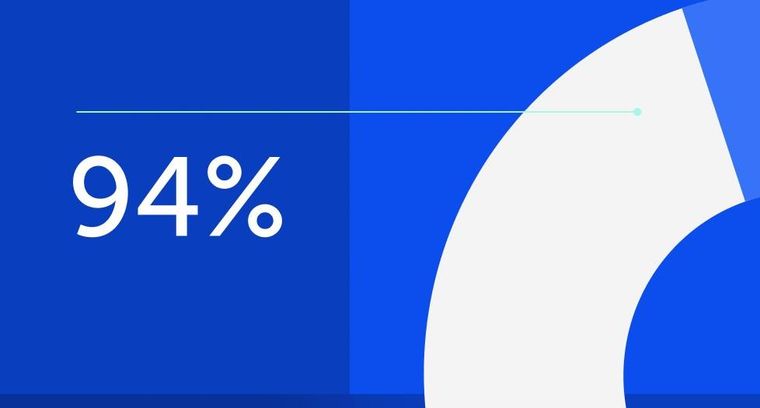
94% of researchers rate our articles as excellent or good
Learn more about the work of our research integrity team to safeguard the quality of each article we publish.
Find out more
ORIGINAL RESEARCH article
Front. Nutr., 17 March 2022
Sec. Nutrition and Metabolism
Volume 8 - 2021 | https://doi.org/10.3389/fnut.2021.741811
This article is part of the Research TopicThe Model of Ramadan Diurnal Intermittent Fasting: Unraveling the Health Implications - Volume 1View all 16 articles
Aim and Background: A growing body of evidence supports the impact of intermittent fasting (IF) on normalizing body weight and that the interaction between body genes and environmental factors shapes human susceptibility to developing obesity. FTO gene is one of these genes with metabolic effects related to energy metabolism and body fat deposition. This research examined the changes in FTO gene expression upon Ramadan intermittent fasting (RIF) in a group of metabolically healthy subjects with overweight and obesity.
Methods: Sixty-three (63) subjects were recruited, of which 57 (17 males and 40 females, mean age 38.4 ± 11.2 years) subjects with overweight and obesity (BMI = 29.89 ± 5.02 kg/m2were recruited and monitored before and at the end of Ramadan month), and 6 healthy subjects with normal BMI (21.4 ± 2.20 kg/m2) recruited only to standardize the reference for normal levels of FTO gene expression. In the two-time points, anthropometric, biochemical, and dietary assessments were undertaken, and FTO gene expression tests were performed using RNA extracted from the whole blood sample.
Results: In contrast to normal BMI subjects, the relative gene expressions in overweight/obese were significantly decreased at the end of Ramadan (−32.30%, 95% CI–0.052 −0.981) in comparison with the pre-fasting state. Significant reductions were found in body weight, BMI, fat mass, body fat percent, hip circumference, LDL, IL-6, TNF-α (P<0.001), and in waist circumference (P < 0.05), whilst HDL and IL-10 significantly increased (P<0.001) at the end of Ramadan in comparison with the pre-fasting levels. Binary logistic regression analysis for genetic expressions showed no significant association between high-energy intake, waist circumference, or obesity and FTO gene expression.
Conclusions: RIF is associated with the downregulation of the FTO gene expression in subjects with obesity, and this may explain, at least in part, its favorable metabolic effects. Hence, RIF presumably may entail a protective impact against body weight gain and its adverse metabolic-related derangements in subjects with obesity.
Obesity is one of the most common prevalent chronic diseases, with its comorbidities and long-term consequent mortality has become a major challenge to global health (1). Obesity is a complicated multifaceted disease that develops from the interaction of cellular, molecular, genetic, metabolic, physiologic, behavioral, cultural, and socioeconomic, influences (2, 3). Notably, cardiovascular disease, diabetes, renal disorders, and neoplasms were the major causes of high body mass index (BMI)-related disability-adjusted life years (DALYs), accounting for 89.3 percent of all high-BMI-related DALYs, with the BMI-related disease burden varying significantly, depending on the Socio-Demographic Index (SDI) (4). Despite the tremendous efforts in the MENA region to combat the problem, yet obesity is still a major health problem due to several factors including the adopted dietary patterns and physical inactivity (5, 6). Genetic predisposing factors represent one of the major contributing factors in the etiopathogenesis of obesity and its consequent complications, with some studies unraveling that high BMI is 25–40% heritable (7). However, to affect body weight, genetic predisposing factors often need to be coupled with environmental and behavioral triggering factors (8, 9).
With the progressive advancement of genome-wide association studies, more than 100 loci have been identified to be associated with obesity and its related traits (10). One of these genetic loci with a strong effect on obesity and related biological functions such as adipogenesis and energy balance regulation, fat mass, and obesity-associated (FTO) gene has emerged as one of the influential genes with remarkable impact (11). Recent large-scale analyses found that the obesity-risk allele (rs9939609 A allele) of the FTO is associated with increased food intake (12, 13), and previous studies also reported that the FTO obesity-risk allele was associated with a reduced response to hunger and satiety after meals in adults and children (14–16).
Nowadays, intermittent fasting (IF) has been looked at as an emerging effective, and costless dietary intervention that helps to promote health and prevent disease and aging (17). Several reports showed the benefits of different styles of IF, including time-restricted eating (TRE, a form of IF that involves confining the eating window to 4–10 h and fasting for the remaining hours of the day) (18), modified fasting regimens allowing 20–25% of energy needs to be consumed on scheduled fasting days, alternate-day fasting. The benefits are well evident for metabolic disorders, as well as cancer, obesity, diabetes, and neurological disorders (17, 19, 20).
Among the widely observed and extensively examined types of IF is the religious form observed during the month of Ramadan (RIF) (21). Ramadan is the ninth month of the lunar calendar, during which healthy adults are mandated to abstain from dawn to sunset, and to refrain from eating and drinking (including water) for a period that extends from 12 to 17 h, depending on the solar season and geographical location (22). This pattern of fasting is associated with dietary (including both food quality and quantity) (23), lifestyles (including sleep quality and quantity) (24) as well as circadian rhythm hormonal changes (25) that may harbor changes in gene expression.
Original research, systematic reviews, and meta-analyses unraveled that observing RIF entails a plethora of beneficial improvements in variable health and metabolic aspects, including reducing the cardiometabolic risk factors such as waist circumference, very low-density-lipoprotein cholesterol, low-density lipoprotein cholesterol (LDL), high-density-lipoprotein cholesterol HDL, total cholesterol (TC), triglycerides (TG), heart rate (HR), systolic blood pressure (SBP), and diastolic blood pressure (DBP) (26, 27). Moreover, RIF is associated reduce body weight (28), body fat content (29) with emphasis on visceral adiposity (30), and ameliorating inflammatory and oxidative stress (31–33), and non-alcoholic fatty liver disease markers in healthy people (34).
