- 1Department of Cardiology, Leiden University Medical Center, Leiden, Netherlands
- 2Department of Endocrinology, Leiden University Medical Center, Leiden, Netherlands
- 3Department of Health Sciences, VU Medical Center, Amsterdam, Netherlands
- 4Department of Immunology, Erasmus MC, University Medical Center Rotterdam, Rotterdam, Netherlands
- 5Laboratory Medical Immunology, Department of Immunology, Erasmus MC, University Medical Center Rotterdam, Rotterdam, Netherlands
- 6Division of Clinical Immunology, Department of Internal Medicine, Erasmus MC, University Medical Center Rotterdam, Rotterdam, Netherlands
- 7Division of Endocrinology, Department of Internal Medicine, Erasmus MC, University Medical Center Rotterdam, Rotterdam, Netherlands
- 8Obesity Center CGG, Erasmus MC, University Medical Center Rotterdam, Rotterdam, Netherlands
Background: COVID-19 is a global challenge to healthcare. Obesity is common in patients with COVID-19 and seems to aggravate disease prognosis. In this review we explore the link between obesity, chronic disease, lifestyle factors and the immune system, and propose societal interventions to enhance global immunity.
Search Strategy and Selection Criteria: We performed three literature searches using the keywords (1) coronavirus AND comorbidities, (2) comorbidities AND immune system, and (3) lifestyle factors AND immune system. Results were screened for relevance by the main author and a total of 215 articles were thoroughly analyzed.
Results: The relationship between obesity and unfavorable COVID-19 prognosis is discussed in light of the impact of chronic disease and lifestyle on the immune system. Several modifiable lifestyle factors render us susceptible to viral infections. In this context, we make a case for fostering a healthy lifestyle on a global scale.
Conclusions: Obesity, additional chronic disease and an unhealthy lifestyle interactively impair immune function and increase the risk of severe infectious disease. In adverse metabolic and endocrine conditions, the immune system is geared toward inflammation. Collective effort is needed to ameliorate modifiable risk factors for obesity and chronic disease on a global scale and increase resistance to viruses like SARS-CoV-2.
Introduction
In recent months the entire globe has been affected by coronavirus disease 2019 (COVID-19), caused by severe acute respiratory syndrome coronavirus 2 (SARS-CoV-2) (1). Worldwide more than 11 million cases of COVID-19 have been detected until July 2020 and over 500,000 cases have proved fatal (2) Despite serious efforts, no effective vaccine has been developed yet. But, even without a vaccine, the impact of subsequent waves of SARS-CoV-2 spreading can most likely be decreased. Among patients with COVID-19, obesity, hypertension, cardiovascular disease (CVD) and type 2 diabetes (T2D) are noticeable risk factors for hospitalization, ICU admission, and death. Apparently, chronic disease is linked with unfavorable outcome in COVID-19. As appropriate lifestyle intervention effectively ameliorates cardiometabolic conditions, public health could play a pivotal role in the battle against SARS-CoV-2 (3). The aim of this review is to summarize the current understanding of the links between lifestyle, metabolic syndrome, obesity, and increased susceptibility to viral infections. In addition, we aim to investigate whether public health interventions can affect resistance to viral infections.
Search Strategy and Selection Criteria
Three separate literature searches were performed in PubMed, EMBASE, and Google Scholar through April 2020 to May 2020. We considered English-language (systematic) review articles and meta-analyses found using the following term combinations: (1) “Coronavirus” and “diabetes/hypertension/obesity/cardiovascular disease/metabolic syndrome” yielding 1,132 articles on April 29, 2020. (2) “Immune system” and “diabetes/hypertension/obesity/cardiovascular disease/metabolic syndrome” yielding 1,364 articles on May 17, 2020. (3) “Immune system” and “lifestyle” yielding 753 results on June 4, 2020. The complete search strategies can be found in Supplementary Material. References lists of identified publications were also screened for relevant studies. If multiple articles were found per subject, preference went to clarity, relevance, and novelty. A total of 215 articles (17, 151, and 47 for search strategies 1, 2, and 3, respectively) were scrutinized for this review.
