- 1School of Applied Health Sciences and Wellness, Division of Exercise Physiology, Ohio University, Athens, OH, United States
- 2College of Education, Department of Physical Education, Qatar University, Doha, Qatar
Tart cherries possess properties that may reduce inflammation and improve glycemic control, however human data on supplementation and the gut microbiota is equivocal. Processing (i.e., juice concentrate, dried, frozen) may affect the properties of tart cherries, and therefore alter their efficacious health benefits. Therefore, the purpose of this study was to investigate the effect of 30 days of supplementation with Montmorency tart cherry (MTC) in concentrate or freeze-dried form on the gut microbiome and markers of inflammation and glycemic control. Healthy participants with no known disease (n = 58, age: 28 ± 10 y, height: 169.76 ± 8.55 cm, body mass: 72.2 ± 12.9 kg) were randomly allocated to four groups and consumed either concentrate or freeze-dried capsules or their corresponding placebos for 30 days. Venous blood samples were drawn at baseline, day 7, 14, and 30 and analyzed for inflammatory markers TNF-alpha, uric acid, C-reactive protein, and erythrocyte sedimentation rate and glycemic control markers glycated albumin, glucose and insulin. A fecal sample was provided at baseline, day 14 and 30 for microbiome analysis. TNF-alpha was significantly lower at 30 vs. 14 days (p = 0.01), however there was no other significant change in the inflammatory markers. Insulin was not changed over time (p = 0.16) or between groups (p = 0.24), nor was glycated albumin different over time (p = 0.08) or between groups (p = 0.56), however glucose levels increased (p < 0.001) from baseline (4.79 ± 1.00 mmol·L−1) to 14 days (5.21 ± 1.02 mmol·L−1) and 30 days (5.61 ± 1.22 mmol·L−1) but this was no different between groups (p = 0.33). There was no significant change in composition of bacterial phyla, families, or subfamilies for the duration of this study nor was there a change in species richness. These data suggest that 30 days of MTC supplementation does not modulate the gut microbiome, inflammation, or improve glycemic control in a healthy, diverse group of adults.
Clinical Trail Registration: https://clinicaltrials.gov/ct2/show/NCT04467372, identifier: NCT04467372.
Introduction
A considerable amount of research has focused on the use of foods and supplements containing anthocyanins and polyphenols to reduce disease risk by mitigating inflammation and improving blood glucose regulation. Globally the incidence of type 2 diabetes is rising and is predicted to rise to nearly 700 million by 2,045 (1). Evidence suggests a diet high in polyphenols and or supplementation with polyphenol-containing supplements can improve risk of developing type 2 diabetes (2), which may be in part be due to the gut microbiota (3). Polyphenols are often found in fruits, spices and herbs, vegetables, and drinks. The microbiota are known to metabolize many polyphenols, altering their bioavailability (4), providing energy and metabolites to surrounding enterocytes, and decreasing systemic inflammation (5). While the gut microbiota of adults is typically constant, changes have been observed from dietary interventions (6), including with polyphenol supplementation. A variety of polyphenol containing substances have been investigated for their role in modulating the microbiota, including cocoa, red wine, powdered blueberries, grape seed extract, and tart cherries, with mixed findings. For example, 4 weeks of supplementing with a high-cocoa flavanol supplement increased bifidobacterial and lactobacilli population, while also decreasing C-reactive protein levels, which was primarily driven by the increased lactobacilli counts (7). Similar findings have been found with red wine consumption for 4 weeks, with increases in bifidobacterial counts driving a decrease in C-reactive protein (8). These authors also found red wine ingestion increased Bacteroides counts. Yamakoshi et al. (9) found 14 days of supplementing with proanthocyanidin-rich grape seed extract increased Bifidobacterium bacterial counts, findings corroborated by Vendrame (10) after 6 weeks of powdered wild blueberry supplementation. This study is particularly interesting, because the authors concluded that the added fiber and high polyphenol content of the powdered supplement led to selective increases in Bifidobacterium (10), which may also occur with freeze-dried powdered Montmorency tart cherry (MTC) supplementation. However, a more recent study investigating MTC concentrate supplementation for 30 days failed to find any changes in bacteria counts. These authors also noted very low levels of Bifidobacterium and Lactobacilli spp. at baseline, which may have led to the lack of significant change. However, Mayta-Apaza found only 5 days of supplementing with MTC concentrate increased Bacteroidetes and decreased Firmicutes in those who had low Bacteroidetes counts at baseline (11). The significance of these changes lies in the fact that Bifidobacteria and Lactobacillus are both known to be probiotics, with a plethora of health benefits (12), while many species of Bacteroidetes are important for digestion and a decrease in their relative abundance along with increases in Firmicutes has been seen in obesity (13). Clearly there are equivocal findings in the literature related to gut microbiome changes with MTC that need further exploration given the potential health impacts. In addition, results of polyphenol supplementation studies are equivocal and assessing different formulations is warranted.
MTC concentrate and freeze-dried powder are known to have high levels of proanthocyanidins, phenolics and antioxidant capacity (14). Unfortunately, there has been no comparison between formulations of tart cherry supplements (freeze-dried powder, concentrate, juice, whole) on inflammation and the gut microbiome in vivo. This is particularly important in the realm of inflammation research because sugar is known to increase the inflammatory response (15, 16) and the freeze-dried powder form of MTC supplements is naturally low in sugar (<1 g) while the juice (variable, depending on formulation) and concentrate (15+ g) are considerably higher. Furthermore, the addition of the skins in freeze-dried cherry powder supplements would increase fiber content and provide polysaccharides as an energy source to Bacteroides (17). While studies of tart cherry have been equivocal on changes in markers of inflammation with variable findings for changes in uric acid (18–23), and C-reactive protein (19–22, 24, 25), investigating the role the gut microbiome may have in modulating the inflammatory response is warranted. In terms of glucose regulation, MTC extract treatment has been shown to inhibit key enzymes in carbohydrate digestion activity, while increasing translocation of glucose transporters, thus improving insulin sensitivity in an in-vitro model (26). However, the published human investigations of MTC and glucose regulation in healthy populations have been equivocal. For example, Lear (25) found no change in fasting glucose or insulin, but the Matsuda index, a marker of insulin sensitivity, decreased after 30 days of supplementation, while Desai (27) found no change in glucose and Chai (28) actually found an increase in glucose and decrease in insulin levels after MTC supplementation. Given these equivocal findings on changes in inflammation and glucose regulation with MTC supplementation, further investigation is warranted.
Therefore, the purpose of this study was to examine the effects of 30 days of supplementing with MTC concentrate or freeze-dried powder on the gut microbiome, inflammation, and glucose regulation. We hypothesized that the polyphenols in the MTC products would influence the gut microbiome composition, which would modulate changes in inflammatory markers and glucose regulation.
Materials and Methods
Participants
This study was approved by the Institutional Review Board at Ohio University (IRB# 18-F-13) and written informed consent was obtained from each respondent prior to entering the study. Participants were recruited with a University-wide email and flyers hung on campus and in the community, then pre-screened for inclusion/exclusion criteria via an online survey and eligibility was confirmed at the baseline visit. Inclusion criteria included being aged 18–50 years, not pregnant, not diabetic, with no unresolved infections or diseases (diabetes, cardiovascular disease, inflammatory or autoimmune disease), and non-smokers. Participants were also free from prescribed anti-inflammatory and corticosteroid use for at least 2 months and had not taken antibiotics within the last year. See Figure 1 for the CONSORT diagram of recruitment and retention and Table 1 for participant demographics.
