- 1College of Engineering, Shenyang Agricultural University, Shenyang, China
- 2Key Laboratory of Development and Application of Rural Renewable Energy, Biogas Institute of Ministry of Agriculture and Rural Affairs, Chengdu, China
- 3Institute of Development Studies, Southwestern University of Finance and Economics, Chengdu, China
- 4College of Chemistry, Sichuan University, Chengdu, China
Biogas slurry, a byproduct of biogas plants, is considered a high-quality bio-organic fertilizer. Despite providing nutrients to crops, biogas slurry may contain a high concentration of heavy metals, leading to food safety problems and endangering human health if such metals are absorbed by plants. Therefore, biogas slurry should undergo systematic risk assessment prior to direct use on farmland to ensure its safety for soils and crops. In this study, the risk of applying biogas slurry in peanut cultivation was comprehensively evaluated. Based on nitrogen contents, different concentrations of biogas slurry were applied in peanut cultivation. The results achieved herein showed that the application of biogas slurry as a nutrient supplier in peanut cultivation would significantly affect the physical and chemical properties of soil and characteristics of the plant and the quality of peanuts. Although the heavy metal content of biogas slurry was within the permitted range, it had potential risks to human health and the environment. Principal component analysis (PCA) showed that biogas slurry was the primary source of heavy metals in soil. After the application of biogas slurry, the contents of As and Hg in the soil increased significantly, which were 11.12 and 26.67 times higher than those in the control soil. The contents of Cu, Zn, Pb, Cd, and As in peanut kernel samples under different levels of biogas slurry application were all lower than the maximum permissible limit set by the Standardization Administration of China. In contrast, the content of Hg in peanut kernels was higher than the maximum permissible limit value of 0.02 mg/kg. Peanut had a higher enrichment capacity of Cd and Zn and a higher migration capacity of Pb. The health risk assessment showed that the long-term consumption of peanuts grown with a high dosage of biogas slurry would be harmful to the health of children aged 2–6 years with a large consumption level.
Introduction
The dry matter and organic dry matter produced daily by livestock worldwide can exceed 20 and 10 million tons, respectively (1); thus, livestock and poultry wastes are the main sources of agricultural non-point-source pollution around the world (2). With a sharp increase in intensification of livestock and poultry farming, China has set strict regulations on both size and location of pig farms to reduce the associated environmental pollution and achieve global sustainable development. At present, the treatment of livestock and poultry manure includes mainly physical methods [sedimentation (3), filtration (4), dry and wet separation (5), and high-temperature drying (6)], chemical methods [precipitation (7), flocculation (8), and advanced oxidation processes (9)], and biochemical methods [aerobic (10) and anaerobic fermentation (11)]. Among these methods, anaerobic fermentation has been receiving large attention for being simple, inexpensive, and environment friendly (12).
The anaerobic fermentation process leads to the formation of a huge amount of biogas slurry that is rich in inorganic elements, such as nitrogen, phosphorus, potassium, and trace elements. The organic components of biogas slurry, such as amino acids, vitamins, and beneficial bacteria, make it suitable as a soil amendment (13). However, a large number of studies have shown that the long-term application of untreated biogas slurry to farmland would bring about crop failure, with weak growth and poor quality. Moreover, the accumulation of heavy metals and antibiotic resistance genes in soil and crops may be transferred to the human body via the food chain, affecting human health and potentially damaging organ functions (14). The overdose of trace elements (such as copper, zinc, and lead), veterinary antibiotics, and other feed additives has contributed to such phenomena (15). Therefore, it is of high importance to assess the risks of biogas slurry application and control the range of application rates (16).
Peanut (Arachis hypogaea L.) is one of the most important oil crops in the world, grown in more than 100 countries, especially in developing countries; it ranks as the second oil crop next to rapeseed (17). Currently, the global peanut cultivation area is estimated at 22.67 million ha with an annual production of 35 million tons (18). As it is rich in proteins, lipids, carbohydrates, minerals, phospholipids, vitamins, and other bioactive ingredients, peanuts play an important role in daily life (19). They are recognized as one of the healthy foods for their comprehensive and relatively balanced nutritional components (20).
As an underground fruiting crop, the root system of peanuts has a strong absorption capacity, resulting in a higher concentration of heavy metals in both shoots and fruits compared with other crops (21). In addition, the kernels can also absorb large amounts of trace elements. Due to soil pollution in planting areas (e.g., due to sewage irrigation, improper fertilization, and excessive pesticides application), the occurrence of excessive contents of heavy metals in peanut production areas is frequent (22). Although some scholars have already investigated the heavy metal pollution in the soils of peanut planting regions (18, 21, 23), no study could be found on the safety risk assessment of applying biogas slurry on the soil and peanut quality.
In light of the abovementioned reasons, the peanut was selected as the field test crop in this study. The potential ecological risks of heavy metals in soil and the growing risks to peanuts under different nitrogen application rates were evaluated. In addition, the estimated daily Intake (EDI) of peanuts and the health risks associated with selected contaminants ingested through peanuts were calculated. The results of this study would help provide a reference for the conversion of biogas by-products into organic fertilizers, which is crucial for the healthy expansion of the peanut industry and the development and implementation of more effective agricultural strategies.
Materials and Methods
Materials and Area
The experiment was conducted from July to November in 2019 at the Scientific Research Base of Shenyang Agricultural University (Shenyang City in Liaoning Province) and lasted for 100 days. Shenyang is located in the southern part of northeast China, with the humid to sub-humid, warm temperate, continental monsoon climate, with plain landform. Peanut (varieties: Nonghua 11) was cultivated in loess brown soil. The biogas slurry used in this study had been collected from a swine manure biogas plant and stored for 2 months before the field application. No pesticide was applied during the experiment.
Experiment Design and Sample Collection
Experiment Design
Each experimental plot was 42 m2 (6.50 m × 6.50 m) and had five equal cropping areas. Two planting ridges (6.20 m × 0.60 m) were set up in each zone, and 10 crops were planted in each row. The row spacing was >0.45 m, and the plant spacing was >0.30 m. An isolation zone was set up between the test plots to avoid the mass transfer between plots. Reasonable application of nitrogen fertilizer is of great significance for the growth of peanuts because the amount of nitrogen required will vary greatly with the different planting methods, planting conditions, and variety yield, the problem of nitrogen fertilizer amount for peanuts has been a concern to scholar for a long time (24, 25). According to different nitrogen rates of biogas slurry, the experimental plots were divided into five groups, including no fertilization, low application rate [8.5 g total nitrogen (TN)/m2·year], European Union application standard (17 g TN/m2·year), domestic average application rate (34 g TN/m2·year) (26), and excessive application (68 g TN/m2·year).