Obesity and Cardiometabolic Diseases are Related to Worse Outcome of COVID-19
The Chinese Center for Disease Control reports higher COVID-19 fatality rates in patients with comorbidities as compared with patients without (4). The fatality rate for patients with COVID-19 without comorbidities was 0.9%, whereas fatality rates in patients with diabetes (7.3%), CVD (10.5%), and hypertension (6.0%) are clearly higher (4). American and European studies confirm the higher chance of fatality and severe disease in patients with comorbidities and add obesity as another risk factor. According to the American Center for Disease Control and Prevention obesity was present in 48.3% of the hospitalized patients with laboratory-confirmed COVID-19 (5). It was also reported that 89.3% of patients with COVID-19 registered in COVID-NET had other underlying conditions, most of them obesity-related: hypertension (49.7%), chronic lung disease (34.6%), diabetes mellitus (28.3%), and CVD (27.8%). Notably, among patients aged 50–64 years, obesity was most prevalent, followed by hypertension and diabetes mellitus. Among those aged >65 years, hypertension was most prevalent, followed by CVD and diabetes mellitus (5).
Furthermore, a study of 4,103 positively tested patients with COVID-19 in New York revealed more frequent hospitalization of patients with obesity (39.8 vs. 14.5%), CVD (44.6 vs. 16.4%), and diabetes (31.8 vs. 5.4%) than in patients without comorbidities (6).
The Relationship Between Obesity and Unfavorable Outcome of COVID-19
Obesity is evidently associated with COVID-19 in terms of prevalence of symptomatic disease, disease severity and mortality (7, 8). Individuals with obesity have an approximately 3-fold increased risk of severe COVID-19 (7). Each unit increase in BMI was associated with a 12% increase in the risk of severe disease and obesity increased the need for invasive mechanical ventilation (7, 8). Furthermore, in patients below 60 years of age, who are generally considered to have a lower risk for severe disease, obesity appears to be a risk factor for hospital admission and need for critical care (9). Interestingly, similar associations were observed in other (viral) infections. During the Influenza A (H1N1) pandemic of 2009–2011, obesity was strongly associated with a worse disease outcome and death (10). Obesity also increases the duration of Influenza A virus shedding in adults, indicative of an impaired immune response that subsequently facilitates influenza transmission (11). Multiple mechanisms in obesity aggravate prognosis in COVID-19 (Table 1). Below we discuss the most important mechanisms known to date.
The Immune System
The immune system uses two sequential responses to react to disturbances in homeostasis, as seen in tissues under physiological or infectious stress. An initial rapid innate immune response, which has only broad specificity for the trigger is followed by a delayed, highly specific adaptive immune response (12). The latter generates long term target-specific immune memory. Both types of responses require specialized white blood cell types, such as granulocytes, macrophages, and natural killer cells that are innate immune populations, while different types of T- and B-lymphocytes constitute the adaptive immune cells. Although often designated distinct immune responses it should be realized that innate and adaptive responses are highly interdependent.
In obesity, both local and systemic immune alterations occur due to metabolic stress (13). In adipose tissue, the anti-inflammatory/immune regulatory primed immune cells (e.g., M2-type macrophages, regulatory T cells (Treg), T-helper (Th)2, type 2 innate lymphoid cells (ILC2) normally present in lean adipose tissue are replaced by increased numbers of pro-inflammatory primed immune cells (e.g., M1 macrophages, Th1, Th17, CD8+ T-cells) that secrete pro-inflammatory cytokines such as IL-1β, IL-6, IL-17, and IFN-γ. This may even be further enhanced by obesity-associated gut inflammation (13). In addition to local adipose tissue immune alterations, systemic immune adaptations are observed in obesity as well, including increased numbers of circulating (inflammatory) monocytes, neutrophils, Th1, Th17, Th22, decreased circulating Treg and elevated pro-inflammatory cytokine levels (14, 15). In concert, these changes lead to a pro-inflammatory state of the immune system in individuals with obesity, characterized by elevated cytokine levels, both locally in the adipose tissue as well as systemically, as depicted in Figure 1. This chronically elevated inflammatory state is thought to stimulate regulatory pathways, which in turn limit the response to an acute trigger such as SARS-CoV-2. This is exemplified by the hampered antiviral type I interferon response in individuals with obesity (16).
