- 1Unità Operativa Dipartimentale Dietetica e Nutrizione Clinica, Dipartimento Medicina Interna, Policlinico San Martino di Genova Istituto di Ricovero e Cura a Carattere Scientifico per l'Oncologia e la Neurologia, Genova, Italy
- 2Unità Operativa Complessa di Medicina Interna e Nutrizione Clinica, Dipartimento ad Attività Integrata di Medicina Interna Scienze Endocrino-Metaboliche e Malattie Infettive, Azienda Ospedaliera Universitaria Policlinico Umberto I, Rome, Italy
Low carbohydrates diets (LCDs), which provide 20–120 g of carbohydrates per day, have long been used as therapeutic options in the treatment of severe obesity, type 2 diabetes mellitus and other morbid conditions, with good results in terms of weight loss and control of the main metabolic parameters, at least in the short and medium term. According to the caloric content and the macronutrient composition, we can classify LCDs in hypocaloric, normoproteic diets [such as the Very Low-Calorie Ketogenic Diet (VLCKD) or the protein-sparing modified fasting (PSMF)], hypocaloric, hyperproteic and hyperlipidic diets (e.g., Atkins, Paleo diets…) and normocaloric, normo-/hyperproteic diets (eucaloric KD), the latter mainly used in patients with brain tumors (gliomas) and refractory epilepsy. In addition to LCD diets, another interesting dietary approach which gained attention in the last few decades is fasting and its beneficial effects in terms of modulation of metabolic pathways, cellular processes and hormonal secretions. Due to the impossibility of using fasting regimens for long periods of time, several alternative strategies have been proposed that can mimic the effects, including calorie restriction, intermittent or alternating fasting, and the so-called fasting mimicking diets (FMDs). Recent preclinical studies have shown positive effects of FMDs in various experimental models of tumors, diabetes, Alzheimer Disease, and other morbid conditions, but to date, the scientific evidence in humans is limited to some opens studies and case reports. The purpose of our narrative review is to offer an overview of the characteristics of the main dietary regimens applied in the treatment of different clinical conditions as well as of the scientific evidence that justifies their use, focusing on low and zero-carb diets and on the different types of fasting.
Low Carbohydrate Diets
The use of low or zero carbohydrate diets has long been a therapeutic option in various morbid conditions. Although over the years there has been a fluctuating position, sometimes unfavorable and sometimes favorable, regarding their use in clinical practice, currently, in light of the evidence of the literature, it finds more and more evidence in its favor, but only in certain clinical conditions (1–18).
It is useful to clarify that the term “high-protein diets,” often used in reference to low-carbohydrate diets, is incorrect because diets characterized by the reduction of the carbohydrate load can also be normo-protein diets.
From a metabolic point of view, low calorie (LCD) diets with low carbohydrate content (20–120 g of carbohydrates/day), which provide 1,000–1,200 calories per day, are indicated in the treatment of obesity as they promote a reduced increase in insulin and an increase in glucagon, which generates greater oxidation of fats (1). However, despite the theory of the insulin carbohydrate model, clinical trials that compared LCD with low fat-isoproteic diets (LFD) reported similar weight loss (2, 3) and fat loss higher when lipid intake but not carbohydrates are reduced (4). Furthermore, a meta-analysis of 32 controlled studies shows that energy expenditure and fat loss are considerably higher with LFDs than isocaloric LCDs (5).
Low calorie diets below 30–50 g of carbohydrate content that causes ketosis and mimics the physiologic state of fasting are called ketogenics: Low Calorie ketogenic diet (LCKD).
With regard to macronutrients, the difference between these diets depends on the percentage of residual calories from fats (hypo, normal or hyper lipids) and proteins.
Low calorie ketogenic diet (LCKD) are diets with carbohydrate intake <30 g/day (13% of the total energy intake), with a relative increase in fats (44%) and proteins (43%) and a total daily energy intake of 800–1,200 (3, 7, 13).
Very low calorie ketogenic diet has an energy intake of <800 cal, with a daily protein intake of about 1.2–1.5 g/kg of ideal body weight (8–14).
Protein sparing modified fast (PSMF) differs because the main source of calories are protein and not fat and therefore the calorie intake is generally lower and corresponds to 400 calories per day, always with a protein intake of 1.2–1.5 g/kg/day of protein (15–21). Carbohydrates are limited to <20–30 g per day. Therefore, VLCKD and PSMF can be considered semi-fasts slightly hyperproteic.
From the point of view of the composition in macronutrients, the difference between these diets is dependent on the percentage of residual calories from fats (low, normal or high lipids) and proteins (low, normal or high proteins).
From a systematic perspective, we can differentiate:
1) Normoproteic low calorie diets (LCD) and low calorie Ketogenic diets (LCKD);
2) normoproteic very low-calorie carbohydrate, hyperlipidic diets [very low calorie Ketogenic diets (VLCKD)];
3) normoproteic, very low-calorie carbohydrate, hypolipidic diets (protein-sparing modified fast);
4) normo or hyperprotein, normocaloric, low carbohydrate diets (eucaloric ketogenic diets) (EKD);
5) hyper-protein, low-calorie, low-carbohydrate, hyperlipidic diets (e.g., Atkins, Scarsdale, Planck, Palaeo)
Each of these types of diets has different pathophysiological bases that provide specific therapeutic indications except for hyperlipidic, protein-rich, low-calorie diets. These diets are related to greater short-term weight loss. Anyway, they are not currently suggested as they could present negative effects on metabolism and intestinal microbiota and certainly cannot be proposed as a healthy lifestyle food model (7, 22–24).
The characteristics and indications proposed for this type of diet are summarized in Table 1, while the approximate percentage of macronutrients is indicated in Figure 1.
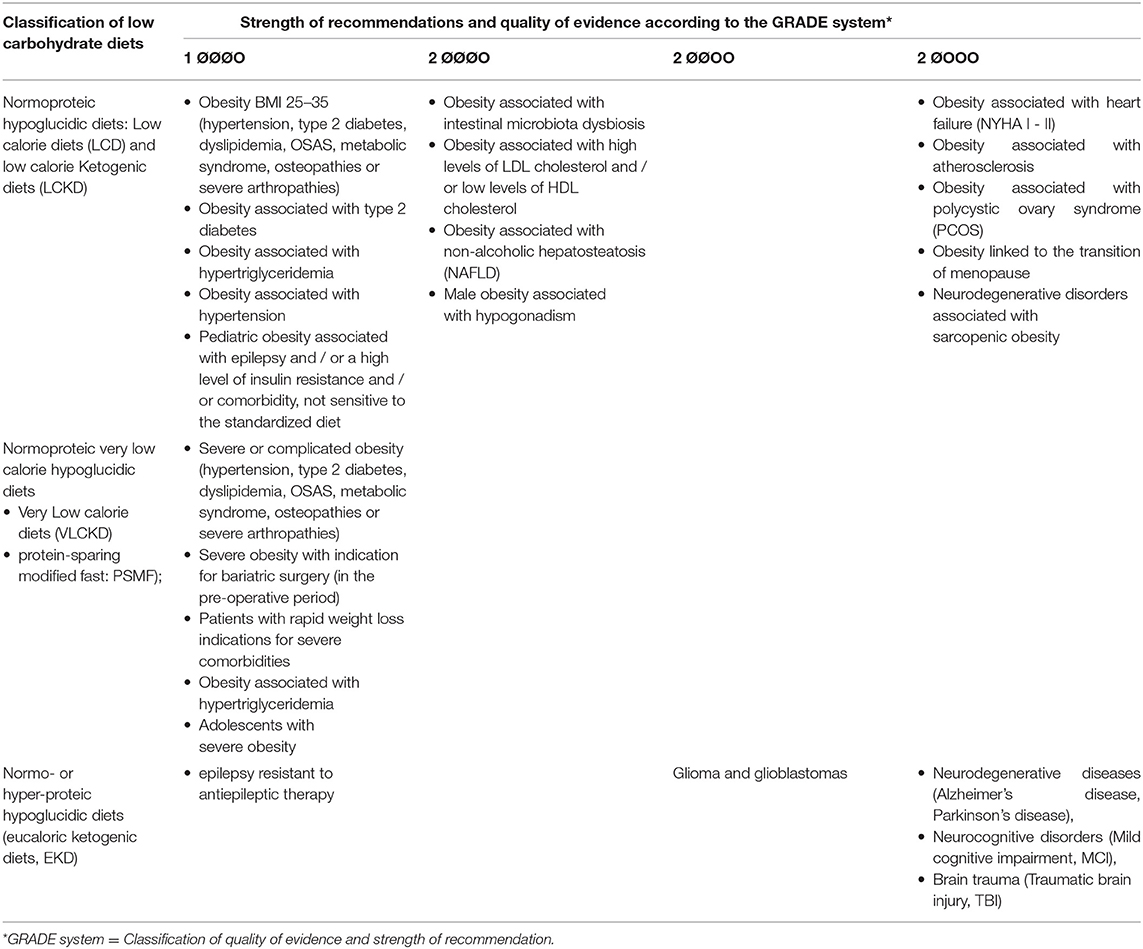
Table 1. Classification of low carbohydrates diets and strength of the recommendations for their use.
Low Calorie Ketogenic Diets
Ketogenic diet in practice is a very low carb diet with a variable fat content and usually normoproteic. In addition, different therapeutic modalities and specific variants are distinguished according to the clinical purpose: obesity, neurological pathologies, congenital metabolic pathologies, etc.
While treating obesity, ketogenic diets differ according to the calories introduced:
Low Calorie Ketogenic Diets (LCKD), Very Low Calorie Ketogenic Diets (VLCKD), modified protein-saving fast (PSMF).
Low Carbohydrates Hypoproteic Low-Calorie Diets (Low Calorie Ketogenic Diets and Very Low Ketogenic Diets)
Over the past decade, a lot of studies have documented evidence of the therapeutic efficacy of LCKD, in obesity (2–4), associated or not with comorbidity and in the preparatory phase for bariatric surgery (7–9) (Table 1).
A VLCKD, according to the guidelines of the European Food Safety Authority 2015 *, provides for the intake of <800 calories per day, a protein content of 1.2–1.5 g/kg of ideal body weight, a minimum amount of carbohydrates <30 g/day, a fat percentage of ≃44%, a minimum content of linoleic acid equal to 11 g/day and α-linolenic acid equal to 1.4 g/day, and vitamins and minerals equal to the daily needs (10–12). In addition to the low calorie intake, the main characteristic of a VLCKD diet is that it provides a reduced carbohydrate intake that stimulates the lipolysis of the storage fat and determines a physiological ketosis. The ketosis that occurs during a VLCKD always remains moderate (ketonemia never exceeding 3 mMol/L) and constitutes a physiological mechanism of energy control widely used by man in any situation of reduced glucose intake.
