- 1Department of Food Science and Technology, Biotechnical Faculty, University of Ljubljana, Ljubljana, Slovenia
- 2Department of Environmental Sciences, Jožef Stefan Institute, Ljubljana, Slovenia
- 3Department of Low and Medium Energy Physics, Jožef Stefan Institute, Ljubljana, Slovenia
Dietary supplements based on algae, known for their nutritional value and bioactive properties, are popular products among consumers today. While commercial algal products are regarded safe by numerous studies, information about the production and origin of such products is scarce. In addition, dietary supplements are not as strictly regulated as food and medicinal drugs. We characterized different algal products (kelps: Laminariales, Spirulina spp., Chlorella spp., and Aphanizomenon flos-aquae), obtained on Slovenian market, based on their elemental composition (X-ray fluorescence, inductively coupled plasma–mass spectrometry), antioxidative potential [DPPH (2,2-diphenyl-1-picrylhydrazyl) assay, total phenolic content], and stable isotope values [carbon (C), nitrogen (N), and sulfur (S); elemental analyzer isotope ratio mass spectrometry (EA-IRMS) method]. Antioxidative potential is consistent among products of the same type, with A. flos-aquae samples having 4.4 times higher antioxidative potential compared to Chlorella spp. and 2.7 times higher compared to Spirulina spp. Levels of toxic trace elements (arsenic, cadmium, mercury, and lead) are below the maximum allowed values and as such do not pose risk to consumers' health. Samples of Spirulina spp. have relatively high δ15N (7.4 ‰ ± 4.4‰) values, which indicate use of organic nitrogen sources in certain samples. Likewise, different elemental composition and isotopic ratios of stable elements (C, N, and S) for the samples with Spirulina spp. or Chlorella spp. are the consequence of using different nutrient sources and algae-growing techniques. Statistical analysis (principal component analysis) has confirmed that all tested A. flos-aquae samples originate from the same source, supposedly Klamath Lake (Oregon, USA). Hawaiian Spirulina pacifica can also be differentiated from all the other samples because of its characteristically high metal content (iron, manganese, zinc, cobalt, nickel, vanadium). Chlorella spp. and Spirulina spp. require further analyses with larger number of samples, as differentiation is not possible based on results of this study.
Introduction
There are numerous algae-based dietary supplements available on the market, which indicates their widespread use among consumers. Dietary supplements are not subject to strict regulations like drugs and imported food. Therefore, continuous evaluation of efficacy, safety, and origin is required to guarantee quality of dietary supplements. Comparison between different products is also complicated because of addition of unknown compounds, which is a common practice among manufacturers. Microalgae (unicellular eukaryotes and cyanobacteria) are interesting organisms to cultivate because of their ability to synthesize bioactive compounds and accumulate minerals and high nutritional value. They are able to grow in modified mediums, including wastewater, which additionally improves economic viability of cultivating microalgae (1). Currently, most producers of microalgae-based commercial products are located in Asia or Australia and show an impressive growth. Production share of food/feed microalgae products owned by European companies is estimated to be approximately 5% of the global market (2).
Microalgae-based products (Spirulina spp.—Arthrospira spp., Chlorella spp., and Aphanizomenon flos-aquae or AFA) have the highest market share among “algal” dietary supplements. Spirulina spp. products, in tablet or powder form, are mostly consumed because of their nutritional profile: protein (60–70%), carbohydrates (14–19%), fat (8%), dietary fibers (3%), vitamins (<1%), and phytochemicals (3, 4). Algae products are also regarded as a significant source of major elements, such as iron (Fe), calcium (Ca), phosphorus (P), potassium (K), sodium (Na), and magnesium (Mg), and trace elements, including manganese (Mn), zinc (Zn), copper (Cu), selenium (Se), and chromium (Cr). Recommended daily amount of aforementioned algae can provide substantial amount of these minerals and even fulfill recommended dietary allowance for iron intake (4, 5). Studies on content of toxic elements and cyanotoxins are scarce (6). Studies (7–10) done on safety of microalgae do not necessarily reflect safety of algal products, as commercial cultivation practice is unknown and not subject to strict regulations. Results acquired from laboratory grown algae are potentially misleading as different growing conditions significantly impact the content of certain elements and synthesized metabolites in algae (1). Determining efficacy and safety of algal products therefore requires analysis and comparison of individual samples from different manufacturers.
Manufacturers provide little to no information regarding the origin and manufacturing practices of their algae-based dietary supplements. Nutrient composition and toxic compounds differ, depending on the location of sample production. Reasons are various environmental conditions and agrotechnical measures. Thus, to ensure quality and safety of the products used in daily nutrition, determination of product's origin is of great importance (11). Variable environmental conditions that influence microalgal growth can consequently affect the stable isotopic composition of carbon, nitrogen, and sulfur (C, N, and S). These parameters along with elemental composition could be used to verify the quality and origin of microalgal products.
The aim of our study is to differentiate commercially available algal products on Slovenian market by characterizing them based on antioxidative potential, elemental composition and stable isotope composition of C, N, and S, as they can reflect different growing conditions, sources of nutrients and origin. To our knowledge, such an approach has not yet been used in any previous study of algae-based supplementary products.
Materials and Methods
Sample Collection
In the present study, 18 samples were obtained from several physical stores and web stores in Slovenia (2018). Dietary supplements were selected based on several types of algae [kelps: Laminariales (n = 2), Spirulina spp. (n = 7), Chlorella spp. (n = 5), and AFA (n = 4)] with different types of production—conventional and organic. The samples were intended for sale on Slovenian market and have declaration in Slovene language (Table 1).
