- State Key Laboratory of Dairy Biotechnology, Shanghai Engineering Research Center of Dairy Biotechnology, Dairy Research Institute, Bright Dairy & Food Co., Ltd., Shanghai, China
Red-Veined Cheese is an experimental internal mold-ripened cheese using red koji powder as the red starter. The objective of this study was to characterize the physicochemical parameters, microorganisms, proteolysis, lipolysis, and volatile profile of the cheese during 33 days of ripening. The gross composition was 56.7% (w/w) for total solids, 45.8% (w/w) for fat-in-dry matter, 2.58% (w/w) for salt content and 37.8% (w/w) for protein. The pH increased from 4.88 to 5.23 during ripening. The Monascus density first rose and then fell, while total mesophilic bacteria count declined steadily throughout the maturation. Proteolysis degree in experimental group was significantly higher than in control group without inoculation of red koji powder. Analysis of the fatty acid profile showed that the internal Monascus-fermentation also promoted lipolysis. A total of 63 volatile compounds, including 12 ketones, 14 alcohols, 15 acids, 13 esters, 5 aldehydes, 3 lactones and 1 phenol, were identified by gas chromatography-mass spectrometry (GC–MS) coupled with headspace solid-phase microextraction (HS-SPME). The main chemical groups of volatile compounds were ketones, alcohols, acids and esters whereas aldehydes, lactones, and phenols represented only minor components. At the end of ripening, the levels of ketones, alcohols, esters were significantly higher in the experimental cheese compared with the control. Our results therefore show that the internal Monascus-fermentation is not only a suitable technology to form red veins in the paste but also an effective method to impact the composition of volatile compounds in cheese, which can thus distinguish it from other internal mold-ripened cheeses and surface-ripened Monascus-fermented cheese.
Introduction
Cheese is one of the most diverse varieties of dairy products. It has become an important contributor to nutritional intake in public health interests because it contains abundant nutrients for the human body including proteins, fat, bioactive peptides, amino acids, free fatty acids and etc (1, 2). With growing public awareness of health, consumer demand for functional dairy products put forward higher requirements. There is an urgent need to develop healthier, more diversified new cheese products to meet the market demand.
Red koji-mold (Monascus sp.) is a well-known industrially mold that produces valuable metabolites such as lovastatin, γ-aminobutyric acid, polysaccharides and etc. (3). Strains belonging to this genera have a time-honored history of being used to prepare fermented foods before they were found to be an important source of various functional compounds (4). Red koji refers to solid fermenting steamed rice with red koji-mold, it is the first Monascus-fermented food. Meanwhile, it is an important ingredient of Asian fermented foods. Traditionally, red koji was used as the red starter to prepare several fermented foods including red-koji wine, vinegar and furu (4), now it is also be used as an alternative to statins for the treatment of dyslipidemia (5).
Monascus-fermented foods have become increasingly popular worldwide (3). However, to date, there are still few cases of applying this fungi genus in cheesemaking. At first, Lorrungrua et al. (6) investigated the possibility of cheese production from soymilk through a combination of Lactobacillus casei and Monascus purpureus. Yu et al. (7) mixed M. purpureus with Penicillium and Geotrichum molds for the ripening of surface-ripened cheese. Wu et al. (8) reported a surface-ripened cheese using Monascus fermentation broth as inoculum.
As far as we know, there is no information available on the development of internal mold-ripened cheese using red koji powder as the sole mold starter, which deserves interest in terms of either its unique creative or potential market value. In this study, we successfully developed a novel internal Monascus-ripened variety, called it Red-Veined Cheese. Its unique technological feature is represented by the sandwich inoculation method of red koji powder during molding.
We aim was to present the details of the manufacturing technology of Red-Veined Cheese, followed by a demonstration of the character portrait of the cheese, mainly focusing on the gross compositional and microbiological status, biochemistry changes, and volatile profile during ripening. The information obtained from this study, can not only contribute to the biochemical knowledge of Monascus-fermented cheese but also help to further improve the quality of this new cheese variety.
Materials and Methods
Materials
Raw whole cow's milk was from Bright Dairy & Food Co., Ltd. (Shanghai, China). Marzyme® 150 MG rennet was supplied by DuPont Danisco (Shanghai, China). Plate count agar and YGC medium were purchased from Qingdao Rishui Biological Technology Co., Ltd. (Qingdao, China). All other chemicals were of analytical grade and purchased from Sigma-Aldrich (Shanghai, China). Monascus purpureus GL-1 (CGMCC No.7603) is stored in China General Microbiological Culture Collection Center (Beijing, China). Flora Danica, a freeze-dried commercial lactic bacteria starter, containing strains Lactococcus lactis ssp. cremoris, Lactococcus lactis ssp. lactis, Lactococcus lactis ssp. diacetylactis and Leuconostoc mesenteroides spp. cremoris, was provided by Chr. Hansen (Denmark).
Preparation of Red Koji Powder
M. purpureus GL-1 was used to prepare red koji powder according to the solid-state fermentation method (9) using rice as the substrate. Experiments were conducted in 250 ml Erlenmeyer flask containing 5 g substrate (powdered rice). The substrate was moistened with salt solution and distilled water in such a way as to obtain Wnal moisture content of 60%. After thorough mixing, the wet substrates were autoclaved at 121°C for 20 min and cooled to room temperature. M. purpureus GL-1 was incubated at 30°C for 7 days and then grinded under sterile conditions. Red koji powder obtained (1.8 × 105 spores/g) was used as the red starter.
Cheese-Making and Sampling
Seven replicas of the experimental cheeses were manufactured from ~30 liters of whole milk which was supplied by a farm of the Bright Dairy and Food Co., Ltd. according to the procedure described in Figure 1. The milk was pasteurized at 73°C for 40 s. Then, calcium chloride solution (final concentration of 9 g/100 mL of milk) and Flora Danica starter (3 g/100 L, Danisco Co. Ltd.) was added to milk at 32°C for the pre-maturation. After ~30 min (acidification up to 20°D), Marzyme® 150 MG rennet was added at a ratio of 4 g/100 L of milk. After 1.5 h, the curd was cut into hazelnut size and was stirred at ~32°C for 20 min. Then 0.2 g of red koji powder was added to each mold (diameter 11 cm, height 11 cm), more concretely: (1) transferring a layer of curd (~2.0 cm thick) to the cylindrical mold; (2) 0.05 g red koji powder was paved evenly on the surface of the curd layer, and a distance of ~1 cm from the edge of the mold was maintained; (3) transferring another layer of curd to the mold; (4) repeating step (2) and (3) until the mold was almost filled up; (5) adding the final layer of curd to encapsulate the whole cheese. After this time, the curd stayed in the molds without pressure for 12 h at 20–22°C, then was dry salted on the surface, dried in a sterile incubator and aged according to the process flow diagram shown in Figure 1. Each sampling point was made up of one of the whole replica (350 g on average) mashed after peeling and stored at −20°C before analysis. Seven replicas without inoculation of red koji powder served as the control. Strict hygienic conditions were maintained throughout the entire cheesemaking process.
Physicochemical Parameter Analysis
Total solids, protein, fat, salt and pH were determined according to the method described by (10). All determinations were made in duplicate.
Microbiological Analysis
Ten grams of cheese were, aseptically sampled, mixed in 90 mL of sterile 0.9% (w/v) NaCl solution. Serial decimal dilutions were prepared in the same diluent. Total mesophilic bacteria (TMB) count on PCA, incubated at 30°C for 3 days. M.purpureus GL-1 counts were enumerated on potato dextrose agar (PDA) after incubation at 25°C for 5 days.
Nitrogen Fraction Analysis
Water-soluble nitrogen (WSN), 12% trichloroacetic acid-soluble nitrogen (TCA-SN) and 5% phosphotungstic acid-soluble nitrogen (PTA-SN) of cheeses were determined as described by Bütikofer et al. (11). Free amino acids (FAA) were analyzed according to the method of Yu et al. (7). All analyses were performed in duplicate.
Analysis of Free Fatty Acids
Free fatty acids (FFAs) of cheese samples were extracted and esterified to methyl esters according to the method described by Felicio et al. (12). The fatty acid methyl esters were quantified by gas chromatography using an Agilent model 7890A-5975C gas chromatograph (Santa Clara, CA, USA) equipped with a flame ionization detector (FID), split injection (1:100). The column used was DB-5 (30 m × 0.25 mm × 0.25 μm i.d). The initial column temperature was programmed to 70°C for 5 min, then rising to 200°C at 25°C/min followed by an increase to 240°C at 2°C/min, then up to 300°C at 20°C/min and remaining at this temperature for 7 min. The carrier gas was helium at a flow rate of 1 mL/min. The split was 1/5, the injection volume 1 μL. The quantification of the methylated samples was made by comparison of their retention times with those of the respective standards (Sigma-Aldrich). The result was expressed as mg FFA/100 g of cheese. All analyses were conducted in duplicate.
