- 1National R&D Center for Edible Fungus Processing Technology, Henan University, Kaifeng, China
- 2Functional Food Engineering Technology Research Center, Kaifeng, China
- 3Joint International Research Laboratory of Food and Medicine Resource Function, Kaifeng, China
- 4School of Chemical Sciences, University of Auckland, Auckland, New Zealand
- 5Medicinal and Aromatic Plants Researches Department, Agricultural Research Center, Horticulture Research Institute, Giza, Egypt
Nutrients can be considered as functional foods, which exert physiological benefits on immune system. The seeds of Nigella sativa, which have many active constituents, are mainly used for medicine, food spice, and nutritional supplements in Egypt. Much attention has been paid to N. sativa seeds for their anticancer, antibacterial, anti-inflammatory, and immune properties. However, their active constituents and mechanisms underlying functions from N. sativa seeds is unclear. Thus, the bioactive constituents with immune regulation in N. sativa seeds were systematically studied. A new compound (3-methoxythymol-6-O-β-D-apiofuranosyl-(1→6)-β-D-glucopyranoside 1) and 11 known compounds (2–12) were separated from the N. sativa seeds by chromatographic methods. Their structures were then elucidated by spectroscopic analysis of MS, UV, IR, 1H-, and 13C-NMR. Furthermore, immunomodulatory effects of those compounds in RAW 264.7 cells were evaluated by phagocytosis, nitric oxide (NO) and cytokine release, related mRNA transcription, and key proteins expression in vitro. Monosaccharide derivatives, Ethyl-α-D-furaarabinose (5), and Ethyl-β-D-fructofuranoside (8) were shown to played bidirectional regulatory roles in immunity and anti-inflammation through the regulation of nuclear factor-κB (NF-κB) signaling pathways. The results showed the active compounds and mechanisms of immune regulation in N. sativa, thus indicating that N. sativa seeds could be used as dietary supplements in immunomodulation.
Introduction
The interaction between inflammation and the immune system is very complex (1). Inflammation is a physiological and pathological reaction caused by the harmful stimulation of living tissue with the vascular system by the internal and external environment (1). A moderate inflammatory reaction can stimulate the immune system and promote the proliferation and activation of immune cells, while an excessive inflammatory reaction will cause immune system dysfunction, resulting in damage to the body (2). Inflammation is the outcome of effective immune response actions that prevent the organism from infections (3). Acute inflammation is the response of the immune system microorganism and environmental stresses and is crucial to tissue healing. On the other hand, chronic inflammation generally refers to chronic illnesses like diabetes and neurodegenerative, cardiovascular, and metabolic diseases (4, 5). When chronic inflammation develops, it can cause pathological change to signaling pathways [especially nuclear factor-κB (NF-κB)] and the signal transducer, which cause an increased level of oxidative stress leading to excessive release of reactive oxygen species (ROS) (6, 7). NF-κB pathway activation leads to the expression of genes regulating immune-regulation, inflammation, apoptosis, and carcinogenesis, with the release of pro-inflammatory cytokines and chemokines (8). Immunotherapy is an effective therapeutics via activating or suppressing the immunologic system through synthetic, natural drugs and antibodies to combat disorders (5). Intake of immune modulators is an essential approach to immunotherapy. Synthetic agents show typical side effects manifested as infection, blood constipation, disorders, and so on, while natural drugs are comparatively safe (9). Some dietary constituents of phytochemicals are essential to the balance and development of the immune system and in the amelioration of chronic inflammation (10).
Diet therapy and diet health gradually became the focus of attention. Diets rich in plants such as spices, fruits, and vegetables were demonstrated to restrict the emergence and growth of chronic illness via the inhibition of chronic inflammation (11, 12). Balanced healthy diets along with nutrient supplementation are also essential to maintain the normal physiology of the human body as it plays an essential role in boosting up individual immunity (13). Nutrient supplementation containing botanicals can meet the physiological and nutritional needs of some patients (14). The use of botanicals has drawn much attention, particularly for minimizing adverse events on the immune system (15).
Nigella sativa, belonging to the family Ranunculaceae, is distributed in southwest Asia, North Africa, and Southern Europe (16). N. sativa seeds are exhibited in time-honored traditions through their usage as wind dispelling agents, diuretics, insect repellents, and dietary supplements (17–19). The seeds of N. sativa have been shown to exert anti-cancer, immunomodulative, anti-inflammatory, anti-bacterial, antioxidant, hypoglycemic, stomach protection, liver protection, and renal protection activities (20–22). The seeds and oil of N. sativa are widely used in food preparation and medicine (23, 24). In addition, literature has shown that the co-delivery of nutrient supplements and drugs could contribute to promising results. N. sativa components as nutritional supplements are effective for the adjuvant treatment of COVID-19 cases (25, 26). In addition, N. sativa seeds and oil has been shown to supplement cardiovascular protective functions for patients with type 2 diabetes mellitus (T2DM) (27). Like other supplements, N. sativa seeds can strengthen the resistance of the immune system to diseases (28). Alshatwi A A found that N. sativa seed extract could stimulate the proliferation of human peripheral blood monocytes, which are usually stimulated by non-phytohemagglutinin through flow cytometry and PCR methods (29).
It is obvious that N. sativa seeds could meet the nutritional need to improve the immune system of an organism (30). Research on the chemical constituents of this genus began in the middle of the 20th century (31), which showed that N. sativa seeds contain various chemical components like essential oils, alkaloids, phenols, saponins, and steroids (32, 33).
Our work focused on the identification of the relevant bioactive compounds in N. sativa seeds. The ethanol extract of N. sativa was investigated and a new monoterpene glucoside, 3-methoxythymol-6-O-β-D-apiofuranosyl-(1→6)-β-D-glucopyranoside, and 11 known compounds were isolated and identified. Then, the anti-inflammatory and immunomodulative activities of the compounds (Figure 1) isolated from N. sativa seeds were evaluated in vitro. The mechanism of the monosaccharide derivatives was demonstrated on RAW264.7 macrophage.
Materials and Methods
Reagents and Instruments
The following technologies and materials were used in this research: UV spectra (Shimadzu, Kyoto, Japan); IR spectra (Bruker Vector 22 spectrophotometer, Bruker Optics GmbH, Ettlingen, Germany); Mass spectra (API QSTAR time-of-flight spectrometer, MDS Sciqaszex, Concord, Ontario, Canada). NMR spectra (Bruker AM-400, Bremerhaven, Germany); silica gel (200–300 and 300–400 mesh, Qingdao Marine Chemical Inc., China); Rp-18 gel (40–63 μm, Merck, Darmstadt, Germany); Sephadex LH-20 (20–150 μm, Amersham Biosciences, Uppsala, Sweden); YMC*GEL ODS-A-HG (50 μm, YMC Co. Ltd., Kyoto, Japan) (34).
The following reagents were used in this research: FBS (Gibco, Grand Island, NE, USA); DMEM, neutral red (Solarbio, Beijing, China); Nitric oxide kit (Nanjing Jiancheng Bioengineering Institute); IL-6 and TNF-α ELISA kit (Beijing 4A Biotech Co., Ltd., Beijing, China); Primer iNOS, TNF-α, IL-6 and Cox-2 (Thermo Fisher Scientific, Shanghai, China); Reactive Oxygen Species Assay Kit (Beyotime Biotechnology, Shanghai, China); PrimeScriptTMRT reagent kit with gDNA Eraser kit and TB Green TM Ex TaqTM II (Tli RNadeH Plus, Accurate Biology, Hunan, China), Bulk kit (TaKaRa, Accurate Biology, Hunan, China); Antibody NF-κB p65, phospho-NF-κB p65, iNOS, COX-2, IκBα, and phospho-IκBα (Cell Signaling, Beverly, MA, USA); LPS (Sigma-Aldrich, St. Louis, MO, USA) (35).