Samples Collection
Before and after planting, the S-shaped sampling method was used to collect soil samples in the 0–30 cm layer, through which the soil background value was recorded as Sorig. After the complete harvesting of the peanuts, soil samples from different plots were recorded as S0 (soil sample from no fertilizer land), S8.5 (soil sample from 8.5 g TN/m2·year land), S17 (soil sample from 17 g TN/m2·year land), S34 (soil sample from 34 g TN/m2·year land), and S68 (soil sample from 68 g TN/m2·year land). After removing weeds, soil pests, stones, and other impurities, all soil samples were naturally air dried, then crushed and passed through a 100-mesh sieve. Table 1 gives the basic physical and chemical properties of the biogas slurry and soil before and after planting.
At maturity, all the plants were harvested, and the samples of roots, stems, leaves, shells, and kernels of the peanut plants were collected separately and kept at −80°C for 12 h followed by freeze-drying and storage at −80°C for later use.
Chemical Analyses
The alkali-hydrolyzed nitrogen (Avail.N.), available phosphorus (Avail.P.), and available potassium (Avail.K.) in biogas slurry were determined according to the Agricultural Standard of the People's Republic of China (27–29). pH (30) was measured by a Seven Compact pH meter (Mettler Toledo, Switzerland). Organic matter (OM) was measured by the potassium dichromate method (31). TN (32) in soils and protein (33) in peanut kernels was determined by an automatic Kjeldahl nitrogen determination apparatus (KD-310, Opsis, Sweden), whereas the hydrolysis diffusion method was used to determine Avail.N. Total phosphorus (TP) and Avail.P. were determined by a spectrophotometer (UV-2600, Shimadzu, Japan). The soil, biogas slurry, and peanut samples were digested by acids (HNO3-HClO4-HF). The contents of Cu, Zn, Pb, Cr, total potassium (TK), Avail.K., and Cd in the samples were determined by atomic absorption spectrophotometry (PinAAcle 900T, PerkinElmer, Waltham, MA, USA). The contents of As and Hg were determined by an atomic fluorescence spectrophotometer (AFS-922, Titan Instruments, Beijing). The main stem height and lateral branch length of peanut plants were directly measured, and the branch number and fruit number per plant were directly counted.
Assessment of Potential Ecological Risk of Heavy Metals
Integrated Pollution Index (IPIN)
The pollution index for single heavy metals (PIi) and IPIN can be used to quantitatively evaluate the extent of heavy metal pollution of soil (34). These indices are calculated using (Equations 1, 2) as follows:
where Ci is the measured content of element i in soil, Ti is the management target value of soil element i, i.e., the reference value in the “Soil Environmental Quality Risk Control Standard for Soil Contamination of Agricultural Land” (Supplementary Table 1) and the parameters are as follows: Zn = 200, Cr = 150, Cu = 50, As = 40, Cd = 0.3, Pb = 90, and Hg = 1.8 (35). IPIavg is the average value of PIi of all heavy metal elements involved, and IPImax is the maximum value.
Potential Ecological Risk Index (RI)
The potential ecological index method comprehensively considers the ecological and toxicological effects of heavy metals to evaluate the degree of soil heavy metal pollution and ecological risk (36), and its calculation formula is as follows:
where is the pollution index of heavy metal, Ci is the measured value of heavy metal, and is the reference ratio of a given heavy metal; the heavy metal background value of the test field soil is used as the reference ratio. is the potential ecological risk index of heavy metals and is the toxicity response parameters of a certain heavy metal. According to the Hakanson potential ecological hazard index, the parameters are: Zn = 1, Cr = 2, Cu = Pb = 5, As = 10, Cd = 30, and Hg = 40 (37).
Biological Enrichment Factor (BCF)
Biological enrichment factor refers to the quantitative relationship incorporating the accumulation and absorption capacity of an organism (38). It is the ratio of the content of a certain element in an organism to the content of this element in its living environment and reflects the risk of, and a degree of damage from, heavy metal accumulation in crops. This experiment mainly explored the biological enrichment of heavy metals in peanut kernels using the following equation:
where Cikernels is the content of heavy metal i in the edible part of crops, Cisoil is the content of heavy metal i in soil.
Transfer Factor (TF)
Transfer factor is expressed as the ratio of the content of heavy metals in leaves to that in the roots of plants (39), which is a measure of the transfer of heavy metals between different plant parts. This experiment mainly explored the transport of heavy metals from roots to leaves and was calculated as follows:
where Cileaf is the content of heavy metal i in leaves, and Ciroot is the content of heavy metal i in roots.
Health Risk Assessment
EDI
Estimated daily intake depends on the heavy metal concentration and daily food consumption of peanuts (40), and EDI (μg kg−1 d−1) is calculated as follows:
where IR is the intake rate of peanuts (kg/person per day; Supplementary Table 2), C is the concentration of heavy metals in peanuts (mg/kg), and WAB is the average weight of a person (kg).
Total Hazard Quotient (THQ)
According to the U.S. Environmental Protection Agency (USEPA) standard methodology, the potential exposure risk of non-carcinogenic substances was indicated by the ratio of EDI to oral reference dose (41). This ratio is called THQ. ADI (the oral reference dose in mg kg−1 d−1) for various elements is: Cu 4 × 10−2, Zn 3 × 10−1, Pb 3.5 × 10−3, Cr 1.5, Cd 3 × 10−3, As 3 × 10−4, and Hg 5 × 10−4 (42, 43). THQ is calculated as follows:
If the THQ value is <1, exposed individuals are unlikely to experience significant toxic effects. If it exceeds 1, the significant toxic effects are likely; as the THQ value increases, so does the likelihood.
Total Target Hazard Quotient (TTHQ)
Total target hazard quotient was used to evaluate the cumulative potential health risks associated with heavy metal accumulated in peanuts. TTHQ is calculated as follows:
If the TTHQ value is <1, the exposed person is unlikely to experience significant toxic effects; if at or above 1, there is a possibility of significant adverse effects due to heavy metal interactions, and this likelihood increases as the TTHQ value increases (44).
Statistical Analysis
Microsoft Office 2019 was used for basic data processing. Origin 2021 was used for drawing figures and performing principal component analysis (PCA). SPSS 22 was used for significance analysis and Canoco5 was used for redundancy analysis.
Results and Discussion
Potential Ecological Risk Assessment of Biogas Slurry Application to Soil
Characteristics of Heavy Metal Content in the Soil in the Root Zone
The comparison between the content of heavy metals in the unplanted and planted soil is shown in Figure 1. Having considered the “Soil Environmental Quality Risk Control Standard for Soil Contamination of Agricultural Land” (35), it was concluded that the soil under consideration had a low risk because the content of heavy metals in each soil sample was smaller than the farmland soil pollution risk reference values. Based on the Chinese agricultural standard “Microbial Organic Fertilizers” (45), the content of heavy metals in biogas slurry used in this experiment did not exceed the permissible limits (Table 2). The contents of As and Hg in the cultivated soil treated with biogas slurry increased significantly, reaching the highest values of 18 and 0.48 mg/kg, respectively, which were 11.12 and 26.67 times higher than those in the control soil. Cd was not detected in the control soil, but its concentration increased in the soil treated with biogas slurry. Such an increase in the concentration of heavy metals in the soil due to the application of biogas slurry needs careful attention. The long-term application of large amounts of biogas slurry will likely cause serious soil heavy metal accumulation. Duan et al. found that after application of biogas slurry for 8 years, the concentration of As, Cd, Cu, and Zn was significantly higher than those in the control (46).