Experimental Procedures
This study was a double-blind randomized control study. Participants completed five total visits for this study. The first visit was to obtain informed consent and explain the procedures thoroughly. The remaining four visits were scheduled for blood draw, blood pressure assessment (Omron HEM-711), body composition assessment (Bioelectrical impedance, InBody USA), and fecal sample collection. These occurred in the morning after a 10 h fast at baseline, and after 7, 14, and 30 days of supplementation. See Figure 2 for testing schematic.
Supplementation Protocol
MTC juice was prepared by diluting 1 fluid ounce of concentrate (King Orchards, Traverse City, MI) with 7 fluid ounces of filtered water in accordance with manufacturer instructions. The placebo was a visually similar carbohydrate- and calorie-matched placebo beverage. Participants were provided with 14, 240 ml (8 oz.) bottles of juice per week for 4 weeks. They were instructed to keep the juice refrigerated until consumption, to shake well at consumption, and to drink two bottles per day, ~8 h apart and not within an hour of exercise. Each bottle of MTC juice contained 77 kcal, 18 g carbohydrate and 0 g fiber and the equivalent of 0.5 lb of MTC per serving, while each bottle of placebo juice contained 67 kcal, 17 g carbohydrate and 0 g fiber. MTC capsules (King Orchards, Traverse City, MI) contained 500 mg of freeze-dried tart cherries, while placebo capsules contained 460 mg cornstarch. Participants were provided with an undisclosed number of capsules and instructed to take two capsules with breakfast each day and to return unused capsules at their next visit. Each MTC capsule contained 1.7 kcal, 0.4 g carbohydrate and 0.2 g fiber and contained 0.92 ounces of cherry skins and pulp. Each placebo capsule contained 1.8 kcal, 0.4 g carbohydrate and 0 g fiber. Independent lab analysis of anthocyanins via high-performance liquid chromatography and total polyphenols via Ultraviolet-Visible Spectroscopy (Certified Laboratories, Mellville, NY) showed MTC juice provided 227 mg anthocyanins and 793 mg total polyphenols per 240 ml bottle while MTC capsules provided 330 mg anthocyanins and 760 mg total polyphenols per capsule. These values are within the ranges reported by previous studies using MTC products (29). Doses were in accordance with previous literature and to ensure similar levels of polyphenols and anthocyanins between formulations. Two capsules were taken at the same time of day rather than spread out for easier compliance. Compliance for both juices was 100%, while it was 92% for placebo capsules and 94% for MTC capsules.
Diet and Exercise Tracking
Following the consent visit and prior to their baseline blood draw, participants completed a 12 month food frequency questionnaire (FFQ) that included portion sizes (Dietary History Questionnaire (DHQ) III, National Cancer Institute) to determine typical dietary patterns (i.e., high, low, or normal intake of carbohydrate, protein, fat, and fiber based on established dietary recommendations). In addition to the FFQ, between the 14 and 30 day visits, participants completed a 3 day food record for 2 weekdays and 1 weekend day, tracking food and beverage intake along with portion sizes. Participants recorded their data in Food Prodigy, a companion program to the Food Processor Nutrition Analysis software (ESHA Research). Data from the 3 days was then exported from the Food Processor Nutrition Analysis program as an excel file for each participant. Finally, every 7 days during the course of the study participants completed an online survey regarding their exercise and dietary habits for the previous 7 days as well as the frequency of their intake of alcohol, anti-inflammatory medications, and the top 100 polyphenol containing foods.
Gut Microbiome Analysis
Fecal samples were collected by participants into a toilet hat (Protocult 100, Ability Building Center, Minneapolis, MN) and aliquoted into three Polypropylene microcentrifuge tubes that were free from DNase/RNase and pyrogens (Eppendorf™ Biopur®). Samples were immediately frozen at −20°C until delivery to the laboratory where they were stored at −80°C. Samples were transported to the lab on ice (Utek, Sonoco ThermoSafe, Arlington Heights, IL) in a thermal insulated tote (Hopkins Medical Products Caledonia, MI).
DNA was extracted from fecal samples using the Qiagen DNeasy PowerSoil kit (Qiagen, 12888-100) per manufacturer's instructions. Isolated genomic DNA was amplified using custom designed primers targeting the 16s rDNA V3-V4 regions with Kapa Biosystems HiFi HotStart ReadyMix (Roche, KK2601). Amplified products were checked for the correct size using the Agilent 2100 Bioanalyzer on a DNA 1000 chip (Agilent, 5067-1504). Each amplified product was dual indexed using Illumina Nextera XT v2 indices (Illumina, FC-131-200X) according to manufacturer's instructions. Prepared libraries were checked for correct sizing and overall quality using the Agilent 2100 Bioanalyzer on a DNA 1000 chip (Agilent, 5067-1504). Libraries were quantified using a Qubit 3.0 Fluorometer (Invitrogen, Q32850) and pooled equimolarly. Prepared libraries were mixed with 10% PhiX and sequenced on an Illumina MiSeq using a 2 × 300 bp (600 cycle) paired-end sequencing kit (Illumina, MS-102-3003). Sequencing reads were downloaded from the BaseSpace server in FASTQ format. Reads were demultiplexed and adaptors removed. Operational Taxonomic Units (OTUs) were generated using QIIME 2.0 and identified against the SILVA database. Species richness and diversity were calculated with the Shannon index and Pielou's evenness (Supplementary Figure). The datasets presented in this study can be found at https://www.ncbi.nlm.nih.gov/bioproject/PRJNA742936.
Blood Sample Analysis
Venous blood samples were collected from an antecubital vein into Ethylenediaminetetraacetic acid (EDTA) and serum separator tubes (SST) immediately before and after 7, 14, and 30 days of supplementation. One milliliter of EDTA blood was used to quantify erythrocyte sedimentation rate (ESR) by the Westegren method (Sedi-Rate, Globe Scientific, Inc., Mahwah, NJ). The remaining EDTA blood was stored at 4°C until centrifugation. SST tubes were stored at room temperature for 30 min then centrifuged with EDTA tubes for 15 min (2,500 × g) and 4°C after which supernatants were collected and stored at −80°C for later analysis. Two milliliter serum were sent to an outside facility (Pathology Laboratories, Inc., Toledo, OH) for measurement of uric acid (UA) and C-reactive protein (CRP). Remaining serum samples were assayed in house in duplicate for TNF-a (BMS223HS, Invitrogen, ThermoFisher Scientific, Vienna, Austria), insulin (Catalog #90095, Crystal Chem, Elk Grove, IL), glucose (Item #120003100P, Eton Bioscience, San Diego, CA), and glycated albumin (Catalog # IT3979, G-Biosciences, St. Louis MO). HOMA-IR was calculated as (insulin x glucose)/405 (30). Reference range for CRP was 0.00–0.744 mg·dL−1 and 3.5 and 7.2 mg·dL−1 for males and females for UA.
