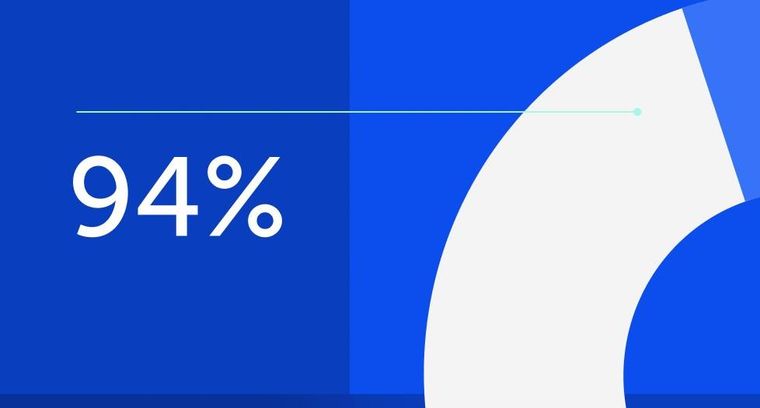
94% of researchers rate our articles as excellent or good
Learn more about the work of our research integrity team to safeguard the quality of each article we publish.
Find out more
REVIEW article
Front. Nutr., 04 June 2021
Sec. Nutrition and Metabolism
Volume 8 - 2021 | https://doi.org/10.3389/fnut.2021.685317
This article is part of the Research TopicSelenium, Human Health and Chronic DiseaseView all 13 articles
This review covers current knowledge of selenium in the dietary intake, its bioavailability, metabolism, functions, biomarkers, supplementation and toxicity, as well as its relationship with diseases and gut microbiota specifically on the symbiotic relationship between gut microflora and selenium status. Selenium is essential for the maintenance of the immune system, conversion of thyroid hormones, protection against the harmful action of heavy metals and xenobiotics as well as for the reduction of the risk of chronic diseases. Selenium is able to balance the microbial flora avoiding health damage associated with dysbiosis. Experimental studies have shown that inorganic and organic selenocompounds are metabolized to selenomethionine and incorporated by bacteria from the gut microflora, therefore highlighting their role in improving the bioavailability of selenocompounds. Dietary selenium can affect the gut microbial colonization, which in turn influences the host's selenium status and expression of selenoproteoma. Selenium deficiency may result in a phenotype of gut microbiota that is more susceptible to cancer, thyroid dysfunctions, inflammatory bowel disease, and cardiovascular disorders. Although the host and gut microbiota benefit each other from their symbiotic relationship, they may become competitors if the supply of micronutrients is limited. Intestinal bacteria can remove selenium from the host resulting in two to three times lower levels of host's selenoproteins under selenium-limiting conditions. There are still gaps in whether these consequences are unfavorable to humans and animals or whether the daily intake of selenium is also adapted to meet the needs of the bacteria.
The organic forms of Se are found as a sulfur amino acid analog, selenomethionine (SeMet), selenocysteine (SeCys), and as methylated derivatives. The inorganic forms correspond to Se salts such as selenate (Se) and selenite (Se) (1). SeMet is found in plant- and animal-origin products as well as in some food supplements (2). On the other hand, SeCys is found primarily in animal-derived food (3), whereas selenium-methylselenocysteine (SeMeCys) is a natural monomethylated organic Se found in some vegetables such as garlic, onion, broccoli, and leeks (2–4). Among the inorganic forms, selenite is present mainly in food supplements, while selenate is found in plant and fish sources (3). These forms of Se have been used to biofortify some vegetables (5, 6).
Brazil nuts, cereals, meat, fish, seafood, milk, and nuts are the best sources of Se (7) (Figure 1). The interaction of fish and seafood with mercury results in insoluble Se derivatives that can reduce its bioavailability (8). In fact, the bioavailability of Se depends primarily on its chemical form. In general, the organic forms are more quickly absorbed and are usually used for the biosynthesis of selenoproteins (9). In addition, the amount of protein, fat, and heavy metal in the diet influence the bioavailability of Se (7–10). High levels of Se are present in some herbal plants such as Astragalus bisulcatus and Brassicaceae (broccoli) (11). Bertholletia excelsa, known as Brazil nut, is one of the highest sources of Se with concentrations that range from 1.80 to 320.80 μg Se/g (12). In addition, the content of Se in the soil has a major influence on the amount of this metal in food, being related to its deficiency and toxicity in some regions. The Se content in the soil usually ranges from 1 to 1.5 μg Se/g, reaching 5.0 μg Se/g in seleniferous soils (13) (Figure 2).
Figure 1. Foods rich in Se with their relative proportions of SeMet, SeCys, SeMeCys, selenate, selenite, and γ-GluMeSeCys. The figure shows the predominance of SeMet in Brazil nuts, wheat grain, fish cod, and chicken (breast). Lamb meat (kidney) is rich in Se mainly in the SeCys form, whereas onion has Se almost exclusively in the form of selenate. Garlic, potato, and broccoli have a balanced proportion of the various forms of Se. Se, selenium; SeMet, selenomethionine; SeCys, selenocysteine; SeMeCys, selenium-methylselenocysteine; γ-GluMeSeCys, γ-glutamyl-Se-methyl-selenocysteine (Figure illustration by Francisco Irochima Pinheiro).
Figure 2. Main countries with documented Se-rich soils. The presence of Se-rich soil is not uniform in the world, but some countries such as Egypt, China, India, Norway, and USA stand out among the others. On the other hand, some plants are more capable of retaining Se, for example - Bertholletia excelsa (Brazil nuts), which is a typical tree of the northern region of Brazil. Se, selenium; SeMet, selenomethionine; SeCys, selenocysteine (Figure illustration by Francisco Irochima Pinheiro).
Dietary Se intake from either organic or inorganic origin is absorbed in the gastrointestinal tract and subsequently transported to the liver, where it is metabolized and used for producing selenoproteins, followed by its distribution to other tissues of the body. Selenoamino acids are actively transported in the duodenum, cecum, and colon through various membrane transport mechanisms, whereas selenate is transported by anion exchangers from the family of the SLC26 gene. On the other hand, there is insufficient evidence on the transport of the other forms of Se (14). Se absorption, metabolism and body distribution are represented in Figure 3.
Figure 3. Se absorption, metabolism, and distribution. After eating Se-rich foods in its organic and/or inorganic form, Se absorption occurs in the duodenum, cecum, and colon. In enterocytes, SeMet and SeCys are absorbed by active transport (systems B0 and b0 + rBAT) while selenate is absorbed by passive transport (anion changers of the SLC26 gene family). After absorption, all forms of Se are converted to H2Se through reactions that occur in the enterocyte and transported in the blood bound LDL, VLDL (mainly). In the liver, H2Se is converted to SePhp and incorporated into selenoproteins in the form of SeCys. Transport to other tissues such as testis, kidneys, and brain occurs mainly in the form of SELENOP through receptor-mediated endocytosis - apoE2 and megaline. Se, selenium; SeMet, selenomethionine; SeCys, selenocysteine; H2Se, hydrogen selenide; LDL, low-density lipoprotein; VLDL, Very low-density lipoprotein; SePhp, selenophosphate; SELENOP, selenoprotein P; apoE2, apolipoprotein E receptor 2 (Figure illustration by Francisco Irochima Pinheiro).
Although the metabolic route differs depending of the Se source, all the absorbed Se is converted to hydrogen selenide (H2Se) in the enterocytes before the specific incorporation of selenocysteine takes place in the active site of the selenoproteins (15). SeMet undergoes transulfurization reactions, in which cystathionine beta-synthetase catalyzes the formation of selenocystathionin, being further converted to SeCys by cystathionine gamma-lyase followed by conversion to H2Se by selenocysteine lyase. SeCys, from both food and the SeMet pathway, will also be reduced to H2Se (16, 17). Alternatively, SeMet can also be incorporated non-specifically into proteins, such as albumin and hemoglobin, replacing methionine (3). As for the inorganic forms, selenate is converted to selenite followed by reduction to H2Se by thioredoxin reductase (TXNRD) and thioredoxin, as well as by glutathione to form selenodiglutathione (GS-Se-SG). Glutathione reductase converts the latter to glutathioselenol (GS-SeH) which reacts with glutathione to form H2Se (3, 18–20). On the other hand, SeMeCys and the synthetic Se derivatives selenobetaine, methylseleninic acid, and methylselenocyanate are converted into methylselenol (CH3)SeH through the enzyme cystathione gamma-lyase, followed by demethylation to become H2Se (21, 22).
In view of this cascade of reactions, all H2Se regardless of its origin will be transported in the blood linked to VLDL and LDL fractions as well as to other proteins (albumin and alpha-globulin). In the liver, H2Se is converted to selenophosphate (SePhp) via selenophosphate synthetase (SEPHS), which will be incorporated into selenoproteins in the form of SeCys. For selenocysteine synthesis, the UGA codon (TGA) is used as an initiation codon, requiring a specialized tRNA (ribonucleic acid carrier), which, after several reactions from the seryl-tRNA, provides information in a targeted manner to the ribosomes that translate mRNAs (messenger ribonucleic acid) to selenoproteins (3, 18–20). Se is transported to tissues such as brain, kidneys, and testicles, mainly in the form of selenoprotein P (SELENOP) through endocytosis mediated by apolipoprotein E receptor 2 (apoE2) and megaline (14).
H2Se can also be methylated by thiol-S-methyltransferase before being excreted. The main form of Se excretion is through urine, however, in cases of excessive consumption, respiratory excretion might occur. Excretion by the lungs occurs when the elimination of Se in the form of trimethyl selenonium (CH3)3Se in the urine becomes saturated, whose elimination occurs mainly in the form of volatile dimethyl selenide (CH3)2Se (23). In situations of moderate consumption of Se, the main monomethylated compound eliminated through kidneys is a seleno sugar namely 1β-methylseleneN-acetyl-D-galactosamine. The non-absorbed Se from food is incorporated into the bile, pancreatic, and intestinal secretions, being eliminated in the feces (23).
Se functionality occurs in the form of selenoproteins that are encoded by the insertion of SeCys by the UGA codon in mRNA under specific conditions. Most of these selenoproteins are involved in the regulation of redox signaling and are grouped into families such as glutathione peroxidases (GPXs), iodothyronine deiodinases (DIOs), TXNRDs, and SELENOP. Thus, the main biomedical applications attributed to Se are related to its antioxidant activity, regulation of thyroid hormone metabolism, anticarcinogenic property, and prevention of cardiovascular diseases. SELENOP acts as the main Se transporter for peripheral tissues in addition to performing extracellular antioxidant function (14, 22).
The evaluation of Se status determines the amount of this biologically active nutrient as a function of intake, retention, and metabolism. Thus, the Se status can be assessed at three levels using biomarkers of intake, retention/excretion, and concentration on tissues as well as biomarkers of functionality (22) (Figure 4).
Figure 4. Se biomarkers. The Se status is evaluated with the purpose of quantifying the biologically active or potentially active nutrient as a function of Se intake, retention, and metabolism in the body. The Se status can be assessed at three levels using specific biomarkers: (1) biomarkers of intake (assessment of food consumption through the food frequency questionnaire); (2) biomarkers of retention/excretion and concentration in tissues (urine, feces, nails, hair, and plasma) and (3) biomarkers of selenium functionality (SELENOP and GPX3 in plasma and GPX1 in erythrocytes, lymphocytes, and tissue samples). Se, selenium; SELENOP, selenoprotein P; GPX1, glutationa peroxidase; GPX3, glutationa peroxidase 3 (Figure illustration by Francisco Irochima Pinheiro).
The assessment of Se intake can be performed using methods of assessing food consumption, such as the food frequency questionnaire. The Se content on foods is estimated using food composition tables (22). Algeria 's population consumes a wide variety of Se-rich foods, such as seafood, meat, eggs, milk, and legumes, however, no significant associations were found between dietary patterns and Se biomarkers, such as Se in plasma, SELENOP, and GPX. Predicting the Se status from food consumption remains a challenge due to the lack of precision of nutrient content in food composition tables, considering the variation in the Se concentration of foods as a function of Se content in the soil (22, 24). In addition, Se from the diet affects colonization of the microbial intestine, which in turn influences the host's Se status and selenoproteoma expression (25).
The retention of Se in the body can be assessed by the difference between the amount of Se ingested and the sum of Se in the urine and feces, which requires the collection of total urine and excreted feces for a few days. Alternatively, it is recommended to evaluate the concentration of creatinine in the urine to reduce the error associated with the variation in urinary excretion. Renal excretion is the main route of elimination of absorbed Se (22). Genetic and environmental factors, as well as body size, age, and sex can influence the retention and excretion of Se in the urine (26). However, studies on Se in urine for biological monitoring are scarce, especially with regard to occupational exposure, in which inhalation is the main route of exposure. After inhalation of high concentrations of Se by workers, inflammatory effects were observed in the respiratory tract (27). An increased intake of Se is reflected rapidly in the increased excretion of Se in the urine (28). The evaluation of Se in urine can be a sensitive parameter for occupational exposures of Se in the short term, but the knowledge about specificity and kinetics of this elimination pathway is still little explored (27).
