- 1Key Lab of Medical Molecular Cell Biology of Shanxi Province, Institutes of Biomedical Sciences, Shanxi University, Taiyuan, China
- 2The Provincial Key Laboratories for Prevention and Treatment of Major Infectious Diseases Shanxi, Institutes of Biomedical Sciences, Shanxi University, Taiyuan, China
The human gastrointestinal tract harbours a complex microbial community, which interacts with the mucosal immune system closely. Gut microbiota plays a significant role in maintaining host health, which could supply various nutrients, regulate energy balance, modulate the immune response, and defence against pathogens. Therefore, maintaining a favourable equilibrium of gut microbiota through modulating bacteria composition, diversity, and their activity is beneficial to host health. Several studies have shown that probiotics and pre-biotics could directly and indirectly regulate microbiota and immune response. In addition, post-biotics, such as the bioactive metabolites, produced by gut microbiota, and/or cell-wall components released by probiotics, also have been shown to inhibit pathogen growth, maintain microbiota balance, and regulate an immune response. This review summarises the studies concerning the impact of probiotics, pre-biotics, and post-biotics on gut microbiota and immune systems and also describes the underlying mechanisms of beneficial effects of these substances. Finally, the future and challenges of probiotics, pre-biotics, and post-biotics are proposed.
Introduction
Over 1,000 bacterial species exist within the human gut, with more than 50 bacterial genera being described (1). The large intestine harbours the highest numbers of bacteria in the gastrointestinal tract, at around 1011-1012 cells per gramme (2). Gut microbiota plays a significant role in maintaining host health, which could supply various nutrients, regulate energy balance, modulate the immune response, and defence against pathogens (3). Therefore, maintaining a favourable equilibrium of gut microbiota is beneficial to host health. Several studies have found that pre-biotics, probiotics, and probiotic-driven metabolites, known as “post-biotic,” are able to improve intestinal microbiota homeostasis, maintain gut barrier integrity, and modulate immune response (Figure 1), and exert beneficial effect to host health by preventing against pathogen invasion and risks of obesity, type 2 diabetes, inflammatory bowel disease, cancer, cardiovascular, liver, and central nervous system disorders (4).
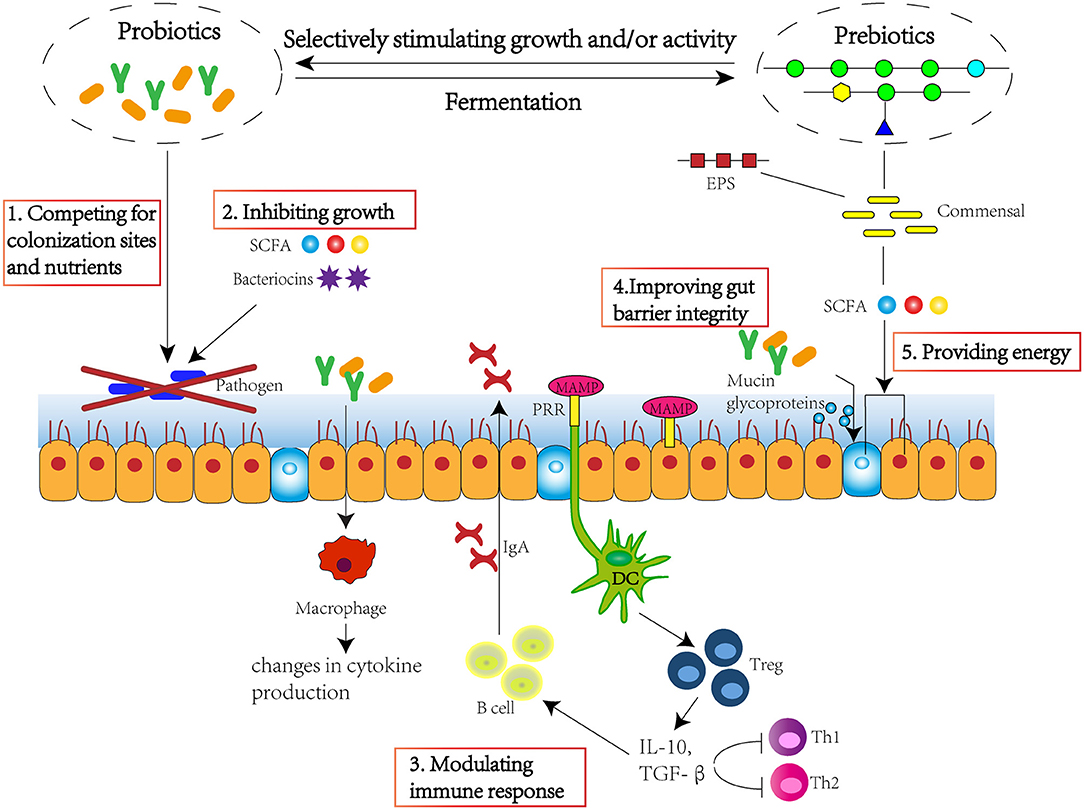
Figure 1. Pre-biotics, probiotics, and post-biotics are able to improve intestinal microbiota homeostasis, maintain gut barrier integrity, and modulate immune response. The approach mechanisms include 1. competing for colonisation sites and nutrients; 2. inhibiting growth through production of SCFA and bacteriocins; 3. modulating immune response through stimulating immune cells and cytokine and immunoglobulin production; 4. improving gut barrier integrity through stimulating the production of mucin glycoproteins; 5. providing energy for epithelium, improving villi growth, crypt development, tight junctions, and mucin production. SCFA, short-chain fatty acid; EPS, exopolysaccharide; MAMP, microorganism-associated molecular patterns; PRR, pattern recognition receptor; DC, dendritic cell; Treg, regulatory T cell; IL-10, interleukin 10; TGF-β, transforming growth factor β.
Effects of Probiotics on Gut Microbiota and Immune Function
At the beginning of the 1900s, Metchnikoff firstly introduced the scientific probiotic concept and hypothesised that longevity in Bulgarian peasants stemmed from their large intakes of fermented milk containing health-promoting microorganisms (now known as probiotics) (5). In 2001 and 2002, probiotics were defined as “live microorganisms that, when administered in adequate amounts, confer a health benefit on the host” by a WHO/FAO working party (6). In 2014, probiotics were stipulated as “defined contents, appropriate viable count at end of shelf life, and suitable evidence for health benefits” and further stated that all probiotics must be “safe for their intended use” by Hill et al. (6). These criteria were then reiterated by the International Scientific Association of Probiotics and Pre-biotics (ISAPP) in a position statement in 2018 (5). Up to now, the list of probiotics that can be used in food includes 35 species or subspecies and has been divided into three categories: lactic acid-producing bacteria (Lactobacillus, Bifidobacterium, and Enterococcus), yeast, and spore-forming Bacillus species. Bifidobacterium spp. and Lactobacillus spp. are generally isolated from healthy human colon or dairy sources (7).
Effects of Probiotics on Gut Microbiota
It is necessary to maintain the balance of the gastrointestinal microbiome, which plays a significant role in many diseases. The gastrointestinal epithelium is the first point of host contact with microorganisms, the infection, and invasion of any pathogenic bacteria will contact the mucosa of the intestinal tract and activate immune response (8). Changes in the composition and diversity of microflora are associated with several gastrointestinal diseases (inflammatory bowel disease, colorectal cancer, or irritable bowel syndrome) and parenteral diseases, such as allergies, bronchial asthma, and cystic fibrosis (9, 10).
Probiotics have been shown to influence gut microbiota in animal studies and human clinical trials (Table 1). Bifidobacterium spp. are primary beneficial bacteria inhabiting in human intestines, and the alterations in the number and composition of microbiota are one of the most common features of diseases, such as Crohn's disease (24), ulcerative colitis (25), respiratory infection disease (11), and autism (26). Probiotics could modulate human intestinal bacteria, inhibiting harmful bacteria, such as Desulfovibrio, and stimulating beneficial bacteria, such as lactic acid bacteria. In one study, probiotic Bifico (Bifidobacterium infantis, Lactobacillus acidophilus, Enterococcus faecalis, and Bacillus cereus) pre-treatment has been found to reduce the risks of colitis-associated cancer and formation of tumour in mice, through decreasing the abundance of colitogenic bacteria, such as Desulfovibrio, Mucispirillum, and Odoribacter, and increasing the abundance of Lactobacillus (12). In another animal study, probiotic yeast Saccharomyces boulardii (S. boulardii) was found to significantly increase the abundance of Bacteroidetes and decrease the abundance of Firmicutes, Proteobacteria, and Tenericutes, which were correlated with the host metabolism alterations, and indicated the potential role of S. boulardii in the treatment of obesity and type 2 diabetes (13). The impacts of probiotics on gut microbiota are also investigated and proved in human clinical trials. In a randomised, double-blind, and placebo-controlled human dietary intervention study, three different doses [5 × 109 CFU/day (high), 1.0 × 109 CFU/day (medium), and 6.5 × 107 CFU/day (low)] of B. lactis HN019 were found to significantly increase levels of bifidobacteria and lactobacilli in elderly compared to placebo. Importantly, this study demonstrated that the lowest dose of B. lactis HN019 (6.5 × 107 CFU/day) was effective in microbiota modulation in the elderly, provided B. lactis HN019 consumption guidelines (14). The mixture of probiotic strains not only showed gut microbiota modulatory effect in healthy volunteers (15) but also in volunteers with recurrent respiratory tract infection disease (11). According to these studies, probiotics could regulate and restore gut microbiota balance through stimulating the growth/activity of beneficial bacteria and suppressing those of harmful bacteria. Although the underlying mechanism of probiotics on gut microbiota modulation remains unclear, probiotics could inhibit the growth of pathogens through the production of short-chain fatty acids (SCFA) and toxins (27) and competition of colonisation sites with pathogens (28). In addition, as more next-generation probiotic strains are identified, the strain-specific effect and effective dose of them need to be investigated.