With the expansion of the nutrigenomic studies, growing attention has been directed toward examining the effect of different regimens of IF on gene expression of variable genes related to human health and diseases (16, 35). However, there is a paucity of studies tackling the effect of RIF and the associated dietary and lifestyle changes on the expression of specific genes related to human health and disease. Among these, only two studies examined the impact of RIF on the anti-oxidative stress genes (TFAM, Nrf2, SOD2) and metabolism-controlling genes (SIRT1, SIRT3) (36), the Circadian Locomotor Output Cycles Kaput (CLOCK) gene, and other genes related to circadian rhythmicity (37). However, the relationship between RIF and obesity- and body fat-controlling gene expressions is still to be investigated. In the former study, RIF was associated with significant increases in the relative expressions of the antioxidant genes (TFAM, SOD2, and Nrf2) in obese subjects in comparison to counterpart expressions of healthy weight subjects, with percent increments of 90.5, 54.1, and 411.5% for the three genes, respectively. However, the metabolism-controlling gene (SIRT3) showed a highly significant downregulation accompanied by a clear trend for reduction in the SIRT1 gene at the end of Ramadan month, with percent decrements of 61.8 and 10.4%, respectively (36). For the latter, profound changes were reported in the diurnal expression of CLOCK, a central component of the circadian molecular clock, during Ramadan compared to the non-fasting month of Sha'aban (the month before Ramadan) (37). One study assessed the association of common polymorphisms in the CLOCK and FTO gene polymorphisms (SNPs) (rs1801260 and rs9939609, respectively) with standardized BMI scores, and the impact of dietary and lifestyle modification in school-age children (38). It was found that sex is a potential modifier for the association between the CLOCK polymorphism and BMI z-scores in school-age children (38) and that the FTO SNP, rs9939609, did not significantly modify the effect of the intervention on BMI z-scores at the follow-up or changes of BMI z-scores (38).
Given the proven impact of RIF in lowering body weight (28), body fatness (29), visceral fat content (30), and satiety and eating-controlling hormones (leptin, adiponectin, ghrelin) (25); it becomes rational to examine the relationship between the observance of RIF and the expression of FTO gene. Considering the preventive effect of IF, and RIF in particular as a unique diurnal TRE model (39), on the above-mentioned obesity-related indicators, and the principal role of FTO in controlling satiety, food intake, body fatness, and obesity risk (11, 13–16); the current work stemmed from the hypothesis that observing RIF will be associated with reduced expression of the FTO gene in fasting people with obesity. Therefore, the current work was designed to find out how the observance of RIF by fasting people with obesity will be associated with changes in the genetic expression of FTO.
In total, 63 subjects were recruited, of which 57 (17 males and 40 females, mean age 38.4 ± 11.2 years) subjects with overweight and obesity (BMI = 29.89 ± 5.02 kg/m2 (were recruited and monitored before and at the end of Ramadan month, and 6 healthy subjects with normal BMI (21.4 ± 2.20 kg/m2) recruited only to standardize the reference for normal levels of FTO gene expression. All subjects who visited the University Hospital Sharjah (UHS), UAE, for screening were recruited for this study. All subjects were Arabs from the Arabian Gulf, Iraq, Egypt, Sudan, Tunisia, and the Levant countries (Syria, Jordan, Lebanon, and Palestine). The study protocol was designed and conducted following the Declaration of Helsinki and approved by the UHS Research Ethics Committee (Reference no. REC/16/12/16/002). All enrolled subjects (n = 63) were provided with an information sheet describing the research plan, objectives, and requirements of participation. Subjects were recruited using personal communication, social media, and institutional emails. All subjects attended the UHS for screening and investigations and provided signed informed consent to participate in this study. Subjects were men and women who were of either normal weight or overweight/obesity (BMI >25 kg/m2) and decided to fast Ramadan and were willing to participate in this study. We have collected basic and sociodemographic data using a self-report questionnaire that covered the medical history and demographic information. The questionnaire was administered in individual face-to-face interviews. A trained research assistant conducted all interviews. The exclusion criteria were a history of metabolic syndrome, diabetes, or cardiovascular disease, taking regular medications for any chronic disease, following a weight-reducing diet, a history of bariatric surgery within the last 6–9 months before commencing Ramadan fasting, and being a pregnant or peri-menopausal woman.
A prospective observational study design was used to investigate the effect of RIF on FTO gene expressions along with variable anthropometric, metabolic, and inflammatory markers in subjects with overweight and obesity. Data were collected at baseline (2–7 days before RIF) and after completing 28–30 consecutive days of diurnal RIF. During the fasting month of Ramadan, individuals abstain from all foods and drinks (including water) from dawn to sunset, with the average fasting duration being 15 h per day. Subjects were not requested to follow any dietary or physical activity regimens or recommendations during any stage of this study. All subjects were asked to pursue habitual lifestyle patterns during both fasting and non-fasting hours. According to Islamic laws of fasting, menstruating women are exempted from observing Ramadan fasting during their period; hence, the fasting period for participating women was less than that for men (23–25 vs. 28–30 days).
Anthropometric measurements were taken at two time points (before and at the end of commencement of 28–30 fasting days). Anthropometric measures of body weight, fat mass, and body fat percentage, and fat-free mass were measured using segmental multi-frequency bioelectrical impedance analysis (DSM-BIA; TANITA, MC-980, Tokyo/Japan) before and at the end of the fasting month. The DSM-BIA machine measured the visceral fat rating (from 0 to 100), and this value was converted into a visceral fat surface area by multiplying the obtained value by 10, consistent with the manufacturer's instructions. Height was measured using a fixed stadiometer to the nearest 0.1 cm. BMI was calculated as weight (kg) divided by height in m2. Waist and hip circumference were measured to the nearest 0.01 m using a non-stretchable measuring tape (Seca, Hamburg/Germany), and their ratio was calculated accordingly.
No special dietary recommendations or food regimens were given to the study subjects during any stage of the study, and all the subjects were asked to pursue their habitual dietary patterns during the eating period before and during Ramadan. Dietary intakes were assessed by trained nutritionists using the 24-h recall technique on 3 days (one weekend day and two weekdays) at the two-time points (before and at the end of Ramadan fasting). Printed two-dimensional food models were used to help study subjects approximate the eaten portion sizes. Dietary intakes of energy (calories), macronutrients (carbohydrates, protein, fats, and water), and micronutrients (vitamins and minerals) were estimated using the Food Processor software (version 10.6 ESHA Research, Salem, OR/USA).
The Dietary Reference Intakes classification for general physical activity level was used to assess subjects' level of physical activity (40). This classification depends on the general physical exercise pattern. Subjects were considered highly active if they performed at least 2 h per day of moderate-intensity physical exercise or 1 h of vigorous exercise in addition to daily living activities. Subjects were considered moderately active if they performed more than 1 h per day of moderate-intensity exercise in addition to daily living activities. Subjects that performed 30 mins to 1 h per day of moderate-intensity physical exercise in addition to daily living activities were considered to have low activity. Finally, subjects who performed daily living activities without other physical exercise were considered sedentary (40).
A sample of 10 ml of blood was collected from subjects at baseline in 3 different tubes including red top (plain) for serum, purple top (EDTA) for plasma and RNA extraction, and gray top (sodium fluoride) for glucose level (before commencing fasting) and at the end of the fasting month. At both time points, blood samples were collected after at least 8 h of fasting. The samples were collected between 11 a.m. and 1 p.m. to eliminate the effect of timing and dietary intake on the measured biochemical parameters and ensure consistency in the duration of fasting for the two-time points. Collected blood samples were divided into two aliquots. One aliquot was centrifuged at 2,500 rpm for 15 min within 1 h of the collection; the serum was aliquoted, coded, and stored at −80°C until it was used for biochemical analysis. The second aliquot was used for RNA extraction, as explained below.