Table 2. The content of heavy metals in biogas slurry and heavy metals limitation for organic fertilizer.
Chen et al. showed that the fungal community composition of paddy soils treated with excessive rates of biogas digestate for a long time was affected by changes in the chemical properties and heavy metal contents (47). It has been shown that the application of biogas slurry could increase the abundance of microorganisms in the soil (48). Microorganisms convert heavy metals to a certain extent. For example, sulfate-reducing bacteria, abundant in biogas slurry, are the main mercury-methylation bacteria (49).
In contrast to As and Hg, the contents of Pb and Cr decreased significantly in different soil samples after the application of biogas slurry. This was attributed to plant absorption of these elements together with nutrients during growth. Plant absorption of some specific elements may be greater than the amount of these elements added via fertilization, leading to a decrease of Pb and Cr contents in the soil after cultivation (26).
Analysis of Soil Pollution Around Roots
The single factor pollution index PIi has five grades in “Specification of Land Quality Geochemical Assessment” (50). The Nemero Composite Pollution Index IPIN is divided into five levels in “The Technical Specification for Soil Environmental Monitoring” (51). In 1980, Hakanson proposed the potential ecological harm index RI with four levels. The evaluation results are shown in Supplementary Table 3.
It can be seen from Table 3 that the integrated pollution coefficients of soil after applying biogas slurry are all <1, indicating that the soil is non-polluted after biogas slurry application (S34 is mildly clean). The potential ecological risk index of soil after applying biogas slurry was >300, indicating that the potential ecological risk to the soil after the application was high, which might have been caused by the high potential ecological risk index of Hg (Figure 2).
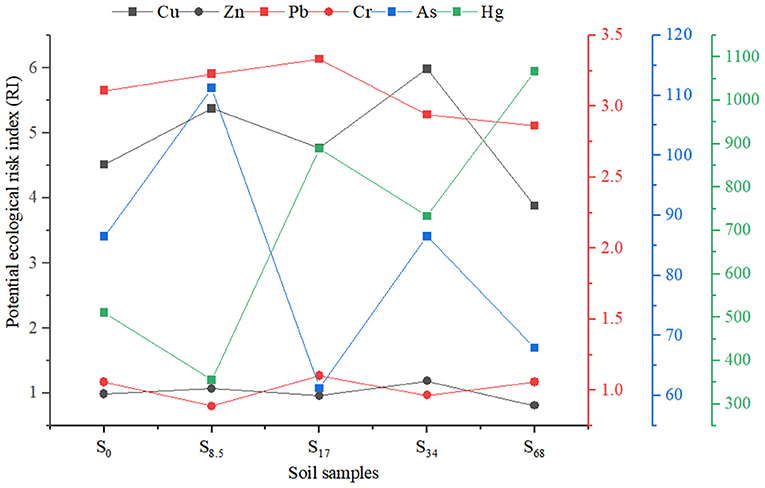
Figure 2. Index of potential ecological risk of heavy metals in soil samples after application of biogas slurry.
Analysis of Heavy Metal Sources in the Soil in the Root Zone
Heavy metals in soil mainly come from the soil parent materials and human activities. PCA can effectively identify the pollution sources of heavy metals in soil (52). The PCA results of heavy metals in the experimental field soil in the present study are shown in Table 4. The first three principal components explained 99.260% of the variation in the heavy metal contents in the experimental field. Figure 3 shows the results of PCA of soil heavy metal content in the study area. The first principal component (PC1) explained 60.296% of the total variation, and the load on the content of Cd, Hg, and As was high (0.480, 0.403, and 0.382, respectively). Considering the results presented in section Characteristics of Heavy Metal Content in Soil in the Root Zone and those shown here, it is inferred that the three metals associated with the PC1 have the same source and are mainly affected by the application of biogas slurry.
The second principal component (PC2) explained 32.870% of the variation, with Cu and Zn having high loads, i.e., 0.631 and 0.642, respectively. Industrial dust, municipal sewage, chemical fertilizers, and pesticide use are the main contributors to Cu and Zn pollution of farmland soil, so it is speculated that the PC2 is mainly affected by human activities, such as sewage irrigation before the experiment.
The third principal component (PC3) explained 6.093% of the variation, and Cr had a high load. The results of the source analysis of Cr in different areas showed that Cr was less affected by human activities (53), so it was speculated that the PC3 was mainly affected by the natural geology of the test area.
Peanut Growth Risk Assessment Due to Biogas Slurry Application
Distribution of Heavy Metals in Plant Organs
As can be seen from Figures 4A–F, the distribution of heavy metals in organs of peanut plants varied among treatments with different nitrogen application rates, which was mainly related to the different absorption capacities of heavy metals in different organs of peanut. In general, heavy metal content in peanut organs was higher at a high than low nitrogen application rate. Cu and Zn are essential trace elements for plant growth. However, when the concentration of Cu and Zn in the soil is higher than a certain critical value, they become toxic to plants. Excessive levels of Zn were found in a small number of peanut kernels in different biogas slurry treatments. The average Cu and Zn contents in peanut grain samples under different nitrogen application rates were significantly lower than 30 and 60 mg/kg, respectively, which are recommended by World Health Organization (69). According to the Limit of Contaminants in “Food Quality Safety and Food Contamination Limits” (54), the content of Pb in peanut grain samples under different nitrogen application rates was lower than the maximum permissible limit of 0.2 mg/kg. The toxicity of Cd would cause leaf yellowing, impaired plant growth, and a significant decrease in pod number (55). As can be seen from Figure 4D, the concentration of Cd in the peanut kernels was below the maximum permissible limit (0.5 mg/kg). Even though a non-essential element for crop growth, Cd can be absorbed by roots. Figure 4E shows that the content of As in peanut kernels under the nitrogen application rates was lower than the maximum permissible limit of 0.1 mg/kg. Cai et al. found that more As was accumulated in peanut shells than kernels (56), which is in line with the findings in the current study. Hg, a metal that is widely distributed in nature, is harmful to crop growth. As depicted in Figure 4F, the content of Hg in peanut kernel samples under different nitrogen application rates exceeded the standard of 0.02 mg/kg.
