- 1Department of Biochemistry and Cancer Institute of the Second Affiliated Hospital, Zhejiang University School of Medicine, Hangzhou, China
- 2Key Laboratory of Cancer Prevention and Intervention of China National Ministry of Education, Hangzhou, China
- 3Department of Orthopedic Surgery, The Second Affiliated Hospital, Zhejiang University School of Medicine, Hangzhou, China
Background: Aberrant homocysteine level is associated with metabolic disorders and DNA damage, which may be involved in the carcinogenesis of hormone-related cancers, but clinical results of observational studies are controversial. In this study, we investigated the causal relationships between plasma homocysteine and breast cancer (BRCA), prostate cancer (PrCa), and renal cell carcinoma (RCC) using Mendelian randomization (MR) analyses.
Design and Methods: To investigate the putative causal associations between homocysteine and the aforementioned three types of cancers, a two-sample MR study was employed for the study. The primary strategy for summary data analyses was the inverse-variance-weighted (IVW) approach. In our study, the single-nucleotide polymorphisms (SNPs) excluded confounding factors through Linkage Disequilibrium (LD). Phenoscanner tests were the instrumental variants (IVs), homocysteine was the exposure, and BRCA, PrCa, and RCC were the outcomes. Single-nucleotide polymorphisms associated with homocysteine were extracted from a large genome-wide association study (GWAS) meta-analysis of European participants (n = 44,147). Summary Statistics of BRCA were obtained from the latest and largest GWAS meta-analysis comprising of 82 studies from Breast Cancer Association Consortium (BCAC) studies, including women of European ancestry (133,384 cases and 113,789 controls); we obtained summary-level data from the GWAS meta-analysis of PrCa comprising 79,148 cases and 61,106 controls of European ancestry, and the dataset of RCC was a sex-specific GWAS meta-analysis comprising of two kidney cancer genome-wide scans for men (3,227 cases and 4,916 controls) and women (1,992 cases and 3,095 controls) of European ancestry. The MR-Egger and weight median analyses were applied for the pleiotropy test.
Results: The results showed null associations between plasma homocysteine levels and overall BRCA (effect = 0.97, 95% CI: 0.90–1.06, P = 0.543), overall PrCa (effect = 1.01, 95% CI: 0.93–1.11, P = 0.774), RCC in men (effect = 0.99, 95% CI: 0.73–1.34, P = 0.929), and RCC in women (effect = 0.89, 95% CI: 0.61–1.31, P = 0.563).
Conclusions: We found no putative causal associations between homocysteine and risk of BRCA, PrCa, and RCC.
Introduction
Homocysteine is derived from the essential amino acid methionine (1) and functions as a metabolic intermediate of the methionine cycle that is essential for one-carbon metabolism (2). Activated by ATP, methionine is converted to S-adenosyl methionine (SAM), a donor for DNA methylation (3) that also leads to producing homocysteine as a crucial intermediate for methionine regeneration. This process requires a 5-methyl-tetra-hydrogen-folate (5-MTHF) unit and a specialized methyltransferase that uses vitamin B12 as a coenzyme (4). Hence, deficiency of folic acid and vitamin B12 may lead to a defective methionine cycle thus the accumulation of the plasma homocysteine level (5, 6), which has been linked to an elevated risk of cardiovascular and metabolic disorders (7–9). Indeed, plasma levels of these one-carbon-unit related metabolites are usually measured for clinical diagnosis of cardiovascular diseases and atherosclerosis. Conversely, reduced plasma homocysteine levels had been reported in some clinical cases of patients who received hormone replacement therapy for post-menopause or severe hypothyroidism (10, 11). On one hand, this negative correlation between hormone and homocysteine levels may be explained by the effects of hormones (estrogen or thyroid hormones) on various enzymes including those involved in methionine metabolism. On the other hand, homocysteine levels have been found to be elevated in the plasma of patients with breast cancer (BRCA), colorectal cancer, primary hepatocellular carcinoma, and many other malignancies (12, 13); this might be manifested by altered methionine cycle that is robust during cancer pathogenesis and is related to facilitated DNA double strand breaks and other mutations. Indeed, elevated homocysteine levels have been linked to tumorigenesis through DNA hypomethylation and inactivation of tumor suppressor genes (14, 15), and it has been noted for some years that plasma homocysteine concentrations are associated with risks of tumorigenesis (16–18). However, the causal relationship between homocysteine and cancers remains controversial. For instance, certain observational studies suggest that homocysteine is a risk factor for some cancers, e.g., BRCA and prostate cancer (PrCa) (19, 20). Other studies, however, suggest otherwise (21–24).
