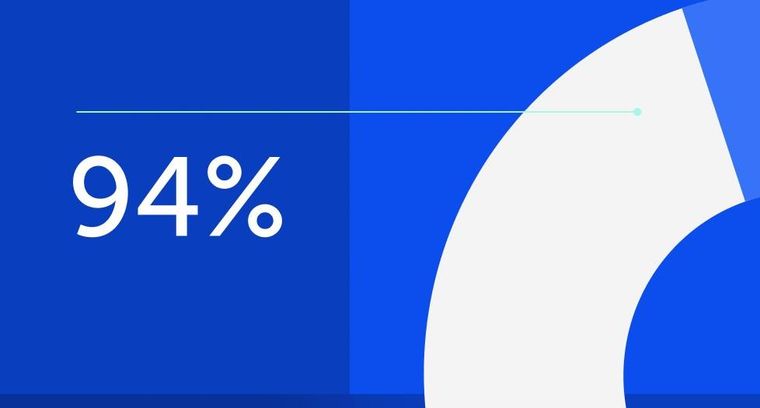
94% of researchers rate our articles as excellent or good
Learn more about the work of our research integrity team to safeguard the quality of each article we publish.
Find out more
REVIEW article
Front. Nutr., 16 July 2021
Sec. Clinical Nutrition
Volume 8 - 2021 | https://doi.org/10.3389/fnut.2021.702802
Very-low-carbohydrate ketogenic diets have been long been used to reduce seizure frequency and more recently have been promoted for a variety of health conditions, including obesity, diabetes, and liver disease. Ketogenic diets may provide short-term improvement and aid in symptom management for some chronic diseases. Such diets affect diet quality, typically increasing intake of foods linked to chronic disease risk and decreasing intake of foods found to be protective in epidemiological studies. This review examines the effects of ketogenic diets on common chronic diseases, as well as their impact on diet quality and possible risks associated with their use. Given often-temporary improvements, unfavorable effects on dietary intake, and inadequate data demonstrating long-term safety, for most individuals, the risks of ketogenic diets may outweigh the benefits.
Very-low-carbohydrate (ketogenic) diets have been promoted for weight loss and, less commonly, for other health reasons. This review summarizes the effects of a ketogenic diet on health conditions for which it has been promoted, as well as potential long-term effects on health.
The term “ketogenic diet” generally refers to a diet that is very low in carbohydrate, modest in protein, and high in fat. This mix of fuels aims to induce ketosis, or the production of ketone bodies that serve as an alternate energy source for neurons and other cell types that cannot directly metabolize fatty acids. Urinary ketone levels are often used as an indicator of dietary adherence (1).
Various ketogenic diets have been studied, as shown in Table 1. The best defined and studied is sometimes called a “classic” ketogenic diet, referring to a very-low-carbohydrate diet that is generally medically supervised, with a 4:1 or 3:1 ratio, by weight, of dietary fat to combined dietary protein and carbohydrate (2).
Other variants allow more protein or carbohydrate (2). Ketogenic diets as typically implemented in scientific studies limit dietary carbohydrate to <50 g per day with varying amounts of fat and protein (3, 4). “Low-carbohydrate diets” refer to carbohydrate intake below the recommended dietary allowance of 130 g/day (3), which may not be low enough to induce ketosis (5).
During prolonged fasting, some tissues, such as muscle, can directly metabolize free fatty acids released from adipose stores. However, much of this fatty acid is converted into ketones in the liver, which can fuel otherwise-obligate glucose consumers like neurons, minimizing mobilization of body protein for gluconeogenesis. However, to induce the liver to make ketones in the fed state, carbohydrate intake must be minimized and fat intake increased. Protein utilization is also altered on a ketogenic diet; the body shunts as much protein as possible to gluconeogenesis, while the minimum necessary amount is used for tissue repair.
Extreme carbohydrate restriction can profoundly affect diet quality, typically curtailing or eliminating fruits, vegetables, whole grains, and legumes and increasing consumption of animal products. Very-low-carbohydrate diets may lack vitamins, minerals, fiber, and phytochemicals found in fruits, vegetables, and whole grains (6–8). Low-carbohydrate diets are often low in thiamin, folate, vitamin A, vitamin E, vitamin B6, calcium, magnesium, iron, and potassium (9). In the absence of multivitamin supplements, individuals on low-carbohydrate diets are at risk of frank nutritional deficiencies (10). Even when consuming only nutrient-dense foods, a 4:1 ketogenic diet is reported to have multiple micronutrient shortfalls, often lacking in vitamin K, linolenic acid, and water-soluble vitamins excluding vitamin B12 (11).
Ketogenic diets are typically low in fiber needed not only for healthful intestinal function but also for microbial production of beneficial colonic short-chain fatty acids (12), which enhance nutrient absorption, stimulate the release of satiety hormones, improve immune function, and have anti-inflammatory and anti-carcinogenic effects (13, 14). Inadequate intake of these microbiota-accessible carbohydrates found in plant cell walls also increases gut permeability, as bacteria extract the carbon they need from the mucus membrane that protects the gastrointestinal tract instead of fiber (15). The relative abundance of certain health-promoting, fiber-consuming bacteria has been found to be reduced in children consuming a ketogenic diet for epilepsy (16). It has been suggested that supplementation of ketogenic diets with fiber and non-digestible carbohydrates might be advisable (16), although data to confirm that supplementation could counteract the effects of very-low-carbohydrate diets on the gut microbiota are lacking.
Intake of other protective dietary components may also be insufficient, such as phytochemicals (e.g., flavanones and anthocyanins), which are not typically included in multivitamins and for which specific intake targets have not been established. Low-carbohydrate diets are also typically high in saturated fat and cholesterol (10).
Worldwide, the lifetime prevalence of epilepsy is 7.6 per 1,000 people (17). According to a 2018 Cochrane Review, most affected individuals can eliminate seizures with medication, but about 30% cannot. Some one-third to one-half of people with drug-resistant epilepsy can reduce seizure frequency by at least 50% with a ketogenic diet (18). The lack of glucose available to fuel neurons is a possible mechanism for action (19).
Long-term adherence is challenging, as food choices are limited and adverse effects are common (18). Micronutrient supplementation is required. Potential health risks accompany the long-term use of such a diet, as described below. Research has shown that modified versions of the ketogenic diet allowing for more carbohydrates have also been somewhat effective in seizure reduction (19). Most studies have not been long term, large scale, nor conducted with adult participants; therefore, more research is needed.
Ketogenic diets can induce weight loss (20–23). In a 2020 meta-analysis of 38 studies lasting 6–12 months and including 6,499 participants, low-carbohydrate diets, defined here as <40% of energy from carbohydrate, led to a small weight loss, compared with low-fat diets, defined as <30% of energy from fat (mean difference −1.30 kg; 95% CI, −2.02 to −0.57), with considerable variability between individuals and between studies. More than half of included studies met criteria for a general ketogenic diet, as defined in Table 1, for part or all of the low-carbohydrate intervention (24).
It has been proposed that weight loss on ketogenic diets may be due to reduced appetite (25), an effect also seen in those following balanced, very-low-energy diets (<800 kcal/day). Since ketosis occurs on both types of diets, though to a lesser degree with very-low-energy diets, it is speculated that ketosis itself may decrease hunger (26). However, findings from a recent trial by Hall et al. suggest that a low-fat vegan diet (10% energy from fat) may be more effective than a ketogenic diet in suppressing appetite (27). Energy expenditure has also been shown to increase on a ketogenic diet, at least in short-term studies (27, 28).
In controlled trials, low-carbohydrate diets appear no more effective than other diets that similarly restrict calories (29), nor are they more effective than other dietary interventions, such as low-fat vegetarian diets, at inducing weight loss (30, 31). A 2013 meta-analysis of randomized controlled trials testing very-low-carbohydrate ketogenic diets (≤50 g carbohydrate/day or ≤10% kcal from carbohydrates) against diets based on modest reductions in fat intake (<30% kcal from fat) for at least 1 year found that ketogenic diets led to marginally more weight loss than reduced-fat diets (weighted mean difference: −0.91 kg; 95% CI, −1.65 kg to −0.17 kg, p = 0.02). However, no statistically significant difference in amount of weight lost was seen between the 2 diets in trials following people for at least 2 years (3).
A 2017 meta-analysis of 9 trials echoed these findings. In studies <12 months long, low-carbohydrate diets (<130 g carbohydrate/day or <26% kcal from carbohydrates) were seen to lead to greater weight loss in people with type 2 diabetes relative to normal- or high-carbohydrate control diets (weighted mean difference: −1.18 kg; 95% CI, −2.32 kg to −0.04 kg; p = 0.04). No advantage was seen relative to control diets in studies of longer duration (weighted mean difference: −0.24 kg; 95% CI, −2.18 kg to 1.7 kg; p = 0.81) (32).