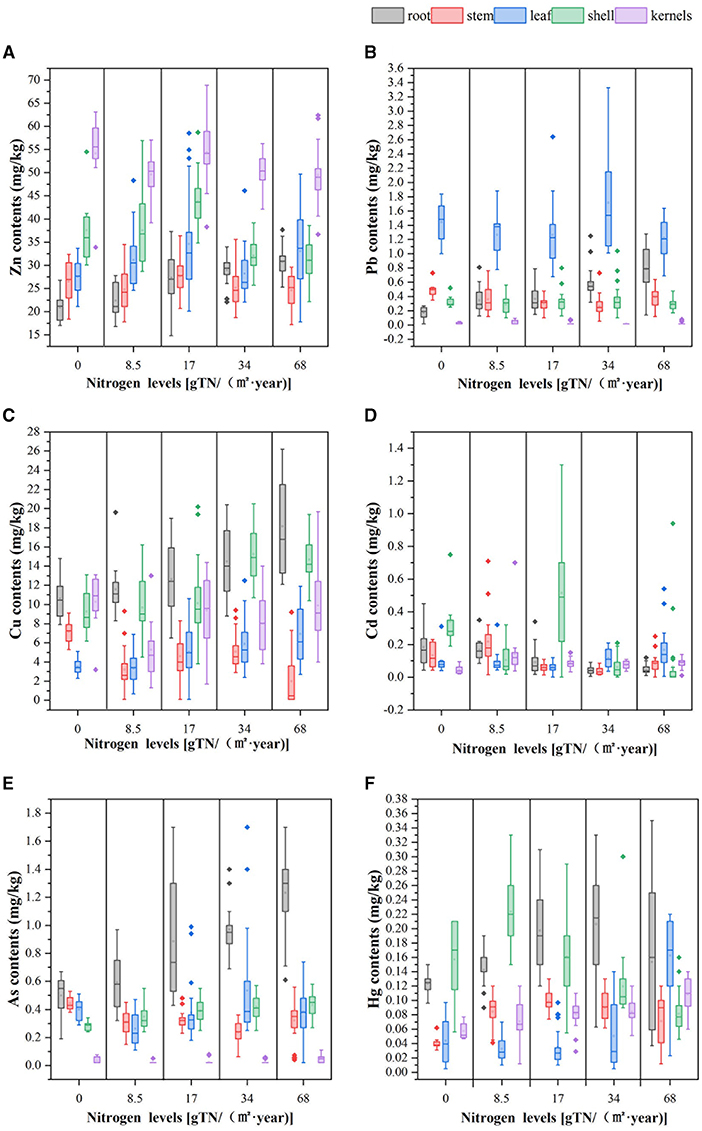
Figure 4. Distribution characteristics of heavy metal content in plant organs. (A) Zn contents, (B) Pb contents, (C) Cu contents, (D) Cd content, (E) As contents, and (F) Hg contents.
Enrichment and Transfer of Heavy Metals in the Soil-Peanut System
The accumulation characteristics of heavy metals in peanuts can be assessed by BCF, which reflects the ability of plants to accumulate heavy metals from the soil. BCF < 0.5 indicates low plant capacity to accumulate heavy metals; 0.5 < BCF <1 indicates some accumulation capacity of heavy metals, and BCF>1 shows that plants have a strong ability to accumulate heavy metals (57). As can be seen in Figure 5, the enrichment capacity of Cd and Zn in the peanut kernels is relatively high. The enrichment ability of Pb and As in the peanut plants was weak [BCF(Pb) and BCF(As) <0.01], and therefore the ecological risk of heavy metals was low. Zhao et al. (58) analyzed bio-accumulation of heavy metals in different parts of peanut, identifying strong bio-accumulation ability for Cu, Zn, and Cd, which was similar to the results of the present study.
The ability of heavy metal translocations from roots to leaves can be expressed as TF. When TF>1, it can be inferred that plants can transfer the heavy metal elements from roots to the aboveground part. When TF <1, plants prevent the transport of heavy metals from roots to the aboveground parts (59). As seen in Figure 6, the TF of Pb in peanuts was high, in contrast, the TF of Cu and As was small, and hence the transfer ability was low. Under a high nitrogen application rate, the TF of Cd increased, suggesting a stimulating effect of high nitrogen supply on Cd distribution to leaves (60).
Correlation Analysis of Peanut and Soil Environment
Table 5 and Figure 7 show the peanut lateral branch length, branch number, fruit number per plant, main stem height, and protein under different fertilization treatments, respectively. It can be concluded that nitrogen application level has a significant influence on the protein content of peanut main stem height, lateral branch length (p < 0.05). With the increase of nitrogen application rate, the main stem height and protein content of peanut plants also showed an increasing trend. Through the redundancy analysis of the correlation between peanut and environmental factors, we revealed the relationship between soil properties and peanut traits after the application of biogas slurry (Figure 8). Total variation was found to be 140.79, and explanatory variables account for 82.6%. The decreasing order of effects of soil factors on the peanut kernels was TN > OM > Avail.P. The contents of Cu and Zn in peanut kernels were positively correlated with the soil TN after biogas slurry application. The branch number and fruit number per plant of peanut were positively correlated with the content of Avail.P in the soil after application of biogas slurry. Li et al. found that peanut protein content was negative correlated with soil OM and Avail.P. after biogas slurry application, which was consistent with the results of this study (61).
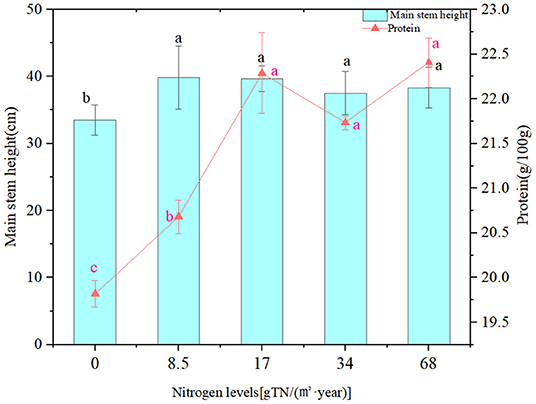
Figure 7. Effects of different fertilization treatments on main stem height and grain protein content of peanut. Lowercase letters a, b indicate significant differences at the level of 0.05. Marked with different letters means the difference is significant, marked with the same letter means the difference is not significant.
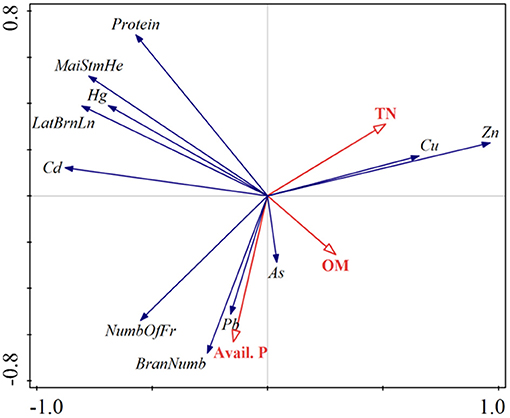
Figure 8. Redundancy analyses of the correlations between botanical character of peanut, protein and metal contents in peanut kernels (blue lines) and soil properties (red lines) using Canoco. MaiStmHe, main stem height; LatBrnLn, lateral branch length; BranNumb, branching number; NumbOfFr, number of fruit per plant.