The measurement of the Se concentration in urine is considered as a potentially viable biomarker of Se status in population studies. Additionally, the concentration of Se in the urine can be used to identify regional variations in the status of Se and might reflect differences in the amount of Se in food according to the type of soil. This evidence supports the need for reviewing the policies of national systems for monitoring micronutrient deficiencies including Se (26).
The concentration of Se in the nail is considered a superior biomarker of Se status, as it provides an integrated measurement of long term exposure (up to 1 year), while blood biomarkers indicate a short term exposure (29). Toenails are considered non-invasive matrices and are used in large epidemiological studies because they present slow growth, easy collection and have less influence from external contamination. The standardization of sample collection, quality control, and analytical techniques are important to consolidate the usefulness of this matrix in epidemiological studies (30). The Se content in nails has a direct relationship with SELENOP and most organic forms of Se, especially SeCys, whereas it has an inverse relationship with the amount of the inorganic forms, such as selenite and selenate. This opposite behavior may be related to the composition of human nails, which are mainly made of proteins rich in cysteine, the latter being able to form complex with Se (31).
There are controversies about the use of Se content in nails and hair as a way to assess the effectiveness of Se supplementation. A systematic review performed with 18 Se supplementation studies found no evidence to support the use of the Se content in the nail and hair as a reliable measurement of effectiveness of Se supplementation (32). Se content in hair has been used to assess long-term Se status in epidemiological studies, offering the advantage of being a low-cost method and easy to store the samples. The Se concentration in hair and nails are excretory forms of Se. Therefore, both reflect the previous status, being more useful as biomarkers in studies of populations with stable dietary patterns (22).
Plasma Se concentration is a more useful biomarker to assess Se status in humans, considering the stability of Se in this compartment (22). A systematic review has recommended the use of plasma Se concentration as a reliable biomarker in supplementation studies with adults of both sexes. The measurement of Se in plasma has shown to be effective in reflecting changes in the amount intake (supplementation) in individuals with intermediate or high Se concentrations at baseline. In addition, this review highlights the usefulness of Se in erythrocytes and whole blood as markers of Se status, both of which are reported as markers of long-term status (32).
Biomarkers of Se functionality include SELENOP, which comprises 20–70% of Se in plasma; GPX3, which comprises 10 to 25% of Se in plasma and GPX1, which can be tested on erythrocytes, lymphocytes, oral cells, and tissue biopsy specimens (22). Plasma SELENOP has been considered a useful biomarker of Se status in populations with relatively low Se intake, but not in populations with high intake that already had high levels of Se before supplementation began (32). SELENOP has shown to be a reliable and sensitive Se status biomarker, providing dose response that can be used to estimate the Se intake required to reach its plateau in the plasma (33). It seems that SELENOP reaches a plateau after supplementation with selenite at doses around 400 μg/day (34).
GPX is one of the main selenoproteins that belongs to the cellular antioxidant defense system. The recommended Se intake was calculated based on optimal plasma GPX3 activity due to the hierarchy of selenoproteins. It also considers the necessary amounts of Se for normal concentrations of other biologically Se compounds (35). A cohort study conducted with 51 participants with adequate Se intake investigated the association between plasma Se, GPX activity, and SELENOP. The results were discrepant between plasma Se concentrations and GPX activity, suggesting other factors may impact the activity of this enzyme such as genetic polymorphisms (36, 37).
Se plays a crucial role in normal physiology and contributes to the pathophysiology of various diseases. Due to its antioxidant and anti-inflammatory properties, several studies have evaluated the impact of Se status in conditions characterized by inflammation and oxidative stress, which includes diabetes, metabolic syndrome, cancer, cardiovascular, and neurodegenerative diseases (38).
Inadequate serum Se levels may increase the risk for the development of several diseases, especially cardiovascular disorders, but it also may lead to cancer, liver diseases, and arthropathies. On the other hand, excessive consumption of Se can cause selenosis, which leads to symptoms such as fatigue, tachycardia, nausea, and diarrhea. Chronic selenosis can cause liver and kidney necrosis, neurological disorders and might compromise the reproductive and immune systems (39).
In three large cohorts, the high serum Se concentration was associated with reduced mortality (40). Another larger study, in which more than 13,000 adults were followed for 12 years, revealed that serum Se greater than or equal to 135 μg/L were associated with reduced cancer mortality (41, 42). Meta-analysis involving 16 prospective studies demonstrated an inverse relationship between Se status and cardiovascular risk (43). Likewise, a systematic review with meta-analysis involving 13 studies revealed that high physiological levels of Se are associated with lower incidence and lower mortality from cardiovascular disease (CVD) (44). In another meta-analysis in which more than 40 thousand participants in randomized clinical trials were included, the authors found that Se supplementation decreases the serum levels of C-reactive protein and increases the levels of GPX, suggesting a positive effect on reduction of inflammation and oxidative stress in cardiovascular diseases (45).
Selenium-binding protein 1 (SELENBP1), an intracellular protein involved in Se metabolism and redox control, has been identified as a circulating biomarker for cardiac events in patients with suspected acute coronary syndrome. At the molecular level, it seems that hypoxia acts as a modulator of SELENBP1, therefore reducing the oxidative stress and controlling the lower oxygen supply (46).
Previous studies have shown that circulating Se plays an important role in the pathogenesis of abnormal glucose metabolism, especially at high concentrations (47, 48). High exposure to Se can affect the expression of the main regulators of glycolysis and gluconeogenesis, through actions mediated by the GPX1 (49), as shown in studies that evidenced that the overexpression of this selenoprotein causes insulin resistance (50).
A review study has elucidated the relationship between Se status and cerebral Se homeostasis via SELENOP. In fact, SELENOP may be involved in some brain disorders, in particular in Alzheimer's disease, providing Se for brain tissue to produce selenoproteins. In addition, it competes with amyloid-β for metal ions and redox-active metals, such as copper and iron. This study points out the involvement of SELENOP in signaling pathways in neuronal and glial tissues, including neuronal calcium homeostasis and excitotoxicity (51).
Brazil nuts (Bertholletia excelsa, family Lecythidaceae) are known to be the richest source of Se with high SeMet content and therefore, it has been widely used in studies of Se supplementation. Regular consumption of Brazil nuts results in optimum plasma Se and erythrocytes concentrations, as well as in better activity of selenoenzymes (52–55) antioxidant state (56), muscular retention (57), and inflammation status (54, 58). It is important to consider genetic variants in selenoprotein genes (55) and pre-stratification of the population prior to starting the trials as a way to avoid possible differentiated responses depending on the Se status in each individual (59). Studies on the effects of supplementation with Brazil-nuts on selenium biomarkers are shown in Table 1.
The effect of Brazil nuts on the human intestinal microbiota is still unknown. It is well-known, however, that Brazil nuts contain fiber, unsaturated fatty acids, and polyphenols that may impact the composition of the gut microbiota and overall gut health. A systematic review with meta-analysis including randomized controlled trials on nut consumption investigated the intake of almonds (n = 5 studies), walnuts (n = 3 studies), and pistachios (n = 1 study) and demonstrated a significant increase in the gut content of genera Clostridium, Dialister, Lachnospira, and Roseburia, as well as a significant decrease in Parabacteroides. The nuts did not show any significant influence on bacterial phyla, bacterial diversity or stool output (60). Other studies have only reported an increase in the abundance of butyrate-producing bacteria after nuts (61, 62) and pistachios (63) intake, without demonstrating any effect on the overall composition of the microbiome.
The Nutritional Prevention of Cancer (NPC) trial showed the effectiveness of supplementing 200 μg/day of Se, as selenized yeast, in reducing the risk of prostate, lung, and colorectal cancers (64). Se supplementation was also reported to decrease CVD and related mortalities (65, 66). In a group of healthy New Zealand men, Se supplementation as selenized yeast (Selplex, 200 μg/day) in the form of SeMet, significantly increased Se levels, improved the TXNDR activity and enhanced DNA stability (59). Due to the positive results from the NPC trial, the Selenium and Vitamin E Cancer Prevention Trial (SELECT) was undertaken in 35,000 healthy US men randomly assigned to 4 groups (selenium-−200 mcg/d from L-selenomethionine, vitamin E-−400 IU/d of all rac-alpha-tocopheryl acetate, selenium + vitamin E, and placebo). Neither selenium nor vitamin E, alone or in combination, was able to prevent prostate cancer in this population (67).
Observational studies and randomized clinical trials conducted with high-dose Se supplementation have shown controversy over the effects on diabetes mellitus, indicating that both excess and deficiency of Se may be associated with higher risks of diabetes mellitus type 2 (DM2) (68–71). In a NPC trial, participants were randomly assigned to receive 200 μg/day of Se (as high-selenium yeast). This group were more likely to develop DM2 than those assigned to placebo. In a randomized controlled study involving patients with DM2 and cardiovascular disease, supplementation with 200 μg Se/day resulted in a significant decrease in insulin, HOMA-IR, C-reactive protein and an increase in total antioxidant capacity (70).
Se toxicity can affect individuals as a result of occasional overdose that usually occurs with intake of incorrectly formulated supplements (72) or due to the excess of Se intake in randomized clinical trials, in which doses of 200 μg/day or more are administered for a substantial period of time (73). Acute toxicity from excessive Se exposure causes stomach pain, headache, respiratory symptoms, changes in blood pressure, vomiting, and nausea. Chronic oral intake of high amounts of Se results in selenosis, a condition characterized by hair loss, deformation and loss of nails, tooth discoloration, garlic breath, gastrointestinal disturbances, skin rash, numbness, paralysis, and occasional hemiplegia (74). Other outcomes have been reported such as dermatitis, increased mortality (73), DM2 (68) and increased incidence of prostate cancer (67), which are also observed in Se deficiency.
Increased mortality has been reported at the highest dose of Se in the Danish PRECISE, a randomized, double-blinded, placebo-controlled, clinical trial performed with four groups treated with 100, 200, or 300 μg Se/day as Se-enriched yeast or placebo yeast. The results of this study warn that a 300-μg/day dose of Se (as Se yeast) taken for 5 years in a country with moderately low Se status can increase all-cause mortality by 10 years later (73).
The levels of dietary exposure that is able to induce selenosis and Se toxicity is difficult to establish due to the fact that toxicity is affected by the chemical form of Se and its bioavailability. Furthermore, interactions of Se with other dietary components, the individual's genotype and intestinal microbiota are also factors that influence the Se toxicity. Even in the face of Danish PRECISE, some populations exposed to excess Se did not develop adverse effects. Such conditions suggest that there are mechanisms for genetic adaptation that might be involved in oscillations in the Se intake, which are mediated by polymorphisms, complexation of SELENOP with toxic elements such as cadmium, arsenic, and mercury forming products of Se excretion (75, 76). The metabolism of Se by intestinal bacteria also favors the excretion of excess of Se (41, 77).
The human digestive tract is inhabited by several microorganisms (bacteria, viruses, fungi, and protozoa) named microbiota, that includes ~100 trillion microorganisms (78). Bacterial cells are distributed unevenly along the gastrointestinal tract with more than 50 types of bacterial phylum. Only Bacteroidetes and Firmicutes are preserved in practically all individuals (79).
Human microbial colonization begins at birth and it is similar to the maternal vaginal microbiota. It is believed that intestinal colonization during birth and breastfeeding is essential to define the composition of the intestinal microbiota later in adulthood, although the determination of the composition of the microbiota is also influenced by several external and internal factors related to the host (80).
The microbiome is capable of encoding more than three million genes. It carries out a variety of metabolic functions not attainable by the human host, which includes the production of some types of vitamins and bioactive compounds, the synthesis of essential and non-essential amino acids, the metabolism of non-digestible carbohydrates and the activity in neural, hormonal, and immunological signaling through the gut-brain axis. Furthermore, it acts on the absorption of nutrients and as an epithelial barrier for pathogens (78). In this sense, imbalances in the intestinal ecosystem or in two-way communication with the brain are associated with gastrointestinal disorders, metabolic diseases, and neurobehavioral disorders. Therefore, strategies have been developed to manipulate the microbiome, with the aim of preventing and/or reversing conditions that are harmful to health (81).
Comparative genomics provides a powerful tool for investigating genes, pathways and evolutionary changes across multiple lineages (82). In the past decade, studies have been conducted evaluating the use of Sec Trait in ~600 bacterial and archaeal genomes, in which the organisms rich in selenoproteins were the anaerobic Deltaproteobacteria and Clostridia classes, especially Syntrophobacter fumarroxidans, with the highest prokaryotic selenoproteoma reported (83).
Traces of Se and related key genes have been evaluated in over 2,300 bacterial and archaea genomes, identifying a phylogenetic and genomic mosaic pattern among organisms using Se in different forms. This profile suggests new genes whose encoded proteins participate in Se metabolism and homeostasis in prokaryotes, such as YedE involved in Se transport, YedF which transcribes redox protein and LysR Se known as specific transcriptional Se-regulator (84).