Statistical Analysis
A priori power analyses for between and within study design to attain 95% power and an alpha of 0.5 were conducted. For microbiota changes, 12 participants per group were needed for an effect size of 1.32 (10, 11). For UA, CRP, and ESR 12 participants per group were needed for an effect size of 0.72 (22), and 0.72 (18, 23), and 0.63 (23), respectively. For glucose a sample size of 12 was needed for an effect size of 0.75 and for insulin a sample size of 16 was needed for an effect size of 0.58 (28). Statistical analysis was completed using Statistical Package for the Social Sciences (SPSS; SPSS 26.0, Chicago, IL, USA). Linear mixed models were used to examine the main effects of time, and treatment, and the interaction effect (time x treatment). For microbiome composition, data was analyzed overall for change in OTUs between groups at baseline, 14 and 30 days. To overcome interindividual variability, a secondary analysis was conducted where participants were divided into groups based on their baseline levels of high (≥10%; n = 23) or low (<10%; n = 35) levels of Bacteroides because they have been shown to be the primary genus impacted by in vitro tart cherry treatment (11). Different covariance structures were systematically fit to the data, and the one that minimized the Hurvich and Tsai's criterion was chosen for the final model. Where a significant F ratio was observed, post-hoc comparisons with LSD-adjusted p-values were used to identify which pairs of means were significantly different. Normality and homogeneity of variance of the residuals were checked using Q-Q plots, and scatter plots, respectively, and deemed plausible in each instance. Two-tailed statistical significance was accepted as p < 0.05. Data are represented as mean ± SD.
Results
Gut Microbiome
There was no significant change in bacterial OTUs over time (F = 0.70, p = 0.50) or between groups (F = 0.19, p = 0.91). When participants were divided by high or low levels of Bacteroides at baseline, there was a significant main effect for time (F = 4.10, p = 0.02) with greater Bacteroides at baseline vs. 14 days (p = 0.05) and 30 days (p = 0.01) and a significant interaction for time and Bacteroides level (F = 7.68, p = 0.001). However, for Firmicutes after dividing between high or low levels of Bacteroides there was no significant main effect for time (F = 0.60, p = 0.55) or group (F = 2.00, p = 0.16). Finally, there were no significant changes in any bacteria phyla or species over time or between groups. OTU data can be seen in Figure 3.
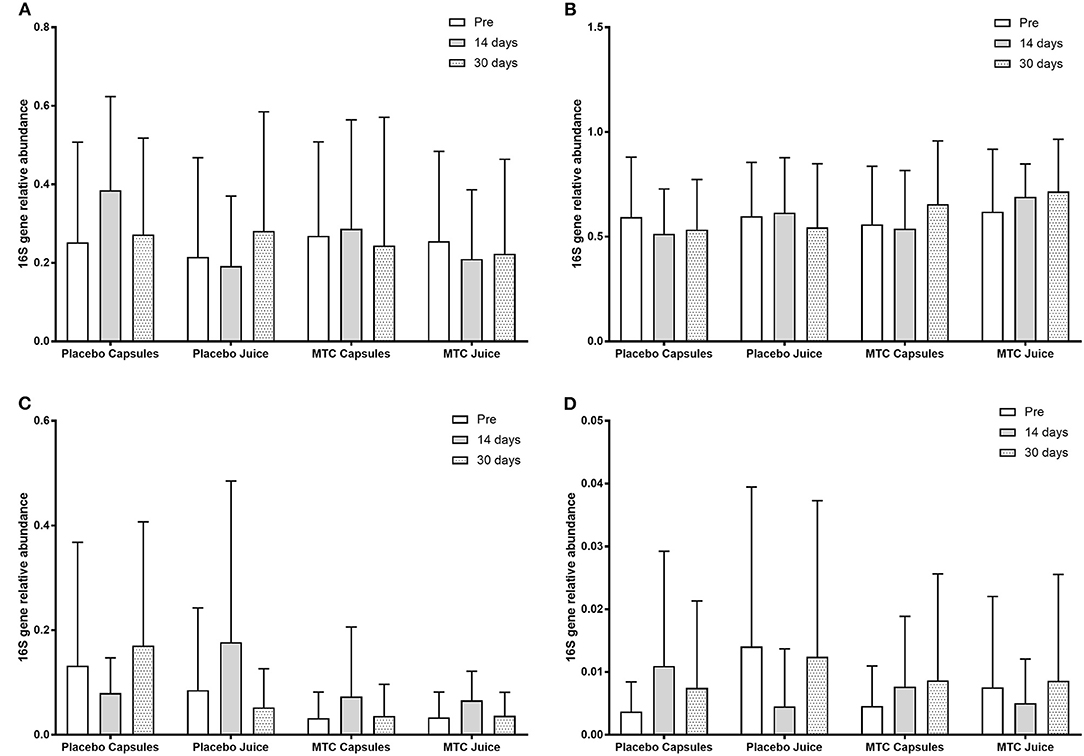
Figure 3. Observed taxonomic units (OTUs) for (A) Bacteroides, (B) Firmicutes, (C) Actinobacteria, and (D) Proteobacteria at baseline and after 14 and 30 days of supplementation with placebo or Montmorency tart cherry (MTC).
Correlations between the gut microbiome and other variables were mostly weak. BMI was positively correlated with Lactobacillus OTUs (r = 0.21, p = 0.01), Bifidobacterium OTUs (r = 0.21, p = 0.01), relative abundance of Actinobacteria (r = 0.23, p < 0.01), and relative abundance of Bacteroidetes (r = −0.25, p < 0.01). Glucose levels were correlated with relative abundances of Bacteroidetes, Firmicutes, Proteobacteria (r = 0.16, p = 0.04 for all), and Faecalibacterium (r = 0.23, p < 0.01). Roseburia OTUs were correlated with CRP (r = 0.19, p = 0.02).
Inflammation
There was no significant change in ESR over time (F = 0.59, p = 0.63) or between groups (F = 1.82, p = 0.15). Similarly, there was no significant change in CRP over time (F = 0.94, p = 0.42) or between groups (F = 1.64, p = 0.19) nor did UA change over time (F = 1.26, p = 0.29) or between groups (F = 0.18, p = 0.91). For TNF-alpha, there was a significant change over time (F = 2.77, p = 0.04) with higher TNF-alpha at 14 vs. 30 days (p = 0.013; mean difference: 0.14 pg·ml−1, 95% CI: 0.02–0.27 pg·ml−1). However, there was no difference between groups (F = 2.37, p = 0.08). Inflammatory marker data can be seen in Figure 4.
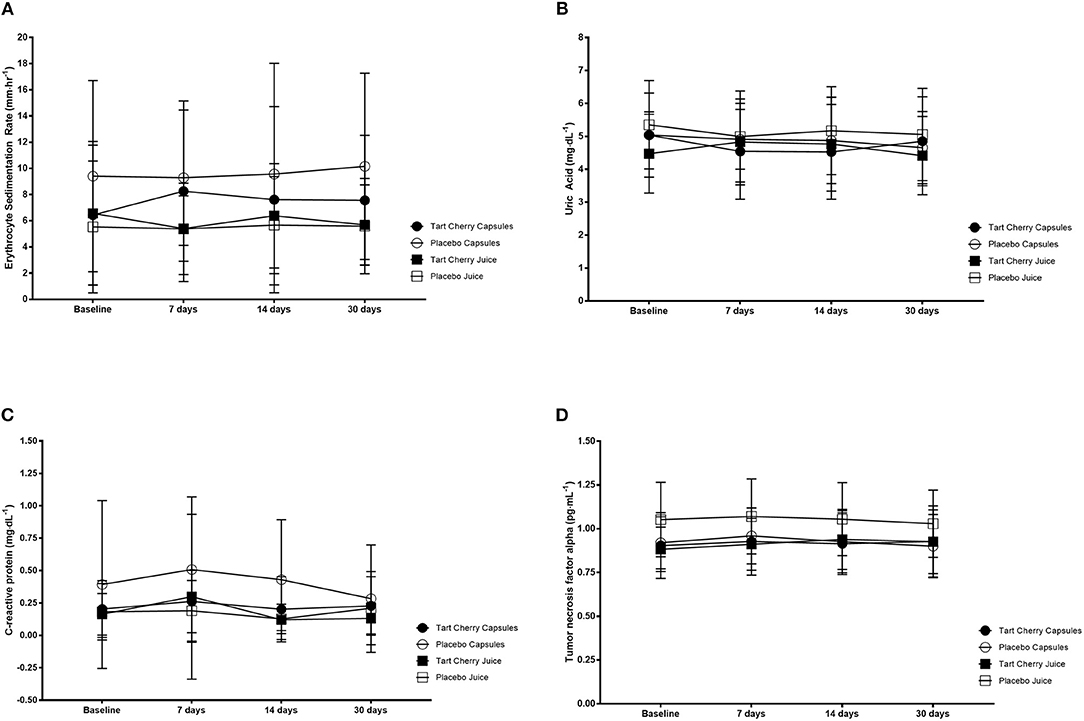
Figure 4. Inflammatory markers including erythrocyte sedimentation rate (A), uric acid (B), C-reactive protein (C) and tumor necrosis factor alpha (D) for each group at baseline and after 14 and 30 days of supplementation with placebo or Montmorency tart cherry (MTC) concentrate or capsules.