At least initially, ketogenic diets may slow fat loss. In a 2016 metabolic ward study by Hall et al., 17 overweight or obese men were provided a baseline diet (50% carbohydrate, 35% fat, and 15% protein, as a percent of energy) for 4 weeks, then a ketogenic diet (5% carbohydrate, 80% fat, 15% protein) for 4 weeks. For 2 weeks after switching from the baseline diet to the ketogenic diet, participants' weight loss accelerated—but fat loss slowed. The authors attributed the additional weight loss primarily to loss of body water. However, loss of body protein may have contributed; urinary nitrogen levels increased through day 11 on the ketogenic diet. In the final 2 weeks on the ketogenic diet, participants' rates of body weight and fat loss rebounded to a rate comparable to that on the baseline diet. As a result, study participants required 4 weeks on a ketogenic diet to lose the same average 0.5 kg of fat lost in the final 2 weeks on a baseline diet. It is not clear whether these effects have longer-term consequences (28).
The 2021 metabolic ward study by Hall et al. tested the effects of both an animal-based ketogenic diet (76% energy from fat, 10% carbohydrate) and a plant-based, low-fat diet (75% carbohydrate, 10% fat) on 20 weight-stable adults, mean age 29.9 years, mean BMI 27.8 kg/m2 (27). Participants were randomized to each diet, which they consumed ad libitum for 2 weeks before immediately crossing over to the other diet. Ad libitum energy intake was 689 kcal/day lower on the low-fat, plant-based diet as compared to the ketogenic diet (p < 0.0001). Reported hunger and satisfaction were similar between groups. Both diets induced weight loss: 1.77 ± 0.32 kg (p < 0.0001) for the ketogenic diet vs. 1.09 ± 0.32 kg (p = 0.003) for the low-fat diet. However, most of the weight lost on the ketogenic diet came from fat-free mass (-1.61 ± 0.27 kg; p < 0.0001); this was not the case with the low-fat diet (−0.16 ± 0.27 kg; p = 0.56). Fat mass did not significantly change during either the first or second week of the ketogenic diet, while the low-fat diet led to significant losses in body fat after both the first and second weeks. This suggests that low-fat, plant-based diets may control appetite better than ketogenic diets. These results also add to evidence suggesting that the rapid initial weight loss observed on ketogenic diets is due predominantly to loss of fat-free mass (e.g., body water, glycogen, protein, and contents of the gastrointestinal tract) (27).
Although ketogenic diets can improve glycemia in pediatric patients with type 1 diabetes, they are generally not used in this population due to the risk of malnutrition, failure to thrive, reduced bone density, hyperlipidemia, poor sleep, amenorrhea, and hypoglycemia. In addition, mood and behavior may be adversely affected (33).
In adults with type 1 diabetes, both favorable and unfavorable outcomes have been observed. A small study of 11 adults with type 1 diabetes reported that a ketogenic diet improved blood glucose control (34). However, the ketogenic diet triggered more frequent and extreme hypoglycemic episodes (6.3 episodes per week compared to 1–2 episodes per week typically reported for those following conventional or otherwise unspecified diets). The majority of participants also developed dyslipidemia. Lipid changes are of particular concern in individuals with diabetes, who are already at heightened risk of cardiovascular events (34).
A comprehensive review strongly discouraged sustained ketosis or hyperketonemia in individuals with type 1 diabetes (35). Due to metabolic irregularities associated with type 1 diabetes, ketone production is elevated, and ketone clearance is diminished. Individuals with elevated ketones are at increased risk for complications of the microvasculature, brain, kidney, and liver compared to those with normal ketone levels. In type 1 diabetes, hyperketonemia is associated with oxidative stress, inflammation, non-alcoholic fatty liver disease, and insulin resistance (35).
Ketogenic diets depress appetite, promote weight loss, reduce blood glucose values, and decrease HbA1c in the short term (21, 36–43). Some studies have reported improved insulin sensitivity (40); the effect appears to be dependent on loss of fat mass (44). In the abovementioned metabolic ward study in which 17 overweight or obese men were provided a baseline diet (50% carbohydrate) for 4 weeks and then a ketogenic diet (5% carbohydrate) for 4 weeks, during the ketogenic diet phase, total cholesterol, low-density lipoprotein cholesterol (LDL-C), and C-reactive protein increased significantly, while fasting insulin and triglycerides decreased. While on the ketogenic diet, insulin sensitivity was impaired when participants were challenged with a baseline diet meal (50% carbohydrates) (45).
In the 2021 metabolic ward trial by Hall et al. comparing the effects of an animal-based ketogenic diet and a plant-based, low-fat diet, the plant-based diet had a greater glycemic load and predictably resulted in higher postprandial glucose and insulin levels than the ketogenic diet. However, glucose tolerance, as determined by an oral glucose tolerance test at the end of each phase, was compromised during the ketogenic phase (average 2-h glucose was 142.6 mg/dL) compared to the plant-based phase (average 2-h blood glucose was 108.5 mg/dL). In addition, high-sensitivity C-reactive protein, a marker of inflammation, was substantially higher while on the ketogenic diet compared to the plant-based diet (2.1 vs. 1.2 mg/L; p = 0.003), although not significantly different from baseline (27).
Another low-carbohydrate diet trial that followed individuals for 1 year found that insulin sensitivity was improved at 6 months but returned to baseline at 1 year (22). In healthy men, a ketogenic diet (83% fat and 2% carbohydrate) reduced insulin's ability to suppress endogenous glucose production (46).
A recent meta-analysis showed that reductions in hemoglobin A1c achieved with carbohydrate-restricted diets typically wane after a few months and that such diets are not more effective than other diets (47).
In other clinical trials with ketogenic diets, diabetes medications are frequently reduced or eliminated (21, 36–43). The beneficial effects of ketogenic diets for people with type 2 diabetes are attributable primarily to weight loss, with benefits appearing to wane over time (48, 49). Few additional negative impacts on global measures of health have been reported in short-term studies on type 2 diabetes (21, 37, 40). Long-term effects have not been elucidated (49).
The prospective Nurses' Health Study found no link between diets lower in carbohydrate and incident type 2 diabetes in women, although those consuming the most vegetable protein and fat had an 18% lower risk (50). The Health Professionals Follow-Up Study found that men consuming diets low in carbohydrate and high in animal protein and fat had a 37% higher risk of being diagnosed with type 2 diabetes than those who scored lowest for this diet style. Those emphasizing vegetable protein and fat on low-carbohydrate diets did not experience increased risk, and for men under 65 years of age, diabetes risk was 22% lower (51).
Dietary staples in ketogenic diets include concentrated fats, meat, poultry, fish, eggs, and cheese, all of which have been associated with increased diabetes risk (52–56). These foods can be high in saturated fat, cholesterol, chemical contaminants, pro-oxidants such as heme iron, and inflammatory compounds such as N-glycolylneuraminic acid (Neu5Gc) and endotoxins. Conversely, foods consistently associated with reduced diabetes risk, including fruits, legumes, whole grains, and several vegetables, are minimized or eliminated (52–56).
Non-alcoholic fatty liver disease (NAFLD) is a serious condition where excess fat is stored in hepatocytes, causing steatosis, which can progress to non-alcoholic steatohepatitis and increase the risk of hepatocellular carcinoma (57–60). Worldwide, the prevalence of NAFLD in adults is estimated to be 25.2%, ranging from a low of 13.5% in Africa to a high of 31.8% in the Middle East, with North America at 24.1% (61). The risk of NAFLD is significantly higher in individuals who have obesity or type 2 diabetes (43–92%) (57, 58, 62).
Hepatic triacylglycerol comes from three sources: de novo lipogenesis, primarily from glucose; lipolysis of stored triglyceride from adipose tissue; and diet-derived fats (58). Most (60–80%) triglyceride is from adipose tissue, 15% is from diet, and 5% is from de novo lipogenesis in healthy people. Triglyceride from de novo lipogenesis is much higher (26%) in individuals with NAFLD (63). Fat derived from de novo lipogenesis and adipose tissue is accelerated by insulin resistance (63).
Several clinical trials have compared low-fat and low-carbohydrate hypocaloric diets in overweight or obese adults and found similar reductions in intrahepatic fat (64–66). Ketogenic diets typically increase intake of saturated fat, cholesterol, and animal protein, all of which are associated with insulin resistance, oxidative stress, and an exacerbated flow of free fatty acids to hepatocytes (57, 62, 63, 67).