Evolutionary trends in the use of Se and selenoproteins indicate more than 5,200 bacterial genomes, with the majority being related to the host, resulting in the largest Se utilization map in this realm. However, of this total, 2/3 of the bacteria do not use Se, suggesting that this ability has been lost over time. Environmental factors and use of Se were also investigated, revealing that Se-cofactor trait (68%) and Sec Trait (37%) appear to favor the conditions of host-associated bacteria, while SeU trait prefers aquatic species that have been isolated mainly from the sea or freshwater (85). These macro-evolutionary trends extend to cell respiration and temperature characteristics, in which anaerobic conditions can significantly promote the use of the Se-cofactor trait and lead to the evolution of new selenoprotein genes. Temperature seems to affect the use of Se, in which thermophilic (<40°C), mesophilic (20–40°C), and psychrophilic (<20°C) conditions favor the use of Sec Trait, Se-cofactor trait (mostly, but non-significant values) and SeU trait, respectively (85, 86). Thus, the human intestine can be a favorable ecosystem for the use of selenium by prokaryotes as the oxygen level in the colon, which is the site with the highest degree of Se absorption, is low and the optimum temperature varies between 25°C and 30°C (87).
Genetic sequencing data carried out with 1,135 Dutch people detected 126 different environmental factors associated with microbiota, including diet, physical activity, diseases, and use of medicines (88). Specific foods and dietary patterns can influence the abundance of different types of bacteria in the intestine. For instance, the low intake of FODMAPs (Fermentable Oligosaccharides, Disaccharides, Monosaccharides, and Polyols) has been identified as a nutritional therapy indicated for the relief of gastrointestinal symptoms reported by patients with irritable bowel syndrome (IBS) and non-celiac sensitivity to gluten (89). Foods rich in fructans (wheat, rye, garlic, and onion) lactose (milk and dairy products), fructose (fruits and processed foods containing syrups), sorbitol, xylitol red fruits, and mushrooms are fermented by intestinal bacteria (Actinobacteria) and yeasts producing hydrogen and methane gases, resulting in bloating symptoms, abdominal pain, and diarrhea (90). In a meta-analysis study with randomized clinical trials, the low FODMAP diet was beneficial for remission of gastrointestinal symptoms in patients with IBS (91). However, the restriction of several foods may lead to a potential inadequacy of micronutrients in patients who follow this dietary recommendation, resulting in significant changes in the microbiota and metabolome, whose duration and clinical relevance are still unknown (92, 93).
Dietary Se influences both the host's selenium status and selenoproteoma expression. The intestinal microbiota can use the ingested Se for the expression of its own selenoproteins. Se affects the composition and colonization of the gut microbiota, which may interfere with the diversity of the microbiota and cause unique effects on microbial composition. About 1/4 of all bacteria have genes that encode selenoproteins. Some of them, such as Escherichia coli, Clostridia, and Enterobacteria classes, are able to colonize the gastrointestinal tract of humans and animals (94). Selenocysteine synthase (SelA) is a pyridoxal phosphate-dependent enzyme (PLP) (95) which catalyzes the formation of selenocysteinyl-tRNA in bacteria from a UGA decoding tRNASec (SelC) loaded with serine and selenophosphate, the product of the enzyme selenophosphate synthetase (SelD). Along with SelB, a specific translation factor of selenocysteinyl-tRNA, SelA, SelC, and SelD are components of bacterial Sec decoding, allowing the incorporation of Sec into specific UGA codons followed by a sequence of insertion of Sec elements (SECIS) (96).
The composition of the microbiota can also be modulated by metals that participate in microbial growth through respiratory mechanisms, as a source of energy for autotrophic growth, as well as to transfer and storage of electrons between cells (86). Manganese, zinc, selenium, and iron act as critical cofactors for bacterial enzymes responsible for DNA replication and transcription, antioxidant action, and cellular respiration (97). Iron and zinc are the metals used by almost all living organisms in metabolic and oxidation-reduction processes (98). Some species require Se for normal metabolic functions, for instance, Escherichia coli has three selenoproteins in its structure (99).
Selenocompounds are found in animal and plant sources with distinct bioavailability. In experimental models using rats, no differences in nutritional availability were observed between selenite, selenate, selenocyanate (SeCN), SeMeCys, SeMet, selenohomolanthionine (SeHLan), selenocenoine (SeCys2), 1β-methyl-acetyl-D-galactosamine (SeSug1), except for trimethylselenonium ion (TMSe) administered orally. The authors discussed these findings based on mechanisms related to gastrointestinal enzymes that can degrade bioselenocompounds into selenocompounds in the intestine (100).
Germ-free mice that were fed with diets with adequate and high Se levels modified their selenoproteoma expression in a similar way to that of the control group but showed higher levels and activity of GPX1 and methionine-R-sulfoxide reductase 1 (MSRB1) in the liver, suggesting partial sequestration of Se by intestinal microorganisms, therefore resulting in limited availability to the host. In these experiments, the genus Parabacteroides of the phylum Bacteriodetes, showed an opposite correlation with Se dietary supplementation. The study concluded that dietary Se affects both the composition of the gut microflora and the colonization of the gastrointestinal tract (99).
Zhai et al. (101) compared the effects of different levels of Se dietary supplements (deficient, adequate, and supranutritional) on the intestinal microbiota of mice. The animals' fecal microbiota transplantation was performed in one of the experiments. Supplementation conducted with different amounts of Se did not significantly alter the mice's intestinal microbiota. It rather induced significant changes in the composition of the gut microbiota. In comparison to the Se-deficient diet, supranutritional Se supplementation significantly decreased the abundance of Dorea sp. and increased the levels of microbes with potential protective effects against colitis and intestinal barrier dysfunction, such as Turicibacter and Akkermansi. Dorea sp. is one of the most common species of the intestinal microbiota that supplies hydrogen and carbon dioxide in the intestine. The authors concluded that Se supplementation can optimize the intestinal flora to protect against intestinal dysfunction.
Although the host and the intestinal microbiota mutually benefit from a symbiotic relationship, these environments can become competitors when the supply of micronutrients becomes limited. On the other hand, the intestinal microbiota favors the biotransformation of Se compounds, characterizing a dubious situation (Figure 5). The Se uptake by intestinal bacteria can negatively influence the expression of selenoproteins in the host, which results in a two to three times lower levels of selenoproteins under Se limiting conditions. The unfavorable consequences of this effect for humans and animals have not yet been evidenced. In view of the high propagated intake of probiotics, the metabolism of Se in these organisms should be investigated in order to assess whether a higher Se intake is recommended (94).
Figure 5. Modulation of the gut microbiota dependent on Se status and biotransformation of Se derivatives. Given the adequate intake of Se, homeostasis occurs due to the beneficial relationship between intestinal and host bacteria resulting in the biotransformation of Se compounds (Se salts metabolized into SeMet and SeCys). Se deficiency results in increased Se uptake by bacteria (Escherichia coli, Clostridia, and Enterobacteria), biotransformation of Se compounds (Se salts metabolized into SeMet and SeCys), decreased expression of selenoproteins by the host, decreased activation of Se immune cells, increased pro-inflammatory cytokines, and increased risk for IBD and cancer. On the other hand, excessive intake of Se causes increased uptake by bacteria such as Turicibacter, Akkermansi, and Lactic acid bacteria (LAB), biotransformation of Se compounds such as selenite (Se) and selenate (Se) which are metabolized into SeMet and SeCys, and increased excretion of volatile compounds from Se. Se, selenium; SeMet, selenomethionine; SeCys, selenocysteine; IBD, inflammatory bowel diseases (Figure illustration by Francisco Irochima Pinheiro).
A study conducted with animal models indicated that the gut microbiota may affect the status of Se and the expression of selenoproteins. The colonization of germ-free (GF) mice has shown to induce the expression of the gastrointestinal form of several selenoproteins, even under conditions of Se-deficient diet. GF mice showed higher GPX and TXNRD1 activities in the intestine and liver, greater expression of GPX1 in the liver and GPX2 in the proximal and distal jejunum and colon, as well as greater activity of GPX1 and GPX2 in the colon. The study indicated that GF animals have less need for Se for selenoprotein biosynthesis than conventionally colonized animals. In addition, it has been observed that colonized animals have a higher risk for developing selenoprotein deficiency when the supply of Se becomes limited (94).
Another study has demonstrated that several inorganic and organic selenocompounds were metabolized to SeMet by the gut microflora of rats and that SeMet was incorporated into bacterial proteins. Proteins containing SeMet, available as a Se pool for the host animal, were accumulated in the gut microflora. The main urinary selenometabolite, SeSug1, was transformed into a nutritionally available selenocompound by the intestinal microflora. Finally, positive effects on the bioavailability of some bioselenocompounds, such as SeCN, MeSeCys, and SeSug1, were observed in the gut microflora (102).
Some bacterial species are able to benefit from Se by triggering some effects on bacterial pathogenesis. Faced with an infection by this type of bacteria, a complex interaction takes place between the host's immune response, the microbial pathogen, the microbiota, and the host's Se status. Bacteria that have Se-dependent enzymes can survive under anaerobic conditions in the mammalian gut. As a result, these bacteria benefit from the host by using Se to increase its virulence and pathogenicity (103).
Se deficiency can leave the individual immunocompromised, allowing the survival of bacteria that do not need Se to establish an infection and cause disease. The host's microbiota may also differ in the presence of Se, which can prevent infection by Se-dependent bacteria, either by competition for Se or by the production of toxic metabolites that can be harmful to pathogenic bacteria (103).
The role of the intestinal microbiota in the excretion of SeMet and selenite has been investigated in rats. It has been reported that the excretion of excess of SeMet and selenite occurs through the production of methylated derivatives of Se and elemental Se from the biotransformation of L-selenomethionine and selenite (104). Another study corroborates this hypothesis by showing that the gut microflora of rats can metabolize L-SeMet to some metabolites (77).
Bacterial count and protein analysis have shown that the number of cells and protein concentrations in the cecum and colon suspensions of rats are similar, but the cecum microbiota of these animals may contain more metabolically active microorganisms for SeMet and selenite compared to those in the colon microbiota. Given the much larger relative size of the colon in humans, the metabolism of Se compounds in the human intestine is likely to occur mainly in the colon. The formation of these volatile compounds of methylated and elemental Se in the intestinal tract points to the role of the microbiota in protecting the host from toxicity due to high doses of Se supplements (104).
Significant increase in the absorption and distribution of cadmium and lead in the blood, gastrointestinal tract, kidneys, liver, and spleen were seen in germ-free mice exposed to cadmium or lead (5, 20, and 100 ppm) for 6 weeks in comparison to non-exposed animals. Thus, it seems that the microbiota act as a protective factor against heavy metals (105).
The role of Se has also been investigated against methylmercury (MeHg) poisoning though the modulation of gut flora and decomposition of this compound. Treatment with selenite for 90 days of rats poisoned with MeHg showed a modulation of flora abundance, specially Bacteroidetes and Firmicutes phyla. An increase in total mercury (THg) was found in fecal samples after treatment with Se on the 30th day. The percentage of MeHg in the poisoned group was between 81 and 105%, while 65–84% was found in the Se treated group, suggesting an increase in MeHg decomposition after treatment with Se (106).
Se and selenoproteins may play an important role in signaling pathways that are involved in the pathogenesis of some diseases, especially IBD (107), cancer (108), thyroid dysfunction (109), and neurogenerative disorders (110). The Se status may impact the expression of nuclear factor-κB (NF-κB) transcription factors and peroxisome proliferator activated receptor (PPAR) γ, which are involved in immune cell activation that ultimately results in various stages of inflammation (107). Thus, Se deficiency and inadequate selenoprotein expression impair innate and adaptive immune responses, especially at the colonic level where an increase in inflammatory cytokines is observed (25). In addition, low intake of Se might result in a phenotype of the gut microbiota that is more susceptible to colitis and infection by Salmonella typhimurium. On the other hand, a diet with sufficient or high levels of Se can optimize the gut microflora for protection against intestinal dysfunctions and chronic diseases (101).
Crohn's disease and ulcerative colitis are IBD characterized by microbial dysbiosis that result in changes in intestinal motility and secretion, visceral hypersensitivity (hyperalgesia), and failure in the intestinal-brain communication (111). Se deficiency is common among patients with IBD, reaching 30.9% of cases (112). The importance of Se in improving IBD is attributed to the ability of the selenoproteins in reducing the inflammatory response (113, 114).
The nuclear factor erythroid factor 2-related factor 2 (Nrf2) also appears to contribute to redox homeostasis in epithelial cells (115). In a study conducted using an animal model of IBD, the lack of Nrf2 led to increased expression of inflammatory cytokines, such as TNFα and IL6 and increased expression of COX2 (116). Nrf2 can also stimulate the expression of TXNRD and GPX under adequate concentrations of Se (117). This relationship was explored in another study that found a positive association between plasma concentration of Se and the expression of Nrf2-related genes (118). In addition, a study showed that the lack of Nrf2 increases NF-?B activity, further intensifying oxidative stress (119).
Zhu et al. (120) investigated the protective effect of Se nanoparticles with Ulva lactuca polysaccharide (ULP-SeNPs) on DSS-induced acute colitis in mice. The main benefits were the reduction of CD68 in the colon, modulation of IL-6 and TNF-α, inactivation of macrophages and suppression of nuclear translocation of NF-κB.