Blood Glucose Regulation
Fasting blood glucose levels steadily rose over the course of the study (F = 9.70, p < 0.001), with greater glucose at 14 days vs. baseline (p = 0.02; mean difference: 5.03 mmol·L−1, 95% CI: 0.75–9.30 mmol·L−1) and 7 days (p = 0.02; mean difference: 4.16 mmol·L−1, 95% CI: 0.52–7.80 mmol·L−1). Similarly, 30 days post was significantly higher vs. baseline (mean difference: 12.20 mmol·L−1, 95% CI: 7.18–17.21 mmol·L−1), 7 days (mean difference: 11.32 mmol·L−1, 95% CI: 6.89–15.76 mmol·L−1), and 14 days (mean difference: 7.17 mmol·L−1, 95% CI: 3.38–10.89 mmol·L−1) (p < 0.01 for all). However, there was no difference between groups (F = 1.17, p = 0.33). Insulin did not change over time (F = 1.79, p = 0.16), nor was it different between groups (F = 1.45, p = 0.24). Similarly, glycated albumin was not different over time (F = 2.39, p = 0.08) or between groups (F = 0.71, p = 0.56). Finally, HOMA-IR was not different over time (F = 1.07, p = 0.37) or between groups (F = 0.58, p = 0.63). Glucose regulation data can be seen in Figure 5.
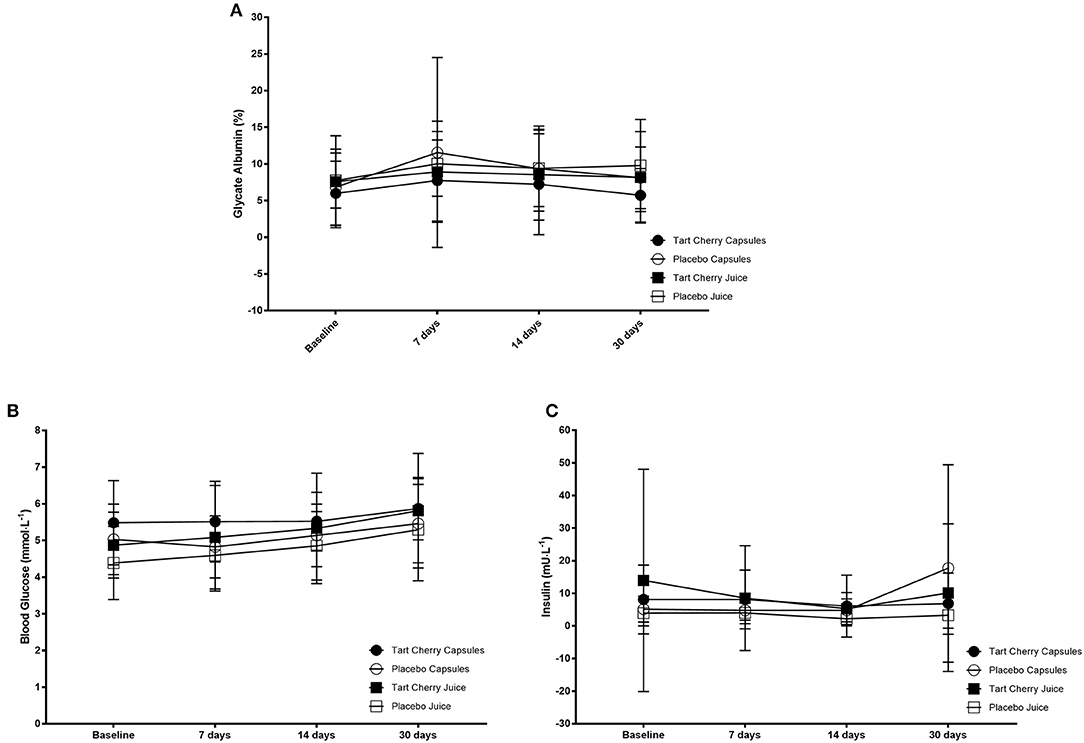
Figure 5. Glucose regulation markers including glycated albumin (A), blood glucose (B), and insulin (C) concentrations for each group at baseline and after 14 and 30 days of supplementation with placebo or Montmorency tart cherry (MTC) concentrate or capsules.
Dietary Intake
Completed FFQ and 3 day diet logs were compiled for 51/58 participants. Typical dietary pattern analysis found that all participants were classified as normal for protein intake, 39 participants were classified as normal, 4 classified as high, and 8 classified as low for carbohydrate intake. For fat, 36 were classified as normal and 15 were classified as high. For fiber, 16 participants were classified as normal, 11 were classified as high, and 24 were classified as low. There was no significant difference between groups in any of the macronutrients analyzed from the 3 day food log in this study (Table 2). Consumption of the top 100 polyphenol containing foods were tracked weekly, results can be found in Supplemental data. Total polyphenol consumption was 5,024 ± 4,599 for the MTC juice group, 4,744 ± 2,387 for the MTC capsule group, 2,519 ± 2,471 for the juice placebo group, and 3,424 ± 3,278 for the capsule placebo group and this was not significant between MTC formulations (X2 = 5.43, p = 0.14), but was higher for MTC vs. placebo group (U = 193, p = 0.02).
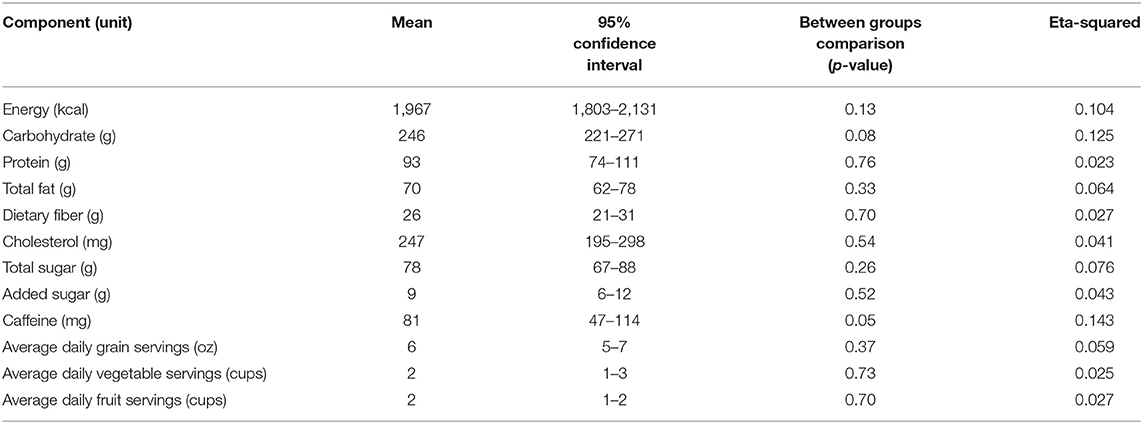
Table 2. Mean daily energy, alcohol, and nutrient intake of participants (n = 51) in the 3-day food log.