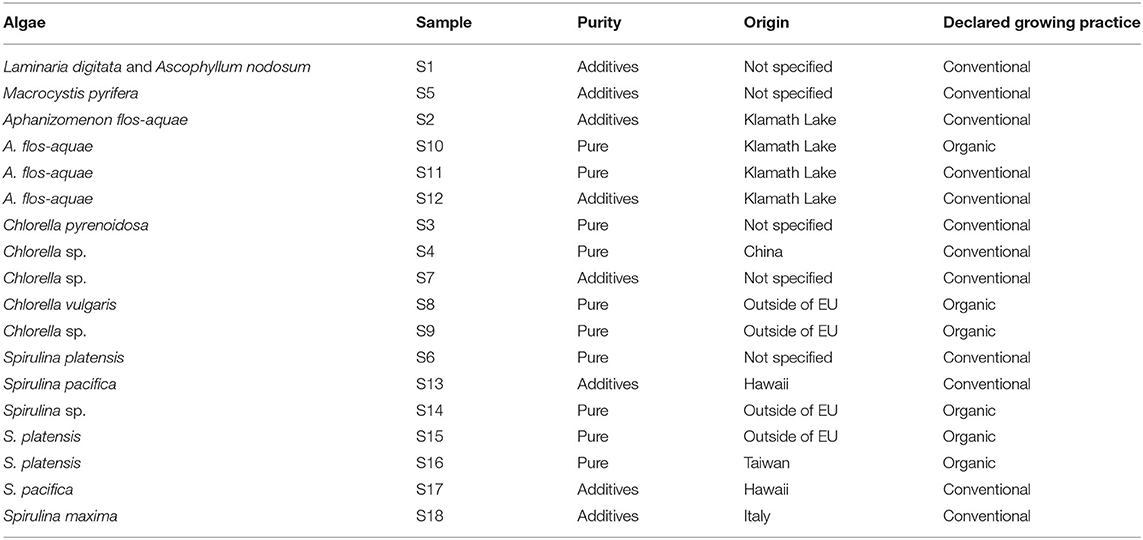
Table 1. List of algae-based dietary supplement samples obtained on Slovenian market with the information on purity, origin, and suggested daily use.
Sample Preparation
Samples in tablet form were ground to fine powder, and samples in capsules were opened. All samples were subsequently stored in powdered form in plastic containers with screw caps. During analysis, all samples were stored at room temperature and kept away from direct sunlight, following the manufacturers' storage guidelines. Sample preparation step was repeated for each individual analysis.
Sample Extract Preparation for Determination of Total Phenolic Compounds and Antioxidative Potential
Five hundred milligrams of fine powder sample was added to 10 mL 80% methanol solution in a centrifuge tube. After vortex mixing it for 5 min, it was incubated in ultrasound bath for 30 min at 40°C. Following incubation, samples were centrifuged for 20 min at 9,400 rcf (at 20°C) and filtrated through filters with 0.32-μm pore width into centrifuge tubes. Ten milliliters of 80% methanol solution was added to resulting sample sediment, and the whole procedure was repeated for each sample. We added 80% methanol solution until 20-mL volume was reached. Sample extracts were prepared in duplicate. Extract of each duplicate sample was stored in four vials, containing 5 mL extract each (total 20 mL per sample duplicate) at −20°C.
Total Phenolic Content
The total phenolic content (TPC) of algal methanolic extracts was determined by Folin–Ciocalteu method (12). Twenty milliliters of Folin–Ciocalteu reagent solution was prepared by mixing MilliQ water (resistivity of 18.2 MΩ*cm (at 25°C) and total organic C value <5 ppb) in ratio 1:10. Mixture in screw cap tube was prepared by adding 0.2 mL Folin–Ciocalteu solution, 0.2 mL sufficiently diluted methanolic sample extract, 1 mL Na carbonate solution (mass concentration of 75 g/L), and 2 mL MilliQ water. After thorough vortex mixing, the mixture was incubated for 2 h in the dark at room temperature, followed by 5-min centrifugation at 2,000 RPM.
Spectrophotometer was calibrated using blind sample. The sample was prepared in the same way as other samples, except that 0.2 mL of 80% methanol was added instead of 0.2-mL sample extract. All measurements were performed at wavelength of 750 nm. Sample dilution ratio was determined for each individual sample by test runs using the same procedure. Kelp samples' total phenolic compounds content was too low to be detected by our method (even after the manipulation of sample dilution ratio in final mixture). Calibration curve for TPC analysis was prepared with gallic acid in triplicate with concentrations 5, 10, 15, and 20 mg/mL. Gallic acid solutions were prepared according to the same protocol as samples, in 80% methanol solution. Results were expressed as mg gallic acid equivalent (GAE)/g solid sample mass.
DPPH Assay
The free radical–scavenging activity of algal extracts were measured by the decrease of absorbance of methanolic solution of 2,2-diphenyl-1-picrylhydrazyl (DPPH) (13). A stock methanolic solution of DPPH (0.0837 μM) was prepared by mixing 3.3 mg DPPH in 100 mL of pure methanol. Absorbance of stock DPPH solution was ~1.1 at 517 nm. Final mixture was prepared by mixing 0.5 mL of sufficiently diluted sample extract and 2.5 mL of methanolic DPPH solution. Blank samples were prepared with 0.5 mL diluted sample extract and 2.5 mL pure methanol. Control sample was prepared with 0.5 mL pure methanol and 2.5 mL methanolic DPPH solution. Absorbance was measured after 30-min incubation period at room temperature in the dark.
Sample dilution ratio was determined for each individual sample by test runs using the same procedure. Antioxidative potential of kelp samples was below detection of our method and could not be determined. On the contrary, AFA samples required dilution in ratio 1:5. Calibration curve for DPPH analysis was prepared with gallic acid in triplicate with concentrations 6, 4.5, 3, 1.5 and 0.75 μg/mL. Like samples, gallic acid was prepared in 80% methanol solution.
Elemental Composition Determination Using X-Ray Fluorescence
X-ray fluorescence (XRF) analysis was performed at Jožef Stefan Institute, Ljubljana. Powdery samples were pressed into tablets. Quality assurance for element analysis was performed using standard reference materials 1573A National Institute of Standards and Technology (NIST) tomato leaves and 1547 NIST peach leaves, acquired from the NIST (Gaithersburg, MD, USA). XRF was used to determine the following elements: bromine (Br), calcium (Ca), chlorine (Cl), iron (Fe), iodine (I), potassium (K), manganese (Mn), phosphorus (P), rubidium (Rb), sulfur (S), silicon (Si), strontium (Sr), titanium (Ti), and zinc (Zn).