Health Risk Assessment of Biogas Slurry Used in Peanut Cultivation
Supplementary Table 2 presents the information on peanut consumers and their consumption and evaluates the intake of heavy metals by different groups via peanuts (62). The EDI (μg kg−1 d−1) values of peanuts from different fertilization treatments were shown in Table 6. Using the Provisional Tolerable Daily Intake (PTDI) as the reference standard, the PTDI values of Cu, Zn, Pb, Cd, As, and Hg in adults were 500, 1,000, 25, 0.83, 2.14, and 5 μg kg−1 d−1, respectively (63). If EDI value is less than PTDI value, it indicates that the health risk of heavy metals in peanuts is small. If EDI value is greater than PTDI value, it indicates that the health risk of heavy metals in peanuts is high. With different consumption levels, EDI values of all metals in children aged 2–6 years and standard adults were lower than PTDI. The EDI values of children aged 2–6 at the average consumption and high consumption level were significantly higher than those of standard adults. The EDI value of Cu and Zn ingested via peanuts in two groups was significantly higher than that of other metals. As can be seen from Figure 4, this was mainly because the contents of these two metals in peanut kernels were relatively high under different nitrogen application rates.
For the same group, the EDI value of different metals was greater at high consumption than average consumption level. Although EDI(Hg) was less than PTDI, Hg in peanut kernels under different nitrogen application rates exceeded the standard according to the results shown in section Distribution of Heavy Metals in Plant Organs. This phenomenon is noteworthy because children at high consumption levels are more likely to ingest Hg by eating peanuts. Hg can cause teratogenicity and chromosomal abnormalities. Chronic mercury poisoning damages the human nervous system. Methylmercury is easily transferred from mother to fetus through the placenta (64). Chen et al. (65) assessed the dietary exposure risk of Cd in peanuts in China and found that the risk from overall dietary exposure is low. At the general consumption level, the exposure of the population is far lower than the provisional tolerable monthly intake (PTMI) value; however, at a high consumption level, 5% of individuals in the population are close to or exceed PTMI value, which carries potential risks.
As can be seen from Table 7, under different fertilization treatments, the THQ value of peanut intake by different groups with different consumption levels was <1. Similar to the EDI value, the THQ value for children aged 2–6 was higher than that of standard adults at both average and high consumption levels. For the same group, THQ values of different metals were larger at high than average consumption level. The THQ value of Cu, As, Hg, and Zn ingested via peanuts in two groups was higher than that of other metals. Although Cu and Zn are essential trace elements for humans, excessive Cu and Zn can adversely affect human health. Excess Cu causes liver and gallbladder problems. Excessive intake of Zn may cause deficiency of other nutrients, such as Ca, P, and Fe and lead to poisoning. Arsenic may cause a systemic disease associated with skin damage, which can harm human skin, respiratory, digestive, urinary, cardiovascular, nervous, hematopoietic, and other systems. The TTHQ was higher under high than low nitrogen application. The TTHQ values for children aged 2–6 with high consumption levels were >1 (except at 8.5 g TN/m2·year), and the TTHQ values of other consumer groups were all <1. TTHQ above 1 indicates that this population is likely to have significant adverse effects due to heavy metal ingestion, and this possibility increases with an increase in TTHQ value at the high nitrogen application rate. Similarly, some studies have also shown potential pollution by the use of biogas slurry in agriculture. Zhang et al. (66) studied the effects of biogas slurry on vegetable crops cultivated in open fields. The results showed that applying digested pig slurry in the vegetable fields could improve soil fertility. The contents of Hg, Zn, Cr, Cd, Pb, and Cu in fertilized soil did not exceed the maximum allowable content for vegetable crop soils in China. However, due to the repeated use of digested pig slurry, the high zinc accumulation should be of concern. Shamsollahi et al. (16) used raw sludge and anaerobically digested sludge for lettuce cultivation. It was found that the application of anaerobically digested sludge could increase the bioaccumulation rate of heavy metals in crops and increase risks to human health. Bian et al. (67) studied the health risks of multi-faceted exposure to heavy metals (Pb, Cd, Cr, Zn, Cu, and As) via water, soil, air, and locally produced food in the Taihu Lake Basin. Their results showed that Zn, Pb, and Cd in soils irrigated with biogas slurry exceeded the soil quality standard values, and the vegetables and grains were contaminated by Pb and Cd, exceeding the allowable limits. Xie et al. (68) applied biogas slurry repeatedly for 4 years (N1) and 10 years (N2) in two vegetable plots to measure heavy metal content (Zn, Cu, Cr, and Pb) and assess the ecological and health risks of short- and long-term fertilization. The results showed long-term fertilization increased the content of heavy metals. In general, N2 had a higher non-carcinogenic and carcinogenic risk index compared with N1. Consistent with the results of the present study, Xie et al. also concluded that children are more sensitive to environmental changes than adults, and more attention should be paid to protecting their health.
Conclusions
In this study, three indicators, i.e., soil ecological risk, peanut growth risk, and human health risk, were comprehensively evaluated to assess the safety of biogas slurry application as a nutrient supplier. According to the results achieved herein, the application of biogas slurry affected the physical and chemical properties of soil, plants' traits and protein content of peanuts, and the content of heavy metals in different parts of soil and in peanuts. As a nutrient source for peanut cultivation, biogas slurry promotes the growth of peanuts; however, it also strengthens the accumulation of heavy metals in the plants and exacerbates soil quality. Moreover, the use of peanuts, fertilized with biogas slurry, can transfer such heavy metals to the bodies of human beings. This risk should not be overlooked because it becomes more pronounced with increased biogas slurry application and will be more dangerous for children. This poses a serious challenge to the replacement of synthetic fertilizers with organic fertilizers in the future. Therefore, long-term monitoring is needed, one the one hand, to understand food safety under crop cultivations with organic fertilizers, such as biogas slurry, and on the other hand, to find solutions over how to reduce and control heavy metals in biogas slurry when it is intended to be applied on farmland.
Data Availability Statement
The original contributions presented in the study are included in the article/Supplementary Material, further inquiries can be directed to the corresponding author/s.
Author Contributions
QL: methodology and investigation. ZZ: writing—original draft, conceptualization, methodology, and data curation. ZX: validation and formal analysis. DL: investigation. ZW: data analysis. YR: supervision. ZM: supervision and project administration. LH: writing—review and editing, supervision, project administration, and funding acquisition. All authors contributed to the article and approved the submitted version.