Extraction and Isolation
The air-dried and powdered N. sativa seeds (5 kg) were degreased with petroleum ether and the residue was extracted by 70% ethanol (3 ×10 L) under room temperature. The ethanol extract (550 g) was separated by silica gel column chromatography eluting with a gradient of CHCl3-MeOH (20:1→1:1, v/v) to afford seven fractions 1–7 by TLC plate analysis. Fr.2 (15 g) was subjected to Rp-18 column chromatography (MeOH-H2O, 20:80→100:0, v/v) to afford five subfractions (Fr.2-1 to Fr.2-6). Sephadex LH-20 column chromatography (MeOH) was performed on 2–4 (300 mg) and then purified by semi-prep. HPLC (MeOH-H2O, 40:60, v/v) was performed to obtain 3 (4 mg), 4 (4 mg), and 5 (25 mg). Fr.3 (50 g) was then separated by Rp-18 column chromatography (MeOH-H2O, 40:90→60:0, v/v), performed by silica gel column chromatography, eluted with a gradient system of EAC-MeOH (10:1→1:1, v/v), purified by Sephadex LH-20 column chromatography (MeOH), and further purified by semi-prep. Another HPLC (MeOH-H2O, 30:70, v/v) was also performed to afford 6 (4 mg), 7 (3.5 mg), and 8 (35 mg). Fraction 4 (19.5 g) was subjected to silica gel column chromatography, eluting with a gradient system of CHCl3-MeOH (8:1→1:1, v/v) to afford five subfractions (Fr.4-1 to Fr.4-5). Fr.4-2 (15 g) was performed by Rp-18 column chromatography (MeOH-H2O, 10:90→100:0, v/v), Sephadex LH-20 column chromatography (MeOH), and further purified by semi-prep. An additional HPLC (MeOH-H2O, 52:48, v/v) to afford 1 (4 mg) and 2 (3 mg) was performed. Fr.6 (100 g) was separated by silica gel column chromatography, eluting with a gradient system of CHCl3-MeOH (8:1→1:1, v/v) to afford five subfractions (Fr.6-1 to Fr.6-5). Fr.6-1 (300 mg) was purified by semi-prep. Once again, HPLC was performed (MeOH-H2O, 28:82, v/v) to afford 9 (7 mg) and 10 (3 mg). Fr.6-3 (150 mg) was purified by Sephadex LH-20 column chromatography (MeOH), further by semi-prep. Another HPLC (MeOH-H2O, 15:85, v/v) to afford 11 (2 mg) was performed. Fr.7 (10 g) was separated by silica gel column chromatography, eluting with a gradient system of CHCl3-MeOH (5:1→1:1, v/v), then Sephadex LH-20 column chromatography (MeOH), and further purified by semi-prep. One more HPLC (MeOH-H2O, 52:48, v/v) was performed to afford 12 (5 mg). Spectral data was seen in Supplementary Materials.
Plant Material
Nigella sativa seeds were provided by the Department of Medicinal and Aromatic Plants, Horticultural Research Institute, Egyptian Agricultural Research Center. The plants of N. sativa were rich in local resources; thus, the collection was permitted.
Cell Culture and Cell Viability Assay
RAW264.7 macrophages were cultured by the same assay as Zhang H (36). Cell viability was assessed by 3-(4,5-Dimethylthiazol-2-yl)-2,5-diphenyltetrazolium bromide (MTT) assay. The starting inoculum of 1 × 105 cells/ml/well was cultured in a 96-well cell culture plate at 100 μl per well-incubating at 37°C with 5% carbon dioxide in an incubator for 24 h. Cells were also exposed to 100-μl culture mediums with different concentrations (6.25, 12.5, 25, 50, 100, 200, and 400 μmol/L) of compounds 5 and 8 for 24 h, respectively. Lipopolysaccharide (LPS, 1 μg/ml) was used as a positive control. In addition, 10 μl/well MTT solution was added in the dark environment, cells were cultured in an incubator for 4 h, and dimethyl sulfoxide (DMSO, 100 μl) was added to each well to solubilize the blue-purple crystal, with the best absorption at 490 nm.
Phagocytic Activity
RAW264.7 cells were seeded in 96-well plates (1 × 106 cells/ml) and exposed to specified concentrations of compounds 5 and 8. The supernatant was treated with 0.075% neutral red solution after 24 h. Cell lysis solution containing 1% acetic acid-anhydrous alcohol (1:1, V:V) was also added into each well, and then the absorbance value was determined at 540 nm.
Measurement of NO
The experiment referred to normal and LPS-induced conditions of the RAW264.7 macrophages model. RAW264.7 cells were seeded in 24-well plates (1 × 106 cells/ml) and treated with compounds 5 and 8 for 24 h under normal culture conditions. The administration group was pretreated with compounds 5 and 8 for 1 h and LPS (1 μg/ml) for 24 h under the LPS-induced inflammation model. Then, supernatants were collected for testing of the nitric oxide (NO) concentration by the Nitric oxide kit according to the instructions of the manufacturer.
Determination of IL-6 and TNF-α
Macrophages were treated as described above, cellular supernatants were collected, and the cytokines [interleukin- (IL-) 6 and tumor necrosis factor- (TNF-) α] were analyzed using corresponding ELISA kits.
qRT-PCR
Cell processing was done similarly to the above methods, and PCR was performed by the same assay as Zhang H (36). The sequences are listed in Table 1.
Western Blot
RAW264.7 cells were collected as described above, and then the total proteins were extracted using the weak RIPA Lysis Buffer. The methods of sodium dodecyl sulfate-polyacrylamide gel electrophoresis (SDS-PAGE) and Western blot were the same as the assay done by Wang Honglin (35). The protein signals were visualized with an ECL chemiluminescence detection kit (Solarbio, Beijing, China) and the band gray value was quantitatively analyzed using the Image J software (36).
Determination of Intracellular ROS
RAW264.7 cells were plated in 6-well plates with 5 × 105 cells/ml cells suspension and cultured at 37°C with 5% CO2 for 24 h. The control group, administration group (compounds 5 and 8), and LPS group (1 μg/ml) were conducted on RAW 264.7 cells, respectively. The supernatant was discarded after 24 h, and the cells were gently rinsed and centrifuged by adding serum-free Dulbecco's modification of eagle's medium (DMEM). Each group was added with 1 ml of 10 μM DCFH-DA to resuspension cells. Cells were incubated at 37°C for 20 min and reversed every 3–5 min to allow the probe to have full contact with the cells. Eventually, the probe was determined by flow cytometry after using a 300-mesh nylon screen.
Statistical Analysis
Experimental data were expressed as mean ± standard deviation, and the numerical statistics were handled using a one-way ANOVA of the SPSS 19.0 software. All column images were made via the GraPhPad Prism 6.0 software.