In this study, we used chemiluminescent immunoassay (CLIA) based on a fully automated clinical chemistry analyzer (Adaltis, Pchem1, Italy) to quantify fasting glucose, total cholesterol (TC), LDL-cholesterol, HDL-cholesterol, and triglycerides (TG) at the two-time points. The pro-and anti-inflammatory cytokines (IL-6 and TNF-α; and IL-10, respectively) were quantified using a multiplex assay (Luminex, Bio-Plex Pro™ Human Cytokine plex Assay).
Blood pressure was measured before blood sampling using a digital blood pressure monitor (GE, USA), with subjects in an erect, seated position after a 5-min resting period.
RNA was extracted using the column-based, Total RNA Purification kit from Norgen, (Thorold, Canada) and reverse transcribed to cDNA by the QuantiTect Reverse Transcription kit from Qiagen (Hilden, Germany), according to the manufacturer's instructions. cDNA and primer concentrations were optimized to obtain a single amplification peak. qPCR reaction was performed at a volume of 20 μl, including 10 ng of cDNA with 5x HOT FIREPol EvaGreen qPCR Mix Plus (ROX) (Solis Biodyne). The cycling conditions included initial activation of the polymerase for 15 min at 95°C, followed by 45 cycles of 15-s denaturation at 94°C, annealing at 55°C for 30 s followed by extension at 72°C for 30 s. The forward and reverse primers used in the study are presented in Table 1. For each sample, the expression of each gene was normalized to the housekeeping gene ribosomal protein L18 subunit (RPL18s); The RPL18s was chosen as it showed less variation among GAPDH and actin housekeeping genes during the initial optimization; at the same time point. (10ng of cDNA and 0.1 uM of each primer per reaction). Three different negative controls were used at this analysis; control (1) no enzyme was added, control (2) no mRNA was added, and control (3) water was added instead of cDNA (NTC control). Therefore, the minimum amount of cDNA, primers and SYBR green have been used per reaction to obtain the specific signal avoiding false amplification. The relative expression was shown as fold change according to Livak and Schmittgen (41) and was presented as mean and standard deviation as described elsewhere (42). Considering the lack of reference range for FTO gene expression, six subjects with normal BMI (21.4 ± 2.20 kg/m2) were recruited only to get the normal FTO expression levels at the two points (before and after Ramadan fasting). For overweight/obese subjects, at each time point, the FTO gene expression was first calculated relative to the housekeeping (RPL18) gene, then as a fold-change compared to the gene expression for the normal reference levels obtained from subjects with normal BMI.
Table 1. Forward and reverse primers were used in genetic analysis for the FTO and housekeeping genes tested.
The statistical analyses were done using Statistical analyses were performed using SPSS 24 (IBM, Armonk, NY, USA). and reported based on the Strengthening the Reporting of Observational Studies in Epidemiology (STROBE) guidelines (43). The primary outcome measure was the change in the genetic expression of the FTO gene between the two-time points. We estimated that 51 subjects would provide 80% power to detect a significant difference of 5% in genetic expression between baseline (pre-fasting) and post-fasting using a two-tailed paired-samples t-test with α = 0.05. With an expected dropout rate of 10%, 56 subjects were planned for enrollment. Tests for normality were included in the model. The variables were expressed as the mean ± standard deviation (SD). Independent sample t-test comparing baseline characteristics between males and females. Two-tailed Paired sample t-tests were used to compare within-subject changes from baseline (pre-fasting) to post-fasting time points. Binary logistic regression [the odds ratio (OR), 95% confidence interval (CI)] was calculated considering genetic expression as dependent variables, and sex (male vs. female), caloric intake (high, >2,000 Kcal vs. low, <2,000 Kcal), waist circumference as independent variables. We recoded the waist circumference variable as high waist circumference or low waist circumference as per the corresponding sex of the participant. The following criteria were used: High, ≥ 102 cm vs. Normal <102 cm for men and High, ≥ 88 cm vs. Normal <88 cm for women. Linear regression was used to determine the relationship between the change in FTO gene expression (dependent variable) before and at the end of Ramadan and biochemical and anthropometric variables (as independent variables). All data were tested at a 5% level of significance (P <0.05).
The intra- and inter-assay coefficient variation (CVs) for the tested biomarkers are found through the repository link: https://www.dropbox.com/scl/fi/pghy4x01f6crvy7boihby/Inter-intra-assays-CVs-DNA-15.9.2021.xlsx?dl=0&rlkey=s5j7v8jj5gstdl878fd2k7rgc.
Fifty-seven (17 males and 40 females, mean age of 38.42 years ± 11.18) overweight/obese subjects (BMI = 29.89 ± 5.02 kg/m2) were recruited and monitored before and at the end of fasting the whole month of Ramadan. The majority of subjects were females (about 70%), and most subjects were married (about 83%), university graduates (around 77%), and sedentary (about 91%). About 91% of the study population were from non-Gulf Cooperation Council (GCC) countries (Table 2).
The basic and anthropometric characteristics of the subjects are shown in Table 3. Bodyweight and composition, glucose homeostasis, blood pressure, and inflammatory markers significantly varied between pre-and post-Ramadan fasting, as shown in Tables 4–6. By the end of the Ramadan fasting month, body weight, BMI, fat mass, body fat percent, waist circumference, and hip circumference significantly (P <0.05) reduced when compared to pre-fasting levels. LDL-C, IL-6, and TNF-α were significantly reduced as well (P <0.05) at the end of the fasting month, while HDL-C and interleukin 10 were significantly increased (P <0.05) (Table 5). Changes in dietary intake are shown in Table 4. Significant increases were reported in the dietary intake of total sugars, PUFA, vitamin C, omega-3 fatty acids, lycopene, and vitamin E in comparison with the pre-fasting intakes, while the intake of protein and cholesterol decreases in comparison with the pre-fasting intakes (Table 6). Results of relative genetic expressions in subjects with overweight/obesity showed significant downregulation in the FTO gene expression at the end of Ramadan in comparison with the pre-fasting level, with a percent reduction of about−32% (95% CI–0.052 −0.981) (Figure 1).
Table 3. Basic anthropometric and cardiac function characteristics of the overweight/obese subjects according to sex variable (n = 57).
Table 4. Changes in anthropometric and cardiac functions measured before and at the end of Ramadan for the overweight/obese subjects (n = 57).
Table 5. Changes in glucose homeostasis and inflammatory markers before and at the end of Ramadan for the overweight/obese subjects (n = 57).
Table 6. Changes in the dietary intake before and at the end of Ramadan for the overweight/obese subjects (n = 57).
Figure 1. Relative (in comparison with healthy subjects with normal BMI) FTO gene expression before and at the end of Ramadan intermittent fasting (RIF) month for the overweight/obese subjects. **P < 0.001.
Binary logistic regression analysis for genetic expressions showed no significant (P > 0.05) association between high-energy intake (≥2,000 kcal vs. <2,000 kcal), waist circumference (High, ≥ 102 cm vs. Normal <102 cm for men and High, ≥ 88 cm vs. Normal <88 cm for women), obesity (BMI ≥ 30 vs. BMI <30) and gene expressions of FTO gene (Supplementary Table 1). Linear regression analysis showed a significant, but weak, positive association between the hip circumference and the FTO gene expression at the end of Ramadan fasting days (Supplementary Table 2).