In epidemiological studies, diets high in saturated fat, trans fat, simple sugars, and animal protein (especially from red and processed meat) (57) and low in dietary fiber and omega-3 fatty acids (62, 68) are thought to contribute to NAFLD. In the Rotterdam Study, those consuming the most animal protein were 54% more likely to have NAFLD than those consuming the least (OR 1.54, 95% CI, 1.20–1.98) (68). Dietary components associated with reduced NAFLD risk include whole grains, nuts and seeds, monounsaturated fats, omega-3 fatty acids, vegetable protein, prebiotic fiber, probiotics, resveratrol, coffee, taurine, and choline (57). In the Tzu Chi Health Study, replacing one serving of soy with fish (or meat) was associated with a 12–13% increased risk of NAFLD. Whole grain intake had an inverse relationship with NAFLD, and those following a vegetarian diet had a 21% lower risk of NAFLD (69).
Lifestyle modifications, particularly diet change, weight loss, and exercise, are the primary modality for treating NAFLD (57, 62, 63). Lifestyle interventions that promote weight loss have been found to reduce liver fat and improve aminotransferase concentrations and insulin sensitivity (48, 57, 58, 62, 63, 68). It has been suggested that achieving ketosis may have a benefit in ameliorating fatty liver (63), but the studies supporting this are limited and typically also restrict energy intake. Long-term safety and specific clinical outcomes have not been determined.
Some have suggested ketogenic diets for cancer patients (70) based on the so-called “Warburg effect,” whereby cancer cells increase glucose uptake and upregulate glycolysis even in the presence of oxygen, preferentially fermenting glucose to lactate (71). By nearly eliminating available glucose, ketogenic diets theoretically stress cancer cells.
Few clinical trials have tested this. A 2018 systematic review of ketogenic diets for the management of gliomas found no randomized clinical trials and just 6 published case series/reports. While the authors could not evaluate the effectiveness of ketogenic diets for cancer survival, they noted that minimal adverse events were reported, suggesting ketogenic diets may be safe in this population (72).
A 2020 systematic review analyzed 13 studies of ketogenic diets as a complementary therapy for standard treatments in a variety of cancers. Studies analyzed were small (n = 2–44); 9 were prospective and 6 were controlled, but just 2 were randomized, and ketogenic diet prescriptions differed between studies. Diet-related adverse events were uncommon and mostly minor, and the diet had a beneficial effect on body composition. Findings were mixed for both overall survival and progression-free survival; beneficial effects were seen in four studies (73). A possible explanation for the lack of a consistent survival benefit is demonstrated in in vitro research suggesting that ketone utilization by cancer cells increases expression of genes associated with high metastatic potential (74). Given potential benefits for body composition, large, well-designed, randomized clinical trials are needed to determine the safety and effectiveness of ketogenic diets in cancer treatment (72, 73).
Long-term data on cancer outcomes with ketogenic diets are lacking. However, food components typical of a ketogenic diet, such as red and processed meats, are linked to increased cancer risk (75–77). Whole grains, fruits, and vegetables are linked to a lower risk of both cancer and all-cause mortality (78, 79), yet, with the exception of non-starchy vegetables, these foods are commonly avoided on ketogenic diets. For example, in one study of a ketogenic diet for type 2 diabetes, researchers encouraged unlimited meat, poultry, seafood, and eggs, while cutting intake of whole grains, fruits, and starchy vegetables and limiting intake of salad vegetables and non-starchy vegetables (21).
By 2050, it is projected that 13.8 million people in the U.S. will have Alzheimer's disease (AD) (80). Given the brain's inability to efficiently utilize glucose in AD, some have proposed ketones as an alternate fuel source for these individuals (81). As reviewed by Włodarek in 2019, small trials have found that increasing blood ketones by supplementing with medium-chain triglycerides does improve some measures of cognitive function in AD, although not necessarily in those with the APOEε4 genotype (82).
No long-term data on ketogenic diets for AD are available, although small, short-term trials have been conducted. A 3-month, weight-maintaining ketogenic diet intervention improved cognition in subjects with mild-to-moderate AD (n = 15), but improvements were lost after a 1-month washout period (83). A 6-week trial of a ketogenic diet in subjects with mild cognitive impairment led to improved memory relative to a control diet (50% of energy from carbohydrates); follow-up data were not available. However, the ketogenic diet was substantially lower in calories, which may have independently reduced insulin resistance (84). In a 2020 review of short-term ketogenic diet and ketone supplement studies in older adults, including those with no dysfunction, mild cognitive impairment, and AD, 6 of 9 controlled trials with clinical endpoints found significant cognitive improvements in the intervention groups, while other trials did not. Whether cognitive gains would be maintained upon discontinuation of the diet/supplement remains unknown due to lack of long-term follow-up (85).
Saturated fat intake, which typically increases on a ketogenic diet, is strongly associated with AD risk. In the Chicago Health and Aging Project, high saturated fat intake was linked to a 2- to 3-fold increased risk of incident AD (86). A 2016 review of international data found that consuming meats, eggs, high-fat dairy such as butter and cheese, and sweets was linked to an increased risk of AD (87). Aside from sweets, consumption of these foods generally increases on a ketogenic diet.
Polyphenol-rich plant foods such as fruits and vegetables are associated with lower AD risk (88) and diets focusing on whole plant foods and limiting animal foods and processed foods, such as the MIND diet, are proven to reduce AD risk (89). Thus, by providing ketones that can be metabolized by neurons in AD, a ketogenic diet could improve symptoms in the short term, but the diet's nutritional profile could increase risk over the long-term in healthy individuals.
The effect of low-carbohydrate diets on plasma lipid concentrations is a major concern. It has long been established that weight loss by any means causes a reduction in total cholesterol of about 2 mg/dL per kilogram lost (90). However, low-carbohydrate diets are often an exception to that rule. In a 2002 6-month study of a very-low-carbohydrate “Atkins” diet by Westman et al., 12 (29%) of the 41 participants had LDL-C elevations. The average increase was 18 mg/dL (91). In a similar 6-month study by Yancy, 30% of participants had LDL-C increases > 10% (92).
In a trial published in 2003 by Foster et al., LDL-C rose 6.2% in a group of low-carbohydrate dieters at 3 months (22). For comparison, LDL-C dropped by 11.1% during this same time period in participants following a conventional low-calorie diet. In a 2004 1-year study, those on a low-carbohydrate diet increased their mean LDL-C from 112 to 120 mg/dL (93). In 2018, Hallberg (94) reported a mean 10% rise in LDL-C in individuals following low-carbohydrate diets, an elevation that persisted during 2 years of follow-up (95). A recent meta-analysis of 5 studies showed that, in individuals with type 2 diabetes, ketogenic diets led to, on average, no substantial change in LDL-C (96).
It is important to note that changes reported in group means do not reflect the change for any given individual. In the 2002 study cited above, while the mean LDL-C increase was 18 mg/dL, one participant's LDL-C concentration increased from 123 to 225 mg/dL (91). In the Yancy study, one participant's LDL-C increased from to 219 mg/dL. Another experienced an LDL-C rise from 184 to 283 mg/dL, and a third developed chest pain and was subsequently diagnosed with coronary heart disease (92). In the Foster study, the standard deviation for the change in LDL-C was 20.4%, indicating that while LDL-C decreased for some, for many participants, LDL-C rose dramatically (22).
Negative effects on blood lipids have also been seen in healthy individuals. A 2018 pilot study of young, fit adults (average age 31) found that 12 weeks on a ketogenic diet led to a weight loss of 3.0 kg in the ketogenic group, with no significant weight change in the control group. However, despite significant weight loss, LDL-C increased by 35% in the ketogenic group (p = 0.048), from 114 mg/dL at baseline to 154 mg/dL at 12 weeks (97).
Some have suggested that LDL-C or LDL particle concentration elevations are of no concern if the increase is mainly in larger LDL particles. There are two problems with this rationale: First is the problem of heterogeneity noted above (i.e., individuals may have significant worsening of their lipid profiles that are not reflected by mean figures). Second, LDL is potentially atherogenic regardless of particle size (98, 99). Data supporting this concern come from the Women's Health Study, a randomized, placebo-controlled trial of low-dose aspirin and vitamin E. As part of the study, LDL particle size was assessed. The hazard ratio for incident cardiovascular disease associated with large LDL particles was 1.44 (indicating a 44% increased risk). For small LDL, it was 1.63 (a 63% increased risk). Both were highly statistically significant. In other words, large LDL particles were strongly atherogenic, albeit less so than small LDL (100).