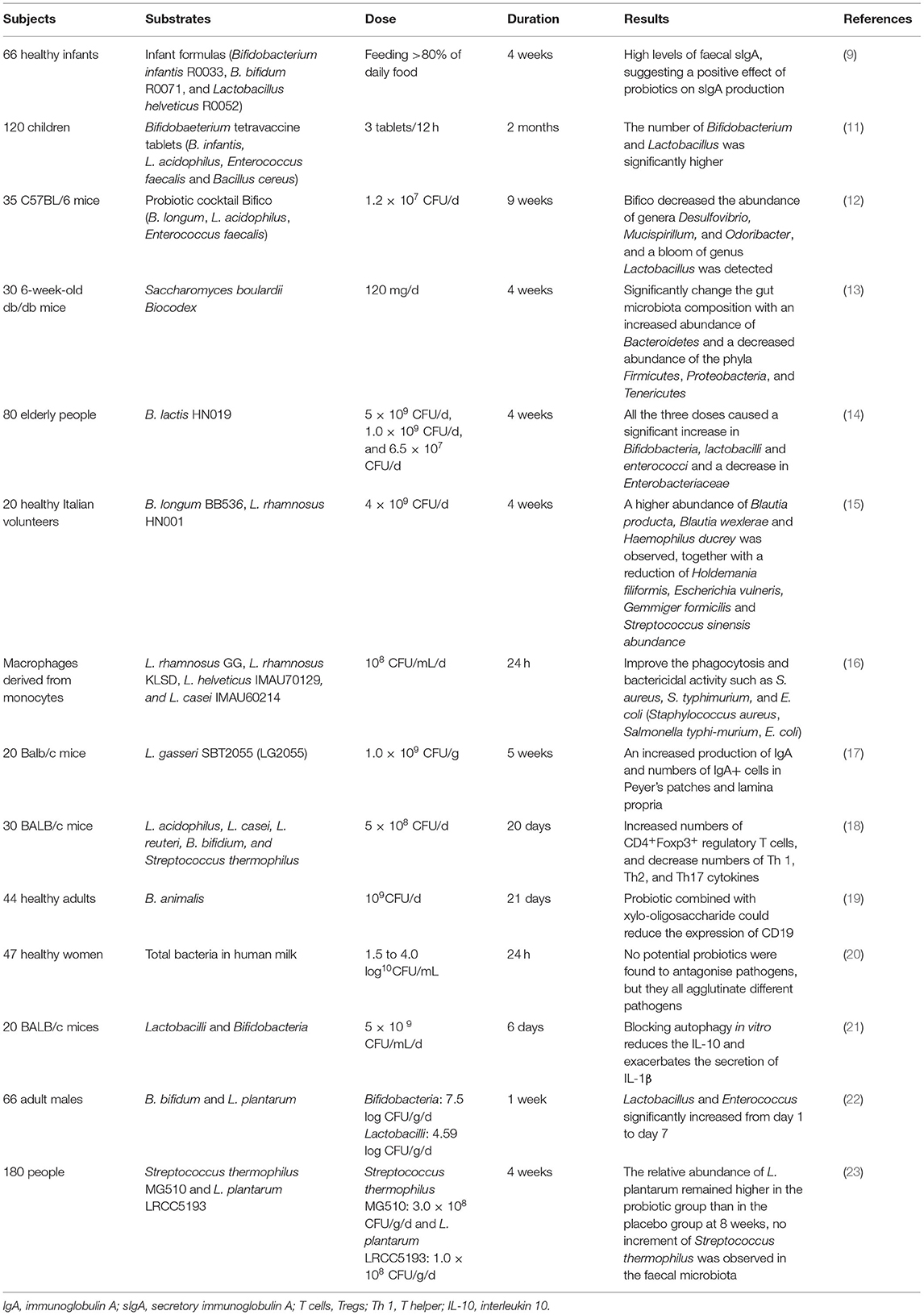
Table 1. Example of studies designed to determine effects of probiotics on gut microbiota and immune function.
Effects of Probiotics on Immune Function
Pattern recognition receptors, such as toll-like receptors (TLR), which are expressed on immune cells, could be recognised by probiotics, after which probiotics could regulate important signalling pathways, producing nuclear factor κb (NF κb) and mitogen-activated protein kinases, and communicate with the host (29). In addition, the innate immune response could be activated, resulting in the production of pro- and anti-inflammatory cytokines or chemokines (8). The effects of probiotics on immunity are summarised in Table 1.
In an in vitro study, four different strains of probiotics [Lactobacillus rhamnosus (L. rhamnosus) GG, L. rhamnosus KLSD, Lactobacillus helveticus (L. helveticus) IMAU70129, and Lacticaseibacillus casei (L. casei) IMAU60214] could stimulate innate immunity by increasing phagocytosis of human monocyte-derived macrophages, levels of reactive oxygen species (ROS), and signalling of NF-κB pp65 and TLR2 (16). Another in vivo study also found similar results, the pre-treatment of Lactobacillus johnsonii (L. johnsonii) NBRC 13952 could enhance the phagocytosis of macrophage cell line RAW264.7 on various pathogens and promote the expression of interleukin-1β (IL-1β) and CD80 (30). The regulatory effects were further investigated in animal and human studies. In an animal study, mice orally administrated with Lactobacillus gasseri (L. gasseri) SBT2055 (LG2055) were found to have increased production of IgA and numbers of IgA+ cells in Peyer's patches and lamina propria, which might result from the stimulation of transforming growth factor β (TGF-β) expression and activation of TLR2 signalling pathways (17). Kwon et al. (18) applied the animal model and found probiotics mixtures L. acidophilus, L. casei, Limosilactobacillus reuteri (L. reuteri), Bifidobacterium bifidum (B. bifidium), and Streptococcus thermophilus (5 × 108 cfu/day) could increase numbers of CD4 + Foxp3+ regulatory T cells (Tregs) and decrease numbers of T helper (Th) 1, Th2, and Th17 cytokines, contributing to inhibit progression and immune disorders in inflammatory bowel disease, atopic dermatitis, and rheumatoid arthritis. Bifidobacterium breve (B. breve) AH1205 and Bifidobacterium longum (B. longum) AH1206 also could promote the expression of transcription factor Foxp3 to induce Tregs in infant mice, which were associated with a protective effect against allergy (31). A human clinical trial also showed that healthy infants fed with infant formulas with B. infantis R0033, Bifidobacterium bifidum (B. bifidum) R0071, and L. helveticus R005 would have a higher level of faecal secretory IgA (sIgA) compared to control, which were associated with enhanced mucosal immunity (9). In addition, probiotic B. infantis 35624 has been shown to increase the proportion of Foxp3+ lymphocytes in peripheral blood in healthy volunteers and decrease levels of pro-inflammatory cytokines and C-reactive protein in psoriasis patients, chronic fatigue syndrome patients, or ulcerative colitis patients (32, 33). Childs and Röytiö (19) conducted a double-blind, placebo-controlled, randomised, factorial cross-over study to investigate the impact of probiotic Bifidobacterium animalis (B. animalis) on immune response and found that probiotics combined with xylo-oligosaccharide could reduce the expression of CD19 on B cells. These findings suggested the crucial role of probiotics in immune function regulation, not only in healthy but also in disease patients through activating important immune signalling pathways and modulating the activity of immune cells. Importantly, the role of probiotics as alternative supplementation in disease treatment and the underlying mechanisms need to be further investigated.
Effects of Pre-biotics on Gut Microbiota and Immune Function
The concept of pre-biotics was introduced by Gibson and Roberfroid when they observed certain non-digestible oligosaccharides were selectively fermented by bifidobacteria (34). The concept of pre-biotics was firstly proposed as a selectively fermented ingredient that results in specific changes, in the composition and/or activity of the gastrointestinal microbiota, thus conferring benefit(s) upon host health (35). Subsequently, with the improved knowledge of gut microbiota composition and underlying mechanisms of pre-biotics (36), the definition of pre-biotics was updated in 2017 as “a substrate that is selectively utilised by host microorganisms conferring a health benefit” by an ISAPP consensus panel led by Gibson (5). Pre-biotics, such as fructooligosaccharides, galactooligosaccharides (GOS), and lactulose, have been found to significantly modulate the balance of the large intestine microorganism community by increasing the number of bifidobacteria and lactic acid bacteria (37). Other nutrients, such as pectin, cellulose, and xylan, are also beneficial to the balance of intestinal microorganisms (38). Pre-biotics play an important role in the prevention of diarrhoea (39), constipation (40), metabolic disease (41), and cancer (42) and confer positive effects on lipid metabolism (43), mineral adsorption (44), and immune regulation (45).
