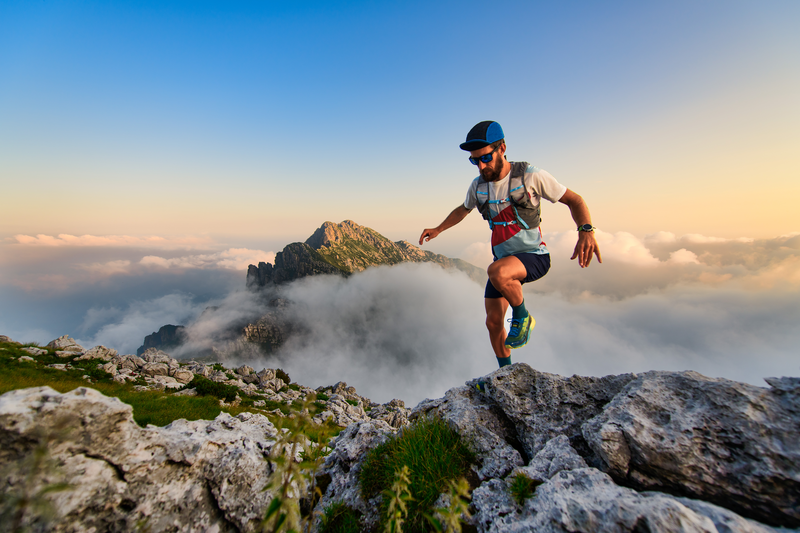
95% of researchers rate our articles as excellent or good
Learn more about the work of our research integrity team to safeguard the quality of each article we publish.
Find out more
ORIGINAL RESEARCH article
Front. Nutr. , 23 July 2021
Sec. Nutrigenomics
Volume 8 - 2021 | https://doi.org/10.3389/fnut.2021.692504
This article is part of the Research Topic Micronutrients and Fatty Acids in Precision Nutrition Strategies View all 12 articles
Background: The sodium iodide symporter is responsible for the transfer of iodine into breast milk and is encoded for by the SLC5A5 gene. The role of genetic variants in the SLC5A5 gene locus in relation to the transfer of iodine from plasma into breast milk in healthy lactating individuals has, to our knowledge, not been explored.
Objective: To identify and characterize possible genetic variants of the SLC5A5 gene in women of African descent living in urban South Africa, and to study associations with breast milk iodine concentrations (BMIC) in lactating women.
Methods: This study is affiliated to the Nutrition during Pregnancy and Early Development (NuPED) cohort study (n = 250 enrolled pregnant women). In a randomly selected sub-sample of 32 women, the SLC5A5 gene was sequenced to identify known and novel variants. Of the identified variants, genotyping of selected variants was performed in all pregnant women who gave consent for genetic analyses (n = 246), to determine the frequency of the variants in the study sample. Urinary iodine concentration (UIC) in spot urine samples and BMIC were measured to determine iodine status. Associations of SLC5A5 genetic variants with BMIC were studied in lactating women (n = 55).
Results: We identified 27 variants from sequencing of gene exomes and 10 variants were selected for further study. There was a significant difference in BMIC between the genotypes of the rs775249401 variant (P = 0.042), with the homozygous GG group having lower BMIC [86.8 (54.9–167.9) μg/L] compared to the (A) allele carriers rs775249401(AG+AA) [143.9 (122.4–169.3) μg/L] (P = 0.042). Of the rs775249401(GG), 49% had UIC <100 μg/L and 61% had BMIC <100 μg/L. On the other hand, 60% of the rs775249401(AG+AA) carriers had UIC <100 μg/L, and none had a BMIC <100 μg/L.
Conclusion: Our results suggest that A-allele carriers of rs775249401(AG+AA) are likely to have higher iodine transfer into breast milk compared to the homozygous GG counterparts. Thus, genetic variations in the SLC5A5 gene may play an important role in the transfer of iodine from plasma into breast milk and may partially explain inter-individual variability in BMIC.
The sodium iodide symporter (NIS) is an intrinsic plasma membrane glycoprotein mediating iodide uptake into thyroid follicular cells. The NIS protein consisting of 643 amino acids is encoded by the SLC5A5 gene, which is located on the forward strand of chromosome 19 (19: 17,982,754–18,005,983, GRCh37.p12) with an open reading frame consisting of 1929 nucleotides arranged as 14 introns and 15 exons (1). The NIS plays a crucial role in iodine metabolism and thyroid regulation (2). Besides thyroid hormone synthesis, the NIS is expressed in breast tissue during late pregnancy and lactation and is responsible for the transfer of iodine from plasma into mammary epithelial cells of lactating breasts (3).