HS-SPME Gas Chromatography Mass Spectrometry
Volatile compound extraction was carried out using 5 g of grated cheese mixed with 8 mL NaH2PO4 solution (25%, w/v) in a 20 mL glass vial sealed with a screw cap with a PTFE-silicon septum according to the method of Mei et al. (13) with modification. Vials were then incubated at 50°C for 45 min. During the first 15 min of incubation, volatiles were allowed to be collected in the headspace and then extracted during the following 30 min using a 50/30 μm divinylbenzene/carboxen/polydimethylsiloxane (DVB/CAR/PDMS) fiber. The fiber was kept for 4 min in the injector at 260°C for desorption of volatiles then analyses were performed on a 7890A-5975C GC-MS system (Agilent Technologies, Santa Clara, CA, USA) equipped with a DB-WAX capillary column (30 m × 0.25 mm × 0.25 μm) (Agilent J &W Scientific, Folsom, CA). The oven temperature was held at 40°C for 5 min, then programmed to 250°C at 5°C/min and held at 250°C for 5 min. Helium was used as a carrier gas at a flow rate of 1 mL/min. The MS temperatures adopted were as follows: interface 280°C, source 230°C, quadrupole 150°C; the acquisition was performed in electron impact mode at 70 eV with 0.7 scans per second and the mass range used was m/z 20–400. Compounds identification was performed by comparison of mass spectra with the data from the NIST 2011 library. Linear retention indexes were also compared with bibliographic data (14). All samples were analyzed in duplicate.
Sensory Analysis
Sensory evaluation of the cheese samples (day 5, 19, and 33 of ripening) and commercial blue cheese (president brand) was carried out according to a previous study (15) with modification. Twelve assessors were recruited and trained. Panelists gave a score between 0 (no perception) and 10 (strong perception) for each attribute, on a structured scale. Cubes of cheese core (roughly 1.5 cm3) were used to test for creamy, acidity, odor, salty, bitter and mushroom.
Statistical Analysis
Means of each group were compared by one-way analysis of variance (ANOVA) followed by Tukey's test (p < 0.05). Comparisons of groups used the unpaired two-tailed t-test at a threshold of 5%. The relationship between variables in volatiles was assessed by the principal component analysis (PCA). Correlation between variables was determined by using the Pearson correlation coefficient with a p-value < 0.05 considered as significant. Statistics were run using SPSS version 22 software (SPSS, Inc., Chicago, IL).
Results and Discussion
Physicochemical and Microbial Parameters
Cheese physicochemical properties and microbial data during 33 days of ripening are summarized in Table 1. The total solids (TS) of the experimental cheese increased (p < 0.001) throughout ripening, finally reaching 56.7 ± 0.4 g/100 g of cheese, which was higher than those reported for Blue Cheese (16), blue-type cheeses (17) and camembert cheese (18).
Protein and fat showed no significant changes (p > 0.05) during maturation. The protein value (around 37.8 g/100 g TS) was in the range of data reported for other internal mold-ripened cheeses (16, 17, 19, 20) and almost overlapping levels measured in Danablu (37.4 g/100 g TS) and Stilton (37.9 g/100 g TS) (16). The fat level (45.8 g/100 g TS on average) was close to that of camembert-type cheese (18), but lower than in blue-veined varieties (16, 17).
As observed in other internal mold-ripened cheeses (19–21), pH neutralization process also occurred during the ripening of cheese samples. The pH value increased steadily over the course of maturation, this is mainly due to lactic acid consumption by the mold and release of ammonia and amines associated with deamination and decarboxylation of free amino acids (22). The pH value (5.22) at the end of ripening was close to that reported in Danablu and Huntsman varieties (pH = 5.3) and lower than levels recorded in other blue-veined cheeses (16, 17).
NaCl concentration increased progressively in the core part may result from the slow diffusion of salt from the surface to the center of the paste (23). The cheese sample aged 33 days showed a salt concentration (2.58 g/100 g TS) and can be classified as high-sodium cheese (24), but the value is less than halved in comparison with values reported for blue cheeses in the literature (16, 17).
The total mesophilic bacteria (TMB) count decreased from 8.42 log CFU/g of cheese on day 0–6.74 log CFU/g of cheese on day 33. A decreasing trend was similarly observed in the control group. However, after day 19, the number of TAMB was approximately an order of magnitude lower than that observed in the experimental cheese (Table 1).
The rapid increase of Monascus density within the first 5 days was due, firstly, to the conditions used (28°C, 85% relative humidity) which favors Monascus growth, and secondly, to the large number of voids distributed internally which offered enough space for mycelium growth. On the other hand, needle puncture also boosted oxygen supply and can help to distribute the mold spores throughout the paste. The Monascus density increased from 2.06 log CFU/g of cheese on day 0 up to 4.99 log units on day 5 (Table 1). After day 3, red veins can be visually observed inside the paste.
Proteolysis
Evolution of different nitrogen fractions during the maturation of Red-Veined Cheese is shown in Table 2. Water-soluble nitrogen (WSN) is an important indicator to evaluate the primary proteolysis of cheese (19). Its value increased from 6.96% (on TN) at day 0–9.67% (on TN) at day 33 and remained at a higher level throughout the ripening process as compared with the control without Monascus inoculation.
TCA-SN fraction, as an index of secondary proteolysis, contains short peptides and FAAs (25). After 12 days of ripening, TCA-SN further increased in the experimental cheese. At day 33, the value has increased by 70% of its initial value. TCA-SN development was found positively correlated with the Monascus density (r = 0.798, p < 0.05), indicating the contribution of Monascus fermentation in the paste to secondary proteolysis of the cheese.
As shown in the table, a significant accumulation of PTA-SN fraction throughout the maturation [from 0.81 ± 0.02% (on TN) at day 0 to 4.94 ± 0.23% (on TN) at day 33] was accompanied by a dramatic enhance in the proportion of PTA-SN to WSN (from 11.6 to 51.1%). Meanwhile, the ratio of PTA-SN (expressed as % TCA-SN) also increased from 19.6% at the beginning to 69.2% at the end of the experiment, demonstrating balance shifting between primary and secondary proteolysis in which the latter gradually predominated. At the end of maturation, PTA-SN accumulation increased 1.8-fold compared to the control.
As expected, the experimental cheese displayed enhanced proteolysis compared with the control. Since the evolution of nitrogen fractions during maturation was mainly attributed to the microbial peptidase activity. The increase in the rate of proteolysis could be probably related to the metabolic activity of M. purpureus GL-1. Additionally, a relatively higher load of lactic acid bacteria in the experimental cheese at the later stage of ripening may also help to promote proteolysis of the cheese.
Figure 2 shows the free amino acid (FAA) profiles during cheese ripening. Most of FAAs displayed increasing trends in the experimental group (Figure 2B). Phe and Pro were decreased with time, possibly because they were consumed by the mold or degraded to amines and other FAAs through the decarboxylation and deamination (26). At day 33, concentrations of Glu, Lys, His, Ser, Tyr, Ile, and Arg were significantly higher (p < 0.01) than that of the control (Figure 2A). The most abundant FAAs at all stages of ripening were Glu, Leu and Tyr, which together accounted for nearly 60% of the total FAA in the experimental cheese, whereas their percent contribution in blue cheese is only ~40% (27). Thr, Ser, Gly, Cys, Val, Met, His, Arg, and Pro make up 23% of the total FAA content in the ripened experimental cheese, while this ratio is over 40% in blue-veined varieties (27). This indicates that there is significant discrimination between Red-Veined Cheese and traditional internal mold-ripened cheese in the FAA profile.