Funding
This work was financially supported by the National Natural Science Foundation of China (Grants No. 31902208), the fundamental research funds for the Central Non-profit Scientific Institution (161001202003_02201), the Sichuan Science and Technology Program (2018JY0543), and the Agricultural Science and Technology Innovation Project of Chinese Academy of Agricultural Sciences (CAAS-ASTIP-2016-BIOMA).
Conflict of Interest
The authors declare that the research was conducted in the absence of any commercial or financial relationships that could be construed as a potential conflict of interest.
Publisher's Note
All claims expressed in this article are solely those of the authors and do not necessarily represent those of their affiliated organizations, or those of the publisher, the editors and the reviewers. Any product that may be evaluated in this article, or claim that may be made by its manufacturer, is not guaranteed or endorsed by the publisher.
Supplementary Material
The Supplementary Material for this article can be found online at: https://www.frontiersin.org/articles/10.3389/fnut.2021.702096/full#supplementary-material
Abbreviations
Cu, copper; EU, European Union; Zn, zinc; WHO, World Health Organization; Pb, lead; USEPA, U.S. Environmental Protection Agency; Cr, chromium; IPIN, integrated pollution index; Cd, cadmium; RI, potential ecological risk index; Hg, mercury; BCF, biological enrichment factor; As, arsenic; TF, transfer factor; TN, total nitrogen; EDI, estimated daily intake; TP, total phosphorus; THQ, total hazard quotient; TK, total potassium; TTHQ, total target hazard quotient; OM, organic matter; PTDI, provisional tolerable daily intake; Avail. N., alkali-hydrolyzed nitrogen; PTMI, provisional tolerable monthly intake; Avail.P., available phosphorus; Avail.K., available potassium.
References
1. Chavez-Fuentes JJ, Capobianco A, Barbuova J, Hutnan M. Manure from our agricultural animals: a quantitative and qualitative analysis focused on biogas production. Waste Biomass Valori. (2017) 8:1749–57. doi: 10.1007/s12649-017-9970-5
2. Sun C, Wu H. Pollution from animal husbandry in China: a case study of the Han River Basin. Water Sci Technol. (2012) 66:872–8. doi: 10.2166/wst.2012.259
3. Huang X, Liu Z, Xue J, Luo Y, Zhang C, Wang Q, et al. Purifying eutrophic wastewater from geese farm with plant floating bed in winter. Pol J Environ Stud. (2021) 30:1171–80. doi: 10.15244/pjoes/125771
4. Chiumenti A, da Borso F, Teri F, Chiumenti R, Piaia B. Full-scale membrane filtration system for the treatment of digestate from a co-disgestion plant. Appl Eng Agric. (2013) 29:985–90. doi: 10.13031/aea.29.10117
5. Cantrell KB, Ro KS, Szogi AA, Vanotti MB, Smith MC, Hunt PG. Green farming systems for the Southeast USA using manure-to-energy conversion platforms. J Renew Sustain Ener. (2012) 4:041401. doi: 10.1063/1.3663846
6. Xuan Z, Zhang X, Zhu H, Xu Y. Study on pollutant characteristics of different types of manure sludge drying condensate. IOP Conf Ser Earth Environ Sci. (2020) 546:042017. doi: 10.1088/1755-1315/546/4/042017
7. Burt CD, Cabrera ML, Rothrock MJ, Kissel DE. Urea hydrolysis and calcium carbonate precipitation in gypsum-amended broiler litter. J Environ Qual. (2018) 47:162–9. doi: 10.2134/jeq2017.08.0337
8. Chini A, Hollas CE, Bolsan AC, Antes FG, Treichel H, Kunz A. Treatment of digestate from swine sludge continuous stirred tank reactor to reduce total carbon and total solids content. Environ Dev Sustain. (2021) 23:12326–41. doi: 10.1007/s10668-020-01170-6
9. Yetilmezsoy K, Sakar S. Improvement of COD and color removal from UASB treated poultry manure wastewater using Fenton's oxidation. J Hazard Mater. (2008) 151:547–58. doi: 10.1016/j.jhazmat.2007.06.013
10. Zhang MY, Liang W, Tu ZN, Li RH, Zhang ZQ, Ali A, et al. Succession of bacterial community during composting: dissimilarity between compost mixture and biochar additive. Biochar. (2021) 3:229–37. doi: 10.1007/s42773-020-00078-8
11. Yin D-M, Mahboubi A, Wainaina S, Qiao W, Taherzadeh MJ. The effect of mono- and multiple fermentation parameters on volatile fatty acids (VFAs) production from chicken manure via anaerobic digestion. Bioresour Technol. (2021) 330:124992. doi: 10.1016/j.biortech.2021.124992
12. de Oliveira ACL, Milagres RS, Orlando WD, Renato ND. Evaluation of Brazilian potential for generating electricity through animal manure and sewage. Biomass Bioenerg. (2020) 139:105654. doi: 10.1016/j.biombioe.2020.105654
13. Zhang J, Wang MY, Cao YC, Liang P, Wu SC, Leung AOW, et al. Replacement of mineral fertilizers with anaerobically digested pig slurry in paddy fields: assessment of plant growth and grain quality. Environ Sci Pollut R. (2017) 24:8916–23. doi: 10.1007/s11356-015-5125-z
14. Bian B, Wu HS, Zhou LJ. Contamination and risk assessment of heavy metals in soils irrigated with biogas slurry: a case study of Taihu basin. Environ Monit Assess. (2015) 187:4377. doi: 10.1007/s10661-015-4377-x
15. Wang H, Dong YH, Yang YY, Toor GS, Zhang XM. Changes in heavy metal contents in animal feeds and manures in an intensive animal production region of China. J Environ Sci. (2013) 25:2435–42. doi: 10.1016/S1001-0742(13)60473-8
16. Shamsollahi HR, Alimohammadi M, Momeni S, Naddafi K, Nabizadeh R, Khorasgani FC, et al. Assessment of the health risk induced by accumulated heavy metals from anaerobic digestion of biological sludge of the lettuce. Biol Trace Elem Res. (2019) 188:514–20. doi: 10.1007/s12011-018-1422-y
17. Wang T, Chen N, Hu DQ, Wang M, Chen MN, Pan LJ, et al. Status and development trend of consumption of peanut in the world. J Peanut Sci. (2014) 43:39–45. doi: 10.14001/j.issn.1002-4093.2014.04.006
18. Yang BL, Zhang CX, Zhang XJ, Wang G, Li L, Geng HR, et al. Survey of aflatoxin B-1 and heavy metal contamination in peanut and peanut soil in China during 2017-2018. Food Control. (2020) 118:107372. doi: 10.1016/j.foodcont.2020.107372
19. Jung M, Kim J, Ahn SM. Factors associated with frequency of peanut consumption in Korea: a national population-based study. Nutrients. (2020) 12:1207. doi: 10.3390/nu12051207
20. Wang SS, Li G. Assessment of cadmium bioaccumulation and distribution in the kernels of peanut widely cultivated in China. Ecotox Environ Safe. (2014) 108:23–8. doi: 10.1016/j.ecoenv.2014.06.029
21. Wang XB, Liu WX, Li ZG, Teng Y, Christie P, Luo YM. Effects of long-term fertilizer applications on peanut yield and quality and plant and soil heavy metal accumulation. Pedosphere. (2020) 30:555–62. doi: 10.1016/S1002-0160(17)60457-0
22. Bian B, Lin C, Lv L. Health risk assessment of heavy metals in soil-plant system amended with biogas slurry in Taihu basin, China. Environ Sci Pollut R. (2016) 23:16955–64. doi: 10.1007/s11356-016-6712-3
23. Dai XH, Bai YZ, Jiang J, Chen XM, Zhou HY, Yin NR, et al. Cadmium in Chinese postharvest peanuts and dietary exposure assessment in associated population. J Agr Food Chem. (2016) 64:7849–55. doi: 10.1021/acs.jafc.6b02639
24. Liu Z, Gao F, Li Y, Zhao J, Wang Y, Wang Z, et al. Grain yield, and nitrogen uptake and translocation of peanut under different nitrogen management systems in a wheat–peanut rotation. Agronomy J. (2020) 112: 1828–38. doi: 10.1002/agj2.20065
25. Gao H, Meng W, Zhang C, Werf W, Zhang Z, Wan S, et al. Yield and nitrogen uptake of sole and intercropped maize and peanut in response to N fertilizer input. Food Energy Secur. (2020) 9:e187. doi: 10.1002/fes3.187
26. Ai P, Jin KD, Alengebawy A, Elsayed M, Meng L, Chen MD, et al. Effect of application of different biogas fertilizer on eggplant production: analysis of fertilizer value and risk assessment. Environ Technol Inno. (2020) 19:101019. doi: 10.1016/j.eti.2020.101019
27. Ministry of Agriculture. Method for Determination of Available Phosphorus in Soil (NY/T1121.7-2014). Beijing: Standards Press of China (2014).