Breast cancer constitutes a malignancy with the highest incidence rate in women worldwide (25). Epidemiological investigations showed that high estrogen levels can significantly change the endocrine environment in vivo and induce the carcinogenesis of BRCA (26, 27). Estrogen triggers transcriptional programs through estrogen receptors and cognate co-activators, and target genes include certain oncogenes that are deregulated in BRCA cells—which are thought to be the primary driving forces for the pathogenesis (28). The wealth of evidence shows that the high incidence of BRCA and ovarian cancer in women (29), along with PrCa and testicular cancer in men, might be related to the disordered hormonal status, presumably owing to the fact that relevant tissues are sensitive to hormones and chemicals that disrupt hormone balance. For instance, serum androgen level is one of the important indicators in the initial diagnosis of the majority of PrCa (30, 31), and clinical endocrine therapy of PrCa is mainly to reduce androgen levels and to inhibit the function of the androgen receptor (32). Renal cell carcinoma may also be hormone-related cancer, given that clinical studies have found that multiple hormone receptors were highly expressed in RCC tumor tissues (33) and abnormal hormone levels are of vital importance for RCC development (34, 35). In a sense, BRCA, PrCa, and RCC are all hormone-related cancers.
Epidemiological/observational studies have limitations because of (potentially) biased results, limited cases, confounding factors, and reverse causation. It is interesting to note that published observational studies on the association of homocysteine, as a potential cancer biomarker, with risks of BRCA and PrCa have drawn inconsistent conclusions (19–24). One limitation of these studies is that it is difficult to interpret the results with confounding and reverse causation. Mendelian randomization (MR) study design has significant strengths by using randomlyassigned SNPs as proxies for exposure to evaluate its causal effects on the outcome in the absence of pleiotropic effects and reverse causality (36, 37), which is unachievable by epidemiological/observational studies. Whether excess homocysteine is the cause of cancer without confounding factors remains unclear. Therefore, we designed a two-sample MR analyses to solve the problem. The MR analyses using genome-wide association study (GWAS) data have been widely employed to establish casual relationships between (genetic) exposure factors and clinical outcomes. According to the genetic law of Mendel's, SNPs are randomly-assigned during gamete formation, which can evaluate the causality between an exposure and an outcome. Mendelian randomization analyses need to satisfy three assumptions: (1) selected instrumental variant (IV) must be associated with the exposure factor; (2) selected IV can only have an effect on the outcome through the exposure; and (3) selected IV cannot be affected by the confounding factors that have an impact upon the association between the exposure and the outcome. In our study, the SNPs excluded confounding factors were the IVs, homocysteine is the exposure, and BRCA, PrCa, and RCC are the outcomes. This study aimed to identify putative causal associations between homocysteine and risks of BRCA, PrCa, and RCC.
Materials and Methods
Genetic Associations With the Plasma Level of Homocysteine
The flow chart of the MR study design is shown in Figure 1. We designed the MR analyses based on the aforementioned three assumptions to assess the associations between homocysteine and risks of BRCA, PrCa, and RCC.
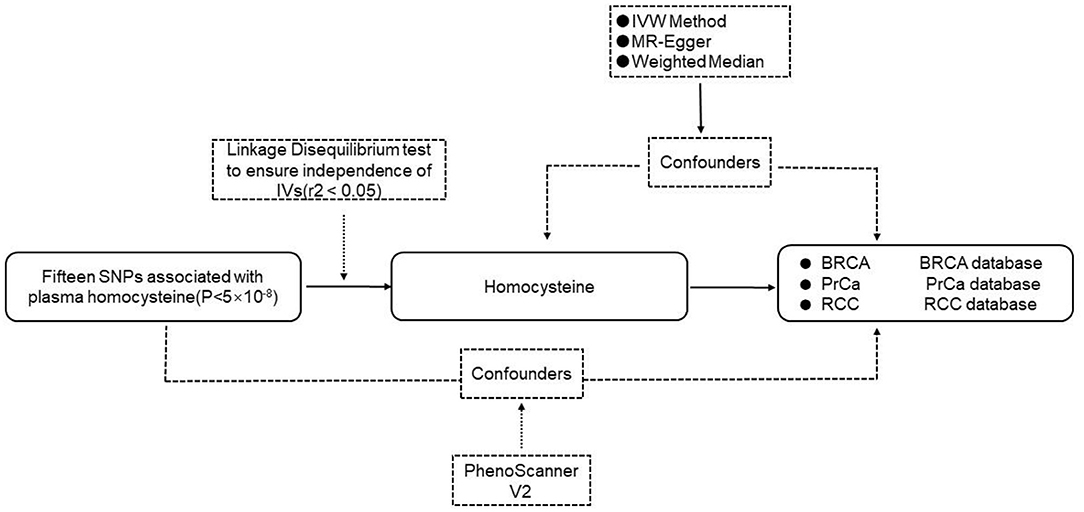
Figure 1. The flow chart of the MR study. IVs, instrumental variables; SNP, single nucleotide polymorphism; IVW, inverse-variance-weighted; BRCA, breast cancer; PrCa, prostate cancer; RCC, renal cell carcinoma.