Effect of Pre-biotics on Gut Microbiota
Pre-biotics could regulate the balance of intestinal microorganisms and improve colon health (46–49) (Table 2). Inulin-type fructans (ITFs) have shown bifidogenic effects in in vitro and human studies (46). Inulin was found to increase bifidobacteria and lactobacilli in faecal samples from the elderly population (67, 68) and healthy adults (69) in vitro. In a human study, 30 obese women were recruited and randomly divided into 2 groups, one group daily received 16g ITF (n = 15), the other group maltodextrin (n = 15) for 3 months. Qualitative and quantitative analyses of faecal microbiota found that ITF intervention increased levels of Bifidobacterium and Faecalibacterium prausnitzii and decreased levels of Bacteroides intestinalis and Bacteroides vulgate compared to maltodextrin (50). In healthy adults, inulin could also modulate gut microbiota composition, with a significant increase in the numbers of bifidobacteria (70). Although inulin has shown modulatory impact on gut microbiota, the impact is related to the degree of polymerization (DP) of inulin. In a mice model study, high-fat-diet feeding obesity mice received inulin with DP ≤ 9 and DP ≥ 23, respectively, for 8 weeks. The results showed the inulin with longer DP could decrease the abundance of Firmicutes and increase the abundance of Bacteroidetes more significantly and perform a more beneficial impact on liver injury (71). In addition, the impact of GOS on gut microbiota was also well-studied. In a mice study, the effect of GOS in prevention and alleviation against Escherichia coli (E. coli) O157 invasion and colonisation was studied. The results showed that GOS could stimulate the growth and activities of beneficial bacteria, such as Akkermansia, Ruminococcaceae, and Bacteroides, and promote the production of SCFA (51). GOS could also increase the abundance of Lactobacillus and Lactococcus in rats with constipation compared to placebo, hence suppression of constipation and exerting a beneficial impact on colon health (72). In addition, GOS has also been shown to modulate gut microbiota with bifidogenic effect in volunteers with gastrointestinal symptoms, such as bloating, flatulence (73), autism (74), and those aged over 60 (67, 68). Other pre-biotics also showed a modulatory impact on gastrointestinal microorganisms. Pre-biotic Mushroom, Bulgaria inquinans (BI) was assessed in male C57BL/6 mice to investigate its impact on gut microbiota. Mice received BI (1 or 2%) for 4 weeks, and the results indicated the decreased diversity of gastrointestinal bacteria, increased abundance of Faecalibaculum and Parabacteroides, and decreased abundance of Allobaculum and Rikenella (52). A study showed that moderate intake of red wine polyphenols could regulate gut microbiota composition in patients with metabolic syndrome, with significantly increased the number of Bifidobacterium, Lactobacillus, and butyrate-producing bacteria (Faecalibacterium prausnitzii and Roseburia) in faeces (41). In a 100 healthy adult study, volunteers consumed placebo, 2′-O-fucosyllactose (2′FL), lacto-N-neotetraose (LNnT), or 2′FL+LNnT (2:1 mass ratio; mix) at 5, 10, or 20 g daily. The results showed that 2′FL and/or LNnT could significantly increase the relative abundance of Actinomycetes and Bifidobacterium and decrease those of Firmicutes and Proteobacteria, hence maintaining gut microbiota balance (53). Although the gastrointestinal microbiota can be modulated by pre-biotics, individual responses can vary. For example, a study aimed to investigate the impact of GOS (0.0, 2.5, 5.0, and 10.0 g GOS) on faecal microbiota of healthy human subjects found that GOS mainly increased the abundance of organisms within the Actinobacteria. However, only 50% of these changes can be detected by individuals. In addition, the response to the GOS varies from individual to individual (75). According to these findings, pre-biotics have been shown to modulate microbiota composition, and these effects could result in alleviating symptoms observed in patients. In addition, different pre-biotics are specific to different bacteria and their effect doses are also different. Therefore, it is important to make scientific results and conclusions reliable and develop proper instructions for consumers.
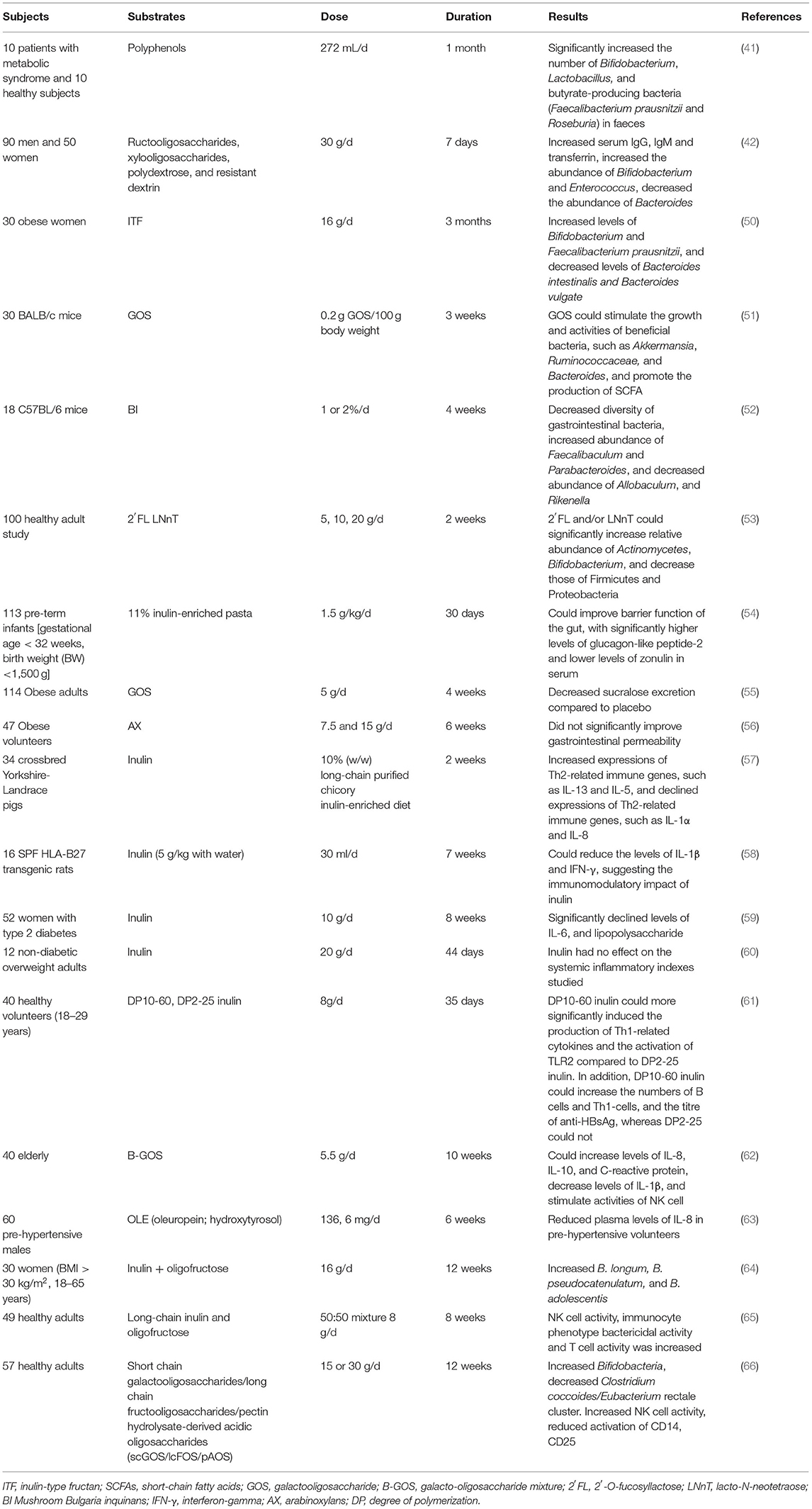
Table 2. Example of studies designed to determine effects of pre-biotics on gut microbiota and immune function.
Effects of Pre-biotics on Immune Function
Protective Effect of Pre-biotics on Intestinal Epithelial Barrier
The protective effects of pre-biotics on intestinal epithelium have been confirmed, and host immunity can be enhanced by improving the integrity of intestinal epithelium (76). In a human study, 11% inulin-enriched pasta could improve the barrier function of the gut, with significantly higher levels of glucagon-like peptide-2 and lower levels of zonulin in serum, hence protecting mucosal barrier integrity and preventing gastrointestinal diseases (54). GOS could also help maintain gut barrier function and improve colon permeability. Obese adults who received 5 g/d GOS for 4 weeks showed a decreased sucralose excretion compared to placebo, suggesting improved barrier function induced by GOS (55). Similar results of GOS were also found in preterm infants (77). Although inulin and GOS could help maintain gut barrier integrity, the impacts of arabinoxylans (AX) remain controversial. In one human study, two different doses of AX (7.5 and 15 g/d) did not significantly improve gastrointestinal permeability in obese volunteers (56). Therefore, the impact of pre-biotics on the integrity and function of the intestinal epithelial barrier needs to be further assessed.
Effects of Pre-biotics on Immune Response
Pre-biotics may also contribute to the regulation of immune response through inhibiting expressions of pro-inflammatory cytokines, stimulating those of anti-inflammatory cytokines, and promoting activities of immune cells, such as macrophages, NK cells, T cells, and B cells (Table 2). In a porcine model study, inulin was found to induce an anti-inflammatory immune response against pathogen infection, with increased expressions of Th2-related immune genes, such as IL-13 and IL-5, and declined expressions of Th2-related immune genes, such as IFNG, IL-1α, and IL-8, which were closely related with microbiota composition (57). In another animal study, 16 SPF HLA-B27 transgenic rats were divided into two groups, the inulin group fed with the combination of chicory-derived long-chain ITFs and short-chain inulin fraction oligofructose, and the control group fed with placebo for 7 weeks. The caecum and colon tissue were collected and analysed for cytokine production. The results showed that pre-biotic intervention could reduce the levels of IL-1β and interferon-gamma (IFN-γ), suggesting the immunomodulatory impact of inulin (58). In addition, inulin could also help to regulate immune markers in patients with type 2 diabetes, with significantly declined levels of tumour necrosis factor-α (TNF-α), IL-6, and lipopolysaccharide (59). However, inulin supplementation could not regulate systemic inflammatory markers in a human study, in which twelve non-diabetic overweight adults daily received 20 g inulin for 44 days (60), the results showed that inulin had no impact on IL-8. Notably, the immunomodulatory impact of inulin depends on the DP or chain length. A double-blind, placebo-controlled human study found that DP10-60 inulin could more significantly induce the production of Th1-related cytokines and the activation of TLR2 compared to DP2-25 inulin. In addition, DP10-60 inulin could increase the numbers of B cells, Th1-cells, and the titre of anti-HBsAg, whereas DP2-25 could not (61). The impact of GOS on the immune response is also investigated. A study, which assessed the influence of Bimuno-GOS (B-GOS) on immune function of 40 elderly, found 5.5 g daily intake of B-GOS for 10 weeks could increase levels of IL-8, IL-10, and C-reactive protein, decrease levels of IL-1β, and stimulate activities of NK cells, suggesting the beneficial effects induced by B-GOS supplementation on ageing population (62). The results were in agreement with previous studies on overweight adults (78), elderly volunteers (79), and in vitro studies (67, 68). Not only inulin and GOS but other pre-biotics also showed immunomodulatory activity. Pre-biotic Mushroom BI was assessed in male C57BL/6 mice to investigate its impact on the host immune response. The results showed that 1 and 2% BI intervention could stimulate the proliferation of T cells from the spleen, and 2% BI could even increase IL-2 production in splenocytes, consequently influencing the peripheral and mucosal immune systems (52). In a randomised, controlled study, polyphenol supplementation reduced plasma levels of IL-8 in pre-hypertensive volunteers (63). In summary, pre-biotics could enhance host immunity in health and disease patients through protecting intestinal barrier function, activating immune cells, and regulating immune signalling pathways.