It has also been proposed that the risk elevation associated with increased LDL-C concentrations may be neutralized to the extent that high-density lipoprotein cholesterol (HDL-C) also rises. However, both Mendelian randomization trials and studies using HDL-elevating agents have not shown benefit regarding cardiovascular risk. In the former category are studies that have examined individuals with naturally occurring genetic variants associated with elevated plasma HDL-C concentrations. These genetic traits are not associated with reduced risk of myocardial infarction unless they also reduce LDL-C (101).
Treatment-induced HDL-C elevations were examined in a meta-analysis of 108 studies including 299,310 participants, which found no associated reduction in the risk of coronary heart disease events, coronary disease mortality, or total mortality (102). The LDL-C/HDL-C ratio was not a better predictor of cardiovascular outcomes than LDL-C alone, and the authors recommended using LDL-C, rather than HDL-C or a ratio of the two, as the therapeutic target.
The evidence of the renal-specific effects of ketogenic diets is limited but worth noting, especially in the context of the unclear long-term benefits of such diets for diabetes and obesity (103). For those without chronic kidney disease (CKD), one of the biggest potential risks of the ketogenic diet is the development of kidney stones, a finding that has been frequently noted in the pediatric epilepsy literature (104, 105). The ketogenic diet's emphasis on high-fat, animal-based foods while excluding many fruits and vegetables promotes a urinary milieu for kidney stones. Dietary animal protein consumption is a well-established promoter of kidney stones (106). The acidosis caused by the ketogenic diet may also encourage stone formation by lowering urinary citrate and pH levels while increasing urinary calcium levels.
Another potential risk of animal-based ketogenic diets for those without CKD is the development of CKD through the consumption of animal fat and protein. In observational studies of populations eating Western diets, high animal fat consumption, as is common with ketogenic diets, has been associated with increased risk of developing albuminuria (107). In a prospective study of nearly 12,000 people over 23 years, high animal protein consumption was associated with a 23% increased hazard ratio of incident CKD (108). Other observational studies of animal protein have shown similar findings (109, 110).
For those with CKD, the high protein content in some ketogenic diets is of concern. While “classic” ketogenic diets are not necessarily high in protein, those used for weight loss often meet the definition of a high-protein diet (>1.5 g/kg/d) by encouraging dieters to consume 1.2–2.0 g/kg/d. Compared to control diets with higher protein content, low protein consumption has been associated with a reduction in the rate of kidney function decline in a meta-analysis of 14 randomized controlled trials (111). High protein consumption facilitates hyperfiltration, a phenomenon of increased blood flow to the glomerulus, which is thought to lead to long-term damage in those with CKD (112). Finally, the acid load from the ketogenic diet may worsen metabolic acidosis and kidney disease in those with CKD (113). The ketogenic diet's acid load comes from the foods consumed (especially those from animal-based sources), ketoacids associated with ketone production, and from the lack of natural alkali found in fruits and vegetables that are often avoided in the ketogenic diet. As such, the ketogenic diet requires further research regarding its long-term renal safety in those with and without CKD.
Approximately 40% of pregnancies in the United States are unplanned (114). Low-carbohydrate diets followed prior to conception or during the periconceptual period are associated with an increased risk of birth defects and gestational diabetes, respectively.
The National Birth Defects Prevention Study found that women who reported consuming low-carbohydrate diets in the year prior to conception (daily carbohydrate intake ≤5th percentile of control mothers, or ~95 g carbohydrate/day) were 30% more likely to have an infant with a neural tube defect (95% CI, 1.02–1.67), specifically anencephaly (OR 1.35; 95% CI, 0.90–2.02) and spina bifida (OR 1.28; 95% CI, 0.95–1.72) (115). For unplanned pregnancies in particular, effect estimates for carbohydrate-restricted diets showed an 89% increased risk of neural tube defects (95% CI, 1.28–2.79) (115).
Use of folate supplements may not mitigate the risk seen with low-carbohydrate diets. In the above study, there was no effect measure modification by folic acid supplement use (115). A 2019 study conducted using data that predated the era of folate-fortified grain products also found an increase in neural tube defects in the offspring of women consuming low-carbohydrate diets in the periconceptual period (OR 2.0; 95% CI, 1.2–3.4), suggesting other factors were contributing (116).
A prospective cohort study evaluating gestational diabetes risk scored women's diets for adherence to a low-carbohydrate diet pattern and dietary fat source. After adjusting for multiple variables including BMI, women consuming the least carbohydrate had a 27% higher risk of gestational diabetes compared to those consuming the most (RR 1.27; 95% CI, 1.06–1.51, p = 0.03). A stronger association was seen for women following a low-carbohydrate diet pattern high in animal products; they had a 36% higher risk of gestational diabetes (RR 1.36; 95% CI, 1.13–1.64, p = 0.003). A vegetable-based low-carbohydrate dietary pattern was not associated with increased risk (117).
The most restrictive ketogenic diets used for epilepsy can cause fatigue, headache, nausea, constipation, hypoglycemia, and acidosis, especially within the first few days to weeks of following the diet (2). Dehydration, hepatitis, pancreatitis, hypertriglyceridemia, hyperuricemia, hypercholesterolemia, hypomagnesemia, and hyponatremia can also occur (82, 118).
A study of 300 users of online forums found that self-administered ketogenic diets may be accompanied by a temporary cluster of symptoms frequently termed “keto flu,” which includes headache, fatigue, nausea, dizziness, “brain fog,” gastrointestinal discomfort, decreased energy, feeling faint, and heartbeat alterations (119). In endurance athletes, 3.5 weeks on a ketogenic diet led to unfavorable effects on markers of bone modeling and remodeling (120).
Longer-term effects can include decreased bone mineral density, nephrolithiasis, cardiomyopathy, anemia, and neuropathy of the optic nerve (82, 121). Ketogenic diets have low long-term tolerability, and are not sustainable for many individuals (48, 49). Diets low in carbohydrate have also been associated with an increased risk of all-cause mortality (122), although recent data suggest that lower-carbohydrate diets can be linked to either higher or lower mortality risk, depending on the quality of the carbohydrate they contain and whether they rely more on animal protein and saturated fat or plant protein and unsaturated fat, respectively (123).
Ketogenic diets reduce seizure frequency in some individuals with drug-resistant epilepsy. These diets can also reduce body weight, although not more effectively than other dietary approaches over the long term or when matched for energy intake. Ketogenic diets can also lower blood glucose, although their efficacy typically wanes within the first few months.
Very-low-carbohydrate diets are associated with marked risks. LDL-C can rise, sometimes dramatically. Pregnant women on such diets are more likely to have a child with a neural tube defect, even when supplementing folic acid. And these diets may increase chronic disease risk: Foods and dietary components that typically increase on ketogenic diets (eg, red meat, processed meat, saturated fat) are linked to an increased risk of CKD, cardiovascular disease, cancer, diabetes, and Alzheimer's disease, whereas intake of protective foods (eg, vegetables, fruits, legumes, whole grains) typically decreases. Current evidence suggests that for most individuals, the risks of such diets outweigh the benefits.
LC and NDB contributed to the organization of the manuscript, reviewed, and approved the submitted version. LC composed the outline and drafted the manuscript. LC, BD, SJ, MJ, JP, MN, and NDB wrote sections of the manuscript. All authors had full access to data and revised and approved the manuscript for publication.
This work was funded by the Physicians Committee for Responsible Medicine.
LC is an employee of the Physicians Committee for Responsible Medicine in Washington, DC, a non-profit organization providing educational, research, and medical services related to nutrition. LC also declares that a trust for her benefit previously held stock in 3M, Abbot Labs, AbbVie, Johnson and Johnson, Mondelez, Nestle, and Walgreens; she is the author of a food and nutrition blog, Veggie Quest; and she is former publications editor and current chair for the Women's Health Dietetic Practice Group within the Academy of Nutrition and Dietetics. MJ and JP received compensation from the Physicians Committee for Responsible Medicine while working on this manuscript. MN is an employee of the Physicians Committee for Responsible Medicine. NDB is an Adjunct Professor of Medicine at the George Washington University School of Medicine. He serves without compensation as president of the Physicians Committee for Responsible Medicine and Barnard Medical Center in Washington, DC, non-profit organizations providing educational, research, and medical services related to nutrition. He writes books and articles and gives lectures related to nutrition and health and has received royalties and honoraria from these sources.
The remaining authors declare that the research was conducted in the absence of any commercial or financial relationships that could be construed as a potential conflict of interest.