Discussion
The current study examined the effects of 30 days of supplementing with MTC concentrate or freeze-dried powder on gut microbiome composition, inflammation, and glucose regulation. We hypothesized that the polyphenols in tart cherry products would influence the gut microbiome composition, which would modulate changes in inflammatory markers and glucose regulation. However, we found no significant alterations in the gut microbiome, and no significant impact of MTC supplementation on inflammation or glucose regulation.
Gut Microbiome
The relationship between polyphenols and the gut microbiome has been established, whereby the degradation of most polyphenols requires host microbes and these microbes in turn utilize the products produced from polyphenol degradation for energy. This degradation of polyphenols often leads to greater bioavailability and biological activity (31) however this is dependent on the host microbiota composition (32). Tart cherries are well-known to be high in polyphenols and two previous investigations have examined the impact of tart cherry supplementation on the composition of the gut microbiome with disparate findings. Mayta-Apaza (11) investigated ingestion of 8 oz. MTC juice for 5 days in 10 participants. When comparing pre-to post-intervention microbiota, very little change was detected. However, the authors determined the need to divide participants into groups based on the baseline relative abundance of Bacteroides, either high or low. In the high Bacteroides group, ingestion of tart cherry juice resulted in a sharp decline in Bacteroides and an increase in Firmicutes (such as Ruminococcus, Clostridium, Streptococcus and Lactobacillus), while the opposite was seen in the low Bacteroides group, with an increase in Bacteroides and decreases in Firmicutes (such as Streptococcus and Lachnospiraceae). These changes may have been due to the underlying diets of the participants, as those in the low Bacteroides group consumed more carbohydrates, sugars, and fibers and the high polyphenol intake from the tart cherry juice resulted in increases in Bacteroides to facilitate breakdown of these polyphenols. When we divided our participants into groups based on high or low baseline levels of Bacteroides, we noted significantly higher Bacteroides in the high vs. low group at baseline and over the 30 days and no change in Firmicute levels, in contrast to the finding of Mayta-Apaza. However, we did not find any significant differences or changes in the other bacteria phyla or species, even if the groups were divided by their baseline Bacteroides levels.
Contrary to the findings of Mayta-Apaza, Lear (25) found no significant impact of 4 weeks supplementing with tart cherry concentrate on gut microbial composition. These authors noted their samples contained very low abundance of Lactobacillus and Bifidobacterium, which should have been more abundant and found their collection and storage methods may have resulted in alterations in their abundances. Nevertheless, our results are very similar to theirs despite our samples having an average 4% relative abundance of Bifidobacterium and 1% for Lactobacillus, well within the ranges of the healthy lower limits for these taxa (33). Early data suggests that the microbiome can be altered with dietary intake strategies, such as exclusively plant or animal based diets (6). For example, 20 days of red wine consumption increased Firmicutes and Bacteroidetes (8) and 30 days consumption of a high polyphenol cocoa drink increased bifidobacterial and lactobacilli populations. However, further analysis suggests these changes are transient, only lasting for 24 h after the consumption of high-fat/low fiber or low-fat/high fiber intake (34). In our study, it is possible that MTC supplementation brought change in microbial composition that might have not been measured with our fecal sample collection times (i.e., we missed the most opportune time for bacterial changes, although admittedly, we do not know what the most opportune timing for microbiome sampling following polyphenol consumption is since the majority of work is after very short (≤ 5 days) or long (>30 days) interventions). Further, Leeming et al. (35) note that the gut microbiota typically reverts back to its baseline state post-intervention, suggesting the need for long term dietary intervention. Therefore, future research should focus on detecting the sufficient time that bring the effective change in microbial composition from the intake of tart cherry supplementation.
It is also interesting to note that dietary intake of our participants did not seem to be a determining factor in whether or not the MTC supplementation altered microbial diversity, in contrast to Mayta-Apaza. Nearly 25% of our sample were considered to have high fat diets, while 40% had low fiber intake. While this likely determined whether a participant had high or low levels of Bacteroidetes at baseline, either the supplements did not provide enough of a stimulus for change, or the participant's dietary patterns were too influential to bring about change. This might indicate a need for greater changes in diet, along with MTC supplementation, to see significant alterations in gut microbiome composition.
Inflammatory Markers
Erythrocyte Sedimentation Rate
When inflammation is present in the body, red blood cells stick to one another and this results in greater sedimentation rates. While this is a non-specific marker of inflammation, it has been measured in two previous studies using tart cherry juice consumption (23, 36). While a 4 week intervention found a modest decline in ESR of 5% (23), another 4 week intervention actually found a 17% increase in ESR (36). Our findings are similar to the latter, as interestingly we found an average 11% increase in ESR over the course of the study, and we did not find a significant difference in ESR between groups. The average values for ESR were well within the normal ranges for the test, indicating there was no measurable inflammation in our participants, which differs from the two previous studies. However, some time points were a bit higher, causing larger intra-individual variability (average CoV: 27%), potentially due to exercise and acute inflammation and suggest this might not be a marker to measure changes in inflammation with tart cherry supplementation in the future in healthy populations.
Uric Acid
Uric acid is produced in the body from the breakdown of purines, and if not metabolized itself, can accumulate resulting in pain and inflammation. There is significant interest in the use of tart cherries to reduce UA and the incidence of gout because much of the research indicates MTC concentrate (18), MTC juice (22, 23) and freeze-dried MTC decrease UA (22). The majority of studies investigating UA and tart cherry are acute in nature, with short supplementation periods (up to 48 h) with decreases of up to 36% during the initial 8 h after consumption (18, 22). Only one of the two longer-term studies (36) found a decrease (19%) in plasma urate while the other found a modest (2%) decline in UA after 120 days of supplementation with MTC concentrate (37). In the current study we also found a modest 2% decline in UA from baseline to 30 days, however there was no significant difference in UA between groups, which is surprising given the literature. Similar to ESR, the majority of the values for our participants were within the normal range, however there was large intra-individual variability (average CoV: 30%), which likely played a role in the lack of significant findings.