This ketosis is completely unlike diabetic ketoacidosis, characterized by: hyperglycemia [blood glucose (BG) > 250 mg/dl]; anion gap metabolic acidosis (pH < 7.30 and bicarbonate < 18 mEq/L); and high ketonemia which reaches 15–20 mMol/L, therefore 5–10 times higher to those of nutritional ketosis.
According to international guidelines, a VLCKD can be used continuously for up to 12 weeks, but it must always be performed under medical surveillance (7–9).
This type of diet achieves the desired weight in less time than conventional low-calorie diets. Usually, an average weight loss of 1–1.5 kg per week is achieved with variations due to gender, body type and individual physical activity.
In addition, with this diet there is an interaction between the satiating effect of proteins and the presence of ketone bodies derived from the use of storage fats, in a better appetite control, always present in traditional low-calorie diets, which is greatly attenuated starting from 36 to 48 h.
In the VLCKD, the use of industrial meal replacements is often used, which may allow greater safety with respect to food components, well-quantified and better balanced (8, 9, 11).
Of course, VLCKD is a transitional method after which, gradually, the return to a correct food style, traditionally based on an accurate balance between the various nutrients: carbohydrates, proteins and fats, must be followed.
Recent studies have demonstrated the validity of VLCKD in comparison with low-carbohydrate (LDC) non-ketogenic diets. In particular, Moreno et al. (13) conclude that VLCKD is well-tolerated and moderate and has transient side effects, and is more effective than a standard very low calorie diet (VLCD). After a year of follow-up, lean body mass was well-preserved among subjects who had lost more than 10% of their initial weight (13); equally Merra et al. (14) showed that a VLCKD was highly effective in terms of reducing body weight without inducing loss of lean mass, thus preventing the risk of sarcopenia (14). Therefore, muscle mass is not affected, but it could be maintained by adequate protein supply.
Normoproteic Low Carb Diets (CHO < 30 g/day): Protein Sparing Modified Fasting
The PSMF diet was developed in 1970 by the working group led by Bistrian and consists in the administration of only proteins for a contribution of 1.2–1.5 g/kg (ideal body weight)/day with supplementation of vitamins and minerals (15). This diet, if controlled in a medical environment, allows excellent results to be obtained even with long-term weight reduction maintenance (16–18) (Table 1). It has recently been shown that the PSMF diet can be used as an effective and safe outpatient method for rapid weight loss in adolescents with severe obesity (19). The calories introduced with this type of diet are very limited, usually < 400 kcal/day. From a nutritional point of view, this diet is not considered complete and, for this reason, nutritional supplementation is necessary. The PSMF regimen in fact involves the intake of vitamins and minerals, such as a multivitamin and 2–3 g of potassium, to compensate the lack of micronutrients due to the scarcity and limited supply of food (18). Furthermore, the consumption of at least 2 liters of calorie free liquid per day is expected (20). From the caloric point of view, the main source is a minimum amount of fat (20 g) in order to reduce the risk of cholelithiasis while the carbohydrate quota is <20 g generally ensured by the use of vegetables, while saving protein is represented by proteins that are supplied in the amount of 1.2–1.5 g/kg on the ideal weight which are actually used for energy in the first 36–48 h of metabolic shift toward ketosis and subsequently for plastic purposes (15). The proteins used are high quality proteins, to a degree that prevents or significantly reduces skeletal muscle loss. Body fat losses correspond to about 0.2 kg/day for women and 0.3 kg/day for men, and therefore in 6 weeks it is possible to obtain an average reduction of 14 kg of fat, limiting the loss of lean mass (21). The benefits of PSMF are not limited to the loss of body fat, but can also include an improvement in blood pressure, blood sugar and lipids (18). With regard to the possible side effects of the PSMF, various aspects should be considered. Since PSMF is a normoproteic diet, no risk of kidney damage is expected in both young and elderly subjects who are unable to respond to the protein increase above 2.5 g/kg/day with an increase in glomerular filtrate (22, 23). For the same reason, there are no risks of stone formation resulting from the acid-base imbalance in calcium metabolism as this risk is observed only with diets with high protein quotas (>2 g/kg/day) also associated with high energy supplies (24). In fact, PSMF could, on the other hand, be involved in improving kidney function and Poplawski et al. have actually shown, in mouse models, that a ketogenic diet regresses, even in histological terms, the process of diabetic nephropathy (25). The authors believe it is plausible that the use of a ketogenic diet for a limited period of time would produce a sustainable regression of the underlying conditions associated with diabetes, significantly resetting gene expression profile (25).
With regard to other possible side effects of the PSMF, various aspects should be considered. In terms of liver complications, it has been known since 1992 that both mild portal inflammation and fibrosis and the risk of gallbladder formation may occur following PSMF. However, subsequent data indicate that to avoid biliary stasis, due to the reduced motility of the gallbladder during PSMF, it is sufficient to introduce a minimum fat content of 10 g/day (26). Concerning the risk of osteoporosis, associated with the increase in calciuria due to acidosis linked to a high protein intake (which does not really exist in this type of diet). According to the Bonjour review, there is no causal relationship between animal proteins and increased incidence of osteoporosis fractures (27). In addition, the increased calciuria that can be observed as a result of increased protein intake from animal and plant sources can be explained by the stimulation of intestinal calcium absorption. It should also be noted that dietary proteins increase IGF-1 which exerts a positive action on bone development and formation (27). However, there is not enough evidence to argue that the benefit of protein on bone leads, in the long run, to a reduction in the risk of osteoporosis fractures (28). Finally, there is one important caveat in the literature: observations of certain deaths that have been observed as a result of ventricular arrhythmias in patients with extended periods of PSMF (16). These deaths have been proven to be the result of the use of hydrolysed collagen proteins and in addition to the lack of integration with ions and vitamins (16). It is therefore essential that a modified fasting protocol with a protein content of at least 1–1.5 g/kg/day, with 20 g of lipids, can be with adequate vitamin, hydroelectrolytic and fiber (20 g/day) and followed under close specialist medical supervision.
Normocaloric Ketogenic Diets (Hyperproteic and Normoproteic) in Pathological Conditions (Not for “weight Loss” Purposes)
Hyperproteic and normoproteic ketogenic diets (KD) have long been used in the treatment of pharmacoresistant epilepsy. In more recent times, some preclinical and clinical evidence support the use of ketogenic diets also in other areas, such as neurodegenerative diseases (Alzheimer's disease, Parkinson's disease), neurocognitive disorders (Mild cognitive impairment, MCI), brain trauma (Traumatic brain injury, TBI) even if the most interesting data come from their use in the neuro-oncological field.
Preclinical Evidence: Experimental Models of Brain Tumors and Ketogenic Diet
There are several studies published on experimental models of different types of malignant neoplasms, treated with the ketogenic diet or in combination with standard therapy (radio and chemotherapy) (Figure 2). Without entering into the details of each individual study and the type of neoplasm taken into account, the effects are globally positive on the survival or reduction of the tumor mass, with some exceptions concerning some melanoma and renal cell lines where the ketogenic diet has a promoter effect against the cancer (30, 31). Several therapeutic mechanisms exist: the reduction of the angiogenic process of the neoplasm, the radiosensitization, the chemosensitization in particular to the PI3K inhibitors, the reduction of the inflammatory processes of the tumor microenvironment and the conservation of the lean mass.
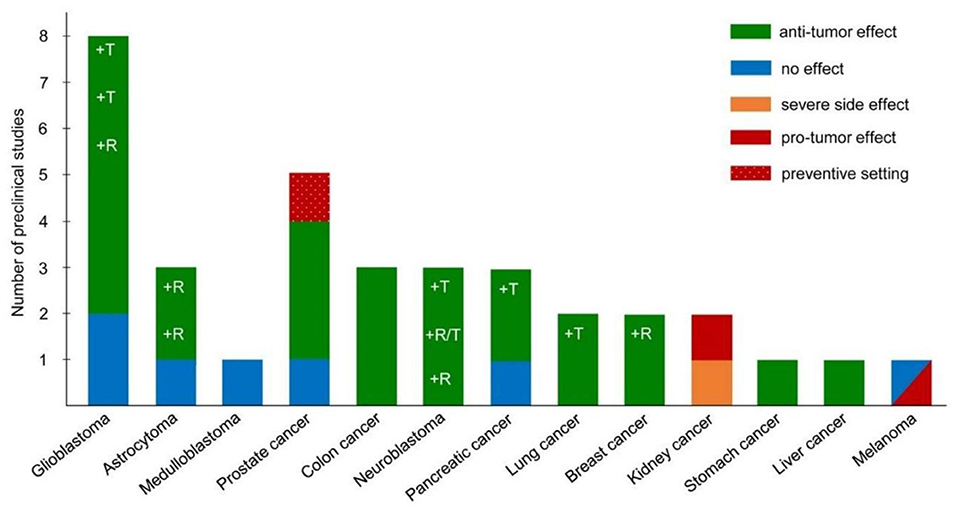
Figure 2. Pre-clinical studies on the ketogenic diet as antineoplastic therapy, adapted (29).
The promoter effects are unclear, as different tumors with similar alterations in mitochondrial oxidative metabolism have produced opposite results with such diets, as in the case of gliomas (anti-neoplastic effect) and renal carcinoma (pro-neoplastic effect). In a breast cancer model, although the neoplastic cells had an enzyme kit capable of using ketones (BDH-1 and OXCT-1, which convert ketone bodies back to Ac-CoA), there was no promoter effect of ketones. On the other hand, an antineoplastic effect has not even been observed (32).
Considering studies on glioma and astrocytoma models, the most used metabolic treatment is the classic 4: 1 or 3: 1 ketogenic diet, ad libitum or energy-restricted. Studies were conducted with both the ketogenic regimen as a unique therapy and in combination with chemotherapy (CT) or radiation therapy (RT). Although the premises based on in vitro models have been very optimistic about the antineoplastic role of beta-hydroxy-methyl-butyrate (BHB) or of aceto-acetate, the results are not always as expected in vivo. Taking into account the experimental models with control group (KD vs. standard diet), the antineoplastic effect of KD does not always occur, while an improvement in survival is evident, and in one study, the complete remission of the tumor when it was associated to RT or CT. The effect also occurs in the absence of traditional RT or CT (Temozolomide) if it is administered with metabolism antagonists of glutamine (6 - diazo - 5 - oxo - L - norleucine, DON) or glucose (2-deoxyglucose), indicating how these models of gliomas are greatly influenced by the host's metabolic and nutritional status (33, 34).
Clinical Evidence of the Ketogenic Diet in CNS Neoplasm
The available human studies are currently very heterogeneous because there is no standardized therapeutic protocol. Ongoing clinical trials seem to favor modified ketogenic diets (MKD), with a less extreme ketogenic ratio (3: 1, 2: 1 or variable as in the modified Atkins diet) and integration of nutritional supplements with MCT to facilitate ketogenesis and l adherence to the diet protocol. Besides anecdotal studies, most prospective studies are open.