Effects of Post-biotics on Gut Microbiota and Immune Function
Post-biotics include cell wall components, such as protein molecules and lipopolysaccharides, extracellular polysaccharides, and microbial metabolites of carbohydrate fermentation or protein degradation, such as SCFA and branched chain fatty acids (80). Several studies have found that post-biotics can exert positive biological functions to the host (4, 81).
Effect of Post-biotic on Gut Microbiota
Post-biotic has great potential to maintain homeostasis of intestinal microbiota and improve intestinal health, through inhibiting the growth and activities of harmful bacteria and stimulating those of beneficial bacteria (Table 3). The impact of metabolic products of probiotic fermentation is well-studied. Cell-free spent media (CFSM) of six probiotics (L. acidophilus EMCC 1324, L. helveticus EMCC 1654, L. plantarum ss. plantarum EMCC 1027, L. rhamnosus EMCC 1105, B. longum EMCC 1547, and B. bifidum EMCC 1334) have been shown to exert strong antibacterial activity to Escherichia coli isolates, with inhibition zones of 11.77–23.10 mm. Notably, CFSM of L. plantarum had the strongest antibacterial activity compared to the other five, with a 64.57% reduction in biofilms of E. coli (88). In another in vitro study, CFSM and biofilm of probiotics L. rhamnosus and L. casei also performed an antifungal activity to Candida albicans (89). Similar findings were also observed in cell-free supernatant (CFS) from L. kunkeei against Candida albicans (90). CFS from L. paracasei CNCM I-4034, B. breve CNCM I-4035, and L. rhamnosus CNCM I-4036 isolated from a faecal sample of breast-feeding infants could suppress the growth of E. coli, Salmonella, and Shigella over 50%, and L. paracasei CNCM I-4034 CFS showed the strongest antimicrobial activity (81%) in vivo. However, when CFS was neutralised, decreased antimicrobial activities were observed, indicating the important role of organic acid and antimicrobial substances produced by probiotic fermentation (91). CFS from probiotic L. rhamnosus GG also exhibited a strong antibacterial activity against E. coli K1, the adhesion, invasion, and translocation of which have been shown to be blocked by CFS in vitro, through stimulating the production of mucin and protecting intestinal barrier function. This was demonstrated in the neonatal rat model, CFS could protect neonatal rats from E. coli K1 infection, through increasing expression levels of Ki67, MUC2, ZO-1, IgA, and mucin and decreasing intestinal barrier permeability (92). Besides, in a broiler model study, the impact of the mixture of CFS from L. plantarum RG14 and inulin on colon health and immune function was investigated. Increased numbers of total caecal microbiota and bifidobacteria, and decreased number of Enterobacteria and E. coli, were observed in birds fed with CFS and inulin compared to birds fed with placebo. In addition, CFS and inulin could stimulate the production of acetic acid in birds, besides, the immune markers were regulated by CFS and inulin, resulting in decreased levels of IFN and TNF-α and increased levels of IL-6. The results illustrated the potential role of post-biotic CFS from L. plantarum RG14 in growth promoters in the poultry industry (82). CFS from L. plantarum RG14 could also improve the nutrients digestion and absorption in newly-weaned lambs, suggested by increasing the number of fibre degrading bacteria, such as Fibrobacter succinogenes and Ruminococcus flavefaciens, and expressions of IGF-1 and MCT-1 (93). These findings demonstrated the antimicrobial and microbiota regulatory activity of post-biotics, however, the bioactive compounds were not identified and separated.
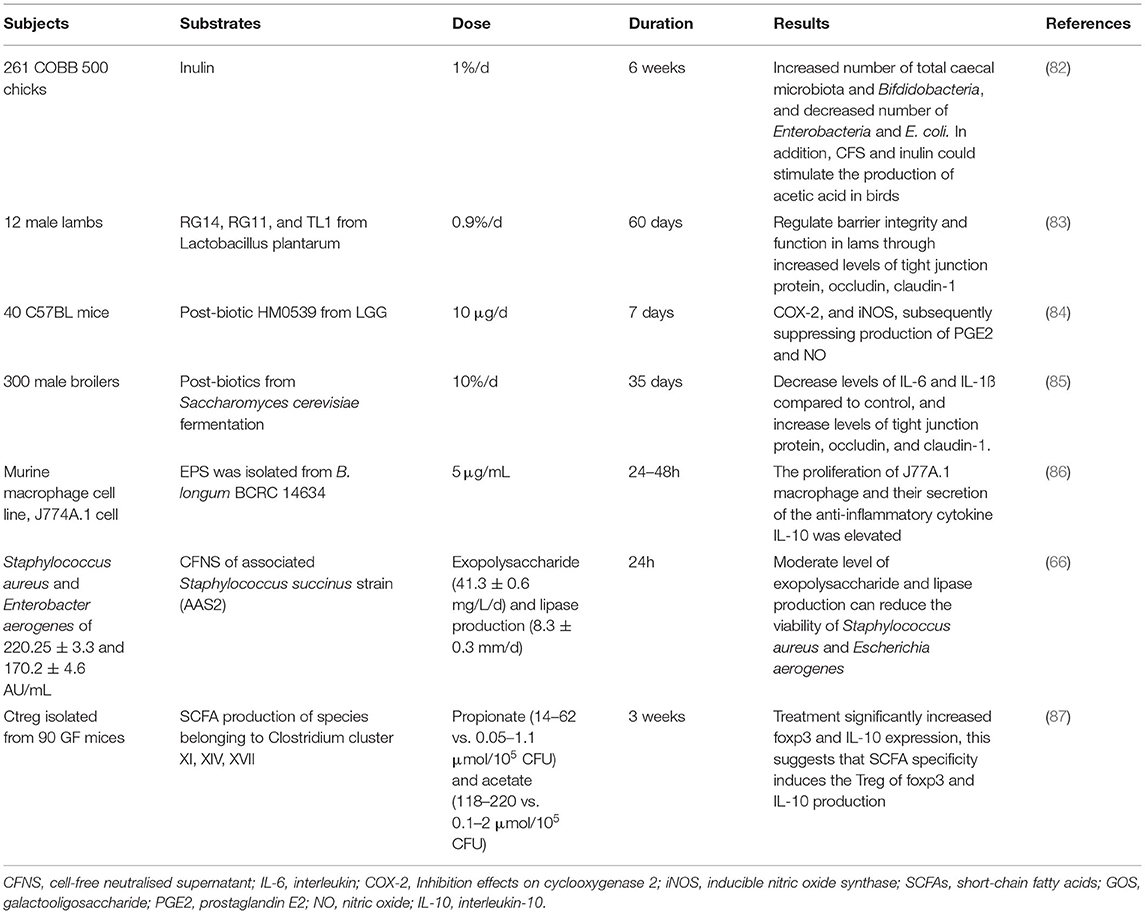
Table 3. Example of studies designed to determine effects of post-biotic on gut microbiota and immune function.
To further explore the underlying mechanism, a few research groups focused on the exopolysaccharides (EPS), and proteins, etc. To investigate the impact of EPS on gut microbiota, an in vitro study applied a batch culture model and compared eleven EPS isolated from different human bifidobacteria with glucose and inulin. The results illustrated that EPS and inulin could stimulate the growth of bifidobacteria and the production of SCFA, resulting in a decreased ratio of acetic acid/propionic acid, which was contrary to glucose fermentation. In addition, the strain-specific impact of EPS was indicated, as EPS from B. pseudocatenulatum contributed to the growth of Desulfovibrio and Faecalibacterium prausnitzii, whereas EPS from B. longum contributed to the growth of Anaerostipes, Prevotella, and/or Oscillospira (94). The results showed EPS from bacteria could be utilised by gastrointestinal microorganisms, hence affecting colon health. The post-biotic could also inhibit the adhesion of pathogens to epithelial cells. A novel soluble protein HM0539 from L. rhamnosus could inhibit the adhesion and invasion of E. coli O157: H7 to HT-29 cell dose-dependently (95). Kaikiri et al. (96) also found that a novel gut microbiota metabolite 10-hydroxy-cis-12-octadecenoic acid (HYA) could alter the faecal microbiota community of NC/Nga mice, although the PCR-denaturing gradient gel electrophoresis analysis did not show detailed bacteria species change induced by HYA. The impact of post-biotics on bacteria is mainly demonstrated in vitro and in vivo, hence further evidence from human clinical trials is required, and post-biotics could be used as alternative antimicrobial compounds and supplementation strategies for disease alleviation or treatment.
Effects of Post-biotics on Immune Function
Protective Effect of Post-biotics on Intestinal Epithelial Barrier
Post-biotics could modulate host immunity by improving gastrointestinal barrier function and inhibiting pathogen translocation. In one study, CFS from L. plantarum fermentation could regulate barrier integrity and function in lambs through increasing levels of tight junction protein, occludin, claudin-1, and CLDN-4 (83). The bioactive compound HM0539 has also been shown to stimulate mucin expression and decline expression levels of MUC2 and zonula occludens-1 (ZO-1) in the neonatal rat model, indicating its intestinal barrier protecting role (97). As shown in the above studies, it is mainly the increased tight junction protein induced by post-biotics that illustrated its positive role in the improvement of intestinal epithelial barrier function. Hence, further immunological, biochemical, and pathological section evaluation from clinical trials is warranted to support this view.
Effects of Post-biotics on Immune Response
Post-biotics may affect the innate and adaptive immune system through the interaction of many cell types along the mucosa, such as B cells, T cells, monocytes, macrophages, NK cells, and dendritic cells (DCs) (98). Cell wall components, including peptidoglycan (99), have been shown could bind to receptors on the surface of monocytes and macrophages, consequently stimulating immune cells to produce cytokines indirectly (100, 101). Tryptophan metabolites can inhibit inflammation by acting on T cell aromatics receptors and stimulating DCs to induce Treg activation through retinoic acid (102).