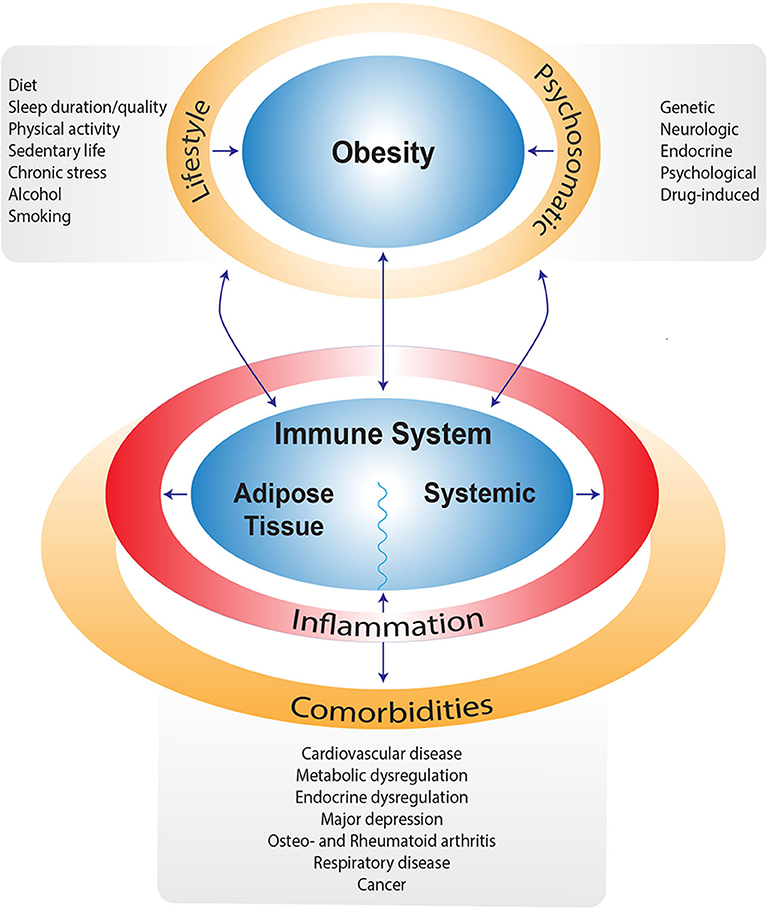
Figure 1. Schematic overview of factors contributing to obesity and/or impairing weight loss. Next to obesity, most of these factors are also directly related to alterations in the immune system leading to a pro-inflammatory state and subsequent comorbidities.
Besides systemic upregulation of pro-inflammatory cytokines, severe COVID-19 is associated with lymphopenia and T cell exhaustion (17). This may be related to functional depletion of the antigen-presenting dendritic cells due to viral infection, since they express both angiotensin-converting-enzyme 2 (ACE2) and the alternative viral receptor DC-SIGN (18). Substantial T-cell apoptotic death might then be caused by T-cell receptor engagement in the absence of appropriate co-stimulation, normally provided by dendritic cells. Moreover, SARS-CoV-2 hampers the function of CD4+ T cells and promotes excessive activation and possibly subsequent exhaustion of CD8+ T cells. Together, these perturbations of T cell subsets likely diminish host antiviral immunity (19). Obesity further worsens this condition as DC maturation appears to be hampered, which in itself already impacts the elicitation of appropriate T-cell responses (20).
Endocrine and Metabolic Dysregulation in Adipose Tissue
Adipose tissue changes play an important role in obesity associated chronic systemic inflammation. Changes in adipokine secretion, fatty acid induced inflammation, oxidative stress, endoplasmic reticulum (ER) stress, and adipose tissue hypoxia are proposed mechanisms (21). Adipocytes secrete adipokines such as leptin and adiponectin, that can modulate the immune system (21, 22). In obesity, leptin secretion is increased. Leptin is pro-inflammatory and stimulates monocyte proliferation and differentiation into macrophages, modulates the activation of natural killer cells, and induces the production of pro-inflammatory cytokines such as TNFα, IL-6, or IL-12 (21). However, leptin inhibits anti-viral type I interferons (16). At the same time, the secretion of adiponectin, which has opposite immunomodulatory effects, is diminished. Adiponectin inhibits phagocytic activity and production of TNFα production, the synthesis of adhesion molecules, the formation of foam cells and stimulates the release of anti-inflammatory cytokines (e.g., IL-10). Nutrient excess and cell expansion in obesity can induce ER stress. ER stress is linked to the production of reactive oxygen species and the activation of inflammatory pathways (21). In addition, adipose tissue expansion in obesity eventually reaches a point where the capacity of local vasculature is insufficient leading to hypoxia. This hypoxic state aggravates the pro-inflammatory profile through further upregulation of leptin, downregulation of adiponectin, and increased production of pro-inflammatory cytokines by resident macrophages (21).