28. Ministry of Agriculture. Determination of Exchangeable Potassium and Non-Exchangeable Potassium Content in Soil (NY/T889-2004). Beijing: Standards Press of China (2004).
29. Hebei Provincial Bureau of Quality and Technical Supervision. Soil Available Nitrogen Determination (DB13/T843-2007). Shijiazhuang City, Hebei Province (2007).
30. Ministry of Agriculture. Method for Determination of soil pH (NY/T1121.2-2006). Beijing: Standards Press of China (2006).
31. Ministry of Agriculture. Organic Fertilizer (NY525-2012). Beijing: Standards Press of China (2012).
32. Ministry of Agriculture. Determination of Total Nitrogen in Soil-Automatic Kjeldahl Apparatus Method (NY/T1121.24-2012). Beijing: Standards Press of China (2012).
33. National Health and Family Planning Commission. Determination of Protein in Food (GB5009.5-2016). Beijing: Standards Press of China (2016).
34. Fan SX, Gan ZT, Li MJ, Zhang ZQ, Zhou Q. Progress of assessment methods of heavy metal pollution in soil. Chin Agric Bull. (2010) 26:310–5.
35. Ministry of Ecological Environment. Soil Environmental Quality Risk Control Standard for Soil Contamination of Agricultural Land (GB15618-2018). Beijing: Standards Press of China (2018).
36. Kumar V, Sharma A, Pandita S, Bhardwaj R, Thukral AK, Cerda A. A review of ecological risk assessment and associated health risks with heavy metals in sediment from India. Int J Sediment Res. (2020) 35:516–26. doi: 10.1016/j.ijsrc.2020.03.012
37. Zhou YL, Yang ZB, Wang QL, Wang CW, Liu F, Song YT, et al. Potential ecological risk assessment and source analysis of heavy metals in soil-crop system in Xiong'an New district. Environ Sci. (2021) 42:2003–15. doi: 10.13227/j.hjkx.202007253
38. Hatami-Manesh M, Mortazavi S, Solgi E, Mohtadi A. Assessing the uptake and accumulation of heavy metals and particulate matter from ambient air by some tree species in Isfahan Metropolis, Iran. Environ Sci Pollut Res Int. (2021) 28:41451–63. doi: 10.1007/s11356-021-13524-2
39. Chen Z, Muhammad I, Zhang Y, Hu W, Lu Q, Wang W, et al. Transfer of heavy metals in fruits and vegetables grown in greenhouse cultivation systems and their health risks in Northwest China. Sci Total Environ. (2021) 766:142663. doi: 10.1016/j.scitotenv.2020.142663
40. Akhtar S, Riaz M, Naeem I, Gong YY, Ismail A, Hussain M, et al. Risk assessment of aflatoxins and selected heavy metals through intake of branded and non-branded spices collected from the markets of Multan city of Pakistan. Food Control. (2020) 112:107132. doi: 10.1016/j.foodcont.2020.107132
41. Yu R, Liu Q, Liu JS, Wang QC, Wang Y. Concentrations of organophosphorus pesticides in fresh vegetables and related human health risk assessment in Changchun, Northeast China. Food Control. (2016) 60:353–60. doi: 10.1016/j.foodcont.2015.08.013
42. Liang H, Wu WL, Zhang YH, Zhou SJ, Long CY, Wen J, et al. Levels, temporal trend and health risk assessment of five heavy metals in fresh vegetables marketed in Guangdong Province of China during 2014-2017. Food Control. (2018) 92:107–20. doi: 10.1016/j.foodcont.2018.04.051
43. Saha N, Mollah MZI, Alam MF, Rahman MS. Seasonal investigation of heavy metals in marine fishes captured from the Bay of Bengal and the implications for human health risk assessment. Food Control. (2016) 70:110–8. doi: 10.1016/j.foodcont.2016.05.040
44. Ullah AKMA, Maksud MA, Khan SR, Lutfa LN, Quraishi SB. Dietary intake of heavy metals from eight highly consumed species of cultured fish and possible human health risk implications in Bangladesh. Toxicol Rep. (2017) 4:574–9. doi: 10.1016/j.toxrep.2017.10.002
45. Ministry of Agriculture. Microbial Organic Fertilizers (NY884-2012). Beijing: Standards Press of China (2012).
46. Duan GL, Zhang HM, Liu YX, Jia Y, Hu Y, Cheng WD. Long-term fertilization with pig-biogas residues results in heavy metal accumulation in paddy field and rice grains in Jiaxing of China. Soil Sci Plant Nutr. (2012) 58:637–46. doi: 10.1080/00380768.2012.726597
47. Chen ZM, Wang Q, Ma JW, Zou P, Yu QG, Jiang LN. Fungal community composition change and heavy metal accumulation in response to the long-term application of anaerobically digested slurry in a paddy soil. Ecotox Environ Safe. (2020) 196:110453. doi: 10.1016/j.ecoenv.2020.110453
48. Mao N, Yang JX, Zhang XB, Wang JC, Ma SR. Effects of different biogas fertilizer treatments on soil microbial number and enzyme activities in vineyards. Tianjin Agric Sci. (2020) 26:41–5. doi: 10.3969/j.issn.1006-6500.2020.08.010
49. Zhu JS, Liao DX, Wang DY, Wang LC, Wang YM, Yin DL, et al. Concentration and edible risk of total mercury and methylmercury in rice paddy irrigated with biogas slurry. Ecol Environ Monit Three Gorges. (2018) 3:75–81. doi: 10.19478/j.cnki.2096-2347.2018.01.12
50. Ministry of Land and Resources. Specification of Land Quality Geochemical Assessment (DZ/T0295-2016). Beijing: Standards Press of China (2016).