Results
Identification of a New Compound
Compound 1 was obtained as a colorless oil and its formula was determined as C22H34O11 by HR-EI-MS at m/z 473.2027 [M-H]− (calcd for C22H34O11, 473.2023). The 1H-NMR spectrum (Table 1) indicated 2 aromatic H-atoms at δH 6.72(s) and 6.95(s), 2 anomeric H-atoms at δ(H) 4.67 (1H, t, J = 3.6 Hz) and 4.96 (1H, d, J = 2.4 Hz, H-1″), and 3 Me groups at 2.14 (3H, s) and 1.20 (6H, d, J = 7 Hz). The 13C-NMR spectrum of 4 (Table 2) revealed 22 C-atom signals, corresponding to a 1,3,4,6- substituted aromatic ring, three Me groups at δ(C) 16.2 and 23.6, a CH group at δ(C) 27.4, a β-D-glucosyl moiety, and a terminal β-D-apiosyl moiety (9, 10). In the HMBC spectrum (Figure 2), the key correlations from H-7/C-5, C-4 and C-3, H-2/C-8, H-9/C-1, and C-8 and C-10, together with 1H-1H-COSY correlations of H-9/H-8 and H-10/H-8 showed that the aglycone of 1 was thymoquinol (11). The key correlations from H-1′ [δH 4.67] to C-6 (δC 149.6) in the HMBC spectrum indicated that β-D-glucosyl moiety was attached to C(6). We also speculated that the β-D-apiose moiety was attached to C(6') and the β-D-glucosyl moiety (12). This conclusion was elucidated by the HMBC spectrum, in which the key correlation from H-1″ [δH 4.96] to C-6′ (δC 68.7) was observed (Figure 2) (9). Hence, the structure of compound 1 was defined as 3-methoxythymol-6-O-β-D-apiofuranosyl-(1→6)-β-D-glucopyranoside.
Effects of Compounds 5 and 8 on Cell Viability of RAW264.7 Macrophages
RAW264.7 cells were exposed to different concentrations of compounds 5 and 8 for 24 h. Results showed that compound 5 had no significant inhibitory effect on the proliferation of RAW264.7 cells at 6.25–400 μmol/L in Figure 3. Compound 8 did not significantly inhibit the proliferation of RAW264.7 cells at 6.25–200 μmol/L, but significantly inhibited the activity cells at 400 μmol/L. Therefore, the concentrations at 50, 100, and 200 μmol/L of compounds 5 and 8 were selected as the concentration gradients for subsequent experiments in Figure 3A.
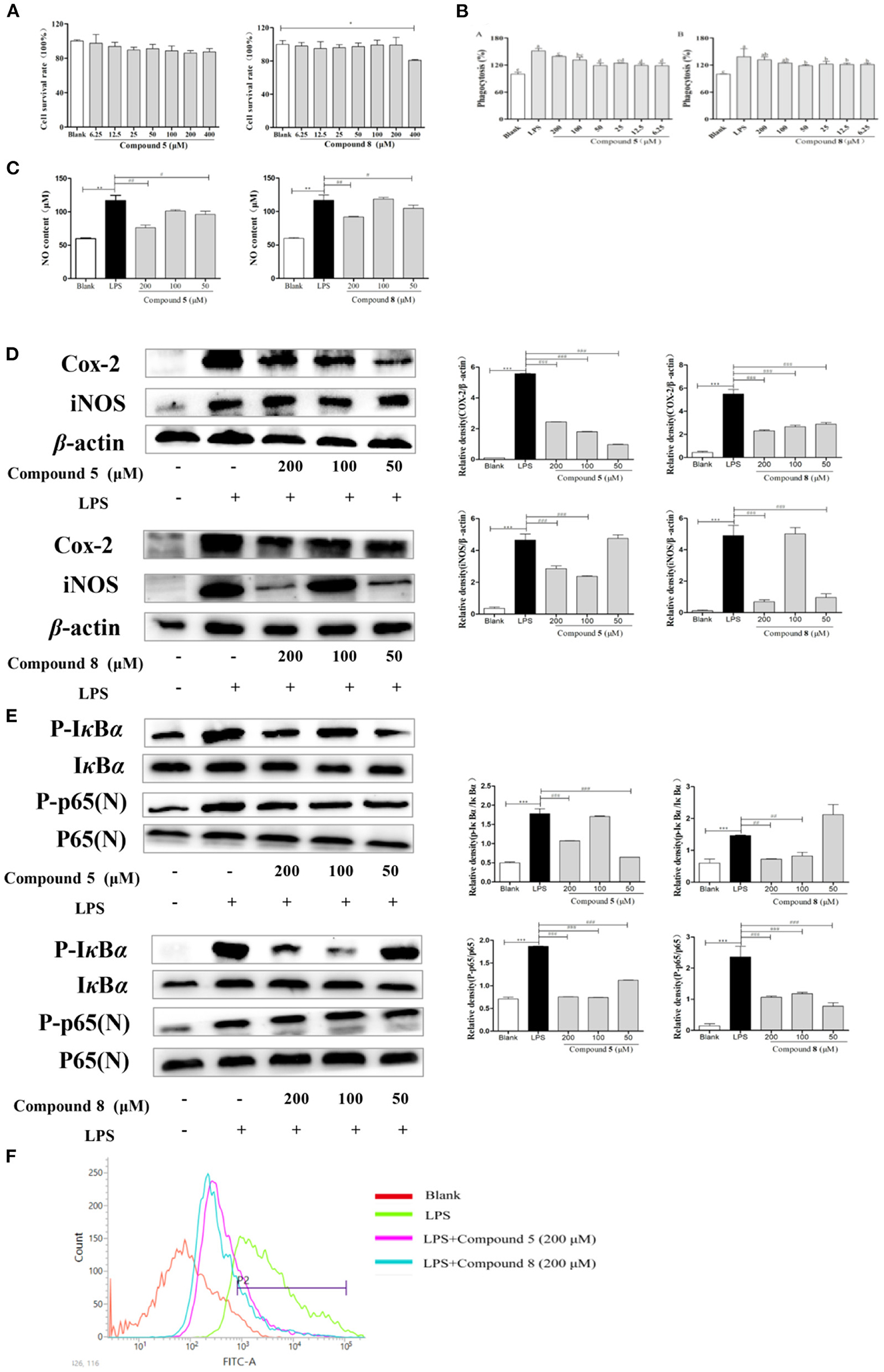
Figure 3. Effects of compounds 5 and 8 on the nuclear factor-κB (NF-κB) pathways in lipopolysaccharide- (LPS) induced RAW264.7 Cells. (A) Cell survival rates. (B) Phagocytic activity of RAW264.7. (C) The expression levels of nitric oxide (NO) production. (D) mRNA and protein expression of cyclooxygenase- (COX) 2 and inducible nitric oxide synthase (iNOS). (E) Expression levels of key proteins in the NF-κB pathways. (F) Production of reactive oxygen species (ROS). β-Actin served as a control. Data shown are means ± SEM. ***p < 0.001, **p < 0.01, *p < 0.05. ###p < 0.001, ##p < 0.01, #p < 0.05.
Effects of Compounds 5 and 8 on Phagocytic Activity
In Figure 3, the phagocytosis of LPS-treated cells was consistent with the expectation, which increased nearly twice more than that of the blank group. Compared with the normal control group, the phagocytic activity of RAW264.7 cells was significantly enhanced after being exposed to compounds 5 and 8 at a concentration of 6.25–200 μmol/L for 24 h in Figure 3B. They could promote the phagocytosis of macrophages at a certain concentration range, which may be essential to immune-regulation.