Respiratory Impairment
Fat deposits in the mediastinum and abdominal cavity reduce compliance of the lungs, chest wall, and entire respiratory system. This likely contributes to respiratory symptoms of obesity such as wheeze, dyspnea, and orthopnea (23). The mechanical effects of obesity produce airway narrowing and closure, increased respiratory system resistance, reduced functional residual capacity, airway hyperresponsiveness, and a ventilation perfusion mismatch, all of which decrease lung function (23). Thus, altered lung function due to mechanical stress probably contributes to the worse prognosis in patients with obesity and COVID-19.
Hemostasis
Obesity is independently associated with both arterial and venous thrombotic events (24). Related mechanisms are increased levels of clotting factors (tissue factor, Factor VII, Factor VIII, and plasminogen activator inhibitor) inflammation and endothelial dysfunction (24). COVID-19 in its severe form is also associated with both serious systemic inflammation and a prothrombotic state, as reflected by a significant increase in fibrinogen and D-dimer levels and high rates of severe pulmonary embolism that forecast a poor prognosis (25). This could constitute another link between obesity and worse outcome in COVID-19 but more research is required to confirm this notion.
ACE2 Receptor, Viral Reservoir and Lymphopenia
Other potential mechanisms through which obesity may lead to worse COVID-19 prognosis are high ACE2 receptor expression and comorbidities. Expression of ACE2—the functional receptor for SARS-CoV-2 and other corona viruses—is upregulated in adipocytes of patients with obesity and diabetes, which turns adipose tissue into a potential target and viral reservoir (11). Potentially, this could result in prolonged viral shedding by adipose tissue, with extended activation of local “preactivated” immune responses and resident cytokine signaling pathways. However, currently, there is no evidence yet for direct SARS-CoV-2 infection of adipose tissue.
Comorbidities
Comorbidities associated with obesity have become major health problems in patients that have been admitted to the hospital (8). Below, we discuss the influence of metabolic syndrome, T2D, CVD, and obstructive sleep apnea (OSA) on the immune system. Obesity associated insulin resistance drives the metabolic syndrome, a condition characterized by a clustering of three or more of the following: central adiposity, elevated blood glucose, elevated plasma triglycerides, elevated blood pressure, and low plasma high density lipoprotein (26). Components of the metabolic syndrome are associated with low-grade chronic inflammation. In metabolic syndrome, levels of leptin are increased aggravating leptin resistance enhancing chronic inflammation. Also, circulating adiponectin levels are decreased in metabolic syndrome, which impairs the resolution of inflammation. In itself, metabolic syndrome increases the risk of T2D and CVD by 2- and 5-fold, respectively (26).
The presence of T2D aggravates the prognosis in patients with COVID-19 (27, 28). It is associated with an approximately 2-fold increase of severe/critical COVID-19 illness, after adjustment for age, sex, obesity, hypertension, and smoking (28). Also, higher ICU admission rates and mortality risk were reported in patients with diabetes and COVID-19 (29). The hazard ratio of death was 1.53 in patients with diabetes after adjustment for age, sex, hypertension, CVD, and cerebrovascular disease (27). Notably, SARS-CoV-1-, MERS-, and influenza-infections are also marked by more severe symptoms in people with diabetes (27). Metabolic disruption, low grade systemic inflammation, and an unbalanced immune response may explain the more serious disease course of infections in T2D (28). This is further discussed in the Diet section.
CVD is a common comorbidity in COVID-19 (5). We did not find any literature that specifically explored the relationship between CVD and the capacity to respond to infectious challenges. Also, hypertension is often reported as a highly frequent comorbidity in severely ill patients with COVID-19 (5). Studies reveal higher ICU admission rates and higher mortality rates in patients with COVID-19 with hypertension (30). However, these data should be interpreted with caution as age and CVD may be important confounders (31). To date, there is no conclusive evidence for an independent causal link between hypertension and increased susceptibility for infectious diseases. Nonetheless, it should be realized that inflammatory conditions caused by innate and adaptive immune responses contribute to, or may even underlie alterations in vasculature, kidney, and the regulating sympathetic nervous system, which together cause hypertension (32).