Bacteria with pro-inflammatory activity, such as Escherichia and Fusobacterium, are increased in patients with IBD, whereas anti-inflammatory species such as Faecalibacterium, Roseburia Clostridium coccoides, Clostridium leptum, prausnitzii, and Bifidobacterium are reduced in this disease (121, 122). Other phyla of bacteria have been associated with the ingestion of Se in individuals with IBD. Dietary Se was positively correlated with the presence of Firmicutes and negatively correlated with Verrucomicrobia in patients with Crohn's disease and ulcerative colitis, respectively (123).
Animals treated with SeCys and selenocystine showed a reduction in the concentration of ROS and malondialdehyde (MDA), as well as an increase in intestinal activity of SOD and GPX, which seems to indicate a protective effect against damage to the gut mucosa. In addition, the levels of IL-1, MCP, IL-6, and TNF-α were significantly reduced in the group treated with SeCys (124).
Xu et al. (125) reported that the administration of Se nanoparticles with Lactobacillus casei ATCC 393 (L. casei 393-SeNPs) protected mice from intestinal barrier dysfunction and oxidative stress associated with enterotoxigenic Escherichia coli infection K88 (ETEC K88), when compared to the animals supplemented with L. casei alone. These findings suggest the ability of L. casei 393-SeNPs in maintaining intestinal epithelial integrity.
The specific link between gut microbiota, selenium status, and cancer is difficult to establish, and multiple mechanisms may be involved in the complex interplay between microbiome, diet, and human host. It has been demonstrated that dietary Se affects both composition of the intestinal microbiota and colonization of the gastrointestinal tract, which, in turn, influence the host Se status and selenoproteome expression (99). The effect of the gut microbiota on selenoproteins and other molecules linked to redox homeostasis and those linked to the WNT/β-catenin signaling pathway may have an impact on the regulation of oxidative stress, apoptosis, inflammation, and immune response, suggesting a direct influence on increased risk of cancer (108).
Considering that Se uptake by intestinal microbiota occurs in conditions of imbalance, it might negatively impact the supply of Se to the host, therefore predisposing to cancer and gut dysfunctions. Deficiency of selenoproteins and molecules linked to redox homeostasis can lead to a gut microbiota phenotype that is more vulnerable to colitis, pathogen infections, and cancer (101). Lower expression of different selenoproteins have been described in colorectal adenomas and cancer tissues, while higher SELENOP concentrations were inversely associated with colorectal cancer risk (126).
Bacteria of the Dorea sp. genus, one of the most common species of the gut microbiota, are increased in conditions of deficiency of Se (101) and are associated with IBS, cancer, multiple sclerosis, and non-alcoholic liver disease (101, 127–130). Se deficiency and inadequate selenoprotein expression impairs innate and adaptive immune responses with higher levels of inflammatory cytokines, especially at colonic level. The effect of the gut microbiota on selenoproteins and other molecules linked to the redox homeostasis may have an impact in the regulation of oxidative stress, apoptosis, inflammation, and immune response, which appears to have a direct influence on cancer risk and development (108, 131).
On the other hand, the administration of probiotics enriched with organic Se seems to by a promising alternative for elimination of pathogenic bacteria in the case of IBD and colon cancer (132). Likewise, Porto et al. (133) showed that oral administration of Saccharomyces cerevisiae enriched with Se reduced eosinophil peroxidase activity, histopathological tissue damage and oxidative stress (lipid peroxidation and nitrite production) in the small intestine of mice. Therefore, clinical studies involving the biological function and bioaccessibility/bioavailability/bioactivity of selenoproteins and selenometabolites in different functional foods enriched with Se and nutraceuticals are highly recommended in order to confirm the findings of preclinical studies.
The thyroid gland contains the highest amount of Se per mg of tissue in the body. Several proteins involved in thyroid metabolism contain Se, namely GPX (type I and II), DIOs, and TXNRD. Resident microbes of the colon metabolize Se, which is not absorbed by the host in the upper gastrointestinal tract. Microbes influence thyroid levels by regulating iodine uptake, degradation, and enterohepatic cycling. In addition, some minerals play an important role on interactions between host and microbiota, particularly selenium, iron, and zinc (134).
Besides having beneficial effects on the activity of the immune system, a healthy gut microbiota positively influences the thyroid function. Although dysbiosis has been found in autoimmune thyroid diseases (AITDs), it has also been reported in patients with thyroid carcinoma, in which an increased number of carcinogenic and inflammatory bacterial strains were observed. In addition, the composition of the gut microbiota has a major influence on the availability of essential micronutrients for the thyroid gland such as Se and zinc, which are co-factors for deiodination reactions that convert thyroxine (T4) into triiodothyronine (T3). Deficiency of these minerals might result from restrictive or unbalanced diets at any stage of life, which leads to a decreased production of thyroid hormones (135, 136).
The microbiota influences the uptake of Se and may alter the availability of L-thyroxine and toxicity of propylthiouracil (PTU) (134). In case of normal levels of Se, the thyoredoxin reductase system and SH-Px protect the thyrocytes from the activity of peroxides, whereas the apoptotic response to H2O2 is increased with Se deficiency (136). For instance, the decrease in the levels of Lactobacillus can interfere with the formation of iodothyronine deiodinases (DIOs) and, consequently, might result in thyroid dysfunctions (109, 137).
Several species of Lactobacillus are able to keep sodium selenite intracellularly as SeCys and SeMet, thus providing a more bioavailable form of Se, whose absorption by human cells is usually poor in its inorganic form (138). Therefore, the decrease in the amount of Lactobacillus in patients with thyroid disease might impart the bioavailability of Se and its role in the transformation of activated thyroid hormone. In addition, Se protects against oxidative damage during the synthesis of other hormones (109).
In a cohort study, the relationship between the gut microbiome, thyroid cancer, and thyroid nodules was confirmed. Among the findings, a relative abundance of Butyricimonas (p < 0.001) and a significant lower amount of Lactobacillus (p < 0.001) was observed in the group with thyroid cancer and in the group of thyroid nodules, respectively (86). The authors point out to the fact that Lactobacillus is an important genus in the human intestine that is able to improve the concentration of various metals in human cells including Se.
In human and rats, it has been proven that large amounts of conjugated iodothyronines can be hydrolyzed in fecal suspension. The diversity and structure of gut microbiota may play several roles in regulating the drug-controlled thyroidal metabolism (139). Some studies have corroborated that thyroid disorders are the causal factor in the relationship with gut microbes. Other studies have demonstrated that bacteria might act as the motivating factor, as thyroid function may be impaired in patients with small intestinal bacterial overgrowth (140, 141). However, the causative role of Se deficiency, thyroid and gut microbiota has not been thoroughly ascertained yet and further clinical studies are highly recommended.
The metabolic potential of gut microbiota has been identified as a contributing factor for the development of CVD (142). The intestinal microbiota produces signaling molecules such as lipopolysaccharide (LPS) and peptidoglycans that interact with host mucosal surface cells, often through the pattern recognition receptors (PRR) (143). In addition, the gut microbiota interacts with the host through the trimethylamine (TMA)/trimethylamine-N-oxide (TMAO) and short-chain fatty acids routes as well as through other routes related to biliary acids. Some of these molecules have shown to functionally interact with ghrelin, leptin, glucagon-like peptide 1 (GLP-1), and peptide YY (PYY), and to stimulate the parasympathetic nervous system. Such activities impact the metabolic processes related to the development of risk factors for CVD (142).
TMAO has gained considerable attention as a potential promoter of atherosclerosis, cardiometabolic diseases, arterial hypertension, ischemic stroke, atrial fibrillation, heart failure, and acute myocardial infarction (142, 144). Mice supplemented with choline or TMAO showed increased risk of thrombosis, in contrast to germ-free mice under the same diet, suggesting that gut microbiota and specific dietary nutrients that enhance TMAO generation seems to modulate platelet function and thrombosis potential in vivo (145).
Phosphatidylcholine and L-carnitine are metabolized by the intestinal microbiota producing trimethylamine gas (TMA), being further metabolized to TMAO by the liver enzymes of the host (144). A variety of enzymes are involved in the production of TMA from dietary components (146). Glycine betaine reductase (GrdH) is an enzyme that requires Se and is responsible for the production of TMA from glycine betaine (147). However, the role of Se in the TMA-generating pathways remains to be elucidated.
The role of gut microbiota in the oxidative stress process occurs through the uric acid metabolism. Higher levels of Escherichia coli result in greater uric acid decomposition, whereas elevated serum uric acid levels in patients with coronary heart disease are related to gut microbiota dysfunction. High levels of serum uric acid increase the production of oxygen free radical and induce endothelial dysfunction (148). Circulating Se is inversely associated with acid uric levels, suggesting the role of selenium in regulating the intracellular redox status (149).
Patients with type 2 diabetes and coronary heart disease have shown reduced hs-CRP, fasting blood glucose, insulin levels, HOMA-IR and increased nitric oxide (NO), total antioxidant capacity (TAC), and glutation (GSH) after use of 200 μg/day of Se and 8 x 109 CFU/day of Lactobacillus acidophilus, Lactobacillus reuteri, Lactobacillus fermentum, and Bifidobacterium bifidum (2 x 109 CFU/g each) for 12 days (150).
The bioavailability of Se on Enterococcus faecium CCDM 922A (EF) and Streptococcus thermophilus CCDM 144 (ST) and their respective forms enriched with Se, SeEF, and SeST, improved their antioxidant status in animal models (151). Selenium works by blocking the activation of nuclear factor-kB through modulation of expression of selenoprotein genes and by inhibiting the production of reactive oxygen species (ROS) (152). Moreover, probiotic may reduce inflammatory factors and oxidative damage by producing short chain fatty acids in the gut and by decreasing the production of free radicals (153). Probiotic and Se co-supplementation in diabetic patients with coronary heart disease showed beneficial effects on indicators of metabolic profiles related to cardiovascular disease.
Despite multiple human clinical studies revealing associations between gut microbiota composition with the development of cardiovascular diseases, few studies have provided mechanistic or causal evidence of a direct role of Se in gut microbiota in this context.
A study has shown that mice fed with a high-fat diet presented high plasma concentrations of LPS, which is a gram-negative bacterial translocation marker that is strongly related to insulin resistance, obesity, and diabetes. However, such LPS-induced metabolic responses were not observed in CD14 mutant mice, suggesting that the LPS/CD14 system may define the intensity of insulin sensitivity and related diseases (154). In this context, the presence of Bifidobacterium was associated with lower concentrations of LPS in the intestine, which resulted in a lower incidence of metabolic diseases (155). In addition to reducing the systemic inflammatory response, Bifidobacterium reduces intestinal permeability in patients with DM2 (156).
The antidiabetic effects of Bifidobacterium were more responsive when administered together with Se. Bifidobacterium enriched with sodium selenite (B. longum DD98, Se-B) mitigated oral glucose tolerance in diabetic mice, suggesting increased insulin sensitivity and protection of pancreatic β cells. These effects were dose dependent indicating the importance of administering adequate doses for better effectiveness of B. longum DD98, Se-B (157). Wei et al. (69) also evaluated the combined supplementation of Se with microorganisms in diabetic C57BL/6 mice, reporting that treatment with aqueous extracts of selenium-enriched Auricularia auricular (AESA) relieved liver damage triggered by oxidative stress in mice with DM.
Other mechanism involved in the prevention and treatment of insulin resistance relates to the production of short-chain fatty acids (SCFAs), especially butyrate (158). Increased concentration of butyrate in DM2 mice supplemented with live multi-strain probiotics was able to reduce HbA1C levels, improving glucose tolerance and insulin resistance (159). In addition, the administration of Se nanoparticles (0.9 mg/kg) demonstrated an increase in butyrate and in the amounts of beneficial bacteria such as Lactobacillus and Faecalibacterium (160). High concentrations of butyric acid, acetic acid, and isobutyric acid were identified in the feces of mice after oral administration of B. longum DD98, Se-B (157).
The positive effects of butyrate on insulin seems to be associated with an increase in the levels of GLP-1, which in turn lowers blood glucose in patients with DM2 (161) demonstrated that the administration of probiotic, VSL # 3, prevented and treated obesity and diabetes in mice. The mechanism discussed involves the probiotic-gut flora-butyrate-GLP-1 axis which is capable of promoting enhanced metabolic efficiency. Considering that supplementation with B. longum DD98, Se-B also resulted in increased secretion of GLP-1 and protected β cells, it has been speculated whether Se acts on this axis as a modulator of the deleterious effects caused by DM (157).
With the discovery that some bacteria species produce chemicals similar to hormones and monoaminal neurotransmitters in the intestine, the microbiota-intestine-brain axis became evident. This bidirectional interaction allows the brain to influence the gastrointestinal functions as well as the immune functions (162). Oral administration of heat-killed Candida kefyr decreased the severity of experimental autoimmune encephalomyelitis (EAE), significantly reduced Th17 cells and increased regulatory T dendritic cells (DC) and CD103+. In addition to changes in intestinal immunity, changes in the microbiome have been observed such as increase in Lactobacillales and decrease in Bacteroides contents (163). In another study, the administration of Lactobacillus reuteri DSM 17938 modulated the immune response in EAE, decreasing TH1/TH17 cells and cytokines IFN-γ/IL-17 (164).
