- 1Shobhaben Pratapbhai Patel School of Pharmacy and Technology Management, Shri Vile Parle Kelavani Mandal's Narsee Monjee Institute of Management Studies, Mumbai, India
- 2Department of Pathology and Laboratory Medicine, Faculty of Medicine, University of Ottawa, Ottawa, ON, Canada
- 3Department of Biotechnology, Maharishi Markandeshwar (Deemed to be University), Mullana, India
Curdlan is an exopolysaccharide, which is composed of glucose linked with β-(1,3)-glycosidic bond and is produced by bacteria, such as Alcaligenes spp., Agrobacterium spp., Paenibacillus spp., Rhizobium spp., Saccharomyces cerevisiae, Candida spp., and fungal sources like Aureobasidium pullulan, Poria cocos, etc. Curdlan has been utilized in the food and pharmaceutical industries for its prebiotic, viscosifying, and water-holding properties for decades. Recently, the usefulness of curdlan has been further explored by the pharmaceutical industry for its potential therapeutic applications. Curdlan has exhibited immunoregulatory and antitumor activity in preclinical settings. It was observed that curdlan can prevent the proliferation of malarial merozoites in vivo; therefore, it may be considered as a promising therapy for the treatment of end-stage malaria. In addition, curdlan has demonstrated potent antiviral effects against human immunodeficiency virus (HIV) and Aedes aegypti virus. It has been suggested that the virucidal properties of curdlans should be extended further for other deadly viruses, such as severe acute respiratory syndrome (SARS), Middle East respiratory syndrome (MERS), and the current severe acute respiratory syndrome coronavirus-2 (SARS-CoV-2/COVID-19). The prebiotic property of curdlan would confer beneficial effects on the host by promoting the growth of healthy microbiota in the gut and consequently help to reduce gastrointestinal disorders. Therefore, curdlan can be employed in the manufacture of prebiotics for the management of various gastrointestinal dysbiosis problems. Studies on the mechanism of action of curdlan-induced suppression in microbial and tumor cells at the cellular and molecular levels would not only enhance our understanding regarding the therapeutic effectiveness of curdlan but also help in the discovery of new drugs and dietary supplements. The primary focus of this review is to highlight the therapeutic interventions of curdlan as an anticancer, anti-malaria, antiviral, and antibacterial agent in humans. In addition, our review provides the latest information about the chemistry and biosynthesis of curdlan and its applications for making novel dairy products, functional foods, and nutraceuticals and also details about the recent patents of curdlan and its derivatives.
Introduction
Exopolysaccharides are natural polymers of high-molecular-weight residual sugar polymers secreted by a wide variety of non-pathogenic microorganisms, including bacteria, yeast, and fungi. The extracellular natural polymeric substances belong to the carbohydrates category and provide many important health benefits, such as promotion of digestibility, biocompatibility, and endurance of long-term stability over a wide range of pH and environmental temperatures (1). Recently, researchers have found promising applications of natural polymeric substances, for example, curdlan, in the pharmaceuticals, food packaging, making biofilms, and in the dairy industry where they improve the viscosity and texture of the food products (2). Overall, curdlan-type exopolysaccharides have many emerging potential applications in making nutraceuticals, functional foods, cosmetics, drugs, and dairy products (3).
Physical Properties of Curdlan
Curdlan is a water-insoluble exopolysaccharide secreted by a wide variety of non-pathogenic bacteria, yeast, and fungi as shown in Table 2. It is comprised of a homopolymer of D-glucose-linked with β-(1,3)-glucan (12). The unique thermal characteristics of curdlan are utilized in biofilm making to improve water barrier capacity and thermal stability in single- and multilayer food packaging (13). Apart from its high thermal stability, curdlan also possesses stability at lower freezing temperatures, and hence is used in frozen foods packaging. According to recent reports, curdlan biofilms with 5% caprolactam have been shown to possess better mechanical strength and other physical properties as compared to other films (14). Curdlan can form two types of gels based on their thermal properties, viz., low-set gel and high-set gel (15). The low-set curdlan gel is produced when the aqueous solution of curdlan is heated up to 60°C and then cooled to 40°C in a thermally reversible process. On the other hand, the high-set curdlan gel is generated when the aqueous solution of curdlan is heated >80°C in a thermally irreversible process (16). In chemical terms, there is a cross-linking between the curdlan micelles in the low-set gel, which are occupied by single-helix molecules through hydrogen bonds, whereas the curdlan micelles are cross-linked by a triple-stranded helix through hydrophobic interactions in the high-set gel (17). Curdlan gels are used for the encapsulation of a number of drugs like indomethacin, salbutamol sulfate, and prednisolone to provide sustained release of these drugs (18). They are also used for grafting copolymers in the pharmaceutical industry (19).
Curdlan is one of the emerging exopolysaccharide, which was discovered and studied by (14, 20). It has the ability to produce polysaccharide-based membrane film, which has proven effective in possessing gas barrier properties (20, 21). Due to its non-toxic attributes, curdlan was approved as a food additive by the US Food and Drug Administration (22). Prebiotics are non-digestible oligosaccharides that promote the growth of beneficial bacteria in the gut. Curdlan being an oligosaccharide has a prebiotic potential and therefore can be used to promote the growth of useful intestinal microbiota (23).
Curdlan has shown a wide range of pharmacotherapy functions, such as anti-HIV, immunomodulation, and antitumor activity (24). A recent study revealed that curdlan can inhibit the invasion of malarial merozoites and therefore can be considered as a possible auxiliary candidate for treating intense malaria (25). Additionally, curdlan has exhibited potential antiviral activity against human immunodeficiency virus (HIV) and Dengue fever virus (26). In view of its virucidal activities, curdlan may be considered a potential candidate against other deadly viruses, such as severe acute respiratory syndrome (SARS), Middle East respiratory syndrome (MERS), and the current severe acute respiratory syndrome coronavirus-2 (SARS-CoV-2/COVID-19).
There are many published reports regarding the therapeutic properties and excipient properties of curdlan (26), but the underlying mechanism of curdlan at the cellular and molecular level remains unknown. This review is intended to highlight the applications of curdlan in drugs and cosmetics, nutraceuticals, and functional foods and in potential industrial usages. In addition, our review provides the latest information regarding the chemistry and microbial sources used for the biosynthesis of curdlan, along with the recent patents applied by the food and drug manufacturers for the utility of curdlan.
Chemistry of Curdlan
Chemical Structures/Formulas of Curdlan Products
Chemical structures and formulas of curdlan compounds are shown in Table 1. The analytical studies have confirmed that curdlan is a D-glucose homopolymer linked in the manner of β-(1,3)-glycosidic bond (30). Curdlan is unbranched and has an average degree of polymerization of around 450. In an alkaline solution of 0.3 Mol, the molecular weight of curdlan is in the range of 5.3 × 104 to 2.0 × 106 Da. The bacterial and fungal species produced curdlan, the average weight lies between 2.06 × 104 and 5.0 × 106 Da (31).
The general molecular formula of curdlan is (C6H10O5)n. It consists of approximately 135 residues of glucosyl, and the infrared spectrum shows a peak of β-arrangement gel at 890 cm−1, while α- arrangement gel shows a peak at 840 cm−1 (32–34).
There is a range of structural variations within the class of β-(1,3)-glucans polysaccharides (35). Curdlan falls into the category of neutral gel having β-(1 → 3)-glycosidic linkage or may also contain few inter- and intra-chain (1 → 6)-linkages, which contain almost 10% succinate known as succinoglycan (36). Curdlan is produced in three different structural forms by the bacterial species (28).
Molecular Conformers of Curdlan
The molecular structures of curdlan in the aqueous systems have been determined by several investigators. For instance, Zhang et al. (37) identified three soluble curdlan conformers, including single-helix, triple-helix, and random coil. The single-stranded curdlan is composed of 2–25 glucose units that exhibit better water solubility with improved permeability. Different biological activities are associated with short disordered curdlan chains (38). Some curdlans have a helical (ordered) conformation at low NaOH concentrations (37). Ikeda and Shishido demonstrated using atomic force microscopy that as alkali concentration increases from 0.19 to 0.24 N, curdlan conformation changes from helix conformation to random coil structure (39). Cheung et al. reported that in 0.3 mol NaOH, the ultrasonic degradation was found to be much faster than in 0.1 mol NaOH, suggesting that curdlan is more sensitive to the degradation in the random-coil conformation than in the helix conformation (40). Saitô et al. confirmed that curdlan is fully soluble in an alkaline solution having a concentration >0.2 N NaOH and exists as a random coil, but the polymer adopts an “ordered state” after neutralization, which consists of a combination of single and triple helices (41). Zhang and Nishinari reported that at low concentrations, curdlan exhibits a relatively extended flexible chain structure in dimethyl sulfoxide, whereas chain entanglement occurs at a higher concentration (42).
Biosynthesis of Curdlan
Microbial Source
Curdlan can be produced as exopolysaccharides by different types of microbes, for example, bacteria, yeast, and fungi. Some major microbes are involved in the biosynthesis of curdlan, and the yields achieved are shown in Table 2.