C-Reactive Protein
CRP is used as a marker for inflammation, typically used to predict cardiovascular disease risk. However, because it is an essential marker of inflammation and elevated CRP has been noted in many inflammatory diseases (38) it can be a valuable tool for evaluating the impact of tart cherry on inflammation. Equivocal findings have been noted with tart cherry supplementation and changes in CRP, potentially due to different lengths of supplementation and doses. Acute interventions with MTC juice or concentrate are equivocal. For example, Bell et al. (18) found a 29% decrease in CRP 5 h after ingestion of 30 or 60 ml MTC concentration, with no difference between the doses, and this reduction was still apparent at 48 h. However, Hillman and Uhranowsky (22) did not find a change in CRP during a 48 h intervention with either 30 or 60 ml MTC juice. Moreover, a 4 week intervention with MTC juice found a non-significant 19% reduction in CRP (23), while other interventions lasting 6 weeks (39) and 12 weeks (36, 40) also failed to find significant reductions in CRP from MTC supplementation. Two investigations that utilized sweet Bing cherries did find significant reductions in CRP both after 3 h (41) and 28 day (42). These different results could be due to formulations, as sweet tart cherries are the only ones known to have anthocyanins in all portions of the fruit (skin, flesh and pit), while tart cherries do not have anthocyanins in their flesh and very little in their pits, though tart cherries have higher antioxidant properties (43). Another reason for differences between studies could be due to type of CRP measured (inflammatory vs. high-sensitivity) or its reported low reproducibility (44). Levels of CRP in our study are similar to other investigations who used healthy participants and MTC supplements and perhaps the lack of change in our study is due to this healthier population used. Indeed studies utilizing participants with high baseline levels of CRP tend to find significant reductions supplementing 4–6 weeks with MTC supplementation (21, 36).
TNF Alpha
TNF-α is a pro-inflammatory cytokine that regulates many body processes including inflammatory reactions by stimulating additional pro-inflammatory cells. TNF-α is not typically present in healthy humans, but increased levels are often found in inflammatory bowel diseases, where it leads to pathological inflammation (45). TNF-α in humans has been decreased (36, 42) or unaffected by cherry ingestion (41, 46, 47), and animal models demonstrate similar results (48, 49). The current study actually found a modest increase (2%) in TNF-α over the course of the study, primarily driven by an increase from baseline to 7 days, with large increases in a few of the participants (10–21% increase in 10/58 participants). TNF-α is positively associated with relative abundance of bacteria such as Ruminococcaceae, Blautia, and Lactobacillus (45), however because we did not see a significant increase in these populations, we cannot speculate that this was the cause. One study has found elevated TNF-α following ingestion of a Jerte Valley cherry-based product, which they speculated was caused by elevations in melatonin content (50), however because we did not measure melatonin content, we cannot be sure what caused TNF-α to increase in our study.
Taken together, the results of this study indicate MTC supplementation in either concentrate or freeze-dried powder has little impact on inflammation in apparently healthy participants, which may in part be due to a lack of sufficient inflammation in participants to observe any effect of the supplements.
Glucose Regulation
Cell-line work demonstrates MTC extract treatment leads to changes in key enzymes related to glucose regulation in diabetes, including alpha amylase (26). This would result in slower peak glucose levels and a longer time for carbohydrate digestion, which may decrease average blood glucose over time. Glucose regulation in the current study was assessed via measurement of glycated albumin, which is reflective of glycemia over a 2–3 week period (51), as well as blood glucose and insulin levels. Glycated albumin and insulin were not significantly changed over time nor were they different between groups, however blood glucose levels steadily rose over the course of the study but were not different between groups. This data is in line with the work by Lear (25) who found a decrease in insulin sensitivity (decreased Matsuda index), with an increase in insulin and no decrease in blood glucose in those supplementing with MTC juice. Additionally, Chai et al. (28) found an increase in blood glucose following 12 weeks of MTC concentrate ingestion, while Kimble et al. (52) found no impact of 12 weeks MTC concentrate ingestion on blood glucose levels. Interestingly, a seven day intervention in patients with metabolic syndrome found a significant decline in blood glucose and an increase in insulin (53), which suggests in the short-term, supplementation may improve insulin sensitivity, but this does not appear to translate to long-term benefits. Our findings show an elevation in glucose without a rise in insulin, which might indicate presence or development of insulin insensitivity, indeed 16 individuals (28%) had a fasting glucose >100 mg·dL−1 (but <126 mg·dL−1) at baseline and an additional 5 participants had elevated glucose levels from 7 days onward. Finally, the difference in sugar composition between the juice and capsule groups were significantly different (17.7 vs. 0.4 g), however there was no significant difference between groups in blood glucose regulation, it appears unlikely the results are due to greater sugar intake from the supplements and further human intervention work with MTC supplements and blood glucose regulation are needed.
Conclusion
The current study hypothesized that the polyphenols in MTC products would influence the gut microbiome composition, which would modulate changes in inflammatory markers and glucose regulation. However, we found no significant alterations in the gut microbiome, and no significant impact of MTC supplementation on inflammation or glucose regulation. These results may partially be due to the use of a healthy population, who did not have inflammatory conditions and thus future work may need to focus on clinical populations. Additionally, time point measurements for the gut microbiome may have missed changes in bacterial composition, therefore additional time course analysis with more frequent measurements may be necessary to see if MTC has any impact on gut microbial composition.
Data Availability Statement
The datasets presented in this study can be found in online repositories. The names of the repository/repositories and accession number(s) can be found below: https://www.ncbi.nlm.nih.gov/bioproject/PRJNA742936.
Ethics Statement
The studies involving human participants were reviewed and approved by Ohio University Institutional Review Board at Ohio University (IRB protocol# 18-F-13). The patients/participants provided their written informed consent to participate in this study.
Author Contributions
AH and BC contributed to conception, design of the study, performed the statistical analysis, and wrote sections of the manuscript. AH organized the database and wrote the first draft of the manuscript. All authors contributed to manuscript revision, read, and approved the submitted version.
Funding
This study was funded by a grant from The Cherry Marketing Institute.
Conflict of Interest
The authors declare that the research was conducted in the absence of any commercial or financial relationships that could be construed as a potential conflict of interest.
Publisher's Note
All claims expressed in this article are solely those of the authors and do not necessarily represent those of their affiliated organizations, or those of the publisher, the editors and the reviewers. Any product that may be evaluated in this article, or claim that may be made by its manufacturer, is not guaranteed or endorsed by the publisher.
Acknowledgments
The authors would like to thank Mr. Chris Brodsky and Ms. Olivia Trickett for their time and effort as research assistants for this project. Additionally, thanks to Dr. William Broach of the Ohio University Genomics Facility for the processing of the fecal samples.