The most recent prospective study considered patients with glioblastoma multiforme (GBM) (WHO grade IV) who underwent surgery and post-operative CT and RT. Nine patients were selected, of whom 6 concluded the study period (14 months, of which 12 in ketosis) (35). Before starting the RT and CT, they were started to a classic 4: 1 normal caloric KD protocol with liquid formula rich in MCT, upon reaching a plasma BHB level of 3 mM they switched to a solid diet with MCT integration (70%) to maintain high ketonemia. Quality of life and neurological aspects did not change during the study and the average survival was 12.8 months (8–19 months), in line with what was expected in the absence of a ketogenic diet (11–13 months), however as reported the authors, the sample is very limited and with great variability of survival. The authors stressed, as already described by Schwartz et al. (36), the need for continuous dietary follow-up, to solve any nutritional problems, adherence and above all to help the patient and family members to remain motivated to follow the diet protocol (35). The only clinical trial published to date, is a pilot study conducted on 20 patients with recurrent GBM, including 8 on the modified ketogenic diet with MCT drink without calorie restriction. Patients were monitored and the ketogenic regimen showed higher latency to tumor growth recovery (progression-free survival) compared to untreated patients (median 6 vs. 3 months). The patients, following the progression of the neoplasm, resumed a cycle of CT (Bevacizumab, anti VEGF drug) maintaining the ketogenic diet and arriving at a survival median of 20.1 months, compared to 16.1 months of a similar cohort of patients treated with the only drug (37). According to a systematic review of the literature on clinical and pre-clinical studies, KD is effective and safe in experimental models, and of possible benefit and safe in patients as adjuvant and neo-adjuvant therapy for the control of neoplasm and associated complications of type neurological. A number of clinical trials are underway to verify safety, compliance and efficacy in enhancing survival (34). Table 2 summarizes the clinical trials.
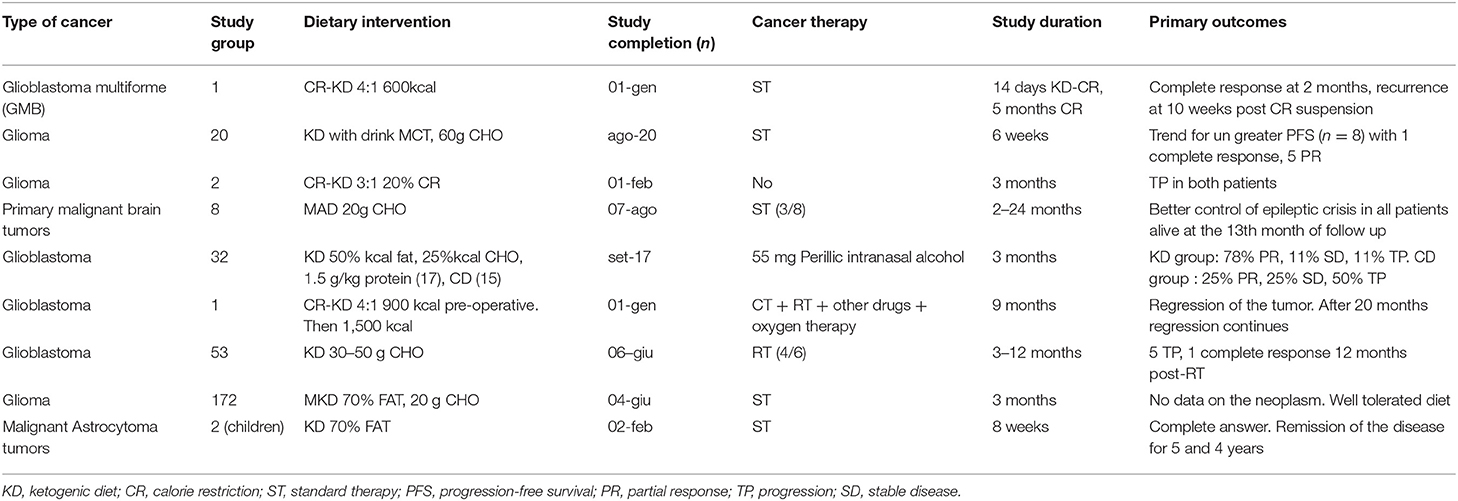
Table 2. Published clinical studies on Eucaloric Ketogenic diet in Neurological cancers, adapted from (30).
The protocols used are still very heterogeneous with one another, both in terms of ketogenic ratio and calorie intake. The choice of the protocol in addition to ensuring adequate ketosis, requires high patient adherence. This aspect is actually essential for benefiting from the metabolic modulation aspect on healthy tissues and for enhancing the effects of therapy on the tumor.
Low-Calorie, Low Carb, Hyperlipidic, Hyperproteic Diets
Scientifically verified low-carbohydrate diets include the Atkins diet (such as Plack, Scarsdale, etc.) and the Paleolithic diet. The most used variant in clinical practice is the one called Atkins diet. This regimen provides an initial “ketogenic” period where carbohydrates (<10–15g/day) (VLCKD) are strongly restricted in favor of a fairly liberalized consumption of various protein foods. Therefore, there is a spontaneously reduced caloric intake due to anorectic effect of ketones and proteins. Endogenous lipolysis provides the necessary Ac-CoA for ketogenesis (38) associated with a high amount of lipids. Popular low-protein high-protein diets, such as Atkins or Zone, produce a significant weight loss in the short term (39, 40), increasing satiety and energy expenditure and body composition (41). On the other hand, in the long term (in clinical trials longer than 1–2 years) there are no differences in weight loss (39, 40, 42–44). Furthermore, hyperlipidic high-protein diets have a higher intake of saturated fats and animal proteins and are associated with an increase in LDL cholesterol values LDL cholesterol (5, 45–48). The Paleolithic diet, also called Paleo or Paleodiet, is based on foods of daily use that imitate the food groups of the Paleolithic hunter-gatherers (49, 50). The diet is high in protein (20–35% energy) and moderate in fats and carbohydrates (22–40% energy, high glycemic index), low omega-6/omega-3 ratio low in sodium, together with a high content of unsaturated fatty acids, antioxidants, fibers, vitamins, and phytochemicals (51). Paleodiet trials showed positive effects in metabolic syndrome (52), increased insulin sensitivity (53) reduced cardiovascular risk factors (54, 55), increased satiety (56, 57) and beneficial modulation of the intestinal microbiota (58). In particular, regarding the Paleodiet for weight loss, scientific evidence indicates a constant reduction of body weight and body fat mass in short (55, 59–61) or long-term studies (62, 63). Moreover, poor adherence over time (59), poor palatability, the presence of a potential risk of deficiency which includes vitamin D, calcium (60) and iodine (64), and high costs are important factors limiting the use of this diet (65).
In conclusion, in the short term, hyperlipidic diets with high protein content with low carbohydrate content show greater effectiveness in terms of weight loss. Furthermore, negative effects on the metabolic level and the microbiota could be considered as a short-term therapeutic tool, but not as a dietary model for life. In addition, in the long term, it has been shown that diets with a different macro-nutrient composition do not have a different efficiency in weight loss.
Fasting Regimes
Apart from the physiological fasting, many treatments have tried over time to achieve the benefits that the fasting condition produces. The basic purpose of fasting is to promote changes in metabolic pathways, cellular processes and hormone secretions (66). The major physiological responses of fasting on health indicators include better insulin sensitivity (67), improved blood pressure levels (68), reduced body fat (69), blood sugar (70), atherogenic lipids (71) and inflammation (72). In the animal model, fasting is associated with interesting outcomes in correcting type 2 diabetes (73) and cardiovascular disease (74). In humans, 12–24 h of fasting are associated with a significant 20% reduction in blood glucose and hepatic glycogen depletion. Under these conditions, the transition to ketogen metabolism occurs (66). In oncology, in preclinical studies, 48-h water fasting is able to prevent DNA damage in healthy cells, usually induced by chemotherapy agents but, in addition to being difficult to accept and completed by patients, it can cause macro and micro-nutritional deficiencies (75–78).
Alternatives to Fasting
The alternative to fasting is represented by continuous calorie restriction, an approach that involves reducing 20–40% of calories continuously, which can cause numerous side effects such as irritability, depression, obsession with food and in cancer patients can lead to malnutrition, a very dangerous condition in this type of patient (78–82). For this reason, another method of daily calorie restriction can be carried out by manipulating meal times and frequencies over time (daily, weekly, monthly). This method refers to calorie restriction and therefore the length of fasting between meals to establish a new kind of strategy.
Intermittent Fasting
Intermittent fasting is the most cited among these methodologies and consists in abstaining from food and calorie drinks for a certain period of time (83, 84). Different variants of intermittent fasting differ in the duration and frequency of fasting cycles. In addition, modified intermittent fasting allows small contributions from energy foods to reduce hunger stimulation (85).
The most common types of intermittent fasting include periodic 5: 2 fasting, alternating 1:1 fasting (Figure 3), hourly food restriction (e.g., 16: 8) (Figure 4), and religious fasting (67–70, 74, 85–99).
In the experimental animal the effects are contradictory: in mice, alternating fasting is not able to reduce muscle insulin resistance induced by high fat diets and is not able to promote changes in weight loss (70, 86, 89).
In humans, although in the short term intermittent fasting allows an average weight loss of 4–10% in periods of 4–24 weeks (68, 69, 72, 87, 88, 100, 101) in the few studies lasting >6 months the reported results they are modest (68, 88, 102–104). In several clinical studies, the absence of adequate control groups suggests that intermittent fasting has not yet been rigorously studied in the long term. Two recent meta-analyses provided a summary of the effects of intermittent energy restriction in intervention studies (84, 105). Both analyses found that no intermittent or continuous calorie restriction was greater than the other for weight loss. Moreover, in a recent comparative real life study of the Mediterranean diet, Paleo diet and intermittent fasting for a period of 1 year, it has been observed that the Mediterranean diet and intermittent fasting give similar results in terms of weight loss but the Mediterranean diet allows a greater benefit in the glycemic control in relation to the consumption of plant foods with a higher fiber content (83). Finally, as regards religious fasts, the data are inconclusive: as regards Ramadan, several studies have reported weight loss (106, 107), while others have not shown any significant changes (90, 108, 109). Very often weight recovery is observed a few weeks after the fasting period (91, 107, 110) while weight loss for daily calorie restriction periods is observed but the results are still short-term. Finally, it should be stressed that fasting without adequate protein content can be harmful for some populations such as children, the elderly and underweight patients.