Neurodegenerative disorders are characterized by an increase in the production of reactive oxygen species (ROS) and a decline in the blood-brain barrier function. Due to the antioxidant property of Se, some selenoproteins play a neuroprotective role (110). TXNRD, for instance, maintains the redox balance and protects the dopaminergic cells, which are prone to oxidative stress in the pathophysiology of Parkinson's disease (165).
Fraga-Silva et al. (166) showed that the administration of Saccharomyces cerevisiae enriched with Se (Selemax) reduced the prevalence of EAE, increased the number of CD103 + dendritic cells and reduced the intestinal inflammatory process compared to the administration of Saccharomyces cerevisiae alone. In addition, Selemax supplementation demonstrated a neuroprotective effect by increasing the expression of protein tau in the CNS. Tau is the main protein associated with the stability of neuronal microtubules along with the MAPs (MAP1 and MAP2) (166). Long-term dietary supplementation (3 months) with Se-enriched yeast (Se-yeast) in triple transgenic mouse model of Alzheimer disease (AD), significantly improved spatial learning, retention of neuronal memory and activity (167).
In a study using humans, probiotic containing Lactobacillus acidophilus, Bifidobacterium bifidum and Bifidobacterium longum (2 × 10 9 UFC/day each) and selenium co-supplementation (200 μg/day, sodium selenite) administered in patients with AD resulted in improved cognitive function and enhanced metabolic profile (168).
Recently, the role of Se in gut health has attracted the interest of the scientific community. This review points out that Brazil nuts, cereals, meat, fish, seafood, milk, and nuts are the best sources of Se and that both deficiency and excess of this metal are related to the occurrence of some diseases. Studies using human and rodent have shown that different doses and sources of Se supplementation can modulate gut microbiota with a positive or negative impact on the host's health (99, 100, 169). The microbial absorption of Se in the large intestine of rats was estimated to be 40–46% of the whole oral dose of Se. In addition, dietetic SeMet increased both fermentation and SCFA production in rats (170). The role of Se in the gut microbiota needs to be better investigated in humans as most studies have been conducted in animal models.
Both the structure and composition of the gut microbiome are significantly affected by genetic and external factors. Among the external factors, dietary pattern is the one that most rapidly alters the gut microbiome in real time, having important role in human health and in the development of chronic diseases (171). Moreover, growth and aging results in physiological changes that modify the gut microbiota (172). The composition of the gut microbiota can also be modulated by metals, therefore requiring a variety of cellular processes such as the system of capture of metal ions by bacteria or high affinity transporters (86). Until now, no specific Se carrier has been identified (84).
A systematic review with meta-analysis including randomized controlled trials on nuts consumption demonstrated a significant increase in the gut content of Clostridium, Dialister, Lachnospira, and Roseburia, as well as a significant decrease in Parabacteroides (60). This finding suggests that high consumption of nuts (a rich source of Se) regulates gut microbiota and promotes the expression of selenoproteins. An in vivo study using mice as the experimental model reported that Se supplementation can optimize the gut microbiota for protection against intestinal dysfunction (101). However, randomized clinical trials are necessary in order to investigate the real impact of Se supplementation on the microbiota and selenoprotein synthesis due to the lack of high-level evidence in the scientific literature.
It has been reported that Se plays a key role in cellular and paracellular permeability, as well as in cellular redox balance and inflammatory cell infiltration (173). Consistent with this finding, Se-deficiency adversely affected the gut barrier function and induced disturbances in the intestinal and immune responses in mice (169). Such events has recently been implicated in several chronic diseases ranging from IBD, DM2, and CVD to cancer and thyroid dysfunctions (135, 136, 169, 174, 175).
The metabolic pathways of selenium biotransformation in gut microbiota remain unclear, even though some bioproducts from Se metabolizing organisms enriched with sodium selenite have been manufactured (137). The rationale behind the use of selenium and probiotic co-supplementation is based on the antioxidant and anti-inflammatory effects of this treatment as observed in the metabolic responses of animal models. For instance, it has been reported that a 4-week probiotic and selenium co-administration to mice under a high-fat diet led to a significant decrease in MDA levels (176). Se-enriched probiotics present themselves as a less toxic alternative to supplementation and have demonstrated a protective effect against liver damage in rats (177) and possible antioxidant, anti-inflammatory, and anti-apoptosis properties (178).
This current review has shown that the composition of the microbiota can be modulated by the dietary Se, in which it can influence both the Se status of the host and the expression of the selenoproteoma. In return, the organism provides the nutrients used by bacteria for energy production and maintenance of their metabolic pathways, therefore characterizing a symbiotic relationship. The gut microbiota can interact with Se for the expression of its own selenoproteins. In addition, some species of intestinal microorganisms can improve the bioavailability of Se and protect against its toxicity. One question that remains unanswered is what constitutes an optimal health-promoting microbiome.
Ultimately, determining the full landscape of host-microbiota interactions and Se status will enable advances in the development of bioproducts involving selenium metabolizing microorganisms, which seems to be a safe alternative for studies about Se supplementation.
LP coordinated the elaboration of the manuscript. RF, KS-E, RC, and LP developed the layout of the manuscript, collected literature, and wrote the manuscript. FP collaborated with the layout of the manuscript and drew all figures. EA translated the entire text to English. LP and EA edited the final version of the manuscript. RF assisted in the reference management. All authors participated in the analysis and interpretation of data as well as in writing the manuscript. All authors approved the submitted version.
The authors declare that the research was conducted in the absence of any commercial or financial relationships that could be construed as a potential conflict of interest.
We thank the Coordination for the Improvement of Higher Education Personnel (Coordenação de Aperfeiçoamento de Pessoal de Nível Superior - CAPES) for the RLUF Master's scholarship, Finance Code 001.
1. Burk R, Hill K. Regulation of selenium metabolism and transport. Annu Rev Nutr. (2015) 35:109–34. doi: 10.1146/annurev-nutr-071714-034250
2. Navarro-alarcon M, Cabrera-vique C. Selenium in food and the human body : a review. Sci Total Environ. (2008) 400:115–41. doi: 10.1016/j.scitotenv.2008.06.024
3. Qazi IH, Angel C, Yang H, Zoidis E, Pan B, Wu Z, et al. Role of selenium and selenoproteins in male reproductive function: a review of past and present evidences. Antioxidants. (2019) 8:268. doi: 10.3390/antiox8080268
4. Kim D, Ku B, Choi E-M. Se-methylselenocysteine stimulates migration and antioxidant response in HaCaT keratinocytes: implications for wound healing. J Trace Elem Med Biol. (2020) 58:126426. doi: 10.1016/j.jtemb.2019.126426
5. Ramos SJ, Faquin V, de Almeida H, Ávila FW, Guilherme LRG, Bastos CEA, et al. Selenato e selenito na produção, nutrição mineral e biofortificação com selênio em cultivares de alface. Rev Bras Cienc do Solo. (2011) 35:1347–55. doi: 10.1590/S0100-06832011000400029
6. Schiavon M, Pilon-Smits EAH. Selenium biofortification and phytoremediation phytotechnologies: a review. J Environ Qual. (2017) 46:10–9. doi: 10.2134/jeq2016.09.0342
7. Fraczek A, Pasternak K. Selenium in medicine and treatment. J Elem. (2013) 18:145–64. doi: 10.5601/jelem.2013.18.1.13
8. Ortuño J, Ros G, Periago MJ, Martinez C, López G, RJ. Importância nutricional del selênio. Arch Latinoam Nutr. (1997) 47:6–13.
9. Surai PF, Fisinin VI. Selenium in pig nutrition and reproduction: boars and semen quality - A review. Asian Aust J Anim Sci. (2015) 28:730–6. doi: 10.5713/ajas.14.0593
10. Kieliszek M, Błazejak S. Current knowledge on the importance of selenium in food for living organisms : a review. Nutrients. (2016) 21:609. doi: 10.3390/molecules21050609
11. Kieliszek M. Selenium–Fascinating microelement, properties and sources in Food. Nutrients. (2019) 24:1298. doi: 10.3390/molecules24071298
12. dos Santos M, da Silva Júnior F, Muccillo-Baisch A. Conteúdo de selênio em alimentos brasileiros: uma revisão dos valores da literatura. J Food Compost Anal. (2017) 58:10–5. doi: 10.1016/j.jfca.2017.01.001
14. Fairweather-tait SJ, Bao Y, Broadley MR, Collings R, Ford D, Hesketh JE, et al. Selenium in human health and disease. Antioxid Redox Signal. (2011) 14:1337–83. doi: 10.1089/ars.2010.3275
15. Daniels LA. Selenium metabolism and bioavailability. Biol Trace Elem Res. (1996) 54:185–99. doi: 10.1007/BF02784430
16. Fairweather-tait SJ, Collings R, Hurst R. Selenium bioavailability : current knowledge and future research. Am J Clin Nutr. (2010) 91:1484–91. doi: 10.3945/ajcn.2010.28674J
17. Rayman MP, Infante HG, Sargent M. Food-chain selenium and human health: spotlight on speciation. Br J Nutr. (2008) 100:238–53. doi: 10.1017/S0007114508922522
18. Xu X, Carlson BA, Mix H, Zhang Y, Saira K, Glass RS, et al. Biosynthesis of selenocysteine on its tRNA in eukaryotes. PLoS Biol. (2007) 5:e4. doi: 10.1371/journal.pbio.0050004
19. Donovan J, Copeland PR. The efficiency of selenocysteine incorporation is regulated by translation initiation factors. J Mol Biol. (2010) 400:659–64. doi: 10.1016/j.jmb.2010.05.026
20. Latrèche L, Duhieu S, Touat-hamici Z, Jean-jean O, Latrèche L, Duhieu S, et al. The differential expression of glutathione peroxidase 1 and 4 depends on the nature of the SECIS element. RNA Biol. (2012) 9:681–90. doi: 10.4161/rna.20147
21. Meuillet E, Stratton S, Cherukuri DP, Goulet AC, Kagey J, Porterfield B, NM. Chemoprevention of prostate cancer with selenium: an update on current clinical trials and preclinical findings. J Cell Biochem. (2004) 91:443–58. doi: 10.1002/jcb.10728
24. Belhadj M, Sarra L, Tani K, Medjati ND, Harek Y, Sahi MD, et al. Se status prediction by food intake as compared to circulating biomarkers in a West Algerian population. Nutrients. (2020) 12:E3599. doi: 10.3390/nu12123599
25. Arias-borrego A, Callejón-leblic B, Calatayud M, Gómez- JL, Collado MC, García-barrera T. Insights into cancer and neurodegenerative diseases through selenoproteins and the connection with gut microbiota - current analytical methodologies. Expert Rev Proteomics. (2019) 16:805–14. doi: 10.1080/14789450.2019.1664292
26. Phiri FP, Ander EL, Lark RM, Bailey EH, Chilima B, Gondwe J, et al. Urine selenium concentration is a useful biomarker for assessing population level selenium status. Environ Int. (2020) 134:105218. doi: 10.1016/j.envint.2019.105218