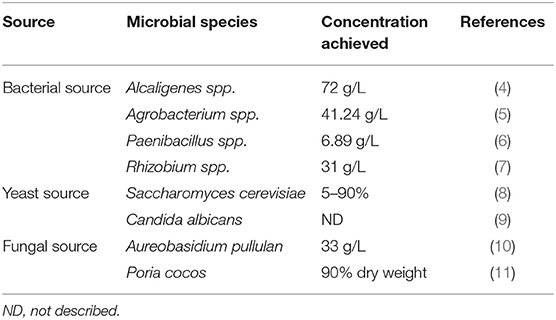
Table 2. Microbial species used in the biosynthesis of curdlan and the yields achieved by different species.
Alcaligenes faecalis is a Gram-negative bacterium, which can be isolated from the soil and can yield maximum curdlan. The NaOH solution was substituted by feeding ammonia water at an initial stage to control pH at 7.0, and then the NaOH solution was added to control pH at 5.6 for the curdlan production after ammonia was consumed. As a result, 72 g/L of curdlan was produced at controlled nitrogen condition (4). Agrobacterium radiobacter is also a Gram-negative soil bacterium, which yields 41.24 g/L of curdlan after 96 h of fermentation (43). Recently, the biological activity as an immune stimulator of peripheral blood mononuclear cells to increase the interferon-γ secretion was shown by curdlan developed by the mutant strain of Agrobacterium sp. ATCC 31750 (ATCC, Manassas, VI, USA), having a molecular weight of 300,000 Da and consisting exclusively of the 1,3-glucose residue linkage (44). Paenibacillus polymyxa is a Gram-positive bacterium, which at 50°C and at pH 7 yields 6.89 g/L of curdlan (6). It is used as a drug delivery carrier for the sustained release of drugs and as a support matrix for the immobilization of enzymes (45). Rhizobium radiobacter is another Gram-negative bacterium, which can yield 31 g/L of curdlan after 72 h of fermentation (46). A recent study confirmed that curdlan obtained from R. radiobacter is highly thermostable with excellent water-holding capacity and can be used as an antioxidant and prebiotic agent. However, further studies are needed to check the biological potential of curdlan produced from R. radiobacter (47).
Biosynthesis of Curdlan
The biosynthesis of extracellular polysaccharides can be divided into three steps. First, substrate uptake for the intracellular formation of polysaccharides and extrusion from the cells. Second, through active transport and group translocation involving substrate phosphorylation, a carbohydrate substratum reaches the cell. Finally, the substrate is guided either along catabolic pathways or those that lead to the synthesis of polysaccharides.
In the biosynthesis of curdlan, uridine diphosphate (UDP)-glucose constitutes the primary precursor that is synthesized via the conversion of glucose-1-phosphate to UDP-glucose by the enzyme UDP-glucose pyrophosphorylase. Later, the polymer structure occurs by the combination with the transition of monosaccharides to the carrier lipid from UDP-glucose. Subsequently, the polymer is extruded from the cells after further chain elongation (Figure 1) (48).
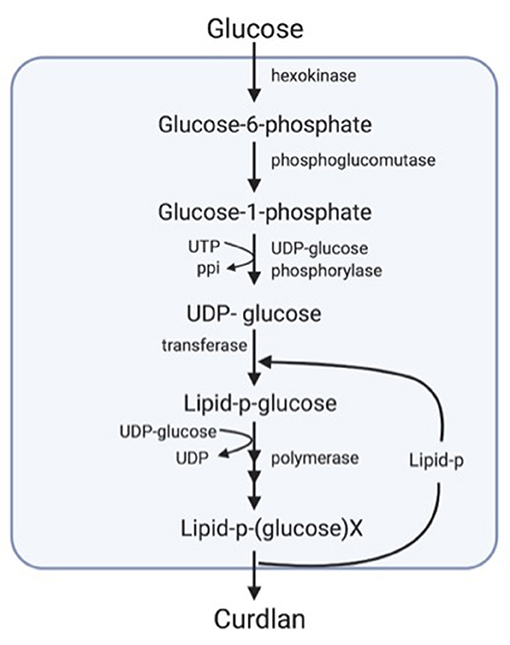
Figure 1. Diagrammatic representation of the biosynthesis of curdlan (33).
A study conducted by Mohsin et al. showed that curdlan can be synthesized from the orange peels using A. faecalis. The cocktail mixture included about 78% water, 10% soluble sugars, 35% cellulose, and 15% pectin from fresh orange peels. However, because of high moisture content, the cost for the preservation of curdlan is high, and hence drying process is an effective approach to store peels. By this technique, the dried orange peels yielded about 23.24 g/L of curdlan. The fermentation substrate showed significant effects on the development of curdlan and presented a novel engineered approach for the production of curdlan in an environment-friendly manner (49).
Applications of Curdlan
Food and Dairy Products
Curdlan gel is a colorless, odorless, and non-toxic substance that has water retention ability. All these versatile properties have led to its use as a food additive in the dairy industry (50, 51). Since curdlan is not easily hydrolyzed by the digestive enzymes, it can be added in prebiotics and to reduce calories and to promote healthy gut microbiota, and thus prevent obesity (52). It is also used as an additive in the preparation of frozen foods, pasta, and canned meat (53).
The hydrothermal hydrolysis of curdlan creates 1,3-gluco-oligosaccharides (GOS), which have proven beneficial in the production of functional foods and biopharmaceuticals (54). On account of the bacteriostatic, biocompatible, biodegradable, and hydrophilic characteristics, the quaternary ammonium salt solution of curdlan (Qcurd) is widely used along with its derivatives to encapsulate several bioactive food components (55). Several curdlan-induced benefits in food and dairy products are summarized in Table 3.
Therapeutic Products
Biologically active β-(1,3)-glucan oligosaccharides were developed by the combination of heat treatment and hydrolysis of curdlan (62). In vitro batch fermentation using human fecal microorganisms showed that the bioactive oligosaccharides significantly increased the population of Lactobacillus in the gut and hence can be considered as a potential prebiotic (63). In vivo studies suggested that glucan oligosaccharide enhanced immunoregulatory activity in cyclophosphamide (CTX)-induced immunosuppressed mice by stimulating the release of nitric oxide (NO), cytokines, and increased immunoglobulins release and splenic lymphocyte proliferation (64).
Curdlan itself and GOS activate macrophages via complement receptor 3 (CR3) and toll-like receptor 2 (TLR2) by mitogen-activated protein kinase (MAPK) pathway and nuclear factor NF-κB pathway. The activated macrophages were found to be non-toxic or compatible with bone marrow-derived macrophages isolated from healthy male C57BL/6J mice. The GOS promoted M1 phenotype polarization of bone marrow-derived macrophages by upregulation of CD11C and CD86 expressions (65). In view of these findings, GOS may be considered safe to enhance immune responses when added to the development of functional foods (66).
Curdlan sulfate (CRDS) is a sulfated glycoconjugate that is biochemically similar to rosette inhibiting drugs like heparin and has been tried for treating severe malaria in 18 African children. CRDS inhibited erythrocyte rosette formation in vitro at 10–100μg/ml in Plasmodium falciparum laboratory strains and revealed anti-malarial activity in clinical isolates (67). Palm sulfopropyl (SP) and palm carboxymethyl/sulfopropyl (CM/SP) curdlan derivatives were able to potentiate the action of actinomycin D or doxorubicin in B16 tumor cells by inhibiting HEp-2 tumor cell growth and by upregulating doxorubicin and actinomycin D cytostatic activities (68). Further clinical trials are needed to establish the long-term safety and efficacy of CRDS in humans.
Barbosa-Lorenzi et al. found that curdlan acts as an agonist for the fungi receptor dectin-1 and induces selective mast cell degranulation without provoking synthesis of leukotriene and cytokine. Hence, curdlan may be employed against fungal infections in human skin (69). Curdlan pellicle produced by Gluconacetobacter xylinus is one of the best bio-based materials because of its super network structure and unique physiochemical properties and could be used for a wide range of clinical and tissue engineering applications (70).
Role of Curdlan as an Adjuvant
In order to enhance the immune response, adjuvants are added to vaccines to produce more antibodies and long-lasting immunity, thus minimizing the required antigen dose. In several studies, β-D-glucan has proven useful as an adjuvant carrier as it is a natural non-digestible polysaccharide that can be easily detected when bound to receptors, such as dectin-1. There is emerging interest in exploring the ability of curdlan to gain endogenous information in the desired organs or cells (71). Protective immunity to Staphylococcus aureus was observed by vaccination of mice with fungal β-glucan particles (GP) loaded with S. aureus antigens. The findings suggest that the glucan particles vaccine system has the potential as a novel approach for developing vaccines against S. aureus (72). Chemo- and radio-therapies cause several side effects in patients with cancer. Neutropenia is one of the most significant side effects of chemotherapy, which disrupts the process of blood formation in the bone marrow and can cause lymphocytopenia, thrombocytopenia, and granulocytopenia and consequently leading to immune system suppression (73). Curdlan represents a novel and promising therapeutic approach in treating cervical cancer and can be used as an adjuvant to reduce side effects of cancer chemotherapy treatment and help in recovering from compromised hematopoiesis in the therapy injured bone marrow (74).