Breast milk is a crucial source of iodine for the breastfeeding infant (4). Thus, adequate breast milk iodine concentration (BMIC) is important for meeting the iodine requirements of infants. Maternal iodine intake, estimated by measuring urinary iodine concentration (UIC), is known to greatly influence BMIC. Therefore, lactating women with insufficient iodine intake reportedly excrete insufficient breast milk iodine to meet the infants' needs (4–6). BMIC of 92 to 150 μg/L have been suggested to provide sufficient iodine to infants (6–8), but consensus on a BMIC threshold to define adequate iodine nutrition has not yet been reached. However, mean and median BMIC values were shown to vary widely between areas, with values typically ranging from <50 μg/L in iodine-deficient areas (4) to 100–150 μg/L in areas of iodine sufficiency (7) but being as high as 150–180 μg/L in areas of adequate iodine supply (9, 10). In a previous cross-sectional study in a convenience sample of 100 lactating women living in a semi-urban area of South Africa, we observed a median BMIC of 179 (126–269) μg/L with large inter-individual variations (11).
Previous research has shown that there is a preferential fractional excretion of iodine in breast milk rather than urine among participants with poor iodine status living in iodine sufficient regions (12). Additionally, women from iodine-deficient regions showed a constant partitioning of iodine into breastmilk. As such, participants with suboptimal iodine status have been shown to present with adequate BMIC. This could be explained by a protective mechanism that allows for a steady supply of iodine to breastfed infants of lactating women with suboptimal iodine status (12, 13), and the NIS is likely to play a major role.
Since there are limited data available on the role of genetic variants in the SLC5A5 gene in relation to breast milk iodine concentration, we aimed to characterize genetic variants in the SLC5A5 gene of women of African descent living in urban South Africa. Further, we investigated the relationship between selected variants with breast milk iodine concentration in lactating women.
The NuPED study was a prospective cohort study conducted in Johannesburg, South Africa from March 2016 to July 2018. The study protocol has be previously published (14). In brief, pregnant women (n = 250) were enrolled if they were between 18 and 39 years of age, <18 weeks gestational age, born in South Africa or a neighboring country, have lived in Johannesburg for at least 12 months, were able to communicate effectively in one of the local languages, non-smoking, and expecting a singleton. Pregnant women were excluded from participation if they reported use of illicit drugs, had a known non-communicable disease such as diabetes, renal disease, history of high blood cholesterol and hypertension, and had a known infectious disease such as tuberculosis or hepatitis, or known serious illness such as cancer, lupus or psychosis. HIV positive women were included in the study. Pregnant women were assessed at <18, 22, and 36 weeks gestation. Follow-up assessments in the women and their infants were performed at 6, 7.5, and 12 months after birth. Of the 250 enrolled women, a total of 98 mother-infant pairs participated in the 6-month follow-up.
In a randomly selected sub-sample of 32 women, the SLC5A5 gene was sequenced to identify and characterize genetic variants. Of the identified variants, genotyping of selected variants was performed in all pregnant women enrolled in the NuPED study and who gave consent for genetic analyses (n = 246), to determine the frequency of the variants in the study sample.
This study was conducted according to the guidelines of the Declaration of Helsinki and all procedures involving human participants were approved by the Human Research Ethics Committees (HREC) of the North-West University (NWU-00186-15-A1 for the NuPED pregnancy phase, NWU-00049-16-A1 for the postnatal phase) and the University of the Witwatersrand, Johannesburg (M150968 and M161045). These studies were also reviewed by the Rahima Moosa Mother and Child Hospital (RMMCH) research review committee, the Gauteng Department of Health, and the Johannesburg Health District's District Research Committee. Further ethical approval was granted for this sub-study by the North-West University HREC (NWU-00455-19-S1 for this study). All participants gave written informed consent.
A venous blood sample was collected into trace element-free ethylenediaminetetraacetic acid (EDTA)-coated vacutainer tubes via venepuncture of the antecubital area of the arm. Blood samples were processed by centrifugation (at 2,000 rpm for 15 min) within 1 h after blood collection to separate plasma, red blood cells and buffy coat. The buffy coat was aliquoted and stored in a 1:1 vol: vol RNAlater (Ambion, Thermo Fischer Scientific) on-site at −20°C for a maximum of seven days. The samples were then transported on dry ice to the Centre of Excellence for Nutrition (CEN) laboratories in Potchefstroom, South Africa and stored at −80°C for genomic DNA (gDNA) isolation.
Genomic DNA (gDNA) was isolated from buffy coat using the Maxwell® 16 instrument and Maxwell® 16 DNA Purification Kit (AS1010) (Promega Corporation) following the manufacturer's instructions. Quantification of gDNA was done using the NanoDrop® ND-1000 UV-Vis Spectrophotometer (Thermo Fischer Scientific).