The current study provides the first evidence of a link between RIF and the FTO gene expression in a cohort of overweight/obese subjects who observed Ramadan fasting (28 days for an average of 15 h/day). There was an association between reduced FTO expression and favorable effects, as demonstrated by suppression of pro-inflammatory markers and improvement of the lipid profile. Above all, such an association was also accompanied by a reduction of BMI and waist/hip ratio denoting that the effect of RIF may be explained, at least in part, by its link to the FTO expression. Several human studies showed beneficial effects of IF (17, 19, 21, 44, 45). Recently, a growing body of evidence suggests substantial health implications for the religious form of IF, with the Ramadan model as one of the most extensively studied forms with variable anthropometric, metabolic, and inflammatory impacts (25–29, 31, 32). The main distinctive features of RIF in comparison to other patterns of IF models are presented in the fact that RIF involves diurnal, dawn to sunset, IF for 29–30 consecutive days with complete abstinence from food and drink, including water. Other models of IF include modified fasting regimens (involves consumption of 20–25% of energy needs on scheduled fasting days such as 5:2 diet), TRE (allows ad libitum nutrient and energy intake within specific time frames, and inducing regular, extended fasting intervals, mostly nocturnal fasting), and alternate-day fasting (allows alternating fasting days with eating days) (45).
In the current study, RIF reduced body weight, BMI, body fat percent, waist circumference; RIF also resulted in a reduction of LDL, with increased HDL. This is in support of the previous reports on the favorable effect of RIF on the cardiometabolic risk factor profile. In our recent meta-analysis, we demonstrated the favorable effect of IF on reducing total cholesterol, LDL, triglyceride levels as well as diastolic blood pressure and heart rate (26). The study included subgroup analysis of age, sex, and duration of fasting (as confounding factors) and the significant favorable effect of RIF was constantly evident in all subgroups. Concordant with our findings in the current and previous studies, Mindikoglu et al. showed that RIF resulted in a significant reduction of BMI, waist circumference, and improvement in blood pressure, with an anti-cancer, anti-diabetes, and anti-aging serum proteome response, providing another dimension for the benefits of IF that is likely to be promoted by cytokine modulation (46).
The observed changes in the total energy and dietary intakes from different macro and micronutrients are repeatedly shown in several studies (30, 32), and consistent with the recent work that compared dietary intakes from different food groups and macronutrients in a comparative study using the year-round dietary intakes (23).
FTO expression is different in underfeeding and fasting conditions and displays tissue-specific differences in mouse models of obesity, but it is not known whether these differences are the cause or the consequence of obesity (47). FTO mRNA expression in mice and humans is broadly distributed in many organs, with notably high levels of expression in the brain and hypothalamus, which regulate energy balance and hunger (48, 49). Previous studies showed that tissue-specific genes may be expressed in a wider variety of tissues. When transcriptomics analysis of peripheral blood mononuclear cells (PBMCs) was compared to that of liver, kidney, stomach, spleen, prostate, lung, heart, colon, and brain, more than 80% of shared differentially expressed genes (50). Also, other reports suggested that PBMCs can represent a surrogate indicator in dietary investigations to identify differentially expressed genes in population studies (51, 52). In mice, the expression of FTO may be influenced by their dietary condition (53). When mice are fasting, there is a strong stimulus to eat, and their hypothalamus FTO mRNA expression is significantly reduced compared to their fed counterparts. Supplementation with the anti-hunger hormone leptin does not reverse this effect, which suggests that the reduced hypothalamic FTO expression observed during fasting is independent of leptin levels (54). These findings show that FTO is downregulated during fasting and increased during feeding and that a decrease in FTO expression or activity might be a signal that encourages overeating and obesity. Noteworthy, the results in rats are different; possibly because of inconsistency in the conditions of different studies and the different sensitivity to starvation among different species. Murine FTO gene expression was shown to be downregulated under fasting conditions, suggesting that obese mouse models mimic the fasted state, possibly contributing to their over-eating (47).
Interestingly, FTO was highly expressed in the cerebellum, salivary gland, and kidney of adult pigs, whereas it was not detected in blood (55). The latter study showed FTO was positively associated with energy intake in the pancreas, and with age in the muscle, adding to the multiple factors that affect FTO expression. Such variation can be explained by different metabolic and secretion activities of different tissues at different age groups. Moreover, as previously described with leptin, diurnal variation may also affect the level of expression of FTO (56). The link of FTO expression to fasting and obesity is not yet fully elucidated in humans, where more factors may interplay to determine this effect. Such factors include the effect of food predilection, dietary patterns, and complexity of gut-brain networking including leptin, ghrelin, among other key players (57). Our current study highlights the reduction of FTO in overweight/obese subjects as a consequence of observing diurnal IF for four consecutive weeks.
Furthermore, the current study showed a reduction in both pro-inflammatory cytokines IL6, TNF-α. Concordantly, in a study by Faris et al. significant reductions in IL-6, IL-1β, and TNF-α were reported in fasting subjects during Ramadan of both sexes, when compared to basal pre-fasting values obtained 1 week before Ramadan (32). Furthermore, this finding is consistent with the results of a meta-analysis and original research showing that RIF is associated with significant reductions in serum proinflammatory cytokines (IL-6, IL-1β, and TNF-α) and hs-CRP, and the oxidative stress marker malondialdehyde and urinary 15-f(2t)-isoprostane (32, 33). The current findings on the significant reductions in lipid profile components (TC, LDL, and TG) and increased HDL are consistent with the systematic reviews and meta-analyses showing that RIF is associated with such improvements in the cardiometabolic risk factors (26, 27). As shown by Faris and colleagues (30), these reductions in the proinflammatory cytokines and other inflammatory adipokines were reported to be associated with significant reductions in visceral adiposity in obese subjects who observed the 4-week dawn to sunset IF of Ramadan. Experimentally, fasting reduced TNF-α in visceral white adipose tissue, IL-1β in subcutaneous tissue, as well as insulin and leptin in the plasma in stressed rats (58).
The current study showed that RIF increased IL-10, which is consistent with a previous study by Faris et al. among obese subjects observing RIF when compared with the pre-fasting levels (30). IL-10 has a strong immune-modulation activity (59). It is thought of as an anti-inflammatory cytokine that can suppress cytokine production from macrophages and the function of neutrophils (60, 61) but can activate CD8+ T cells and natural killer (NK) cells for anti-viral immunity, denoting its dual role in immunity (62, 63). Intriguingly, the IL-10 signaling pathway was one of the top Differentially Expressed Genes (DEGs) in COVID-19 infected normal epithelium vs. mock-infected cells (64) and could be, along with the reduction in other metabolic and inflammatory risk factors, involved in the plausible protective effect of Ramadan fasting against the COVID-19 infection (65).
FTO expression did not correlate with high-energy intake, waist circumference, or obesity as shown by the binary logistic regression analysis performed. These findings denote that RIF exerts its beneficial effects independently from the dietary and anthropometric factors, through different pathways that may or may not involve weight reduction and lower energy intake. Such dissociation between the beneficial effect of IF and caloric restriction is supported by previous work on rodents (66) who found that IF has beneficial effects in experimental mice reported on glucose regulation and neuronal resistance to injury that are independent of caloric intake. Several proteins interact with the FTO; the most significant of which is Melanocortin receptor 4 (MCR4) that is co-expressed with the FTO in some species. The MCR4 is involved in energy balance as well as somatic growth (67).