Sample Preparation for Inductively Coupled Plasma–Mass Spectrometry
Samples were digested using an UltraWAVE digestion system (Millestone, Italy); 0.05–0.1 g of sample was weighted directly into Teflon vial, followed by addition of 2 mL of 65% HNO3 (Suprapur, Merck, Germany). Digestion temperature program was as followed: from room temperature to 240°C in 20 min, held on 240°C for 15 min, and then cooled to 40°C (approximately 1 h). Maximum pressure was set at 100 bar. Loading gas (N2) was set at 25 bar and room temperature.
Digested samples were transferred into plastic vials and filled to 10-mL mark with MilliQ water. Samples were additionally diluted with 5% HNO3 in 1:5 ratio. Because of visible residuals, the samples were filtered through 0.45-μm hydrophilic syringe filters (Millipor Millex-HV, Merck, Germany). Quality assurance for element analysis was performed using standard reference material BCR-414 (trace elements in plankton) with known elemental composition. Reagents' blanks were prepared according to the same protocol as samples.
Elemental Composition Determination Using Inductively Coupled Plasma–Mass Spectrometry
Inductively coupled plasma–mass spectrometry (ICP-MS) analysis was performed on an Agilent 8800 triple quadrupole instrument (Agilent Technologies, California, USA). Calibration curve for mercury (Hg) content determination was prepared using NIST 3133 Hg standard solution in the following concentrations: 0, 0.1, 0.5, 1, and 5 ng/mL. Elements [V, Mn, Co, Ni, Cu, Zn, As, Se, Sr, Mo, Cd, Pb and Fe] were determined using MULTI XVI (Merck, Germany) multielement standard solution for ICP-MS. Calibration curve was prepared in 5% HNO3 by using the following concentrations of MULTI XVI: 0, 0.1, 0.5, 1, 5, 10, 50, 100, and 250 ng/mL.
Each sample was analyzed in dilutions (with 5% HNO3) to 10 and 100 mL. Samples S1, S4, S7, and S10 were prepared in duplicate (two parallels for 10-mL dilution, two parallels for 100-mL dilution). ICP-MS was used for determination of following trace elements: As, Cd, Co, Cu, Hg, Mn, Mo, Ni, Pb, Se, Sr, V, and Zn.
Stable Isotope Ratio Analysis of Light Elements Using EA-IRMS
Stable isotope ratios of 13C/12C, 15N/14N, and 34S/32S were expressed as δ values in ‰ according to the following equation (14):
where E represents element (C, N, S), R is isotope ratio between heavier “i” and lighter “j” isotopes (13C/12C, 15N/14N, 34S/32S) in the “sample” and reference material (“ref”). Values for C were expressed relative to V-PDB (Vienna-Pee Dee Belemnite) standard, N values relative to AIR, and S values relative to V-CDT (Vienna Cañon Diablo Troilite) standard.
Stable isotope ratios of light elements (13C/12C, 15N/14N, 34S/32S) in algae samples were simultaneously determined by isotope ratio mass spectrometry with preparation system for solid samples IsoPrime 100–Vario PYRO Cube (OH/CNS Pyrolyser/Elemental Analyzer, Elementar Analysensysteme GmbH, Germany). Four milligrams of sample and 4 mg of tungsten oxide (WO3) were weighted directly into tin capsules, sealed, and placed into the automatic sampler of the elemental analyzer. Each sample was measured in triplicate, and the average value was considered. Quality assurance for stable isotope ratio analysis was performed using the following reference materials: USGS-43: δ13C = −21.28 ± 0.10‰, δ15N = +8.44 ± 0.10‰, δ34S = +10.46 ± 0.22‰; B2155 Protein Sercon: δ13C = −26.98 ± 0.13‰, δ15N = +5.94 ± 0.08‰, δ34S = +6.32‰ ± 0.8‰; Casein Protein CRP: δ13C = −20.34 ± 0.09‰, δ15N = +5.62 ± 0.19‰, δ34S = +4.18‰ ± 0.74‰. Measurement precision value was ±0.2‰ for δ13C, and ± 0.3‰ for δ15N and δ34S.
Statistical Analysis
Statistical calculations were carried out using the XL-STAT software package (Addinsoft, Long Island, NY, USA, 2019). First, basic statistics were applied to the data. Because most of the data were not normally distributed (Shapiro-Wilk test, p < 0.05), the nonparametric Mann–Whitney U test was used for comparison of element content between different microalgae products. In all analyses, p < 0.05 was considered as statistically significant.
Further, principal component analysis (PCA) was applied. PCA is an unsupervised pattern recognition multivariate statistical tool able to analyze numerical dataset structured in an M observations/N variables table and recognize underlying patterns in the dataset. The results of such analysis are displayed as biplots, which are simultaneous representations of variables and observations in the space of selected two PCA axes (e.g., PC1/PC2). The biplots enable visualization and increase interpretability of relation and trends among observations and variables on a two-dimensional map and identify similarities and differences among observations, association with variables, and also impact and role of a particular variable in discrimination and clustering of observations.
PCA was used to enable identification of characteristic parameters that are able to discriminate samples based on antioxidative potential, stable isotope composition of light elements and elemental composition. Samples of kelps (Laminariales) were excluded from PCA, because of relatively low content of algae in dietary supplement products and significant physiological differences compared to microalgae.
Results and Discussion
Total Phenolic Content and Antioxidative Potential
Average values of TPC of different dietary supplements based on algae, expressed as mg GAE/g solid sample, were 12.3 ± 1.2 for samples of Chlorella spp., 23.2 ± 6.4 for Spirulina spp., 96.4 ± 7.5 for AFA, and 1.6 ± 1.6 for kelp (Figure 1). AFA samples contained the highest amounts of TPC, followed by Spirulina spp. and Chlorella spp. For comparison, Al-Dhabi and Valan Arasu (15) determined TPC values of 2.4–24.4 mg GAE/g sample for Spirulina spp. Kelp samples had negligible TPC content, presumably due to the low algae content in the sampled product itself (13–14% according to the declaration). It should be noted that kelp samples were not homogenous (brown algal parts tabletized with filler); therefore, the measurements might be incorrect.
