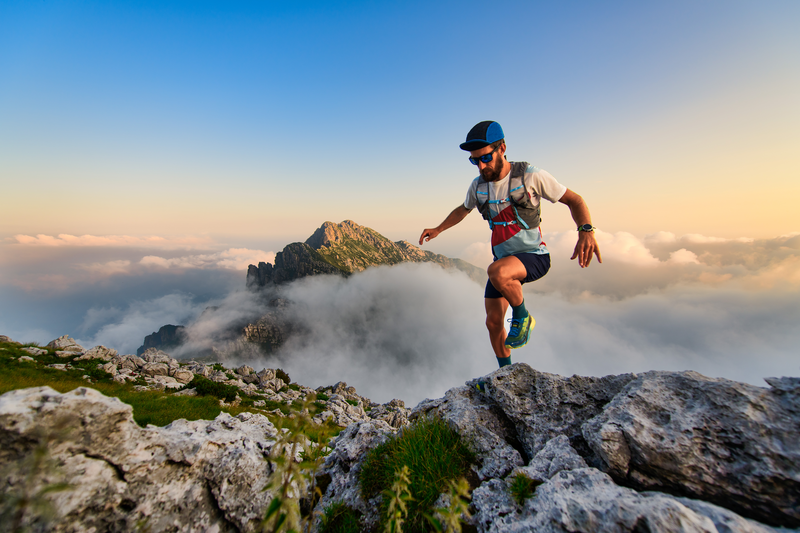
94% of researchers rate our articles as excellent or good
Learn more about the work of our research integrity team to safeguard the quality of each article we publish.
Find out more
ORIGINAL RESEARCH article
Front. Nutr. , 16 September 2021
Sec. Nutrigenomics
Volume 8 - 2021 | https://doi.org/10.3389/fnut.2021.751388
This article is part of the Research Topic The Epigenetic Mechanism of Intestinal Immune Response and Its Nutritional Regulation View all 8 articles
Accumulating evidence shows that the γ-amino butyric acid (GABA)ergic system affects the functions of different organs, and liver is one of the most sex-dimorphic organs in animals. However, whether and how the GABAergic system influences liver function in a sex-specific manner at the intrinsic molecular level remains elusive. In this study, firstly, we find that the levels of GABA are significantly increased in the livers of female mice with GABA transporter (GAT)-2 deficiency (KO) whereas it only slightly increased in male GAT-2 KO mice. Apart from the amino acid profiles, the expressions of toll-like receptors (TLRs) also differ in the livers of female and male KO mice. Moreover, RNA-seq results show 2,227 differentially expressed genes (DEGs) in which 1,030 are upregulated whereas 1,197 that are downregulated in the livers of female KO mice. Notably, oxidative phosphorylation, non-alcoholic fatty liver disease, Huntington's disease, and peroxisome proliferator-activated receptor (PPAR) signaling pathways are highly enriched by GAT-2 deficiency, indicating that these pathways probably meditate the effects of GAT-2 on female liver functions, on the other hand, only 1,233 DEGs, including 474 are upregulated and 759 are downregulated in the livers of male KO mice. Interestingly, retinol metabolism, PPAR signaling pathway, and tuberculosis pathways are substantially enriched by GAT-2 deficiency, suggesting that these pathways may be responsible for the effects of GAT-2 on male liver functions. Collectively, our results reveal the sex-dimorphic effects of GAT-2 in guiding liver functions, and we propose that targeting the GABAergic system (e.g., GATs) in a sex-specific manner could provide previously unidentified therapeutic opportunities for liver diseases.
The γ-aminobutyric acid transporter (GAT) family, which includes GAT-1, GAT-2, GAT-3, and BGT-1, is the important regulator in controlling the intracellular and extracellular γ-amino butyric acid (GABA) concentration (1, 2). GAT-1 or GAT-3 mainly expresses in the central nervous system and regulates the GABA activity in the central nervous system (3). On the contrary, GAT-2 is predominantly found in the peripheral organ, especially in liver and kidney, and it is considered as the GABA and taurine transporter in the liver (4). Except for the transport function, the physiological roles for GATs have also been described. For example, it has been demonstrated that GAT-2 could affect immune cell fates [e.g., macrophage polarization (5), Th1 differentiation (6), and Th17 responses (7)] and orchestrate the inflammatory responses of lipopolysaccharide-induced sepsis, infection-induced pneumonia, and high-fat diet-induced obesity in vivo (5, 8). Nevertheless, the physiological roles for GATs in directing the functions of peripheral organs are still enigmatic.