In clinical trials, β-(1,3)-glucan has been used as adjuvant therapy with significant beneficial effects on the survival and quality of life of patients with cancer through the activation of the immune system (75). In comparison with the aluminum adjuvant, curdlan increased the immunogenicity of the hepatitis B vaccine and promoted an immune response in mice by increasing the specific antibody response to hepatitis B surface antigen (HBsAg) (76). Sulfated yeast β-glucan showed an excellent adjuvant effect on the influenza vaccine (H5N1) in a mouse model. The anti-influenza vaccine increased the influx of macrophages and dendritic cells, increased antigen-specific T cell levels, and induced splenocyte proliferation in mice (77). In another study done on mice, curdlan alone substantially boosted the immune response against influenza through stimulation of lymphocyte proliferation and enhanced cytokine production (78). Well-designed clinical studies are warranted to verify the results obtained in animal models.
Curdlan as an Antioxidant and Anti-inflammation Agent
It has been hypothesized that β-(1,3)-glucan products are capable of increasing the lifespan, slowing the emergence of age-related biomarkers and exerting the antioxidant impact of Nothobranchius guentheri and hence may be potentially useful for promoting health and well-being and extension of lifespan in humans (79). Recent studies have shown that beta-glucans can decrease hyperglycemia, hyperlipidemia, and high blood pressure. By minimizing oxidative stress-induced mitochondrial damage, curdlan/β-(1,3)-glucan may contribute to new approaches to manage diabetes mellitus associated complications, neurodegenerative disorders, and cardiovascular diseases (80). It has also been suggested that curdlan obtained from S. cerevisiae protects the human plasma components from strong biological oxidants and inflammatory mediators due to its free radical scavenging capability (81). A study conducted on isolated rat hepatocytes showed that curdlan obtained from the fungal source can capture free radicals and may be a possible candidate for prophylaxis and treatment against depleted uranium toxic effects (82). Furthermore, the systemic administration and local application of beta-glucan were effective against burn-induced oxidative tissue damage in rats (83).
Protection Against Hyperglycemia in Patients With Diabetes Infected With COVID-19
Curdlan obtained from yeast Aureobasidium pullulans, also known as biological response modifier glucan (BRMG), has the ability to bring the blood sugar levels under control and has the potential in enhancing and regulating immune parameters. Fasting plasma glucose (FPG) level is one of the indicators of COVID-19 infection, which causes high mortality among patients with diabetes and is responsible for causing serious pathological complications in vital organs. Consequently, BRMG may help to control FPG levels and may be used as a preventive measure against the COVID-19 virus (84). COVID-19 has also been linked to an increased risk of mortality in patients with comorbidities including diabetes. Diabetics have a higher risk of disease severity in COVID-19 because they have a higher incidence of inflammatory processes due to persistent glucose recognition by C-type lectin receptors (84). Reduced PI3K/Akt activity is a crucial factor in the development of diabetes. Dectin-1 (belongs to the C-type lectin receptors family), complement receptor-3, lactosylceramide, and scavenger and toll-like receptors are among the receptors that BRMGs activate. The immune system of patients with diabetes is typically impaired. The immune system can be boosted by BRMG (85). Diabetes is linked to glycation-induced chronic inflammation of beta cells (β-cells) in the pancreas and elevated hemoglobin A1C (HbA1C) and glycated hemoglobin levels with clinical relevance as a marker of diabetes diagnosis and glycemic regulation. The immune-boosting activity of BRMG is linked to its potential anti-glycation effect (normalization of HbA1c) in patients with diabetes (86). In addition, the development of a hypercoagulable prothrombotic state is favored by an imbalance between coagulation and fibrinolysis, with increased levels of clotting factors and relative inhibition of the fibrinolytic system, endothelial dysfunction, and enhanced platelet aggregation and activation, all of which are complications of diabetes, explains the high risk of patients with diabetes to COVID-19 in terms of intensity/severity (85). Yeast BRMGs have been found to regulate the coagulation activation by controlling the cytokine activity and reversing diabetes-induced oxidative stress, which causes clotting parameters to be disrupted due to increased platelet aggregation and thrombin levels (87). Under the prevailing circumstances, it is of utmost importance to reduce COVID-19-induced mortality and serious complications such as coagulopathy, especially among the vulnerable populations (85). In order to fight against COVID-19-like pathogens, BRMG may be used to boost the immune system in humans. BRMG may also prevent blood clotting from occurring due to radiation exposure or diabetes-induced oxidative stress that enhances platelet aggregation and increases thrombin levels. It was reported that BRMG increases the level of IL8, while decreases the levels of several interleukins (IL-1β, IL-2, IL-4, IL-6, and IL-12), tumor necrosis factor (TNF)-alpha, interferon-gamma (IFN-γ), and active soluble fas-ligand (sFasL) levels, thereby maintaining the effective optimal defense against hyper-inflammation-free viral infections (87). Hyperinflammation results by preventing the onset of apoptosis and by increasing the development of sFAS and by suppressing the cytokine storms through a cascade of molecular events involved in activating Treg cells and reducing IL6, and at the same time preventing the chemoattraction of monocytes and macrophages, T cells, NK cells, and dendritic cells by reducing CXCL10 and CCL2 cells (88). Overall, BRMG enhances the immune function in the upper respiratory tract to protect against microbial pathogens (89). The immune boosting activity is one of the most important attributes of β-glucans. Keeping in view these observations, further clinical trials are required to validate the antiviral and immune system prophylaxis of BRMG.
The virucidal properties of BRMG should be explored further for other deadly viruses, including the lethal viruses like SARS, MERS, and the virulent strains of SARS-CoV-2/COVID-19. Although SARS-CoV-2 is primarily a respiratory virus, the viral host receptor angiotensin-converting enzyme 2 (ACE2) is found in the cytoplasm of gastrointestinal epithelial cells, and the viral nucleocapsid protein is found in the cytoplasm of rectal, duodenal, and gastric epithelial cells, suggesting that the intestine may play a role in COVID-19 pathogenesis and may be a possible route of infection. β-glucans with immune effects in the intestine could thus be a beneficial supplementation method for the COVID-19 treatment (90). A β-glucan extract from the edible shiitake mushroom Lentinus edodes was recently confirmed to have a differential in vitro immunomodulatory and pulmonary cytoprotective effects, suggesting that it may be used to treat COVID-19. According to the findings, β-glucans delivered as a customized cocktail may be a good match for a potential nutraceutical-based COVID-19 intervention (91). In a randomized, placebo-controlled, and double-blind trial, Mah et al. found that the severity of upper respiratory tract infection (URTI) was lower among marathon runners (n = 76) who consumed dairy beverages containing 250 mg soluble baker's yeast beta-glucan (Wellmune® Austin, TX, USA) as opposed to athletes given placebo (n = 133). The severity was evaluated by the Jackson index, and results showed that the yeast beta-glucan significantly reduced the severity of nasal discharge and URTI in marathon runners (92).
Therefore, the above evidence suggests the application of curdlan in the treatment of hyperglycemia in patients infected with COVID-19.
Wound Healing Properties of Curdlan
β-Glucans increase wound repair by increasing the infiltration of macrophages, which promote tissue granulation, collagen deposition, and skin re-epithelialization. Curdlan-based wound dressings have a great stability and resistance to wound protease, prevent bacterial infections, and are effective in wound healing. According to a clinical study conducted by Majtan and Jesenak, the application of 3% cream containing curdlan isolated from S. cerevisiae caused 55.23% reduction in ulcers after 90 days of treatment (93). In humans, fungal β-D-glucans reduce blood cholesterol and improve absorption of glucose in cells and thereby help in wound healing (94).
Immunomodulation Functions of Curdlan
Besides the above-mentioned usages, curdlan appears to have some other immunomodulation applications. Recently, Song et al. reported improvement in the in vivo humoral and cell-mediated immune responses in aged mice after the antigen challenge thereby suggesting the antiaging efficacy of curdlan (95). Curdlan derivatives also have anti-allergic activities, which have been verified in animal models and clinical trials in humans (96). Burg et al. observed that the daily oral administration of fungal β-glucan (400 μg/day) significantly reduced the influx of eosinophils into the lungs of ovalbumin challenged mice compared with control counterparts, thereby suggesting the protective action of curdlan against the development of asthma (97). The overactive type II helper T-lymphocytes response is thought to occur in individuals who experience allergic asthma attacks. It has been observed that β-(1,3)-glucan attenuates Th2 cytokines response by modulating the activity of macrophages, which produce pro-inflammatory mediators, such as prostaglandin E2, tumor growth factor, and IL-10 (98). Some investigators suggest that curdlan may be helpful in the prevention of chronic fatigue syndrome because it increases the proliferation of splenic lymphocytes and enhances the production of cytokines together with the stimulation of humoral and cellular immunity (99).