Massively parallel next-generation sequencing (NGS) was performed using the Ion Torrent platform on the subset of 32 randomly selected samples. A custom Ion Ampliseq panel was designed with the online Ampliseq designer (https://ampliseq.com/, last accessed July 2020). This customized panel included the SLC5A5 gene locus spanning a target region size of 20.83 kbp with 20 amplicons covering 100% of the targeted sequence. Library preparation was performed on the Ion Chef® as per the manufactures specifications. A total of 10 ng gDNA (0.67 ng/μl) was used as input volume for library preparation. Library and template preparation were done with the Ion AmpliSeq™ Kit for Chef DL8 for 32 reactions (Cat number: A29024,) and the Ion 510™ and Ion 520™ and Ion 530™ Kit—Chef (Cat number A34019), respectively. The entire coding region of each selected gene, including flanking regions of introns-exons was sequenced according to 200bp chemistry, using an Ion 530™ Chip Kit-4 Reactions (Cat number: A27763) and the Ion S5™ System (Cat number: A27212, ThermoFisher, MA, USA).
Data analysis of raw reads obtained from the Ion S5™ System was done with the Torrent Suite (v.5.8). The fastq files were uploaded to the sequence reads archive Bioproject PRJNA735618. The primary analysis included signal calling and base-calling. Quality control of the bases was filtered according to a Phred quality score of 20, depth > 10 and quality score of >500 frets were met. The sequence files were aligned against Genome Reference Consortium Human Build 37 (hg19), followed by coverage analysis and variant calling using the coverage analysis and variantCaller plugins from the Torrent Suite, respectively. Secondary data analyses of the variant caller files were annotated, filtered and mined following an in-house pipeline (15).
In the subset of 32 sequenced samples, variants that passed the quality control assessment were considered for validation in the entire sample set using the iPLEX® MassARRAY system from Agena Bioscience™. IPLEX assays were designed and analyses were performed by the service provider Inqaba Biotech (Inqaba Biotechnology Pretoria South Africa). Assays were designed using the Assay Design Suite (ADS) software and dbSNP for metadata. gDNA was amplified in 96 microtiter plates using iPLEX reagent kits and a nano dispenser RS1000 was used to transfer samples from microtiter plates to a SpectroCHIP® array. Data were obtained from the SpectroCHIP® array using the MassARRAY® analyser. Reports were automatically generated by Typer (Company). Genotype calls were made in real-time during MALDI-TOF analysis and data was automatically saved to the MassARRAY database. Variants were assessed for quality, and tested for adherence to Hardy-Weinberg equilibrium (HWE) (16) by using Haploview and modified Pearson chi-square (χ2) test. Adherence to HWE was set at P < 0.001.
From the 98 women participating in the 6-month follow-up, we collected a midstream spot urine sample (10–40 ml) into clean polystyrene cups between 07:00 and 12:00 noon, and approximately 5 ml was decanted into iodine-free screw-capped cups. The research team ensured that the urine samples were not used for any routine assessments using dipsticks (potential contamination with iodine). Samples were aliquoted and stored on-site at −20°C for a maximum of 7 days. Thereafter, samples were transported on dry ice to the CEN laboratories, for storage at −80°C until analysis.
Urinary iodine concentration (UIC) in spot urine samples was measured in duplicate using the Pino modification of the Sandell-Kolthoff reaction with spectrophotometric detection at CEN laboratories (17, 18). All analyses were done using nanopure grade water and all laboratory glassware and plasticware were acid washed before use. Internal and external controls were used to ensure the quality of the analysis. Iodine concentrations in spot urine samples are expressed as median concentrations (μg/L). A UIC cut-off of <100 μg/L was used to indicate insufficient iodine intake in lactating women (19).
From 58 lactating women who participated in the 6-month follow-up, a breast milk (foremilk) sample (≈5 ml) was collected by manual expression into an iodine-free screw-capped cup before feeding the infant. Iodine concentrations in breast milk (in μg/L) were measured using a multi-collector inductively coupled plasma mass spectrometer [MC-ICP-MS (Finnigan NEPTUNE, Thermo Scientific™ Waltham, MA, USA)] as described by Dold et al. (10). A BMIC cut-off of <100 μg/L was used to indicate inadequate maternal iodine intake (20).
Individual UIC and BMIC measures were used to calculate the estimated daily iodine excretion through the urine and breast milk by assuming a total daily urine volume of 1.5 L (12, 21, 22) and breast milk volume of 0.78 L (12, 22). Estimated total daily iodine excretion was calculated as the iodine excretion in urine added to the iodine excretion in breast milk (12). Furthermore, fractional iodine excretion in urine and breast milk as percentages of estimated total daily iodine excretion were also calculated (12, 22).