Pharmacologic treatments cannot reset the circadian clock rhythm; thus, there is an urgent need for an effective intervention to reset the circadian clock and prevent metabolic syndrome and metabolic syndrome-induced cancers (68, 69). However, IF practiced exclusively during human activity hours can reset the circadian rhythm. Therefore, resetting the disrupted circadian clock in humans by consecutive daily IF could provide a primary strategy to improve metabolic syndrome and reduce the incidence of metabolic syndrome-induced cancer (68, 69). RIF upregulated several key regulatory proteins that play a key role in tumor suppression, DNA repair, insulin signaling, glucose, and lipid metabolism, circadian clock, cytoskeletal remodeling, immune system, and cognitive function (70).
Nonetheless, our results indicate a lack of association between FTO gene expression and caloric intake by the fasting people. This notion may appear inconsistent with the evident association between intakes of calories, carbohydrates, and fats with the FTO genotype (67), given some studies that showed a correlation of the FTO risk allele and high FTO gene expression (68, 69). Moreover, it has been reported that the FTO genotype may influence dietary macronutrient intakes, body weight, energy balance, appetite, and hormone secretion (70, 71). The SNPs of the FTO gene are likely associated with food intake and obesity through modifying the expression of other genes (72). Until now, there is no strong evidence for the association of FTO A risk allele and level of gene expression. There is a recognized association of the A risk allele of FTO rs9939609 and overweight worldwide (48, 71) in several Arab populations (72–75). In this study, we did not investigate the subjects' genotypes, as the study group is not from the same ethnicity. In our previous study on the Emirati population, the rs9939609 AA genotype was significantly associated with higher BMI; in females, but not in males (76). In another study by our group, subjects with rs9939609 AA genotype showed significantly higher fasting glucose compared to other genotypes, with a trend of higher insulin levels and HOMA2-IR (77). We recently correlated the FTO genotypes, as well as FGF21 genotypes to dietary patterns in the Emirati population (78). Whether the outcome of a caloric intervention is affected by FTO rs 9939609 A risk allele is weakly evident (79).
A few limitations should be considered when interpreting the findings of the current work. First, causality cannot be inferred, as the design is observational prospective in nature. Hence, undetected confounding factors could be involved in the downregulation or upregulation of the tested FTO gene upon RIF. Changes in circadian rhythm and sleep patterns that have been reported to affect the expression of some genes (37) are among the factors that may be implicated in changing FTO gene expression. Tissue- and age-specific variations of FTO expression also add to the complexity of the interpretation of its expression in the blood. Although the practice of physical exercise did not change during Ramadan month in comparison with the pre-fasting stage, still this factor may be of paramount effect, and objective measurements have to be applied in measuring physical exercise levels in the forthcoming studies.
RIF is linked to the downregulation of FTO gene expression in subjects with obesity, which might explain, at least in part, its beneficial metabolic benefits. Consequently, RIF may have a preventive effect against body weight increase and associated negative metabolic-related derangements in overweight/obese people, possibly through modulation of FTO gene expression.
The original contributions presented in the study are included in the article/Supplementary Material, further inquiries can be directed to the corresponding author/s.
The studies involving human participants were reviewed and approved by Research Ethics Committee, University of Sharjah. The patients/participants provided their written informed consent to participate in this study.
MF, MS-A, and MM: conceptualization and validation. MF, MM, and LM: data curation and methodology. DA: formal analysis. MF, MS-A, and WA-R: funding acquisition, supervision, and project administration. MF, MS-A, MM, and LM: investigation. MF and WA-R: resources. MF and WA-R: software. LM, MM, and DA: visualization. MF and MS-A: writing—original draft. MF, MS-A, and WA-R: writing—review and editing. All authors contributed to the article and approved the submitted version.
Vice-Chancellor Research has supported this work and Graduate Studies Office/the University of Sharjah, Grant No. (VCRG/R1061/2016). MS-A was funded by the University of Sharjah targeted Grant (1801090141-P) and the MBRU-Al-Mahmeed Research Award 2019, WA-R was funded by the University of Sharjah Grant 16010501020-P.
The authors declare that the research was conducted in the absence of any commercial or financial relationships that could be construed as a potential conflict of interest.
All claims expressed in this article are solely those of the authors and do not necessarily represent those of their affiliated organizations, or those of the publisher, the editors and the reviewers. Any product that may be evaluated in this article, or claim that may be made by its manufacturer, is not guaranteed or endorsed by the publisher.
The authors express their deep thanks to the volunteers for their commitment and enthusiasm during research execution. Thanks are due to Ms. Arwa Fawzan, Ms. Alzahraa Alchaar, and Ms. Yara Hasibi for their significant assistance in data collection.
The Supplementary Material for this article can be found online at: https://www.frontiersin.org/articles/10.3389/fnut.2021.741811/full#supplementary-material
1. Hruby A, Hu FB. The epidemiology of obesity: a big picture. PharmacoEconomics. (2015) 33:673–89. doi: 10.1007/s40273-014-0243-x
2. GBD 2015 Obesity Collaborators, Afshin A, Forouzanfar MH, Reitsma MB, Sur P, Estep K, et al. Health effects of overweight and obesity in 195 countries over 25 years. N Engl J Med. (2017) 377:13–27. doi: 10.1056/NEJMoa1614362
3. Azevedo PS, Minicucci MF, Zornoff LAM. Obesity: a growing multifaceted problem. Arquivos brasileiros de cardiologia. (2015) 105:448–9. doi: 10.5935/abc.20150133
4. Stanaway JD, Afshin A, Gakidou E, Lim SS, Abate D, Abate KH. Global, regional, and national comparative risk assessment of 84 behavioural, environmental and occupational, and metabolic risks or clusters of risks for 195 countries and territories, 1990–2017: a systematic analysis for the Global Burden of Disease Stu. The Lancet. (2018). 392:1923–94. doi: 10.1016/S0140-6736(18)32225-6
5. Dai H, Alsalhe TA, Chalghaf N, Riccò M, Bragazzi NL, Wu J. The global burden of disease attributable to high body mass index in 195 countries and territories, 1990–2017: an analysis of the global burden of disease study. PLOS Med. (2020) 17:e1003198. doi: 10.1371/journal.pmed.1003198
6. Musaiger AO, Al Hazzaa HM, Al-Qahtani A, Elati J, Ramadan J, AboulElla NA, et al. Strategy to combat obesity and to promote physical activity in Arab countries. Diabet Metabol Syndrome Obesity: Targ Therapy. (2011) 4:89. doi: 10.2147/DMSO.S17322
7. Anderson PM, Butcher KF. Childhood obesity: trends and potential causes. Fut Child. (2006) 2006:19–45. doi: 10.1353/foc.2006.0001
8. Sahoo K, Sahoo B, Choudhury AK, Sofi NY, Kumar R Bhadoria. Childhood obesity: causes and consequences. J Fam Med Prim Care. (2015) 4:187–92. doi: 10.4103/2249-4863.154628