27. Greiner A, Hildebrand J, Uter RFW, Göen HDT. Evaluation of urinary selenium as a biomarker of human occupational exposure to elemental and inorganic selenium. Int Arch Occup Env Heal. (2019) 93:325–35. doi: 10.1007/s00420-019-01489-2
28. Jäger T, Drexler H, Göen T. Human metabolism and renal excretion of selenium compounds after oral ingestion of sodium selenate dependent on trimethylselenium ion (TMSe) status. Arch Toxicol. (2016) 90:149–58. doi: 10.1007/s00204-014-1380-x
29. Satia JA, King IB, Morris JS, Stratton K, White E. Toenail and plasma levels as biomarkers of selenium exposure. Ann Epidemiol. (2006) 16:53–8. doi: 10.1016/j.annepidem.2005.02.011
30. Gutiérrez-gonzález E, García-esquinas E, De Larrea-baz NF, Salcedo-bellido I, Navas-acien A, Lope V, et al. Toenails as biomarker of exposure to essential trace metals: a review. Environ Res. (2019) 179:108787. doi: 10.1016/j.envres.2019.108787
31. Filippini T, Ferrari A, Michalke B, Grill P, Vescovi L, Salvia C, et al. Toenail selenium as an indicator of environmental exposure : a cross-sectional study. Mol Med Rep. (2017) 15:3405–12. doi: 10.3892/mmr.2017.6388
32. Ashton K, Hooper L, Harvey LJ, Hurst R. Methods of assessment of selenium status in humans : a systematic. Am J Clin Nutr. (2009) 89:2025S–39S. doi: 10.3945/ajcn.2009.27230F
33. Hurst R, Armah CN, Dainty JR, Hart DJ, Teucher B, Goldson AJ, et al. Establishing optimal selenium status : results of a randomized, double-blind, placebo-controlled trial. Am J Clin Nutr. (2010) 91:923–31. doi: 10.3945/ajcn.2009.28169
34. Brodin O, Hackler J, Misra S, Wendt S, Sun Q. Selenoprotein P as biomarker of selenium status in clinical trials with therapeutic dosages of selenite. Nutrients. (2020) 12:1067. doi: 10.3390/nu12041067
35. Thomson CD. Assessment of requirements for selenium and adequacy of selenium status: a review. Eur J Clin Nutr. (2004) 58:391–402. doi: 10.1038/sj.ejcn.1601800
36. Müller SM, Dawczynski C, Wiest J, Lorkowski S, Kipp AP, Schwerdtle T. Functional Biomarkers for the selenium status in a human nutritional intervention study. Nutrients. (2020) 12:676. doi: 10.3390/nu12030676
37. Lubos E, Loscalzo J, Handy DE. Glutathione peroxidase-1 in health and disease: from molecular mechanisms to therapeutic opportunities. Antioxid Redox Signal. (2011) 15:1957–97. doi: 10.1089/ars.2010.3586
38. Ibrahim SAZ, Kerkadi A, Agouni A. Selenium and health : an update on the situation in the Middle East and North Africa. Nutrients. (2019) 11:1457. doi: 10.3390/nu11071457
39. Kang D, Lee J, Wu C, Guo X, Lee BJ, Chun J-S, et al. The role of selenium metabolism and selenoproteins in cartilage homeostasis and arthropathies. Exp Mol Med Vol. (2020) 52:1198–208. doi: 10.1038/s12276-020-0408-y
40. Rayman MP. Selenium and human health. Lancet J. (2012) 379:1256–68. doi: 10.1016/S0140-6736(11)61452-9
41. Rayman MP. Selenium intake, status, and health: a complex relationship. Hormones. (2020) 19:9–14. doi: 10.1007/s42000-019-00125-5
42. Bleys J, Navas-Acien A, Guallar E. Serum selenium levels and all-cause, cancer, and cardiovascular mortality among US adults. Arch Intern Med. (2008) 168:404–10. doi: 10.1001/archinternmed.2007.74
43. Zhang X, Liu C, Gui J, Song Y. Selenium status and cardiovascular diseases: meta-analysis of prospective observational studies and randomized controlled trials. Eur J Clin Nutr. (2016) 70:162–9. doi: 10.1038/ejcn.2015.78
44. Kuria A, Tian H, Li M, Wang Y, Aaseth J, Zang J, et al. Selenium status in the body and cardiovascular disease: a systematic review and meta-analysis. Crit Rev Food Sci Nutr. (2020) 17:1–10. doi: 10.1080/10408398.2020.1803200
45. Ju W, Li X, Li Z, Wu G, Fu X, Yang X, et al. The effect of selenium supplementation on coronary heart disease: a systematic review and meta-analysis of randomized controlled trials. J Trace Elem Med Biol. (2017) 44:8–16. doi: 10.1016/j.jtemb.2017.04.009
46. Kühn E, Slagman A, Kühn-Heid E, Seelig J, Schwiebert C, Minich W, et al. Circulating levels of selenium-binding protein 1 (SELENBP1) are associated with risk for major adverse cardiac events and death. J Trace Elem Med Biol. (2019) 52:247–53. doi: 10.1016/j.jtemb.2019.01.005
47. Kim J, Chung HS, Choi M, Roh YK, Yoo HJ, Park JH, et al. Association between serum selenium level and the presence of diabetes mellitus : a meta-analysis of observational studies. Diabetes Metab J. (2019) 43:447–60. doi: 10.4093/dmj.2018.0123
48. Vinceti M, Filippini T, Rothman KJ. Selenium exposure and the risk of type 2 diabetes: a systematic review and meta-analysis. Eur J Epidemiol. (2018) 33:789–810. doi: 10.1007/s10654-018-0422-8
49. Zhou J, Huang K, LX. Selenium and diabetes - evidence from animal studies. Free Radic Biol Med. (2013) 65:1548–56. doi: 10.1016/j.freeradbiomed.2013.07.012
50. Mcclung JP, Roneker CA, Mu W, Lisk DJ, Langlais P, Liu F, et al. Development of insulin resistance and obesity in mice overexpressing cellular glutathione peroxidase. Proc Natl Acad Sci USA. (2004) 101:8852–7. doi: 10.1073/pnas.0308096101
51. Solovyev N. Selenoprotein P and its potential role in Alzheimer' s disease. Hormones. (2020) 19:73–9. doi: 10.1007/s42000-019-00112-w
52. Thomson CD, Chisholm A, Mclachlan SK, Campbell JM. Brazil nuts : an effective way to improve selenium status. Am J Clin Nutr. (2008) 87:379–84. doi: 10.1093/ajcn/87.2.3
53. Cominetti C, De Bortoli MC, Garrido AB, Cozzolino SMF. Brazilian nut consumption improves selenium status and glutathione peroxidase activity and reduces atherogenic risk in obese women. Nutr Res. (2012) 32:403–7. doi: 10.1016/j.nutres.2012.05.005
54. Duarte GB, Reis BZ, Rogero MM, Vargas-mendez E, Júnior FB, Cercato C, et al. Consumption of Brazil nuts with high selenium levels increased inflammation biomarkers in obese women: a randomized controlled trial. Nutrition. (2019) 63–64:162–8. doi: 10.1016/j.nut.2019.02.009
55. Donadio JLS, Rogero MM, Guerra-shinohara EM, Barbosa F, Desmarchelier C, Borel P, et al. Genetic variants in selenoprotein genes modulate biomarkers of selenium status in response to Brazil nut supplementation (the SU. BRA. NUT study). Clin Nutr. (2018) 38:1–10. doi: 10.1016/j.clnu.2018.03.011
56. Huguenin GVB, Oliveira GMM, Moreira ASB, Saint Pierre TD, Gonçalves RA, Pinheiro-mulder AR, et al. Improvement of antioxidant status after Brazil nut intake in hypertensive and dyslipidemic subjects. Nutr J. (2015) 29:1–10. doi: 10.1186/s12937-015-0043-y
57. Behne D, Alber D, Kyriakopoulos A. Long-term selenium supplementation of humans: selenium status and relationships between selenium concentrations in skeletal muscle and indicator materials. Jl Trace Elem Med Biol. (2010) 24:99–105. doi: 10.1016/j.jtemb.2009.12.001
58. Colpo E, Da Vilanova C, Reetz LG, Duarte M, Farias I, Meinerz DF, et al. Brazilian nut consumption by healthy volunteers improves in fl ammatory parameters. Nutrition. (2014) 30:459–65. doi: 10.1016/j.nut.2013.10.005
59. Karunasinghe N, Han DY, Zhu S, Duan H, Ko Y, Yu JF, et al. Effects of supplementation with selenium, as selenized yeast, in a healthy male population from New Zealand. Nutr Cancer. (2013) 65:355–66. doi: 10.1080/01635581.2013.760743
60. Creedon AC, Hung ES, Berry SE, Whelan K. Nuts and their e ff ect on gut microbiota, gut function and symptoms in adults : a systematic review and meta-analysis of randomised controlled trials. Nutrients. (2020) 12:2347. doi: 10.3390/nu12082347
61. Byerley L, Samuelson D, Blanchard IE, Luo M, Lorenzen B, Banks S, et al. Changes in the gut microbial communities following addition of walnuts to the diet. J Nutr Biochem. (2017) 48:94–102. doi: 10.1016/j.jnutbio.2017.07.001
62. Holscher H, Taylor A, Swanson K, Novotny J, Baer D. Almond consumption and processing affects the composition of the gastrointestinal microbiota of healthy adult men and women: a randomized controlled trial. Nutrients. (2018) 10:126. doi: 10.3390/nu10020126
63. Ukhanova M, Wang X, Baer D, Novotny J, Fredborg M, Mai V. Effects of almond and pistachio consumption on gut microbiota composition in a randomised cross-over human feeding study. Br J Nutr. (2014) 111:2146–52. doi: 10.1017/S0007114514000385
64. Clark L, Combs G, Turnbull B, Slate E, Chalker D, Al E. Effects of selenium supplementation for cancer prevention in patients with carcinoma of the skin. A randomized controlled trial. Nutritional Prevention of Cancer Study Group. JAMA. (1996) 276:957–1963.
65. Salonen JT, Alfthan G, Huttunen JK, Pikkarainen J, Puska P. Association between cardiovascular death and myocardial infarction and serum selenium in a matched-pair longitudinal study. Lancet. (1982) 320:175–9. doi: 10.1016/S0140-6736(82)91028-5
66. Virtamo J, Valkeila E, Alfthan G, Punsar S, Huttunen JK, Karvonen MJ. Serum selenium and the risk of coronary heart disease and stroke. Am J Epidemiol. (1985) 122:276–82.
67. Lippman SM, Klein EA, Goodman PJ, Scott LM, Thompson IM, Al E. Effect of selenium and vitamin E on risk of prostate cancer and other cancers: the selenium and vitamin E Cancer Prevention Trial (SELECT). JAMA. (2009) 301:39–51. doi: 10.1001/jama.2008.864
68. Stranges S, Marshall JR, Natarajan R, Donahue RP, Al E. Effects of long-term selenium supplementation on the incidence of type 2 diabetes: a randomized trial. Ann Intern Med. (2007) 147:217–23. doi: 10.7326/0003-4819-147-4-200708210-00175
69. Wei J, Zeng C, Gong Q, Yang H, Li X, Lei G, et al. The association between dietary selenium intake and diabetes : a cross-sectional study among middle-aged and older adults. Nutr J. (2015) 14:18. doi: 10.1186/s12937-015-0007-2
70. Farrokhian A, Bahmani F, Taghizadeh M, Mirhashemi SM, Aarabi MH, Raygan, et al. Selenium supplementation affects insulin resistance and serum hs-CRP in patients with type 2 diabetes and coronary heart disease. Horm Metab Res. (2016) 48:263–8. doi: 10.1055/s-0035-1569276
71. Kohler LN, Florea A, Kelley CP, Chow S, Hsu P, Batai K, et al. Higher plasma selenium concentrations are associated with increased odds of prevalent type 2 diabetes. J Nutr. (2018) 148:1333–40. doi: 10.1093/jn/nxy099
72. Morris JS, Crane SB. Selenium toxicity from a misformulated dietary supplement, adverse health effects, and the temporal response in the nail biologic monitor. Nutrients. (2013) 5:1024–57. doi: 10.3390/nu5041024
73. Rayman MP, Winther KH, Pastor-barriuso R, Cold F. Effect of long-term selenium supplementation on mortality: results from a multiple-dose, randomised controlled trial. Free Radic Biol Med. (2018) 1:46–54. doi: 10.1016/j.freeradbiomed.2018.02.015
74. Yang GQ, Wang SZ, Zhou RH, SS. Endemic selenium intoxication of humans in China. Am J Clin Nutr. (1983) 37:872–81. doi: 10.1093/ajcn/37.5.872
75. Suzuki KT, Sasakura C, Yoneda S. Binding sites for the (Hg-Se) complex on selenoprotein P. Biochim Biophys Acta. (1998) 1429:102–12. doi: 10.1016/s0167-4838(98)00221-0
76. Liu Y, Zhang W, Zhao J, Lin X, Liu J, Cui L, et al. Selenoprotein P as the major transporter for mercury in serum from methylmercury-poisoned rats. J Trace Elem Med Biol. (2018) 50:589–95. doi: 10.1016/j.jtemb.2018.04.013
77. Krittaphol W, McDowell A, Thomson CD, Tucker IG, Fawcett JP, Mikov M. An improved HPLC method for the investigation of L-selenomethionine metabolism in rat gut contents. Biomed Chromatogr. (2009) 23:1169–74. doi: 10.1002/bmc.1239