Raw data were captured in Microsoft Access and 20% of all data were randomly checked for correctness. All genetic, UIC and BMIC, data were captured in Excel Windows XP (Microsoft, Seattle, WA, USA). Data processing and statistical analysis of data were performed using SPSS software (SPSS Inc, Chicago, IL, USA).
Data were tested for normality using the Kolmogorov-Smirnov test. UIC and BMIC data were log-transformed for further analysis. For UIC, values above the cut-off indicative of excessive iodine intake (UIC >500 μg/L) were considered outliers. These UIC outliers (n = 2) were excluded from the analysis because high intakes of iodine have previously been reported to lead to improved BMIC in an individual that harbored a SLC5A5 variant associated with the lower transfer of iodine into breast milk (23). Normally and non-normally distributed data are expressed as means ± standard deviation (SD) and medians (25th percentile, 75th percentile), respectively. Categorical data are expressed as frequencies and percentages.
Participants were stratified according to UIC categories (UIC <100 μg/L and UIC ≥100 μg/L) or BMIC categories (BMIC <100 μg/L and BMIC ≥100 μg/L). The between-group analyses were performed using the Mann Whitney U test. Overlaid scatterplots were used to depict the relationship between total daily iodine excretion, fractional iodine excretion in breast milk and fractional iodine excretion in urine. Unadjusted general linear models were performed to compare UIC and BMIC between genetic variants with the recessive genetic model (GG vs. GA + AA) as categorical variables. For the significant models, effect sizes were calculated using Cohens' d and partial eta squared. Significance was set at p < 0.05.
Genomic DNA was isolated from 246 samples and the SLC5A5 gene of 32 randomly selected samples were sequenced following a targeted gene sequencing approach. Variants were quality controlled and 27 genetic variations passed the quality control assessment. Of the 27 variants, 26 had known annotation and one was novel located in the 5' untranslated region (UTR). Of the annotated variants, six were coding and 13 were in intronic regions, whereas the remaining seven variants were located in the 5' UTR (Table 1). Variants were inspected for possible functionality based on genomic position as well as association with regulatory sites and or available literature. Ten variants (rs121909177(C/T), rs4808708(G/A), rs7255301(G/A), rs73520743(A/C), rs112076606(A/G), rs73520745(G/A), rs775249401(G/A), rs34850953(G/T), rs8103545(C/T) and the novel variant) were validated in all 246 samples (see Table 2). All variants adhered to Hardy-Weinberg Equilibrium (HWE). The minor allele frequencies (MAF) of the variants studied in the lactating women (n = 55) compared well with the MAF of the total group. In the total group of pregnant women (n = 246), none of the women were homozygous for the alternative alleles for rs121909177(C/T), rs73520745(G/A), rs73520743(A/C), and rs8103545(C/T). In addition, the lactating women had no homozygosity for the alternative alleles of rs7255301(G/A) and rs34850953(G/T) variants too. The total study sample (n = 246) was monomorphic for the CC genotype of the novel variant (19: 17983034) and the lactating women all harbored the CC genotype for rs121909177(C/T) (Table 2).
Table 2. Molecular characteristics of SLC5A5 genetic variants among pregnant women (n = 264) and the subset of lactating women (n = 55).
A total of 246 women from the NuPED study consented to participate in the genetic study of which 98 mothers and their infants were assessed at 6-months postpartum. Of these, 58 women indicated to breastfeed their infants and provided a breastmilk sample. Characteristics of the women who were lactating (n = 58) and non-lactating (n = 40) at 6 months postpartum are given in Table 3. The two groups were similar in age, height, weight, BMI, MUAC and UIC (Table 3). The median UIC of all women (n = 98) was 104.7 (66.2–154.0) μg/L, whereas the lactating and non-lactating women had a median UIC of 95 (64–146) μg/L and 122 (73–207) μg/L, respectively (P = 0.259). The lactating women had a median BMIC of 99.4 (60.1–167.9) μg/L. Of the lactating women, 53% had a UIC <100 μg/L and 51% had a median BMIC <100 μg/L. Lactating women had a total estimated iodine excretion of 227.2 (167.4–346.7) μg/d, with 36% iodine excreted in breast milk and 64% in urine.
An unadjusted general linear model was applied to study the associations of BMIC and UIC in the subset of lactating women (n = 55) with the recessive genetic model for rs4808708(G/A), rs7255301(G/A), rs73520743(A/C), rs112076606(A/G), rs73520745(G/A), rs775249401(G/A); rs34850953(G/T) and rs8103545(C/T) (Table 4). There was a significant difference in UIC between the genotypes of the rs4808708(G/A) variant (P = 0.05). Homozygote rs4808708(GG) had higher UIC compared to (A)-allele carriers rs4808708(AG+AA) (P = 0.05), whereas BMIC were comparable between the two genotypes (P = 0.612) for the same variant. BMIC were different between the rs775249401(G/A) genotypes, whereby the homozygous GG genotype had lower BMIC compared to the (A)-allele carriers rs775249401(AG+AA) (P = 0.042). No difference in UIC were apparent for rs775249401(G/A) genotypes. The variant rs112076606(A/G) showed a trend toward a significant association with both BMIC and UIC (P = 0.051 and P = 0.081). The homozygotes AA genotype had higher median concentrations compared to the G-allele carriers rs112076606(GA+GG).