Liver is an organ mainly for metabolism and also plays a critical role in detoxification, biosynthesis of serum proteins, endocrine, and immune homeostasis (9, 10). More importantly, the liver is one of the most sexually dimorphic organs in gene expressions due to the different metabolic needs for male and female animals (11). For a long time, mice have been used as models for liver diseases such as non-alcoholic fatty liver disease (NAFLD), cirrhosis, fibrosis, and tumorigenesis (12–15). Also, studies have shown that there are significant gender differences in liver metabolism of male and female mice (16). Indeed, sex hormones (the conversion of testosterone to estradiol) meditate sexual dimorphism in the liver (11, 17). However, whether other endogenous signals modulate sexual dimorphism in the liver or influence liver functions in a sex-specific manner remains largely unclear.
In this study, the hepatic tissues of the wild type and GAT-2−/− mice are performed by analyzing amino acids, quantitative reverse transcription PCR (RT-qPCR), and transcriptome sequencing. As the connection between GAT-2 gene and liver function is not fully elucidated, we hope to analyze the amino acid profile and differentially expressed genes that are related to inflammation, like toll-like receptors (TLRs) family, and provide a basis for researching the link between GAT-2 and liver function in different sexes.
The GAT2−/− mice (KO) have been described previously (7); the mice were housed in pathogen-free colonies (temperature, 20–30°C, relative humidity, 50–60%, 12 h dark/12 h light), and they had free choice of standard rodent feed and drinking water. The homozygous wild-type (WT) mice and KO mice were bred separately and serve as further studies.
Ten 9-week-old WT mice (half male and half female) and ten 9-week-old KO mice (half male and half female) were selected randomly in this study. The mice were sacrificed, and the livers were collected at necropsy. The samples were frozen in liquid nitrogen immediately and stored at −80°C for further analysis.
After accurately weighing the tissue samples (40.0 mg) in a centrifuge tube, 400 μl ultrapure water was added and then vortexed for 4.5 min. Then, 200 μl of homogenates was added to 800 μl of methanol–acetonitrile (50:50, V/V). The mixture was vortexed and sonicated in ice water at 4°C for 10 min and then centrifuged at 19,000 g for 15 min at 4°C. The supernatant was taken and vacuum-dried at 60°C for 90 min and then dried with nitrogen to obtain a dry substance. The dry substance was dissolved with 200 μl methanol–water (50:50, V/V) and sonicated in ice water at 4°C for 10 min before centrifugation at 19,000 g for 15 min at 4°C. The supernatant was filtered through a 0.22 μm membrane filter. The experiments were performed on a Thermo Fisher Scientific UPLC system (Dionex UltiMate 3000) coupled with a mass spectrometer (Q-Exactive Focus). Xcalibur software (version 3.0) was used for instrument control, data acquisition, and data analysis.
Total RNA of liver tissue was extracted using TRIZOL (Invitrogen, CA, USA) and then treated with DNase I (Invitrogen, CA, USA) according to the instruction of the manufacturer. The RNA quality was assessed by an RNA 6000 Nano Assay Kit and determined by Agilent Bioanalyser2100 (Agilent Technologies, CA, USA), and the RNA concentration was detected spectrophotometrically at 260 nm by the ND-2000 (NanoDrop Technologies, DE, USA).
Complementary DNA (cDNA) was reverse transcribed using the RevertAcidTMfrst strand cDNA synthesis kit (TaKaRa, Qingdao, China). The amplification reactions were performed using an ABI Prism 7900 HT sequence detection system (Applied Biosystems, Foster, CA, USA). Primers were designed using PrimerPremier 5.0 and β-actin was used as an internal control to normalize target gene transcript levels. The relative levels of genes were presented in terms of 2−(ΔΔCt), where ΔCt = (Cttarget – Ctβ−actin) treatment – (Cttarget−Ctβ−actin)control.
Total RNA was purified by using the RNA purification bead and fragmented into short fragments with 150–200 bp in length. Next, the short fragments were used as a template for synthesizing the strand cDNA using TruSeq Stranded mRNA LT Sample Prep Kit (Illumina, CA, USA) according to the instructions of the manufacturer, and the purification of strand cDNA was carried out using the Agencourt AMPureXP (Beckman Coulter, CA, USA). Then, the purified strand cDNA was carried out to the end reparation, “A” base addition, and sequencing adapt religation. Finally, cDNA was amplification and used to construct the library, which was sequenced on the Illumina sequencing platform (HiSeqTM 2500).
Raw data (raw reads) in fast q format were removed after ligation with NGS QC Tool kit software firstly, and then, the clean reads were obtained after removing the low-quality reads and reads containing ploy-N. The Q20, Q30, GC content, and sequence duplication level of the resultant clean sequences were calculated, and the high-quality clean reads were used for the further analysis.