Recent Patents of Curdlan
Besides other functional and biological properties, curdlan has been identified as an antiviral and antibacterial substance. As an immunomodulatory agent, curdlan can bind as a phagocytic receptor ligand and can act as dectin-1 agonist (69). In addition, curdlan can act as C-type lectin receptor and hence can achieve targeted immunotherapy (100). Apart from this, curdlan has unique gelling properties and thus can be applied to increase elasticity and the overall gel strength (13). Curdlan can also be used to form edible preservative films (14, 15). Recent patents of curdlan are mostly concentrated toward its immunomodulatory functions and improvement of physical properties of foods and drugs in the packaging processes. A comprehensive list of recent patents on curdlan, its applications, inventors, and patent holders are summarized in Table 4.
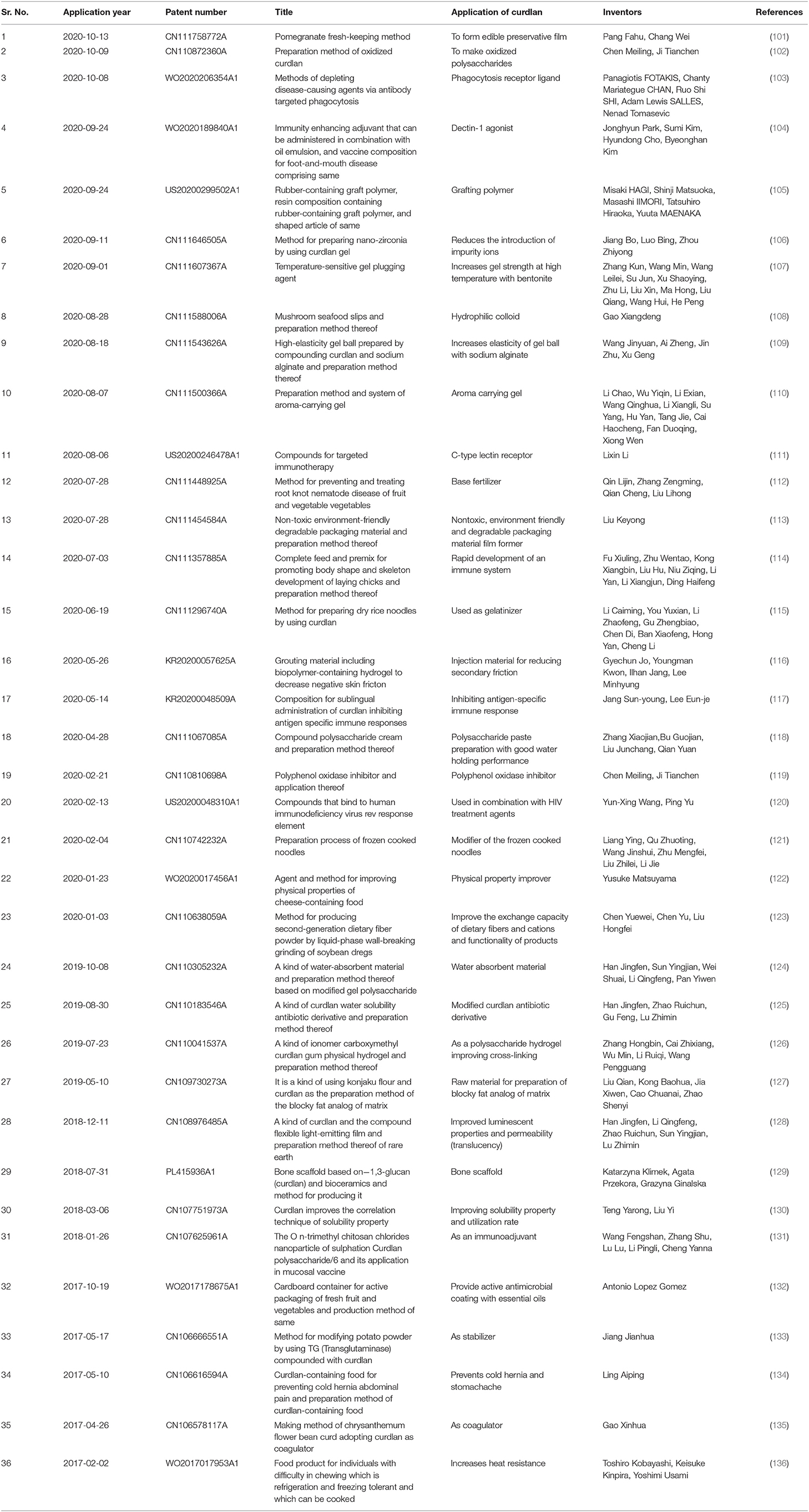
Table 4. Recent patents on curdlan showing patent holders, therapeutic products and a wide array of industrial applications.
Conclusions And Future Directions
A wide variety of exopolysaccharides or curdlans are produced by using bacteria, fungi, and yeast. The unique physicochemical properties of soluble and insoluble curdlans have been found highly valuable materials in different industries, such as dairy, food manufacturing, cosmetics, and pharmaceuticals. As shown in Table 4, curdlan hydrocolloids play very important roles in making novel functional foods, pharmaceuticals, and dairy products like prebiotics. Some curdlans are dectin-1 receptor agonists and hence can be employed as adjuvant carriers for vaccines, and their immunomodulatory activity can be beneficial in cancer chemotherapy and prevention of autoimmune disorders. A number of curdlans have shown antiaging, antidiabetic, antioxidant, anti-inflammation, wound healing, and cardio- and neuro-protection capabilities. Some curdlans have revealed the potential for treating malaria, pathogenic bacteria, and viral diseases (HIV and SARS-CoV-2/COVID-19). However, further well-designed studies are needed to verify the preliminary results obtained in a limited number of clinical observations.
It is also important to evaluate the immunomodulatory activities of different curdlans in suitable animal models and randomized and well-controlled clinical studies in humans. More research is also warranted to understand the mechanism of action of curdlans at the cellular and molecular levels to develop novel products. In addition, there is a need to study the formulation characteristic of curdlans under different environmental conditions for creating stable formulations with long-lasting shelf lives.
The current patents on curdlan are mostly concentrated toward biopolymers, curdlan gels, polysaccharide-based membrane film, immunomodulatory functions, and improvement of physical properties of foods and drugs in the packaging processes. Microencapsulation and nanotechnology studies are needed for the development and delivery of antiviral, anticancer, and parenteral therapies of curdlan. Good manufacturing procedures and quality control methods must be used in making curdlan preparations.
Author Contributions
GK, HB, and HT visualized the presented idea, contributed to manuscript writing, and supervised the project. VC, AV, and SB-P contributed in doing literature searches and prepared manuscript draft. GK and HB revised and approved the manuscript. All authors contributed to the article and approved the submitted version.
Conflict of Interest
The authors declare that the research was conducted in the absence of any commercial or financial relationships that could be construed as a potential conflict of interest.
References
1. Zhang R, Belwal T, Li L, Lin X, Xu Y, Polymers ZL-C, 2020 undefined. Recent Advances in Polysaccharides Stabilized Emulsions for encapsulation and Delivery of Bioactive Food Ingredients: A Review. Elsevier. (2020). Available online at: https://www.sciencedirect.com/science/article/pii/S0144861720305622 (accessed November 18, 2020).
2. Pereira L, Gheda SF, Ribeiro-Claro PJA. Analysis by vibrational spectroscopy of seaweed polysaccharides with potential use in food, pharmaceutical, cosmetic industries. Int J Carbohydr Chem. (2013) 2013:537202. doi: 10.1155/2013/537202
3. Rana S Biological LU-IJ of 2020 undefined. Microbial Exopolysaccharides: Synthesis Pathways, Types and Their Commercial Applications. Elsevier. (2020). Available online at: https://www.sciencedirect.com/science/article/pii/S0141813020329378 (accessed November 18, 2020).