Table 4. Association of SLC5A5 variants with breast milk iodine concentrations and urinary iodine concentrations (n = 55).
Furthermore, the estimated breast milk iodine excretion for rs775249401(GG) was 67.7 (42.9–131.1) μg/d, while estimated breast milk iodine excretion of the (A)-allele carriers of rs775249401(AG+AA) was 112.2 (95.5–132.1) μg/d. All the (A)-allele carriers (100%) of rs775249401(AG+AA) had a BMIC above 100 μg/L, whereas 39.5% the homozygous GG [rs775249401(GG)] had a BMIC ≥100 μg/L. Figure 1 shows the associations between estimated total daily iodine excretion and fractional excretion in urine and breast milk for rs775249401(G/A). The homozygotes GG genotype had a higher fraction of iodine excreted in urine (63.2%) than in breast milk (36.8%) (Figure 1A). The fractional excretion of iodine in urine and breast milk remained constant across the range of estimated total daily iodine excretion. In the (A)-allele carriers rs775249401(AG+AA), fractional iodine excretion in urine and breast milk plotted against total daily iodine excretion (Figure 1B) shows that a higher fraction of iodine was excreted in breast milk than urine when estimated total daily iodine excretion was lower than 350 μg/day, while the fraction of iodine excreted in breast milk was lower than urine above this threshold.
Figure 1. Association between estimated daily iodine excretion and fractional iodine excretion in breast milk and urine for (A) the homozygous G group (n = 43) and (B) the (A) allele carriers (AA + AG) (n = 10). The open data points represent fractional excretion of iodine in urine and the filled data points, the fractional excretion in breast milk. The dotted line is the regression line for fractional excretion of iodine in urine. The solid line is the regression line for fractional excretion of iodine in breast milk.
Figure 2 shows the association between estimated total daily iodine excretion and fractional excretion in urine and breast milk in homozygotes GG (rs775249401(GG)) and in the (A)-allele carriers of rs775249401(AG+AA), stratified by UIC (UIC <100 μg/L vs. UIC≥100 μg/L). The fractional excretion of iodine in breast milk for rs775249401(GG) with UIC <100 μg/L was lower than in urine when estimated total daily iodine excretion was low, but higher with higher estimated total daily iodine excretion and exceeded the proportion of iodine excreted in urine (Figure 2A). In contrast, fractional excretion of iodine in breast milk of the(A)-allele carriers with UIC <100 μg/L, was higher than in urine when the estimated total daily iodine excretion was low, but lower with a higher estimated total daily iodine excretion (Figure 2B). In both the rs775249401(GG) and rs775249401(AG+AA) genotype groups with UIC ≥100 μg/L, fractional excretion of iodine in breast milk was lower than fractional excretion of iodine into the urine (Figures 2C,D, respectively) and remained constant across estimated total daily iodine excretion. The association between rs775249401(G/A) and BMIC had a Cohens' d of 0.88 and a partial eta squared η2 = 0.078.
Figure 2. Association between estimated daily iodine and fractional iodine excretion in breast milk and urine (A) the homozygous G group (rs775249401(GG)) with UIC <100 μg/L (n = 21), (B) the (A) allele carriers for the rs775249401(AG+AA) with UIC <100 μg/L (n = 6), (C) the homozygous G group (rs775249401(GG)) with UIC ≥100 μg/L (n = 22) and (D) the (A) allele carriers for the rs775249401(AG+AA) with UIC ≥100 μg/L (n = 4). The open data points represent fractional excretion of iodine in urine and the filled data points, the fractional excretion in breast milk. The dotted line is the regression line for fractional excretion of iodine in urine. The solid line is the regression line for fractional excretion of iodine in breast milk.
To our knowledge, this is the first study to assess the role of genetics in the transfer of iodine from plasma to breast milk in healthy lactating women of African descent using a targeted NGS approach. Genetic variants in the SLC5A5 gene in women of African descent living in urban South Africa were characterized and studied in the context of iodine transfer from plasma to breast milk during lactation. Our results suggest rs775249401(G/A) to be a candidate variant for mediating the transfer of iodine from plasma into breast milk, specifically among lactating women with poor iodine status. The function of the variant may not be observed when the iodine status is adequate, suggesting that adequate iodine intake may counter the low iodine transfer associated with rs775249401(GG).