Candidate IVs linked to plasma homocysteine concentrations were selected from a study that included data from 44,147 participants of European ancestry on total plasma homocysteine concentrations (38). This meta-analysis included individual-level data from 10 cohorts. Plasma homocysteine levels were measured in each cohort by Liquid chromatography–tandem mass spectrometry, high performance liquid chromatography, and enzymatic methods. The participants from each cohort were genotyped by using the Affymetrix 5.0 GeneChip 500K 500K (Thermo Fisher), Illumina Infinium HumanHap610K, 550, and Illumina HumanCNV370 BeadChips (Illumina). The exclusion criteria for SNPs in each cohort [minor allele frequency (MAF) <1%, P-value for the Hardy–Weinberg equilibrium (HWE) <1.0 × 10−6, call rate <95%] were used. General characteristics of the contributing cohorts and study population are described elsewhere (38). Briefly, the age of the participants of each cohort ranged from 17 to 79 years. Except for the four cohorts [The TwinsUK cohort (TUK1&TUK2), Women's Genome Health Study (WGHS), and Nurses' Health Study (NHS)], which are mainly composed of women, the number of men and the number of women in the rest of the cohorts are roughly the same (women: 45–58%).
IVs Selection and Validation
To single out the genetic variables linked to plasma homocysteine concentrations, a genome-wide significance (P < 5 × 10−8) was set as the standard to ensure a close relationship between SNPs and plasma homocysteine levels. A total of 18 SNPs were obtained from a study of total homocysteine level datasets. Among them, three SNPs (rs1801133, rs957140, and rs12921383) were weeded out because of the linkage disequilibrium (LD) to conform the independence of selected IVs (r2 < 0.05) (Supplementary Table 1). Given the primary assumption of MR analyses that IVs can only affect the outcome through the exposure, we also performed a search on the Phenoscanner website to detect the pleiotropic effects of the selected IVs and removed one SNP (rs12921383) due to its link to skin cancer; in fact, this one overlapped with the aforementioned three SNPs. Eventually, the remaining 15 SNPs were selected to analyze the relationships between genetic variants for homocysteine levels and the risk of BRCA. The characteristics of the 15 SNPs are shown in Table 1 and Supplementary Table 2.
Study Outcomes
Breast cancer, PrCa, and RCC were the outcomes. We selected the latest published GWAS meta-analysis that contained the most complete clinical data available, on three types of cancers, respectively. The sources of datasets are shown in Table 2.
Summary statistics of overall BRCA were obtained from the latest and largest GWAS meta-analysis comprising of 82 studies from the Breast Cancer Association Consortium (BCAC), including women of European ancestry (133,384 cases and 113,789 controls) [BRCA–GWAS] (39). Two Illumina iSelect genotyping arrays, iCOGS and OncoArray, were applied for sample genotyping in this study. Single-nucleotide polymorphisms with MAF < 0.005 were excluded.
For PrCa, we extracted summary-level data from the GWAS meta-analysis of Prostate Cancer Association Group to Investigate Cancer-Associated Alterations in the Genome (PRATICAL) consortium comprising of 79,148 cases and 61,106 disease-free controls of European ancestry [PrCa–GWAS] (40). This GWAS meta-analysis included the PrCa GWAS meta-analysis published in 2014, and the summary data of PrCa samples from 52 studies genotyping on the OncoArray analysis published in 2017. The QC criteria excluded SNPs with P-value for HWE < 1.0 × 10−12 in cases or P < 10−7 in controls, the call rate <95%, or with concordance <98%.
The dataset of RCC was a sex-specific GWAS meta-analysis comprising of two kidney cancer genome-wide scans for men (3,227 cases and 4,916 controls) and women (1,992 cases and 3,095 controls) of European ancestry [RCC–GWAS] (41). HumanHap 317k, 550, or 610Q were conducted to genotype the selected participants. Standard QC procedures (P-value for HWE <1.0 ×10–7, call rate <90%, and MAF < 0.05) were applied.
Statistical Analyses
In our study, three different statistical methods of two-sample MR analyses were employed. First of all, the inverse-variance-weight (IVW) approach (42) was applied for the primary two-sample MR analyses to quantify the causal associations between plasma homocysteine concentrations and the risk of three types of cancers. Briefly, the ratio of coefficients was calculated in the IV outcome regression to evaluate the causal effects. ME-Egger regression approach was used to examine the horizontal pleiotropy between IVs and the three types of cancers, independent of homocysteine levels. The intercept differs from zero (p > 0.05) and indicates no horizontal pleiotropy. In addition, a weighted median method (WM), an effective statistical tool that only needs half of the effective SNPs, was used as proof for the IVW approach by calculating the median value of selected estimates of IVs (43).