We downloaded the reference genome and annotation files from the Mus musculus Genome Informatics (MGI, http://www.informatics.jax.org). We then used Bowtie2 (18) to build an index of the reference genome and TopHat (19) to align the paired-end clean reads with the reference genome.
The fragments per kilobase transcriptome per million mapped reads (FPKM) value was used to quantify transcripts expression, it was calculated using cufflinks, and the read counts of each gene were obtained by htseq-count. Differentially expressed genes (DEGs) were identified using the DESeq (2012) functions estimate Size Factors and nbinom Test. A P < 0.05 was set as the threshold for significantly differential expression. Hierarchical cluster analysis of DEGs was performed to explore the pattern of genes expression. Gene ontology (GO) enrichment and Kyoto Encyclopedia of Genes and Genomes (KEGG) pathway enrichment analysis of DEGs were, respectively, performed using R based on the hypergeometric distribution.
Data in this study were presented as means ± SEM and analyzed using Prism 6.0 (GraphPad Software, Inc., La Jolla, CA, USA). The data that conformed to normal distribution and had equal variance were analyzed by unpaired t-test between two groups; data that conformed to normal distribution and had unequal variance were analyzed by unpaired t-test with Welch's correction between two groups; data that did not conform to normal distribution were analyzed by non-parametric test between the two groups. Mean values were considered as statistically different at P < 0.05.
Considering GAT-2 is the transporter for GABA, and liver is a crucial metabolically active organ, we analyzed the amino acid profile of livers in male and/or female mice with or without GAT-2 deficiency. In the livers of male KO mice, seven amino acids (glutamate, sarcosine, cystathionine, isoleucine, 5-hydroxylysine, 3-methylhistidine, and arginine) increased, but three amino acids (taurine, urea, and b-alanine) decreased (Figures 1A,B; Supplementary Figure 1A). Compared with WT mice, only two amino acids (sarcosine and GABA) increased in the livers of female KO mice (Figures 1A,B; Supplementary Figure 1B). The levels of GABA significantly increased in the livers of female KO mice, whereas it only slightly increased in male KO mice. These findings suggest that GAT-2 influences amino acid metabolism in liver of male mice and female mice in a different manner.
Figure 1. Common amino acids analysis by UPLC. (A) γ-amino butyric acid (GABA) level in livers of male (left) and female (right) mice. (B) Common amino acid level in livers of male (up) and female (down) mice. Data were analyzed with unpaired t-test and represented as means ± SEM except indicated.
Given that liver also serves as an important immune organ, we investigated whether GAT-2 affects the immunologic functions of livers in male and/or female mice. Here, we selected 10 genes, such as TLR1, TLR2, TLR3, TLR4, TLR5, TLR6, TLR7, TLR8, TLR9, and Myd88, which are related to TLR signaling pathways. RT-qPCR analysis revealed that TLR1, TLR2, TLR6, and TLR8 were downregulated in the livers of male KO mice (Figure 2A), whereas only TLR1 and TLR6 were downregulated in the livers of female KO mice (Figure 2B). Similarly, these findings indicate that GAT-2 may have a broader effect on TLR signaling of liver in male mice than those in female mice.
Figure 2. Quantitative reverse transcription PCR (qRT-PCR) validation expression profile of genes related to toll-like receptors (TLRs). [(A) male, (B) female]. Ten individual genes involved in toll-like receptor signaling pathways were analyzed by qRT-PCR. Data were analyzed with unpaired t-test and represented as means ± SEM except indicated. *P < 0.05.
Subsequently, we determined the sex-specific influence of GAT-2 on liver function at the intrinsic molecular level by performing the RNA-seq method. After Illumina sequencing, the sequencing and assembly are displayed in Table 1. Totally, we achieved 60,629,043 and 59,038,390 raw reads in WT female and male mice libraries, and 58,760,836 and 60,090,923 raw reads in KO female and male mice libraries, respectively. After quality control, we retained 59,052,921 and 57,646,065 clean reads in WT female and male mice libraries, and 57,398,020 and 58,618,110 in KO female and male mice libraries, respectively. The GC content of WT female and male mice was 48.00 and 47.33%, respectively. The GC content of KO female and male mice was 48.17 and 47.50%, respectively.
We then mapped the clean reads to the Mus musculus Genome Informatics (MGI, http://www.informatics.jax.org), and the results showed that approximately 92.07 and 81.92% of clean reads in WT female and male mice, respectively, were matched with the reference genome, and approximately 90.61 and 82.13% of clean reads in KO female and male mice, respectively, were matched with the reference genome. Furthermore, 80.69 and 68.94% clean reads in WT female and male mice, respectively, and 79.54 and 69.52% clean reads in KO female and male mice, respectively, were uniquely mapped to the reference genome. However, more than 40% of the clean reads were non-splice reads in each library (Table 1).