1. Newman JC, Verdin E. β-Hydroxybutyrate: a signaling metabolite. Annu Rev Nutr. (2017) 37:51–76. doi: 10.1146/annurev-nutr-071816-064916
2. Roehl K, Sewak SL. Practice paper of the academy of nutrition and dietetics: classic and modified ketogenic diets for treatment of epilepsy. J Acad Nutr Diet. (2017) 117:1279–92. doi: 10.1016/j.jand.2017.06.006
3. Bueno NB, de Melo IS, de Oliveira SL, da Rocha Ataide T. Very-low-carbohydrate ketogenic diet v. low-fat diet for long-term weight loss: a meta-analysis of randomised controlled trials. Br J Nutr. (2013) 110:1178–87. doi: 10.1017/S0007114513000548
4. Kirkpatrick CF, Bolick JP, Kris-Etherton PM, Sikand G, Aspry KE, Soffer DE, et al. Review of current evidence and clinical recommendations on the effects of low-carbohydrate and very-low-carbohydrate (including ketogenic) diets for the management of body weight and other cardiometabolic risk factors: a scientific statement from the National Lipid Association Nutrition and Lifestyle Task Force. J Clin Lipidol. (2019) 13:689–711.e1. doi: 10.1016/j.jacl.2019.08.003
5. Institute of Medicine. Dietary Reference Intakes: The Essential Guide to Nutrient Requirements. Washington, DC: The National Academies Press (2006).
6. Liu RH. Health-promoting components of fruits and vegetables in the diet. Adv Nutr. (2013) 4:384s−92s. doi: 10.3945/an.112.003517
7. Slavin JL, Lloyd B. Health benefits of fruits and vegetables. Adv Nutr. (2012) 3:506–16. doi: 10.3945/an.112.002154
8. Patterson MA, Maiya M, Stewart ML. Resistant starch content in foods commonly consumed in the United States: a narrative review. J Acad Nutr Diet. (2020) 120:230–44. doi: 10.1016/j.jand.2019.10.019
9. Freedman MR, King J, Kennedy E. Popular diets: a scientific review. Obes Res. (2001) 9(Suppl. 1):1s−40s. doi: 10.1038/oby.2001.113
10. Bilsborough SA, Crowe TC. Low-carbohydrate diets: what are the potential short- and long-term health implications? Asia Pac J Clin Nutr. (2003) 12:396–404.
11. Zupec-Kania BA, Spellman E. An overview of the ketogenic diet for pediatric epilepsy. Nutr Clin Pract. (2008) 23:589–96. doi: 10.1177/0884533608326138
12. Holscher HD. Dietary fiber and prebiotics and the gastrointestinal microbiota. Gut Microbes. (2017) 8:172–84. doi: 10.1080/19490976.2017.1290756
13. Paoli A, Mancin L, Bianco A, Thomas E, Mota JF, Piccini F. Ketogenic diet and microbiota: friends or enemies? Genes. (2019) 10:534. doi: 10.3390/genes10070534
14. Jeffery IB, O'Toole PW. Diet-microbiota interactions and their implications for healthy living. Nutrients. (2013) 5:234–52. doi: 10.3390/nu5010234
15. Daïen CI, Pinget VP, Tan JK, Macia L. Detrimental impact of microbiota-accessible carbohydrate-deprived diet on gut and immune homeostasis: an overview. Front Immunol. (2017) 8:548. doi: 10.3389/fimmu.2017.00548
16. Lindefeldt M, Eng A, Darban H, Bjerkner A, Zetterström CK, Allander T, et al. The ketogenic diet influences taxonomic and functional composition of the gut microbiota in children with severe epilepsy. NPJ Biofilms Microbiomes. (2019) 5:5. doi: 10.1038/s41522-018-0073-2
17. Fiest KM, Sauro KM, Wiebe S, Patten SB, Kwon CS, Dykeman J, et al. Prevalence and incidence of epilepsy: a systematic review and meta-analysis of international studies. Neurology. (2017) 88:296–303. doi: 10.1212/WNL.0000000000003509
18. Martin-McGill KJ, Jackson CF, Bresnahan R, Levy RG, Cooper PN. Ketogenic diets for drug-resistant epilepsy. Cochrane Database Syst Rev. (2018) 11:CD001903. doi: 10.1002/14651858.CD001903.pub4
19. D'Andrea Meira I, Romão TT, Pires do Prado HJ, Krüger LT, Pires MEP, da Conceição PO. Ketogenic diet and epilepsy: what we know so far. Front Neurosci. (2019) 13:5. doi: 10.3389/fnins.2019.00005
20. Dashti HM, Mathew TC, Hussein T, Asfar SK, Behbahani A, Khoursheed MA, et al. Long-term effects of a ketogenic diet in obese patients. Exp Clin Cardiol. (2004) 9:200–5.
21. Westman EC, Yancy WS, Mavropoulos JC, Marquart M, McDuffie JR. The effect of a low-carbohydrate, ketogenic diet versus a low-glycemic index diet on glycemic control in type 2 diabetes mellitus. Nutr Metab. (2008) 5:36. doi: 10.1186/1743-7075-5-36
22. Foster GD, Wyatt HR, Hill JO, McGuckin BG, Brill C, Mohammed BS, et al. A randomized trial of a low-carbohydrate diet for obesity. N Engl J Med. (2003) 348:2082–90. doi: 10.1056/NEJMoa022207
23. Dashti HM, Al-Zaid NS, Mathew TC, Al-Mousawi M, Talib H, Asfar SK, et al. Long term effects of ketogenic diet in obese subjects with high cholesterol level. Mol Cell Biochem. (2006) 286:1–9. doi: 10.1007/s11010-005-9001-x
24. Chawla S, Tessarolo Silva F, Amaral Medeiros S, Mekary RA, Radenkovic D. The effect of low-fat and low-carbohydrate diets on weight loss and lipid levels: a systematic review and meta-analysis. Nutrients. (2020) 12:3774. doi: 10.3390/nu12123774
25. Westman EC, Feinman RD, Mavropoulos JC, Vernon MC, Volek JS, Wortman JA, et al. Low-carbohydrate nutrition and metabolism. Am J Clin Nutr. (2007) 86:276–84. doi: 10.1093/ajcn/86.2.276
26. Gibson AA, Seimon RV, Lee CM, Ayre J, Franklin J, Markovic TP, et al. Do ketogenic diets really suppress appetite? A systematic review and meta-analysis. Obes Rev. (2015) 16:64–76. doi: 10.1111/obr.12230
27. Hall KD, Guo J, Courville AB, Boring J, Brychta R, Chen KY, et al. Effect of a plant-based, low-fat diet versus an animal-based, ketogenic diet on ad libitum energy intake. Nat Med. (2021) 27:344–53. doi: 10.1038/s41591-020-01209-1
28. Hall KD, Chen KY, Guo J, Lam YY, Leibel RL, Mayer LE, et al. Energy expenditure and body composition changes after an isocaloric ketogenic diet in overweight and obese men. Am J Clin Nutr. (2016) 104:324–33. doi: 10.3945/ajcn.116.133561
29. Academy of Nutrition and Dietetics Evidence Analysis Library. In Adults, How Effective, in Terms of Weight Loss and Maintenance, are Low Carbohydrate Diets (Defined as <35% kcals From Carbohydrate)? (2006). Available online at: https://www.andeal.org/topic.cfm?cat=2891&evidence_summary_id=250138 (accessed January 29, 2021).