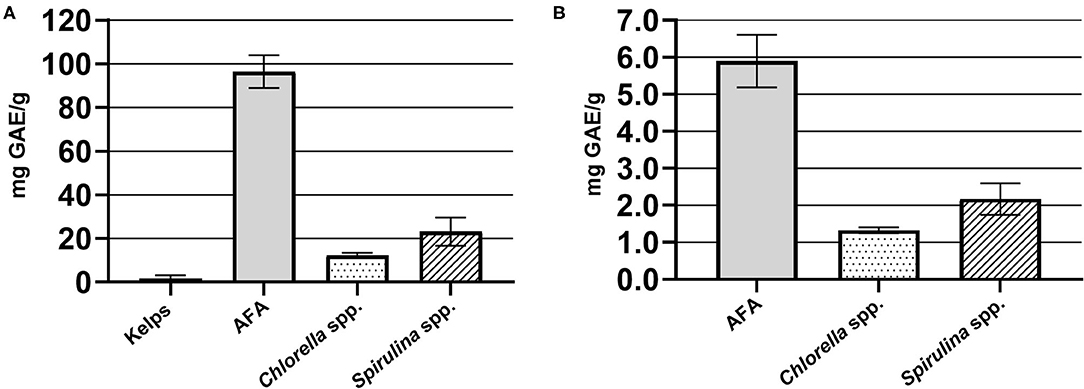
Figure 1. (A) Average total phenolic compounds (Folin–Ciocalteu method); (B) average antioxidative potential values (DPPH method) in different types of algae-based dietary supplement samples, expressed as mg gallic acid equivalent (GAE)/g solid sample.
Average antioxidative potential values, determined by the DPPH method, for different types of algae-based dietary supplement samples (expressed as mg GAE/g solid sample) were 1.33 ± 0.08 for Chlorella spp., 2.17 ± 0.43 for Spirulina spp., and 5.90 ± 0.71 for AFA (Figure 1). Antioxidative potential of kelp was significantly lower in comparison to other algae samples and as such could not be measured by using our method.
AFA samples showed the highest measured antioxidative potential, which was 4.4 times the value of Chlorella spp. samples and 2.7 times the value of Spirulina spp. samples. There were no significant differences among similar products from different manufacturers regarding antioxidative potential. The latter is evident from the relatively small deviations in measured values among sample groups with the same algae type (Figure 1). Consequently, antioxidative potential allows discrimination of samples between different algae types. It should be noted that presented results of antioxidative potential do not assess the efficacy in relation to health benefits of algal products, as our research goal is mainly characterization of different product types.
Stable Isotope Composition of Light Elements
δ13C value in algae is reflected by their C source (16). Average δ13C value of Spirulina spp. samples was −23.0‰ ± 4.0‰. Unusually high δ13C value of −17.4‰ of sample S18 could be explained by declared addition of corn maltodextrin, which has characteristic δ13C value of C4 plants (from −15‰ to −12‰) (17). Chlorella spp. had similar δ13C values with an average value of −27.5‰ ± 5.7‰, with sample S3 having the lowest value of −37.1‰ (Table 2). The low δ13C value determined in Chlorella spp. could be related to the growing conditions in a closed system. Closed systems are closed bioreactors with higher control over growing conditions (pH and temperature), higher photosynthetic efficiency, lower water evaporation rate, and lower CO2 loss to the atmosphere (18). It was found that the δ13C value in a closed system exhibits lower δ13C value of CO2. Because of relatively small deviation among samples of Spirulina spp. and Chlorella spp., we assume they utilize similar sources of and CO2 during cultivation, presumably ones that have shown to be the most efficient from manufacturer's point of view.
Spirulina spp. samples had an average δ15N value of 7.4‰ ± 4.4‰, indicating organic source of N in samples with high δ15N values (>6‰), possibly due to wastewater use (19). This also applies to kelp samples. Higher δ15N values also indicate usage of modified mediums for algae cultivation, as the latter can significantly improve economic viability of the project. One sample (S6) of Spirulina platensis differed from other samples with δ15N value of 1.2‰, indicating inorganic source of N or molecular N (air) fixation. Differences in δ15N values between samples can also be explained by other factors, such as (i) different climate, which is hard to control in open bioreactors, and (ii) recycling of growth medium (1). Samples of Chlorella spp. generally showed lower δ15N values (3.5 ± 6.0‰) compared to Spirulina spp. samples. This is probably due to Chlorella manufacturers using less optimized and modified growing methods compared to Spirulina manufacturing. Spirulina is able to grow in saline environments (8), which is exploited to prevent contamination by other microorganisms when growing in “low-quality” media. As original media use mostly inorganic source of N (1), we can assume that most samples were grown using modified media. Lack of information from manufacturers makes interpretation of results rather difficult, as we do not have any insight into geographical factors that may affect fractionation.
AFA samples had similar stable isotope composition of C and N, which indicates that samples originate from the same source (all AFA products are declared to originate from Klamath Lake, OR). Small deviations in stable isotope composition of C and N in sample S2 were probably due to presence of additives in the product. Relatively high values of δ13C (−15.5 ± 1.1‰) in AFA samples might indicate photosynthetic fixation of CO2 from air as their primary source of C. Values of δ15N were around zero (0.3 ± 1.1‰), indicating fixation of molecular N from air.
The δ34S values in algae-based dietary supplement samples ranged from −3.0 to 16.8‰, with the lowest values observed in Chlorella spp. and the highest in kelps (brown algae). Variability in δ34S values among the samples of same algae species (with the exception of AFA) was on average higher for C and N. This variability can be explained by different origins of samples or differences in organic load during growing conditions. However, the information regarding the distribution of the δ34S values of aquatic resources and organisms is scarce. There are three potential sources of S in algae, depending on the proximity to the ocean, geology, and redox chemistry. For example, the δ34S of marine sulfate and vegetation near the ocean are approximately +20‰ but decrease to +6‰ over 100 km (20, 21). In the Hawaiian Islands, δ34S values of sulfates from volcanic ash and basalt-derived soils ranging from 6.3 to 15.4‰ (22) have been reported and are also in agreement with our data.