78. Bull MJ, Plummer NT. Part 1: the human gut microbiome in health and disease. Integr Med. (2014) 13:17–22.
79. Lozupone CA, Stombaugh JI, Gordon JI, Jansson JK, Knight R. Diversity, stability and resilience of the human gut microbiota. Nature. (2012) 489:220–30. doi: 10.1038/nature11550
80. Milani C, Duranti S, Bottacini F, Casey E, Turroni F, Mahony J, et al. The first microbial colonizers of the human gut: composition, activities, and health implications of the infant gut microbiota. Microbiol Mol Biol Rev. (2017) 81:e00036–17. doi: 10.1128/MMBR.00036-17
81. Fassarella M, Blaak EE, Penders J, Nauta A, Smidt H, Zoetendal EG. Gut microbiome stability and resilience: elucidating the response to perturbations in order to modulate gut health. Gut. (2021) 70:595–605. doi: 10.1136/gutjnl-2020-321747
83. Zhang Y, Gladyshev VN. Comparative genomics of trace elements: emerging dynamic view of trace element utilization and function. Chem Rev. (2009) 109:4828–61. doi: 10.1021/cr800557s
84. Lin J, Peng T, Jiang L, Ni JZ, Liu Q, Chen L, et al. Comparative genomics reveals new candidate genes involved in selenium metabolism in prokaryotes. Genome Biol Evol. (2015) 7:664–76. doi: 10.1093/gbe/evv022
85. Peng T, Lin J, Xu YZ, Zhang Y. Comparative genomics reveals new evolutionary and ecological patterns of selenium utilization in bacteria. ISME J. (2016) 10:2048–59. doi: 10.1038/ismej.2015.246
86. Zhang J, Zhang F, Zhao C, Xu Q, Liang C, Al E. Dysbiosis of the gut microbiome is associated with thyroid cancer and thyroid nodules and correlated with clinical index of thyroid function. Endocrine. (2019) 34:564–74. doi: 10.1007/s12020-018-1831-x
87. Koziolek M, Grimm M, Becker D. Al. E. Investigation of pH and temperature profiles in the GI tract of fasted human subjects using the intellicap(®) system. J Pharm Sci. (2015) 1041:2855–63. doi: 10.1002/jps.24274
88. Zhernakova A, Kurilshikov A, Bonder MJ, Tigchelaar EF, Schirmer M, Vatanen T, et al. Population-based metagenomics analysis reveals markers for gut microbiome composition and diversity. Science. (2016) 352:565–9. doi: 10.1126/science.aad3369
89. Magge S, Lembo A. Low-FODMAP diet for treatment of irritable bowel syndrome. Gastroenterol Hepatol. (2012) 8:739–45.
90. Lewis JD, Abreu MT. Diet as a trigger or therapy for inflammatory bowel diseases. Gastroenterology. (2017) 152:398–414.e6. doi: 10.1053/j.gastro.2016.10.019
91. Zhan YL, Zhan YA, Dai SX. Is a low FODMAP diet beneficial for patients with inflammatory bowel disease? A meta-analysis and systematic review. Clin Nutr. (2018) 37:123–9. doi: 10.1016/j.clnu.2017.05.019
92. Staudacher HM. Nutritional, microbiological and psychosocial implications of the low FODMAP diet. J Gastroenterol Hepatol. (2017) 32:16–9. doi: 10.1111/jgh.13688
93. Eswaran S, Dolan RD, Ball SC, Jackson K, Chey W. The impact of a 4-Week low-FODMAP and mNICE diet on nutrient intake in a sample of US adults with irritable bowel syndrome with diarrhea. J Acad Nutr Diet. (2020) 120:641–9. doi: 10.1016/j.jand.2019.03.003
94. Hrdina J, Banning A, Kipp A, Loh G, Blaut M, Brigelius-Flohé R. The gastrointestinal microbiota affects the selenium status and selenoprotein expression in mice. J Nutr Biochem. (2009) 20:638–48. doi: 10.1016/j.jnutbio.2008.06.009
95. Itoh Y, Bröcker MJ, Sekine S, Hammond G, Suetsugu S, Söll D, et al. Decameric SelA•tRNA(Sec) ring structure reveals mechanism of bacterial selenocysteine formation. Science. (2013) 340:75–8. doi: 10.1126/science.1229521
96. Kryukov GV, Gladyshev VN. The prokaryotic selenoproteome. EMBO Rep. (2004) 5:538–43. doi: 10.1038/sj.embor.7400126
97. Lopez CA, Skaar EP. The impact of dietary transition metals on host-bacterial interactions. Cell Host Microbe. (2018) 23:737–48. doi: 10.1016/j.chom.2018.05.008
98. Capdevilla DA, Wang J, Giedroc DP. Bacterial strategies to maintain zinc metallostasis at the host-pathogen interface. J Biol Chem. (2016) 291:20858–68. doi: 10.1074/jbc.R116.742023
99. Kasaikina MV, Kravtsova MA, Lee BC, Seravalli J, Peterson DA, Walter J, et al. Dietary selenium affects host selenoproteome expression by influencing the gut microbiota. FASEB J. (2011) 25:2492–9. doi: 10.1096/fj.11-181990
100. Takahashi K, Suzuki N, Ogra Y. Effect of gut microflora on nutritional availability of selenium. Food Chem. (2020) 319:126537. doi: 10.1016/j.foodchem.2020.126537
101. Zhai Q, Cen S, Li P, Tian F, Al E. Effects of dietary selenium supplementation on intestinal barrier and immune responses associated with its modulation of gut microbiota. Environ Sci Technol Lett. (2018) 5:724–30. doi: 10.1021/acs.estlett.8b00563
102. Takahashi K, Suzuki N, Ogra Y. Bioavailability comparison of nine bioselenocompounds in vitro and in vivo. Int J Mol Sci. (2017) 18:506. doi: 10.3390/ijms18030506
103. Sumner S, Markley R, Kirimanjeswara G. Role of selenoproteins in bacterial pathogenesis. Biol Trace Elem Res. (2019) 192:69–82. doi: 10.1007/s12011-019-01877-2
104. Krittaphol W, McDowell A, Thomson CD, Mikov M, Fawcett JP. Biotransformation of L-selenomethionine and selenite in rat gut contents. Biol Trace Elem Res. (2011) 139:188–96. doi: 10.1007/s12011-010-8653-x
105. Breton J, Daniel C, Dewulf J, Pothion S, Froux N, Sauty M, et al. Gut microbiota limits heavy metals burden caused by chronic oral exposure. Toxicol Lett. (2013) 222:132–8. doi: 10.1016/j.toxlet.2013.07.021
106. Liu Y, Ji J, Zhang W, Suo Y, Zhao J, Lin X, et al. Selenium modulated gut flora and promoted decomposition of methylmercury in methylmercury-poisoned rats. Ecotoxicol Environ Saf. (2019) 185:109720. doi: 10.1016/j.ecoenv.2019.109720
107. Nettleford SK, Prabhu KS. Selenium and selenoproteins in gut inflammation-a review. Antioxidants. (2018) 7:36. doi: 10.3390/antiox7030036
108. Peters KM, Carlson BA, Gladyshev VN, Tsuji PA. Selenoproteins in colon cancer. Free Radic Biol Med. (2018) 1:14–25. doi: 10.1016/j.freeradbiomed.2018.05.075
109. Köhrle J. Selenium and the thyroid. Curr Opin Endocrinol Diabetes Obes. (2013) 20:441–8. doi: 10.1097/01.med.0000433066.24541.88
110. Solovyev N, Drobyshev E, Blume B, Michalke B. Selenium at the neural barriers: a review. Front Neurosci. (2021) 15:630016. doi: 10.3389/fnins.2021.630016
111. Carabotti M, Scirocco A, Maselli MA, Severi C. The gut-brain axis: interactions between enteric microbiota, central and enteric nervous systems. Ann Gastroenterol. (2015) 28:203–9.
112. Han YM, Yoon H, Lim S, Sung MK, Al E. Risk factors for vitamin D, zinc, and selenium deficiencies in Korean patients with inflammatory bowel disease. Gut Liver. (2017) 11:363–9. doi: 10.5009/gnl16333
113. Kudva AK, Shay AE, Prabhu KS. Selenium and inflammatory bowel disease. Am J Physiol Gastrointest Liver Physiol. (2015) 309:G71–7. doi: 10.1152/ajpgi.00379.2014
114. Tian T, Wang Z, Zhang J. Pathomechanisms of oxidative stress in inflammatory bowel disease and potential antioxidant therapies. Oxid Med Cell Longev. (2017) 2017:4535194. doi: 10.1155/2017/4535194
115. Nunes C, Teixeira N, Serra D, Freitas V, Almeida L, Laranjinha J. Red wine polyphenol extract efficiently protects intestinal epithelial cells from inflammation via opposite modulation of JAK/STAT and Nrf2 pathways. Toxicol Res. (2016) 5:53–65. doi: 10.1039/C5TX00214A
116. Khor T, Huang M, Kwon K, Chan J, Reddy B, Kong A. Nrf2-Deficient mice have an increased susceptibility to dextran sulfate sodium–induced colitis. Cancer Res. (2006) 2006:24. doi: 10.1158/0008-5472
117. Brigelius-Flohé R, Müller M, Lippmann D, Kipp AP. The yin and yang of nrf2-regulated selenoproteins in carcinogenesis. Int J Cell Biol. (2012) 2012:486147. doi: 10.1155/2012/486147
118. Reszka E, Wieczorek E, Jablonska E, Janasik B, Fendler W, Wasowicz W. Association between plasma selenium level and NRF2 target genes expression in humans. J Trace Elem Med Biol. (2015) 30:102–6. doi: 10.1016/j.jtemb.2014.11.008
119. Pan H, Wang H, Wang X, Zhu L, Mao L. The absence of Nrf2 enhances NF-κB-dependent inflammation following scratch injury in mouse primary cultured astrocytes. Mediators Inflamm. (2012) 2012:217580. doi: 10.1155/2012/217580
120. Zhu C, Zhang S, Song C, Zhang Y, Ling Q, Hoffmann PR, et al. Selenium nanoparticles decorated with Ulva lactuca polysaccharide potentially attenuate colitis by inhibiting NF-κB mediated hyper inflammation. J Nanobiotechnol. (2017) 15:20. doi: 10.1186/s12951-017-0252-y
121. Lovasz BD, Golovics PA, Vegh Z, Lakatos PL. New trends in inflammatory bowel disease epidemiology and disease course in Eastern Europe. Digest Liver Dis. (2013) 45:269–76. doi: 10.1016/j.dld.2012.08.020
122. Brown K, DeCoffe D, Molcan E, Gibson DL. Diet-induced dysbiosis of the intestinal microbiota and the effects on immunity and disease. Nutrients. (2012) 4:1095–119. doi: 10.3390/nu4081095
123. Weng YJ, Gan HY, Li X, Huang Y, Li ZC, Deng HM, et al. Correlation of diet, microbiota and metabolite networks in inflammatory bowel disease. J Dig Dis. (2019) 20:447–59. doi: 10.1111/1751-2980.12795
124. Shi C, Yue F, Shi F, Qin Q, Al E. Selenium-containing amino acids protect dextran sulfate sodium-induced colitis via ameliorating oxidative stress and intestinal inflammation. J Inflamm Res. (2021) 14:89–95. doi: 10.2147/JIR.S288412
125. Xu C, Guo Y, Qiao L, Ma L, Cheng Y, Roman A. Biogenic synthesis of novel functionalized selenium nanoparticles by Lactobacillus casei ATCC 393 and its protective effects on intestinal barrier dysfunction caused by enterotoxigenic Escherichia coli K88. Front Microbiol. (2018) 9:1129. doi: 10.3389/fmicb.2018.01129
126. Hughes DJ, Fedirko V, Jenab M, Schomburg L, Méplan C, Freisling H, et al. Selenium status is associated with colorectal cancer risk in the European prospective investigation of cancer and nutrition cohort. Int J Cancer. (2015) 135:1149–61. doi: 10.1002/ijc.29071
127. Saulnier DM, Riehle K, Mistretta TA, Diaz MA, Al E. Gastrointestinal microbiome signatures of pediatric patients with irritable bowel syndrome. Gastroenterology. (2011) 141:1782–91. doi: 10.1053/j.gastro.2011.06.072
128. Yi Y, Shen L, Shi W, Xia F, Al E. Gut microbiome components predict response to neoadjuvant chemoradiotherapy in patients with locally advanced rectal cancer: a prospective, longitudinal study. Clin Cancer Res. (2020) 27:1329–40. doi: 10.1158/1078-0432
129. Chen J, Chia N, Kalari KR, Yao JZ, Novotna M, Paz Soldan MM, et al. Multiple sclerosis patients have a distinct gut microbiota compared to healthy controls. Sci Rep. (2016) 27:28484. doi: 10.1038/srep28484
130. Del Chierico F, Nobili V, Vernocchi P, Russo A, De Stefanis C, Gnani D, et al. Gut microbiota profiling of pediatric nonalcoholic fatty liver disease and obese patients unveiled by an integrated meta-omics-based approach. Hepatology. (2017) 65:451–64. doi: 10.1002/hep.28572
131. Barrett CW, Reddy VK, Short SP, Motley AK, Lintel MK, Bradley AM, et al. Selenoprotein P influences colitis induced tumorigenesis by mediating stemness and oxidative damage. J Clin Invest. (2015) 125:2646–60. doi: 10.1172/JCI76099
132. Mego M, Májek J, Konceková R, Ebringer L, Cierniková S, Rauko P, et al. Intramucosal bacteria in colon cancer and their elimination by probiotic strain Enterococcus faecium M-74 with organic selenium. Folia Microbiol. (2005) 50:443–7. doi: 10.1007/BF02931427
133. Porto B, Monteiro C, Souza É, Leocádio P, Alvarez-Leite J, Generoso S, et al. Treatment with selenium-enriched Saccharomyces cerevisiae UFMG A-905 partially ameliorates mucositis induced by 5-fluorouracil in mice. Cancer Chemother Pharmacol. (2019) 84:117–26. doi: 10.1007/s00280-019-03865-8
134. Fröhlich E, Wahl R. Microbiota and thyroid interaction in health and disease. Trends Endocrinol Metab. (2019) 30:479–90. doi: 10.1016/j.tem.2019.05.008
135. Knezevic J, Starchl C, Tmava Berisha A, Amrein K. Thyroid-gut-axis: how does the microbiota influence thyroid function? Nutrients. (2020) 12:1769. doi: 10.3390/nu12061769
136. Mezzomo TR, Nadal J. Efeito dos nutrientes e substâncias alimentares na função tireoidiana e no hipotireoidismo. Demetra. (2016) 11:427–43. doi: 10.12957/demetra.2016.18304
137. Martínez FG, Moreno-Martin G, Pescuma M, Madrid-Albarrán Y, Mozzi F. Biotransformation of selenium by lactic acid bacteria: formation of seleno-nanoparticles and seleno-amino acids. Front Bioeng Biotechnol. (2020) 8:506. doi: 10.3389/fbioe.2020.00506
138. Pessione E. Lactic acid bacteria contribution to gut microbiota complexity: lights and shadows. Front Cell Infect Microbiol. (2012) 22:86. doi: 10.3389/fcimb.2012.00086
139. Yao Z, Zhao M, Gong Y, Chen W, Wang Q, Fu Y, et al. Relation of gut microbes and l-thyroxine through altered thyroxine metabolism in subclinical hypothyroidism subjects. Front Cell Infect Microbiol. (2020) 10:495. doi: 10.3389/fcimb.2020.00495
140. Feng J, Zhao F, Sun J, Lin B, Zhao L, Liu Y, et al. E. Alterations in the gut microbiota and metabolite profiles of thyroid carcinoma patients. Int J Cancer. (2019) 144:2728–45. doi: 10.1002/ijc.32007/
141. Konrad P, Chojnacki J, Kaczka A, Pawłowicz M, Rudnicki C, Chojnacki C. [Thyroid dysfunction in patients with small intestinal bacterial overgrowth]. Pol Merkur Lekarski. (2018) 44:15–8.