4. Wu J, Zhan X, Liu H, Zheng Z. Enhanced Production of Curdlan by Alcaligenes faecalis by Selective Feeding with Ammonia Water during the Cell Growth Phase of Fermentation. Chin J Biotechnol. (2008) 24:1035–9. doi: 10.1016/S1872-2075(08)60049-7
5. Liang Y, Zhu L, Gao M, Zheng Z, Wu J, Zhan X. Influence of Tween-80 on the production and structure of water-insoluble curdlan from Agrobacterium sp. Int J Biol Macromol. (2018) 106:611–9. doi: 10.1016/j.ijbiomac.2017.08.052
6. Rafigh SM, Yazdi AV, Vossoughi M, Safekordi AA, Ardjmand M. Optimization of culture medium and modeling of curdlan production from Paenibacillus polymyxa by RSM and ANN. Int J Biol Macromol. (2014) 70:463–73. doi: 10.1016/j.ijbiomac.2014.07.034
7. Ben Salah R, Jaouadi B, Bouaziz A, Chaari K, Blecker C, Derrouane C, et al. Fermentation of date palm juice by curdlan gum production from Rhizobium radiobacter ATCC 6466TM: Purification, rheological and physico-chemical characterization. LWT - Food Sci Technol. (2011) 44:1026–34. doi: 10.1016/j.lwt.2010.11.023
8. Lesage G, Bussey H. Cell Wall Assembly in Saccharomyces cerevisiae. Microbiol Mol Biol Rev. (2006) 70:317–43. doi: 10.1128/mmbr.00038-05
9. Miura NN, Adachi Y, Yadomae T, Tamura H, Tanaka S, Ohno N. Structure and biological activities of β-glucans from yeast and mycelial forms of Candida albicans. Microbiol Immunol. (2003) 47:173–82. doi: 10.1111/j.1348-0421.2003.tb03382.x
10. Bozoudi D, Tsaltas D. The multiple and versatile roles of Aureobasidium pullulans in the vitivinicultural sector. Fermentation. (2018) 4:1–15. doi: 10.3390/fermentation4040085
11. Mahapatra S, Banerjee D. Fungal exopolysaccharide: production, composition and applications. Microbiol Insights. (2013) 6:MBI.S10957. doi: 10.4137/mbi.s10957
12. Food Additive Status List | FDA (2020). Available online at: https://www.fda.gov/food/food-additives-petitions/food-additive-status-list (accessed November 18, 2020).
13. Barcelos MCS, Vespermann KAC, Pelissari FM, Molina G. Current status of biotechnological production and applications of microbial exopolysaccharides. Crit Rev Food Sci Nutr. (2020) 60:147595. doi: 10.1080/10408398.2019.1575791
14. Harada T, Misaki A, Saito H. Curdlan: A bacterial gel-forming β-1, 3-glucan. Arch Biochem Biophys. (1968) 124:292–8. doi: 10.1016/0003-9861(68)90330-5
15. Fu Y, Cheng L, Meng Y, Li S, Zhao X, Du Y, polymers HY-C, 2015 undefined. Cellulosimicrobium Cellulans Strain E4-5 Enzymatic Hydrolysis of Curdlan for Production of (1→3)-Linked β-d-Glucan Oligosaccharides. Elsevier. (2020). Available online at: https://www.sciencedirect.com/science/article/pii/S0144861715007602 (accessed November 18, 2020).
16. Yan J, Cai W, Wang C, Yu Y, Zhang H, YY-F 2020 undefined. Macromolecular Behavior, Structural Characteristics and Rheological Properties of Alkali-Neutralization Curdlan at Different Concentrations. Elsevier. (2020). Available online at: https://www.sciencedirect.com/science/article/pii/S0268005X19312263 (accessed November 18, 2020).
17. Evansi SG, Morrison' D, Kaneko2 Y, Havlikl I. The Effect of Curdlan Sulphate on Development in vitro of Plasmodium Falciparum. (2020). Available online at: https://academic.oup.com/trstmh/article/92/1/87/1876813 (accessed November 18, 2020).
18. Ichiyama K, Gopala Reddy SB, Zhang LF, Chin WX, Muschin T, Heinig L, et al. Sulfated polysaccharide, curdlan sulfate, efficiently prevents entry/fusion and restricts antibody-dependent enhancement of dengue virus infection in vitro: a possible candidate for clinical application. PLoS Negl Trop Dis. (2013) 7:2188. doi: 10.1371/journal.pntd.0002188
19. Coatings UB-P in O, 2017 undefined. Experimental Investigation of Novel Curdlan/Chitosan Coatings on Packaging Paper. Elsevier (2020). Available online at: https://www.sciencedirect.com/science/article/pii/S030094401730156X (accessed November 18, 2020).
20. Mangolim C, Silva T da, chemistry VF-F, 2017 undefined. Use of FT-IR, FT-Raman and Thermal Analysis to Evaluate the Gel Formation of Curdlan Produced by Agrobacterium sp. IFO 13140 and Determination of its Rheological. Elsevier. (2020). Available online at: https://www.sciencedirect.com/science/article/pii/S0308814617305800 (accessed November 18, 2020).
21. Zhu J, Wang Q, Han L, Zhang C, Wang Y, KT-… APAM, 2020 undefined. Effects of Caprolactam Content on Curdlan-Based Food Packaging Film and Detection by Infrared Spectroscopy. Elsevier. (2020). Available online at: https://www.sciencedirect.com/science/article/pii/S1386142520309215 (accessed November 18, 2020).
22. Jiang S, Zhao S, Jia X, Wang H, Zhang H, Liu Q, et al. Thermal gelling properties and structural properties of myofibrillar protein including thermo-reversible and thermo-irreversible curdlan gels. Food Chem. (2020) 311:126018. doi: 10.1016/j.foodchem.2019.126018
23. Funami T, Yada H, Nakao Y. Curdlan properties for application in fat mimetics for meat products. J Food Sci. (1998) 63:283–7. doi: 10.1111/j.1365-2621.1998.tb15727.x
24. Wu C, Yuan C, Chen S, Liu D, Ye X, Hu Y. The effect of curdlan on the rheological properties of restructured ribbonfish (Trichiurus spp.) meat gel. Food Chem. (2015) 179:222–231. doi: 10.1016/j.foodchem.2015.01.125
25. Zhan XB, Lin CC, Zhang HT. Recent advances in curdlan biosynthesis, biotechnological production, and applications. Appl Microbiol Biotechnol. (2012) 93:525–31. doi: 10.1007/s00253-011-3740-2
26. Cai W-D, Zhu J, Wu L-X, Qiao Z-R, Li L, Yan J-K. Preparation, characterization, rheological and antioxidant properties of ferulic acid-grafted curdlan conjugates. Food Chem. (2019) 300:125221. doi: 10.1016/j.foodchem.2019.125221
27. Li X, Cheung PCK. Application of natural β-glucans as biocompatible functional nanomaterials. Food Sci Hum Wellness. (2019) 8:315–9. doi: 10.1016/j.fshw.2019.11.005
28. Verma DK, Niamah AK, Patel AR, Thakur M, Singh Sandhu K, Chávez-González ML, et al. Noe Aguilar Chemistry and microbial sources of curdlan with potential application and safety regulations as prebiotic in food and health. Food Res Int. (2020) 133:109136. doi: 10.1016/j.foodres.2020.109136
29. Castellane TCL, de Sousa BFS, Lemos EG de M. Exopolysaccharide produced from Rhizobium spp. - An interesting product for industry and environment. Curr Appl Polym Sci. (2019) 3:157–66. doi: 10.2174/2452271603666191016143811
30. Zhai W, Iwata T. Synthesis and properties of curdlan branched and linear mixed ester derivatives. Polym Degrad Stab. (2019) 161:50–56. doi: 10.1016/j.polymdegradstab.2019.01.008
31. Hundschell CS, Wagemans AM. Rheology of common uncharged exopolysaccharides for food applications. Curr Opin Food Sci. (2019) 27:1–7. doi: 10.1016/j.cofs.2019.02.011
32. Zhang R, Edgar KJ. Properties, chemistry, and applications of the bioactive polysaccharide curdlan. Biomacromolecules. (2014) 15:1079–96. doi: 10.1021/bm500038g
33. West TP. Production of the polysaccharide curdlan by agrobacterium species on processing coproducts and plant lignocellulosic hydrolysates. Fermentation. (2020) 6:16. doi: 10.3390/fermentation6010016
34. Kalyanasundaram GT, Doble M, Gummadi S. Production and downstream processing of (1 → 3)-β- D-glucan from mutant strain of Agrobacterium sp. ATCC 31750. AMB Express. (2012) 2:1–10. doi: 10.1186/2191-0855-2-31
35. Han B, Baruah K, Cox E, Vanrompay D, Bossier P. Structure-functional activity relationship of β-glucans from the perspective of immunomodulation: a mini-review. Front Immunol. (2020) 11:1–8. doi: 10.3389/fimmu.2020.00658
36. Gieroba B Sroka-Bartnicka A Kazimierczak P Kalisz G Pieta IS Nowakowski R . Effect of gelation temperature on the molecular structure and physicochemical properties of the curdlan matrix: Spectroscopic and microscopic analyses. Int J Mol Sci. (2020) 21:1–18. doi: 10.3390/ijms21176154
37. Zhang L, Wang C, Cui S, Wang Z, Zhang X. Single-molecule force spectroscopy on curdlan: Unwinding helical structures and random coils. Nano Lett. (2003) 3:1119–24. doi: 10.1021/nl034298d
38. Feng X, Li F, Ding M, Zhang R, Shi T. Molecular dynamic simulation: conformational properties of single-stranded curdlan in aqueous solution. Carbohydr Polym. (2020) 250:116906. doi: 10.1016/j.carbpol.2020.116906
39. Ikeda S, Shishido Y. Atomic force microscopy studies on heat-induced gelation of Curdlan. J Agric Food Chem. (2005) 53:786–91. doi: 10.1021/jf048797r
40. Cheung YC, Yin J, Wu JY. Effect of polysaccharide chain conformation on ultrasonic degradation of curdlan in alkaline solution. Carbohydr Polym. (2018) 195:298–302. doi: 10.1016/j.carbpol.2018.04.118
41. Saitô H, Ohki T, Sasaki T. A 13C Nuclear Magnetic Resonance Study of Gel-Forming (1~3)-β-d-Glucans. Evidence of the presence of single-helical conformation in a resilient gel of a curdlan-type polysaccharide 13140 from Alcaligenes faecalis var. myxogenes IFO 13140. Biochemistry. (1977) 16:908–14. doi: 10.1021/bi00624a015
42. Zhang H, Nishinari K. Characterization of the conformation and comparison of shear and extensional properties of curdlan in DMSO. Food Hydrocoll. (2009) 23:1570–8. doi: 10.1016/j.foodhyd.2008.11.001
43. Gao H, Xie F, Zhang W, Tian J, Zou C, Jia C, et al. Characterization and improvement of curdlan produced by a high-yield mutant of Agrobacterium sp. ATCC 31749 based on whole-genome analysis. Carbohydr Polym. (2020) 245:116486. doi: 10.1016/j.carbpol.2020.116486
44. Shih IL, Yu JY, Hsieh C, Wu JY. Production and characterization of curdlan by Agrobacterium sp. Biochem Eng J. (2009) 43:33–40. doi: 10.1016/j.bej.2008.08.006
45. Liang T-W, Wang S-L. Recent advances in exopolysaccharides from Paenibacillus spp.: production, isolation, structure, and bioactivities. Mar Drugs. (2015) 13:1847–63. doi: 10.3390/md13041847
46. Gao H, Yang L, Tian J, Huang L, Huang D, Zhang W, et al. Characterization and rheological properties analysis of the succinoglycan produced by a high-yield mutant of Rhizobium radiobacter ATCC 19358. Int J Biol Macromol. (2020) 166:61–70. doi: 10.1016/j.ijbiomac.2020.10.087
47. Kavitake D, Delattre C, Devi PB, Pierre G, Michaud P, Shetty PH. Andhare Physical and functional characterization of succinoglycan exopolysaccharide produced by Rhizobium radiobacter CAS from curd sample. Int J Biol Macromol. (2019) 134:1013–21. doi: 10.1016/j.ijbiomac.2019.05.050
48. Sutherland IW. Microbial exopolysaccharide synthesis department of microbiology, university of Edinburgh, West Mains Road, Edinburgh, EH9 3JG, Scotland The fate of a carbohydrate (or other) substrate supplied to an exopolysaccharide-producing microbial cell de. Extracellular Microbial Polysaccharides. (1977) 4:40–57.