9. Dolton P, Xiao M. The intergenerational transmission of body mass index across countries. Econ Hum Biol. (2017) 24:140–52. doi: 10.1016/j.ehb.2016.11.005
10. Hägg S, Ganna A, Van Der Laan SW, Esko T, Pers TH, Locke AE, et al. Gene-based meta-analysis of genome-wide association studies implicates new loci involved in obesity. Hum Mol Genetics. (2015) 24:6849–60. doi: 10.1093/hmg/ddv379
11. Merkestein M, Laber S, McMurray F, Andrew D, Sachse G, Sanderson J, et al. FTO influences adipogenesis by regulating mitotic clonal expansion. Nat Commun. (2015) 6:1–9. doi: 10.1038/ncomms7792
12. Qi Q, Kilpeläinen TO, Downer MK, Tanaka T, Smith CE, Sluijs I, et al. FTO genetic variants, dietary intake and body mass index: insights from 177 330 individuals. Hum Mol Genetics. (2014) 23:6961–72. doi: 10.1093/hmg/ddu411
13. Qi Q, Downer MK, Kilpeläinen TO, Taal HR, Barton SJ, Ntalla I, et al. Dietary intake, FTO genetic variants, and adiposity: a combined analysis of over 16,000 children and adolescents. Diabetes. (2015) 64:2467–76. doi: 10.2337/db14-1629
14. den Hoed M, Westerterp-Plantenga MS, Bouwman FG, Mariman EC, Westerterp K. Postprandial responses in hunger and satiety are associated with the rs9939609 single nucleotide polymorphism in FTO. Am J Clinic Nutri. (2009) 90:1426–32. doi: 10.3945/ajcn.2009.28053
15. Wardle J, Carnell S, Haworth CM, Farooqi IS, O'Rahilly S, Plomin R. Obesity associated genetic variation in FTO is associated with diminished satiety. J Clinic Endocrinol Metabol. (2008) 93:3640–3. doi: 10.1210/jc.2008-0472
16. Xiang L, Wu H, Pan A, Patel B, Xiang G, Qi L, et al. FTO genotype and weight loss in diet and lifestyle interventions: a systematic review and meta-analysis. Am J Clinic Nutri. (2016) 103:1162–70. doi: 10.3945/ajcn.115.123448
17. de Cabo R, Mattson MP. Effects of intermittent fasting on health, aging, and disease. New Engl J Med. (2019) 381:2541–51. doi: 10.1056/NEJMra1905136
18. Gabel K, Cienfuegos S, Kalam F, Ezpeleta M, Varady K. A time-restricted eating to improve cardiovascular health. Curr Atherosclero Rep. (2021) 23:1–9. doi: 10.1007/s11883-021-00922-7
19. Di Francesco A, Di Germanio C, Bernier M, de Cabo. R. A time to fast. Science. (2018) 362:770–5. doi: 10.1126/science.aau2095
20. Wilhelmi de Toledo F, Grundler F, Sirtori CR, Ruscica M. Unravelling the health effects of fasting: a long road from obesity treatment to healthy life span increase and improved cognition. Annal Med. (2020) 52:147–61. doi: 10.1080/07853890.2020.1770849
21. Patterson RE, Laughlin GA, Sears DD, LaCroix AZ, Marinac C, Gallo LC, et al. Intermittent fasting and human metabolic health. J Acad Nutri Dietetics. (2015) 115:1203–12. doi: 10.1016/j.jand.2015.02.018
22. Abdelrahim D, Faris ME, Hassanein M, Shakir AZ, Yusuf AM, Almeneessier AS, et al. Impact of Ramadan diurnal intermittent fasting on hypoglycemic events in patients with type 2 diabetes: a systematic review of randomized controlled trials and observational studies. Front Endocrinol. (2021) 12:4423. doi: 10.3389/fendo.2021.624423
23. Shatila H, Baroudi M, Ahmad RES, Chehab R, Forman MR, Abbas N, et al. Impact of Ramadan fasting on dietary intakes among healthy adults: a year-round comparative study. Front Nutri. (2021) 21:689788. doi: 10.3389/fnut.2021.689788
24. Faris ME, Jahrami HA, Alhayki FA, Alkhawaja NA, Ali AM, Aljeeb SH, et al. Effect of diurnal fasting on sleep during Ramadan: a systematic review and meta-analysis. Sleep Breath. (2020). 24:771–82. doi: 10.1007/s11325-019-01986-1
25. Al-Rawi N, Madkour M, Jahrami H, Salahat D, Alhasan F, BaHammam A, et al. Effect of diurnal intermittent fasting during Ramadan on ghrelin, leptin, melatonin, and cortisol levels among overweight and obese subjects: A prospective observational study. PloS ONE. (2020) 15:e0237922. doi: 10.1371/journal.pone.0237922
26. Jahrami HA, Faris ME, Janahi AI, Janahi MI, Abdelrahim DN, Madkour MI, et al. Does four-week consecutive, dawn-to-sunset intermittent fasting during ramadan affect cardiometabolic risk factors in healthy adults? a systematic review, meta-analysis, and meta-regression. Nutri Metabol Cardiovascul Dis. (2021) 21:2. doi: 10.1016/j.numecd.2021.05.002
27. Faris ME, Jahrami HA, Alsibai J, Obaideen AA. Impact of Ramadan diurnal intermittent fasting on the metabolic syndrome components in healthy, non-athletic Muslim people aged over 15 years: a systematic review and meta-analysis. Br J Nutri. (2020) 123:1–22. doi: 10.1017/S000711451900254X
28. Jahrami HA, Alsibai J, Clark CC, Faris ME. A systematic review, meta-analysis and meta-regression of the impact of diurnal intermittent fasting during Ramadan on body weight in healthy subjects aged 16 years and above. Euro J Nutri. (2020) 20:161. doi: 10.1007/s00394-020-02216-1
29. Fernando H, Zibellini J, Harris RA, Seimon RV, Sainsbury A. Effect of Ramadan fasting on weight and body composition in healthy non-athlete adults: a systematic review and meta-analysis. Nutrients. (2019) 11:478. doi: 10.3390/nu11020478
30. Faris ME, Madkour MI, Obaideen AK, Dalah EZ, Hasan HA, Radwan H, et al. Effect of Ramadan diurnal fasting on visceral adiposity and serum adipokines in overweight and obese individuals. Diabet Res Clinic Pract. (2019) 153:166–175. doi: 10.1016/j.diabres.2019.05.023
31. Faris ME, Madkour MI, Obaideen AK, Dalah EZ, Hasan HA, Radwan H, et al. Impact of diurnal intermittent fasting during Ramadan on inflammatory and oxidative stress markers in healthy people: Systematic review and meta-analysis. J Nutri Intermed Metabol. (2019) 15:18–26. doi: 10.1016/j.jnim.2018.11.005
32. Faris ME, Kacimi S, Ref'at A, Fararjeh MA, Bustanji YK, Mohammad MK, et al. Intermittent fasting during Ramadan attenuates proinflammatory cytokines and immune cells in healthy subjects. Nutri Res. (2012) 32:947–55. doi: 10.1016/j.nutres.2012.06.021
33. Faris ME, Hussein RN, Al-Kurd RAA, Al-Fararjeh MA, Bustanji YK, Mohammad MK. Impact of Ramadan intermittent fasting on oxidative stress measured by urinary 15–isoprostane. J Nutri Metabol. (2012) 12:80924. doi: 10.1155/2012/802924
34. Faris ME, Jahrami H, Abdelrahim D, Bragazzi N, BaHammam A. The effects of Ramadan intermittent fasting on liver function in healthy adults: a systematic review, meta-analysis, and meta-regression. Diabet Res Clinic Pract. (2021) 178:108951. doi: 10.1016/j.diabres.2021.108951
35. Franzago M, Santurbano D, Vitacolonna E, Stuppia L. Genes and diet in the prevention of chronic diseases in future generations. Int J Mol Sci. (2020) 21:2633. doi: 10.3390/ijms21072633
36. Madkour MI, El-Serafi AT, Jahrami HA, Sherif NM, Hassan RE, Awadallah S. Ramadan diurnal intermittent fasting modulates SOD2, TFAM, Nrf2, sirtuins (SIRT1. SIRT3) gene expressions in subjects with overweight and obesity. Diabetes Res Clinic Pract. (2019) 2019:107801. doi: 10.1016/j.diabres.2019.107801
37. Ajabnoor GM, Bahijri S, Shaik NA, Borai A, Alamoudi AA, Al-Aama JY, et al. Ramadan fasting in Saudi Arabia is associated with altered expression of CLOCK, DUSP and IL-1alpha genes, as well as changes in cardiometabolic risk factors. PloS One. (2017) 12:e0174342. doi: 10.1371/journal.pone.0174342
38. Meng Y, Lohse B, Cunningham-Sabo L. Sex modifies the association between the CLOCK variant rs1801260 and BMI in school-age children. PloS One. (2020) 15:e0236991. doi: 10.1371/journal.pone.0236991
39. Ismail S, Manaf RA, Mahmud A. Comparison of time-restricted feeding and Islamic fasting: a scoping review. East Mediterr Health J. (2019) 2:11. doi: 10.26719/emhj.19.011
40. Otten J, Hellwig J, Meyers L. Institute of Medicine. 2006. Dietary Reference Intakes: The Essential Guide to Nutrient Requirements. Washington, DC: The National Academies Press (2006).