All analyses were conducted by “Mendelian Randomization” package in R version 3.6.1.
Results
Causal Associations With Diverse Cancers
To establish putative causal associations between homocysteine concentrations and BRCA, PrCa, and RCC, we performed IVW analyses to estimate causal effects. The associations between genetic variants for plasma concentrations of homocysteine and all the outcomes are listed in Supplementary Tables 3–5. Our study suggested null significant causal associations between homocysteine concentrations and three types of cancers.
The outcomes suggested no evidence that homocysteine levels had causal effects on overall BRCA (effect = 0.97, 95% CI: 0.90–1.06, P = 0.543; Figure 2; Supplementary Table 6).
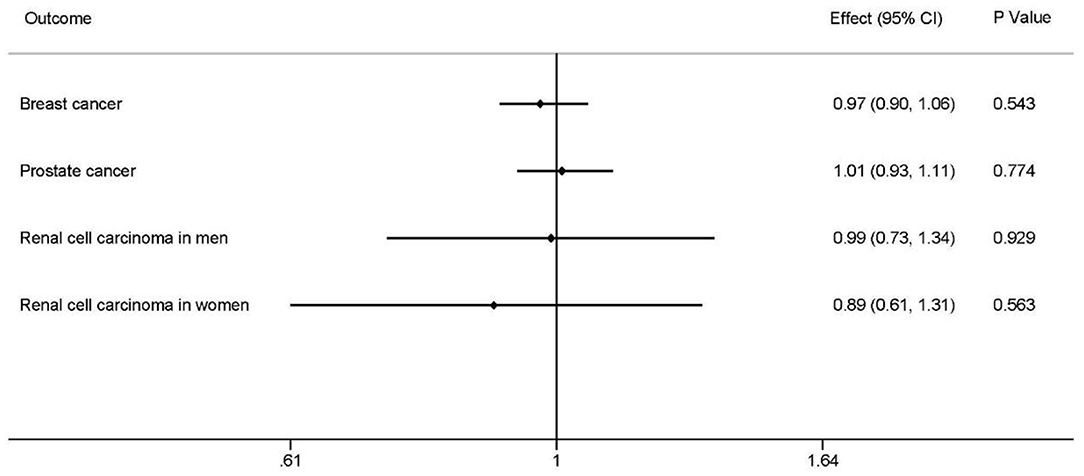
Figure 2. Causal associations between homocysteine levels and overall breast cancer, overall prostate cancer, renal cell carcinoma in men, and renal carcinoma in women. The effects, 95% CIs, and P-values of associations are contained. Effect, the combined causal effect; CI, confidence interval; P-value, P-value of the causal estimate.
There was no significant relationship between homocysteine levels and overall PrCa (effect = 1.01, 95% CI: 0.93–1.11, P = 0.774; Figure 2; Supplementary Table 6).
Inverse-variance-weight analyses showed no effects of homocysteine concentrations on RCC of different sexes, RCC in men (effect = 0.99, 95% CI: 0.73–1.34, P = 0.929), and RCC in women (effect = 0.89, 95% CIl: 0.61–1.31, P = 0.563) (Figure 2; Supplementary Table 6).
Sensitivity Analyses
The MR-Egger and the weight medium methods were used to further analyze the influence of pleiotropic effects on the final outcome. A similar conclusion was drawn according to the sensitivity analyses. The MR-Egger test showed non-zero intercept values (p > 0.05), which indicated little horizontal pleiotropy (Supplementary Table 7). Similar results were observed in the WM analyses (Supplementary Table 7). All the results confirmed no pleiotropy effects on our estimated associations.
Discussion
This two-sample MR study suggested no putative relationships between plasma homocysteine levels and the risk of BRCA, PrCa, and RCC. In addition, the levels of homocysteine were not significantly related to the risk of BRCAs with different ER expression status and RCC of different sexes.
As a plasma component involved in one-carbon metabolism, homocysteine has attracted attention in diverse clinical studies. Higher plasma homocysteine levels tend to be associated with metabolic disorders, even cancer; but the conclusions drawn from previous observational studies on associations between levels of plasma homocysteine with cancer risk are controversial given that other studies found no evidence for casual associations between genetically-determined plasma homocysteine levels with risk of hormone-related cancers. In an EPIC–Varese cohort study (23), researchers recruited 276 BRCA cases to study on the role that one-carbon metabolism plays in breast carcinogenesis. The team concluded that high homocysteine levels were not linked to BRCA risks; however, others found that increased homocysteine levels were inversely related to multiple cancers (17–19). Some case control studies also found that SNPs existed in genes involved in homocysteine metabolism. These polymorphisms were reported to be closely related to different cancers. These controversial findings may be explained by the following reasons: (1) The sample size of these observational studies might not be large enough to draw a valid conclusion; (2) establishing causality is a much more difficult task which many epidemiological techniques cannot achieve; (3) observational studies are prone to residual confounding factors, such as diversified nutrients involved in one-carbon metabolism, which results in biased conclusion, and thus inaccuracy. It is still unknown whether homocysteine is one of the causes of cancers or the result. Therefore, our MR analyses wanted to explore whether homocysteine is the cause of cancer without confounding factors. Our findings that plasma homocysteine levels had no causal effect on the risk of cancer are consistent with the EPIC–Varese cohort study.