Both fold change and p-value or false discovery rate (FDR) were chosen to compare and analyze the differential expression of the Unigenes in two groups, and we screened differently expressed genes between the two groups based on the criteria |log2(fold change) |>0.0 and P < 0.05. The MA figures and heatmaps are shown in Figures 3A,B. Totally, 2,227 DEGs were identified in female KO mice, in which 1,030 genes were upregulated and 1,197 genes were downregulated (Figure 3C). However, only 1,233 DEGs were identified in male KO mice, in which 474 genes were upregulated and 759 genes were downregulated (Figure 3D). Interestingly, our RNA-seq data also demonstrated that GAT-2 may have a broader effect on TLR signaling of liver in male mice than those in female mice, evidenced by TLR1, TLR2, TLR3, TLR4, TLR6, and TLR8 that were downregulated in male KO mice (Supplementary Figure 2A), whereas only TLR1, TLR3, and TLR5 were downregulated and Myd88 were upregulated in KO female mice (Supplementary Figure 2B).
Figure 3. GABA transporter 2_(GAT2) deficiency alters the transcriptomic profile in the livers of male and female KO mice. (A) MA plot and heatmap analysis of upregulated (red) or downregulated genes (green) in male KO and wild-type (WT) livers (n = 3). (B) MA figure and heatmap analysis of upregulated (red) or downregulated genes (green) in female KO and WT livers (n = 3). (C,D) [(C), male; (D), female]. The number of up-/downregulated differentially expressed genes (DEGs) in female and male KO livers compared with WT livers, respectively. The red bars represented genes that were upregulated in KO compared to WT, while the green bars represented genes that were downregulated.
After achieving the DEGs, we performed the GO enrichment analysis and functional annotation of DEGs. In the GO analysis, gene functions were divided into three categories, namely, biological process (BP), cellular component (CC), and molecular function (MF). The threshold value for significant enrichment of a GO term was P < 0.05. The top 10 differences in the significance of GO terms in BP, CC, and MF are shown in Figure 4. In the biological process, negative regulation of cell adhesion and cell activation was the most significant categories in male KO mice (Figure 4A, left), whereas cholesterol metabolic process and steroid metabolic process were the most significant categories in female KO mice (Figure 4B, left), and as for cellular component, membrane raft and membrane microdomain were the most significant in male KO mice (Figure 4A, middle), while the most significant categories were inner mitochondrial membrane protein complex and respiratory chain complex in female KO mice (Figure 4B, middle); in molecular function, oxidoreductase activity and monooxygenase activity were the most significant GO terms in male KO mice (Figure 4A, right), while enzyme activator activity and transcription factor activity were the most significant GO terms in KO female mice (Figure 4B, right).
Figure 4. Functional GO categories of DEGs (KO vs WT). (A) GO classification in male KO and WT livers, including biological process, cellular component, and molecular function. (B) GO classification in female KO and WT livers, including biological process, cellular component, and molecular function. Y-axis: The logarithmic value of P-value, X-axis: GO classification.
Kyoto Encyclopedia of Genes and Genomes was the main public database for pathways (20). In total, 284 and 277 different pathways were detected in KO female and male mice, respectively. The most significant 20 pathways are shown in Figure 5. Among these pathways, retinol metabolism, PPAR signaling pathway, and tuberculosis were the most three significant pathways in male KO mice (Figure 5A), whereas oxidative phosphorylation, NAFLD, and Huntington's disease were the most three significant pathways in KO female mice (Figure 5B). Interestingly, the PPAR signaling pathway showed a similar p-value in both male and female KO mice pathway enrichment analysis. Overall, our RNA-seq data suggest that GAT-2 influences liver function in a sex-specific manner at the intrinsic molecular level.
Figure 5. Scatterplot of enriched KEGG pathways for DEGs. [(A) male, (B) female]. Sizes of bubbles indicate the number of DEGs in the corresponding pathway, and the colors of the bubble represent P-value after multiple hypothesis test.