Fast-Mimicking Diet
The Fast-mimicking diet (FMD) is a dietary regimen, to be carried out under strict medical supervision, which provides for a very low calorie intake (300–1,100 kcal/day), as well as low is the introduction of carbohydrates and proteins in order to mimic water fasting but with possible better compliance by patients (75–77). Patients receive standardized portions of vegetable broths, soups, juices, dried fruit bars, herbal teas and in addition micronutrient supplements. Protocols provide that fast-mimicking diet is observed for 2–5 consecutive days a month. Several studies have shown efficacy in the experimental animal in various morbid conditions such as diabetes and tumors, but in humans the data are limited to open studies or case reports. The only randomized phase 2 study is a cross over that compared a group treated with three cycles of FMD with a group treated with a normal calorie diet (111). Subsequently, the group with normocaloric diet was treated with 3 cycles of FMD and the FMD group with control diet, which also was normocaloric. The result is easy to interpret: with the cross over it simply doubled in number of patients but the comparison between FMD remained the normocaloric diet. Obviously it is paradoxical to think that a fast is compared with a normocaloric diet because the results are necessarily spurious.
The absence of comparison between a low-calorie diet and a FMD is a severe bias. As a matter of facts the study demonstrated that a 3 cycles of fasting reduce body weight, waist circumference, BMI, total body and trunk fat, systolic blood pressure and IGF-1 compared to a normocaloric diet. But not only, although there is no real control group, the authors also point out that, from a post-hoc analysis, the subjects who had high risk factors or metabolic markers associated with metabolic syndrome and age-related diseases (such as a high body mass index, elevated blood pressure, high blood glucose levels, triglycerides, CRP, cholesterol and IGF-1), were significantly improved compared with non-risk individuals (111).
In fact, the same results could be observed with any low-calorie regimen conducted for the same period, even more on high-risk individuals, where it is well-known that weight loss is related to the reduction of metabolic risk factors and pressure.
Therefore, the study does not yield any actual conclusions or indications.
Consequently, it is necessary that rigorous studies are conducted with real control arms with low calorie diets. This study simply strengthens the evidence for calorie restriction in preventing chronic degenerative diseases, malignant tumors and longevity.
As regards tumors, several preclinical studies have shown that fasting diets or fast-mimicking diets exert powerful anticancer effects in experimental animals, both in solid tumor models (such as breast, lung, and gliomas) and in hematological tumors. These dietary approaches can reduce nutrient levels/factors that promote proliferation, particularly glucose, IGF1 and insulin, increase ketones body level which help to slow tumor growth and promote antitumor immunity and sensitization of cancer cells to the action of the immune system (112, 113).
By contrast, fasting, unlike other dietetic approaches, induces “starvation” modality in cells.
In fact, fasting can activate a sustained evolutionary molecular response to metabolic stress in normal cells, inhibiting their proliferation, increasing the maintenance of self with effect of protection from chemotherapy and toxic agents (114). This mechanism can be noteworthy if we consider the damage often caused by these drugs, the side effects of which can be serious or even lethal for the lesions suffered by epithelial and non-epithelial tissues. In this way, it is possible, at least in part, to explain the observed reduction of the side effects of anti-cancer treatments if the fasting mimic diet is simultaneously followed. On the contrary, cancer cells have a reverse effect (differential stress response) (114) with inhibition of stress response.
The reduction in the availability of glucose in neoplastic cells determined by FMD causes a switch from aerobic glycolysis (Warburg effect) (Figure 5) toward oxidative phosphorylation and beta-oxidation of fatty acids, a necessary condition to allow cell growth in a nutrient-poor environment (115).
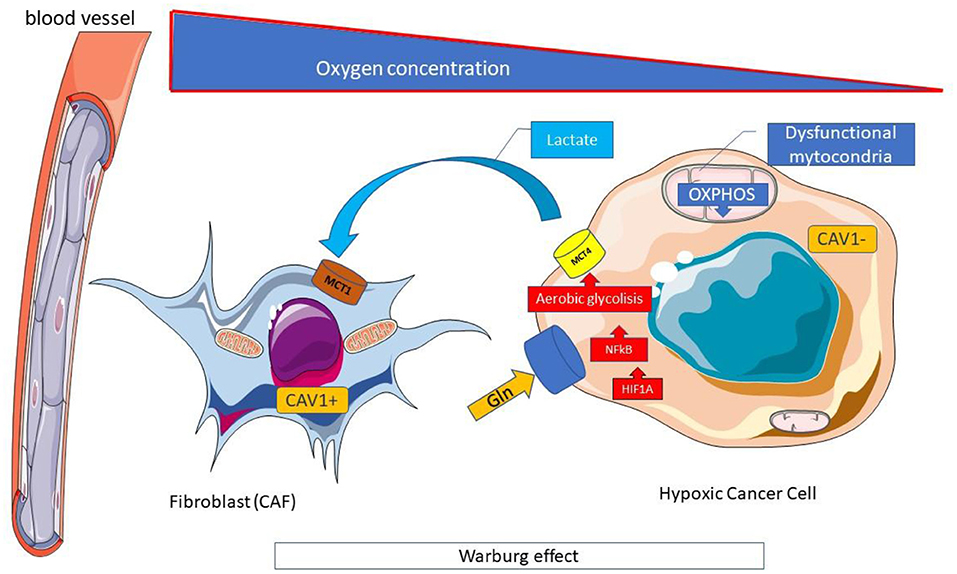
Figure 5. Warburg effect. The Warburg effect is a form of modified cell metabolism observed in many neoplastic cells where, unlike normal differentiated cells, which rely primarily on mitochondrial oxidative phosphorylation to generate the energy needed for cellular processes, relies on aerobic glycolysis. Aerobic glycolysis is an apparently inefficient way of generating adenosine 5'-triphosphate (ATP), compared to oxidative phosphorylation which allows the production of ATP by the oxidative disruption of pyruvate in mitochondria. However, the rate of glucose metabolism through aerobic glycolysis is higher, so that lactate production from glucose takes place 10–100 times faster than complete glucose oxidation in mitochondria. The metabolic difference observed by Warburg adapts cancer cells to hypoxic (oxygen deficient) conditions within solid tumors and derives largely from the same mutations of oncogenes and tumor suppressor genes that cause the other abnormal characteristics of cancer cells. Hypoxic conditions could induce HIF1A, a major regulator of glucose metabolism, and activate the expression of key enzymes for glycolysis. The high use of glucose in aerobic glycolysis, in addition to the high production of ATP, and the increase in the pathway of pentose phosphates, both essential for the anabolic processes necessary to support cell proliferation, is associated with the production of high levels of lactate and the acidification of the tumor microenvironment which plays a favorable role to the growth of neoplastic. CAV, Caveolin; Gln, Glutamine; MCT, Monocarboxylated transporter; OXPHOS, Oxidative phosphorylation.
The increase in beta-oxidation in the mitochondria, in turn, causes an increase in ROS production and, at the same time a decrease in the cell's antioxidant defenses (glutathione) occurs; the two processes amplify oxidative stress and promote the activity of chemotherapy (115). There are, however, many doubts about this, as the Warburg effect in many cell lines can be thwarted by what is referred to as the “reverse Warburg effect” (Figure 6): a new model of cancer metabolism, in which cancer cells breathe by feeding on lactic acid produced by neighboring fibroblasts (116).
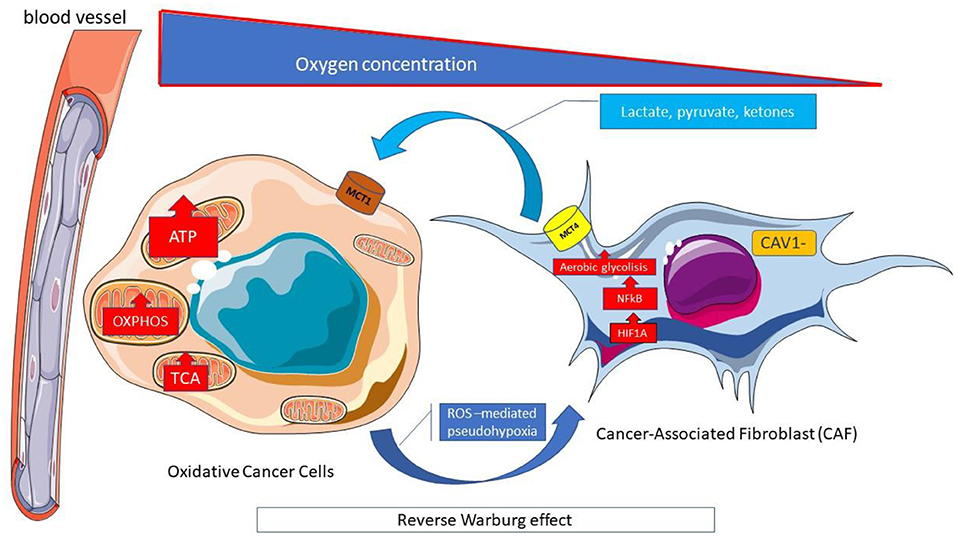
Figure 6. Reverse Warburg effect. Cells within tumors interact metabolically with the transfer of catabolites from supporting stromal cells to adjacent tumor cells. The reverse Warburg effect describes when the aerobic glycolysis of stromal fibroblasts associated with cancer metabolically supports adjacent cancer cells. The stromal-cancer metabolic coupling, allows cancer cells to generate ATP from substrates (lactate, pyruvate, ketonic bodies) provided by stromal cells, increase proliferation and reduce apoptosis. The Monocarboxylated transporter 4 MCT4 transporter is involved in the release of monocarboxylates from the tumor-associated fibroblast. It is regulated by catabolic transcription factors such as hypoxia inducible factor 1 alpha (HIF1A) and kappa-light-chain-enhancer nuclear factor of activated B-cells (NF-κB), and is highly expressed in cancer-associated fibroblasts. In contrast, MCT1 allows the absorption of these catabolites by neoplastic cells where it is highly expressed. ATP, Adenosine triphosphate; CAV, Caveolin; HIF1A; Hypoxia-inducible factor 1-alpha; MCT, Monocarboxylated transporter; NF-κB, Nuclear factor kappa B; OXPHOS, Oxidative phosphorylation; ROS, Reactive oxygen species; TCA, Tricarboxylic acid.
Indeed, in normal tissues, glucose is converted to pyruvate and transported to mitochondria for oxidative phosphorylation (OXPHOS). In Many types of tumors, some cancer cell (in particular cancer stem cells) express high levels of Caveolin 1 (Cav1) which regulates genes encoding glycolytic enzymes, glucose transporters and stimulate glycolysis, irrespective of the presence of oxygen without ATP generation by mitochondria (aerobic glycolysis or Warburg effect), in a crosstalk with Hypoxia-inducible factor 1-alpha (HIF-1α) (117, 118).