142. Tang W, Kitai T, Hazen S. Gut microbiota in cardiovascular health and disease. Circ Res. (2017) 120:1183–96. doi: 10.1161/CIRCRESAHA
143. Larsson E, Tremaroli V, Lee Y, Koren O, Nookaew I, Fricker A, et al. Analysis of gut microbial regulation of host gene expression along the length of the gut and regulation of gut microbial ecology through myd88. Gut. (2012) 61:1124–31. doi: 10.1136/gutjnl-2011-301104
144. Gatarek P, Kaluzna-Czaplinska J. Trimethylamine N-oxide (TMAO) in human health. EXCLI J. (2021) 20:301–19. doi: 10.17179/excli2020-3239
145. Zhu W, Gregory JC, Org E, Buffa JA, Gupta N, Wang Z, et al. Gut microbial metabolite TMAO enhances platelet hyperreactivity and thrombosis risk. Cell. (2016) 165:111–24. doi: 10.1016/j.cell.2016.02.011
146. Simó C, García-Cañas V. Dietary bioactive ingredients to modulate the gut microbiota-derived metabolite TMAO. New opportunities for functional food development. Food Funct. (2020) 11:6745–76. doi: 10.1039/d0fo01237h
147. Andreesen J. Glycine metabolism in anaerobes. Antonie Van Leeuwenhoek. (1994) 66:223–37. doi: 10.1007/BF00871641
148. Liu H, Zhuang J, Tang P, Li J, Xiong X, Deng H. The role of the gut microbiota in coronary heart disease. Curr Atheroscler. (2020) 22:1107. doi: 10.1007/s11883-020-00892-2
149. Ruggiero C, Cherubini A, Guralnik J, Semba R, Maggio M, Ling S, et al. The interplay between uric acid and antioxidants in relation to physical function in older persons. J Am Geriatr Soc. (2007) 55:1206–15. doi: 10.1111/j.1532-5415.2007.01260.x
150. Raygan F, Ostadmohammadi V, Asemi Z. The effects of probiotic and selenium co-supplementation on mental health parameters and metabolic profiles in type 2 diabetic patients with coronary heart disease: a randomized, double-blind, placebo-controlled trial. Clin Nutr. (2019) 38:1594–8. doi: 10.1016/j.clnu.2018.07.017
151. Krausova G, Kana A, Vecka M, Hyrslova I, Stankova B, Kantorova V, et al. In vivo bioavailability of selenium in selenium-enriched Streptococcus thermophilus and Enterococcus faecium in CD IGS rats. Antioxidants. (2021) 10:463. doi: 10.3390/antiox10030463
152. Zeng J, Zhou J, Huang K. Effect of selenium on pancreatic proinflammatory cytokines in streptozotocin-induced diabetic mice. J Nutr Biochem. (2009) 20:530–6. doi: 10.1016/j.jnutbio.2008.05.012
153. Markowiak-Kopeć P, Slizewska K. The effect of probiotics on the production of short-chain fatty acids by human intestinal microbiome. Nutrients. (2020) 12:1107. doi: 10.3390/nu12041107
154. Cani PD, Amar J, Iglesias MA, Poggi M, Knauf C, Bastelica D, et al. Metabolic endotoxemia initiates obesity and insulin. Diabetes. (2007) 56:1761–72. doi: 10.2337/db06-1491
155. Schwiertz A, Taras D, Schäfer K. Al. E. Microbiota and SCFA in lean and overweight healthy subjects. Obesity. (2010) 18:190–5. doi: 10.1038/oby.2009.167
156. Samah S, Ramasamy K, Lim S, Neoh C. Probiotics for the management of type 2 diabetes mellitus: a systematic review and meta-analysis. Diabetes Res Clin Pr. (2016) 118:172–82. doi: 10.1016/j.diabres.2016.06.014
157. Zhao D, Zhu H, Gao F, Qian Z, Mao W, Yin Y, et al. Antidiabetic effects of selenium-enriched Bifidobacterium longum DD98 in type 2 diabetes model of mice. Food Funct. (2020) 11:6528–41. doi: 10.1039/D0FO00180E
158. Gao Z, Yin J, Zhang J, Ward RE, Martin RJ, Lefevre M, et al. Butyrate improves insulin sensitivity and increases energy expenditure in mice. Diabetes. (2009) 58:1509–17. doi: 10.2337/db08-1637
159. Li X, Xu Q, Jiang T, Fang S, Wang S, Zhao J, et al. A comparative study of the antidiabetic effects exerted by live and dead multi-strain probiotics in the type 2 diabetes model of mice. Food Funct. (2016) 7:4851–60. doi: 10.1039/C6FO01147K
160. Gangadoo S, Dinev I, Chapman J, Hughes RJ, Van T, Moore RJ, et al. Selenium nanoparticles in poultry feed modify gut microbiota and increase abundance of Faecalibacterium prausnitzii. Appl Microbiol Biotechnol. (2018) 102:1455–66. doi: 10.1007/s00253-017-8688-4
161. Yadav H, Lee JH, Lloyd J, Walter P, Rane SG. Beneficial metabolic effects of a probiotic via butyrate-induced GLP-1 hormone secretion. J Biol Chem. (2013) 288:25088–97. doi: 10.1074/jbc.M113.452516
162. Marietta E, Horwath I, Taneja V. Microbiome, immunomodulation, and the neuronal system. Neurotherapeutics. (2018) 15:23–30. doi: 10.1007/s13311-017-0601-4
163. Takata K, Tomita T, Okuno T, Kinoshita M, Koda T, Honorat JA, et al. Dietary yeasts reduce inflammation in central nerve system via microflora. Ann Clin Transl Neurol. (2015) 2:56–66. doi: 10.1002/acn3.153
164. He B, Hoang TK, Tian X, Taylor CM, Blanchard E, Luo M, et al. Lactobacillus reuteri reduces the severity of experimental autoimmune encephalomyelitis in mice by modulating gut microbiota. Front Immunol. (2019) 10:385. doi: 10.3389/fimmu.2019.00385
165. Lopert P, Day BJ, Patel M. Thioredoxin reductase deficiency potentiates oxidative stress, mitochondrial dysfunction and cell death in dopaminergic cells. PLoS ONE. (2012) 7:e50683. doi: 10.1371/journal.pone.0050683
166. de Campos Fraga-Silva TF, Mimura L, de Oliveira L, Dos Santos Toledo JH, Borim PA, Zorzella-Pezavento, et al. Selenization of S. cerevisiae increases its protective potential in experimental autoimmune encephalomyelitis by triggering an intestinal immunomodulatory loop. Sci Rep. (2020) 10:22190. doi: 10.1038/s41598-020-79102-7
167. Zhang ZH, Wen L, Wu QY, Chen C, Zheng R, Liu Q, et al. Long-term dietary supplementation with selenium-enriched yeast improves cognitive impairment, reverses synaptic deficits, and mitigates tau pathology in a triple transgenic mouse model of Alzheimer's disease. J Agric Food Chem. (2017) 65:4970–9. doi: 10.1021/acs.jafc.7b01465
168. Tamtajia O, Heidari-soureshjanib R, Mirhosseinic N, Kouchakiad E, Al E. Probiotic and selenium co-supplementation, and the effects on clinical, metabolic and genetic status in Alzheimer's disease: a randomized, double-blind, controlled trial. Clin Nutr. (2019) 38:2569–75. doi: 10.1016/j.clnu.2018.11.034
169. Zhai Q, Xiao Y, Li P, Tian F, Zhao J, Zhang H, et al. Varied doses and chemical forms of selenium supplementation differentially affect mouse intestinal physiology. Food Funct. (2019) 10:5398–412. doi: 10.1039/c9fo00278b
170. Kim J, Combs GF. Effects of selenium on colonic fermentation in the rat. Biol Trace Elem Res. (1997) 56:215–24. doi: 10.1007/BF02785394
171. Hills RJ, Pontefract B, Mishcon H, Black C, Sutton S, Theberge C. Gut microbiome: profound implications for diet and disease. Nutrients. (2019) 11:1613. doi: 10.3390/nu11071613
172. Henrick B, Hutton A, Palumbo M, Casaburi G, Mitchell R, Underwood M, et al. Elevated fecal pH indicates a profound change in the breastfed infant gut microbiome due to reduction of Bifidobacterium over the past century. mSphere. (2018) 3:e00041–18. doi: 10.1128/mSphere.00041-18
173. Cao C, Fan R, Zhao J, Zhao X, Yang J, Zhang Z, et al. Impact of 761 exudative diathesis induced by selenium deficiency on LncRNAs and their 762 roles in the oxidative reduction process in broiler chick veins. Oncotarget. (2017) 763:20695–705. doi: 10.18632/oncotarget
174. Woldeamlak B, Yirdaw K, Biadgo B. Role of gut microbiota in type 2 diabetes mellitus and its complications: novel insights and potential intervention strategies. Gastroenterology. (2019) 74:314–20. doi: 10.4166/kjg.2019.74.6.314
175. Franzosa EA, Sirota-Madi A, Avila-Pacheco J, Fornelos N, Al E. Gut microbiome structure and metabolic activity in inflammatory bowel disease. Nat Microbiol. (2019) 4:293–305. doi: 10.1038/s41564-018-0306-4
176. Nido S, Shituleni S, Mengistu B, Liu Y, Khan A, Gan F, et al. Effects of selenium-enriched probiotics on lipid metabolism, antioxidative status, histopathological lesions, and related gene expression in mice fed a high fat diet. Biol Trace Elem Res. (2016) 171:399–409. doi: 10.1007/s12011-015-0552-8
177. Liu Y, Liu Q, Ye G, Khan A, Liu J, Gan F, et al. Protective effects of selenium-enriched probiotics on carbon tetrachloride-induced liver fibrosis in rats. J Agric Food Chem. (2015) 63:242–9. doi: 10.1021/jf5039184
Keywords: selenium, gut microbiota, selenocompounds, selenoproteins, selenium metabolism
Citation: Ferreira RLU, Sena-Evangelista KCM, de Azevedo EP, Pinheiro FI, Cobucci RN and Pedrosa LFC (2021) Selenium in Human Health and Gut Microflora: Bioavailability of Selenocompounds and Relationship With Diseases. Front. Nutr. 8:685317. doi: 10.3389/fnut.2021.685317
Received: 24 March 2021; Accepted: 11 May 2021;
Published: 04 June 2021.
Edited by:
Lucia A. Seale, University of Hawaii, United StatesCopyright © 2021 Ferreira, Sena-Evangelista, de Azevedo, Pinheiro, Cobucci and Pedrosa. This is an open-access article distributed under the terms of the Creative Commons Attribution License (CC BY). The use, distribution or reproduction in other forums is permitted, provided the original author(s) and the copyright owner(s) are credited and that the original publication in this journal is cited, in accordance with accepted academic practice. No use, distribution or reproduction is permitted which does not comply with these terms.
*Correspondence: Lucia Fatima Campos Pedrosa, bGZjcGVkcm9zYUBnbWFpbC5jb20=
Disclaimer: All claims expressed in this article are solely those of the authors and do not necessarily represent those of their affiliated organizations, or those of the publisher, the editors and the reviewers. Any product that may be evaluated in this article or claim that may be made by its manufacturer is not guaranteed or endorsed by the publisher.
Research integrity at Frontiers
Learn more about the work of our research integrity team to safeguard the quality of each article we publish.