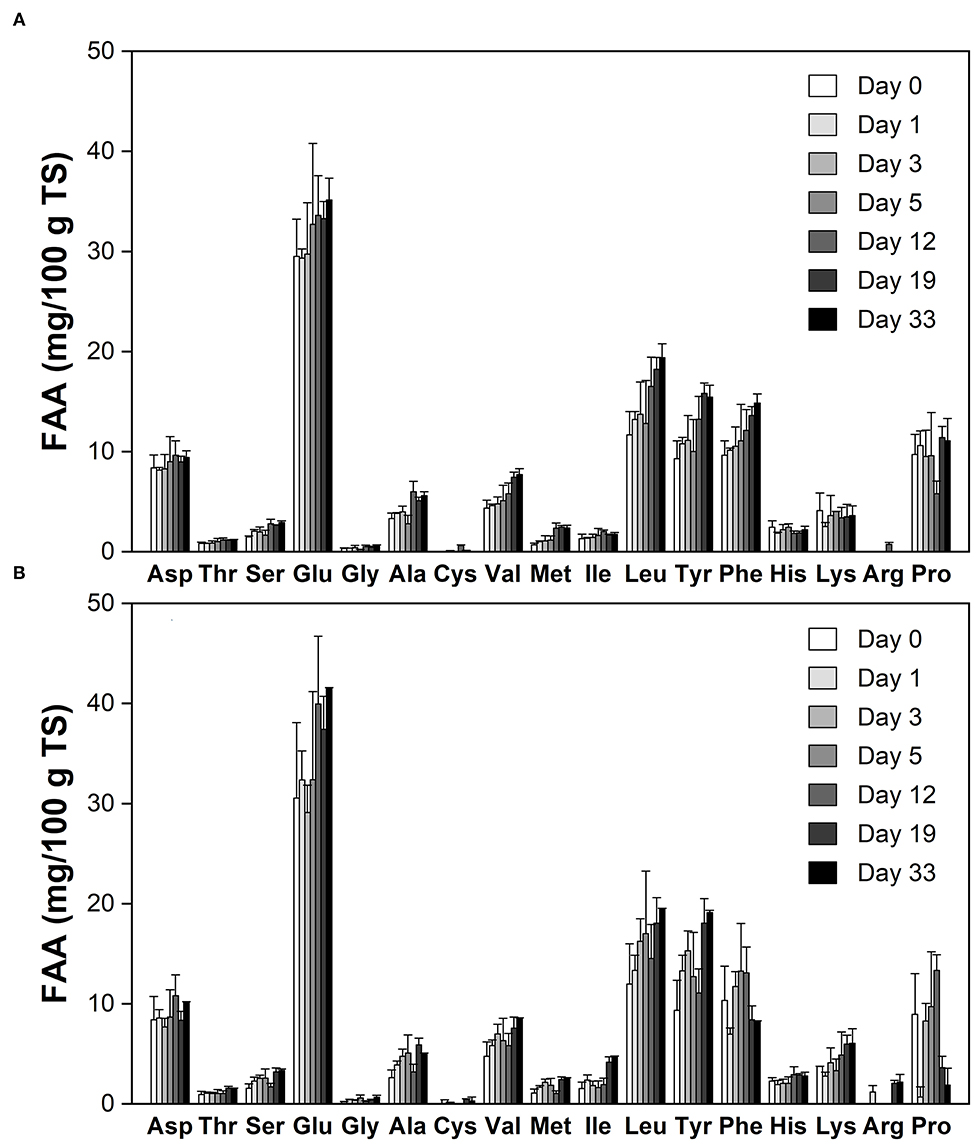
Figure 2. Free amino acid (FAA) profiles during cheese ripening. (A) The control without inoculation of red koji powder; (B) Red-Veined Cheese. Error bars indicate standard deviations from the mean.
The total FAA remained at a low level until the end of ripening (Table 2), indicating that one month's maturity might have been too short compared with that of blue-veined varieties (21, 28, 29) to promote great differences in proteolysis degree.
Lipolysis
Changes in fatty acid composition of cheese during 33 days of ripening are summarized in Table 3. The total FFA content of the experimental cheese nearly doubled throughout the maturation, indicating that the cheese went through substantial lipolysis during ripening. At day 33, palmitic acid (C16 : 0) is the most abundant in the ripened experimental cheese, followed by oleic acid (C18 : 1), accounting together for 52.3% of total free fatty acids (TFFA). Compared with the control, long-chain saturated fatty acids (LCSFA) decreased by 7%, while long-chain unsaturated fatty acids (LCUSFA) and Short-chain fatty acids (SCFA) increased by 45 and 12%, respectively.
Changes in the relative abundance of free fatty acids (FFAs) with different chain lengths and saturations are shown in Figure 3. It can be seen that the mass fractions did not change for FFAs with varying chain lengths within the first 5 days. Over the remainder of the maturation period, the fraction of LCSFA decreased from 62 to 51% whereas the proportion of LCUFA increased from 36 to 44%. In contrast, similar changes were not observed in the control group. This difference could be due at least in part to the metabolic activity of M. purpureus GL-1. For the SCFA fraction, its proportion increased progressively over time, with similar trends seen in both groups. At day 33, SCFA only represented ~5% of Total FFA in the experimental cheese, which may be due to their consumption by the mold, or more likely, converted to other volatile compounds to contribute to cheese flavor (30).
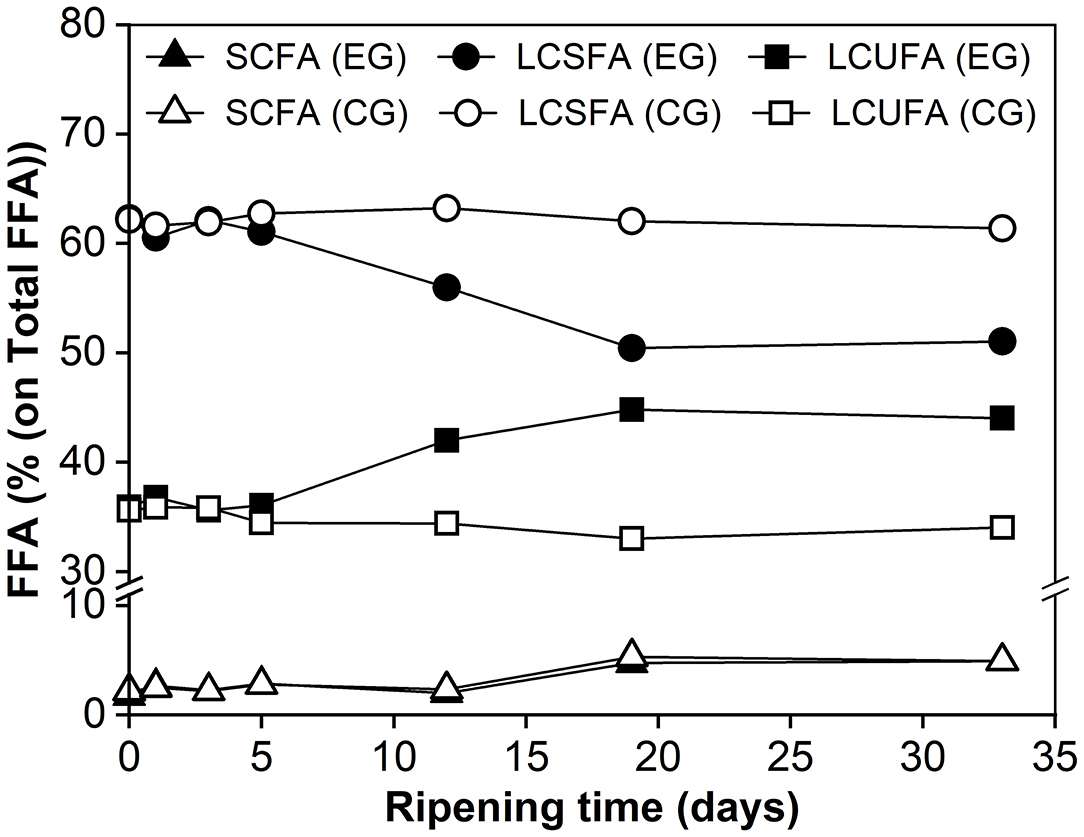
Figure 3. The relative abundance of free fatty acids (FFAs) categorized based on chain length and saturation degree during cheese ripening. SCFA, short-chain fatty acids (C6 : 0, C8 : 0, C10 : 0); LCSFA, long-chain saturated fatty acids (C12 : 0, C14 : 0, C16 : 0 and C18 : 0); LCUFA, long-chain unsaturated fatty acids (C16 : 1, C18 : 1, C18 : 2); EG, experimental group; CG, control group without inoculation of red koji powder.
The Total FFA content reached a final amount of 1469.19 mg/100 g of cheese (Table 3), which was higher than those of Camembert (681–5,066 mg/kg of cheese) and Brie (2,678 mg/kg of cheese), but lower than those reported for blue cheese (over 26 g/kg of cheese). The latter might be because the maturation takes a much shorter time than blue cheese. Additionally, the percentage of unsaturated fatty acids (44%) was higher in the ripened experimental cheese than in traditional internal mold-ripened and surface-ripened cheeses (30). At the late stage of ripening, the total FFA content showed a significantly higher level (p < 0.05) in the experimental group, suggesting that internal Monascus-fermentation did promote cheese lipolysis.