γ-amino butyric acid (GABA)ergic system consists of GABA, GABA receptors, glutamate decarboxylase (GAD), vesicular inhibitory amino acid transporter (VIAAT), GATs, and GABA transaminase (GABA-T). Studies show that the GABAergic system not only has inhibitory functions in the central nervous system but also plays vital roles in modulating the immune system. For example, GABA inhibits the development of proinflammatory T-cell responses (21). Besides the development of immune cells, GABA also modulates the release of inflammatory cytokines from peripheral blood mononuclear cells and T cells (22). GAD, and GABA receptors and transporters have been found in immune cells (e.g., macrophages and T cells) and could determine the fates of these immune cells (5, 7). Thus, these findings draw much attention in exploring the role of the GABAergic system in regulating the peripheral system and organs (including liver).
Liver is one of the most sexual-dimorphism organs and mainly for metabolism (23–25). Firstly, we analyzed the levels of GABA and other amino acids in murine livers. Interestingly, we demonstrate that GABA significantly increases in livers of KO female mice whereas slightly increases in the livers of male KO mice, indicating that GAT-2 deletion seems to affect the transport of GABA in livers of mice. Additionally, sarcosine increases in livers of both female and male KO mice, which could function as a potentially important metabolic intermediary of cancer cell invasion and aggressivity (26, 27). Isoleucine can improve the function of the immune system, including immune organs, cells, and reactive substances (28), whereas higher levels of glutamate, 5-hydroxylysine, and 3-methylhistidine may cause adverse health effects (29–31). Considering that GAT-2 deficiency mainly increases these aforementioned amino acids in the livers of male mice, it could be concluded that GAT-2 has a different immunomodulatory role in regulating the hepatic functions of male mice. However, the potential mechanisms still need to be further revealed.
Toll-like receptors are a group of important proteins involved in innate immunity and also serve as a bridge connecting non-specific immunity and specific immunity (32). They could recognize moderated microbial structures, such as bacterial lipopolysaccharide and viral double-stranded RNA, and activate signaling pathways that lead to immune responses against microbial infections (33). All TLRs share a traditional adaptor protein-MyD88 and also activate a common signaling pathway that culminates in the activation of nuclear factor–κB (NF-κB) transcription factors and the mitogen-activated protein kinases (MAPKs) extracellular signal (34, 35). Therefore, the expressions of TLR1, TLR2, TLR3, TLR4, TLR5, TLR6, TLR7, TLR8, TLR9, and Myd88 that are related to TLR signaling pathways are taken as the proxy for exploring the immunomodulatory role of GAT-2 on the hepatic functions. Intriguingly, both results from RT-qPCR and RNA-seq highlight that GAT-2 deficiency significantly lowers the expressions of TLR1/2/6/8 in male murine livers whereas it only highly downregulates TLR1 in the livers of female mice.
To further investigate the effects of GAT-2 on the livers at the molecular level, we perform the transcriptome analysis between the liver of WT mice and GAT-2−/−mice. After quality control, we have retained 59,052,921 and 57,646,065 clean reads in WT female and male mice libraries, 57,398,020 and 58,618,110 in KO female and male mice libraries, respectively, and the GC content is between 40 and 60%. These data mean that the sequence coverage of two libraries is enough for transcriptional analysis. Notably, we find that the DEG number of female KO mice is much larger in male KO mice (2,227 vs. 1,233), which indicates that the broader effects of GAT-2 on transcriptomic profile in the livers of female mice.
Differentially expressed genes are involved in a wide variety and different functions including biological process, cellular component, and molecular function (36). We can seek their differences more specifically in this study from KEGG analysis. Moreover, KEGG analysis shows that oxidative phosphorylation (OXPHOS) and NAFLD are the most significant pathways in KO female mice, whereas retinol metabolism and the PPAR signaling pathway are the most significant pathways in KO male mice. These findings indicate that GAT-2 might influence the metabolism of female and male liver functions through different pathways. OXPHOS is one of the dominant metabolic routes mainly for adenosine triphosphate (ATP) production in the cell (5, 37). NAFLD is defined as the excessive deposition of fat in hepatocytes, excluding alcohol and other specific factors that may damage the liver (13). Moreover, it has been shown that retinol promotes normal growth and development of the host (38). PPAR signaling pathway regulates the expression of genes associated with lipid metabolism, fat formation, maintenance of metabolic homeostasis, and inflammation (39). Therefore, GAT-2 might regulate excessive fat deposition and inflammation in the livers of female mice, whereas GAT-2 could affect normal growth and development of livers in male mice involving retinol metabolism and PPAR signaling pathway. Interestingly, the PPAR signaling pathway is significantly enriched in both male and female GAT-2 KO mice, which might attribute to the increase of GABA and/or affected TLRs. Nevertheless, well-designed experiments for validating the aforementioned results are needed.
Collectively, our results reveal a sex-dimorphic influence of GAT-2 on murine liver functions at the molecular level, which could provide a basis for further discussing the physiological and pathophysiological role of GAT-2.