We observed that in individuals harboring the rs775249401(GG) genotype fractional excretion of iodine into the breast milk was lower than fractional excretion of iodine into urine with low iodine status, while in the rs775249401(AG+AA) group fractional excretion of iodine into the breast milk was higher even when iodine status was low. Thus, lactating women carrying an (A) allele for rs775249401(AG+AA) (19%; 10/53) had an adaptive advantage to maintain optimal levels of BMIC despite suboptimal iodine status. Our findings suggest a positive genetic drift from the ancestral rs775249401(GG) to the alternative allele to rs775249401(AG+AA), which leads to preferential excretion of iodine into the breast milk when iodine status is low. This preferential excretion of iodine in breast milk rather than in urine is likely a result of an increased expression of the NIS in the lactating breast and further supports the plausibility of a regulatory potential by the rs775249401(G/A).
The rs775249401(G/A) variant is located in a promoter region between exon 4 and 5 (24), which is responsible for transcriptional control. It is speculated that the variant interferes with the affinity of transcription factors (TFs) to bind to their idyllic binding sites (25), thus altering affinity to transcription factors (25). The adaptation observed in our study suggests a physiological benefit for the infant, in that it ensures sufficient iodine in breast milk, especially during periods of low iodine intakes. Furthermore, the rs775249401(G/A) variant is mainly present in African and not in Caucasian populations according to the Genome Aggregation Database (26). The variant rs775249401(G/A) has a minor allele frequency (MAF) of 0.028 in this study population, which is higher than reported in the genome-wide association study for Africa populations (0.015) (24). A total of 14 missense and non-sense mutations in the SLC5A5 gene have been previously described in association with iodide transport defects in the thyroid (27). However, a better understanding of the impact that genetic variations in the SLC5A5 gene have on BMIC is as important.
Out of the 10 variants explored in our study, only rs775249401 was significantly associated with BMIC. One other variant, rs4808708(G/A), was associated with UIC but not BMIC. The variant rs112076606(A/G) showed a trend toward significance with both BMIC and UIC, whereby participants with the genotype rs112076606(AA) had a higher BMIC and UIC compared to rs112076606 (AG+GG) carriers, suggesting AA homozygotes to have a higher overall iodine excretion compared to their G allele carrier counterparts.
To our knowledge, this is the first study to assess the role of genetics in the transfer of iodine from plasma to breast milk in healthy lactating women. Furthermore, our participants were healthy participants with no known history of thyroid disease. However, a major limitation of this study is the small sample size; based on an a posteriori sample size calculation, our study was only powered to determine associations of medium to large effect sizes. Thus, exploring the relationship of variants in the SLC5A5 gene locus in other studies with larger sample sizes is highly recommended.
Our results indicate that genetics may play an important role in the transfer of iodine into breast milk. The SLC5A5 gene variant rs775249401(G/A) seems to be a candidate variant for further investigation. The A-allele carriers of rs775249401(AG+AA) are likely to have higher iodine transfer into breast milk when in an iodine-deficient state compared to the homozygous GG group. Our results suggest that genetic variations in the SLC5A5 gene may play an important role in the transfer of iodine from plasma into breast milk and may partially explain variability in BMIC independent of maternal iodine intake. Therefore, these findings could contribute toward the body of evidence to improve precision nutrition strategies.
The data generated for the study are deposited in the sequence read archive (SRA), bioproject number (PRJNA735618), https://www.ncbi.nlm.nih.gov/bioproject/PRJNA735618.
This study was conducted according to the guidelines laid down in the Declaration of Helsinki, and all procedures involving research study participants were approved by the Human Research Ethics Committee of the North-West University and the University of the Witwatersrand, Johannesburg. Permission to perform the NuPED study was given by the CEO of RMMCH, the RMMCH research review committee, the Gauteng Department of Health and the Johannesburg Health District's District Research Committee. Written informed consent was obtained from all participants before enrolment. Consent for genetic testing were obtained.
LZ, JB, JN, and SS conceptualized and designed the study and wrote the first draft of the manuscript. SS, JB, LZ, EAS, LM, and CMS executed the study and collected data. LZ, MS, JB, and SS performed biochemical and statistical analyses. All authors critically evaluate the manuscript.
The NuPED study was supported in part by the National Research Foundation of South Africa, Unique Grant No. 99374 (EAS) (https://www.nrf.ac.za/) and the South African Medical Research Council under a Self-Initiated Research Grant (CMS) (http://www.mrc.ac.za/). The NuPED study has received further financial support from the University of South Africa's Research Department for the grant Academic Qualification Improvement Programme (EAS) (www.unisa.ac.za). This sub-study was funded by the South Africa Sugar Association (Project 254) and ETH, Zurich.
The authors declare that the research was conducted in the absence of any commercial or financial relationships that could be construed as a potential conflict of interest.
