution or reproduction is permitted which does not comply with these terms.
*Correspondence: Sarah L. McKune, c21ja3VuZUB1ZmwuZWR1
†These authors have contributed equally to this work and share first authorship
‡These authors share senior authorship
Disclaimer: All claims expressed in this article are solely those of the authors and do not necessarily represent those of their affiliated organizations, or those of the publisher, the editors and the reviewers. Any product that may be evaluated in this article or claim that may be made by its manufacturer is not guaranteed or endorsed by the publisher.
Research integrity at Frontiers
Learn more about the work of our research integrity team to safeguard the quality of each article we publish.