The datasets presented in this study can be found in online repositories. The names of the repository/repositories and accession number(s) can be found in the article/Supplementary Material.
The animal study was reviewed and approved by Laboratory Animal Ethical Commission of the South China Agricultural University.
JF and CZ designed the research and analyzed data. JF, QZ, ZW, and CH performed the experiments and analyzed the data. JF wrote the manuscript. CZ revised the manuscript. All authors contributed to the article and approved the submitted version.
This work was supported by the Guangdong Basic and Applied Basic Research Foundation (2019B1515210002).
The authors declare that the research was conducted in the absence of any commercial or financial relationships that could be construed as a potential conflict of interest.
All claims expressed in this article are solely those of the authors and do not necessarily represent those of their affiliated organizations, or those of the publisher, the editors and the reviewers. Any product that may be evaluated in this article, or claim that may be made by its manufacturer, is not guaranteed or endorsed by the publisher.
The Supplementary Material for this article can be found online at: https://www.frontiersin.org/articles/10.3389/fnut.2021.751388/full#supplementary-material
Supplementary Figure 1. Uncommon amino acids analysis by UPLC. [(A) male, (B) female]. Data were analyzed with unpaired t-test and represented as means ± SEM except indicated. *P < 0.05.
Supplementary Figure 2. RNA-seq validation expression profile of genes related to TLRs. [(A) male, (B) female]. *P < 0.05, **P < 0.01, ***P < 0.001, ****P < 0.0001.
Supplementary Table 1. The full list of DEGs.
Supplementary Table 2. The full list of enriched GO terms.
Supplementary Table 3. Detailed information on the enriched KEGG pathways.
1. Conti F, Minelli A, Melone M. GABA transporters in the mammalian cerebral cortex: localization, development and pathological implications. Brain Res Brain Res Rev. (2004) 45:196–212. doi: 10.1016/j.brainresrev.2004.03.003
2. Madsen KK, White HS, Schousboe A. Neuronal and non-neuronal GABA transporters as targets for antiepileptic drugs. Pharmacol Therapeut. (2010) 125:394–401. doi: 10.1016/j.pharmthera.2009.11.007
3. Chiu CS, Brickley S, Jensen K, Southwell A, Mckinney S, Cull-Candy S, et al. GABA transporter deficiency causes tremor, ataxia, nervousness, and increased GABA-induced tonic conductance in cerebellum. J Neurosci. (2005) 25:3234–45. doi: 10.1523/Jneurosci.3364-04.2005
4. Zhou Y, Holmseth S, Hua R, Lehre AC, Olofsson AM, Poblete-Naredo I, et al. The betaine-GABA transporter (BGT1, slc6a12) is predominantly expressed in the liver and at lower levels in the kidneys and at the brain surface. Am J Physiol-Renal. (2012) 302:F316–28. doi: 10.1152/ajprenal.00464.2011
5. Xia Y, He F, Wu X, Tan B, Chen S, Liao Y, et al. GABA transporter sustains IL-1beta production in macrophages. Sci Adv. (2021) 7:eabe9274. doi: 10.1126/sciadv.abe9274
6. Ding X, Chang Y, Wang S, Yan D, Yao J, Zhu G. Transcriptomic analysis of the effect of GAT-2 deficiency on differentiation of mice naive T cells into Th1 cells in vitro. Front Immunol. (2021) 12:667136. doi: 10.3389/fimmu.2021.667136
7. Ren W, Liao Y, Ding X, Jiang Y, Yan J, Xia Y, et al. Slc6a13 deficiency promotes Th17 responses during intestinal bacterial infection. Mucosal Immunol. (2019) 12:531–44. doi: 10.1038/s41385-018-0111-7
8. Miwa M, Tsuboi M, Noguchi Y, Enokishima A, Nabeshima T, Hiramatsu M. Effects of betaine on lipopolysaccharide-induced memory impairment in mice and the involvement of GABA transporter 2. J Neuroinflamm. (2011) 8:1–13. doi: 10.1186/1742-2094-8-153
9. Fabregat I. Exploring liver physiology, pathology, TGF-beta, EMT, stemness and new developments in liver cancer. Hepat Oncol. (2017) 4:9–13. doi: 10.2217/hep-2017-0007