It is believed that the underlying presence of OSA facilitates susceptibility to SARS-CoV-2 infection, and increases the risk for severe COVID-19 and the overall mortality of the disease (33). The CORONADO study shows that treated OSA is independently associated with risk of death by day 7 (34). Research suggests that when controlling for obesity, the presence of OSA is associated with decreased lung function, decreased lung-transfer factor for carbon monoxide, and, importantly, increased lung inflammation (35). Obesity plays a pivotal role in the pathogenesis of OSA and weight loss of approximately 7–11% leads to clinically significant and meaningful improvement of OSA (36). Lifestyle modification with reduced calorie intake and increased physical activity forms the foundation of all weight loss interventions and seems to have weight-independent benefits in OSA (36). Below we discuss the potential benefits of lifestyle adaptations in light of COVID-19 and its underlying risk factors.
The Influence of Lifestyle Factors on the Immune System
When discussing the relation between obesity and the severity of COVID-19, it is important to emphasize that obesity is a complex disease. Next to societal and lifestyle factors, numerous other factors contribute to weight gain or impair weight loss. For example, medication may promote weight gain, as well as endocrine or hypothalamic disease, genetic syndromes or psychiatric conditions (37). It is counterproductive to blame people for their obesity or for severe progression of COVID-19. The obesity stigma is a serious problem in this context, and guiding people in their attempt to lose weight in a respectful way is of crucial importance (38, 39). Furthermore, when intensive lifestyle intervention turns out to be insufficient to lose weight, other obesity treatments such as pharmacotherapy or bariatric surgery should be considered to attain a healthier weight (40).
The American Endocrine Society and the European Association for the Study of Obesity both include lifestyle interventions, bariatric surgery and pharmacotherapy in recommended weight reduction strategies for people with obesity (41, 42). Besides weight reduction effects, bariatric surgery and pharmacotherapy have also been shown to positively affect the immune system in obese patients (43–46). The rate of weight loss for optimal immune system function is yet unclear. It is known that relatively fast weight reduction through bariatric surgery as well as more gradual weight loss through lifestyle modification both improve immune system markers (44, 47). On the other hand, severe caloric restriction, as well as malnutrition, reduces immunity and renders one more susceptible for disease (48, 49). Nevertheless, the negative impact of obesity on general health and responses to infections, including COVID-19 as discussed above, warrants vigorous lifestyle intervention (47).
Lifestyle features (nutrition, physical activity, sleep, stress, alcohol, smoking) interact with genetics to disrupt physiology and cause disease (50). In this context, a low grade inflammatory process appears to play a central role in many distinct chronic non-communicable disorders (51). Since lifestyle is consequently at the root of these diseases, it follows that lifestyle intervention should be an integral part of their treatment. Accordingly, a recent study shows that lifestyle intervention can partly reverse the pro-inflammatory phenotype associated with obesity (47). Even though the decline in pro-inflammatory markers was correlated with a decline of waist circumference and markers of metabolic syndrome, metabolic improvement was not required for immunological recovery. This suggests that lifestyle factors can directly affect the function of the immune system. Below we discuss the influence of diet, physical activity, stress, smoking, alcohol and sleep on the immune system as depicted in Figure 2.