Other cancer cells (observed in breast, ovarian, prostate, liver, colon, pancreatic, and head and neck squamous cell cancer) can reprogram cancer-associated fibroblasts (CAF) that express monocarboxylate transporter 4 (MCT4) to undergo aerobic glycolysis and secrete energy-rich nutrients (lactate, pyruvate, beta-hydroxybutyrate, acetate). The CAF is obliged to feed cancerous cells that express MCT1 and have a high mitochondrial oxidative metabolism (reverse Warburg effect). These cancer cells overexpress monocarboxylate transporter1 (MCT1) (Figure 6). High stromal levels of MCT1 expression in cancer cells and high MCT4 expression in the stroma are specifically associated with poor overall survival (119).
MCT 4 expression in CAF starts from the production of reactive oxygen species (ROS) by cancer cells which freely spread in the microenvironment and enter into the adiacent CAF, causing oxidative stress (116). Oxidative stress leads to the activation of HIF-1α and NFκB (ROS-mediated pesudo-hypoxia) (Figure 5). HIF-1α triggers angiogenesis and aerobic glycolysis and moreover causes the loss of stromal Caveolin 1 (Cav-1) that amplifies oxidative stress through a “positive feedforward control” (Figure 6).
The microenvinronmental cancer metabolism could be more complex (120). As a matter of fact a Multicompartment Metabolism Model is described similarly to the Reverse Warburg Effect. In this multicompartment model the cancer cell compartment is divided into a highly proliferative population (oxidative cancer cells with MCT1 expression) and a relatively less proliferative population (Hypoxic cancer cells) (Figure 7).
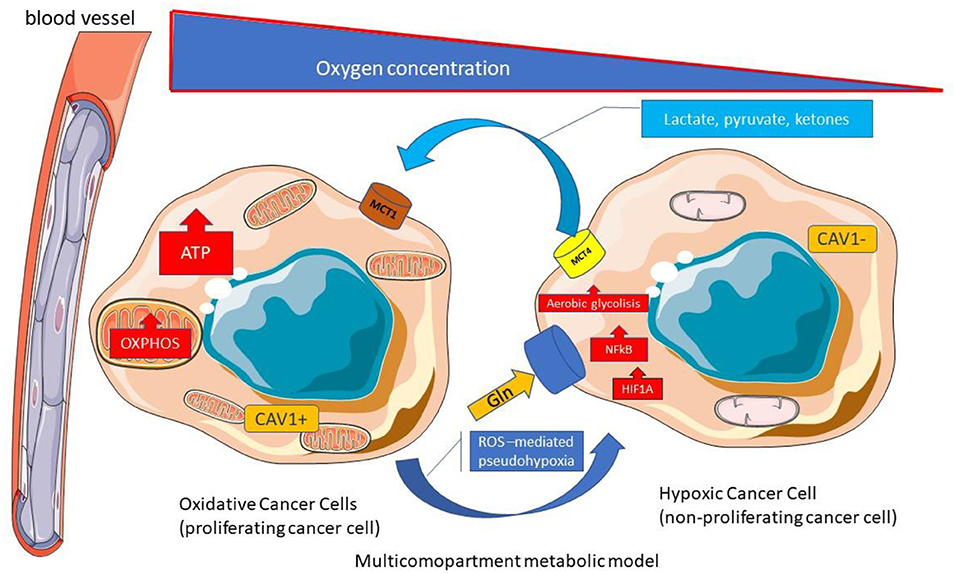
Figure 7. Multicompartment metabolic model. Multicompartimental metabolism model is similar to the reverse Warburg effect. In the multicompartimental model, the cell compartment of cancer cells is divided into a very proliferative compartment (consisting of oxidative cancerous cells with MCT1 expression) and a less proliferative compartment (consisting of hypoxic cancer cells). ATP, Adenosine triphosphate; CAV, Caveolin; Gln, Glutamine; HIF1A, Hypoxia-inducible factor 1-alpha; MCT, Monocarboxylated transporter; NF-κB, Nuclear factor kappa B; OXPHOS, Oxidative phosphorylation; TCA Tricarboxylic acid; ROS; Reactive Oxygen species.
The CAF and hypoxic cancer cells with low proliferation rates guarantee nutrients for proliferative cancer cells in the Multicompartment Metabolism Model (119). The authors suggest that multiple types of metabolism models could be found in the different cancer phenotypes and even into different areas within a single tumor (119).
Furthermore, the hypothesis of using calorie and protein restriction cannot always be used in all types of cancer. Leucine and glucose intake could provoke in some cancer type a less activation of many anabolic pathways and cell proliferation, by inhibiting apoptosis and the activation of mTOR-c1. In this regard the reduction of leucine and glucose do not stimulate growth factor receptors (VEGF, IGF-1, EGF, etc.) and insulin, without activating phosphatidylinositol-3-kinase (PI3K) and AKT.
In fact, in some tumors the PI3K/AKT/mTOR pathway can be hyperactivated regardless of endocrine signals or the availability of nutrients. Cancer cell lines that present the PI3K mutation are in fact unresponsive to the effect of food energy restriction (120).
Studying a type of diet such as DMD/FMD, which provides only a few days a month of calorie restriction, could be a new complementary approach to standard drug therapies in the treatment of cancer; a cyclic fast of this type could be well-accepted by patients and if the data were to reflect the results on experimental animals, it could increase the tolerability and efficacy of chemotherapy agents and reduce side effects. It seems that this can be a feasible, well-tolerated and relatively safe dietary treatment, but it is essential to wait for convincing results from controlled clinical studies and large cases, in order to prevent the risk of aggravation of malnutrition which is instead of high frequency in many patients oncology.
Indeed, one of the main problems related to the applicability of these types of dietary regimens in humans, and in particular in cancer patients, is the high risk of exacerbating nutritional deficiencies, malnutrition, weight loss and sarcopenia related to the cancer itself and to anticancer treatments, at least in predisposed or fragile patients. Therefore, a comprehensive nutritional assessment must be performed before including cancer patients in a clinical trial with a fasting regimen and close clinical monitoring for the entire clinical trial is equally essential.
Recently, under an accurate nutritional control periodic fasting or FMD increases the anti-cancer activity of tamoxifen and fulvestrant, delays resistance to these agents and, in combination with fulvestrant and palbociclib, causes tumor regression and reverses acquired resistance to these two drugs. A pivotal cause for the enhancement of ET anti-tumor activity by fasting or FMD appears to be the reduction in blood insulin, IGF1 and leptin, with the consequent inhibition of the PI3K–AKT–mTOR pathway, at least in part through the upregulation of EGR1 and PTEN (121). These results have been obtained thank's to a multimodality nutritional treatment: alternating FMD cycle with oral nutritional supplement (121).
Malnutrition related to the disease is one of the main causes of death and morbidity in patients with cancer, in these cases cachexia leads to weight loss and muscle wasting. The incidence and prevalence of malnutrition in patients are between 40 and 80%, so it is a very serious and by no means negligible problem (122).
It is now widely known that nutritional parameters, and in particular body composition such as phase angle (PhA), a parameter closely related to the severity of cancer malnutrition, represents an important predictor of reduced survival in cancer patients undergoing anticancer treatments (123, 124).
Author Contributions
SS was responsible for the conceptualization and design of the study and writing the manuscript. MM was responsible for the conceptualization and design and review the manuscript. All authors contributed to the article and approved the submitted version.
Conflict of Interest
The authors declare that the research was conducted in the absence of any commercial or financial relationships that could be construed as a potential conflict of interest.
The reviewer CL declared a shared affiliation with one of the authors MM to the handling editor at time of review.
References
1. Ludwig DS, Willett WC, Volek JS, Neuhouser ML. Dietary fat: from foe to friend? Science. (2018) 362:764–70. doi: 10.1126/science.aau2096
2. Foster GD, Wyatt HR, Hill JO, Makris AP, Rosenbaum DL, Brill C, et al. Weight and metabolic outcomes after 2 years on a low-carbohydrate versus low-fat diet: a randomized trial. Ann Intern Med. (2010) 153:147–57. doi: 10.7326/0003-4819-153-3-201008030-00005
3. Ebbeling CB, Feldman HA, Klein GL, Wong JMW, Bielak L, Steltz SK, et al. Effects of a low carbohydrate diet on energy expenditure during weight loss maintenance: randomized trial. BMJ. (2018) 363:k4583. doi: 10.1136/bmj.k4583
4. Hall KD, Bemis T, Brychta R, Chen KY, Courville A, CRayner EJ, et al. Calorie for calorie, dietary fat restriction results in more body fat loss than carbohydrate restriction in people with obesity. Cell Metab. (2015) 22:427–36. doi: 10.1016/j.cmet.2015.07.021
5. Hall KD, Guo J. Obesity energetics: body weight regulation and the effects of diet composition. Gastroenterology. (2017) 152:1718–27.e1713. doi: 10.1053/j.gastro.2017.01.052
6. Swiglo BA, Murad MH, Schünemann HJ, Kunz R, Vigersky RA, Guyatt GH, et al. A case for clarity, consistency, and helpfulness: state-of-the-art clinical practice guidelines in endocrinology using the grading of recommendations, assessment, development, and evaluation system. J Clin Endocrinol Metab. (2008) 93:666–73. doi: 10.1210/jc.2007-1907
7. Freire R. Scientific evidence of diets for weight loss: different macronutrient composition, intermittent fasting, and popular diets. Nutrition. (2020) 69:110549. doi: 10.1016/j.nut.2019.07.001
8. Muscogiuri G, Barrea L, Laudisio D, Pugliese G, Salzano C, Savastano S, et al. The management of very low-calorie ketogenic diet in obesity outpatient clinic: a practical guide. J Trans Med. (2019) 17:356. doi: 10.1186/s12967-019-2104-z
9. Caprio M, Infante M, Moriconi E, Armani A, Fabbri A, Mantovani G, et al. Very-low-calorie ketogenic diet [VLCKD] in the management of metabolic diseases: systematic review and consensus statement from the Italian Society of Endocrinology [SIE]. J Endocrinol Invest. (2019) 42:1365–86. doi: 10.1007/s40618-019-01061-2
10. Bueno NB, de Melo IS, de Oliveira SL, da Rocha Ataide T. Very-low-carbohydrate ketogenic diet v. low-fat diet for long-term weight loss: a meta-analysis of randomized controlled trials. Br J Nutr. (2013) 110:1178–87.21. doi: 10.1017/S0007114513000548
11. Paoli A, Rubini A, Volek JS, Grimaldi KA. Beyond weight loss: a review of the therapeutic uses of very-low-carbohydrate [ketogenic] diets. Eur J Clin Nutr. (2013) 67:789–96. doi: 10.1038/ejcn.2013.116
12. Cicero AF, Benelli M, Brancaleoni M, Dainelli G, Merlini D, Negri R. Middle and long-term impact of a very low-carbohydrate ketogenic diet on cardiometabolic factors: a multi-center, cross-sectional, clinical study. High Blood Press Cardiovasc Prev. (2015) 22:389–94. doi: 10.1007/s40292-015-0096-1
13. Moreno B, Bellido D, Sajoux I, Goday A, Saavedra D, Crujeiras AB, et al. Comparison of a very low-calorie-ketogenic diet with a standard low-calorie diet in the treatment of obesity. Endocrine. (2014) 47:793–805. doi: 10.1007/s12020-014-0192-3
14. Merra G, Miranda R, Barrucco S, Gualtieri P, Mazza M, Moriconi E, et al. Very-low-calorie ketogenic diet with aminoacid supplement versus very low restricted-calorie diet for preserving muscle mass during weight loss: a pilot double-blind study. Eur Rev Med Pharmacol Sci. (2016) 20:2613–21.