The plasma homocysteine levels were not found to be significantly elevated in early stage cancers, as opposed to a sharp increase in their advanced stages (17). In addition, pre-menopausal and post-menopausal patients with BRCA showed different levels of plasma homocysteine (44). Moreover, upon patients receiving surgery or chemotherapy, their plasma homocysteine levels increased significantly leading to a higher incidence of thromboembolism (45), one of the most frequent complications caused by hyperhomocysteinemia (46). Regarding why homocysteine concentrations differ among different stages of cancer development whereas they lack association with cancer risk, we speculate that homocysteine might not be an independent risk factor, that is, it exerts effects by interplaying with other exposure factors. At an early stage of cancers, the homeostatic level of homocysteine may suffice to support cancer cell growth in conjunction with other methionine cycle components such as 5-MTHF, the methyl-group carrier, or other metabolite(s) like folate. An observational study suggested that women with higher homocysteine levels and low folate levels may be at risk of increased BRCA (16). It was indeed suggested that one or more micronutrients involved in homocysteine metabolism may act in combination to associate with the risk of cancer. At advanced stages, more cancer cells may encounter elevated metabolic stresses, and more nutrient-available cells can secrete homocysteine to avoid homocysteine toxicity and maintain cellular homocysteine homeostasis for those more metabolically stressed cancer cells. Such “cross-feeding” could be common among metabolically heterogeneous cancer cells. In this capacity, therefore, any role of homocysteine metabolism is likely to be complex, possibly involving a dual cancer-initiate effect in cooperation with other risk factors.
Mendelian randomization can utilize genetic instruments to analyze a large number of patient samples because of increasingly/readily more available published data from diverse GWAS datasets, which can significantly increase the accuracy thus the credibility of analyses. To the best of our knowledge, this is the first two-sample MR study that has accurately evaluated potential associations between plasma homocysteine level and cancer risk. The advantage of this MR approach is that genetic variation is a long-term and stable cause of exposure. Compared with randomized controlled trials, it is elaborated directly and is not subject to interferences by confound factors like social environment and lifestyle. In this particular study, the LD analyses were used to rule out three SNPs, and the MR-Egger intercept test and sensitivity analyses showed no pleiotropic effect of the 15 selected SNPs, which additionally increases the credibility of our study.
However, our study is not without limitations. The data that the MR analyses utilized have been collected from earlier published available GWAS summary data that have been adjusted. Further, the grouped data did not allow us to effectively explore the associations between homocysteine levels with different stages of cancer and different hormone status of different cancers. In a broader sense, the methionine cycle is but one of the pathways of one-carbon metabolism; further studies are needed to explore whether other biochemical components involved in one-carbon-fixation pathways, such as those engaged in de novo nucleotide biosynthetic pathways, are associated with risks of cancer development.
Conclusions
In conclusion, our study demonstrated no evidence of causal associations between plasma homocysteine and cancers of the BRCA, PrCa, and RCC via a two-sample MR approach. Herein, our MR study suggested that homocysteine alone might not be useful as a dynamic biomarker alone for the risks of the aforementioned three cancers.
Data Availability Statement
The original contributions presented in the study are included in the article/Supplementary Material, further inquiries can be directed to the corresponding author/s.
Author Contributions
QH, YS, and YaL conceived the study design and drafted the manuscript. ZY, ZQ and JL participated in data extraction and data analysis. XJ and YiL did the data checking and analysis. All authors critically reviewed the manuscript.
Funding
This study received funds from the China National 973 Project (2014CB542003), the China Natural Sciences Foundation Project (81372179), and the Zhejiang Provincial SciTech Commission Project (2014C03048-2) to YaL.
Conflict of Interest
The authors declare that the research was conducted in the absence of any commercial or financial relationships that could be construed as a potential conflict of interest.
Publisher's Note
All claims expressed in this article are solely those of the authors and do not necessarily represent those of their affiliated organizations, or those of the publisher, the editors and the reviewers. Any product that may be evaluated in this article, or claim that may be made by its manufacturer, is not guaranteed or endorsed by the publisher.