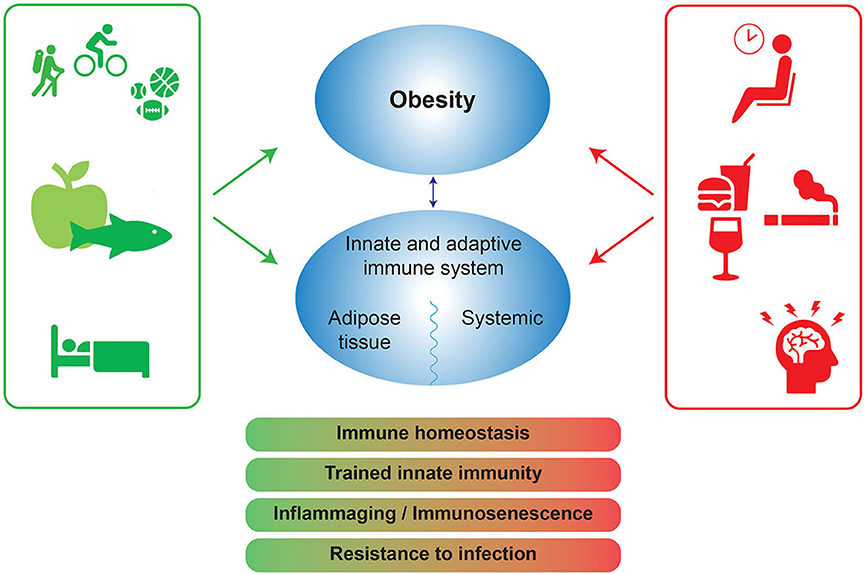
Figure 2. Positive and negative lifestyle factors influence obesity and the immune system. The negative lifestyle factors (red) can lead to a disbalance in immune homeostasis, trained innate immunity, inflammaging, and a decrease in infection resistance. Positive factors (green) can protect immune homeostasis and potentially increase resistance to infection. Trained innate immunity: long term functional reprogramming of innate immune cells, which is evoked by exogenous or endogenous stimuli and which leads to a usually enhanced response toward a second challenge after return to a non-activated state. Inflammaging: chronic low-grade inflammation that occurs with aging and is characterized by dysregulated inflammatory responses, in general resulting in increased inflammatory responses and diminished responses to pathogens like influenza or SARS-CoV-2.
Diet
In affluent countries, chronic overeating of diets comprising a high proportion of ultra-processed energy-dense and nutrient-poor foods and drinks induces systemic low-grade inflammation, also referred to as “metaflammation” (52). These Western diet-components are thought to induce so-called “trained innate immunity,” i.e., the functional reprogramming of innate immune cells by exogenous or endogenous stimuli. Training leads to a more vigorous response toward a second activating challenge, and this condition may be retained for a long period. This innate memory gives rise to an elevated inflammatory set point of innate immune cells, which is mediated through alterations in their progenitor cells (53). Exposure to adverse metabolic stimuli induces epigenetic changes during early development of these immature innate immune cells, which facilitate the expression of inflammatory genes. The mechanisms explaining inappropriate adaptive immune activation against pathogens in the presence of enhanced innate-driven inflammation are far from clear at present. They might include immune suppression by activated myeloid cells or regulatory T- and B-cells as well as inappropriate skewing of adaptive responses (54).
The enhanced inflammatory set point in obesity is reminiscent of changes occurring during aging, which is also characterized by increase of inflammatory parameters and designated as “inflammaging” (55). In fact, alterations in DNA methylation in blood cells and other tissues can be used as epigenetic marker of biological age, the ‘epigenetic clock' (56). Lifestyle-related risk factors, including obesity and smoking, accelerate epigenetic aging (55). Moreover, obesity may promote thymic involution, leading to diminished output of naïve T-lymphocytes, which accelerates immunosenescence (57).
Dampening inflammation by adequate intake of fruit and vegetables is essential for prevention of chronic metabolic diseases, and sufficient consumption thereof is reflected by inhibition of epigenetic aging (55). Many fruits and vegetables, e.g., tomatoes, garlic, berries, apples, broccoli, grapes and olives, have anti-inflammatory effects (58). A number of vitamins (A, B6, B12, folate, C, D, and E) and trace elements (zinc, copper, selenium, iron) have been demonstrated to play important and complementary roles in supporting both the innate and adaptive immune systems (59). Foods such as nuts, meat, eggs, shellfish, and wholegrains are good sources of many of these trace elements. In addition, omega-3 fatty acids derived from fatty fish have anti-inflammatory action (59). There is evidence that omega-3 fatty acids can control the cytokine storm in acute respiratory distress syndrome (ARDS) (60). Such a diet is consistent with current healthy eating guidelines (60).
Foods containing probiotic and prebiotic substances can maintain a healthy microbiota, which can also benefit the immune system (60). The gut microbiota plays a role in educating and regulating the immune system. Gut dysbiosis is a feature of obesity, chronic disease and many infectious diseases, and has been described in COVID-19. Dietary approaches to achieve a healthy microbiota can also benefit the immune system. Indeed, optimal nutrient intake promotes optimal immune function and may limit the impact of novel, more virulent pathogenic viruses (59). It must be emphasized that severe caloric restriction, as well as malnutrition, reduces immunity and renders one more susceptible for disease (48, 49). Extreme and prolonged reduction of caloric intake or crash diets are therefore strongly discouraged.