Elemental Analysis
The results of the elemental composition in microalgae supplements are presented in Supplementary Table 1. No statistically significant difference between different types of algae supplements was observed for Si, V, Mn, Ti, Co, Ni, Rb, Cu, Se, Mn, Fe, and Hg. A significantly higher content of Ca was observed for Spirulina spp. products, whereas Chlorella spp. displayed the highest P level. This observation agrees with the study performed by Rzymski et al. (7). AFA products exhibited high Ca and Mo concentrations that differ statistically significantly from other products. The highest concentrations of Sr, Br, and Cl were determined in kelps samples and differ significantly from other products mainly from Chlorella spp. Zn levels did not differ between Spirulina spp. and Chlorella spp., but they are significantly higher than those observed in AFA and kelps.
Iron Content
Average Fe content (mg Fe/g solid sample) in samples of Chlorella spp. was 1.00 ± 0.52, Spirulina spp. 1.36 ± 1.33, AFA 0.44 ± 0.05, and kelps 0.13 ± 0.01 (Figure 2). High deviation among Spirulina spp. samples is due to higher Fe content in Hawaiian Spirulina pacifica samples (3.29 ± 0.27). Iron content in Spirulina reflects the Fe content in the medium used for cultivation (23).
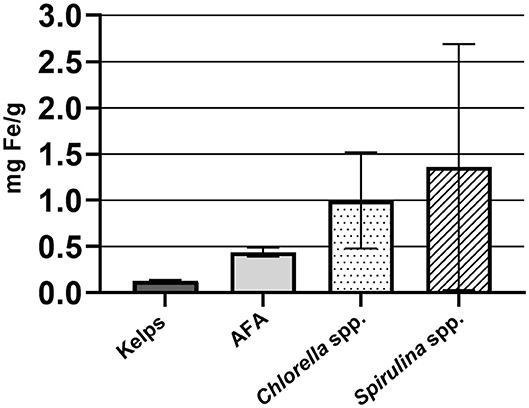
Figure 2. Average iron content in algal dietary supplement samples, expressed as mg iron/g solid sample.
Iodine Content
Iodine content in kelp samples was 183 mg/kg solid sample for S1 and 221 mg/kg for S5, with 3% and 11% deviation from values declared on the product. Variability of I content in kelp-based supplements is therefore lower compared to edible seaweed (24). Other algae samples had I content below the limit of detection of XRF method.
Toxic Elements Content in Algal Dietary Supplements
Statistically significant difference in As concentration was found between Chlorella spp. and Spirulina spp. samples and AFA and kelp-based algae. Average total arsenic (As) content of samples was 0.26 ± 0.17 mg/kg for Chlorella spp. and 0.73 ± 0.96 mg/kg for Spirulina spp. samples (Figure 3). AFA and kelp-based samples had higher total As content, which was between 3.5 and 6.5 mg/kg solid sample. At the time of writing, European Commission (25) has not set upper tolerable level for As content in dietary supplements. It should be noted that our analysis determined only total As. For health risk assessment, determination of As species is required as the inorganic form of As is more toxic compared to the organic form.
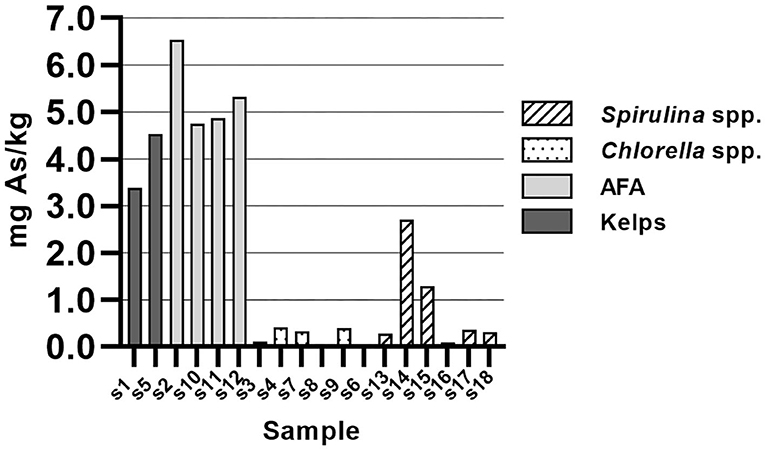
Figure 3. Arsenic content in samples of algal dietary supplements, expressed as mg total arsenic/kg solid sample.
Cd content was below maximum allowed value for dietary supplements of 1.0 mg/kg, set by European Commission (25) (Figure 4). Kelp sample (S5) had notable Cd content of 0.55 mg/kg solid sample and as such differs significantly from other samples. Other samples had Cd content between 0.011 and 0.064 mg/kg. With respect to Cd content, none of the samples pose risk to consumers' health.
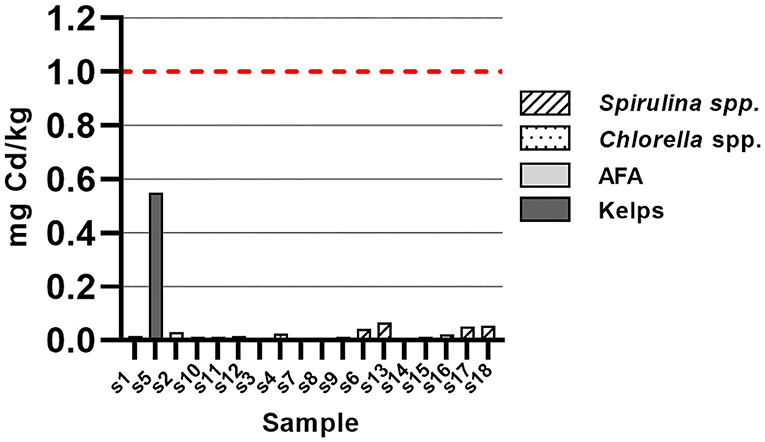
Figure 4. Cadmium content in samples of algal dietary supplements, expressed as mg total cadmium/kg solid sample.