10. Wang H, Liang X, Gravot G, Thorling CA, Crawford DH, Xu ZP, et al. Visualizing liver anatomy, physiology and pharmacology using multiphoton microscopy. J Biophotonics. (2017) 10:46–60. doi: 10.1002/jbio.201600083
11. Della Torre S, Mitro N, Meda C, Lolli F, Pedretti S, Barcella M, et al. Short-term fasting reveals amino acid metabolism as a major sex-discriminating factor in the liver. Cell Metab. (2018) 28:256. doi: 10.1016/j.cmet.2018.05.021
12. Sato T, Tsuchiya A, Owaki T, Kumagai M, Motegi S, Iwasawa T, et al. Severe steatosis and mild colitis are important for the early occurrence of hepatocellular carcinoma. Biochem Bioph Res Co. (2021) 566:36–44. doi: 10.1016/j.bbrc.2021.05.097
13. Zhou J, Zhao Y, Guo YJ, Zhao YS, Liu H, Ren J, et al. A rapid juvenile murine model of nonalcoholic steatohepatitis (NASH): Chronic intermittent hypoxia exacerbates Western diet-induced NASH. Life Sci. (2021) 276:119403. doi: 10.1016/j.lfs.2021.119403
14. Yan LK, Zhang X, Fu J, Liu Q, Lei XH, Cao ZY, et al. Inhibition of the transient receptor potential vanilloid 3 channel attenuates carbon tetrachloride-induced hepatic fibrosis. Biochem Bioph Res Co. (2021) 558:86–93. doi: 10.1016/j.bbrc.2021.04.065
15. Barthet VJA, Brucoli M, Ladds MJGW, Nossing C, Kiourtis C, Baudot AD, et al. Autophagy suppresses the formation of hepatocyte-derived cancer-initiating ductular progenitor cells in the liver. Sci Adv. (2021) 7:eabf9141. doi: 10.1126/sciadv.abf9141
16. Kwo PY, Ramchandani VA, O'Connor S, Amann D, Carr LG, Sandrasegaran K, et al. Gender differences in alcohol metabolism: relationship to liver volume and effect of adjusting for body mass. Gastroenterology. (1998) 115:1552–7. doi: 10.1016/s0016-5085(98)70035-6
17. Zheng W, Xu H, Lam SH, Luo H, Karuturi RK, Gong Z. Transcriptomic analyses of sexual dimorphism of the zebrafish liver and the effect of sex hormones. PLoS ONE. (2013) 8:e53562. doi: 10.1371/journal.pone.0053562
18. Langmead B, Salzberg SL. Fast gapped-read alignment with Bowtie 2. Nat Methods. (2012) 9:357–9. doi: 10.1038/Nmeth.1923
19. Trapnell C, Pachter L, Salzberg SL. TopHat: discovering splice junctions with RNA-Seq. Bioinformatics. (2009) 25:1105–11. doi: 10.1093/bioinformatics/btp120
20. Kanehisa M, Goto S. KEGG: kyoto encyclopedia of genes and genomes. Nucleic Acids Res. (2000) 28:27–30. doi: 10.1093/nar/28.1.27
21. Tian JD, Lu YX, Zhang HW, Chau CH, Dang HN, Kaufman DL. Gamma-aminobutyric acid inhibits T cell autoimmunity and the development of inflammatory responses in a mouse type 1 diabetes model. J Immunol. (2004) 173:5298–304. doi: 10.4049/jimmunol.173.8.5298
22. Bhandage AK, Jin Z, Korol SV, Shen QJ, Pei Y, Deng QL, et al. GABA regulates release of inflammatory cytokines from peripheral blood mononuclear cells and CD4(+) T cells and is immunosuppressive in type 1 diabetes. Ebiomedicine. (2018) 30:283–94. doi: 10.1016/j.ebiom.2018.03.019
23. Goossens GH, Jocken JWE, Blaak EE. Sexual dimorphism in cardiometabolic health: the role of adipose tissue, muscle and liver. Nat Rev Endocrinol. (2021)17:47–66. doi: 10.1038/s41574-020-00431-8
24. Torre D, Lolli F, Ciana P, Maggi A. Sexual dimorphism and estrogen action in mouse liver. Adv Exp Med Biol. (2017) 1043:141–51. doi: 10.1007/978-3-319-70178-3_8
25. Rui L. Energy metabolism in the liver. Compr Physiol. (2014) 4:177–97. doi: 10.1002/cphy.c130024
26. Heger Z, Rodrigo MAM, Michalek P, Polanska H, Masarik M, Vit V, et al. Sarcosine up-regulates expression of genes involved in cell cycle progression of metastatic models of prostate cancer. PLoS ONE. (2016) 11:e0165830. doi: 10.1371/journal.pone.0165830
27. Sreekumar A, Poisson LM, Rajendiran TM, Khan AP, Cao Q, Yu JD, et al. Metabolomic profiles delineate potential role for sarcosine in prostate cancer progression (vol 457, pg 910, 2009). Nature. (2013) 499:504–04. doi: 10.1038/nature12288