30. Gardner CD, Kiazand A, Alhassan S, Kim S, Stafford RS, Balise RR, et al. Comparison of the Atkins, Zone, Ornish, and LEARN diets for change in weight and related risk factors among overweight premenopausal women: the A TO Z Weight Loss Study: a randomized trial. JAMA. (2007) 297:969–77. doi: 10.1001/jama.297.9.969
31. Dansinger ML, Gleason JA, Griffith JL, Selker HP, Schaefer EJ. Comparison of the Atkins, Ornish, Weight Watchers, and Zone diets for weight loss and heart disease risk reduction: a randomized trial. JAMA. (2005) 293:43–53. doi: 10.1001/jama.293.1.43
32. Meng Y, Bai H, Wang S, Li Z, Wang Q, Chen L. Efficacy of low carbohydrate diet for type 2 diabetes mellitus management: a systematic review and meta-analysis of randomized controlled trials. Diabetes Res Clin Pract. (2017) 131:124–31. doi: 10.1016/j.diabres.2017.07.006
33. McClean AM, Montorio L, McLaughlin D, McGovern S, Flanagan N. Can a ketogenic diet be safely used to improve glycaemic control in a child with type 1 diabetes? Arch Dis Child. (2019) 104:501–4. doi: 10.1136/archdischild-2018-314973
34. Leow ZZX, Guelfi KJ, Davis EA, Jones TW, Fournier PA. The glycaemic benefits of a very-low-carbohydrate ketogenic diet in adults with type 1 diabetes mellitus may be opposed by increased hypoglycaemia risk and dyslipidaemia. Diabet Med. (2018) 35:1258–63. doi: 10.1111/dme.13663
35. Kanikarla-Marie P, Jain SK. Hyperketonemia and ketosis increase the risk of complications in type 1 diabetes. Free Radic Biol Med. (2016) 95:268–77. doi: 10.1016/j.freeradbiomed.2016.03.020
36. Yancy WS, Jr., Foy M, Chalecki AM, Vernon MC, Westman EC. A low-carbohydrate, ketogenic diet to treat type 2 diabetes. Nutr Metab. (2005) 2:34. doi: 10.1186/1743-7075-2-34
37. Dashti HM, Mathew TC, Khadada M, Al-Mousawi M, Talib H, Asfar SK, et al. Beneficial effects of ketogenic diet in obese diabetic subjects. Mol Cell Biochem. (2007) 302:249–56. doi: 10.1007/s11010-007-9448-z
38. Hussain TA, Mathew TC, Dashti AA, Asfar S, Al-Zaid N, Dashti HM. Effect of low-calorie versus low-carbohydrate ketogenic diet in type 2 diabetes. Nutrition. (2012) 28:1016–21. doi: 10.1016/j.nut.2012.01.016
39. Goday A, Bellido D, Sajoux I, Crujeiras AB, Burguera B, García-Luna PP, et al. Short-term safety, tolerability and efficacy of a very low-calorie-ketogenic diet interventional weight loss program versus hypocaloric diet in patients with type 2 diabetes mellitus. Nutr Diabetes. (2016) 6:e230. doi: 10.1038/nutd.2016.36
40. Colica C, Merra G, Gasbarrini A, De Lorenzo A, Cioccoloni G, Gualtieri P, et al. Efficacy and safety of very-low-calorie ketogenic diet: a double blind randomized crossover study. Eur Rev Med Pharmacol Sci. (2017) 21:2274–89.
41. Saslow LR, Mason AE, Kim S, Goldman V, Ploutz-Snyder R, Bayandorian H, et al. An online intervention comparing a very low-carbohydrate ketogenic diet and lifestyle recommendations versus a plate method diet in overweight individuals with type 2 diabetes: a randomized controlled trial. J Med Internet Res. (2017) 19:e36. doi: 10.2196/jmir.5806
42. McKenzie AL, Hallberg SJ, Creighton BC, Volk BM, Link TM, Abner MK, et al. A novel intervention including individualized nutritional recommendations reduces hemoglobin A1c level, medication use, and weight in type 2 diabetes. JMIR Diabetes. (2017) 2:e5. doi: 10.2196/diabetes.6981
43. Vilar-Gomez E, Athinarayanan SJ, Adams RN, Hallberg SJ, Bhanpuri NH, McKenzie AL, et al. Post hoc analyses of surrogate markers of non-alcoholic fatty liver disease (NAFLD) and liver fibrosis in patients with type 2 diabetes in a digitally supported continuous care intervention: an open-label, non-randomised controlled study. BMJ Open. (2019) 9:e023597. doi: 10.1136/bmjopen-2018-023597
44. Murphy EA, Jenkins TJ. A ketogenic diet for reducing obesity and maintaining capacity for physical activity: hype or hope? Curr Opin Clin Nutr Metab Care. (2019) 22:314–9. doi: 10.1097/MCO.0000000000000572
45. Rosenbaum M, Hall KD, Guo J, Ravussin E, Mayer LS, Reitman ML, et al. Glucose and lipid homeostasis and inflammation in humans following an isocaloric ketogenic diet. Obesity. (2019) 27:971–81. doi: 10.1002/oby.22468
46. Bisschop PH, de Metz J, Ackermans MT, Endert E, Pijl H, Kuipers F, et al. Dietary fat content alters insulin-mediated glucose metabolism in healthy men. Am J Clin Nutr. (2001) 73:554–9. doi: 10.1093/ajcn/73.3.554
47. Goldenberg JZ, Day A, Brinkworth GD, Sato J, Yamada S, Jönsson T, et al. Efficacy and safety of low and very low carbohydrate diets for type 2 diabetes remission: systematic review and meta-analysis of published and unpublished randomized trial data. BMJ. (2021) 372:m4743. doi: 10.1136/bmj.m4743
48. Kosinski C, Jornayvaz FR. Effects of ketogenic diets on cardiovascular risk factors: evidence from animal and human studies. Nutrients. (2017) 9:E517. doi: 10.3390/nu9050517
49. Brouns F. Overweight and diabetes prevention: is a low-carbohydrate-high-fat diet recommendable? Eur J Nutr. (2018) 57:1301–12. doi: 10.1007/s00394-018-1636-y
50. Halton TL, Liu S, Manson JE, Hu FB. Low-carbohydrate-diet score and risk of type 2 diabetes in women. Am J Clin Nutr. (2008) 87:339–46. doi: 10.1093/ajcn/87.2.339
51. de Koning L, Fung TT, Liao X, Chiuve SE, Rimm EB, Willett WC, et al. Low-carbohydrate diet scores and risk of type 2 diabetes in men. Am J Clin Nutr. (2011) 93:844–50. doi: 10.3945/ajcn.110.004333
52. Qian F, Liu G, Hu FB, Bhupathiraju SN, Sun Q. Association between plant-based dietary patterns and risk of type 2 diabetes: a systematic review and meta-analysis. JAMA Intern Med. (2019) 179:1335–44. doi: 10.1001/jamainternmed.2019.2195
53. Okada E, Takahashi K, Nakamura K, Ukawa S, Takabayashi S, Nakamura M, et al. Dietary patterns and abnormal glucose tolerance among Japanese: findings from the National Health and Nutrition Survey, 2012. Public Health Nutr. (2019) 22:2460–8. doi: 10.1017/S1368980019000120
54. Bellou V, Belbasis L, Tzoulaki I, Evangelou E. Risk factors for type 2 diabetes mellitus: an exposure-wide umbrella review of meta- analyses. PLoS ONE. (2018) 13:e0194127. doi: 10.1371/journal.pone.0194127
55. Shu L, Shen XM, Li C, Zhang XY, Zheng PF. Dietary patterns are associated with type 2 diabetes mellitus among middle-aged adults in Zhejiang Province, China. Nutr J. (2017) 16:81. doi: 10.1186/s12937-017-0303-0
56. Jannasch F, Kröger J, Schulze MB. Dietary patterns and type 2 diabetes: a systematic literature review and meta-analysis of prospective studies. J Nutr. (2017) 147:1174–82. doi: 10.3945/jn.116.242552
57. Perdomo CM, Frühbeck G, Escalada J. Impact of nutritional changes on nonalcoholic fatty liver disease. Nutrients. (2019) 11:677. doi: 10.3390/nu11030677
58. Schugar RC, Crawford P. Low-carbohydrate ketogenic diets, glucose homeostasis, and nonalcoholic fatty liver disease. Curr Opin Clin Nutr Metab Care. (2012) 15:374–80. doi: 10.1097/MCO.0b013e3283547157
59. Ore A, Akinloye OA. Oxidative stress and antioxidant biomarkers in clinical and experimental models of non-alcoholic fatty liver disease. Medicina. (2019) 55:26. doi: 10.3390/medicina55020026
60. Romero-Gómez M, Zelber-Sagi S, Trenell M. Treatment of NAFLD with diet, physical activity and exercise. J Hepatol. (2017) 67:829–46. doi: 10.1016/j.jhep.2017.05.016
61. Younossi ZM, Koenig AB, Abdelatif D, Fazel Y, Henry L, Wymer M. Global epidemiology of nonalcoholic fatty liver disease-Meta-analytic assessment of prevalence, incidence, and outcomes. Hepatology. (2016) 64:73–84. doi: 10.1002/hep.28431
62. Eslamparast T, Tandom P, Raman M. Dietary composition independent of weight loss in the management of non-alcoholic fatty liver disease. Nutrients. (2017) 9:800. doi: 10.3390/nu9080800
63. Marchesini G, Petta S, Grave RD. Diet, weight loss, and liver health in nonalcoholic fatty liver disease: pathophysiology, evidence, and practice. Hepatology. (2016) 63:2032–43. doi: 10.1002/hep.28392
64. De Luis DA, Aller R, Izaola O, Gonzalez Sagrado M, Conde R. Effect of two different hypocaloric diets in transaminases and insulin resistance in nonalcoholic fatty liver disease and obese patients. Nutr Hosp. (2010) 25:730–5.