Supplementary Material
The Supplementary Material for this article can be found online at: https://www.frontiersin.org/articles/10.3389/fnut.2021.733057/full#supplementary-material
References
1. Cho NH, Shaw JE, Karuranga S, Huang Y, da Rocha Fernandes JD, Ohlrogge AW, et al. Diabetes atlas: global estimates of diabetes prevalence for 2017 and projections for 2045. Diabetes Res Clin Pract. (2018) 138:271–81. doi: 10.1016/j.diabres.2018.02.023
2. Xiao JB, Högger P. Dietary polyphenols and type 2 diabetes: current insights and future perspectives. Curr Med Chem. (2015) 22:23–38. doi: 10.2174/0929867321666140706130807
3. Gurung M, Li Z, You H, Rodrigues R, Jump DB, Morgun A, et al. Role of gut microbiota in type 2 diabetes pathophysiology. EBioMedicine. (2020) 51:102590. doi: 10.1016/j.ebiom.2019.11.051
4. Cardona F, Andrés-Lacueva C, Tulipani S, Tinahones FJ, Queipo-Ortuño MI. Benefits of polyphenols on gut microbiota and implications in human health. J Nutr Biochem. (2013) 24:1415–22. doi: 10.1016/j.jnutbio.2013.05.001
5. Thursby E, Juge N. Introduction to the human gut microbiota. Biochem J. (2017) 474:1823–36. doi: 10.1042/BCJ20160510
6. David LA, Maurice CF, Carmody RN, Gootenberg DB, Button JE, Wolfe BE, et al. Diet rapidly and reproducibly alters the human gut microbiome. Nature. (2014) 505:559–63. doi: 10.1038/nature12820
7. Tzounis X, Rodriguez-Mateos A, Vulevic J, Gibson GR, Kwik-Uribe C, Spencer JP. Prebiotic evaluation of cocoa-derived flavanols in healthy humans by using a randomized, controlled, double-blind, crossover intervention study. Am J Clin Nutr. (2011) 93:62–72. doi: 10.3945/ajcn.110.000075
8. Queipo-Ortuño MI, Boto-Ordóñez M, Murri M, Gomez-Zumaquero JM, Clemente-Postigo M, Estruch R, et al. Influence of red wine polyphenols and ethanol on the gut microbiota ecology and biochemical biomarkers. Am J Clin Nutr. (2012) 95:1323–34. doi: 10.3945/ajcn.111.027847
9. Yamakoshi J, Tokutake S, Kikuchi M, Kubota Y, Konishi H, Mitsuoka T. Effect of proanthocyanidin-rich extract from grape seeds on human fecal flora and fecal odor. Microb Ecol Health Dis. (2001) 13:25–31. doi: 10.1080/089106001750071672
10. Vendrame S, Guglielmetti S, Riso P, Arioli S, Klimis-Zacas D, Porrini M. Six-week consumption of a wild blueberry powder drink increases bifidobacteria in the human gut. J Agric Food Chem. (2011) 59:12815–20. doi: 10.1021/jf2028686
11. Mayta-Apaza AC, Pottgen E, De Bodt J, Papp N, Marasini D, Howard L, et al. Impact of tart cherries polyphenols on the human gut microbiota and phenolic metabolites in vitro and in vivo. J Nutr Biochem. (2018) 59:160–72. doi: 10.1016/j.jnutbio.2018.04.001
12. Kechagia M, Basoulis D, Konstantopoulou S, Dimitriadi D, Gyftopoulou K, Skarmoutsou N, et al. Health benefits of probiotics: a review. ISRN Nutr. (2013) 2013:481651. doi: 10.5402/2013/481651
13. Marchesi JR, Adams DH, Fava F, Hermes GDA, Hirschfield GM, Hold G, et al. The gut microbiota and host health: a new clinical frontier. Gut. (2016) 65:330–9. doi: 10.1136/gutjnl-2015-309990
14. Ou B, Bosak KN, Brickner PR, Iezzoni DG, Seymour EM. Processed tart cherry products-comparative phytochemical content, in vitro antioxidant capacity and in vitro anti-inflammatory activity. J Food Sci. (2012) 77:H105–12. doi: 10.1111/j.1750-3841.2012.02681.x
15. Aeberli I, Gerber PA, Hochuli M, Kohler S, Haile SR, Gouni-Berthold I, et al. Low to moderate sugar-sweetened beverage consumption impairs glucose and lipid metabolism and promotes inflammation in healthy young men: a randomized controlled trial. Am J Clin Nutr. (2011) 94:479–85. doi: 10.3945/ajcn.111.013540
16. Bruun JM, Maersk M, Belza A, Astrup A, Richelsen B. Consumption of sucrose-sweetened soft drinks increases plasma levels of uric acid in overweight and obese subjects: a 6-month randomised controlled trial. Eur J Clin Nutr. (2015) 69:949–53. doi: 10.1038/ejcn.2015.95
17. Comstock LE. Importance of glycans to the host-bacteroides mutualism in the mammalian intestine. Cell Host Microbe. (2009) 5:522–6. doi: 10.1016/j.chom.2009.05.010
18. Bell P, Gaze D, Davison G, George T, Scotter M. Howatson G. Montmorency tart cherry (Prunus cerasus L) concentrate lowers uric acid, independent of plasma cyanidin-3-O-glucosiderutinoside. J Funct Foods. (2014) 11:82–90. doi: 10.1016/j.jff.2014.09.004
19. Howatson G, McHugh MP, Hill JA, Brouner J, Jewell AP, Van Someren KA, et al. Influence of tart cherry juice on indices of recovery following marathon running. Scand J Med Sci Sports. (2010) 20:843–52. doi: 10.1111/j.1600-0838.2009.01005.x
20. McCormick R, Peeling P, Binnie M, Dawson B, Sim M. Effect of tart cherry juice on recovery and next day performance in well-trained Water Polo players. J Int Soc Sports Nutr. (2016) 13:41. doi: 10.1186/s12970-016-0151-x
21. Schumacher HR, Pullman-Mooar S, Gupta SR, Dinnella JE, Kim R, McHugh MP. Randomized double-blind crossover study of the efficacy of a tart cherry juice blend in treatment of osteoarthritis (OA) of the knee. Osteoarthritis Cartilage. (2013) 21:1035–41. doi: 10.1016/j.joca.2013.05.009
22. Hillman AR, Uhranowsky K. Acute ingestion of montmorency tart cherry reduces serum uric acid but has no impact on high sensitivity C-reactive protein or oxidative capacity. Plant Foods Hum Nutr. (2021) 76:83–9. doi: 10.1007/s11130-021-00879-7
23. Martin KR, Coles KM. Consumption of 100% tart cherry juice reduces serum urate in overweight and obese adults. Curr Dev Nutr. (2019) 3:nzz011. doi: 10.1093/cdn/nzz011
24. Kent K, Charlton KE, Jenner A, Roodenrys S. Acute reduction in blood pressure following consumption of anthocyanin-rich cherry juice may be dose-interval dependant: a pilot cross-over study. Int J Food Sci Nutr. (2016) 67:47–52. doi: 10.3109/09637486.2015.1121472
25. Lear R, O'Leary M, O'Brien Andersen L, Holt CC, Stensvold CR, van der Giezen M, et al. Tart cherry concentrate does not alter the gut microbiome, glycaemic control or systemic inflammation in a middle-aged population. Nutrients. (2019) 11:1063. doi: 10.3390/nu11051063
26. Kirakosyan A, Gutierrez E, Ramos Solano B, Seymour EM, Bolling SF. The inhibitory potential of Montmorency tart cherry on key enzymes relevant to type 2 diabetes and cardiovascular disease. Food Chem. (2018) 252:142–6. doi: 10.1016/j.foodchem.2018.01.084
27. Desai T, Bottoms L, Roberts M. The effects of montmorency tart cherry juice supplementation and FATMAX exercise on fat oxidation rates and cardio-metabolic markers in healthy humans. Eur J Appl Physiol. (2018) 118:2523–39. doi: 10.1007/s00421-018-3978-9
28. Chai SC, Davis K, Wright RS, Kuczmarski MF, Zhang Z. Impact of tart cherry juice on systolic blood pressure and low-density lipoprotein cholesterol in older adults: a randomized controlled trial. Food Funct. (2018) 9:3185–94. doi: 10.1039/C8FO00468D
29. Sabou VR, O'Leary MF, Liu Y, Brown PN, Murch S, Bowtell JL. Review of analytical methods and reporting of the polyphenol content of tart cherry supplements in human supplementation studies investigating health and exercise performance effects: recommendations for good practice. Front Nutr. (2021) 8:652094: doi: 10.3389/fnut.2021.652094