Samples of kelps (S1) and AFA (S2) exceeded maximum allowed value of Hg in dietary supplements by factor of 3.5 and 4.4, respectively (Figure 5). Maximum allowed value of Hg in dietary supplements is set at 0.1 mg/kg by European Commission (25). Hg content of other samples was below maximum allowed value. One sample of Chlorella spp. (S8) had Hg content below LOD. Despite the exceeded values of Hg in two samples, it should be noted that maximum Hg content for fish is set much higher (compared to dietary supplements) at 1.0 mg/kg fish muscle (25). By ingesting manufacturer's recommended daily dose of sample (4.02 g) with highest Hg content, we would ingest 1.76 μg of Hg. In contrast, eating 100 g of fish flesh with Hg content at limit (1.0 mg/kg) would equate to ingesting 0.1 mg Hg, which is 57 times higher than daily dose of sample (S2) with the highest Hg content.
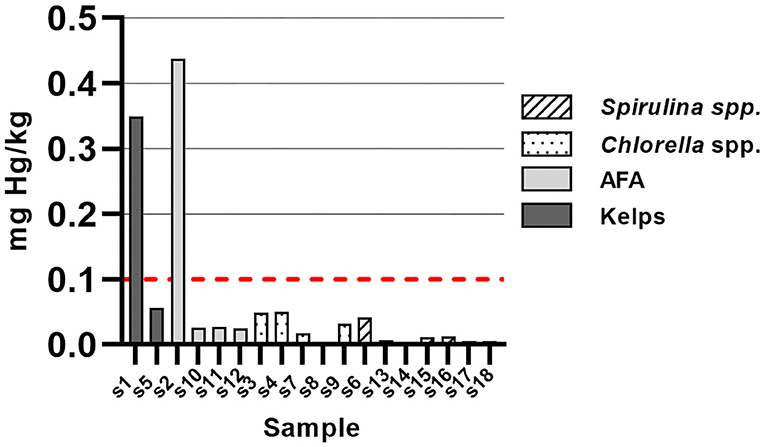
Figure 5. Mercury content in samples of algal dietary supplements, expressed as mg total mercury/kg solid sample.
Pb content was below maximum allowed value (3.0 mg/kg) (25) in all samples of algal dietary supplements (Figure 6). Kelps sample (S1) had Pb content below LOD. Pb content of samples was 0.35 ± 0.22, 0.23 ± 0.19, and 0.02 ± 0.00 mg/kg for Spirulina spp., Chlorella spp., and AFA, respectively, where Spirulina spp. differ significantly from AFA samples. In contrast with our results, Rzymski et al. (7) report of average Pb content of 2.6 ± 1.3 mg/kg for Chlorella spp. samples and 2.6 ± 1.9 mg/kg for Spirulina spp. samples, where 40% of Chlorella spp. and 30% of Spirulina spp. samples exceeded maximum allowed value.
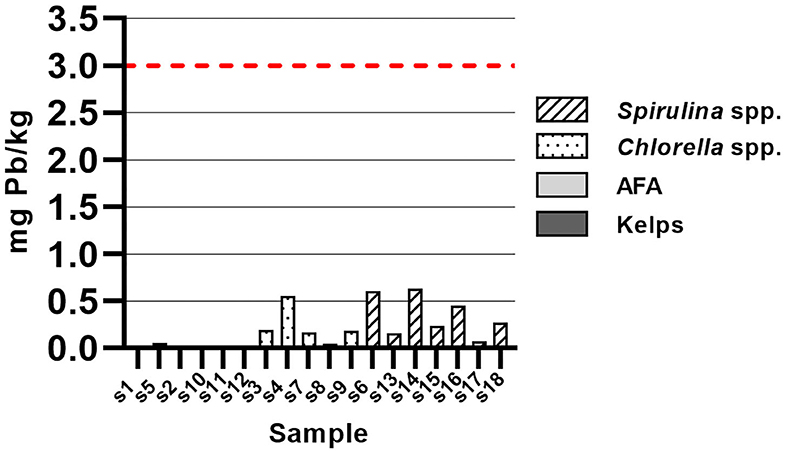
Figure 6. Lead content in samples of algal dietary supplements, expressed as mg total Pb/kg solid sample.
Toxic elements such as Hg, Cd, Pb, and As were detected only in trace amounts and as such do not pose risk to consumers' health, which is in agreement with other studies (7, 10). It should be noted that algal products also require determination of algal toxins to fully evaluate their safety (6).
PCA of Microalgae Samples
AFA-based products are distinguishable from other samples (Figure 7). They have characteristically high antioxidative potential (TPC and DPPH); high Mo, Ca, and Sr content; low P content; relatively low δ15N values; and high δ13C values. Sample S2 slightly deviates from AFA group, possibly due to additives (all other AFA samples are declared as pure). Based on our PCA, we can claim that AFA samples originate from the same source, supposedly Klamath Lake, OR. Interestingly, sample S10 is declared as organic, whereas other samples have no such declaration. Such labeling discrepancy is unexpected, considering all our AFA samples are advertised to originate from Klamath Lake.