In addition, post-biotics could influence immune response by affecting the immune signalling pathway through modulating inflammatory cytokines. Butyrate, an important bacteria fermentation product, was shown to facilitate monocyte polarised to M2 macrophage and suppress the pro-inflammatory immune response in mice, with increased expression of Arg1 and activation of H3K9/STAT6 signalling pathway (101). Lipoteichoic acid produced by L. plantarum was shown to inhibit inflammation response induced by the viral pathogen in porcine intestinal epithelial cells. The results showed lipoteichoic acid could reduce levels of IL-8 and suppress ERK phosphorylation, p38 kinase, and NF-κB activation in a dose-dependent manner (103). The post-biotic HM0539 not only exhibited an inhibition effect against the pathogens but also an immunomodulatory effect. In in vitro cell culture and dextran sulphate sodium (DSS)-induced murine colitis model, HM0539 showed an inhibition effect on cyclooxygenase 2 (COX-2), and inducible nitric oxide synthase (iNOS), subsequently suppressing the production of prostaglandin E2 (PGE2) and nitric oxide (NO), TLR4-MyD88, and NF-κB signalling pathways, and hence might serve as a candidate strategy in inflammatory bowel disease treatment (84). Metabolic products from Pediococcus acidilactici, L. reuteri, E. faecium, and L. acidophilus fermentation were assessed in broiler chicks challenged with Clostridium perfringens infection. The immunomodulatory activity of these metabolic products was illustrated by the downregulating pro-inflammatory response, especially the Kyoto Encyclopaedia of Genes and Genomes (KEGG) signalling pathways (104). An animal experiment investigated the influence of post-biotics from Saccharomyces cerevisiae fermentation product in male broilers and found they could decrease levels of IL-6, NF-κB, and IL-1ß compared to control, and increase levels of tight junction protein, occludin, and claudin-1, indicating their anti-inflammatory activities and protective role in barrier function (85). An in vivo study also showed that EPS from B. animalis subsp. Lactis could modulate the immune response, inducing the downregulation of pro-inflammatory cytokines (105). In ex vivo cultures of mucosa from diarrhoea patients, CFS of L. casei DG could downregulate pro-inflammatory immune response, upregulate anti-inflammatory immune response, via reducing expression levels of IL-1α, IL-6, IL-8, and TLR-4, and increasing those of IL-10 (106).
The impacts of the cell wall on immune response were also compared by a few studies. The cell wall components of probiotic Ganeden Bacillus coagulans 30 (GBC30) were shown to stimulate the maturation of monocyte in human peripheral blood mononuclear cell (PBMC) culture. The shift of monocyte toward macrophage and DCs was observed with GBC30 cell wall components, with higher CD80 and CD86 expressions (107). Furthermore, an in vivo study indicated the immunomodulatory activity of GBC30 cell wall components through suppressing the production of ROS, increasing phagocytosis and migration of PBMC, enhancing the proportion of NK cells, stimulating the production of anti-inflammatory cytokine production, such as IL-4, and IL-10, and decreasing pro-inflammatory cytokine production, such as TNF-α and IFN-γ, consequently balancing Th1/Th2 immune response (86). The immunomodulatory activity of cell wall components and CFS from seventeen lactic acid bacteria strains were assessed in another in vivo study. The results showed that IL-10 could be stimulated by CFS of all strains in peripheral blood mononuclear cells (PMBC) culture. In addition, cell wall components and CFS could both modulate the anti-inflammatory immune response, whereas, cell wall components modulate the pro-inflammatory immune response and stimulate the activity of Tregs more strongly (108).
Autophagy plays a crucial role in the innate and adaptive immune response, regulating cell homeostasis, and modulating the renewal of cellular proteins and organelles. It has recently been found an imbalance of T cells could be caused by the deficiency in autophagy, resulting in intestinal inflammation (109). The whole peptidoglycan of B. bifidum showed antitumour impact in BALB/c male nude mice via increasing gene expression of bax and decreasing that of bcl-2, subsequently inducing cell apoptosis (110). Peptidoglycan expressed on L. fermentum BGHV110 (HV110) was isolated, and its impact on autophagy was investigated in acetaminophen (APAP)-induced hepatotoxicity in HepG2 cells. The results illustrated that activation of PINK1-dependent autophagy could be stimulated by HV110 supplementation, which increased LC3 protein conversion and p62/SQSTM1 protein degradation (111).
These findings suggested that the immunomodulatory activity of post-biotic mainly depended on their ability to differentially regulate the production of anti-inflammatory and pro-inflammatory cytokines and the balance of Th1 and Th2. They can effectively regulate the gene expression of immune cells and the interference of transcription factors, thus driving the differentiation of the immune system. In addition, post-biotics may exert a significant effect on autophagy, hence influencing host immune response.
Conclusion
This review summarised the positive impacts of probiotics, pre-biotics, and post-biotics on gastrointestinal health and immune function. Several in vitro, in vivo, and clinical studies have confirmed that they play a significant role in maintaining intestinal microorganism equilibrium and regulating immune response, consequently conferring benefits to host health. Although the underlying mechanism remains to be further investigated, supplementations of probiotics, pre-biotics, and post-biotics could lower colonic pH, produce antibacterial molecules, stimulate the growth and activities of beneficial bacteria, and suppress the growth of pathogens. In addition, they also play an important role in the modulation of host immunity, via improving gut barrier function, preventing pathogen translocation, altering pattern recognition receptor expression, modulating crucial signalling pathways, stimulating immune cell activities, and balancing Th1/Th2 immune response. Notably, the efficacies of probiotics, pre-, and post-biotics are typically dose-dependent, especially exhibiting species-specific for probiotic strains and structure-activity relationships for pre- and post-biotics. In addition, the profile of host microorganisms and immunity may differ based on the age, gender, exercise, health conditions, geography, etc, of the host, hence, it is necessary to consider these factors and assess increasingly quantitative and qualitative impacts of pre-biotics, probiotics, and post-biotics on gut microbiota and immune system. Besides, the underlying mechanisms should be fully illustrated via combining in vitro, in vivo, and clinical studies and biochemical evaluations.
Author Contributions
All authors listed have made a substantial, direct, and intellectual contribution to the work and approved it for publication.
Funding
This work was supported by the Science and Technology Innovation Project of Colleges and Universities of Shanxi Province (2019L0074).
Conflict of Interest
The authors declare that the research was conducted in the absence of any commercial or financial relationships that could be construed as a potential conflict of interest.
Publisher's Note
All claims expressed in this article are solely those of the authors and do not necessarily represent those of their affiliated organizations, or those of the publisher, the editors and the reviewers. Any product that may be evaluated in this article, or claim that may be made by its manufacturer, is not guaranteed or endorsed by the publisher.
References
1. Adak A, Khan MR. An insight into gut microbiota and its functionalities. Cell Mol Life Sci. (2019) 76:473–93. doi: 10.1007/s00018-018-2943-4
2. Fujimura KE, Sitarik AR, Havstad S, Lin DL, Levan S, Fadrosh D, et al. Neonatal gut microbiota associates with childhood multisensitized atopy and T cell differentiation. Nat Med. (2016) 22:1187–91. doi: 10.1038/nm.4176
3. Takiishi T, Fenero CIM, Câmara NOS. Intestinal barrier and gut microbiota: shaping our immune responses throughout life. Tissue Barriers. (2017) 5:e1373208. doi: 10.1080/21688370.2017.1373208
4. Tsai YL, Lin TL, Chang CJ, Wu TR, Lai WF, Lu CC, et al. Probiotics, prebiotics and amelioration of diseases. J Biomed Sci. (2019) 26:3. doi: 10.1186/s12929-018-0493-6
5. Gibson GR, Hutkins R, Sanders ME, Prescott SL, Reimer RA, Salminen SJ, et al. Expert consensus document: the international scientific association for probiotics and prebiotics (ISAPP) consensus statement on the definition and scope of prebiotics. Nat Rev Gastroenterol Hepatol. (2017) 14:491–502. doi: 10.1038/nrgastro.2017.75