Effects of Compounds 5 and 8 on NO Secretion in LPS-Induced RAW264.7 Cells
There is a significant increase in the concentration of NO in normal cells after LPS stimulation, increasing to approximately twice as much as that in the blank group, which indicated that the model was successful. However, the excessive expression of NO was inhibited significantly by the high-dose and low-dose groups of compounds 5 and 8 after LPS stimulation, while the middle-dose group showed significant effects in Figure 3C.
Effects of Compounds 5 and 8 on Gene and Protein Expression of iNOS and COX-2 in LPS-Induced RAW264.7 Cells
The increase of NO during inflammation was generally attributed to the upregulation of inducible nitric oxide synthase (iNOS) and cyclooxygenase- (COX) 2, respectively. In Figure 3D, LPS significantly stimulated the transcription of targeted mRNA, while compounds 5 and 8 reversed the mRNA upregulation. Compounds 5 and 8 at 50, 100, or 200 μmol/L, significantly reduced COX-2 and iNOS protein production in LPS-induced macrophages, which was in accordance with the PCR results.
Effects of Compounds 5 and 8 on the Expression of Proteins in NF-κB Signaling Pathways in LPS-Induced RAW264.7 Cells
NF-κB signaling pathways are key cellular signaling pathways mediated by LPS. The results showed that the protein expressions of p-IκBα and p-NF-κB p65 were significantly upregulated in RAW264.7 cells stimulated by LPS compared with the blank group. These results indicated that compounds 5 and 8 could inhibit the over-expression of key proteins p-IκBα and N-NF-κB p65 in the NF-κB pathways of RAW264.7 cells in Figure 3E, thereby inhibiting the over-activation of the NF-κB pathways and playing an anti-inflammatory role.
Effects of Compounds 5 and 8 on LPS-Induced Oxidative Stress
To release abundant ROS, LPS is generally used to stimulate RAW264.7 cells. These ROS mediate inflammatory signals in cells. As is shown in Figure 3F, compared with the blank group, the peak shape of the LPS group shifted significantly to the right, indicating that LPS improved the production of oxygen, while compounds 5 and 8 at 200 μmol/L could inhibit the excessive production of reactive oxygen in stimulated RAW264.7 cells.
Effects of Compounds 5 and 8 on NO Secretion in Normal Cultured RAW264.7 Cells
As shown in Figure 4A, compounds 5 and 8 in high- and medium-dose groups significantly promoted the production of NO in normal cultured RAW264.7 cells, while there was no significant effect at 50 μmol/L.
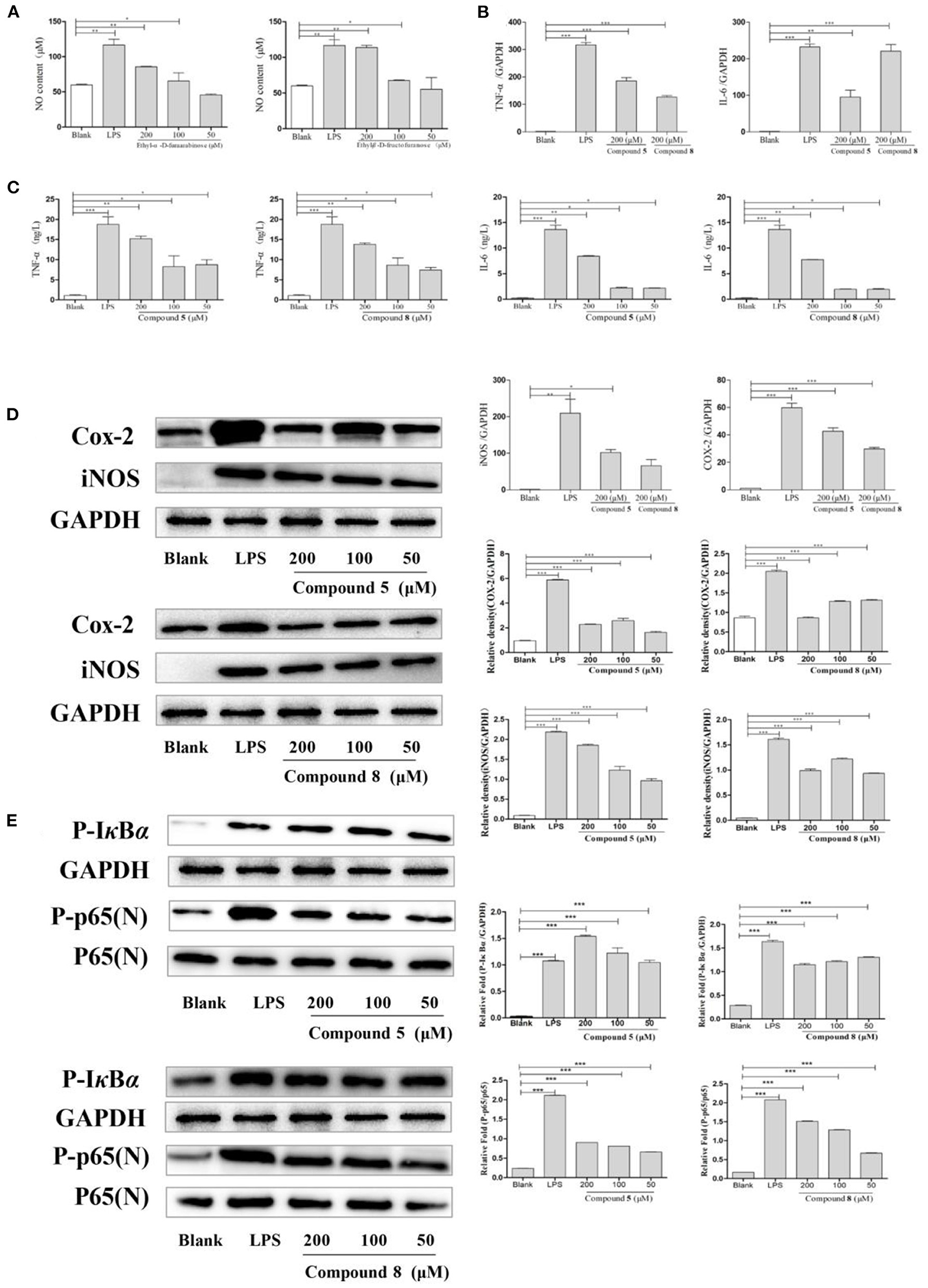
Figure 4. Effects of compounds 5 and 8 on the nuclear factor-κB (NF-κB) signaling pathways in normal cultured RAW264.7 cells. (A) Expression of nitric oxide (NO) production. (B) Inflammatory cytokine tumor necrosis factor- (TNF-) α, interleukin- (IL-) 6 mRNA transcription. (C) Secretion of TNF-α, IL-6. (D) mRNA and protein expression of cyclooxygenase- (COX-) 2 and inducible nitric oxide synthase (iNOS). (E) Expression of key proteins in the NF-κB signaling pathways. Glyceraldehyde-3-phosphate dehydrogenase (GAPDH) served as control. Data shown are means ± SEM. ***p < 0.001, **p < 0.01, *p < 0.05.