28. Gu CS, Mao XB, Chen DW, Yu B, Yang Q. Isoleucine plays an important role for maintaining immune function. Curr Protein Pept Sci. (2019) 20:644–51. doi: 10.2174/1389203720666190305163135
29. de la Fuente-Sandoval C, Leon-Ortiz P, Favila R, Stephano S, Mamo D, Ramirez-Bermudez J, et al. Higher levels of glutamate in the associative-striatum of subjects with prodromal symptoms of schizophrenia and patients with first-episode psychosis. Neuropsychopharmacology. (2011) 36:1781–91. doi: 10.1038/npp.2011.65
30. Herbert KR, Williams GM, Cooper GJS, Brimble MA. Synthesis of glycosylated 5-hydroxylysine, an important amino acid present in collagen-like proteins such as adiponectin. Org Biomol Chem. (2012) 10:1137–44. doi: 10.1039/c1ob06394d
31. Neumann S, Welling H, Bilzer T, Thuere S. Myopathy and alterations in serum 3-methylhistidine in dogs with liver disease. Res Vet Sci. (2008) 84:178–84. doi: 10.1016/j.rvsc.2007.05.014
32. Fitzgerald KA, Kagan JC. Toll-like receptors and the control of immunity. Cell. (2020) 180:1044–66. doi: 10.1016/j.cell.2020.02.041
33. Moynagh PN. Toll-like receptor signalling pathways as key targets for mediating the anti-inflammatory and immunosuppressive effects of glucocorticoids. J Endocrinol. (2003) 179:139–44. doi: 10.1677/joe.0.1790139
34. Shuto T, Xu H, Wang B, Han J, Kai H, Gu XX, et al. Activation of NF-kappa B by nontypeable Hemophilus influenzae is mediated by toll-like receptor 2-TAK1-dependent NIK-IKK alpha /beta-I kappa B alpha and MKK3/6-p38 MAP kinase signaling pathways in epithelial cells. Proc Natl Acad Sci U S A. (2001) 98:8774–9. doi: 10.1073/pnas.151236098
35. Barton GM, Medzhitov R. Toll-like receptor signaling pathways. Science. (2003) 300:1524–5. doi: 10.1126/science.1085536
36. Ashburner M, Ball CA, Blake JA, Botstein D, Butler H, Cherry JM, et al. Gene ontology: tool for the unification of biology. The gene ontology consortium. Nat Genet. (2000) 25:25–9. doi: 10.1038/75556
37. Li Y, Jia A, Wang Y, Dong L, Wang Y, He Y, et al. Immune effects of glycolysis or oxidative phosphorylation metabolic pathway in protecting against bacterial infection. J Cell Physiol. (2019) 234:20298–309. doi: 10.1002/jcp.28630
38. Chen L, Khillan JS. A novel signaling by vitamin A/retinol promotes self renewal of mouse embryonic stem cells by activating PI3K/Akt signaling pathway via insulin-like growth factor-1 receptor. Stem Cells. (2010) 28:57–63. doi: 10.1002/stem.251
Keywords: GAT-2, sex dimorphism, liver, amino acids, transcriptome
Citation: Fu J, Zhang Q, Wu Z, Hong C and Zhu C (2021) Transcriptomic Analysis Reveals a Sex-Dimorphic Influence of GAT-2 on Murine Liver Function. Front. Nutr. 8:751388. doi: 10.3389/fnut.2021.751388
Received: 01 August 2021; Accepted: 17 August 2021;
Published: 16 September 2021.
Edited by:
Xihong Zhou, Institute of Subtropical Agriculture (CAS), ChinaReviewed by:
Haiwen Zhang, Hainan University, ChinaCopyright © 2021 Fu, Zhang, Wu, Hong and Zhu. This is an open-access article distributed under the terms of the Creative Commons Attribution License (CC BY). The use, distribution or reproduction in other forums is permitted, provided the original author(s) and the copyright owner(s) are credited and that the original publication in this journal is cited, in accordance with accepted academic practice. No use, distribution or reproduction is permitted which does not comply with these terms.
*Correspondence: Congrui Zhu, Y29uZ3J1aXpAa3N1LmVkdQ==
†These authors have contributed equally to this work
Disclaimer: All claims expressed in this article are solely those of the authors and do not necessarily represent those of their affiliated organizations, or those of the publisher, the editors and the reviewers. Any product that may be evaluated in this article or claim that may be made by its manufacturer is not guaranteed or endorsed by the publisher.
Research integrity at Frontiers
Learn more about the work of our research integrity team to safeguard the quality of each article we publish.