All claims expressed in this article are solely those of the authors and do not necessarily represent those of their affiliated organizations, or those of the publisher, the editors and the reviewers. Any product that may be evaluated in this article, or claim that may be made by its manufacturer, is not guaranteed or endorsed by the publisher.
We would like to thank Dr. Amy Wise, Prof. A. Coovadia, Prof. H. Lombaard, Dr. E. Loock, Dr. R. Adams, Dr. R. Strehlau, Prof. M. Faber, Dr. O. Sotunde, Dr. M. Rothman, Dr. L. Siziba, and Mrs. C. Cooke, the sonographers and nurses of Rahima Moosa Mother and Child Hospital, the nurses at the primary healthcare clinics, the fieldworkers, and the study participants. We like to thank Prof. F. H. van der Westhuizen and Prof. A. A. van Dijk for the use of the IonTorrent sequencing facility, Biochemistry, North-West University.
1. Smanik PA, Liu Q, Furminger TL, Ryu K, Xing S, Mazzaferri EL, et al. Cloning of the human sodium iodide symporter. Biochem Biophys Res Commun. (1996) 226:339–45. doi: 10.1006/bbrc.1996.1358
2. Darrouzet E, Lindenthal S, Marcellin D, Pellequer J-L, Pourcher T. The sodium/iodide symporter: state of the art of its molecular characterization. Biochim Biophys Acta. (2014) 1838:244–53. doi: 10.1016/j.bbamem.2013.08.013
3. Tazebay UH, Wapnir IL, Levy O, Dohan O, Zuckier LS, Zhao QH, et al. The mammary gland iodide transporter is expressed during lactation and in breast cancer. Nat. Med. (2000) 6:871–8. doi: 10.1038/78630
4. Mulrine HM, Skeaff SA, Ferguson EL, Gray AR, Valeix P. Breast-milk iodine concentration declines over the first 6 mo postpartum in iodine-deficient women. Am J Clin Nutr. (2010) 92:849–56. doi: 10.3945/ajcn.2010.29630
5. Bouhouch RR, Bouhouch S, Cherkaoui M, Aboussad A, Stinca S, Haldimann M, et al. Direct iodine supplementation of infants versus supplementation of their breastfeeding mothers: a double-blind, randomised, placebo-controlled trial. Lancet Diabetes Endocrino. (2014) 2:197–209. doi: 10.1016/S2213-8587(13)70155-4
6. Dold S, Zimmermann MB, Baumgartner J, Davaz T, Galetti V, Braegger C, et al. A dose-response crossover iodine balance study to determine iodine requirements in early infancy. Am J Clin Nutr. (2016) 104:620–8. doi: 10.3945/ajcn.116.134049
7. Azizi F, Smyth P. Breastfeeding and maternal and infant iodine nutrition. Clin Endocrinol. (2009) 70:803–9. doi: 10.1111/j.1365-2265.2008.03442.x
8. Dror DK, Allen LH. Iodine in human milk: a systematic review. Adv Nutr. (2018) 9:347S−57S. doi: 10.1093/advances/nmy020
9. Semba RD, Delange F. Iodine in human milk: perspectives for infant health. Nutr Rev. (2001) 59:269–78. doi: 10.1111/j.1753-4887.2001.tb05512.x
10. Dold S, Baumgartner J, Zeder C, Krzystek A, Osei J, Haldimann M, et al. Optimization of a new mass spectrometry method for measurement of breast milk iodine concentrations (BMIC) and an assessment of the effect of analytic method and timing of within-feed sample collection on BMIC. Thyroid. (2015) 26:287–95. doi: 10.1089/thy.2015.0317
11. Osei J, Andersson M, Van Der Reijden O, Dold S, Smuts C, Baumgartner J. Breast milk iodine concentrations, iodine status and thyroid function of breastfed infants aged 2-4 months and their mothers residing in a South African township. J Clin Res Pediatr Endocrinol. (2016) 8:381–91. doi: 10.4274/jcrpe.2720
12. Dold S, Zimmermann MB, Aboussad A, Cherkaoui M, Jia Q, Jukic T, et al. Breast milk iodine concentration is a more accurate biomarker of iodine status than urinary iodine concentration in exclusively breastfeeding women, 2. J Nutr. (2017) 147:528–37. doi: 10.3945/jn.116.242560
13. Nazeri P, Dalili H, Mehrabi Y, Hedayati M, Mirmiran P, Azizi F. Breast milk iodine concentration rather than maternal urinary iodine is a reliable indicator for monitoring iodine status of breastfed neonates. Biol Trace Elem Res. (2018) 185:71–7. doi: 10.1007/s12011-018-1246-9
14. Symington EA, Baumgartner J, Malan L, Zandberg L, Ricci C, Smuts CM. Nutrition during pregnancy and early development (NuPED) in urban South Africa: a study protocol for a prospective cohort. BMC Pregnancy Childbirth. (2018) 18:308. doi: 10.1186/s12884-018-1943-6
15. Schoonen M, Seyffert A, Van Der Westhuizen FH, Smuts I. A bioinformatics pipeline for rare genetic diseases in South African patients. S Afr J Sci. (2019) 115:1–3. doi: 10.17159/sajs.2019/4876
16. Hardy GH. Mendelian proportions in a mixed population. Science. (1908) 28:49–50. doi: 10.1126/science.28.706.49
17. Pino S, Fang SL, Braverman LE. Ammonium persulfate: a safe alternative oxidizing reagent for measuring urinary iodine. Clin Chem. (1996) 42:239–43. doi: 10.1093/clinchem/42.2.239
18. Jooste PL, Strydom E. Methods for determination of iodine in urine and salt. Best Pract Res Clin Endocrinol Metab. (2010) 24:77–88. doi: 10.1016/j.beem.2009.08.006
19. WHO (World Health Organization). Urinary iodine concentrations for determining iodine status in populations. Geneva: World Health Organization (2013).