6. Hill C, Guarner F, Reid G, Gibson GR, Merenstein DJ, Pot B, et al. Expert consensus document. The international scientific association for probiotics and prebiotics consensus statement on the scope and appropriate use of the term probiotic. Nat Rev Gastroenterol Hepatol. (2014) 11:506–14. doi: 10.1038/nrgastro.2014.66
7. Sanders ME. Probiotics in 2015: their scope and use. J Clin Gastroenterol. (2015) 49 (Suppl. 1):S2–6. doi: 10.1097/MCG.0000000000000350
8. Metzger RN, Krug AB, Eisenächer K. Enteric virome sensing-its role in intestinal homeostasis and immunity. Viruses. (2018) 10:146. doi: 10.3390/v10040146
9. Xiao L, Gong C, Ding Y, Ding G, Xu X, Deng C, et al. Probiotics maintain intestinal secretory immunoglobulin A levels in healthy formula-fed infants: a randomised, double-blind, placebo-controlled study. Benef Microbes. (2019) 10:729–39. doi: 10.3920/BM2019.0025
10. Vernocchi P, Del Chierico F, Fiocchi AG, El Hachem M, Dallapiccola B, Rossi P, et al. Understanding probiotics' role in allergic children: the clue of gut microbiota profiling. Curr Opin Allergy Clin Immunol. (2015) 15:495–503. doi: 10.1097/ACI.0000000000000203
11. Li KL, Wang BZ, Li ZP, Li YL, Liang JJ. Alterations of intestinal flora and the effects of probiotics in children with recurrent respiratory tract infection. World J Pediatr. (2019) 15:255–61. doi: 10.1007/s12519-019-00248-0
12. Song H, Wang W, Shen B, Jia H, Hou Z, Chen P, et al. Pretreatment with probiotic bifico ameliorates colitis-associated cancer in mice: transcriptome and gut flora profiling. Cancer Sci. (2018) 109:666–77. doi: 10.1111/cas.13497
13. Everard A, Matamoros S, Geurts L, Delzenne NM, Cani PD. Saccharomyces boulardii administration changes gut microbiota and reduces hepatic steatosis, low-grade inflammation, and fat mass in obese and type 2 diabetic db/db mice. mBio. (2014) 5:e01011–14. doi: 10.1128/mBio.01011-14
14. Ahmed M, Prasad J, Gill H, Stevenson L, Gopal P. Impact of consumption of different levels of Bifidobacterium lactis HN019 on the intestinal microflora of elderly human subjects. J Nutr Health Aging. (2007) 11:26–31. doi: 10.1016/j.jaging.2006.02.002
15. Toscano M, De Grandi R, Stronati L, De Vecchi E, Drago L. Effect of Lactobacillus rhamnosus HN001 and Bifidobacterium longum BB536 on the healthy gut microbiota composition at phyla and species level: a preliminary study. World J Gastroenterol. (2017) 23:2696–704. doi: 10.3748/wjg.v23.i15.2696
16. Rocha-Ramírez LM, Pérez-Solano RA. probiotic lactobacillus strains stimulate the inflammatory response and activate human macrophages. J Immunol Res. (2017) 2017:4607491. doi: 10.1155/2017/4607491
17. Sakai F, Hosoya T, Ono-Ohmachi A, Ukibe K, Ogawa A, Moriya T, et al. Lactobacillus gasseri SBT2055 induces TGF-β expression in dendritic cells and activates TLR2 signal to produce IgA in the small intestine. PLoS ONE. (2014) 9:e105370. doi: 10.1371/journal.pone.0105370
18. Kwon HK, Lee CG, So JS, Chae CS, Hwang JS, Sahoo A, et al. Generation of regulatory dendritic cells and CD4+Foxp3+ T cells by probiotics administration suppresses immune disorders. Proc Natl Acad Sci USA. (2010) 107:2159–64. doi: 10.1073/pnas.0904055107
19. Childs CE, Röytiö H, Alhoniemi E, Fekete AA, Forssten SD, Hudjec N, et al. Xylo-oligosaccharides alone or in synbiotic combination with Bifidobacterium animalis subsp. lactis induce bifidogenesis and modulate markers of immune function in healthy adults: a double-blind, placebo-controlled, randomised, factorial cross-over study. Br J Nutr. (2014) 111:1945–56. doi: 10.1017/S0007114513004261
20. Damaceno QS, Souza JP, Nicoli JR, Paula RL, Assis GB, Figueiredo HC, et al. Evaluation of potential probiotics isolated from human milk and colostrum. Probiotics Antimicrob Proteins. (2017) 9:371–9. doi: 10.1007/s12602-017-9270-1
21. Zaylaa M, Alard J, Kassaa IA, Peucelle V, Boutillier D, Desramaut J, et al. Autophagy: a novel mechanism involved in the anti-inflammatory abilities of probiotics. Cell Physiol Biochem. (2019) 53:774–93. doi: 10.33594/000000172
22. Kirpich IA, Solovieva NV, Leikhter SN, Shidakova NA, Lebedeva OV, Sidorov PI, et al. Probiotics restore bowel flora and improve liver enzymes in human alcohol-induced liver injury: a pilot study. Alcohol. (2008) 42:675–82. doi: 10.1016/j.alcohol.2008.08.006
23. Yoon JY, Cha JM, Oh JK, Tan PL, Kim SH, Kwak MS, et al. Probiotics ameliorate stool consistency in patients with chronic constipation: a randomized, double-blind, placebo-controlled study. Dig Dis Sci. (2018) 63:2754–64. doi: 10.1007/s10620-018-5139-8
24. Lichtenstein L, Avni-Biron I, Ben-Bassat O. Probiotics and prebiotics in crohn's disease therapies. Best Pract Res Clin Gastroenterol. (2016) 30:81–8. doi: 10.1016/j.bpg.2016.02.002
25. Derikx LA, Dieleman LA, Hoentjen F. Probiotics and prebiotics in ulcerative colitis. Best Pract Res Clin Gastroenterol. (2016) 30:55–71. doi: 10.1016/j.bpg.2016.02.005
26. Patusco R, Ziegler J. Role of probiotics in managing gastrointestinal dysfunction in children with autism spectrum disorder: an update for practitioners. Adv Nutr. (2018) 9:637–50. doi: 10.1093/advances/nmy031
27. Prabhurajeshwar C, Chandrakanth RK. Probiotic potential of Lactobacilli with antagonistic activity against pathogenic strains: an in vitro validation for the production of inhibitory substances. Biomed J. (2017) 40:270–83. doi: 10.1016/j.bj.2017.06.008
28. Sherman PM, Ossa JC, Johnson-Henry K. Unraveling mechanisms of action of probiotics. Nutr Clin Pract. (2009) 24:10–4. doi: 10.1177/0884533608329231
29. Palm NW, de Zoete MR, Flavell RA. Immune-microbiota interactions in health and disease. Clin Immunol. (2015) 159:122–7. doi: 10.1016/j.clim.2015.05.014
30. Jaffar N, Okinaga T, Nishihara T, Maeda T. Enhanced phagocytosis of aggregatibacter actinomycetemcomitans cells by macrophages activated by a probiotic lactobacillus strain. J Dairy Sci. (2018) 101:5789–98. doi: 10.3168/jds.2017-14355
31. Lyons A, O'Mahony D, O'Brien F, MacSharry J, Sheil B, Ceddia M, et al. Bacterial strain-specific induction of Foxp3+ T regulatory cells is protective in murine allergy models. Clin Exp Allergy. (2010) 40:811–9. doi: 10.1111/j.1365-2222.2009.03437.x
32. Konieczna P, Groeger D, Ziegler M, Frei R, Ferstl R, Shanahan F, et al. Bifidobacterium infantis 35624 administration induces Foxp3 T regulatory cells in human peripheral blood: potential role for myeloid and plasmacytoid dendritic cells. Gut. (2012) 61:354–66. doi: 10.1136/gutjnl-2011-300936
33. Groeger D, O'Mahony L, Murphy EF, Bourke JF, Dinan TG, Kiely B, et al. Bifidobacterium infantis 35624 modulates host inflammatory processes beyond the gut. Gut Microbes. (2013) 4:325–39. doi: 10.4161/gmic.25487
34. Gibson GR, Roberfroid MB. Dietary modulation of the human colonic microbiota: introducing the concept of prebiotics. J Nutr. (1995) 125:1401–12. doi: 10.1093/jn/125.6.1401
35. Sheveleva SA. [Probiotics, prebiotics and probiotic products. Current status]. Vopr Pitan. (1999) 68:32–40.
36. Scott KP, Grimaldi R, Cunningham M, Sarbini SR, Wijeyesekera A, Tang MLK, et al. Developments in understanding and applying prebiotics in research and practice-an ISAPP conference paper. J Appl Microbiol. (2020) 128:934–49. doi: 10.1111/jam.14424
37. Macfarlane S, Macfarlane GT, Cummings JH. Review article: prebiotics in the gastrointestinal tract. Aliment Pharmacol Ther. (2006) 24:701–14. doi: 10.1111/j.1365-2036.2006.03042.x
38. Markowiak P, Slizewska K. Effects of probiotics, prebiotics, and synbiotics on human health. Nutrients. (2017) 9:1021. doi: 10.3390/nu9091021
39. Rose L, Rose J, Gosling S, Holmes M. Efficacy of a probiotic-prebiotic supplement on incidence of diarrhea in a dog shelter: a randomized, double-blind, placebo-controlled trial. J Vet Intern Med. (2017) 31:377–82. doi: 10.1111/jvim.14666
40. Chu JR, Kang SY, Kim SE, Lee SJ, Lee YC, Sung MK. Prebiotic UG1601 mitigates constipation-related events in association with gut microbiota: a randomized placebo-controlled intervention study. World J Gastroenterol. (2019) 25:6129–44. doi: 10.3748/wjg.v25.i40.6129
41. Moreno-Indias I, Sánchez-Alcoholado L, Pérez-Martínez P, Andrés-Lacueva C, Cardona F, Tinahones F, et al. Red wine polyphenols modulate fecal microbiota and reduce markers of the metabolic syndrome in obese patients. Food Funct. (2016) 7:1775–87. doi: 10.1039/C5FO00886G
42. Xie X, He Y, Li H, Yu D, Na L, Sun T, et al. Effects of prebiotics on immunologic indicators and intestinal microbiota structure in perioperative colorectal cancer patients. Nutrition. (2019) 61:132–42. doi: 10.1016/j.nut.2018.10.038
43. Angelino D, Martina A, Rosi A, Veronesi L, Antonini M, Mennella I, et al. Glucose- and lipid-related biomarkers are affected in healthy obese or hyperglycemic adults consuming a whole-grain pasta enriched in prebiotics and probiotics: a 12-week randomized controlled trial. J Nutr. (2019) 149:1714–23. doi: 10.1093/jn/nxz071
44. Abrams SA, Griffin IJ, Hawthorne KM, Liang L, Gunn SK, Darlington G, et al. A combination of prebiotic short- and long-chain inulin-type fructans enhances calcium absorption and bone mineralization in young adolescents. Am J Clin Nutr. (2005) 82:471–6. doi: 10.1093/ajcn/82.2.471