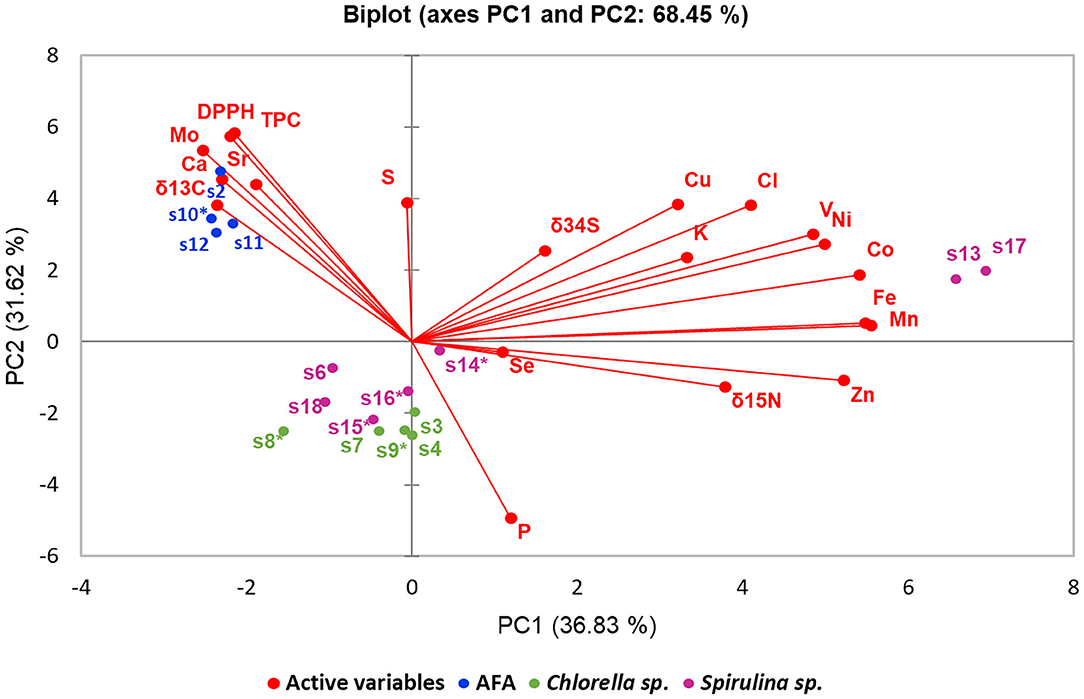
Figure 7. Principal component analysis of algal dietary supplements analysis results (antioxidative potential, elemental, and stable isotope composition data). The biplot shows data of samples as dots (scores) and analysis results (loadings) as vectors. Samples marked with * are declared as organic.
Samples of Chlorella spp. and Spirulina spp. cannot be reliably distinguished using PCA (with exception of Hawaiian S. pacifica) because of lack of characteristic parameters of respective microalgae (Figure 7). Organically grown Spirulina spp. and Chlorella spp. also do not exhibit any characteristic parameters, including δ15N values, where high values usually indicate assimilation of organic N originating from wastewater. Two S. pacifica. samples (S13 and S17), originating from Hawaii, are well separated from other samples based on Fe, Mn, Zn, Co, Ni, V, K, Cl, Cu, and δ15N values and δ34S values, whereas the sample from Italy (S18) cannot be distinguished from other Spirulina spp. samples originating from non-EU countries.
Hawaiian S. pacifica samples S13 and S17 (Figure 7) significantly differ from other analyzed samples. That is largely due to significantly higher content of elements such as Co, Mn, Fe, Ni, V, and Zn compared to other samples, which is shown by their respective loadings (Figure 7).
By combining results from stable isotope composition, antioxidative potential, and elemental composition, we can reliably discriminate S. pacifica and AFA from our samples. Discrimination between Chlorella spp. and Spirulina spp. is not possible based on our results because of insufficient number of samples and scarce information provided by the manufacturers.
Data Availability Statement
The raw data supporting the conclusions of this article will be made available by the authors, without undue reservation.
Author Contributions
NO, NP, and JK: Conceptualization. JK, NP, NO, and JM: Methodology. MJ, JK, and MN: Validation. JK, MN, and MJ: Formal analysis. JK, MJ, JM, and MN: Investigation. NP, NO, and MN: Resources. JK: Writing—original draft preparation and visualization. JK, MJ, NO, NP, and MN: Writing—review and editing. NP and NO: Supervision, project administration, and funding acquisition. All authors contributed to the article and approved the submitted version.
Funding
This research was funded by Slovenian Research Agency (P4-0121, P1-0143 and J4-1773: Lactic acid fermentation for enrichment of microalgae biomass with new nutrients).
Conflict of Interest
The authors declare that the research was conducted in the absence of any commercial or financial relationships that could be construed as a potential conflict of interest.
Acknowledgments
We would also like to acknowledge the technical support provided by Stojan Žigon and Doris Potočnik for stable isotope analysis. We would also like to thank our colleague Miha Bahun for providing help in the laboratory.
Supplementary Material
The Supplementary Material for this article can be found online at: https://www.frontiersin.org/articles/10.3389/fnut.2020.618503/full#supplementary-material
References
1. Costa JAV, Freitas BCB, Rosa GM, Moraes L, Morais MG, Mitchell BG. Operational and economic aspects of Spirulina-based biorefinery. Bioresour Technol. (2019) 292:121946. doi: 10.1016/j.biortech.2019.121946
2. Vigani M, Parisi C, Rodríguez-Cerezo E, Barbosa MJ, Sijtsma L, Ploeg M, et al. Food and feed products from micro-algae: Market opportunities and challenges for the EU. Trends Food Sci Technol. (2015) 42:81–92. doi: 10.1016/j.tifs.2014.12.004
3. Holman BWB, Malau-Aduli AEO. Spirulina as a livestock supplement and animal feed. J Anim Physiol Anim Nutr (Berl). (2013) 97:615–23. doi: 10.1111/j.1439-0396.2012.01328.x
4. Tokuşoglu O ÜMK. Biomass Nutrient profiles of three microalgae. J Food Sci. (2003) 68:1144–48. doi: 10.1111/j.1365-2621.2003.tb09615.x
5. Sharoba A. Nutritional value of spirulina and its use in the preparation of some complementary baby food formulas. J Food Dairy Sci. (2014) 5:517–38. doi: 10.21608/jfds.2014.53033
6. Grosshagauer S, Kraemer K, Somoza V. The true value of spirulina. J Agric Food Chem. (2020) 68:4109–115. doi: 10.1021/acs.jafc.9b08251
7. Rzymski P, Budzulak J, Niedzielski P, Klimaszyk P, Proch J, Kozak L, et al. Essential and toxic elements in commercial microalgal food supplements. J Appl Phycol. (2019) 31:3567–79. doi: 10.1007/s10811-018-1681-1
8. ANSES. Risks associated with the consumption of food supplements containing Spirulina. (2017) 33:1–38. Available online at: https://www.anses.fr/en/content/anses-opinion-risks-associated-consumption-food-supplements-containing-spirulina
9. De Morais MG, Vaz BDS, De Morais EG, Costa JAV. Biologically active metabolites synthesized by microalgae. Biomed Res Int. (2015) 2015:835761. doi: 10.1155/2015/835761
10. Al-Dhabi NA. Heavy metal analysis in commercial Spirulina products for human consumption. Saudi J Biol Sci. (2013) 20:383–8. doi: 10.1016/j.sjbs.2013.04.006
11. Drivelos SA, Georgiou CA. Multi-element and multi-isotope-ratio analysis to determine the geographical origin of foods in the European Union. TrAC - Trends Anal Chem. (2012) 40:38–51. doi: 10.1016/j.trac.2012.08.003
12. Singleton VL, Rossi JA, Jr J. Colorimetry of total phenolics with phosphomolybdic-phosphotungstic acid reagents. Am J Enol Vitic. (1965) 16:144–58.