15. Bistrian DR, Winterer J, Blackburn GL, Young V, Sherman M. Effect of a protein-sparing diet and brief fast on nitrogen metabolism in mildly obese subjects. J Lab Clin Med. (1977) 89:1030–5.
16. Blackburn GL, Bray GA. Management of Obesity by Severe Caloric Restriction. Littleton: PSG Publishing Company, Inc. (1985).
17. Avenell A, Brown TJ, McGee MA, Campbell MK, Grant AM, Broom J, et al. What are the long term benefits of weight reducing diets in adults? A systematic review of randomized controlled trials. J Hum Nutr Diet. (2004) 17:317–35. doi: 10.1111/j.1365-277X.2004.00531.x
18. Walters JK, Hoogwerf BJ, Reddy SS. The protein sparing modified fast for obesity-related medical problems. Cleve Clin J Med. (1997) 64:242–4 doi: 10.3949/ccjm.64.5.242
19. Bakhach M, Shah V, Harwood T, Lappe S, Bhesania N, Mansoor S, et al. The protein-sparing modified fast diet: an effective and safe approach to induce rapid weight loss in severely obese adolescents. Glob Pediatr Health. (2016) 3:2333794X15623245. doi: 10.1177/2333794X15623245
20. Styne DM, Arslanian SA, Connor EL, Farooqi IS, Murad MH, Silverstein JH. Pediatric obesity-assessment, treatment, and prevention: an endocrine society clinical practice guideline. J Clin Endocrinol Metab. (2017) 102:709–57. doi: 10.1210/jc.2016-2573
21. Van Gaal LF, Snyders D, De Leeuw IH, Bekaert JL. Anthropometric and calorimetric evidence for the protein sparing effects of a new protein supplemented low calorie preparation. Am J Clin Nutr. (1985) 41:540–4. doi: 10.1093/ajcn/41.3.540
22. Martin WF, Cerundolo LH, Pikosky MA, Gaine PC, Maresh CM, Armstrong LE, et al. Effects of dietary protein intake on indexes of hydration. J Am Dietetic Assoc. (2006) 106:587–9. doi: 10.1016/j.jada.2006.01.011
23. Walrand S, Short KR, Bigelow ML, Sweatt AJ, Hutson SM, Nair KS. Functional impact of high protein intake on healthy elderly people. Am J Physiol Endocrinol Metab. (2008) 295:E921–8. doi: 10.1152/ajpendo.90536.2008
24. Reddy ST, Wang CY, Sakhaee K, Brinkley L, Pak CY. Effect of low-carbohydrate high-protein diets on acid-base balance, stone-forming propensity, and calcium metabolism. Am J Kidney Dis. (2002) 40:265–74. doi: 10.1053/ajkd.2002.34504
25. Poplawski MM, Mastaitis JW, Isoda F, Grosjean F, Zheng F, Mobbs CV. Reversal of diabetic nephropathy by a ketogenic diet. PLoS ONE. (2011) 6:e18604. doi: 10.1371/journal.pone.0018604
26. Festi D, Colecchia A, Larocca A, Villanova N, Mazzella G, Petroni ML, et al. Review: low caloric intake and gall-bladder motor function. Alimentary Pharmacol Ther. (2000) 14 (Suppl. 2):51–3. doi: 10.1046/j.1365-2036.2000.014s2051.x
27. Bonjour JP. Dietary protein: an essential nutrient for bone health. J Am Coll Nutr. (2005) 24:526S−36S. doi: 10.1080/07315724.2005.10719501
28. Darling AL, Millward DJ, Torgerson DJ, Hewitt CE, Lanham-New SA. Dietary protein and bone health: a systematic review and meta-analysis. Am J Clin Nutr. (2009) 90:1674–92. doi: 10.3945/ajcn.2009.27799
29. Weber DD, Aminazdeh-Gohari S, Kofler B. Ketogenic diet in cancer therapy. Aging. (2018) 10:164–5. doi: 10.18632/aging.101382
30. Weber DD, Aminzadeh-Gohari S, Tulipan J, Catalano L, Feichtinger RG, Kofler B. Ketogenic diet in the treatment of cancer – Where do we stand? Mol Metab. (2019) 33:102–21. doi: 10.1016/j.molmet.2019.06.026
31. Woolf EC, Scheck AC. The ketogenic diet for the treatment of malignant glioma. J Lipid Res. (2015) 56:5–10. doi: 10.1194/jlr.R046797
32. Bartmann C, Janaki Raman SR, Flöter J, Schulze A, Bahlke K, Willingstorfer J, et al. Beta-hydroxybutyrate [3-OHB] can influence the energetic phenotype of breast cancer cells, but does not impact their proliferation and the response to chemotherapy or radiation. Cancer Metab. (2018) 6:8. doi: 10.1186/s40170-018-0180-9
33. Abdelwahab MG, Fenton KE, Preul MC, Rho JM, Lynch A, Stafford P, et al. The ketogenic diet is an effective adjuvant to radiation therapy for the treatment of malignant glioma. PLoS ONE. (2012) 7:e36197. doi: 10.1371/journal.pone.0036197
34. Winter SF, Loebel F, Dietrich J. Role of ketogenic metabolic therapy in malignant glioma: a systematic review. Crit Rev Oncol Hematol. (2017) 112:41–58. doi: 10.1016/j.critrevonc.2017.02.016
35. van der Louw EJTM, Olieman JF, van den Bemt PMLA, Bromberg JEC, Oomen-de Hoop E, Neuteboom RF, et al. Ketogenic diet treatment as adjuvant to standard treatment of glioblastoma multiforme: a feasibility and safety study. Ther Adv Med Oncol. (2019) 11:1758835919853958. doi: 10.1177/1758835919882584
36. Schwartz KA, Noel M, Nikolai M, Chang HT. Investigating the ketogenic diet as treatment for primary aggressive brain cancer: challenges and lessons learned. Front Nutr. (2018) 5:11. doi: 10.3389/fnut.2018.00011
37. Rieger J, Bähr O, Maurer GD, Hattingen E, Franz K, Brucker D, et al. ERGO: a pilot study of ketogenic diet in recurrent glioblastoma. Int J Oncol. (2014) 44:1843–52. doi: 10.3892/ijo.2014.2382
38. Kossoff EH, Dorward JL. The modified Atkins diet. Epilepsia. (2008) 49:37–41. doi: 10.1111/j.1528-1167.2008.01831.x
39. Gardner CD, Kiazand A, Alhassan S, Kim S, Stafford RS, Balise RR, et al. Comparison of the Atkins, Zone, Ornish, and LEARN diets for change in weight and related risk factors among overweight premenopausal women: the A TO Z Weight Loss Study: a randomized trial. JAMA. (2007) 297:969–77. doi: 10.1001/jama.297.9.969
40. Shai I, Schwarzfuchs D, Henkin Y, Shahar DR, Witkow S, Greenberg I, et al. Weight loss with a low-carbohydrate, Mediterranean, or low-fat diet. N Engl J Med. (2008) 359:229–41. doi: 10.1056/NEJMoa0708681
41. Westerterp-Plantenga MS, Lemmens SG, Westerterp KR. Dietary protein—its role in satiety, energetics, weight loss and health. Br J Nutr. (2012) 108:S105–12. doi: 10.1017/S0007114512002589
42. Gardner CD, Trepanowski JF, Del Gobbo LC, Hauser ME, Rigdon J, Ioannidis JPA, et al. Effect of low-fat vs low-carbohydrate diet on 12-month weight loss in overweight adults and the association with genotype pattern or insulin secretion: the DIETFITS randomized clinical trial. JAMA. (2018) 319:667–79. doi: 10.1001/jama.2018.0245
43. Truby H, Baic S, deLooy A, Fox KR, Livingstone MBE, Logan CM, et al. Randomised controlled trial of four commercial weight loss programmes in the UK: initial findings from the BBC “diet trials.” BMJ. (2006) 332:1309–14. doi: 10.1136/bmj.38833.411204.80
44. Dalle Grave R, Calugi S, Gavasso I, El Ghoch M, Marchesini G. A randomized trial of energy-restricted high-protein versus high-carbohydrate, low-fat diet in morbid obesity. Obesity. (2013) 21:1774–81. doi: 10.1002/oby.20320
45. Mansoor N, Vinknes KJ, Veierød MB, Retterstøl K. Effects of low-carbohydrate diets v. low-fat diets on body weight and cardiovascular risk factors: a metaanalysis of randomised controlled trials. Br J Nutr. (2016) 115:466–79. doi: 10.1017/S0007114515004699
46. Retterstøl K, Svendsen M, Narverud I, Holven KB. Effect of low carbohydrate high fat diet on LDL cholesterol and gene expression in normal-weight, young adults: a randomized controlled study. Atherosclerosis. (2018) 279:52–61. doi: 10.1016/j.atherosclerosis.2018.10.013
47. Sacks FM, Bray GA, Carey VJ, Smith SR, Ryan DH, Anton SD, et al. Comparison of weight-loss diets with different compositions of fat, protein, and carbohydrates. N Engl J Med. (2009) 360:859–73. doi: 10.1056/NEJMoa0804748
48. Eaton SB, Konner M. Paleolithic nutrition. A consideration of its nature and current implications. N Engl J Med. (1985) 312:283–9. doi: 10.1056/NEJM198501313120505
49. Cordain L, Eaton SB, Sebastian A, Mann N, Lindeberg S, Watkins BA, et al. Origins and evolution of the Western diet: health implications for the 21st century. Am J Clin Nutr. (2005) 81:341–54. doi: 10.1093/ajcn.81.2.341
50. Cordain L, Miller JB, Eaton SB, Mann N, Holt SH, Speth JD. Plant-animal subsistence ratios and macronutrient energy estimations in worldwide hunter gatherer diets. Am J Clin Nutr. (2000) 71:682–92. doi: 10.1093/ajcn/71.3.682
51. Pastore RL, Brooks JT, Carbone JW. Paleolithic nutrition improves plasma lipid concentrations of hypercholesterolemic adults to a greater extent than traditional heart-healthy dietary recommendations. Nutr Res. (2015) 35:474–9. doi: 10.1016/j.nutres.2015.05.002
52. Manheimer EW, van Zuuren EJ, Fedorowicz Z, Pijl H. Paleolithic nutrition for metabolic syndrome: systematic review and meta-analysis. Am J Clin Nutr. (2015) 102:922–32. doi: 10.3945/ajcn.115.113613
53. Jonsson T, Ahr_en B, Pacini G, Sundler F, Wierup N, Steen S, et al. A Paleolithic diet confers higher insulin sensitivity, lower C-reactive protein and lower blood pressure than a cereal-based diet in domestic pigs. Nutr Metab. (2006) 3:39. doi: 10.1186/1743-7075-3-39