45. Goehring KC, Marriage BJ, Oliver JS, Wilder JA, Barrett EG, Buck RH. Similar to those who are breastfed, infants fed a formula containing 2'-fucosyllactose have lower inflammatory cytokines in a randomized controlled trial. J Nutr. (2016) 146:2559–66. doi: 10.3945/jn.116.236919
46. Meyer D, Stasse-Wolthuis M. The bifidogenic effect of inulin and oligofructose and its consequences for gut health. Eur J Clin Nutr. (2009) 63:1277–89. doi: 10.1038/ejcn.2009.64
47. Duncan SH, Louis P, Thomson JM, Flint HJ. The role of pH in determining the species composition of the human colonic microbiota. Environ Microbiol. (2009) 11:2112–22. doi: 10.1111/j.1462-2920.2009.01931.x
48. Segata N. Gut microbiome: westernization and the disappearance of intestinal diversity. Curr Biol. (2015) 25:R611–3. doi: 10.1016/j.cub.2015.05.040
49. Holscher HD. Dietary fiber and prebiotics and the gastrointestinal microbiota. Gut Microbes. (2017) 8:172–84. doi: 10.1080/19490976.2017.1290756
50. Dewulf EM, Cani PD, Claus SP, Fuentes S, Puylaert PG, Neyrinck AM, et al. Insight into the prebiotic concept: lessons from an exploratory, double blind intervention study with inulin-type fructans in obese women. Gut. (2013) 62:1112–21. doi: 10.1136/gutjnl-2012-303304
51. Zou Y, Wang J, Wang Y, Peng B, Liu J, Zhang B, et al. Protection of Galacto-Oligosaccharide against E. coli O157 colonization through enhancing gut barrier function and modulating gut microbiota. Foods. (2020) 9:1710. doi: 10.3390/foods9111710
52. Sang H, Xie Y, Su X, Zhang M, Zhang Y, Liu K, et al. Mushroom bulgaria inquinans modulates host immunological response and gut microbiota in mice. Front Nutr. (2020) 7:144. doi: 10.3389/fnut.2020.00144
53. Elison E, Vigsnaes LK, Rindom Krogsgaard L, Rasmussen J, Sørensen N, McConnell B, et al. Oral supplementation of healthy adults with 2'-O-fucosyllactose and lacto-N-neotetraose is well tolerated and shifts the intestinal microbiota. Br J Nutr. (2016) 116:1356–68. doi: 10.1017/S0007114516003354
54. Russo F, Linsalata M, Clemente C, Chiloiro M, Orlando A, Marconi E, et al. Inulin-enriched pasta improves intestinal permeability and modifies the circulating levels of zonulin and glucagon-like peptide 2 in healthy young volunteers. Nutr Res. (2012) 32:940–6. doi: 10.1016/j.nutres.2012.09.010
55. Krumbeck JA, Rasmussen HE, Hutkins RW, Clarke J, Shawron K, Keshavarzian A, et al. Probiotic bifidobacterium strains and galactooligosaccharides improve intestinal barrier function in obese adults but show no synergism when used together as synbiotics. Microbiome. (2018) 6:121. doi: 10.1186/s40168-018-0494-4
56. Salden BN, Troost FJ, Wilms E, Truchado P, Vilchez-Vargas R, Pieper DH, et al. Reinforcement of intestinal epithelial barrier by arabinoxylans in overweight and obese subjects: a randomized controlled trial: arabinoxylans in gut barrier. Clin Nutr. (2018) 37:471–80. doi: 10.1016/j.clnu.2017.01.024
57. Myhill LJ, Stolzenbach S, Hansen TVA, Skovgaard K, Stensvold CR, Andersen LO, et al. Mucosal barrier and Th2 immune responses are enhanced by dietary inulin in pigs infected with trichuris suis. Front Immunol. (2018) 9:2557. doi: 10.3389/fimmu.2018.02557
58. Hoentjen F, Welling GW, Harmsen HJ, Zhang X, Snart J, Tannock GW, et al. Reduction of colitis by prebiotics in HLA-B27 transgenic rats is associated with microflora changes and immunomodulation. Inflamm Bowel Dis. (2005) 11:977–85. doi: 10.1097/01.MIB.0000183421.02316.d5
59. Dehghan P, Pourghassem Gargari B, Asghari Jafar-abadi M. Oligofructose-enriched inulin improves some inflammatory markers and metabolic endotoxemia in women with type 2 diabetes mellitus: a randomized controlled clinical trial. Nutrition. (2014) 30:418–23. doi: 10.1016/j.nut.2013.09.005
60. Chambers ES, Byrne CS, Morrison DJ, Murphy KG, Preston T, Tedford C, et al. Dietary supplementation with inulin-propionate ester or inulin improves insulin sensitivity in adults with overweight and obesity with distinct effects on the gut microbiota, plasma metabolome and systemic inflammatory responses: a randomised cross-over trial. Gut. (2019) 68:1430–8. doi: 10.1136/gutjnl-2019-318424
61. Vogt LM, Elderman ME, Borghuis T, de Haan BJ, Faas MM, de Vos P. Chain length-dependent effects of inulin-type fructan dietary fiber on human systemic immune responses against hepatitis-B. Mol Nutr Food Res. (2017) 61:171. doi: 10.1002/mnfr.201700171
62. Vulevic J, Juric A, Walton GE, Claus SP, Tzortzis G, Toward RE, et al. Influence of galacto-oligosaccharide mixture (B-GOS) on gut microbiota, immune parameters and metabonomics in elderly persons. Br J Nutr. (2015) 114:586–95. doi: 10.1017/S0007114515001889
63. Lockyer S, Rowland I, Spencer JPE, Yaqoob P, Stonehouse W. Impact of phenolic-rich olive leaf extract on blood pressure, plasma lipids and inflammatory markers: a randomised controlled trial. Eur J Nutr. (2017) 56:1421–32. doi: 10.1007/s00394-016-1188-y
64. Salazar N, Dewulf EM, Neyrinck AM, Bindels LB, Cani PD, Mahillon J, et al. Inulin-type fructans modulate intestinal bifidobacterium species populations and decrease fecal short-chain fatty acids in obese women. Clin Nutr. (2015) 34:501–7. doi: 10.1016/j.clnu.2014.06.001
65. Lomax AR, Cheung LV, Noakes PS, Miles EA, Calder PC. Inulin-Type β2-1 fructans have some effect on the antibody response to seasonal influenza vaccination in healthy middle-aged humans. Front Immunol. (2015) 6:490. doi: 10.3389/fimmu.2015.00490
66. Khusro A, Aarti C, Barbabosa-Pilego A, Rojas Hernández S. Anti-pathogenic, antibiofilm, and technological properties of fermented food associated Staphylococcus succinus strain AAS2. Prep Biochem Biotechnol. (2019) 49:176–83. doi: 10.1080/10826068.2019.1566149
67. Liu Y, Gibson GR, Walton GE. An in vitro approach to study effects of prebiotics and probiotics on the faecal microbiota and selected immune parameters relevant to the elderly. PLoS ONE. (2016) 11:e0162604. doi: 10.1371/journal.pone.0162604
68. Liu Y, Gibson GR, Walton GE. A three-stage continuous culture approach to study the impact of probiotics, prebiotics and fat intake on faecal microbiota relevant to an over 60s population. J Funct Foods. (2017) 32 (Supple. C):238–47. doi: 10.1016/j.jff.2017.02.035
69. Liu C, Kolida S, Charalampopoulos D, Rastall RA. An evaluation of the prebiotic potential of microbial levans from Erwinia sp. 10119. J Funct Foods. (2019) 64:103668. doi: 10.1016/j.jff.2019.103668
70. Reimer RA, Soto-Vaca A, Nicolucci AC, Mayengbam S, Park H, Madsen KL, et al. Effect of chicory inulin-type fructan-containing snack bars on the human gut microbiota in low dietary fiber consumers in a randomized crossover trial. Am J Clin Nutr. (2020) 111:1286–96. doi: 10.1093/ajcn/nqaa074
71. Du H, Zhao A, Wang Q, Yang X, Ren D. Supplementation of inulin with various degree of polymerization ameliorates liver injury and gut microbiota dysbiosis in high fat-fed obese mice. J Agric Food Chem. (2020) 68:779–87. doi: 10.1021/acs.jafc.9b06571
72. Kim MG, Jo K, Chang YB, Suh HJ, Hong KB. Changes in the gut microbiome after galacto-oligosaccharide administration in loperamide-induced constipation. J Pers Med. (2020) 10:161. doi: 10.3390/jpm10040161
73. Vulevic J, Tzortzis G, Juric A, Gibson GR. Effect of a prebiotic galactooligosaccharide mixture (B-GOS®) on gastrointestinal symptoms in adults selected from a general population who suffer with bloating, abdominal pain, or flatulence. Neurogastroenterol Motil. (2018) 30:e13440. doi: 10.1111/nmo.13440
74. Grimaldi R, Cela D, Swann JR, Vulevic J, Gibson GR, Tzortzis G, et al. In vitro fermentation of B-GOS: impact on faecal bacterial populations and metabolic activity in autistic and non-autistic children. FEMS Microbiol Ecol. (2017) 93:fiw233. doi: 10.1093/femsec/fiw233
75. Davis LM, Martínez I, Walter J, Goin C, Hutkins RW. Barcoded pyrosequencing reveals that consumption of galactooligosaccharides results in a highly specific bifidogenic response in humans. PLoS ONE. (2011) 6:e25200. doi: 10.1371/journal.pone.0025200
76. Akram W, Garud N, Joshi R. Role of inulin as prebiotics on inflammatory bowel disease. Drug Discov Ther. (2019) 13:1–8. doi: 10.5582/ddt.2019.01000
77. Westerbeek EA, van den Berg A, Lafeber HN, Fetter WP, van Elburg RM. The effect of enteral supplementation of a prebiotic mixture of non-human milk galacto-, fructo- and acidic oligosaccharides on intestinal permeability in preterm infants. Br J Nutr. (2011) 105:268–74. doi: 10.1017/S0007114510003405
78. Vulevic J, Juric A, Tzortzis G, Gibson GR. A mixture of trans-galactooligosaccharides reduces markers of metabolic syndrome and modulates the fecal microbiota and immune function of overweight adults. J Nutr. (2013) 143:324–31. doi: 10.3945/jn.112.166132
79. Vulevic J, Drakoularakou A, Yaqoob P, Tzortzis G, Gibson GR. Modulation of the fecal microflora profile and immune function by a novel trans-galactooligosaccharide mixture (B-GOS) in healthy elderly volunteers. Am J Clin Nutr. (2008) 88:1438–46. doi: 10.3945/ajcn.2008.26242