30. Matthews DR, Hosker JP, Rudenski AS, Naylor BA, Treacher DF, Turner RC. Homeostasis model assessment: insulin resistance and beta-cell function from fasting plasma glucose and insulin concentrations in man. Diabetologia. (1985) 28:412–9. doi: 10.1007/BF00280883
31. Trošt K, Ulaszewska MM, Stanstrup J, Albanese D, De Filippo C, Tuohy KM, et al. Host: microbiome co-metabolic processing of dietary polyphenols - An acute, single blinded, cross-over study with different doses of apple polyphenols in healthy subjects. Food Res Int. (2018) 112:108–28. doi: 10.1016/j.foodres.2018.06.016
32. Possemiers S, Bolca S, Verstraete W, Heyerick A. The intestinal microbiome: a separate organ inside the body with the metabolic potential to influence the bioactivity of botanicals. Fitoterapia. (2011) 82:53–66. doi: 10.1016/j.fitote.2010.07.012
33. Almonacid DE, Kraal L, Ossandon FJ, Budovskaya YV, Cardenas JP, Bik EM, et al. 16S rRNA gene sequencing and healthy reference ranges for 28 clinically relevant microbial taxa from the human gut microbiome. PLoS ONE. (2017) 12:e0176555. doi: 10.1371/journal.pone.0176555
34. Wu GD, Chen J, Hoffmann C, Bittinger K, Chen Y-Y, Keilbaugh SA, et al. Linking long-term dietary patterns with gut microbial enterotypes. Science. (2011) 334:105–8. doi: 10.1126/science.1208344
35. Leeming ER, Johnson AJ, Spector TD, Le Roy CI. Effect of diet on the gut microbiota: rethinking intervention duration. Nutrients. (2019) 11:862. doi: 10.3390/nu11122862
36. Martin KR, Burrell L, Bopp J. Authentic tart cherry juice reduces markers of inflammation in overweight and obese subjects: a randomized, crossover pilot study. Food Funct. (2018) 9:5290–300. doi: 10.1039/C8FO01492B
37. Schlesinger N. Pilot studies of cherry juice concentrate for gout flare prophylaxis. J Arthritis. (2012) 1:1–5. doi: 10.4172/2167-7921.1000101
38. Dhingra R, Gona P, Nam B-H, D'Agostino RB, Wilson PWF, Benjamin EJ, et al. Reactive protein, inflammatory conditions and cardiovascular disease risk. Am J Med. (2007) 120:1054–62. doi: 10.1016/j.amjmed.2007.08.037
39. Lynn A, Mathew S, Moore CT, Russell J, Robinson E, Soumpasi V, et al. Effect of a tart cherry juice supplement on arterial stiffness and inflammation in healthy adults: a randomised controlled trial. Plant Foods Hum Nutr Dordr Neth. (2014) 69:122–7. doi: 10.1007/s11130-014-0409-x
40. Dodier T, Anderson KL, Bothwell J, Hermann J, Lucas EA, Smith BJUS. Montmorency tart cherry juice decreases bone resorption in women aged 65-80 years. Nutrients. (2021) 13:544. doi: 10.3390/nu13020544
41. Jacob RA, Spinozzi GM, Simon VA, Kelley DS, Prior RL, Hess-Pierce B, et al. Consumption of cherries lowers plasma urate in healthy women. J Nutr. (2003) 133:1826–9. doi: 10.1093/jn/133.6.1826
42. Kelley DS, Adkins Y, Reddy A, Woodhouse LR, Mackey BE, Erickson KL. Sweet bing cherries lower circulating concentrations of markers for chronic inflammatory diseases in healthy humans. J Nutr. (2013) 143:340–4. doi: 10.3945/jn.112.171371
43. Chaovanalikit A, Wrolstad RE. Anthocyanin and polyphenolic composition of fresh and processed cherries. J Food Sci. (2004) 69:FCT73–83. doi: 10.1111/j.1365-2621.2004.tb17859.x
44. Chrismas B, Taylor L, Smith A, Pemberton P, Siegler JC, Midgley AW. Reproducibility of measurement techniques used for creatine kinase, interleukin-6 and high-sensitivity C-reactive protein determination over a 48 h period in males and females. Meas Phys Educ Exerc Sci. (2018) 22:191–9. doi: 10.1080/1091367X.2017.1412317
45. Mendes V, Galvão I, Vieira AT. Mechanisms by which the gut microbiota influences cytokine production and modulates host inflammatory responses. J Interferon Cytokine Res. (2019) 39:393–409. doi: 10.1089/jir.2019.0011
46. Levers K, Dalton R, Galvan E, Goodenough C, O'Connor A, Simbo S, et al. Effects of powdered Montmorency tart cherry supplementation on an acute bout of intense lower body strength exercise in resistance trained males. J Int Soc Sports Nutr. (2015) 12:41. doi: 10.1186/s12970-015-0102-y
47. Levers K, Dalton R, Galvan E, O'Connor A, Goodenough C, Simbo S, et al. Effects of powdered Montmorency tart cherry supplementation on acute endurance exercise performance in aerobically trained individuals. J Int Soc Sports Nutr. (2016) 13:22. doi: 10.1186/s12970-016-0133-z
48. Martin KR, Wooden A. Tart cherry juice induces differential dose-dependent effects on apoptosis, but not cellular proliferation, in MCF-7 human breast cancer cells. J Med Food. (2012) 15:945–54. doi: 10.1089/jmf.2011.0336
49. Seymour EM, Lewis SK, Urcuyo-Llanes DE, Tanone II, Kirakosyan A, Kaufman PB, et al. Regular tart cherry intake alters abdominal adiposity, adipose gene transcription, and inflammation in obesity-prone rats fed a high fat diet. J Med Food. (2009) 12:935–42. doi: 10.1089/jmf.2008.0270
50. Garrido M, González-Gómez D, Lozano M, Barriga C, Paredes SD, Rodríguez AB, et al. Jerte valley cherry product provides beneficial effects on sleep quality. Influence on aging. J Nutr Health Aging. (2013) 17:553–60. doi: 10.1007/s12603-013-0029-4
51. Wright LA-C, Hirsch IB. Metrics beyond hemoglobin A1C in diabetes management: time in range, hypoglycemia, and other parameters. Diabetes Technol Ther. (2017) 19:S-16-S-26. doi: 10.1089/dia.2017.0029
52. Kimble R, Keane KM, Lodge JK, Howatson G. The influence of tart cherry (Prunus cerasus, cv montmorency) concentrate supplementation for 3 months on cardiometabolic risk factors in middle-aged adults: a randomised, placebo-controlled trial. Nutrients. (2021) 13:1417. doi: 10.3390/nu13051417
Keywords: polyphenol, cherry, montmorency, microbiome, glucose, inflammation
Citation: Hillman AR and Chrismas BCR (2021) Thirty Days of Montmorency Tart Cherry Supplementation Has No Effect on Gut Microbiome Composition, Inflammation, or Glycemic Control in Healthy Adults. Front. Nutr. 8:733057. doi: 10.3389/fnut.2021.733057
Received: 29 June 2021; Accepted: 19 August 2021;
Published: 16 September 2021.
Edited by:
Karen M. Keane, Galway-Mayo Institute of Technology, IrelandReviewed by:
Malachy P. McHugh, Nicholas Institute of Sports Medicine and Athletic Trauma (NISMAT), United StatesAna Rodriguez-Mateos, King's College London, United Kingdom
Terun Desai, University of Hertfordshire, United Kingdom
Copyright © 2021 Hillman and Chrismas. This is an open-access article distributed under the terms of the Creative Commons Attribution License (CC BY). The use, distribution or reproduction in other forums is permitted, provided the original author(s) and the copyright owner(s) are credited and that the original publication in this journal is cited, in accordance with accepted academic practice. No use, distribution or reproduction is permitted which does not comply with these terms.
*Correspondence: Angela R. Hillman, aGlsbG1hbiYjeDAwMDQwO29oaW8uZWR1