54. Jonsson T, Granfeldt Y, Ahr_en B, Branell US, Palsson G, Hansson A, et al. Beneficial effects of a Paleolithic diet on cardiovascular risk factors in type 2 diabetes: a randomized cross-over pilot study. Cardiovasc Diabetol. (2009) 8:35. doi: 10.1186/1475-2840-8-35
55. Ghaedi E, Mohammadi M, Mohammadi H, Ramezani-Jolfaie N, Malekzadeh J, Hosseinzadeh M, et al. Effects of a Paleolithic diet on cardiovascular disease risk factors: a systematic review and meta-analysis of randomized controlled trials. Adv Nutr. (2019) 10:634–46. doi: 10.1093/advances/nmz007
56. Jonsson T, Granfeldt Y, Erlanson-Albertsson C, Ahrén B, Lindeberg S. A Paleolithic diet is more satiating per calorie than a mediterranean-like diet in individuals with ischemic heart disease. Nutr Metab. (2010) 7:85. doi: 10.1186/1743-7075-7-85
57. Bligh HF, Godsland IF, Frost G, Hunter KJ, Murray P, MacAulay K, et al. Plantrich mixed meals based on Palaeolithic diet principles have a dramatic impact on incretin, peptide YY and satiety response, but show little effect on glucose and insulin homeostasis: an acute-effects randomised study. Br J Nutr. (2015) 113:574–84. doi: 10.1017/S0007114514004012
58. Spreadbury I. Comparison with ancestral diets suggests dense acellular carbohydrates promote an inflammatory microbiota, and may be the primary dietary cause of leptin resistance and obesity. Diabetes Metab Syndr Obes. (2012) 5:175–89. doi: 10.2147/DMSO.S33473
59. Jonsson T, Granfeldt Y, Lindeberg S, Hallberg AC. Subjective satiety and other experiences of a Paleolithic diet compared to a diabetes diet in patients with type 2 diabetes. Nutr J. (2013) 12:105. doi: 10.1186/1475-2891-12-105
60. Osterdahl M, Kocturk T, Koochek A, W€andell PE. Effects of a short-term intervention with a paleolithic diet in healthy volunteers. Eur J Clin Nutr. (2008) 62:682–5. doi: 10.1038/sj.ejcn.1602790
61. Otten J, Stomby A, Waling M, Isaksson A, Tellstrom A, Lundlin-Olsson L, et al. Benefits of a Paleolithic diet with and without supervised exercise on fat mass, insulin sensitivity, and glycemic control: a randomized controlled trial in individuals with type 2 diabetes. Diabetes Metab Res Rev. (2017) 33:e2828. doi: 10.1002/dmrr.2828
62. Mellberg C, Sandberg S, Ryberg M, Eriksson M, Brage S, Larsson C, et al. Long term effects of a Palaeolithic-type diet in obese postmenopausal women: a 2-year randomized trial. Eur J Clin Nutr. (2014) 68:350–7. doi: 10.1038/ejcn.2013.290
63. Otten J, Mellberg C, Ryberg M, Sandberg S, Kullberg J, Lindahl B, et al. Strong and persistent effect on liver fat with a Paleolithic diet during a two-year intervention. Int J Obes. (2016) 40:747–53. doi: 10.1038/ijo.2016.4
64. Manousou S, Sta_ l M, Larsson C, Mellberg C, Lindahl B, Eggersten R, et al. A Paleolithic-type diet results in iodine deficiency: a 2-year randomized trial in postmenopausal obese women. Eur J Clin Nutr. (2018) 72:124–9. doi: 10.1038/ejcn.2017.134
65. Pitt CE. Cutting through the Paleo hype: the evidence for the Palaeolithic diet. Aust Fam Physician. (2016) 45:35–8.
66. Mattson MP, Moehl K, Ghena N, Schmaedick M, Cheng A. Intermittent metabolic switching, neuroplasticity and brain health. Nat Rev Neurosci. (2018) 19:63–80. doi: 10.1038/nrn.2017.156
67. Halberg N, Henriksen M, S€oderhamn N, Stallknecht B, Ploug T, Schjerling P, et al. Effect of intermittent fasting and refeeding on insulin action in healthy men. J Appl Physiol. (2005) 99:2128–36. doi: 10.1152/japplphysiol.00683.2005
68. Varady KA, Bhutani S, Church EC, Klempel MC. Short-term modified alternate- day fasting: a novel dietary strategy for weight loss and cardioprotection in obese adults. Am J Clin Nutr. (2009) 90:1138–43. doi: 10.3945/ajcn.2009.28380
69. Eshghinia S, Mohammadzadeh F. The effects of modified alternate-day fasting diet on weight loss and CAD risk factors in overweight and obese women. J Diabetes Metab Disord. (2013) 12:4. doi: 10.1186/2251-6581-12-4
70. Anson RM, Guo Z, de Cabo R, Iyun T, Rios M, Hagepanos A, et al. Intermittent fasting dissociates beneficial effects of dietary restriction on glucose metabolism and neuronal resistance to injury from calorie intake. Proc Natl Acad Sci USA. (2003) 100:6216–20. doi: 10.1073/pnas.1035720100
71. Varady KA. Intermittent versus daily calorie restriction: which diet regimen is more effective for weight loss? Obes Rev. (2011) 12:e593–601. doi: 10.1111/j.1467-789X.2011.00873.x
72. Johnson JB, Summer W, Cutler RG, Martin B, Hyun DH, Dixit VD, et al. Alternate day calorie restriction improves clinical findings and reduces markers of oxidative stress and inflammation in overweight adults with moderate asthma. Free Radic Biol Med. (2007) 42:665–74. doi: 10.1016/j.freeradbiomed.2006.12.005
73. Cheng CW, Villani V, Buono R, Wei M, Kumar S, Yilmaz OH, et al. Fasting-mimicking diet promotes Ngn3-driven β-cell regeneration to reverse diabetes. Cell. (2017) 168:775–88.e12. doi: 10.1016/j.cell.2017.01.040
74. Mager DE, Wan R, Brown M, Cheng A, Wareski P, Abernathy DR, et al. Caloric restriction and intermittent fasting alter spectral measures of heart rate and blood pressure variability in rats. FASEB J. (2006) 20:631–7. doi: 10.1096/fj.05-5263com
75. de Groot S, Vreeswijk MP, Welters MJ, Gravesteijn G, Boei JJ, Jochems A, et al. The effects of short-term fasting on tolerance to [neo] adjuvant chemotherapy in HER2-negative breast cancer patients: a randomised pilot study. BMC Cancer. (2015) 15:652. doi: 10.1186/s12885-015-1663-5
76. Dorff TB, Groshen S, Garcia A, Shah M, Tsao-Wei D, Pham H, et al. Safety and feasibility of fasting in combination with platinum-based chemotherapy. BMC Cancer. (2016) 16:360. doi: 10.1186/s12885-016-2370-6
77. Bauersfeld SP, Kessler CS, Wischnewsky M, Jaensch A, Steckhan N, Stange R, et al. The effects of short-term fasting on quality of life and tolerance to chemotherapy in patients with breast and ovarian cancer:a randomised cross-over pilot study. BMC Cancer. (2018) 18:476. doi: 10.1186/s12885-018-4353-2
78. Arends J, Bachmann P, Baracos V, Barthelemy N, Bertz H, Bozzetti F, et al. ESPEN guidelines on nutrition in cancer patients. Clin Nutr. (2017) 36:11–48. doi: 10.1016/j.clnu.2016.07.015
79. Fontana L, Patridge L. Promoting health and longevity through diet: from model organisms to humans, Cell. (2015) 161:106–18. doi: 10.1016/j.cell.2015.02.020
80. Holloszy JO, Fontana L. Caloric restriction in humans. Exp Gerontol. (2007) 42:709–12. doi: 10.1016/j.exger.2007.03.009
81. Dirks AJ, Leeuwenburgh C. Caloric restriction in humans: potential pitfalls and health concerns. Mech Ageig Dev. (2006) 127:1–7. doi: 10.1016/j.mad.2005.09.001
82. Das SK, Roberts SB, Bhapkar MV, Villareal DT, Fontana L, Martin CK, et al. Body-composition changes in the comprehensive assessment of long-term effects of reducing intake of energy [CALERIE]-2 study: a 2-y randomised controlled trial of calorie restriction in non obese humans. Am J Clin Nutr. (2017) 105:913–27. doi: 10.3945/ajcn.116.137232
83. Jospe MR, Roy M, Brown RC, Haszard JJ, Meredith-Jones K, Fangupo LJ, et al. Intermittent fasting, Paleolithic, or Mediterranean diets in the real world: exploratory secondary analyses of a weight-loss trial that included choice of diet and exercise. Am J Clin Nutr. 111:503–14. doi: 10.1093/ajcn/nqz330
84. Headland M, Clifton P, Carter S, Keogh J. Weight-loss outcomes: a systematic review and meta-analysis of intermittent energy restriction trials lasting a minimum of 6 months. Nutrients. (2016) 8:354. doi: 10.3390/nu8060354
85. Antoni R, Johnston KL, Collins AL, Robertson MD. Effects of intermittent fasting on glucose and lipid metabolism. Proc Nutr Soc. (2017) 76:361–8. doi: 10.1017/S0029665116002986
86. Goodrick CL, Ingram DK, Reynolds MA, Freeman JR, Cider N. Effects of intermittent feeding upon body weight and lifespan in inbred mice: interaction of genotype and age. Mech Ageing Dev. (1990) 55:69–87. doi: 10.1016/0047-6374(90)90107-Q
87. Catenacci VA, Pan Z, Ostendorf D, Brannon S, Gozansky WS, Mattson MP, et al. A randomized pilot study comparing zero-calorie alternate-day fasting to daily caloric restriction in adults with obesity. Obesity. (2016) 24:1874–83. doi: 10.1002/oby.21581
88. Harvie MN, Pegington M, Mattson MP, Frystyk J, Dillon B, Evans G, et al. The effects of intermittent or continuous energy restriction on weight loss and metabolic disease risk markers: a randomized trial in young overweight women. Int J Obes. (2011) 35:714–27. doi: 10.1038/ijo.2010.171
89. Higashida K, Fujimoto E, Higuchi M, Terada S. Effects of alternate-day fasting on high-fat diet-induced insulin resistance in rat skeletal muscle. Life Sci. (2013) 93:208–13. doi: 10.1016/j.lfs.2013.06.007