Volatile Compounds
Table 4 summarizes 63 volatile compounds identified during the ripening of Red-Veined Cheese by the HS-SPM-GC-MS method, including 12 Ketones, 14 alcohols, 15 acids, 13 esters and 5 aldehydes, 3 lactones and 1 phenol.
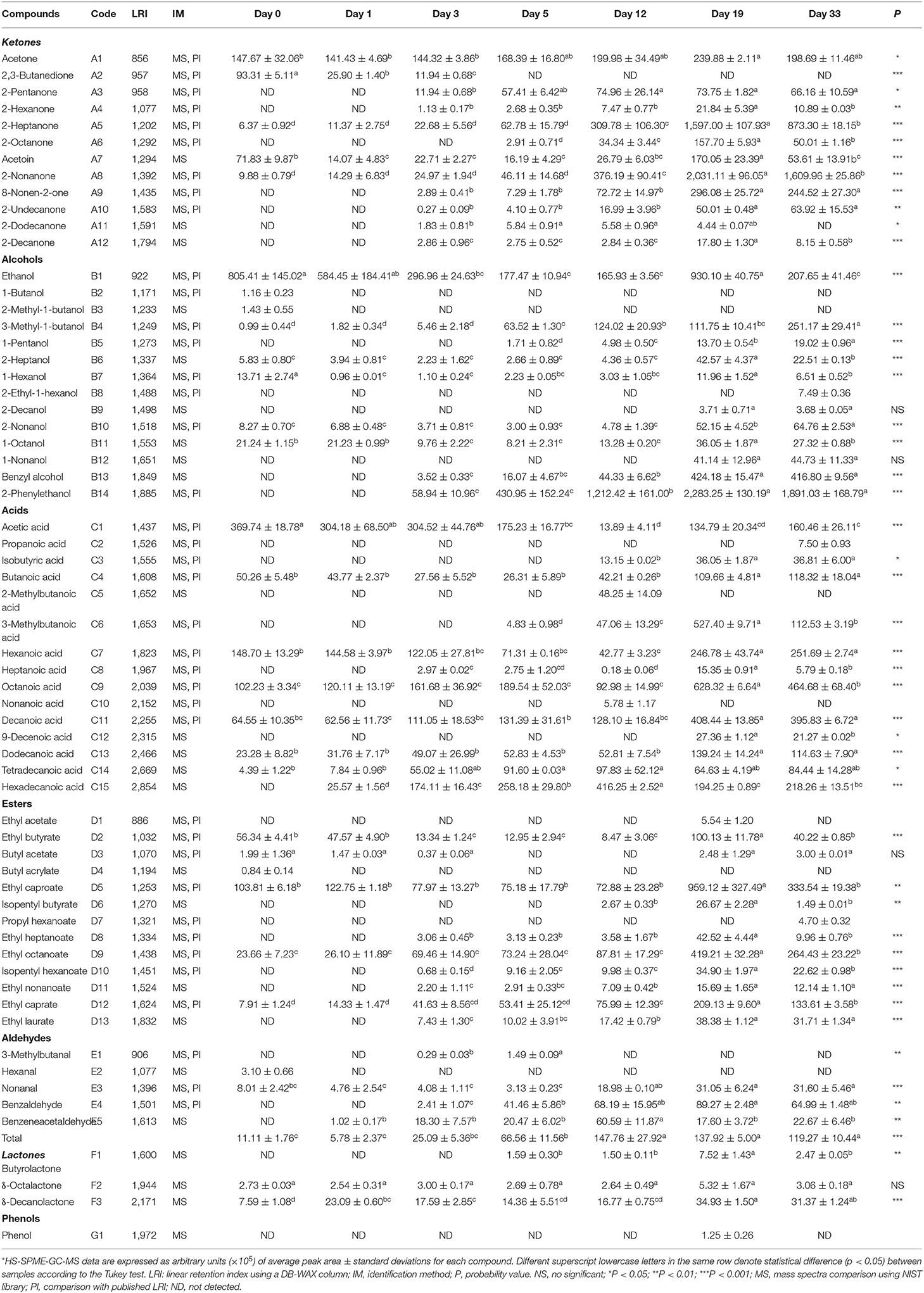
Table 4. Volatile compounds identified in Red-Veined Cheese by HS-SPME-GC-MS during the different ripening stages.
Ketones
Ketones consisted of 12 components. The majority being methyl ketones. At the end of ripening, the GC-MS total peak area of this chemical group accounted for 35% of all volatiles. The most abundant 2-alkanones were the ones with an odd number of carbon atoms. Among which, 2-nonanone was the most abundant compound, next is 2-heptanone, together representing ~80% of total ketones. In blue cheeses, they are characteristic flavor components which are formed during enzymatic lipolysis of FFAs (31). Although ketones was the most abundant group in the ripened experimental cheese, its percentage in neutral volatiles (38%) is significantly lower than that observed in blue cheeses (50–70%) (32, 33).
Alcohols
Alcohols became the second abundant flavor component after 2 weeks, accounting for more than 30% of the total volatiles. Most of them belong to primary alcohols. Secondary alcohols detected (from C3 to C9) in Red-Veined Cheese are typical flavor alcohols in blue cheese (34). 2-alkanols (i.e., 2-heptanol, 2-nonanol and 2-decanol), which are also important for blue cheese flavor, were only minor components in this cheese. Moreover, the proportion of alcohols in neutral volatiles (41.4%) was significantly higher in the ripened cheese than in French blue-veined varieties (<30%) (33).
3-Methyl-1-butanol, typical methyl alcohol in blue cheese deriving from the metabolism of leucine (35), was also detected in the experimental cheese. But it was not found in the control group (Supplementary Table 1). At day 33, 3-methyl-1-butanol represented 8.5% of total alcohols, a value equal to that of Gorgonzola (32), higher than Bleu des Causses (3%), but significantly lower than those measured for Bleu d'Auvergne (39%) and Roquefort (33–44%) (33).
Two aromatic alcohols (2-phenylethanol and benzyl alcohol) accumulated in the experimental cheese over time. Their concentrations increased by 118 and 32 times within 1 month, respectively (Table 4). In comparison, they were almost undetectable in the control throughout the entire maturation (Supplementary Table 1). 2-Phenylethanol is one of the most odorous alcohols in camembert and cheddar and can give cheese a very pleasant rose-like fragrance. Its appearance was previously reported to be related to the transformation of phenylalanine by yeasts (18). However, in this study, yeasts were not detected in any cheese samples. Since the control cheese was deficient in these two aromatic alcohols, the reason for their accumulation in the experimental cheese might be owed to the metabolic activity of M. purpureus GL-1. Because of their accumulation, Red-Veined Cheese showed a distinctive alcohol profile, which is characterized by a fairly high level of aromatic alcohols (77.9% of total alcohol) and a low level of 2-alkanol (3.1%). In addition, we also noticed that 2-phenylethanol and benzyl alcohol were nearly undetectable in the surface-ripened MC over the course of maturation (8). This suggests that the internal fermentation of M. purpureus GL-1 could be the main reason for the accumulation of these two aromatic alcohols.
Acids
A total of 15 acids were identified during the maturation period. Most of which are n-alkanoic acids. By the end of ripening, the content of acids increased 2.6 times and represented 21.8% of the total volatiles, which ranked behind ketones and alcohols. n-acids constituted 91.4% of the total acid peak area. Among them, the ones with even carbon atoms predominated and accounted for 90.8% of the total, while the ones with odd carbon numbers presented only in a tiny amount (0.7% of the total).
Three branched-chain acids (isobutyric acid, 2-methylbutanoic acid and 3-methylbutanoic acid) were detected during maturation. They are important flavor acids for blue cheese (32) and derive from the metabolism of valine, isoleucine and leucine, respectively (35). This suggests that amino acid metabolism is also one of the major pathways involved in flavor formation of Red-Veined Cheese. In comparison, no branched-chain acids were found in the control, indicating that Monascus fermentation may play a critical role in the formation of branched-chain acids.
Esters
Major flavor components of Red-Veined Cheese include esters (9.4% of total volatiles). Thirteen·esters were identified. The dominant ones were ethyl esters of free fatty acids. Compared with the control, the content of ethyl esters of free fatty acids (from C4 to C10) was significantly increased (p < 0.05) in the experimental cheese. Besides, we found that propyl isoamyl esters of n-acids such as isopentyl butyrate, propyl hexanoate and isopentyl hexanoate were unique to the experimental group but absent from the control (Table 4 and Supplementary Table 1). At day 33, ethyl caproate, ethyl octanoate and ethyl caprate constituted the backbone of esters profile of the experimental cheese (85.3% of total esters area), they are characteristic of mellow fruity and floral notes (32). It is reasonable to assume that these esters are also important volatiles responsible for the flavor of Red-Veined Cheese.