Effects of Compounds 5 and 8 on the Secretion of Cytokines and mRNA Expression in RAW264.7 Cells in Normal Cultured RAW264.7 Cells
In Figure 4C, compared with the blank group, the release levels of TNF-α and IL-6 by RAW264.7 cells treated with LPS or compounds were significantly increased. The results showed that compounds 5 and 8 could promote the release of TNF-α and IL-6 in RAW264.7 cells, but the optimal dose was 200 μmol/L. On this basis, the effects of compounds 5 and 8 (200 μmol/L, respectively), on the mRNA levels of cytokines in normal cultured RAW264.7 cells were determined by qRT-PCR. As shown in Figure 4B, mRNA transcription of TNF-α and IL-6 was significantly raised following LPS treatment in the positive control group. Furthermore, compared with the control group, compounds 5 and 8 (200 μmol/L, respectively), significantly increased the levels of TNF-α and IL-6. These indicated that compounds 5 and 8 could upregulate the mRNA levels of TNF-α and IL-6 in macrophages and promote the production of IL-6 and TNF-α.
Effects of Compounds 5 and 8 on iNOS and COX-2 in Normal Cultured RAW264.7 Cells
To determine the effects of compounds 5 and 8 (200 μmol/L, respectively), on the mRNA expression of iNOS and COX-2 in cells, PCR was used. Western blot was applied to measure the effects of compounds 5 and 8 (50, 100, and 200 μmol/L, respectively), on the expression of COX-2 and iNOS under normal culture conditions. As shown in Figure 4D, LPS could significantly increase the production of iNOS and COX-2 mRNA. High-dose groups of compounds 5 and 8 could significantly upregulate the mRNA transcription levels of COX-2 and iNOS in normal cultured cells. Moreover, compared with the blank group, compounds 5 and 8 significantly promoted the production of COX-2 and iNOS proteins. In summary, compounds 5 and 8 could significantly promote the production of COX-2 and iNOS at the gene and protein levels.
Effects of Compounds 5 and 8 on the Expression of Proteins in NF-κB Signaling Pathway in Normal Cultured RAW264.7 Cells
The NF-κB pathway is crucial to cellular and body immunity, inflammatory response, and apoptosis. As shown in Figure 4E, the results showed that, compared with the blank group, compounds 5 and 8 could promote the expression of key proteins p-IκBα and p-p65 in the NF-κB signaling pathways of RAW264.7 cells in normal culture, thus moderately activating NF-κB signaling pathways and enhancing immunity.
Discussion
Our immune response system is continuously conducting on protecting the host from microbes by recognizing, answering, and acting on antigens (37). Generally, macrophages are crucial to innate cellular immune responses and play a significant role in the phagocyte cell system, which is programmed to identify, engulf, and eliminate apoptotic cells, bacteria, etc. (10, 38). The immune system functions to maintain the physiological health of the body and protect it from pathogens through an increased release of inflammatory cytokines (39). Cytokines released by different cells with specific effects on cellular signaling through combination to their receptors on the cell surface are essential modulators of the immune process via a complex network engaged in various immune processes, which include proliferation, phagocytosis, and inflammation (40, 41).
The NF-κB pathway is crucial to cellular and body immunity, inflammatory response, and apoptosis. The NF-κB pathway activation leads to the expression of genes associated with inflammation and immunoregulation and the release of pro-inflammatory cytokines and chemokines, which eventually results in the transcription of genes (42). It participates in many biological processes such as apoptosis, cellular immune regulation, inflammatory reaction, and tumorigenesis by regulating the expression of related inflammatory factors, chemokines, growth factors, COX-2, and nitric oxide synthase (NOS) (43). Thus, reducing the translocation of NF-κB can decrease the production of pro-inflammatory cytokines and inflammatory mediators like iNOS and COX-2 (44). The NF-κB in the cytoplasm binds to its inhibitor IκB in the resting state to form a trimer p50-p65-IκB, which is in an inactive state. When stimulated by LPS, IκB is phosphorylated by its kinase IKK and under the action of the ubiquitin enzyme, p50-p65-IκB is disintegrated and NF-κB translocation into the nucleus is phosphorylated and activated, which mediates a series of downstream reactions (Figure 5) (45).
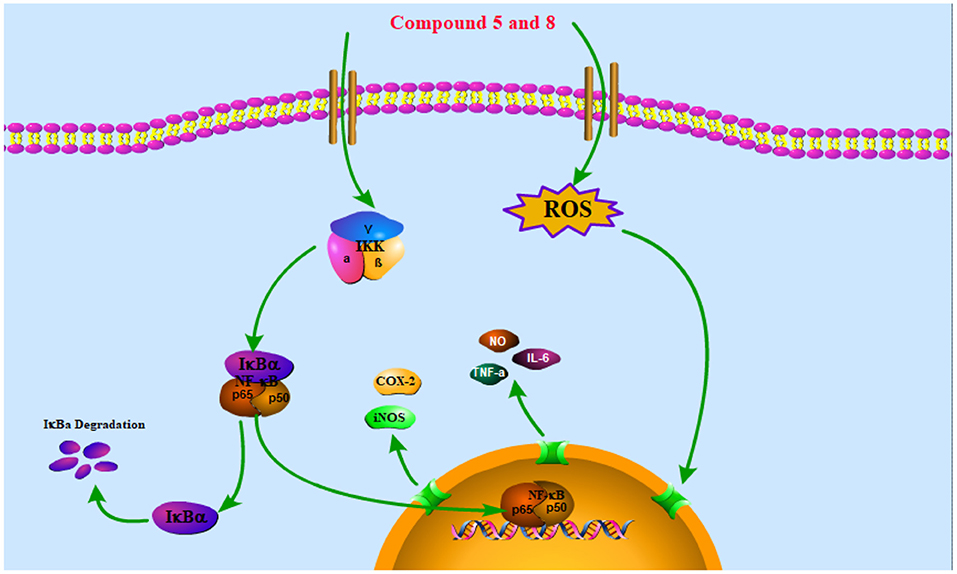
Figure 5. Schematic of the potential mechanisms of the effects of compounds 5 and 8 on the NF-κB signaling pathway in RAW264.7 macrophages. Compounds 5 and 8 exert anti-inflammatory and immune bidirectional regulation by promoting reactive oxygen species (ROS) generation and affecting the nuclear factor-κB (NF-κB) signaling pathway as discussed in the text. The arrows indicate the direction of the signaling pathway.
In this study, monosaccharide derivatives isolated from N. sativa seeds were studied to illustrate their effect on RAW 264.7 macrophage. To evaluate the immunobiological activity of these monosaccharide derivatives, studies based on various immune responses containing cytotoxicity, phagocytosis, transcription, and expression were used. As a result, we found two monosaccharide derivatives (5, 8) (Figure 1) that showed a two-way regulation of immunity and anti-inflammation on normal and LPS-stimulated RAW264.7 macrophages in vitro through regulating the expression of key proteins in the NF-κB pathway. Monosaccharide derivatives might be possibly used in immunomodulating on the basis of their function to affect the macrophage. In addition, investigation has also shown that arabinoxylans with immunomodulatory effect could be considered effective bioactive food supplements associated with many health amelioration functions (46). In vitro immunobiological evaluation of chitin- and chitosan-derived oligosaccharides on RAW 264.7 cells obtained results that indicated a beneficial immunomodulation effect referring to the development of cytokine release, phagocytosis, cell proliferation, and respiratory burst (10).
Nutrition plays a significant role in every stage of the immune response. In this regard, food containing balanced nutrients with therapeutic functions play an essential role in promoting the immunity of individuals (46). Nutritional interventions, particularly the beneficial constituents with special immune and anti-inflammatory effects, show the potential to regulate and enhance the immune system (47, 48). Since the assessment of the efficacy of plants is laborious, conducting research on more homogeneous populations to take the heterogeneity of the plant preparations into account should be considered in the future (49). Despite the fact that dietary supplements have appealing and considerable interest to nutritionists, the safety and effectiveness of these dietary supplements are still a highly controversial issue (50, 51).