49. Mohsin A, Sun J, Khan IM, Hang H, Tariq M, Tian X, et al. Sustainable biosynthesis of curdlan from orange waste by using Alcaligenes faecalis: A systematically modeled approach. Carbohydr Polym. (2019) 205:626–35. doi: 10.1016/j.carbpol.2018.10.047
50. Lee CH, Chin KB. Evaluation of various salt contents on quality characteristics with or without curdlan of pork myofibrillar protein gels and the development of low-salt pork sausages. Int J Food Sci Technol. (2019) 54:550–7. doi: 10.1111/ijfs.13969
51. Puertas A, Dueñas M, Marieta C. Supramolecular structure and renaturation of a (1 → 3)-β-d-glucan compared with curdlan and scleroglucan. Fibers. (2014) 2:255–63. doi: 10.3390/fib2030255
52. Aljewicz M, Florczuk A, Dabrowska A. Influence of β-glucan structures and contents on the functional properties of low-fat ice cream during storage. Polish J Food Nutr Sci. (2020) 70:233–40. doi: 10.31883/pjfns/120915
53. Cai Z, Zhang H. Recent progress on curdlan provided by functionalization strategies. Food Hydrocoll. (2017) 68:128–35. doi: 10.1016/j.foodhyd.2016.09.014
54. Wang D, Kim DH, Yoon JJ, Kim KH. Production of high-value β-1,3-glucooligosaccharides by microwave-assisted hydrothermal hydrolysis of curdlan. Process Biochem. (2017) 52:233–7. doi: 10.1016/j.procbio.2016.11.005
55. Cai WD, Qiu WY, Ding ZC, Wu LX, Yan JK. Conformational and rheological properties of a quaternary ammonium salt of curdlan. Food Chem. (2019) 280:130–8. doi: 10.1016/j.foodchem.2018.12.059
56. Xin Y, Lee JY, Kim J, Kim Y. Effect of curdlan on textural and cooking qualities of noodles made with tofu. J Food Process Preserv. (2018) 42:13661. doi: 10.1111/jfpp.13661
57. Qian Y, Bian L, Wang K, Chia WY, Khoo KS, Zhang C, et al. Preparation and characterization of curdlan/nanocellulose blended film and its application to chilled meat preservation. Chemosphere. (2020) 266:128948. doi: 10.1016/j.chemosphere.2020.128948
58. Lee CH, Chin KB. Changes in physicochemical properties of pork myofibrillar protein combined with corn starch and application to low-fat pork patties. Int J Food Sci Technol. (2020) 55:157–64. doi: 10.1111/ijfs.14272
59. Jiang S, Cao C, Xia X, Liu Q, Kong B. Enhancement of the textural and gel properties of frankfurters by adding thermo-reversible or thermo-irreversible curdlan gels. J Food Sci. (2019) 84:1068–77. doi: 10.1111/1750-3841.14595
60. Malaka R, Ohashi T, Baco S. Effect of bacteria exopolysaccharide on milk gel formation. Open J For. (2013) 3:10–2. doi: 10.4236/ojf.2013.34b004
61. Zhang H, Zhang F, Yuan R. Applications of natural polymer-based hydrogels in the food industry. In: Hydrogels Based on Natural Polymers. Elsevier (2020). p. 357–410. doi: 10.1016/B978-0-12-816421-1.00015-X
62. Kumagai Y, Okuyama M, Kimura A. Heat treatment of curdlan enhances the enzymatic production of biologically active β-(1,3)-glucan oligosaccharides. Carbohydr Polym. (2016) 146:396–401. doi: 10.1016/j.carbpol.2016.03.066
63. Shi Y, Liu J, Yan Q, You X, Yang S, Jiang Z. In vitro digestibility and prebiotic potential of curdlan (1 → 3)-β-D-glucan oligosaccharides in Lactobacillus species. Carbohydr Polym. (2018) 188:17–26. doi: 10.1016/j.carbpol.2018.01.085
64. Tang J, Zhen H, Wang N, Yan Q, Jing H, Jiang Z. Curdlan oligosaccharides having higher immunostimulatory activity than curdlan in mice treated with cyclophosphamide. Carbohydr Polym. (2019) 207:131–42. doi: 10.1016/j.carbpol.2018.10.120
65. Liu J, Tang J, Li X, Yan Q, Ma J, Jiang Z. Curdlan (Alcaligenes faecalis) (1 → 3)-β-d-Glucan Oligosaccharides Drive M1 phenotype polarization in murine bone marrow-derived macrophages via activation of MAPKs and NF-κB pathways. Molecules. (2019) 24:4251. doi: 10.3390/molecules24234251
66. Jia X, Wang C, Du X, Peng H, Liu L, Xiao Y, et al. Specific hydrolysis of curdlan with a novel glycoside hydrolase family 128 β-1,3-endoglucanase containing a carbohydrate-binding module. Carbohydr Polym. (2021) 253:117276. doi: 10.1016/j.carbpol.2020.117276
67. Kyriacou HM, Steen KE, Raza A, Arman M, Warimwe G, Bull PC, et al. In vitro inhibition of Plasmodium falciparum rosette formation by curdlan sulfate. Antimicrob Agents Chemother. (2007) 51:1321–6. doi: 10.1128/AAC.01216-06
68. Bădulescu M, Apetrei N, AL-R 2009 undefined. Curdlan Derivatives Able to Enhance Cytostatic Drugs activity on Tumor Cells. academia.edu. (2008). Available online at: http://www.academia.edu/download/47964581/Curdlan_derivatives_able_to_enhance_cyto20160810-1572-1c4s1d5.pdf#page=11 (accessed November 18, 2020).
69. Barbosa-Lorenzi VC, Peyda S, Scheynius A, Nilsson G, Lunderius-Andersson C. Curdlan induces selective mast cell degranulation without concomitant release of LTC4, IL-6 or CCL2. Immunobiology. (2017) 222:647–50. doi: 10.1016/j.imbio.2016.12.001
70. Fang J, Kawano S, Tajima K, Kondo T. In vivo curdlan/cellulose bionanocomposite synthesis by genetically modified gluconacetobacter xylinus. Biomacromolecules. (2015) 16:3154–60. doi: 10.1021/acs.biomac.5b01075
71. Su Y, Li X, Lam KL, Cheung PCK. pH-sensitive PEG-coated hyper-branched β-D-glucan derivative as carrier for CpG oligodeoxynucleotide delivery. Carbohydr Polym. (2020) 246:116621. doi: 10.1016/j.carbpol.2020.116621
72. Paterson MJ, Caldera J, Nguyen C, Sharma P, Castro AM, Kolar SL, et al. Harnessing antifungal immunity in pursuit of a Staphylococcus aureus vaccine strategy. PLOS Pathog. (2020) 16:e1008733. doi: 10.1371/journal.ppat.1008733
73. Akramiene D, Akramiene D, Kondrotas A, DidŽiapetriene J, Kevelaitis E. APŽVALGINIS STRAIPSNIS Correspondence to Effects of b-Glucans on the Immune System. (2007). Available online at: https://www.mdpi.com/1010-660X/43/8/597 (accessed November 26, 2020).