65. Kirk E, Reeds DN, Fink BN, Mayurranjan SM, Patterson BW, Klein S. Dietary fat and carbohydrates differentially alter insulin sensitivity during caloric restriction. Gastroenterology. (2009) 136:1552–60. doi: 10.1053/j.gastro.2009.01.048
66. Haufe S, Engeli S, Kast P, Böhnke J, Utz W, Haas V, et al. Randomized comparison of reduced fat and reduced carbohydrate hypocaloric diets on intrahepatic fat in overweight and obese human subjects. Hepatology. (2011) 53:1504–14. doi: 10.1002/hep.24242
67. Westerbacka J, Lammi K, Häkkinen AM, Rissanen A, Salminen I, Aro A, et al. Dietary fat content modifies liver fat in overweight nondiabetic subjects. J Clin Endocrinol Metab. (2005) 90:2804–9. doi: 10.1210/jc.2004-1983
68. Alferink LJ, Kiefte-de Jong JC, Erler NS, Veldt BJ, Schoufour JD, de Knegt RJ, et al. Association of dietary macronutrient composition and non-alcoholic fatty liver disease in an ageing population: the Rotterdam Study. Gut. (2019) 68:1088–98. doi: 10.1136/gutjnl-2017-315940
69. Chiu TH, Lin MN, Pan WH, Chen YC, Lin CL. Vegetarian diet, food substitution, and nonalcoholic fatty liver. Ci Ji Yi Xue Za Zhi. (2018) 30:102–9. doi: 10.4103/tcmj.tcmj_109_17
70. Huebner J, Marienfeld S, Abbenhardt C, Ulrich C, Muenstedt K, Micke O, et al. Counseling patients on cancer diets: a review of the literature and recommendations for clinical practice. Anticancer Res. (2014) 34:39–48.
71. Liberti MV, Locasale JW. The Warburg effect: how does it benefit cancer cells? Trends Biochem Sci. (2016) 41:211–8. doi: 10.1016/j.tibs.2015.12.001
72. Martin-McGill KJ, Srikandarajah N, Marson AG, Tudur Smith C, Jenkinson MD. The role of ketogenic diets in the therapeutic management of adult and paediatric gliomas: a systematic review. CNS Oncol. (2018) 7:CNS17. doi: 10.2217/cns-2017-0030
73. Klement RJ, Brehm N, Sweeney RA. Ketogenic diets in medical oncology: a systematic review with focus on clinical outcomes. Med Oncol. (2020) 37:14. doi: 10.1007/s12032-020-1337-2
74. Martinez-Outschoorn UE, Prisco M, Ertel A, Tsirigos A, Lin Z, Pavlides S, et al. Ketones and lactate increase cancer cell “stemness,” driving recurrence, metastasis and poor clinical outcome in breast cancer: achieving personalized medicine via Metabolo-Genomics. Cell Cycle. (2011) 10:1271–86. doi: 10.4161/cc.10.8.15330
75. Bouvard V, Loomis D, Guyton KZ, Grosse Y, Ghissassi FE, Benbrahim-Tallaa L, et al. Carcinogenicity of consumption of red and processed meat. Lancet Oncol. (2015) 16:1599–600. doi: 10.1016/S1470-2045(15)00444-1
76. Farvid MS, Cho E, Chen WY, Eliassen AH, Willett WC. Dietary protein sources in early adulthood and breast cancer incidence: prospective cohort study. BMJ. (2014) 348:g3437. doi: 10.1136/bmj.g3437
77. Farvid MS, Cho E, Chen WY, Eliassen AH, Willett WC. Adolescent meat intake and breast cancer risk. Int J Cancer. (2015) 136:1909–20. doi: 10.1002/ijc.29218
78. Aune D, Giovannucci E, Boffetta P, Fadnes LT, Keum N, Norat T, et al. Fruit and vegetable intake and the risk of cardiovascular disease, total cancer and all-cause mortality-a systematic review and dose-response meta-analysis of prospective studies. Int J Epidemiol. (2017) 46:1029–56. doi: 10.1093/ije/dyw319
79. Zhang B, Zhao Q, Guo W, Bao W, Wang X. Association of whole grain intake with all-cause, cardiovascular, and cancer mortality: a systematic review and dose-response meta-analysis from prospective cohort studies. Eur J Clin Nutr. (2018) 72:57–65. doi: 10.1038/ejcn.2017.149
80. Hebert LE, Weuve J, Scherr PA, Evans DA. Alzheimer disease in the United States (2010-2050) estimated using the 2010 census. Neurology. (2013) 80:1778–83. doi: 10.1212/WNL.0b013e31828726f5
81. Broom GM, Shaw IC, Rucklidge JJ. The ketogenic diet as a potential treatment and prevention strategy for Alzheimer's disease. Nutrition. (2019) 60:118–21. doi: 10.1016/j.nut.2018.10.003
82. Włodarek D. Role of ketogenic diets in neurodegenerative diseases (Alzheimer's disease and Parkinson's disease). Nutrients. (2019) 11.E169. doi: 10.3390/nu11010169
83. Taylor MK, Sullivan DK, Mahnken JD, Burns JM, Swerdlow RH. Feasibility and efficacy data from a ketogenic diet intervention in Alzheimer's disease. Alzheimers Dement (NY). (2017) 4:28–36. doi: 10.1016/j.trci.2017.11.002
84. Krikorian R, Shidler MD, Dangelo K, Couch SC, Benoit SC, Clegg DJ. Dietary ketosis enhances memory in mild cognitive impairment. Neurobiol Aging. (2012) 33:425.e19-27. doi: 10.1016/j.neurobiolaging.2010.10.006
85. Lilamand M, Porte B, Cognat E, Hugon J, Mouton-Liger F, Paquet C. Are ketogenic diets promising for Alzheimer's disease? A translational review. Alzheimers Res Ther. (2020) 12:42. doi: 10.1186/s13195-020-00615-4
86. Morris MC, Evans DA, Bienias JL, Tangney CC, Bennett DA, Aggarwal N, et al. Dietary fats and the risk of incident Alzheimer disease. Arch Neurol. (2003) 60:194–200. doi: 10.1001/archneur.60.2.194
87. Grant WB. Using multicountry ecological and observational studies to determine dietary risk factors for Alzheimer's disease. J Am Coll Nutr. (2016) 35:476–89. doi: 10.1080/07315724.2016.1161566
88. Lefèvre-Arbogast S, Gaudout D, Bensalem J, Letenneur L, Dartigues JF, Hejblum BP, et al. Pattern of polyphenol intake and the long-term risk of dementia in older persons. Neurology. (2018) 90:e1979–88. doi: 10.1212/WNL.0000000000005607
89. Morris MC, Tangney CC, Wang Y, Sacks FM, Bennett DA, Aggarwal NT. MIND diet associated with reduced incidence of Alzheimer's disease. Alzheimers Dement. (2015) 11:1007–114. doi: 10.1016/j.jalz.2014.11.009
90. Dattilo AM, Kris-Etherton PM. Effects of weight reduction on blood lipids and lipoproteins: a meta-analysis. Am J Clin Nutr. (1992) 56:320–8. doi: 10.1093/ajcn/56.2.320
91. Westman EC. Effect of 6-month adherence to a very low carbohydrate diet program. Am J Med. (2002) 113:30–6. doi: 10.1016/S0002-9343(02)01129-4
92. Yancy WS. A low-carbohydrate, ketogenic diet versus a low-fat diet to treat obesity and hyperlipidemia: a randomized, controlled trial. Ann Int Med. (2004) 140:769–77. doi: 10.7326/0003-4819-140-10-200405180-00006
93. Stern L. The effects of low-carbohydrate versus conventional weight loss diets in severely obese adults: one-year follow-up of a randomized trial. Ann Int Med. (2004) 140:778–85. doi: 10.7326/0003-4819-140-10-200405180-00007
94. Hallberg SJ, McKenzie AL, Williams PT, Bhanpuri NH, Peters AL, Campbell WW, et al. Effectiveness and safety of a novel care model for the management of type 2 diabetes at 1 year: an open-label, non-randomized, controlled study. Diabetes Ther. (2018) 9:583–612. doi: 10.1007/s13300-018-0373-9
95. Athinarayanan SJ, Adams RN, Hallberg SJ, McKenzie AL, Bhanpuri NH, Campbell WW, et al. Long-term effects of a novel continuous remote care intervention including nutritional ketosis for the management of type 2 diabetes: a 2-year non-randomized clinical trial. Front Endocrinol (Lausanne). (2019) 10:348. doi: 10.3389/fendo.2019.00348
96. Yuan X, Wang J, Yang S, Gao M, Cao L, Li X, et al. Effect of the ketogenic diet on glycemic control, insulin resistance, and lipid metabolism in patients with T2DM: a systematic review and meta-analysis. Nutr Diabetes. (2020) 10:38. doi: 10.1038/s41387-020-00142-z