N. sativa seeds are quite safe and effective for treating patients with chronic disease (52–55). Thymoquinone obtained in N. sativa seeds can reduce oxidative stress and recover the balance between anti- and pro-inflammatory cytokines (56). Another study indicated that supplementing 2 g/day of N. sativa oil capsules showed considerable improvements on cardiometabolic parameters of high-density lipoprotein cholesterol (HDL-C), low-density lipoprotein cholesterol (LDL-C), and glutamic-oxaloacetic transaminase (GOT) levels (57) of the serum. However, further research into clinical situations might be required to evaluate the functions of N. sativa seeds on the inflammation and immune mechanisms responsible for this behavior. Every supplementation could be considered prudently by the nutritionist and used within the recommended safety dose (58). In addition, monosaccharide derivatives are generally found at low levels in our diet, providing essential contribution by some dietary habits. More growing research with reliable and advanced technologies could be carried out to assess the dietary intake of monosaccharide derivatives in the future.
N. sativa seeds were applied as value immune modulators in the therapy of chronic diseases (14). Thus, taking both properties of the compounds and the optimal dose into account, research on N. sativa food supplements can provide fundamental information regarding body health suggestions with N. sativa in the future, especially against chronic inflammation which leads to some diseases. Our research indicated that an N. sativa supplement might be beneficial as a complementary method for therapy of immune and inflammatory complications in patients.
Conclusion
A new monoterpene glucoside (1) and 11 known compounds (2–12) were isolated and identified in N. sativa seeds. Monosaccharide derivatives 5 and 8 exerted bidirectional regulatory effects on immunity and anti-inflammation through NF-κB signaling pathways. The mechanism of immunity may relate to the increased release of cytokines and the level of mRNA transcription in normal RAW264.7 cells, and anti-inflammatory properties may associate with the inhibition of the excessive release of pro-inflammatory cytokines and excessive transcription at the mRNA level under LPS stimulation. These data will be valuable for further research on N. sativa seeds as a dietary supplement for immune-modulation.
Data Availability Statement
The original contributions presented in the study are included in the article/Supplementary Material, further inquiries can be directed to the corresponding author/s.
Author Contributions
YN and BW performed the experiments, wrote, and prepared the original draft. LZ analyzed and summarized data. CM and ZL critically reviewed the manuscript. AA contributed to the data acquisition. GW and DS-W supervised project administration. WK provided resources, funding, and reviewed the manuscript. All authors contributed to the article and approved the submitted version.
Funding
This work was funded by the Research on Precision Nutrition and Health Food, Department of Science and Technology of Henan Province (CXJD2021006).
Conflict of Interest
The authors declare that the research was conducted in the absence of any commercial or financial relationships that could be construed as a potential conflict of interest.
Publisher's Note
All claims expressed in this article are solely those of the authors and do not necessarily represent those of their affiliated organizations, or those of the publisher, the editors and the reviewers. Any product that may be evaluated in this article, or claim that may be made by its manufacturer, is not guaranteed or endorsed by the publisher.
Supplementary Material
The Supplementary Material for this article can be found online at: https://www.frontiersin.org/articles/10.3389/fnut.2021.722813/full#supplementary-material
Abbreviations
NO, nitric oxide; COX, cyclooxygenase; IL, interleukin; NF-κB, nuclear factor-κB; gDNA, genomic DNA; PVDF, polyvinylidene fluoride; RNA, ribonucleic acid; PBS, phosphate buffered saline; iNOS, inducible nitric oxide synthase; FBS, fatal bovine serum; PCR, polymerase chain reaction; IκB, inhibitor of NF-κB; LPS, lipopolysaccharide; ELISA, enzyme-linked immunosorbent assay; DMSO, dimethyl sulfoxide; cDNA, complementation deoxyribonucleic acid; BCA, butyleyanoacrylate; GAPDH, glyceraldehyde-3-phosphate dehydrogenase; TNF, tumor necrosis factor; DMEM, Dulbecco's modification of eagle's medium; SDS-PAGE, sodium dodecyl sulfate-polyacrylamide gel electrophoresis; MTT, 3-(4, 5-Dimethylthiazol-2-yl)-2,5-diphenyltetrazolium bromide; COVID-19, coronavirus disease 2019; T2DM, type 2 diabetes mellitus; ROS, reactive oxygen species; HDL-C, high-density lipoprotein cholesterol; LDL-C, low-density lipoprotein cholesterol; GOT, glutamic-oxaloacetic transaminase; HMBC, heteronuclear multiple-bond correlation; HPLC, high-pressure liquid chromatography; TLC, thin layer chromatography.
References
1. Li M, Dong L, Du H, Bao Z, Lin S. Potential mechanisms underlying the protective effects of Tricholoma matsutake singer peptides against LPS-induced inflammation in RAW264.7 macrophages. Food Chem. (2021) 2021:129452. doi: 10.1016/j.foodchem.2021.129452
2. Mohammad NS, Fazel NS, Antoni S, Xiao JB, Dehpour AR, Shirooie S, et al. Anti-inflammatory effects of Melatonin: a mechanistic review. Rit Rev Food Sci. (2018) 1:62. doi: 10.1080/10408398.2018.1487927
3. Calder PC, Ahluwalia N, Brouns F, Buetler T, Clement K, Cunningham K, et al. Dietary factors and low-grade inflammation in relation to overweight and obesity. Brit J Nutr. (2011) 106:S5. doi: 10.1017/S0007114511005460
4. Sun HX, Zhang J, Chen FY, Chen XF, Zhou ZH, Wang H. Activation of RAW264.7 macrophages by the polysaccharide from the roots of Actinidia eriantha and its molecular mechanisms. Carbohydr Polym. (2015) 121:388–402. doi: 10.1016/j.carbpol.2014.12.023