Supplementary Material
The Supplementary Material for this article can be found online at: https://www.frontiersin.org/articles/10.3389/fnut.2021.645371/full#supplementary-material
Abbreviations
BRCA, breast cancer; PrCa, prostate cancer; MR, mendelian randomization; RCC, renal cell carcinoma; SNP, single-nucleotide polymorphism; GWAS, genome-wide association studies; IVW, inverse-variance-weighted; IV, instrumental variable; LD, linkage disequilibrium; MAF, minor allele frequency; HWE, Hardy-Weinberg equilibrium; TUK1&TUK2, the twinsUK cohort; WGHS, women's genome health study; NHS, nurses' health study.
References
1. Eriksson S, Prigge JR, Talago EA, Arner ES, Schmidt EE. Dietary methionine can sustain cytosolic redox homeostasis in the mouse liver. Nat Commun. (2015). 6:6479. doi: 10.1038/ncomms7479
2. Ducker GS, Rabinowitz JD. One-carbon metabolism in health and disease. Cell Metab. (2017). 25:27–42. doi: 10.1016/j.cmet.2016.08.009
3. Mudd SH, Brosnan JT, Brosnan ME, Jacobs RL, Stabler SP, Allen RH, et al. Methyl balance and transmethylation fluxes in humans. Am J Clin Nutr. (2007). 85:19–25. doi: 10.1093/ajcn/85.1.19
4. Pajares MA, Perez-Sala D. Betaine homocysteine S-methyltransferase: just a regulator of homocysteine metabolism? Cell Mol Life Sci. (2006). 63:2792–803. doi: 10.1007/s00018-006-6249-6
5. Perla-Kajan J, Twardowski T, Jakubowski H. Mechanisms of homocysteine toxicity in humans. Amino Acids. (2007). 32:561–72. doi: 10.1007/s00726-006-0432-9
6. McNulty H, Scott JM. Intake and status of folate and related B-vitamins: considerations and challenges in achieving optimal status. Br J Nutr. (2008). 99(Suppl 3):S48–54. doi: 10.1017/S0007114508006855
7. Casas JP, Bautista LE, Smeeth L, Sharma P, Hingorani AD. Homocysteine and stroke: evidence on a causal link from Mendelian randomisation. Lancet. (2005). 365:224–32. doi: 10.1016/S0140-6736(05)70152-5
8. Wald DS, Law M, Morris JK. Homocysteine and cardiovascular disease: evidence on causality from a meta-analysis. BMJ. (2002). 325:1202. doi: 10.1136/bmj.325.7374.1202
9. Holmes MV, Newcombe P, Hubacek JA, Sofat R, Ricketts SL, Cooper J, et al. Effect modification by population dietary folate on the association between MTHFR genotype, homocysteine, and stroke risk: a meta-analysis of genetic studies and randomised trials. Lancet. (2011). 378:584–94. doi: 10.1016/S0140-6736(11)60872-6
10. Bicikova M, Hampl R, Hill M, Stanicka S, Tallova J, Vondra K. Steroids, sex hormone-binding globulin, homocysteine, selected hormones and markers of lipid and carbohydrate metabolism in patients with severe hypothyroidism and their changes following thyroid hormone supplementation. Clin Chem Lab Med. (2003). 41:284–92. doi: 10.1515/CCLM.2003.045
11. Guimarães DA, Cardoso J, Dusse LM, Franco RM, Franco Hde A, Alvim TC, et al. Effect of oral hormone replacement therapy on plasma homocysteine levels. Acta Obstet Gynecol Scand. (2006). 85:1304–6. doi: 10.1080/00016340600676136
12. Sun CF, Haven TR, Wu TL, Tsao KC, Wu JT. Serum total homocysteine increases with the rapid proliferation rate of tumor cells and decline upon cell death: a potential new tumor marker. Clin Chim Acta. (2002). 321:55–62. doi: 10.1016/S0009-8981(02)00092-X
13. Wu LL, Wu JT. Hyperhomocysteinemia is a risk factor for cancer and a new potential tumor marker. Clin Chim Acta. (2002). 322:21–8. doi: 10.1016/S0009-8981(02)00174-2
14. Ames BN. DNA damage from micronutrient deficiencies is likely to be a major cause of cancer. Mutat Res. (2001). 475:7–20. doi: 10.1016/S0027-5107(01)00070-7
15. Duthie SJ, Narayanan S, Brand GM, Pirie L, Grant G. Impact of folate deficiency on DNA stability. J Nutr. (2002). 132(8 Suppl):2444S−9S. doi: 10.1093/jn/132.8.2444S
16. Lin J, Lee IM, Song Y, Cook NR, Selhub J, Manson JE, et al. Plasma homocysteine and cysteine and risk of breast cancer in women. Cancer Res. (2010). 70:2397–405. doi: 10.1158/0008-5472.CAN-09-3648
17. Gatt A, Makris A, Cladd H, Burcombe RJ, Smith JM, Cooper P, et al. Hyperhomocysteinemia in women with advanced breast cancer. Int J Lab Hematol. (2007). 29:421–5. doi: 10.1111/j.1751-553X.2007.00907.x