20. Jorgensen A, O'leary P, James I, Skeaff S, Sherriff J. Assessment of breast milk iodine concentrations in lactating women in Western Australia. Nutrients. (2016) 8:699. doi: 10.3390/nu8110699
21. Kirk AB, Kroll M, Dyke JV, Ohira S, Dias RA, Dasgupta PK. Perchlorate, iodine supplements, iodized salt and breast milk iodine content. Sci Total Environ. (2012) 420:73–8. doi: 10.1016/j.scitotenv.2012.01.045
22. IOM (Institute of Medicine). Dietary reference intakes for vitamin A, vitamin K, arsenic, boron, chromium, copper, iodine, iron, manganese, molybdenum, nickel, silicon, vanadium and zinc. Washington DC: National Academy Press (2001).
23. Mizokami T, Fukata S, Hishinuma A, Kogai T, Hamada K, Maruta T, et al. Iodide transport defect and breast milk iodine. Eur Thyroid J. (2016) 5:145–8. doi: 10.1159/000446496
24. Cunningham F, Achuthan P, Akanni W, Allen J, Amode MR, Armean IM, et al. Ensembl 2019. Nucleic Acids Res. (2019) 47:D745–51.
25. Suzuki Y, Tsunoda T, Sese J, Taira H, Mizushima-Sugano J, Hata H, et al. Identification and characterization of the potential promoter regions of 1031 kinds of human genes. Genome Res. (2001) 11:677–84. doi: 10.1101/gr.GR-1640R
26. Karczewski KJ, Francioli LC, Tiao G, Cummings BB, Alföldi J, Wang Q, et al. Variation across 141,456 human exomes and genomes reveals the spectrum of loss-of-function intolerance across human protein-coding genes. Nature. (2020) 581:434–43. doi: 10.1038/s41586-020-2308-7
Keywords: breast milk, iodine concentration, lactating women, SLC5A5 gene, sodium iodide symporter, urinary iodine concentration
Citation: Siro SS, Baumgartner J, Schoonen M, Ngounda J, Malan L, Symington EA, Smuts CM and Zandberg L (2021) Characterization of Genetic Variants in the SLC5A5 Gene and Associations With Breast Milk Iodine Concentration in Lactating Women of African Descent: The NUPED Study. Front. Nutr. 8:692504. doi: 10.3389/fnut.2021.692504
Received: 08 April 2021; Accepted: 22 June 2021;
Published: 23 July 2021.
Edited by:
L. Joseph Su, University of Arkansas for Medical Sciences, United StatesReviewed by:
Bibiana Garcia-Bailo, University of Toronto, CanadaCopyright © 2021 Siro, Baumgartner, Schoonen, Ngounda, Malan, Symington, Smuts and Zandberg. This is an open-access article distributed under the terms of the Creative Commons Attribution License (CC BY). The use, distribution or reproduction in other forums is permitted, provided the original author(s) and the copyright owner(s) are credited and that the original publication in this journal is cited, in accordance with accepted academic practice. No use, distribution or reproduction is permitted which does not comply with these terms.
*Correspondence: Lizelle Zandberg, TGl6ZWxsZS5aYW5kYmVyZ0Bud3UuYWMuemE=
Disclaimer: All claims expressed in this article are solely those of the authors and do not necessarily represent those of their affiliated organizations, or those of the publisher, the editors and the reviewers. Any product that may be evaluated in this article or claim that may be made by its manufacturer is not guaranteed or endorsed by the publisher.
Research integrity at Frontiers
Learn more about the work of our research integrity team to safeguard the quality of each article we publish.