41. Livak KJ, Schmittgen TD. Analysis of relative gene expression data using real-time quantitative PCR and the 2– ΔΔCT method. Methods. (2001) 25:402–408. doi: 10.1006/meth.2001.1262
42. Maher S, Kolieb E, Sabik NA, Abd-Elhalim D, El-Serafi AT, El-Wazir Y. Comparison of the osteogenic differentiation potential of mesenchymal cells isolated from human bone marrow, umbilical cord blood and placenta derived stem cells. Beni-Suef Univ J Basic Appl Sci. (2015) 4:80–5. doi: 10.1016/j.bjbas.2015.02.011
43. Von Elm E, Altman DG, Egger M, Pocock SJ, Gøtzsche PC, Vandenbroucke JP. The Strengthening the Reporting of Observational Studies in Epidemiology (STROBE) statement: guidelines for reporting observational studies. Annals Intern Med. (2007) 147:573–7. doi: 10.7326/0003-4819-147-8-200710160-00010
44. Mattson MP, de Cabo R. Effects of intermittent fasting on health, aging, and disease. New Engl J Med. (2020) 382:1773. doi: 10.1056/NEJMc2001176
45. Patterson RE, Sears DD. Metabolic effects of intermittent fasting. Ann Rev Nutri. (2017) 37:371–393. doi: 10.1146/annurev-nutr-071816-064634
46. Mindikoglu AL, Abdulsada MM, Jain A, Jalal PK, Devaraj S, Wilhelm ZR, et al. Intermittent fasting from dawn to sunset for four consecutive weeks induces anticancer serum proteome response and improves metabolic syndrome. Scientific Rep. (2020) 10:18341–41. doi: 10.1038/s41598-020-73767-w
47. Fawcett KA, Barroso I. The genetics of obesity: FTO leads the way. Trends in genetics : TIG. (2010) 26:266–274. doi: 10.1016/j.tig.2010.02.006
48. Frayling TM, Timpson NJ, Weedon MN, Zeggini E, Freathy RM, Lindgren CM, et al. A common variant in the FTO gene is associated with body mass index and predisposes to childhood and adult obesity. Science. (2007) 316:889–94. doi: 10.1126/science.1141634
49. Scott LJ, Mohlke KL, Bonnycastle LL, Willer CJ, Li Y, Duren WL, et al. A genome-wide association study of type 2 diabetes in Finns detects multiple susceptibility variants. Science. (2007) 316:1341–5. doi: 10.1126/science.1142382
50. Liew C-C, Ma J, Tang HC, Zheng R, Dempsey AA. The peripheral blood transcriptome dynamically reflects system wide biology: a potential diagnostic tool. J Lab Clinic Med. (2006) 147:126–32. doi: 10.1016/j.lab.2005.10.005
51. Rudkowska I, Raymond C, Ponton A, Jacques H, Lavigne C, Holub BJ, et al. Validation of the use of peripheral blood mononuclear cells as surrogate model for skeletal muscle tissue in nutrigenomic studies. Omics: J Integrat Biol. (2011) 15:1–7. doi: 10.1089/omi.2010.0073
52. Hofmann T, Klenow S, Borowicki A, Gill CI, Pool-Zobel BL, Glei M. Gene expression profiles in human peripheral blood mononuclear cells as biomarkers for nutritional in vitro and in vivo investigations. Genes Nutri. (2010) 5:309–19. doi: 10.1007/s12263-010-0170-1
53. Fredriksson R, Hägglund M, Olszewski PK, Stephansson O, Jacobsson JA, Olszewska AM, et al. The obesity gene, FTO, is of ancient origin, up-regulated during food deprivation and expressed in neurons of feeding-related nuclei of the brain. Endocrinology. (2008) 149:2062–71. doi: 10.1210/en.2007-1457
54. Stratigopoulos G, Padilla SL, LeDuc CA, Watson E, Hattersley AT, McCarthy MI, et al. Regulation of Fto/Ftm gene expression in mice and humans. Am J Physiol-Regulatory Integrat Comparat Physiol. (2008) 294:R1185–96. doi: 10.1152/ajpregu.00839.2007
55. Ferenc K, PilŽys T, Garbicz D, Marcinkowski M, Skorobogatov O, Dylewska M, et al. Intracellular and tissue specific expression of FTO protein in pig: changes with age, energy intake and metabolic status. Scientific Rep. (2020) 10:1–17. doi: 10.1038/s41598-020-69856-5
56. Kettner NM, Mayo SA, Hua J, Lee C, Moore DD, Fu L. Circadian dysfunction induces leptin resistance in mice. Cell Metabol. (2015) 22:448–59. doi: 10.1016/j.cmet.2015.06.005
57. Mizuno TM. Fat mass and obesity associated (FTO) gene and hepatic glucose and lipid metabolism. Nutrients. (2018) 10:1600. doi: 10.3390/nu10111600
58. Speaker KJ, Paton MM, Cox SS, Fleshner M. A single bout of fasting (24 h) reduces basal cytokine expression and minimally impacts the sterile inflammatory response in the white adipose tissue of normal weight f344 rats. Mediat Inflamm. (2016) 2016:1–13. doi: 10.1155/2016/1698071
59. Murray PJ. The primary mechanism of the IL-10-regulated antiinflammatory response is to selectively inhibit transcription. Proceed Nat Acad Sci. (2005) 102:8686–91. doi: 10.1073/pnas.0500419102
60. Krause P, Morris V, Greenbaum JA, Park Y, Bjoerheden U, Mikulski Z, et al. IL-10-producing intestinal macrophages prevent excessive antibacterial innate immunity by limiting IL-23 synthesis. Nat Commun. (2015) 6:1–12. doi: 10.1038/ncomms8055
61. Kessler B, Rinchai D, Kewcharoenwong C, Nithichanon A, Biggart R, Hawrylowicz CM, et al. Interleukin 10 inhibits pro-inflammatory cytokine responses and killing of Burkholderia pseudomallei. Scientific Rep. (2017) 7:1–11. doi: 10.1038/srep42791
62. Mocellin S, Panelli MC, Wang E, Nagorsen D, Marincola FM. The dual role of IL-10. Trends Immunol. (2003) 24:36–43. doi: 10.1016/S1471-4906(02)00009-1
63. Couper KN, Blount DG, Riley EM. IL-10: the master regulator of immunity to infection. J Immunol. (2008) 180:5771–7. doi: 10.4049/jimmunol.180.9.5771
64. Al Heialy S, Hachim MY, Senok A, Gaudet M, Abou Tayoun A, Hamoudi R, et al. Regulation of angiotensin- converting enzyme 2 in obesity: implications for COVID-19. Front Physiol. (2020) 11:1194. doi: 10.3389/fphys.2020.555039