5. Maione F, Russo R, Khan H, Mascolo N. Medicinal plants with anti-inflammatory activities. Nat Prod Res. (2016) 30:1343–52. doi: 10.1080/14786419.2015.1062761
6. Xie Z, Wang Y, Huang J, Qian N, Shen G, Chen L. Anti-inflammatory activity of polysaccharides from Phellinus linteus by regulating the NF-κB translocation in LPS-stimulated RAW264.7 macrophages. Int J Biol Macromol. (2019) 129:61–7. doi: 10.1016/j.ijbiomac.2019.02.023
7. Xiang H, Sun DX, Cui C. Hypoglycemic polysaccharides from Auricularia auricula and Auricularia polytricha inhibit oxidative stress, NF-κB signaling and proinflammatory cytokine production in streptozotocin-induced diabetic mice. Food Sci Hum Well. (2021) 10:87–93. doi: 10.1016/j.fshw.2020.06.001
8. Zhe R, Tao Q, Qiu F, Song YL, Lin DD, Ma YF, et al. Immunomodulatory effects of hydroxyethylated Hericium erinaceus polysaccharide on macrophages RAW264.7. Int J BiolMacromol. (2017) 105:879. doi: 10.1016/j.ijbiomac.2017.07.104
9. Chung JW, Oh MJ, Cho YH, Moon GJ, Kim GM, Chung CS, et al. Distinct roles of endothelial dysfunction and inflammation in intracranial atherosclerotic stroke. Eur Neurol. (2017) 77:211–9. doi: 10.1159/000460816
10. Tsvetkov YE, Pauloviová E, Pauloviová L, Farka P, Nifantiev NE. Synthesis of biotin-tagged chitosan oligosaccharides and assessment of their immunomodulatory activity. Front Chem. (2020) 8:554732. doi: 10.3389/fchem.2020.554732
11. Wu X, Schauss AG. Mitigation of inflammation with foods. J Agri Food Chem. (2012) 60:6703–17. doi: 10.1021/jf3007008
12. Ruan MC, Bu YR, Wu FJ, Zhang SJ, Chen RL, Li N, et al. Chronic consumption of thermally processed palm oil or canola oil modified gut microflora of rats. Food Sci Hum Well. (2020) 10:94–102. doi: 10.1016/j.fshw.2020.06.005
13. Thirumdas R, Kothakota A, Pandiselvam R, Bahrami A, Barba FJ. Role of food nutrients and supplementation in fighting against viral infections and boosting immunity: A review. Trends Food Sci Technol. (2021) 110:66–77. doi: 10.1016/j.tifs.2021.01.069
14. El-Hack M, Alagawany M, Farag MR, Tiwari R, Karthik K, Dhama K. Nutritional, healthical and therapeutic efficacy of black cumin, in animals, poultry and humans. Internat J Pharmacol. (2016) 12:232–48. doi: 10.3923/ijp.2016.232.248
15. Grivennikov SI, Karin M. Dangerous liaisons: STAT3 and NF-κB collaboration and crosstalk in cancer. Cytokine Growth F R. (2010) 21:11–9. doi: 10.1016/j.cytogfr.2009.11.005
16. Li YL, Wang ZS, Liu B, Chen WY, Liu JY, Muhuyati W. Advance in research on chemical constituents and pharmacological effects of Nigella seeds. Chin Pharm J. (2016) 51:1157–61. doi: 10.11669/cpj.2016.14.001
17. Maojudai Y. Research values and the utilization prospects of Nigella plants. J Xinjiang Normal University. (2011) 30:37–41. doi: 10.3969/j.issn.1008-9659.2011.01.008
18. Ali BH, Blunden G. Pharmacological and toxicological properties of Nigella sativa. Phytother Res. (2003) 17:299. doi: 10.1002/ptr.1309
19. Niu Y, Zhou L, Meng LJ, Chen ST, Ma CY, Liu ZH, et al. Recent progress on chemical constituents and pharmacological effects of the genus Nigella. Evid-based Compl Alt. (2020) 2020:1–15. doi: 10.1155/2020/6756835
20. Ahmad A, Husain A, Mujeeb M, Khan SA, Najmi AK, Siddique NA, et al. A review on therapeutic potential of Nigella sativa: A miracle herb. Asian Pac J Trop Bio. (2013) 3:337–52. doi: 10.1016/S2221-1691(13)60075-1
21. Ahmed AF, He N, Xia ZY, Kang WY. Total phenolic and flavoniod content and antioxidant properties of Nigella sativa L. seeds. Curr Top Nutraceut Res. (2018) 16:147–54.
22. Dong J, Liang Qx, Niu Y, Jiang SJ, Kang WY. Effects of Nigella sativa seed polysaccharides on type 2 diabetic mice and gut microbiota. Int J Biol Macromol. (2020) 159:725–38. doi: 10.1016/j.ijbiomac.2020.05.042
23. Zahra GN, Shahrzad HK, Mohammad HB. Preclinical and clinical effects of Nigella sativa and its constituent, thymoquinone: A review. J Ethnopharmacol. (2016) 190:372–86. doi: 10.1016/j.jep.2016.06.061
24. Ma C, Liu C, Ahmed A F, Niu YY, Kang WY. Optimum extraction technology for the seed oil of Nigella sativa L. J Food Quality. (2019) 2019:1–6. doi: 10.1155/2019/2592731
25. Sayed S, Aboonq M S, Rashedy A, Aljehani YT, Soliman TM. Promising preventive and therapeutic effects of TaibUVID nutritional supplements for COVID-19 pandemic: towards better public prophylaxis and treatment (A retrospective study). Am J Blood Res. (2020) 10:266–82.
26. Koshak AE, Koshak EA, Mobeireek AF, Badawi MA, Madani TA. Nigella sativa supplementation to treat symptomatic mild COVID-19: A structured summary of a protocol for a randomised, controlled, clinical trial. Trials. (2020) 21:703. doi: 10.1186/s13063-020-04647-x
27. Kooshki A, Tofighiyan T, Rastgoo N, Rakhshani MH, Mohammad M. Effect of Nigella sativa oil supplement on risk factors for cardiovascular diseases in patients with type 2 diabetes mellitus. Phytother Res. (2020) 34:1–6. doi: 10.1002/ptr.6707
28. Ruan Q, Chen YH. Nuclear factor-κB in immunity and inflammation: the Treg and Th17 connection. Curr Topics Innate Immunity II. (2012) 2012:207–21. doi: 10.1007/978-1-4614-0106-3_12
29. Alshatwi Ali A. Bioactivity-guided identification to delineate the immunomodulatory effects of methanolic extract of Nigella sativa seed on human peripheral blood mononuclear cells. Chin J Integr Med. (2014) 2:1–6. doi: 10.1007/s11655-013-1534-3
30. Sandhya A, Kannayiram G. Pharmacological, bioactive screening of medicinal plant Nigella sativa and the derived compound thymoquinone: An in vitro study. Int Jl Res Pharml Sci. (2020) 11:2458–65. doi: 10.26452/ijrps.v11i2.2239
31. Rahman AU, Hasan SS, Choudhary MI, et al. Nigellidine- a new indazole alkaloid from the seeds of Nigella sativa. Tetrahedron Lett. (1995) 36:1993–6. doi: 10.1016/0040-4039(95)00210-4
32. Gupta M, Mehta BK. A comprehensive review on secondary metabolites of Nigella sativa (Seeds). Res J Chem Sci. (2017) 7:16–29.