Other Compounds
Other groups of volatiles such as aldehydes, lactones and phenol represented minor fractions. No accumulation of aldehydes was observed during maturation, probably because they were reduced to the corresponding alcohols (36). Three aldehydes (nonanal, benzaldehyde and benzeneacetaldehyde) were identified in the experimental cheese at the end of ripening, among which, benzaldehyde was the most abundant, its concentration was 7 times that found in the control (Table 4 and Supplementary Table 1). Benzeneacetaldehyde with broom flower notes (32) existed only in the experimental cheese. Micro amounts of lactones and phenol were detected in the cheese headspace during ripening. In addition to providing creamy coconut notes and contribute to buttery feeling, δ-lactones can also help smooth overall cheese flavor by reducing the influence of sharpness of free fatty acids (37).
Sensory Analysis and Principal Component Analysis of Volatiles
As shown in Table 5, the sensory attributes of the Red-veined cheese (5, 19, and 33 days ripening) and commercial blue cheese (President Blue Cheese, from a local market) were assessed. At 19 and 33 days of storage, Red-veined cheese had a stronger mushroom note than 5 days and control 33 days. These flavor attributes had no different significantly between the 9 and 33 days of storage cheese and commercial blue cheese, but had a less odor and bitter than commercial blue cheese, which maybe related with the low level of proteolysis and lipolysis in Red-veined cheese, FAA and FFA lower than those reported for blue cheese which were mentioned above. Because The bitter taste and ammoniacal flavor and aroma, common to cheeses matured by molds, are also due to the intense proteolytic activity during the maturation process. The intense lipolytic activity also contributes greatly to the unique characteristic flavor of each type of cheese, since each resulting fatty acid has intense and different aroma and its presence speeds up the process of oxidative rancidity of lipids (38).
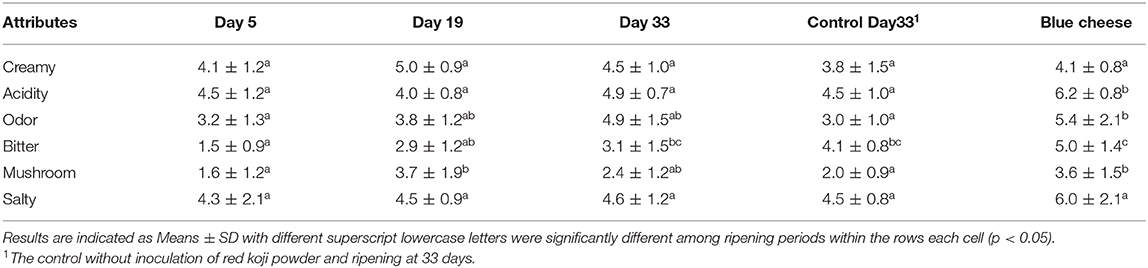
Table 5. Sensory evaluation of the Red-Veined Cheese during ripening and commercial Blue cheese (President brand).
Principal component analysis (PCA) was applied as a means to visualize any relation among the volatile data of Red-Veined Cheese. All input variables in Table 3 were included in the PCA and Biplot (score plot overlapped to loadings plot) was used because it can display relationships between volatiles/cheese samples and PCs at the same time. For clarity, only compounds contributing to the main part of data variation have been identified in Figure 4. The first two principal components (PC1 and PC2) explain 79.8% of the total variance (PC1 accounts for 61.9% and PC2 for 17.9%). The experimental cheeses with different ripen time were separated on the PC1-PC2 plane. Younger samples are located on the negative PC1, while on the positive area were more ripened ones. 0-days-old cheese sample is more correlated to 2,3-Butanedione (A2) and acetic acid (C1) compared to other samples whereas a 12-days-old sample to 2-methylbutanoic acid (C5). Most of the flavor compounds that can explain data variability are concentrated at the positive extreme of PC1, mainly composed of methyl ketones, primary branched and aromatic alcohols, FFAs and straight-chain esters. This is consistent with the fact that these compounds were mostly isolated at relatively higher concentrations from cheeses ripened for 19 and 33 days. The 19-day-old sample is typically correlated to ethyl caproate (D5), whereas the main characteristic compound of 33-day-old sample was 2-phenylethanol (B14). Exploring the area between sample points of days 19 and 33, we noticed that nearly 70% of the compounds in the figure falling into this sector characterized by floral and fruity notes, mainly including methyl ketones (2-Heptanone, 2-Octanone, 2-Nonanone, 2-Undecanone), aromatic alcohols (Benzyl alcohol, 2-Phenylethanol), ethyl esters of free fatty acids (Ethyl octanoate, Ethyl caprate) and lactone (δ-Decanolactone) (39). Their amounts began to rise sharply and far exceeded the control group after about 2 weeks of ripening.
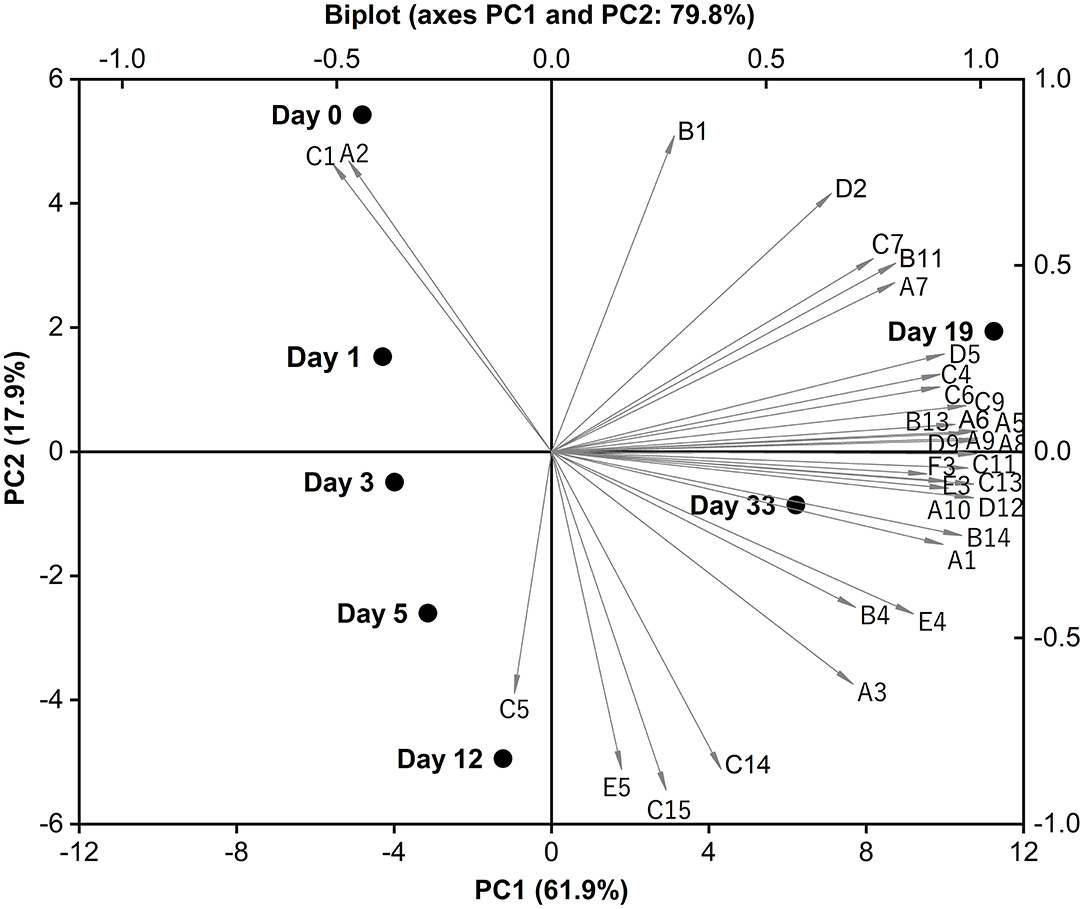
Figure 4. PCA analysis of the volatile profile of Red-Veined Cheese. References: A1 (Acetone), A2 (2,3-Butanedione), A3 (2-Pentanone), A5 (2-Heptanone), A6 (2-Octanone), A7 (Acetoin), A8 (2-Nonanone), A9 (8-Nonen-2-one), A10 (2-Undecanone), B1 (Ethanol), B4 (3-Methyl-1-butanol), B11 (1-Octanol), B13 (Benzyl alcohol), B14 (2-Phenylethanol), C1 (Acetic acid), C4 (Butanoic acid), C5 (2-Methylbutanoic acid), C6 (3-Methylbutanoic acid), C7 (Hexanoic acid), C9 (Octanoic acid), C11 (Decanoic acid), C13 (Dodecanoic acid), C14 (Tetradecanoic acid), C15 (Palmitic acid), D2 (Ethyl butyrate), D5 (Ethyl caproate), D9 (Ethyl octanoate), D12 (Ethyl caprate), E3 (Nonanal), E4 (Benzaldehyde), E5 (Benzeneacetaldehyde), F3 (δ-Decanolactone).