65. Faris ME, Salem ML, Jahrami HA, Madkour MI, BaHammam AS. Ramadan intermittent fasting and immunity: an important topic in the era of COVID-19. Annal Thoracic Med. (2020) 15:125. doi: 10.4103/atm.ATM_151_20
66. Anson RM, Guo Z, de Cabo R, Iyun T, Rios M, Hagepanos A, et al. Intermittent fasting dissociates beneficial effects of dietary restriction on glucose metabolism and neuronal resistance to injury from calorie intake. Proceed Nat Acad Sci. (2003) 100:6216–6220. doi: 10.1073/pnas.1035720100
67. Szklarczyk D, Morris JH, Cook H, Kuhn M, Wyder S, Simonovic M, et al. The STRING database in 2017: quality-controlled protein–protein association networks, made broadly accessible. Nucleic Acids Res. (2016) 2016:gkw937. doi: 10.1093/nar/gkw937
68. Pothiwala P, Jain SK, Yaturu S. Metabolic syndrome and cancer. Metabol Syndrome Relat Disord. (2009) 7:279–88. doi: 10.1089/met.2008.0065
69. Li X-M, Delaunay F, Dulong S, Claustrat B, Zampera S, et al. Cancer inhibition through circadian reprogramming of tumor transcriptome with meal timing. Cancer Res. (2010) 70:3351–60. doi: 10.1158/0008-5472.CAN-09-4235
70. Mindikoglu AL, Abdulsada MM, Jain A, Choi JM, Jalal PK, Devaraj S, et al. Intermittent fasting from dawn to sunset for 30 consecutive days is associated with anticancer proteomic signature and upregulates key regulatory proteins of glucose and lipid metabolism, circadian clock, DNA repair, cytoskeleton remodeling, immune system and cognitive function in healthy subjects. J Proteomics. (2020) 217:103645. doi: 10.1016/j.jprot.2020.103645
71. Hunt SC, Stone S, Xin Y, Scherer CA, Magness CL, Iadonato SP, et al. Association of the FTO gene with BMI. Obesity. (2008) 16:902–904. doi: 10.1038/oby.2007.126
72. Cyrus C, Ismail MH, Chathoth S, Vatte C, Hasen M, Al Ali A. Analysis of the impact of common polymorphisms of the FTO and MC4R genes with the risk of severe obesity in Saudi Arabian population. Genetic Test Mol Biomark. (2018) 22:170–7. doi: 10.1089/gtmb.2017.0218
73. Al-Serri A, Al-Bustan SA, Kamkar M, Thomas D, Alsmadi O, Al-Temaimi R, et al. Association of FTO rs9939609 with obesity in the Kuwaiti population: a public health concern? Med Princip Pract. (2018) 27:145–51. doi: 10.1159/000486767
74. Khan SM, El Hajj Chehadeh S, Abdulrahman M, Osman W, Al Safar H. Establishing a genetic link between FTO and VDR gene polymorphisms and obesity in the Emirati population. BMC Med Genetics. (2018) 19:1–9. doi: 10.1186/s12881-018-0522-z
75. Sabarneh A, Ereqat S, Cauchi S, AbuShamma O, Abdelhafez M, Ibrahim M, et al. Common FTO rs9939609 variant and risk of type 2 diabetes in Palestine. BMC Med Genet. (2018) 19:1–6. doi: 10.1186/s12881-018-0668-8
76. Saber-Ayad M, Manzoor S, Radwan H, Hammoudeh S, Wardeh R, Ashraf A, et al. The FTO genetic variants are associated with dietary intake and body mass index amongst Emirati population. PloS ONE. (2019) 14:e0223808. doi: 10.1371/journal.pone.0223808
77. Saber-Ayad M, Manzoor S, El Serafi A, Mahmoud I, Hammoudeh S, Rani A, et al. The FTO rs9939609 “A” allele is associated with impaired fasting glucose and insulin resistance in Emirati population. Gene. (2019) 681:93–98. doi: 10.1016/j.gene.2018.09.053
78. Naja F, Itani L, Hammoudeh S, Manzoor S, Abbas N, Radwan H, et al. Dietary Patterns and their associations with the FTO and FGF21 gene variants among Emirati adults. Front Nutri. (2021) 8:211. doi: 10.3389/fnut.2021.668901
79. Dorling JL, Belsky DW, Racette SB, Das SK, Ravussin E, Redman LM, et al. Association between the FTO rs9939609 single nucleotide polymorphism and dietary adherence during a 2-year caloric restriction intervention: exploratory analyses from CALERIE™ phase 2. Experiment Gerontol. (2021) 2021:111555. doi: 10.1016/j.exger.2021.111555
Keywords: caloric restriction, intermittent fasting, obesity, nutrigenomics, Ramadan, time-restricted eating, gene expression, Middle East
Citation: Madkour MI, Malhab LJB, Abdel-Rahman WM, Abdelrahim DN, Saber-Ayad M and Faris ME (2022) Ramadan Diurnal Intermittent Fasting Is Associated With Attenuated FTO Gene Expression in Subjects With Overweight and Obesity: A Prospective Cohort Study. Front. Nutr. 8:741811. doi: 10.3389/fnut.2021.741811
Received: 15 July 2021; Accepted: 20 December 2021;
Published: 17 March 2022.
Edited by:
Anne Marie Minihane, University of East Anglia, United KingdomReviewed by:
Anestis Dougkas, Institut Paul Bocuse, FranceCopyright © 2022 Madkour, Malhab, Abdel-Rahman, Abdelrahim, Saber-Ayad and Faris. This is an open-access article distributed under the terms of the Creative Commons Attribution License (CC BY). The use, distribution or reproduction in other forums is permitted, provided the original author(s) and the copyright owner(s) are credited and that the original publication in this journal is cited, in accordance with accepted academic practice. No use, distribution or reproduction is permitted which does not comply with these terms.
*Correspondence: Maha Saber-Ayad, bXNhYmVyQHNoYXJqYWguYWMuYWU=; MoezAlIslam E. Faris, bWZhcmlzQHNoYXJqYWguYWMuYWU=
Disclaimer: All claims expressed in this article are solely those of the authors and do not necessarily represent those of their affiliated organizations, or those of the publisher, the editors and the reviewers. Any product that may be evaluated in this article or claim that may be made by its manufacturer is not guaranteed or endorsed by the publisher.
Research integrity at Frontiers
Learn more about the work of our research integrity team to safeguard the quality of each article we publish.