80. Collado MC, Vinderola G, Salminen S. Postbiotics: facts and open questions. A position paper on the need for a consensus definition. Benef Microbes. (2019) 10:711–9. doi: 10.3920/BM2019.0015
81. Teame T, Wang A, Xie M, Zhang Z, Yang Y, Ding Q, et al. Paraprobiotics and postbiotics of probiotic lactobacilli, their positive effects on the host and action mechanisms: a review. Front Nutr. (2020) 7:570344. doi: 10.3389/fnut.2020.570344
82. Kareem KY, Loh TC, Foo HL, Asmara SA, Akit H. Influence of postbiotic RG14 and inulin combination on cecal microbiota, organic acid concentration, and cytokine expression in broiler chickens. Poult Sci. (2017) 96:966–75. doi: 10.3382/ps/pew362
83. Izuddin WI, Humam AM, Loh TC. Dietary postbiotic lactobacillus plantarum improves serum and ruminal antioxidant activity and upregulates hepatic antioxidant enzymes and ruminal barrier function in post-weaning lambs. Antioxidants. (2020) 9:250. doi: 10.3390/antiox9030250
84. Li Y, Yang S, Lun J, Gao J, Gao X, Gong Z, et al. Inhibitory effects of the Lactobacillus rhamnosus GG effector protein HM0539 on inflammatory response through the TLR4/MyD88/NF-κB axis. Front Immunol. (2020) 11:551449. doi: 10.3389/fimmu.2020.551449
85. Chuang WY, Hsieh YC, Lin LJ, Chang SC, Lee TT. Saccharomyces cerevisiae and phytase co-fermentation wheat bran on growth, antioxidation, immunity and intestinal morphology in broilers. Asian Austra J Anim Sci. (2020) 34:1157–68. doi: 10.5713/ajas.20.0399
86. Jensen GS, Benson KF, Carter SG, Endres JR. GanedenBC30 cell wall and metabolites: anti-inflammatory and immune modulating effects in vitro. BMC Immunol. (2010) 11:15. doi: 10.1186/1471-2172-11-15
87. Smith PM, Howitt MR, Panikov N, Michaud M, Gallini CA, Bohlooly YM, et al. The microbial metabolites, short-chain fatty acids, regulate colonic Treg cell homeostasis. Science. (2013) 341:569–73. doi: 10.1126/science.1241165
88. Abdelhamid AG, Esaam A, Hazaa MM. Cell free preparations of probiotics exerted antibacterial and antibiofilm activities against multidrug resistant E. coli. Saudi Pharma J. (2018) 26:603–7. doi: 10.1016/j.jsps.2018.03.004
89. Song YG, Lee SH. Inhibitory effects of Lactobacillus rhamnosus and Lactobacillus casei on candida biofilm of denture surface. Arch Oral Biol. (2017) 76:1–6. doi: 10.1016/j.archoralbio.2016.12.014
90. Ebrahimi M, Sadeghi A. Postbiotic and anti-aflatoxigenic capabilities of lactobacillus kunkeei as the potential probiotic LAB isolated from the natural honey. Probiotics Antimicrob Proteins. (2021) 13:343–55. doi: 10.1007/s12602-020-09697-w
91. Muñoz-Quezada S, Bermudez-Brito M, Chenoll E, Genovés S, Gomez-Llorente C, Plaza-Diaz J, et al. Competitive inhibition of three novel bacteria isolated from faeces of breast milk-fed infants against selected enteropathogens. Br J Nutr. (2013) 109 (Suppl. 2):S63–9. doi: 10.1017/S0007114512005600
92. He X, Zeng Q, Puthiyakunnon S, Zeng Z, Yang W, Qiu J, et al. Lactobacillus rhamnosus GG supernatant enhance neonatal resistance to systemic Escherichia coli K1 infection by accelerating development of intestinal defense. Sci Rep. (2017) 7:43305. doi: 10.1038/srep43305
93. Izuddin WI, Loh TC. Effects of postbiotic supplementation on growth performance, ruminal fermentation and microbial profile, blood metabolite and GHR, IGF-1 and MCT-1 gene expression in post-weaning lambs. BMC Vet Res. (2019) 15:315. doi: 10.1186/s12917-019-2064-9
94. Salazar N, Gueimonde M, Hernandez-Barranco AM, Ruas-Madiedo P, de los Reyes-Gavilan CG. Exopolysaccharides produced by intestinal bifidobacterium strains act as fermentable substrates for human intestinal bacteria. Appl Environ Microbiol. (2008) 74:4737–45. doi: 10.1128/AEM.00325-08
95. Zhang H, Gao J, He X, Gong Z, Wan Y, Hu T, et al. [The postbiotic HM0539 from Lactobacillus rhamnosus GG prevents intestinal infection by enterohemorrhagic E. coli O157: H7 in mice]. Nan Fang Yi Ke Da Xue Xue Bao. (2020) 40:211–8. doi: 10.12122/j.issn.1673-4254.2020.02.12
96. Kaikiri H, Miyamoto J, Kawakami T, Park SB, Kitamura N, Kishino S, et al. Supplemental feeding of a gut microbial metabolite of linoleic acid, 10-hydroxy-cis-12-octadecenoic acid, alleviates spontaneous atopic dermatitis and modulates intestinal microbiota in NC/nga mice. Int J Food Sci Nutr. (2017) 68:941–51. doi: 10.1080/09637486.2017.1318116
97. Gao J, Li Y, Wan Y, Hu T, Liu L, Yang S, et al. A novel postbiotic from Lactobacillus rhamnosus GG with a beneficial effect on intestinal barrier function. Front Microbiol. (2019) 10:477. doi: 10.3389/fmicb.2019.00477
98. Dunand E, Burns P. Postbiotics produced at laboratory and industrial level as potential functional food ingredients with the capacity to protect mice against Salmonella infection. J Appl Microbiol. (2019) 127:219–29. doi: 10.1111/jam.14276
99. Frede S, Stockmann C, Freitag P, Fandrey J. Bacterial lipopolysaccharide induces HIF-1 activation in human monocytes via p44/42 MAPK and NF-kappaB. Biochem J. (2006) 396:517–27. doi: 10.1042/BJ20051839
100. Singh S, Bhatia R, Singh A, Singh P, Kaur R, Khare P, et al. Probiotic attributes and prevention of LPS-induced pro-inflammatory stress in RAW264.7 macrophages and human intestinal epithelial cell line (Caco-2) by newly isolated Weissella cibaria strains. Food Funct. (2018) 9:1254–64. doi: 10.1039/C7FO00469A
101. Ji J, Shu D, Zheng M, Wang J, Luo C, Wang Y, et al. Microbial metabolite butyrate facilitates M2 macrophage polarization and function. Sci Rep. (2016) 6:24838. doi: 10.1038/srep24838
102. Haase S, Haghikia A, Wilck N, Müller DN, Linker RA. Impacts of microbiome metabolites on immune regulation and autoimmunity. Immunology. (2018) 154:230–8. doi: 10.1111/imm.12933
103. Kim KW, Kang SS, Woo SJ, Park OJ, Ahn KB, Song KD, et al. Lipoteichoic acid of probiotic Lactobacillus plantarum attenuates poly I:C-induced IL-8 production in porcine intestinal epithelial cells. Front Microbiol. (2017) 8:1827. doi: 10.3389/fmicb.2017.01827
104. Johnson CN, Kogut MH, Genovese K, He H, Kazemi S, Arsenault RJ. Administration of a postbiotic causes immunomodulatory responses in broiler gut and reduces disease pathogenesis following challenge. Microorganisms. (2019) 7:268. doi: 10.3390/microorganisms7080268
105. Hidalgo-Cantabrana C, Nikolic M, López P, Suárez A, Miljkovic M, Kojic M, et al. Exopolysaccharide-producing Bifidobacterium animalis subsp. lactis strains and their polymers elicit different responses on immune cells from blood and gut associated lymphoid tissue. Anaerobe. (2014) 26:24–30. doi: 10.1016/j.anaerobe.2014.01.003
106. Compare D, Rocco A, Coccoli P, Angrisani D, Sgamato C, Iovine B, et al. Lactobacillus casei DG and its postbiotic reduce the inflammatory mucosal response: an ex-vivo organ culture model of post-infectious irritable bowel syndrome. BMC Gastroenterol. (2017) 17:53. doi: 10.1186/s12876-017-0605-x
107. Benson KF, Redman KA, Carter SG, Keller D, Farmer S, Endres JR, et al. Probiotic metabolites from Bacillus coagulans GanedenBC30 support maturation of antigen-presenting cells in vitro. World J Gastroenterol. (2012) 18:1875–83. doi: 10.3748/wjg.v18.i16.1875
108. Ashraf R, Vasiljevic T, Smith SC, Donkor ON. Effect of cell-surface components and metabolites of lactic acid bacteria and probiotic organisms on cytokine production and induction of CD25 expression in human peripheral mononuclear cells. J Dairy Sci. (2014) 97:2542–58. doi: 10.3168/jds.2013-7459
109. Matsuzawa-Ishimoto Y, Shono Y. Autophagy protein ATG16L1 prevents necroptosis in the intestinal epithelium. J Exp Med. (2017) 214:3687–705. doi: 10.1084/jem.20170558
110. Li-sheng W, Ling-jia P, Li S, Yong S, Ya-li Z, Dian-yuan Z. The apoptosis of experimental colorectal carcinoma cells induced by peptidoglycan of bifidobacterium and the expression of apoptotic regulating genes. Chin J Cancer Res. (1999) 11:184–7. doi: 10.1007/s11670-999-0008-9
Keywords: probiotics, pre-biotics, post-biotics, gut microbiota, immune system
Citation: Liu Y, Wang J and Wu C (2022) Modulation of Gut Microbiota and Immune System by Probiotics, Pre-biotics, and Post-biotics. Front. Nutr. 8:634897. doi: 10.3389/fnut.2021.634897
Received: 29 November 2020; Accepted: 02 December 2021;
Published: 03 January 2022.
Edited by:
Gemma Emily Walton, University of Reading, United KingdomReviewed by:
Alberto Finamore, Council for Agricultural and Economics Research (CREA), ItalyJulio Plaza-Diaz, Children's Hospital of Eastern Ontario (CHEO), Canada
Copyright © 2022 Liu, Wang and Wu. This is an open-access article distributed under the terms of the Creative Commons Attribution License (CC BY). The use, distribution or reproduction in other forums is permitted, provided the original author(s) and the copyright owner(s) are credited and that the original publication in this journal is cited, in accordance with accepted academic practice. No use, distribution or reproduction is permitted which does not comply with these terms.
*Correspondence: Yue Liu, eXVlbGl1JiN4MDAwNDA7c3h1LmVkdS5jbg==; Changxin Wu, Y3h3MjAmI3gwMDA0MDtzeHUuZWR1LmNu