33. Morikawa T, Xu FM, Kashima YS, Matsuda H, Ninomiya K, Yoshikawa M. Novel dolabellane-type diterpene alkaloids with lipid metabolism promoting activities from the seeds of Nigella sativa. Org Lett. (2004) 6:869–72. doi: 10.1002/chin.200432213
34. Liu ZH, Ma RJ, Yang L, Li JY, Zhou J. Triterpenoids and iridoids from Patrinia scabiosaefolia. Fitoterapia. (2017) 119:130–5. doi: 10.1016/j.fitote.2017.04.011
35. Wang HL, Xu XQ, Yin ZH, Wang MK, Wang BG, Ma CY, et al. Activation of RAW264.7 cells by PCp-I, a polysaccharide from Psoralea corylifolia L, through NF-κB/MAPK signalling pathway. Int J Immunopath Ph. (2021) 35:1–12. doi: 10.1177/20587384211010058
36. Zhang H, Guo Q, Liang Z, Wang M, Wang B, Sun DX, et al. Anti-inflammatory and antioxidant effects of Chaetoglobosin Vb in LPS-induced RAW264.7 cells: Achieved via the MAPK and NF-κB signaling pathways. Food Chem Toxicol. (2020) 147:111915. doi: 10.1016/j.fct.2020.111915
37. Fernandez MI, Pedron T, Tournebize R, Olivo-Marin JC, Sansonetti PJ, Phalipon A. Anti-inflammatory role for intracellular dimeric immunoglobulin a by neutralization of lipopolysaccharide in epithelial cells. Immunity. (2003) 18:739–49. doi: 10.1016/S1074-7613(03)00122-5
38. Li C, Cui Y, Lu J, Meng L, Ma C, Liu Z, et al. Spectrum-effect relationship of immunologic activity of Ganoderma lucidum by UPLC-MS/MS and component knock-out method. Food Sci Hum Well. (2021) 10:278–88. doi: 10.1016/j.fshw.2021.02.019
39. Ilaria P, Cristiana M, Giuseppa M, Francesca I, Mauro S. Flavonoids and immune function in human: a systematic review. Crit Rev Food Sci. (2015) 55:383–95. doi: 10.1080/10408398.2012.656770
40. Mollazadeh H, Arrigo FG, Christopher NB, Matteo P, Muhammed M, Amirhossein S. Immune modulation by curcumin: The role of interleukin-10. Crit Rev Food Sci Nutr. (2017) 59:89–101. doi: 10.1080/10408398.2017.1358139
41. He J, Lu X, Wei T, Dong YQ, Cai Z, Tang L, et al. Asperuloside and asperulosidic acid exert an anti-inflammatory effect via suppression of the NF-κB and MAPK signaling pathways in LPS-induced RAW 264.7 macrophages. Int J Mol Sci. (2018) 19:2027. doi: 10.3390/ijms19072027
42. Tak PP, Firestein GS. NF-κB: A key role in inflammatory diseases. J Clin Investig. (2001) 107:711. doi: 10.1172/JCI11830
43. Liu C, Guo Y, Zhao F, Qin H, Lu H, Fang L, et al. Potential mechanisms mediating the protective effects of a peptide from walnut (Juglans mandshurica Maxim.) against hydrogen peroxide induced neurotoxicity in PC12 cells. Food Funct. (2019) 10:3491–501. doi: 10.1039/C8FO02557F
44. Craig R, Larkin A, Mingo AM, Thuerauf DJ, Andrews C, McDonough PM. p38 MAPK and NF-κB collaborate to induce interleukin-6 gene expression and release evidence for a cytoprotective autocrine signaling pathway in a cardiac myocyte model system. J Biol Chem. (2000) 275:23814–24. doi: 10.1074/jbc.M909695199
45. Viatour P, Merville MP, Bours V, Chariot A. Phosphorylation of NF-κB and IκB proteins: Implications in cancer and inflammation. Trends Biochem Sci. (2005) 30:43–52. doi: 10.1016/j.tibs.2004.11.009
46. Levine R. Monosaccharides in Health and Disease. Annu Rev Nutr. (1986) 6:211–24. doi: 10.1146/annurev.nu.06.070186.001235
47. Barrea L, Muscogiuri G, Frias-Toral E, Laudisio D, Pugliese G, Castellucci B, et al. Nutrition and immune system: from the Mediterranean diet to dietary supplementary through the microbiota. Crit Rev Food Sci. (2020) 3:1–25. doi: 10.1080/10408398.2020.1792826
48. Li X, Cheung P. Application of natural β-glucans as biocompatible functional nanomaterials. Food Sci Hum Well. (2019) 8:315–9. doi: 10.1016/j.fshw.2019.11.005
49. Riaz M, Rahman NU, Zia-Ul-Haq M, Jaffar HZ, Manea R. Ginseng: A dietary supplement as immune-modulator in various diseases. Trends Food Sci Tech. (2018) 83:12–30. doi: 10.1016/j.tifs.2018.11.008
50. Marik PE, Flemmer M. Do dietary supplements have beneficial health effects in industrialized nations: What is the evidence? JPEN-Parenter Enter Nutr. (2012) 36:159–68. doi: 10.1177/0148607111416485
51. Dwyer JT, Coates PM, Smith MJ. Dietary supplements: regulatory challenges and research resources. Nutrients. (2018) 10:41. doi: 10.3390/nu10010041
52. Gholamnezhada Z, Keyhanmanesh R, Boskabady MH. Anti-inflammatory, antioxidant and immunomodulatory aspects of Nigella sativa for its preventive and bronchodilatory effects on obstructive respiratory diseases: A review of basic and clinical evidence. J Funct Foods. (2015) 17:910–27. doi: 10.1016/j.jff.2015.06.032
53. Eid AM, Elmarzugi NA, Abu Ayyash LM, Sawafta MN, Daana HI. A review on the cosmeceutical and external applications of Nigella sativa. J Trop Med-US. (2017) 22:1–6. doi: 10.1155/2017/7092514
54. Umar S, Zargan J, Umar K, Ahmad S, Katiyar CK. Modulation of the oxidative stress and inflammatory cytokine response by thymoquinone in the collagen induced arthritis in Wistar rats. Chem Biol Interact. (2012) 197:40–6. doi: 10.1016/j.cbi.2012.03.003
55. Lei X, Lv X, Meng L, Yang ZR, Ji MY, Guo XF, et al. Thymoquinone inhibits growth and augments 5-fluorouracil-induced apoptosis in gastric cancer cells both in vitro and in vivo. Biochem Bioph Res Co. (2012) 417:864–8. doi: 10.1016/j.bbrc.2011.12.063
56. Elsherbiny NM, Maysarah NM, El-Sherbiny M, Al-Gayyar MM. Renal protective effects of thymoquinone against sodium nitrite-induced chronic toxicity in rats: Impact on inflammation and apoptosis. Life Sci. (2017) 180:1–8. doi: 10.1016/j.lfs.2017.05.005
57. Razmpoosh E, Safi S, Nadjarzadeh A, Hossien F, Nooshin A, Mahta M, et al. The effect of Nigella sativa supplementation on cardiovascular risk factors in obese and overweight women: a crossover, double-blind, placebo-controlled randomized clinical trial. Eur J Nutr. (2020) 60:1863–74. doi: 10.1007/s00394-020-02374-2
Keywords: Nigella sativa, monoterpene glucoside, monosaccharide derivatives, immune, anti-inflammatory, RAW264.7
Citation: Niu Y, Wang B, Zhou L, Ma C, Waterhouse GIN, Liu Z, Ahmed AF, Sun-Waterhouse D and Kang W (2021) Nigella sativa: A Dietary Supplement as an Immune-Modulator on the Basis of Bioactive Components. Front. Nutr. 8:722813. doi: 10.3389/fnut.2021.722813
Received: 09 June 2021; Accepted: 16 July 2021;
Published: 17 August 2021.
Edited by:
Quancai Sun, Jiangsu University, ChinaReviewed by:
Guoxun Chen, The University of Tennessee, Knoxville, United StatesXia Ma, Shanghai Institute of Technology, China
Copyright © 2021 Niu, Wang, Zhou, Ma, Waterhouse, Liu, Ahmed, Sun-Waterhouse and Kang. This is an open-access article distributed under the terms of the Creative Commons Attribution License (CC BY). The use, distribution or reproduction in other forums is permitted, provided the original author(s) and the copyright owner(s) are credited and that the original publication in this journal is cited, in accordance with accepted academic practice. No use, distribution or reproduction is permitted which does not comply with these terms.
*Correspondence: Zhenhua Liu, bGl1emhlbmh1YTYyMyYjeDAwMDQwOzE2My5jb20=; Adel F. Ahmed, YWRlbGZhaG1pXzIwMDgmI3gwMDA0MDt5YWhvby5jb20=; Dongxiao Sun-Waterhouse, ZHhzdW43MiYjeDAwMDQwO2hvdG1haWwuY29t; Wenyi Kang, a2FuZ3dlbnkmI3gwMDA0MDtob3RtYWlsLmNvbQ==
†These authors have contributed equally to this work