Overall, the experimental cheese was richer in volatiles. More than a quarter of compounds identified were not found in the control group (Table 4 and Supplementary Table 1). They include 3 ketones (2-pentanone, 2-hexanone,8-nonen-2-one), 3 alcohols (2-methyl-1-butanol, 3-methyl-1-butanol, benzyl alcohol), 5 acids (propanoic acid, isobutyric acid, 2-methylbutanoic acid, 3-methylbutanoic acid, nonanoic acid), 4 esters (isopentyl butyrate, propyl hexanoate, isopentyl hexanoate, ethyl nonanoate) and 1 aldehyde (benzeneacetaldehyde). Among which, benzyl alcohol and benzeneacetaldehyde impart cheese a rosy note, esters of n-acids give a sweet fruity scent, while almost all branched FFAs with sweaty smell disappeared at the end of ripening. At day 33, ten substances in the experimental group exhibited significantly higher concentrations compared with the control (p < 0.001), including aromatic alcohol and aldehyde (2-phenylethanol, benzaldehyde), methyl ketones (heptanone, 2-octanone, 2-nonanone, and 2-undecanone) and straight-chain esters (ethyl caproate, ethyl octanoate, ethyl caprate, and ethyl laurate). Most of them provide fruity notes. Of particular note, 2-phenylethanol, flavor alcohol with rosy aroma (39), accumulated extensively in the experimental cheese with the extension of ripening time. But it was almost undetectable in the control group. More than that, 2-phenylethanol was also undetected in surface-ripened MC (8). This indicates that the accumulation of this aromatic alcohol was associated not only with the metabolic activity of M. purpureus GL-1 but also with the growth position of the mold mycelia. During the 33 days of ripening, the total peak area of all volatiles in the experimental cheese had increased about 4-fold and finally reached 9,147.71 × 105 arbitrary units, which was 3 times that of the control. At this point, ketones, alcohols, esters and aldehydes were 11.5, 10.2, 4.4, and 3.7 times that of the control, respectively. But the amount of total acids was only 89.2% of that in the control (Figure 5). Alcohols and esters, which are responsible for mild flavors, prevailed in the experimental group, together accounting for 42% of the total volatiles at the end of ripening. This value is higher than those reported for Roquefort (11–17%), Bleu des Causses (19%), Bleu d'Auvergne (24%) and Gorgonzola (39%). For acids in the ripened experimental cheese, its percentage (22%) is much below the value of blue-veined varieties (50–70%) (32, 33). In comparison, in the control group, acids predominated, accounting for 73% of the total volatiles at day 33. No significant content increases in other chemical groups were observed except for esters (Supplementary Table 1). These comparisons show clearly that it is the fermentation of M. purpureus GL-1 inside the cheese matrix that lets the ripened cheese form a distinct flavor composition, which can distinguish it from other traditional internal mold-ripened cheeses.
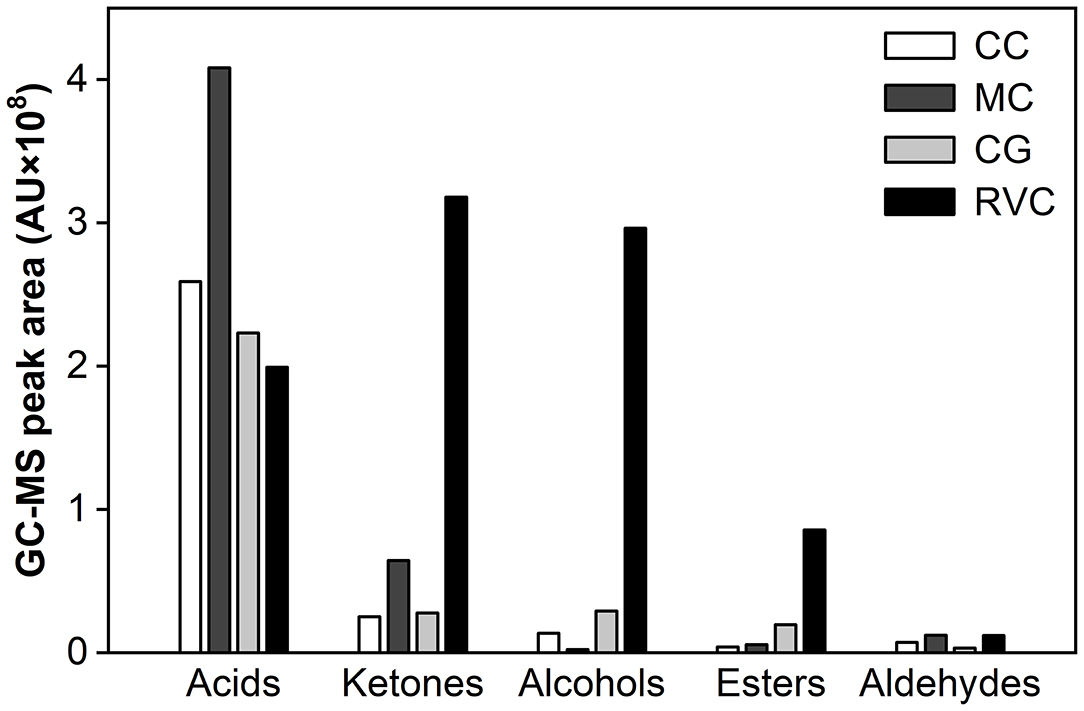
Figure 5. The effects of Monascus-fermentation on the flavor composition of Red-Veined Cheese (RVC) and surface-ripened Monascus-fermented cheese (MC) (8) after 33 days of ripening. CG, control group without inoculation of red koji powder; CC, control group of surface-ripened MC.
Additionally, when compared with the surface-ripened MC using the same strain of M. purpureus (8), more volatiles substances produced during the whole ripening time in the experimental cheese, including 6 alcohols (1-butanol, 2-methyl-1-butanol, 1-pentanol, 2-decanol, 1-octanol), 3 acids (propanoic acid, 3-methylbutanoic acid, palmitic acid), 4 esters (butyl acetate, propyl hexanoate, ethyl nonanoate, ethyl laurate) and 1 aldehyde (hexanal) (Table 4). At the end of ripening, most of them were still present in the cheese. Among which,1-pentanol, 1-octanol, 2-phenylethanol, 3-methylbutanoic acid, palmitic acid, ethyl nonanoate and ethyl laurate showed content accumulation (p < 0.001) from the fifth day of maturation (Table 4). Besides, two aromatic alcohols (benzyl alcohol and 2-phenylethanol) were abundant in the experimental cheese but barely detectable in surface-ripened MC (8). For flavor substances that can be detected in both types of Monascus-fermented cheese, straight-chain fatty acids with pungent smell (from C2 to C8) showed higher concentrations in the MC while the level of ketones with floral and fruity aroma (2-heptanone, 2-octanone, 2-nonanone, and 2-undecanone) were higher in the experimental cheese. After the same length of maturation (33 days), the amounts of alcohols, esters and ketones in the experimental cheese were 141-, 15-, and 5-fold higher, respectively, than those in surface-ripened MC. Whereas, the total acid content was only 48.8% of that found in the MC (Figure 5).
Another interesting finding was there were clear differences in formation between the experimental cheese and surface-ripened MC (Figure 6). At the onset of maturation, alcohols in the experimental cheese had the highest proportion among all chemical groups (39.8% of GC-MS total peak area of volatiles), acids ranked second (35.4%). As maturation continues, ketones continued to increase with its peak at day 33 (34.9%). Alcohols fluctuation over time was observed. At the end of ripening, it became the second most abundant volatile component (32.5%). Acids rose first and then dropped with its peak at day 3 (53.7%) and finally ranked third (21.9%) at day 33 (Figure 6B). In contrast, flavor development in surface-ripened MC (Figure 6D) is entirely different from that of the experimental cheese, but similar to the pattern of its control group (Figure 6C), which is characterized by a consistently high level of carboxylic acids throughout the maturation progress. The percentage of acids to the total volatiles remained the highest throughout the whole ripening period and increased from 57% at day 0 to 82.9% at day 33, while ketones, alcohols and esters decreased from 19.3, 15.3, and 8.0 to 13.1, 0.4, and 1.2%, respectively (Figure 6D). Also, the comparison revealed that the flavor formation of the control in this study (Figure 6A) was highly similar to that of the control group of the MC (Figure 6C). Since the same mold strain was employed as the red starter in this study, the difference in flavor between these two different Monascus-fermented varieties further confirms that the growth position of Monascus mycelium is a very important factor for the metabolic generation which is closely related to the formation of volatile metabolites during cheese ripening. This finding was consistent with the previous research results about Aspergillus oryzae (40).