90. McNeil J, Mamlouk MM, Duval K, Schwartz A, Nardo Junior N, Doucet E. Alterations in metabolic profile occur in normal-weight and obese men during the Ramadan fast despite no changes in anthropometry. J Obes. (2014) 2014:482547. doi: 10.1155/2014/482547
91. Sadeghirad B, Motaghipisheh S, Kolahdooz F, Zahedi MJ, Haghdoost AA. Islamic fasting and weight loss: a systematic review and meta-analysis. Public Health Nutr. (2014) 17:396–406. doi: 10.1017/S1368980012005046
92. Baumeier C, Kaiser D, Heeren J, Scheja L, John C, Weise C, et al. Caloric restriction and intermittent fasting alter hepatic lipid droplet proteome and diacylglycerol species and prevent diabetes in NZO mice. Biochim Biophys Acta. (2015) 1851:566–76. doi: 10.1016/j.bbalip.2015.01.013
93. Soeters MR, Lammers NM, Dubbelhuis PF, Ackermans M, Jonkers-Schuitema CF, Fliers E, et al. Intermittent fasting does not affect whole-body glucose, lipid, or protein metabolism. Am J Clin Nutr. (2009) 90:1244–51. doi: 10.3945/ajcn.2008.27327
94. Hatori M, Vollmers C, Zarrinpar A, DiTacchio L, Bushong EA, Gill S, et al. Timerestricted feeding without reducing caloric intake prevents metabolic diseases in mice fed a high-fat diet. Cell Metab. (2012) 15:848–60. doi: 10.1016/j.cmet.2012.04.019
95. Chaix A, Zarrinpar A, Miu P, Panda S. Time-restricted feeding is a preventative and therapeutic intervention against diverse nutritional challenges. Cell Metab. (2014) 20:991–1005. doi: 10.1016/j.cmet.2014.11.001
96. Chowdhury EA, Richardson JD, Tsintzas K, Thompson D, Betts JA. Effect of extended morning fasting upon ad libitum lunch intake and associated metabolic and hormonal responses in obese adults. Int J Obes. (2016) 40:305–11. doi: 10.1038/ijo.2015.154
97. Moro T, Tinsley G, Bianco A, Marcolin G, Pacelli QF, Battaglia G, et al. Effects of eight weeks of time-restricted feeding [16/8] on basal metabolism, maximal strength, body composition, inflammation, and cardiovascular risk factors in resistance-trained males. J Transl Med. (2016) 14:290. doi: 10.1186/s12967-016-1044-0
98. Sutton EF, Beyl R, Early KS, Cefalu WT, Ravussin E, Peterson CM. Early timerestricted feeding improves insulin sensitivity, blood pressure, and oxidative stress even without weight loss in men with prediabetes. Cell Metab. (2018) 27:1212–21.e1213. doi: 10.1016/j.cmet.2018.04.010
99. Kul S, Savas E, €Ozt€urk ZA, Karada_g G. Does Ramadan fasting alter body weight and blood lipids and fasting blood glucose in a healthy population? A metaanalysis. J Relig Health. (2014) 53:929–42. doi: 10.1007/s10943-013-9687-0
100. Liu H, Javaheri A, Godar RJ, Murphy J, Ma X, Rohatgi N, et al. Intermittent fasting preserves beta-cell mass in obesity-induced diabetes via the autophagylysosome pathway. Autophagy. (2017) 13:1952–68. doi: 10.1080/15548627.2017.1368596
101. Bhutani S, Klempel MC, Kroeger CM, Trepanowski JF, Varady KA. Alternate day fasting and endurance exercise combine to reduce body weight and favorably alter plasma lipids in obese humans. Obesity. (2013) 21:1370–9. doi: 10.1002/oby.20353
102. Greenway FL. Physiological adaptations to weight loss and factors favouring weight regain. Int J Obes. (2015) 39:1188e96. doi: 10.1038/ijo.2015.59
103. Varady KA, Hellerstein MK. Alternate-day fasting and chronic disease prevention: a review of human and animal trials. Am J Clin Nutr. (2007) 86:7e13. doi: 10.1093/ajcn/86.1.7
104. Klempel MC, Kroeger CM, Varady KA. Alternate day fasting [ADF] with a high-fat diet produces similar weight loss and cardioprotection as ADF with a low-fat diet. Metabolism. (2013) 62:137e43. doi: 10.1016/j.metabol.2012.07.002
105. Harris L, McGarty A, Hutchison L, Ells L, Hankey C. Short-term intermittent energy restriction interventions for weight management: a systematic review and meta-analysis. Obes Rev. (2017) 19:1–13. doi: 10.1111/obr.12593
106. Al-Hourani HM, Atoum MF. Body composition, nutrient intake and physical activity patterns in young women during Ramadan. Singapore Med J. (2007) 48:906–10.
107. Hajek P, Myers K, Dhanji AR, West O, McRobbie H. Weight change during and after Ramadan fasting. J Public Health. (2012) 34:377–81. doi: 10.1093/pubmed/fdr087
108. Yucel A, Degirmenci B, Acar M, Albayrak R, Haktanir A. The effect of fasting month of Ramadan on the abdominal fat distribution: assessment by computed tomography. Tohoku J Exp Med. (2004) 204:179–87. doi: 10.1620/tjem.204.179
109. Lamri-Senhadji MY, El Kebir B, Belleville J, Bouchenak M. Assessment of dietary consumption and time-course of changes in serum lipids and lipoproteins before, during and after Ramadan in young Algerian adults. Singapore Med J. (2009) 50:288–94.
110. Fahrial Syam A, Suryani Sobur C, Abdullah M, Makmun D. Ramadan fasting decreases body fat but not protein mass. Int J Endocrinol Metab. (2016) 14:e29687. doi: 10.5812/ijem.29687
111. Wei M, Brandhorst S, Shelehchi M, Mirzaei H, Cheng CW, Budniak J, et al. Fasting-mimicking diet and markers/risk factors for aging, diabetes, cancer, and cardiovascular disease. Sci Transl Med. (2017) 9:eaai8700. doi: 10.1126/scitranslmed.aai8700
112. Newman JC, Verdin E. Ketone bodies as signaling metabolites. Trends Endocrinol Metab. (2014) 25:42–52. doi: 10.1016/j.tem.2013.09.002
113. Lu Z, Die J, Wu G, Shen J, Collins R, Chen W, et al. Fasting selectively blocks development of acute lymphoblastic leukaemia via leptin-receptor upregulation. Nat Med. (2017) 23:79–90. doi: 10.1038/nm.4252
114. Raffaghello L, Lee C, Safdie FM, Wei M, Madia F, Bianchi G, et al. Starvation-dependent differential stress resistance protects normal but not cancer cells against high-dose chemotherapy. Proc Natl Acad Sci USA. (2008) 105:8215–20. doi: 10.1073/pnas.0708100105
115. Nencioni A, Caffa I, Cortellino S, Longo VD. Fasting and cancer: molecular mechanisms and clinical application. Nat Rev Cancer. (2018) 18:707–19. doi: 10.1038/s41568-018-0061-0
116. Wilde L, Roche M, Domingo-Vidal M, Tanson K, Philp N, Curry J, et al. Metabolic coupling and the Reverse Warburg Effect in cancer: implications for novel biomarker and anticancer agent development. Semin Oncol. (2017) 44:198–203. doi: 10.1053/j.seminoncol.2017.10.004
117. Nwosu ZC, Ebert MP, Dooley S, Meyer C. Caveolin-1 in the regulation of cell metabolism: a cancer perspective. Mol Cancer. (2016) 15:71. doi: 10.1186/s12943-016-0558-7
118. Vander Heiden MG, Cantley LC, Thompson CB. Understanding the Warburg effect: the metabolic requirements of cell proliferation. Science. (2009) 324:1029–33. doi: 10.1126/science.1160809
119. Bovenzi CD, Hamilton J, Tassone P, Johnson J, Cognetti DM, Luginbuhl A, et al. Prognostic indications of elevated MCT4 and CD147 across cancer types: a meta-analysis. BioMed Res Int. (2015) 2015:242437. doi: 10.1155/2015/242437
120. Kalaany NY, Sabatini DM. Prognostic indications of elevated MCT4 and CD147 across cancer types: tumours with PI3K activation are resistant to dietary restriction. Nature. (2009) 458:725–31. doi: 10.1038/nature07782
121. Caffa I, Spagnolo V, Vernieri C, Valdemarin F, Becherini P, Wei M, et al. Fasting-mimicking diet and hormone therapy induce breast cancer regression. Nature. (2020) 583:620–4. doi: 10.1038/s41586-020-2502-7
122. Lis CG, Gupta D, Lammersfeld CA, Markman M, Vashi PG. Role of nutritional status in predicting quality of life outcomes in cancer—A systematic review of the epidemiological literature. Nutr J. (2012) 11:27. doi: 10.1186/1475-2891-11-27
123. Sukkar SG, Giacosa A, Frascio F. Clinical validation of bioelectrical impedance [BIA] in malnourished cancer patients. RINPE. (1993) 11:78–88.
Keywords: low-carbohydrate diet, ketogenic diet, obesity, fasting, protein sparing modified fasting, fast-mimicking diet, low calorie diets, very low calorie diet
Citation: Sukkar SG and Muscaritoli M (2021) A Clinical Perspective of Low Carbohydrate Ketogenic Diets: A Narrative Review. Front. Nutr. 8:642628. doi: 10.3389/fnut.2021.642628
Received: 16 December 2020; Accepted: 28 May 2021;
Published: 12 July 2021.
Edited by:
Franco Scaldaferri, Catholic University of the Sacred Heart, ItalyReviewed by:
Antonio Herbert Lancha Jr, University of São Paulo, BrazilCarla Lubrano, Sapienza University of Rome, Italy
Marco Pizzoferrato, Catholic University of the Sacred Heart, Italy
Copyright © 2021 Sukkar and Muscaritoli. This is an open-access article distributed under the terms of the Creative Commons Attribution License (CC BY). The use, distribution or reproduction in other forums is permitted, provided the original author(s) and the copyright owner(s) are credited and that the original publication in this journal is cited, in accordance with accepted academic practice. No use, distribution or reproduction is permitted which does not comply with these terms.
*Correspondence: Samir Giuseppe Sukkar, c2FtaXJzdWtrYXJAeWFob28uaXQ=