51. State Environmental Protection Administration. The Technical Specification for Soil Environmental Monitoring (HJ/T166-2004). Beijing: Standards Press of China (2004).
52. Wu H, Xu C, Wang J, Xiang Y, Ren M, Qie H, et al. Health risk assessment based on source identification of heavy metals: a case study of Beiyun River, China. Ecotox Environ Safe. (2021) 213:112046. doi: 10.1016/j.ecoenv.2021.112046
53. Wang Y, Guo G, Zhang D, Lei M. An integrated method for source apportionment of heavy metal(loid) s in agricultural soils and model uncertainty analysis. Environ Pollut. (2021) 276:116666. doi: 10.1016/j.envpol.2021.116666
54. National Health and Family Planning Commission. Food Quality Safety and Food Contamination Limits. Beijing: Standards Press of China (2017).
55. Wang F, Wang JG, Liu D W, Li L, Wan SB, Zhang H, et al. Cadmium concentration and translocation in paddy fields with different peanut varieties. Chin J Oil Crop Sci. (2019) 41:568–76. doi: 10.7505/j.issn.1007-9084.2019.04.011
56. Cai K, Yu QH, Zhao ZY, Wang WJ, Zhao M. As and Hg accumulation and transformation rules in different varieties of peanut plants. Chin Agric Sci Bull. (2010) 26:167–72.
57. Xing CB, Hao LB, Zhao XY, Zhao YY, Tang SD, Zang LB, et al. Distribution and migration characteristics of heavy metals in soil-plant system in Songbei Pb-Zn mining area, Xingcheng, Liaoning province. Sci Technol Eng. (2020) 20:406.
58. Zhao M, Cai K, Ren Y, Sun YH, Wang WJ, Chen JM, et al. Bioaccumulation and transformation of Cu, Zn, Pb, Cd and Cr in different peanut (Arachis hypogaea L.) cultivars. J Peanut Sci. (2010) 39:1–7. doi: 10.3969/j.issn.1002-4093.2010.03.001
59. Liu Y, Zhuang P, Li ZA, Zou B, Wang G, Li NY, et al. Effects of fertiliser and intercropping on cadmium uptake by maize. Chem Ecol. (2013) 29:489–500. doi: 10.1080/02757540.2013.810720
60. Tan CQ, He QF, Qin YY, Shen WH, Liu X Cao.Y.Y. Effect of nitrogen application on seedling growth and cadminm uptake and distribution in Acacia mangium × Acacia auriculiformis under cadmium stress. J Plant Nutr Fertil. (2017) 23:1326–34. doi: 10.11674/zwyf.17011
61. Li XH, Guo HH, Yang LP, Yang P, Wan SB. Effects of soil fertility on peanut quality. J Anhui Agric Sci. (2010) 38:5500–2. doi: 10.13989/j.cnki.0517-6611.2010.10.070
62. Ding X, Wu L, Li P, Zhang Z, Zhou H, Bai Y, et al. Risk assessment on dietary exposure to aflatoxin B-1 in post-harvest peanuts in the Yangtze river ecological region. Toxins. (2015) 7:4157–74. doi: 10.3390/toxins7104157
63. Dai HW, Song XX, Xin JL, Huang BF. Research progress on health risk assessment of heavy metals via consumption of rice in China. J Anhui Agric Sci. (2016) 44:60–2+77. doi: 10.13989/j.cnki.0517-6611.2016.29.021
64. Teng W, Liu Q, Li Q, Liu YB. Hazard and Risk Assessment of Heavy Metal Pollution to Agricultural Products. Beijing: Chemical Industry Press (2010).
65. Chen ZJ, Song W, Li PW, Ding XX, Wang M, Qian YZ. Survey and dietary risk assessment of cadmium in peanut produced in China. J Agro Environ Sci. (2012) 31:237–44.
66. Zhang SH, Hua YM, Deng LW. Nutrient status and contamination risks from digested pig slurry applied on a vegetable crops field. Int J Environ Res Public Health. (2016) 13:406. doi: 10.3390/ijerph13040406
67. Bian B, Zhou LJ, Li L, Lv L, Fan YM. Risk assessment of heavy metals in air, water, vegetables, grains, and related soils irrigated with biogas slurry in Taihu Basin, China. Environ Sci Pollut R. (2015) 22:7794–807. doi: 10.1007/s11356-015-4292-2
68. Xie JY, Li FP, Mei B. Pollution and health risk assessment of heavy metals following repeated biogas slurry application in two vegetable fields. IOP Conf Ser Earth Environ Sci. (2021) 647:012209. doi: 10.1088/1755-1315/647/1/012209
Keywords: risk assessment, biogas slurry, heavy metal, peanuts, cultivation
Citation: Liu Q, Zhao Z, Xue Z, Li D, Wen Z, Ran Y, Mei Z and He L (2021) Comprehensive Risk Assessment of Applying Biogas Slurry in Peanut Cultivation. Front. Nutr. 8:702096. doi: 10.3389/fnut.2021.702096
Received: 29 April 2021; Accepted: 31 August 2021;
Published: 14 October 2021.
Edited by:
Tong Wu, Research Center for Eco-Environmental Sciences (CAS), ChinaReviewed by:
Pranita Jaiswal, Indian Council of Agricultural Research (ICAR), IndiaShikun Cheng, University of Science and Technology Beijing, China
Hupenyu Allan Mupambwa, Sam Nujoma Marine and Coastal Resources Research Center (SANUMARC), Namibia
Copyright © 2021 Liu, Zhao, Xue, Li, Wen, Ran, Mei and He. This is an open-access article distributed under the terms of the Creative Commons Attribution License (CC BY). The use, distribution or reproduction in other forums is permitted, provided the original author(s) and the copyright owner(s) are credited and that the original publication in this journal is cited, in accordance with accepted academic practice. No use, distribution or reproduction is permitted which does not comply with these terms.
*Correspondence: Li He, aGVsaUBjYWFzLmNu; Zili Mei, bWVpemlsaUBjYWFzLmNu