18. Chou YC, Lee MS, Wu MH, Shih HL, Yang T, Yu CP, et al. Plasma homocysteine as a metabolic risk factor for breast cancer: findings from a case-control study in Taiwan. Breast Cancer Res Treat. (2007). 101:199–205. doi: 10.1007/s10549-006-9278-9
19. Wu X, Zou T, Cao N, Ni J, Xu W, Zhou T, et al. Plasma homocysteine levels and genetic polymorphisms in folate metablism are associated with breast cancer risk in chinese women. Hered Cancer Clin Pract. (2014). 12:2. doi: 10.1186/1897-4287-12-2
20. Gohlke JH, Lloyd SM, Basu S, Putluri V, Vareed SK, Rasaily U, et al. Methionine-homocysteine pathway in African-American prostate cancer. JNCI Cancer Spectr. (2019). 3:pkz019. doi: 10.1093/jncics/pkz019
21. Wu K, Helzlsouer KJ, Comstock GW, Hoffman SC, Nadeau MR, Selhub J. A prospective study on folate, B12, and pyridoxal 5′-phosphate (B6) and breast cancer. Cancer Epidemiol Biomarkers Prev. (1999). 8:209–17.
22. Zhang SM, Willett WC, Selhub J, Hunter DJ, Giovannucci EL, Holmes MD, et al. Plasma folate, vitamin B6, vitamin B12, homocysteine, and risk of breast cancer. J Natl Cancer Inst. (2003). 95:373–80. doi: 10.1093/jnci/95.5.373
23. Agnoli C, Grioni S, Krogh V, Pala V, Allione A, Matullo G, et al. Plasma riboflavin and vitamin B-6, but not homocysteine, folate, or vitamin b-12, are inversely associated with breast cancer risk in the European prospective investigation into cancer and nutrition-varese cohort. J Nutr. (2016). 146:1227–34. doi: 10.3945/jn.115.225433
24. Collin SM, Metcalfe C, Refsum H, Lewis SJ, Zuccolo L, Smith GD, et al. Circulating folate, vitamin B12, homocysteine, vitamin B12 transport proteins, and risk of prostate cancer: a case-control study, systematic review, and meta-analysis. Cancer Epidemiol Biomarkers Prev. (2010). 19:1632–42. doi: 10.1158/1055-9965.EPI-10-0180
25. Bray F, Ferlay J, Soerjomataram I, Siegel RL, Torre LA, Jemal A. Global cancer statistics 2018: GLOBOCAN estimates of incidence and mortality worldwide for 36 cancers in 185 countries. CA Cancer J Clin. (2018). 68:394–424. doi: 10.3322/caac.21492
26. Crandall CJ, Aragaki AK, Cauley JA, McTiernan A, Manson JE, Anderson G, et al. Breast tenderness and breast cancer risk in the estrogen plus progestin and estrogen-alone women's health initiative clinical trials. Breast Cancer Res Treat. (2012). 132:275-85. doi: 10.1007/s10549-011-1848-9
27. Yager JD, Davidson NE. Estrogen carcinogenesis in breast cancer. N Engl J Med. (2006). 354:270–82. doi: 10.1056/NEJMra050776
28. Perou CM, Sorlie T, Eisen MB, van de Rijn M, Jeffrey SS, Rees CA, et al. Molecular portraits of human breast tumours. Nature. (2000). 406:747–52. doi: 10.1038/35021093
29. Iqbal J, Kahane A, Park AL, Huang T, Meschino WS, Ray JG. Hormone levels in pregnancy and subsequent risk of maternal breast and ovarian cancer: a systematic review. J Obstet Gynaecol Can. (2019). 41:217–22. doi: 10.1016/j.jogc.2018.03.133
30. Cuzick J, Thorat MA, Andriole G, Brawley OW, Brown PH, Culig Z, et al. Prevention and early detection of prostate cancer. Lancet Oncol. (2014). 15:e484–92. doi: 10.1016/S1470-2045(14)70211-6
31. Wang G, Zhao D, Spring DJ, DePinho RA. Genetics and biology of prostate cancer. Genes Dev. (2018). 32:1105–40. doi: 10.1101/gad.315739.118
32. Hammerer P, Madersbacher S. Landmarks in hormonal therapy for prostate cancer. BJU Int. (2012). 110(Suppl 1):23–9. doi: 10.1111/j.1464-410X.2012.11431.x
33. Dunzendorfer U, Drahovsky D, Schmidt-Gayk H. [Peptide hormones LH, FSH, TSH, prolactin, beta-HCG and PTH in patients with urogenital tumors]. Onkologie. (1981). 4:188–92. doi: 10.1159/000214908
34. Zucchetto A, Talamini R, Dal Maso L, Negri E, Polesel J, Ramazzotti V, et al. Reproductive, menstrual, and other hormone-related factors and risk of renal cell cancer. Int J Cancer. (2008). 123:2213–6. doi: 10.1002/ijc.23750
35. Concolino G, Marocchi A, Conti C, Tenaglia R, Di Silverio F, Bracci U. Human renal cell carcinoma as a hormone-dependent tumor. Cancer Res. (1978). 38(11 Pt 2):4340–4.