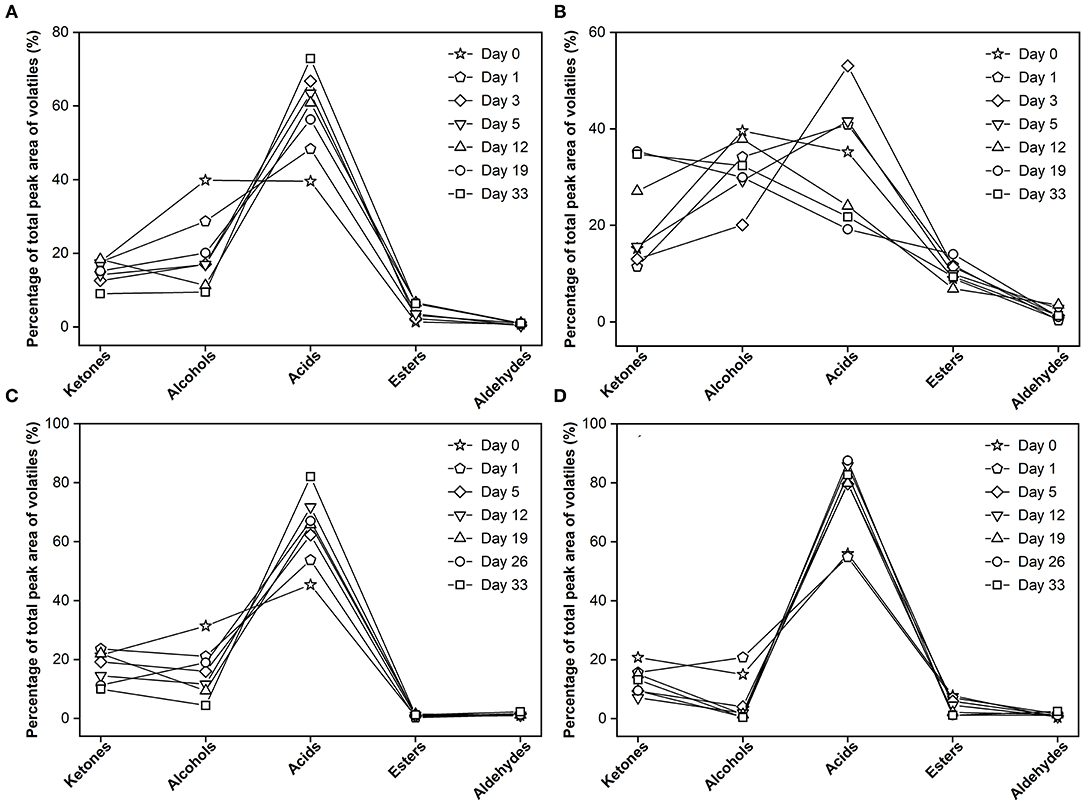
Figure 6. Changes in flavor profile across the 33 days. (A) control group without inoculation of red koji powder, (B) experimental group, (C) control group of surface-ripened Monascus-fermented cheese (MC) (8), (D) surface-ripened MC.
Conclusions
This is the first report on developing a novel internal mold-ripened cheese variety through the inoculation of red koji powder into the paste. It is a new Monascus-fermented variety that has a style similar to blue-veined cheese. Because of the bright red color of the veins, it is named as Red-Veined Cheese. Compared to the control, a higher proteolysis and lipolysis degree indicate that the use of internal Monascus-fermentation to accelerate cheese ripening is successful. More than that, the remarkable increase in the kind and quantity of volatile compounds also gives the experimental cheese a distinctive flavor profile, which distinguishes it not only from other internal mold-ripened cheeses but also from surface-ripened Monascus-fermented cheese previously reported. From a broader perspective, the result of the present study not only adds an important branch to the family tree of mold-ripened cheese but also provides a useful addition to Monascus-fermented food. Furthermore, this study proved that the growth position of Monascus mycelium might be one of the key reasons for the discrepancy in the flavor development between Red-Veined Cheese and surface-ripened Monascus-fermented cheese. This finding provides not only a valuable reference for flavor improvement of Monascus-fermented cheese but also lays a foundation for the industrialization of high-quality Monascus-fermented food in the future.
Data Availability Statement
The original contributions generated for the study are included in the article/Supplementary Material, further inquiries can be directed to the corresponding author/s.
Author Contributions
ZL and CY designed experiments. JJ and ZZ carried out experiments, analyzed experimental results and sequencing data, and wrote the manuscript. All authors contributed to the article and approved the submitted version.
Conflict of Interest
JJ, ZZ, ZL, and CY were employed by the company Bright Dairy & Food Co., Ltd.
Acknowledgments
The authors would like to gratefully acknowledge the National Key R&D Program of China (2018YFC1604205) and the Shanghai Engineering Center of Dairy Biotechnology (19DZ2281400). The authors also wish to thank the public platform of the State Key Laboratory of Dairy Biotechnology.
Supplementary Material
The Supplementary Material for this article can be found online at: https://www.frontiersin.org/articles/10.3389/fnut.2021.649611/full#supplementary-material
References
1. Fanqiang M, Chen R, Zhu X, Lu Y, Nie T, Lu F, et al. Newly effective milk-clotting enzyme from Bacillus subtilis and its application in Cheese Making. J Agric Food Chem. (2018) 66:6162–9. doi: 10.1021/acs.jafc.8b01697
2. Feeney E, Nugent A, Nulty B, Walton J, Flynn A, Gibney E. An overview of the contribution of dairy and cheese intakes to nutrient intakes in the Irish diet: results from the National Adult Nutrition Survey. Br J Nutr. (2015) 1:1–9. doi: 10.1017/S000711451500495X
3. Srianta I, Ristiarini S, Sen S, Zhang BB, Xu G, Blanc P. Recent research and development of Monascus fermentation products. Int Food Res J. (2014) 21:1–12. Available online at: http://www.ifrj.upm.edu.my/21%20(01)%202014/1%20IFRJ%2021%20(01)%202014%20Srianta%20211.pdf
4. Rahayu YYS, Yoshizaki Y, Yamaguchi K, Okutsu K, Futagami T, Tamaki H, et al. Key volatile compounds in red koji-shochu, a Monascus-fermented product, and their formation steps during fermentation. Food Chem. (2017) 224:398–406. doi: 10.1016/j.foodchem.2016.12.005
5. Burke F. Red yeast rice for the treatment of dyslipidemia. Curr Atheroscler Rep. (2015) 17:495. doi: 10.1007/s11883-015-0495-8
6. Lorrungrua C, Sinma K, Pantagrud P, Wannasiris S, Mahabandha K, Khucharoen K. Red cheese production from soymilk by Monascus purpureus and Lactobacillus casei. J Appl Sci. (2014) 14:2819–24. doi: 10.3923/jas.2014.2819.2824
7. Yu H, Liu Z, Hang F, Mo B. Effect of Monascus sp. as an adjunct starter on physicochemical properties and proteolysis in semi-hard cheeses during ripening. Food Sci Biotechnol. (2016) 25:785–93. doi: 10.1007/s10068-016-0133-4
8. Wu S, Yu H, Liu Z, You C. Influence of Monascus purpureus BD-M-4 on the physicochemical properties, proteolysis and volatile compounds of surface mould-ripened cheese. Food Sci Biotechnol. (2018) 28:129–38. doi: 10.1007/s10068-018-0459-1
9. Babitha S, Soccol CR, Pandey A. Solid-state fermentation for the production of Monascus pigments from jackfruit seed. Bioresour Technol. (2007) 98:1554–60. doi: 10.1016/j.biortech.2006.06.005
10. Prieto B, Urdiales R, Franco I, Tornadijo ME, Fresno JM, Carballo J. Biochemical changes in Picón Bejes-Tresviso cheese, a Spanish blue-veined variety, during ripening. Food Chem. (1999) 67:415–21. doi: 10.1016/S.0308-8146(99)00140-5
11. Bütikofer U, Rüegg M, Ardö Y. Determination of nitrogen fractions in cheese: evaluation of a collaborative study. LWT Food Sci Technol. (1993) 26:271–5. doi: 10.1006/fstl.1993.1056
12. Felicio TL, Esmerino EA, Vidal VAS, Cappato LP, Garcia RKA, Cavalcanti RN, et al. Physico-chemical changes during storage and sensory acceptance of low sodium probiotic Minas cheese added with arginine. Food Chem. (2016) 196:628–37. doi: 10.1016/j.foodchem.2015.09.102
13. Mei J, Guo Q, Wu Y, Li Y, Yu H. Study of proteolysis, lipolysis, and volatile compounds of a Camembert-type cheese manufactured using a freeze-dried Tibetan kefir co-culture during ripening. Food Sci Biotechnol. (2015) 24:393–402. doi: 10.1007/s10068-015-0052-9
14. Mallia S, Fernández-García E, Olivier Bosset J. Comparison of purge and trap and solid phase microextraction techniques for studying the volatile aroma compounds of three European PDO hard cheeses. Int Dairy J. (2005) 15:741–58. doi: 10.1016/j.idairyj.2004.11.007
15. Duval P, Chatelard-Chauvin C, Gayard C, Rifa E, Bouchard P, Hulin S, et al. Changes in biochemical and sensory parameters in industrial blue-veined cheeses in different packaging. Int Dairy J. (2018) 77:89–99. doi: 10.1016/j.idairyj.2016.10.004