74. Chaichian S, Moazzami B, Sadoughi F, Haddad Kashani H, Zaroudi M, Asemi Z. Functional activities of beta-glucans in the prevention or treatment of cervical cancer. J Ovarian Res. (2020) 13:1–12. doi: 10.1186/s13048-020-00626-7
75. Eiman A. β -Glucans and their Applications in Cancer Therapy: Focus o.: Ingenta Connect. Anti-Cancer Agents Med Chem. (2013) 13:709–19. doi: 10.2174/1871520611313050005
76. Li P, Tan H, Xu D, Yin F, Cheng Y, Zhang X, et al. Effect and mechanisms of curdlan sulfate on inhibiting HBV infection and acting as an HB vaccine adjuvant. Carbohydr Polym. (2014) 110:446–55. doi: 10.1016/j.carbpol.2014.04.025
77. Wang M, Zhang L, Yang R, Fei C, Wang X, Zhang K, et al. Improvement of immune responses to influenza vaccine (H5N1) by sulfated yeast beta-glucan. Int J Biol Macromol. (2016) 93:203–7. doi: 10.1016/j.ijbiomac.2016.06.057
78. Vetvicka V, Vannucci L, Sima P. β-glucan as a new tool in vaccine development. Scand J Immunol. (2020) 91:12833. doi: 10.1111/sji.12833
79. Song L, Zhou Y, Ni S, Wang X, Yuan J, Zhang Y, et al. Dietary intake of β-glucans can prolong lifespan and exert an antioxidant action on aged fish Nothobranchius guentheri. Rejuvenation Res. (2020) 23:293–301. doi: 10.1089/rej.2019.2223
80. Alp H, Varol S, Celik M, MA-E 2012 undefined. Protective Effects of Beta Glucan and Gliclazide on Brain Tissue and Sciatic nerve of Diabetic Rats Induced by Streptozosin. (2020). Available online at: http://downloads.hindawi.com/journals/edr/2012/230342.pdf (accessed November 23, 2020).
81. Saluk-Juszczak J, Krolewska K, Wachowicz B. (1 → 3)-β-D-Glucan inhibits a dual mechanism of peroxynitrite stroke. Int J Biol Macromol. (2011) 48:488–94. doi: 10.1016/j.ijbiomac.2011.01.010
82. Pourahmad J, Shaki F, Tanbakosazan F, Ghalandari R, Hossein Ali Ettehadi, Dahaghin E. Protective effects of fungal β-(1 → 3)-D-glucan against oxidative stress cytotoxicity induced by depleted uranium in isolated rat hepatocytes. Hum Exp Toxicol. (2011) 30:173–81. doi: 10.1177/0960327110372643
83. Toklu HZ, Sener G, Jahovic N, Uslu B, Arbak S, Yegen BÇ. β-glucan protects against burn-induced oxidative organ damage in rats. Int Immunopharmacol. (2006) 6:156–69. doi: 10.1016/j.intimp.2005.07.016
84. Ikewaki N, Iwasaki M, Abraham SJK. Biological response modifier glucan through balancing of blood glucose may have a prophylactic potential in COVID-19 patients. J Diabetes Metab Disord. (2020) 19:1–4. doi: 10.1007/s40200-020-00664-4
85. Ikewaki N, Rao KS, Archibold AD, Iwasaki M, Senthilkumar R, Preethy S, et al. Coagulopathy associated with COVID-19 – perspectives & preventive strategies using a biological response modifier glucan. Thromb J. (2020) 18:1–8. doi: 10.1186/s12959-020-00239-6
86. Cerf ME. Beta cell dysfunction and insulin resistance. Front Endocrinol. (2013) 4:37. doi: 10.3389/fendo.2013.00037
87. -Kashoury E, Fattah SMA, Ramadan LA, El-Denshary ES. The Role of Yeast Beta Glucan on Blood Coagulation in Streptozotocin-Induced Diabetes and Irradiated Rats. (2016). Available online at: http://www.esnsa-eg.com/download/researchFiles/(3)60-2015.pdf (accessed November 23, 2020).
88. Rao KS, Suryaprakash V, Senthilkumar R, Preethy S, Katoh S, Ikewaki N, et al. Role of immune dysregulation in increased mortality among a specific subset of COVID-19 patients and immune-enhancement strategies for combatting through nutritional supplements. Front Immunol. (2020) 11:1548. doi: 10.3389/fimmu.2020.01548
89. Dharsono T, Rudnicka K, Wilhelm M, Schoen C. Effects of yeast (1,3)-(1,6)-beta-glucan on severity of upper respiratory tract infections: a double-blind, randomized, placebo-controlled study in healthy subjects. J Am Coll Nutr. (2019) 38:40–50. doi: 10.1080/07315724.2018.1478339
90. Ikewaki N, Iwasaki M, Kurosawa G, Rao K-S, Lakey-Beitia J, Preethy S, et al. β-glucans: wide-spectrum immune-balancing food-supplement-based enteric (β-WIFE) vaccine adjuvant approach to COVID-19. Hum Vaccin Immunother. (2021) 2:1–6. doi: 10.1080/21645515.2021.1880210
91. Murphy EJ, Masterson C, Rezoagli E, O'Toole D, Major I, Stack GD, et al. β-Glucan extracts from the same edible shiitake mushroom Lentinus edodes produce differential in-vitro immunomodulatory and pulmonary cytoprotective effects - Implications for coronavirus disease (COVID-19) immunotherapies. Sci Total Environ. (2020) 732:139330. doi: 10.1016/j.scitotenv.2020.139330
92. Mah E, Kaden VN, Kelley KM, Liska DJ. Soluble and insoluble yeast β -glucan differentially affect upper respiratory tract infection in marathon runners: a double-blind, randomized placebo-controlled trial. J Med Food. (2020) 23:416–9. doi: 10.1089/jmf.2019.0076
93. Majtan J, Jesenak M. β-Glucans: multi-functional modulator of wound healing. Molecules. (2018) 23:806. doi: 10.3390/molecules23040806
94. Borchani C, Fonteyn F, Jamin G, Destain J, Willems L, Paquot M, et al. Structural characterization, technological functionality, and physiological aspects of fungal β-D-glucans: a review. Crit Rev Food Sci Nutr. (2016) 56:1746–52. doi: 10.1080/10408398.2013.854733
95. Song L, Yuan J, Ni S, Zhou Y, Wang X, Chen Y, et al. Enhancement of adaptive immune responses of aged mice by dietary intake of β-glucans, with special emphasis on anti-aging activity. Mol Immunol. (2020) 117:160–7. doi: 10.1016/j.molimm.2019.10.019
96. Bashir KMI, Choi JS. Clinical and physiological perspectives of β-glucans: the past, present, and future. Int J Mol Sci. (2017) 18:1906. doi: 10.3390/ijms18091906
97. Burg AR, Quigley L, Jones A V, O'Connor GM, Boelte K, McVicar DW, et al. Orally administered β-glucan attenuates the Th2 response in a model of airway hypersensitivity. Springerplus. (2016) 5:1–10. doi: 10.1186/s40064-016-2501-1
98. Talbott SM, Talbott JA, Talbott TL, Dingler E. β -Glucan supplementation, allergy symptoms, and quality of life in self-described ragweed allergy sufferers. Food Sci Nutr. (2013) 1:90–101. doi: 10.1002/fsn3.11
99. Vetvicka V, Vetvickova J. β-Glucan Attenuates Chronic Fatigue Syndrome in Murine Model. (2015). Available online at: www.jnsci.org/content/112 (accessed November 23, 2020).
100. Vono M, Eberhardt CS, Mohr E, Auderset F, Christensen D, Schmolke M, et al. Overcoming the neonatal limitations of inducing germinal centers through liposome-based adjuvants including C-type lectin agonists trehalose dibehenate or Curdlan. Front Immunol. (2018) 9:28. doi: 10.3389/fimmu.2018.00381
101. Fahu P, Wei C. Pomegranate Fresh-Keeping Method. CN Patent No 111758772A. Nanyang: China National Intellectual Property Administration (2020).
102. Meiling C, Tianchen J. Preparation Method of Oxidized Curdlan. CN Patent No 110872360A. Wuxi: China National Intellectual Property Administration (2020).
103. Fotakis P, Chan CM, Shi RS, Salles AL, Tomasevic N. Methods of Depleting Disease Causing Agents via Antibody Targeted Phagocytosis. WO Patent No 2020206354A1. San Carlos, CA: World Intellectual Property Organization (2020).