36. Latvala A, Ollikainen M. Mendelian randomization in (epi)genetic epidemiology: an effective tool to be handled with care. Genome Biol. (2016). 17:156. doi: 10.1186/s13059-016-1018-9
37. Gala H, Tomlinson I. The use of Mendelian randomisation to identify causal cancer risk factors: promise and limitations. J Pathol. (2020). 250:541–54. doi: 10.1002/path.5421
38. van Meurs JB, Pare G, Schwartz SM, Hazra A, Tanaka T, Vermeulen SH, et al. Common genetic loci influencing plasma homocysteine concentrations and their effect on risk of coronary artery disease. Am J Clin Nutr. (2013). 98:668–76. doi: 10.3945/ajcn.112.044545
39. Zhang H, Ahearn TU, Lecarpentier J, Barnes D, Beesley J, Qi G, et al. Genome-wide association study identifies 32 novel breast cancer susceptibility loci from overall and subtype-specific analyses. Nat Genet. (2020). 52:572–81. doi: 10.1038/s41588-020-0609-2
40. Schumacher FR, Al Olama AA, Berndt SI, Benlloch S, Ahmed M, Saunders EJ, et al. Association analyses of more than 140,000 men identify 63 new prostate cancer susceptibility loci. Nat Genet. (2018). 50:928–36. doi: 10.1038/s41588-018-0142-8
41. Laskar RS, Muller DC, Li P, Machiela MJ, Ye Y, Gaborieau V, et al. Sex specific associations in genome wide association analysis of renal cell carcinoma. Eur J Hum Genet. (2019). 27:1589–98. doi: 10.1038/s41431-019-0455-9
42. Burgess S, Butterworth A, Thompson SG. Mendelian randomization analysis with multiple genetic variants using summarized data. Genet Epidemiol. (2013). 37:658–65. doi: 10.1002/gepi.21758
43. Bowden J, Davey Smith G, Haycock PC, Burgess S. Consistent estimation in Mendelian randomization with some invalid instruments using a weighted median estimator. Genet Epidemiol. (2016). 40:304–14. doi: 10.1002/gepi.21965
44. Love RR, Anker G, Yang Y, Refsum H, Ueland PM, Lonning PE, et al. Serum homocysteine levels in postmenopausal breast cancer patients treated with tamoxifen. Cancer Lett. (1999). 145:73–7. doi: 10.1016/S0304-3835(99)00233-5
45. Stathopoulou A, Vlachonikolis I, Mavroudis D, Perraki M, Kouroussis C, Apostolaki S, et al. Molecular detection of cytokeratin-19-positive cells in the peripheral blood of patients with operable breast cancer: evaluation of their prognostic significance. J Clin Oncol. (2002). 20:3404–12. doi: 10.1200/JCO.2002.08.135
Keywords: homocysteine, breast cancer, prostate cancer, renal cell carcinoma, Mendelian randomization
Citation: He Q, Yang Z, Sun Y, Qu Z, Jia X, Li J, Lin Y and Luo Y (2021) The Impact of Homocysteine on the Risk of Hormone-Related Cancers: A Mendelian Randomization Study. Front. Nutr. 8:645371. doi: 10.3389/fnut.2021.645371
Received: 23 December 2020; Accepted: 23 July 2021;
Published: 24 August 2021.
Edited by:
Catherine Frances Hughes, Ulster University, United KingdomReviewed by:
Cecilie Kyrø, Danish Cancer Society Research Center (DCRC), DenmarkRikard Landberg, Chalmers University of Technology, Sweden
Copyright © 2021 He, Yang, Sun, Qu, Jia, Li, Lin and Luo. This is an open-access article distributed under the terms of the Creative Commons Attribution License (CC BY). The use, distribution or reproduction in other forums is permitted, provided the original author(s) and the copyright owner(s) are credited and that the original publication in this journal is cited, in accordance with accepted academic practice. No use, distribution or reproduction is permitted which does not comply with these terms.
*Correspondence: Yan Luo, bHVveWFuMjAxMSYjeDAwMDQwO3pqdS5lZHUuY24=
†These authors have contributed equally to this work