16. Fernandez-Salguero J. Internal mould–ripened cheeses: characteristics, composition and proteolysis of the main European blue vein varieties. Ital J Food Sci. (2004) 16:437–45. Available online at: http://www.chiriottieditori.it/images/stories/IJFS%20archivio/IJFS164.pdf
17. Lawlor JB, Delahunty CM, Sheehan J, Wilkinson MG. Relationships between sensory attributes and the volatile compounds, non-volatile and gross compositional constituents of six blue-type cheeses. Int Dairy J. (2003) 13:481–94. doi: 10.1016/S0958-6946(03)00048-7
18. Guizani N, Kasapis S, Al Attabi Z, Al-Ruzeiki M. Microbiological, physicochemical, and biochemical changes during ripening of camembert cheese made of pasteurized cow's milk. Int J Food Prop. (2002) 5:483–94. doi: 10.1081/JFP-120015486
19. Diezhandino I, Fernández D, González L, McSweeney PLH, Fresno JM. Microbiological, physico-chemical and proteolytic changes in a Spanish blue cheese during ripening (Valdeón cheese). Food Chem. (2015) 168:134–41. doi: 10.1016/j.foodchem.2014.07.039
20. Prieto B, Franco I, Fresno JM, Bernardo A, Carballo J. Picón Bejes-Tresviso blue cheese: an overall biochemical survey throughout the ripening process. Int Dairy J. (2000) 10:159–67. doi: 10.1016/S0958-6946(00)00032-7
21. Madkor S, Fox PF, Shalabi SI, Metwalli NH. Studies on the ripening of stilton cheese: Proteolysis. Food Chem. (1987) 25:13–29. doi: 10.1016/0308-8146(87)90050-1
22. Fox PF, Cogan TM, Guinee TP. Chapter 25 - factors that affect the quality of cheese. In: McSweeney PLH, Fox PF, Cotter PD, Everett DW, editors. Cheese. 4th edition. San Diego: Academic Press (2017). p. 617–41.
23. Lee HJ, Olson NF, Lund DB. Diffusion of salt, fatty acids, and esterases in mozzarella cheese. J Dairy Sci. (1980) 63:513–22. doi: 10.3168/jds.S0022-0302(80)82965-1
24. Matera J, Luna AS, Batista DB, Pimentel TC, Moraes J, Kamimura BA, et al. Brazilian cheeses: a survey covering physicochemical characteristics, mineral content, fatty acid profile and volatile compounds. Food Res Int. (2018) 108:18–26. doi: 10.1016/j.foodres.2018.03.014
25. Sousa MJ, Ardö Y, McSweeney PLH. Advances in the study of proteolysis during cheese ripening. Int Dairy J. (2001) 11:327–45. doi: 10.1016/S0958-6946(01)00062-0
26. Schormüller J. The chemistry and biochemistry of cheese ripening. In: Chichester CO, Mrak EM, Stewart GF, editors. Advances in Food Research. Manhattan: Academic Press (1968). p. 231–334.
27. Zarmpoutis IV, McSweeney PLH, Fox PF. Proteolysis in blue-veined cheese: an intervarietal study. Irish J Agric Food Res. (1997) 36:219–29. https://www.jstor.org/stable/25562306
28. El-Sheikh MM, El-Senaity MH, Youssef YB, Shahein NM, Rabou NS. Effect of ripening conditions on the properties of Blue cheese produced from cow's and goat's milk. J Am Sci. (2011) 7:485–90. Available online at: http://www.jofamericanscience.org/journals/am-sci/am0701/63_4549am0701_485_490.pdf
29. Ramos M, Cáceres I, Polo C, Alonso L, Juarez M. Effect of freezing on soluble nitrogen fraction of Cabrales cheese. Food Chem. (1987) 24:271–8. doi: 10.1016/0308-8146(87)90102-6
30. Collins YF, McSweeney PLH, Wilkinson MG. Lipolysis and free fatty acid catabolism in cheese: a review of current knowledge. Int Dairy J. (2003) 13:841–66. doi: 10.1016/S0958-6946(03)00109-2
31. Majcher MA, Myszka K, Gracka A, Grygier A, Jeleń HH. Key odorants of lazur, a polish mold-ripened cheese. J Agric Food Chem. (2018) 66:2443–8. doi: 10.1021/acs.jafc.6b04911
32. Moio L, Piombino P, Addeo F. Odour-impact compounds of Gorgonzola cheese. J Dairy Res. (2000) 67:273–85. doi: 10.1017/S0022029900004106
33. Gallois A, Langlois D. New results in the volatile odorous compounds of French cheeses. Le Lait. (1990) 70:89–106. doi: 10.1051/lait:199028
34. Engels WJM, Dekker R, de Jong C, Neeter R, Visser S. A comparative study of volatile compounds in the water-soluble fraction of various types of ripened cheese. Int Dairy J. (1997) 7:255–63. doi: 10.1016/S0958-6946(97)00003-4
35. Yvon M, Rijnen L. Cheese flavour formation by amino acid catabolism. Int Dairy J. (2001) 11:185–201. doi: 10.1016/S0958-6946(01)00049-8
36. Cantor MD, van den Tempel T, Hansen TK, Ardö Y. Chapter 37 - blue cheese. In: McSweeney PLH, Fox PF, Cotter PD, Everett DW, editors. Cheese. 4th ed. San Diego: Academic Press (2017). p. 929–54.
37. Di Cagno R, Banks J, Sheehan L, Fox PF, Brechany EY, Corsetti A, et al. Comparison of the microbiological, compositional, biochemical, volatile profile and sensory characteristics of three Italian PDO ewes' milk cheeses. Int Dairy J. (2003) 13:961–72. doi: 10.1016/S0958-6946(03)00145-6
38. Galli BD, Martin JGP, da Silva PPM, Porto E, Spoto MHF. Sensory quality of Camembert-type cheese: relationship between starter cultures and ripening molds. Int J Food Microbiol. (2016) 234:71–5. doi: 10.1016/j.ijfoodmicro.2016.06.025
39. Curioni PMG, Bosset JO. Key odorants in various cheese types as determined by gas chromatography-olfactometry. Int Dairy J. (2002) 12:959–84. doi: 10.1016/S0958-6946(02)00124-3
Keywords: internal mold-ripened cheese, red koji powder, Monascus, sandwich inoculation, ripening, proteolysis, lipolysis, volatile compounds
Citation: Jiao J, Zheng Z, Liu Z and You C (2021) Study of the Compositional, Microbiological, Biochemical, and Volatile Profile of Red-Veined Cheese, an Internal Monascus-Ripened Variety. Front. Nutr. 8:649611. doi: 10.3389/fnut.2021.649611
Received: 14 January 2021; Accepted: 25 March 2021;
Published: 16 April 2021.
Edited by:
Edy Sousa De Brito, Embrapa Agroindústria Tropical, BrazilReviewed by:
José S. Câmara, Universidade da Madeira, PortugalKieran N. Kilcawley, Teagasc, Ireland
Copyright © 2021 Jiao, Zheng, Liu and You. This is an open-access article distributed under the terms of the Creative Commons Attribution License (CC BY). The use, distribution or reproduction in other forums is permitted, provided the original author(s) and the copyright owner(s) are credited and that the original publication in this journal is cited, in accordance with accepted academic practice. No use, distribution or reproduction is permitted which does not comply with these terms.
*Correspondence: Zhenmin Liu, bGl1emhlbm1pbkBicmlnaHRkYWlyeS5jb20=; Chunping You, eW91Y2h1bnBpbmdAYnJpZ2h0ZGFpcnkuY29t
†These authors have contributed equally to this work