13. Brand-Williams W, Cuvelier ME, Berset C. Use of a free radical method to evaluate antioxidant activity. LWT - Food Sci Technol. (1995) 28:25–30. doi: 10.1016/S0023-6438(95)80008-5
14. Gannes LZ, Del Rio CM, Koch P. Natural abundance variations in stable isotopes and their potential uses in animal physiological ecology. Comp Biochem Physiol A Mol Integr Physiol. (1998) 119:725–37. doi: 10.1016/S1095-6433(98)01016-2
15. Al-Dhabi NA, Valan Arasu M. Quantification of phytochemicals from commercial spirulina products and their antioxidant activities. Evidence-based complement. Altern Med. (2016) 2016:7631864. doi: 10.1155/2016/7631864
16. Gu B, Alexander V. Stable carbon isotope evidence for atmospheric CO2 uptake by cyanobacterial surface scums in a eutrophic lake. Appl Environ Microbiol. (1996) 62:1803–4. doi: 10.1128/aem.62.5.1803-1804.1996
17. Von Caemmerer S, Ghannoum O, Pengelly JJL, Cousins AB. Carbon isotope discrimination as a tool to explore C4 photosynthesis. J Exp Bot. (2014) 65:3459–70. doi: 10.1093/jxb/eru127
18. Pires JCM, Alvim-Ferraz MCM, Martins FG, Simões M. Carbon dioxide capture from flue gases using microalgae: Engineering aspects and biorefinery concept. Renew Sustain Energy Rev. (2012) 16:3043–53. doi: 10.1016/j.rser.2012.02.055
19. Zhang Y, Li F, Zhang Q, Li J, Liu Q. Tracing nitrate pollution sources and transformation in surface- and ground-waters using environmental isotopes. Sci Total Environ. (2014) 490:213–22. doi: 10.1016/j.scitotenv.2014.05.004
20. Wadleigh MA, Schwarcz HP, Kramer JR. Isotopic evidence for the origin of sulphate in coastal rain. Tellus, Ser B Chem Phys Meteorol. (1996) 48:44–59. doi: 10.3402/tellusb.v48i1.15665
21. Wadleigh MA, Blake DM. Tracing sources of atmospheric sulphur using epiphytic lichens. Environ Pollut. (1999) 106:265–71. doi: 10.1016/S0269-7491(99)00114-1
22. Mizota C, Sasaki A. Sulfur isotope composition of soils and fertilizers: Differences between Northern and Southern Hemispheres. Geoderma. (1996) 71:77–93. doi: 10.1016/0016-7061(95)00091-7
23. Sukumaran P, Dahlan FL, Omar H, Ismail A. Macro - and micronutrients status in Arthrospira platensis grown in Fresh water and brackish water medium. Int J Curr Microb Appl Sci. (2014) 3:384–91.
24. Teas J, Pino S, Critchley A, Braverman LE. Variability of iodine content in common commercially available edible seaweeds. Thyroid. (2004) 14:836–41. doi: 10.1089/thy.2004.14.836
25. European Parliament. EC no 629/2008. Off J Eur Union. (2008) 3.7.2008:2006–9. Available online at: https://eur-lex.europa.eu/eli/reg/2008/629/oj
Keywords: algae, Spirulina, Chlorella, Aphanizomenon flos-aquae, antioxidative potential, stable isotopes, elemental composition, toxic elements
Citation: Kejžar J, Jagodic Hudobivnik M, Nečemer M, Ogrinc N, Masten Rutar J and Poklar Ulrih N (2021) Characterization of Algae Dietary Supplements Using Antioxidative Potential, Elemental Composition, and Stable Isotopes Approach. Front. Nutr. 7:618503. doi: 10.3389/fnut.2020.618503
Received: 17 October 2020; Accepted: 29 December 2020;
Published: 05 February 2021.
Edited by:
Dana Alina Magdas, National Institute for Research and Development of Isotopic and Molecular Technologies, RomaniaReviewed by:
Gabriela Cristea, National Institute for Research and Development of Isotopic and Molecular Technologies, RomaniaHock Eng Khoo, Guilin University of Technology, China
Copyright © 2021 Kejžar, Jagodic Hudobivnik, Nečemer, Ogrinc, Masten Rutar and Poklar Ulrih. This is an open-access article distributed under the terms of the Creative Commons Attribution License (CC BY). The use, distribution or reproduction in other forums is permitted, provided the original author(s) and the copyright owner(s) are credited and that the original publication in this journal is cited, in accordance with accepted academic practice. No use, distribution or reproduction is permitted which does not comply with these terms.
*Correspondence: Nataša Poklar Ulrih, bmF0YXNhLnBva2xhckBiZi51bmktbGouc2k=