104. Park J, Kim S, Cho H, Kim B. Immunity Enhancing Adjuvant That Can Be Administered in Combination With Oil Emulsion, and Vaccine Composition for Foot-And-Mouth Disease Comprising Same. Gimcheon-si: WO Patent No 2020189840A1. World Intellectual Property Organization (2020).
105. Hagi M, Matsuoka S, Iimori M, Hiraoka T, Maenaka Y. Rubber-Containing Graft Polymer, Resin Composition Containing Rubber-Containing Graft Polymer, and Shaped Article of Same. US Patent No 20200299502A1. Charlotte, NC: United States Patent and Trademark Office (2020).
106. Bo J, Bing L, Zhiyong Z. Method for Preparing Nano Zirconia by Using Curdlan Gel. CN Patent No 111646505A. Ningde: China National Intellectual Property Administration (2020).
107. Kun Z, Min W, Leilei W, Jun S, Shaoying X, Li Z, et al. Temperature-Sensitive Gel Plugging Agent. CN Patent No 111607367A. Tianjin: China National Intellectual Property Administration (2020).
108. Xiangdeng G. Mushroom Seafood Slips and Preparation Method Thereof. CN Patent No 111588006A. Fuzhou: China National Intellectual Property Administration (2020).
109. Jinyuan W, Zheng A, Zhu J, Geng X. High-Elasticity Gel Ball Prepared by Compounding Curdlan and Sodium Alginate and Preparation Method Thereof. CN Patent No 111543626A. Shanghai: China National Intellectual Property Administration (2020).
110. Chao L, Yiqin W, Exian L, Qinghua W, Xiangli L, Yang S, et al. Preparation Method and System of Aroma-Carrying Gel. CN Patent No 111500366A. Kunming: China National Intellectual Property Administration (2020).
111. Li L. Compounds for Targeted Immunotherapy. US Patnent No 20200246478A1. Beijing: United States Patent and Trademark Office (2020).
112. Lijin Q, Zengming Z, Cheng Q, Lihong L. Method for Preventing and Treating Root Knot Nematode Disease of Fruit and Vegetables. CN Patent No 111448925A. Chifeng: China National Intellectual Property Administration (2020).
113. Keyong L. Non-Toxic Environment-Friendly Degradable Packaging Material and Preparation Method Thereof. CN Patent No 111454584A. Chifeng: China National Intellectual Property Administration (2020).
114. Xiuling F, Wentao Z, Xiangbin K, Hu L, Ziqing N, Yan L, et al. Complete Feed and Premix for Promoting Body Shape and Skeleton Development of Laying Chicks and Preparation Method Thereof. CN Patent No 111357885A. Huaiyang: China National Intellectual Property Administration (2020).
115. Caiming L, Yuxian Y, Zhaofeng L, Zhengbiao G, Di C, Xiaofeng B, et al. Method for Preparing Dry Rice Noodles by Using Curdlan. CN Patent No 111296740A. Wuxi: China National Intellectual Property Administration (2020).
116. Jo G, Kwon Y, Jang I, Minhyung L. Grouting Material Including Biopolymer-Containing Hydrogel to Decrease Negative Skin Fricton. KR Patent No 20200057625A. Daejeon: Korean Intellectual Property Office (2020).
117. Sun-young J, Eun-je L. Composition for Sublingual Administration of Curdlan Inhibiting Antigen Specific Immune Responses. KR Patent No 20200048509A. Suwon-si: Korean Intellectual Property Office (2020).
118. Xiaojian Z, Guojian B, Junchang L, Yuan Q. Compound Polysaccharide Cream and Preparation Method Thereof. CN Patent No 111067085A. Sheng: China National Intellectual Property Administration (2020).
119. Meiling C, Tianchen J. Polyphenol Oxidase Inhibitor and Application Thereof. CN Patent No 110810698A. Wuxi: China National Intellectual Property Administration (2020).
120. Wang Y, Yu P. Compounds That Bind to Human Immunodeficiency Virus Rev Response Element. US Patent No 20200048310A1. Washington, DC: United States Patent and Trademark Office (2020).
121. Ying L, Zhuoting Q, Jinshui W, Mengfei Z, Zhilei L, Jie L. Preparation Process of Frozen Cooked Noodles. CN Patent No 110742232A. Zhengzhou: China National Intellectual Property Administration (2020).
122. Hongbin Z, Zhixiang C, Min W, Ruiqi L, Pengguang W. Agent and Method for Improving Physical Properties of Cheese-Containing Food. WO Patent No 2020017456A1. Tokyo: World Intellectual Property Organization (2020).
123. Yuewei C, Yu C, Hongfei L. Method for Producing Second-Generation Dietary Fiber Powder by Liquid-Phase Wall-Breaking Grinding of Soybean Dregs. CN Patent No 110638059A. Beijing: China National Intellectual Property Administration (2020).
124. Jingfen H, Yingjian S, Shuai W, Qingfeng L, Yiwen P. (2019). A Kind of Water-Absorbent Material and Preparation Method Thereof Based on Modified Gel Polysaccharide. CN Patent No 110305232A. Hohhot: China National Intellectual Property Administration.
125. Jingfen H, Ruichun Z, Feng G, Zhimin L. A Kind of Curdlan Water Solubility Antibiotic Derivative and Preparation Method Thereof. CN Patent No 110183546A. Hohhot: China National Intellectual Property Administration (2019).
126. Hongbin Z, Zhixiang C, Min W, Ruiqi L, Pengguang W. A Kind of Ionomer Carboxymethyl Curdlan Gum Physical Hydrogel and Preparation Method Thereof. CN Patent No 110041537A. Shanghai: China National Intellectual Property Administration (2019).
127. Qian L, Baohua K, Xiwen J, Chuanai C, Shenyi Z. It is a Kind of Using Konjaku Flour and Curdlan as the Preparation Method of the Blocky Fat Analogue of Matrix. CN Patent No 109730273A. Harbin: China National Intellectual Property Administration (2019).
128. Jingfen H, Qingfeng L, Ruichun Z, Yingjian S, Zhimin L. A Kind of Curdlan and the Compound Flexible Light-Emitting Film and Preparation Method Thereof of Rare Earth. CN Patent No 108976485A. Hohhot: China National Intellectual Property Administration (2018).
129. Klimek K, Przekora A, Ginalska G. Bone Scaffold Based on -1,3-Glucan (Curdlan) and Bioceramics and Method for Producing It. PL Patent No 415936A1. Lublin: Polish Patent Office (2018).
130. Yarong T, Yi L. Curdlan Improves the Correlation Technique of Solubility Property. CN Patent No 107751973A. Shanghai: China National Intellectual Property Administration (2018).
131. Fengshan W, Shu Z, Lu L, Pingli L, Yanna C. The O n-Trimethyl Chitosan Chlorides Nanoparticle of Sulphation Curdlan Polysaccharide/6 and Its Application in Mucosal Vaccine. CN Patent No 107625961A. Jinan: China National Intellectual Property Administration (2018).
132. Gomez AL. Cardboard Container for Active Packaging of Fresh Fruit and Vegetables and Production Method of Same. WO Patent NO 2017178675A1. Cartagena: World Intellectual Property Organization (2017).
133. Jianhua J. Method for Modifying Potato Powder by Using TG (Transglutaminase) Compounded with Curdlan. CN Patent No 106666551A. Suzhou: China National Intellectual Property Administration (2017).
134. Aiping L. Curdlan-Containing Food for Preventing Cold Hernia Abdominal Pain and Preparation Method of Curdlan-Containing Food. CN Patent No 106616594A. Shanghai: China National Intellectual Property Administration (2017).
135. Xinhua G. Making Method of Chrysanthemum Flower Bean Curd Adopting Curdlan as Coagulator. CN Patent No 106578117A. Yantai: China National Intellectual Property Administration (2017).
Keywords: curdlan sulfate, succinoglucan, immunomodulatory activity, anti-viral, anti-malaria, anti-cancer, functional food, β-(1,3)-glucan oligosaccharides
Citation: Chaudhari V, Buttar HS, Bagwe-Parab S, Tuli HS, Vora A and Kaur G (2021) Therapeutic and Industrial Applications of Curdlan With Overview on Its Recent Patents. Front. Nutr. 8:646988. doi: 10.3389/fnut.2021.646988
Received: 28 December 2020; Accepted: 27 May 2021;
Published: 28 June 2021.
Edited by:
Xingbin Yang, Shaanxi Normal University, ChinaReviewed by:
Uma Devi Palanisamy, Monash University Malaysia, MalaysiaCatarina Lourenço-Lopes, University of Vigo, Spain
Copyright © 2021 Chaudhari, Buttar, Bagwe-Parab, Tuli, Vora and Kaur. This is an open-access article distributed under the terms of the Creative Commons Attribution License (CC BY). The use, distribution or reproduction in other forums is permitted, provided the original author(s) and the copyright owner(s) are credited and that the original publication in this journal is cited, in accordance with accepted academic practice. No use, distribution or reproduction is permitted which does not comply with these terms.
*Correspondence: Ginpreet Kaur, ginpreet.kaur@nmims.edu