97. Kephart WC, Pledge CD, Roberson PA, Mumford PW, Romero MA, Mobley CB, et al. The three-month effects of a ketogenic diet on body composition, blood parameters, and performance metrics in CrossFit Trainees: a pilot study. Sports. (2018) 6:E1. doi: 10.3390/sports6010001
98. Robinson JG. What is the role of advanced lipoprotein analysis in practice? J Am Coll Cardiol. (2012) 60:2607–15. doi: 10.1016/j.jacc.2012.04.067
99. AACC Lipoproteins and Vascular Diseases Division Working Group on Best Practices, Cole TG, Contois JH, Csako G, McConnell JP, Remaley AT, et al. Association of apolipoprotein B and nuclear magnetic resonance spectroscopy-derived LDL particle number with outcomes in 25 clinical studies: assessment by the AACC Lipoprotein and Vascular Diseases Division Working Group on Best Practices. Clin Chem. (2013) 59:752–70. doi: 10.1373/clinchem.2012.196733
100. Mora S, Otvos JD, Rifai N, Rosenson RS, Buring JE, Ridker PM. Lipoprotein particle profiles by nuclear magnetic resonance compared with standard lipids and apolipoproteins in predicting incident cardiovascular disease in women. Circulation. (2009) 119:931–9. doi: 10.1161/CIRCULATIONAHA.108.816181
101. Voight BF, Peloso GM, Orho-Melander M, Frikke-Schmidt R, Barbalic M, Jensen MK, et al. Plasma HDL cholesterol and risk of myocardial infarction: a mendelian randomisation study. Lancet. (2012) 380:572–80. doi: 10.1016/S0140-6736(12)60312-2
102. Briel M, Ferreira-Gonzalez I, You JJ, Karanicolas PJ, Akl EA, Wu P, et al. Association between change in high density lipoprotein cholesterol and cardiovascular disease morbidity and mortality: systematic review and meta-regression analysis. BMJ. (2009) 338:b92 doi: 10.1136/bmj.b92
103. Joshi S, Ostfeld RJ, McMacken M. The ketogenic diet for obesity and diabetes-enthusiasm outpaces evidence. JAMA Intern Med. (2019) 179:1163–4. doi: 10.1001/jamainternmed.2019.2633
104. Furth SL, Casey JC, Pyzik PL, Neu AM, Docimo SG, Vining EP, et al. Risk factors for urolithiasis in children on the ketogenic diet. Pediatr Nephrol. (2000) 15:125–8. doi: 10.1007/s004670000443
105. McNally MA, Pyzik PL, Rubenstein JE, Hamdy RF, Kossoff EH. Empiric use of potassium citrate reduces kidney-stone incidence with the ketogenic diet. Pediatrics. (2009) 124:e300–4. doi: 10.1542/peds.2009-0217
106. Tracy CR, Best S, Bagrodia A, Poindexter JR, Adams-Huet B, Sakhaee K, et al. Animal protein and the risk of kidney stones: a comparative metabolic study of animal protein sources. J Urology. (2014) 192:137–41. doi: 10.1016/j.juro.2014.01.093
107. Lin J, Hu FB, Curhan GC. Associations of diet with albuminuria and kidney function decline. Clin J Am Soc Nephrol. (2010) 5:836–43. doi: 10.2215/CJN.08001109
108. Haring B, Selvin E, Liang M, Coresh J, Grams ME, Petruski-Ivleva N, et al. Dietary protein sources and risk for incident chronic kidney disease: results from the Atherosclerosis Risk in Communities (ARIC) Study. J Ren Nutr. (2017) 27:233–42. doi: 10.1053/j.jrn.2016.11.004
109. Lew QJ, Jafar TH, Koh HW, Jin A, Chow KY, Yuan JM, et al. Red meat intake and risk of ESRD. J Am Soc Nephrol. (2017) 28:304–12. doi: 10.1681/ASN.2016030248
110. Mirmiran P, Yuzbashian E, Aghayan M, Mahdavi M, Asghari G, Azizi F. A prospective study of dietary meat intake and risk of incident chronic kidney disease. J Ren Nutr. (2020) 30:111–8. doi: 10.1053/j.jrn.2019.06.008
111. Yan B, Su X, Xu B, Qiao X, Wang L. Effect of diet protein restriction on progression of chronic kidney disease: a systematic review and meta-analysis. PLoS ONE. (2018) 13:e0206134. doi: 10.1371/journal.pone.0206134
112. Kalantar-Zadeh K, Fouque D. Nutritional management of chronic kidney disease. N Engl J Med. (2017) 377:1765–76. doi: 10.1056/NEJMra1700312
113. Banerjee T, Crews DC, Wesson DE, Tilea AM, Saran R, Ríos-Burrows N, et al. High dietary acid load predicts ESRD among adults with CKD. J Am Soc Nephrol. (2015) 26:1693–700. doi: 10.1681/ASN.2014040332
114. Ahrens KA, Thoma ME, Copen CE, Frederiksen BN, Decker EJ, Moskosky S. Unintended pregnancy and interpregnancy interval by maternal age, National Survey of Family Growth. Contraception. (2018) 98:52–5. doi: 10.1016/j.contraception.2018.02.013
115. Desrosiers TA, Siega-Riz AM, Mosley BS, Meyer RE. Low carbohydrate diets may increase risk of neural tube defects. Birth Defects Res. (2018) 110:901–9. doi: 10.1002/bdr2.1198
116. Shaw GM, Yang W. Women's periconceptional lowered carbohydrate intake and NTD-affected pregnancy risk in the era of prefortification with folic acid. Birth Defects Res. (2019) 111:248–53. doi: 10.1002/bdr2.1466
117. Bao W, Bowers K, Tobias DK, Olsen SF, Chavarro J, Vaag A, et al. Prepregnancy low-carbohydrate dietary pattern and risk of gestational diabetes mellitus: a prospective cohort study. Am J Clin Nutr. (2014) 99:1378–84. doi: 10.3945/ajcn.113.082966
118. Kang HC, Chung DE, Kim DW, Kim HD. Early- and late-onset complications of the ketogenic diet for intractable epilepsy. Epilepsia. (2004) 45:1116–23. doi: 10.1111/j.0013-9580.2004.10004.x
119. Bostock ECS, Kirkby KC, Taylor BV, Hawrelak JA. Consumer reports of “keto flu” associated with the ketogenic diet. Front Nutr. (2020) 7:20. doi: 10.3389/fnut.2020.575713
120. Heikura IA, Burke LM, Hawley JA, Ross ML, Garvican-Lewis L, Sharma AP, et al. A short-term ketogenic diet impairs markers of bone health in response to exercise. Front Endocrinol (Lausanne). (2020) 10:880. doi: 10.3389/fendo.2019.00880
121. Hoyt CS, Billson FA. Optic neuropathy in ketogenic diet. Br J Ophthalmol. (1979) 63:191–4. doi: 10.1136/bjo.63.3.191
122. Noto H, Goto A, Tsujimoto T, Noda M. Low-carbohydrate diets and all-cause mortality: a systematic review and meta-analysis of observational studies. PLoS ONE. (2013) 8:e55030. doi: 10.1371/journal.pone.0055030
Keywords: ketogenic diet, very-low-carbohydrate diet, low-carbohydrate diet, obesity, disease prevention
Citation: Crosby L, Davis B, Joshi S, Jardine M, Paul J, Neola M and Barnard ND (2021) Ketogenic Diets and Chronic Disease: Weighing the Benefits Against the Risks. Front. Nutr. 8:702802. doi: 10.3389/fnut.2021.702802
Received: 29 April 2021; Accepted: 10 June 2021;
Published: 16 July 2021.
Edited by:
Jasminka Z. Ilich, Florida State University, United StatesReviewed by:
Maria Montserrat Diaz Pedrosa, State University of Maringá, BrazilCopyright © 2021 Crosby, Davis, Joshi, Jardine, Paul, Neola and Barnard. This is an open-access article distributed under the terms of the Creative Commons Attribution License (CC BY). The use, distribution or reproduction in other forums is permitted, provided the original author(s) and the copyright owner(s) are credited and that the original publication in this journal is cited, in accordance with accepted academic practice. No use, distribution or reproduction is permitted which does not comply with these terms.
*Correspondence: Lee Crosby, LCrosby@pcrm.org
Disclaimer: All claims expressed in this article are solely those of the authors and do not necessarily represent those of their affiliated organizations, or those of the publisher, the editors and the reviewers. Any product that may be evaluated in this article or claim that may be made by its manufacturer is not guaranteed or endorsed by the publisher.
Research integrity at Frontiers
Learn more about the work of our research integrity team to safeguard the quality of each article we publish.