Experimental evidence of the effects of diet in relation to COVID-19 is still lacking. However, it is generally accepted that a diet rich in vegetables, fruit, nuts, legumes, fish, and ‘healthy' dietary fats is associated with a lower risk of chronic non-communicable diseases as well as improved immune function (61). A range of nutrients or bioactive compounds has been proposed to explain this association. Examples are polyphenols, vitamin D, n-3 PUFA in autoimmune and inflammatory disorders, and vitamin D, vitamin E, zinc, and probiotics in reduction of infection (62). A balanced whole foods version of a vegetarian or Mediterranean diet fits this description. Conversely, a diet that is rich in energy-dense, nutrient-poor foods (i.e., consisting of ultra-processed foods with high levels of refined carbohydrates, added sugar and fats) is related to an increased risk of non-communicable diseases and impaired immune function (63). Moreover, a recent study found that ultra-processed food stimulated additional intake of approximately 500 kcal/day when compared to content-matched unprocessed diet (64).
Physical Activity
Similar to the Western diet, a sedentary lifestyle is associated with abdominal adiposity, a pro-inflammatory state and increased risk of infection (65). Besides facilitating weight loss, regular physical activity decreases the activation of the immune system and improves immunosurveillance and immunocompetence (65). Regular exercise may limit or delay inflammaging (66). Subsequently, a physically active lifestyle reduces the risk of contracting a range of communicable diseases including viral and bacterial infections (65, 66).
The near-daily practice of moderate intensity exercise (e.g., walking or cycling) reduces upper respiratory tract infection symptom days by 40–50% (67). There is a dose-response relationship between the intensity of regular exercise and the risk for infection (65). Exercise intensity is commonly expressed as the Metabolic Equivalent of Task (MET) which is the ratio of a person's working metabolic rate relatively to their resting metabolic rate. Light to moderate exercise (MET 2–5.9, e.g., walking, vacuum cleaning or yoga) is better than inactivity (MET <2, e.g., writing or desk work) but vigorous exercise (MET 6–8.7, e.g., running, basketball or swimming) is even better. On similar note, short periods of maximum intensity training (High-intensity interval training or HIIT) seem to be a valuable addition to moderate-intensity continuous training in terms of enhancing cardiorespiratory fitness, metabolic hemostasis, vascular function and immune system function (68, 69). However, prolonged and intensive or (near) maximal intensity exercise is associated with an increased illness risk (67).
Substantial reduction in acute respiratory infection illness is detectable as soon as after 8 weeks of moderate intensity exercise (70). Most studies used a moderate intensity program of 30–45 min/day for 5 days/ week (67). This is in line with the Global recommendations on Physical Activity for Health on which most national guidelines are based (71). Thus, adoption of a physically active lifestyle by a substantial percentage of the population would probably curtail infectious pandemics such as COVID-19 and non-infectious pandemics such as obesity (65).
Stress
Different emotional states, or exposure to psychological stressors, are associated with enhanced susceptibility to, or increased severity of disease through nervous system-induced alterations in innate and adaptive immunity (72). A main component of the stress response is cortisol, which production and secretion are regulated by the hypothalamus-pituitary-adrenal axis (73). Cortisol has many functions in the human body, such as mediating the stress response, and regulating metabolism as well as, inflammatory-, and immune function (73). Cortisol is ordinarily anti-inflammatory and confines the immune response, but chronic hypercortisolemia can lead to resistance of the immune system. The accumulation of stress hormones gives rise to an increased production of inflammatory cytokines that compromises the immune response (74). It has been demonstrated that individuals reporting higher levels of stress are more likely to develop clinical symptoms during experimental respiratory viral infection (72). Behavioral interventions targeted at alleviating stress, promoting heightened states of relaxation and encouraging moderate physical activity have been shown to bolster anti-viral immune responses and decrease markers of inflammation (75). A meta-analysis focusing on psychological interventions to alleviate stress showed positive effects on the immune system. It even suggested a positive relation between the frequency of self-reported of stress-reducing practice and a more adequate functioning of the immune system (76). Besides the impact on inflammatory markers, stress also promotes other negative lifestyle factors such as unhealthy eating and poor sleeping quality (77).
Smoking
Nicotine and other tobacco components have several pro- as well as anti-inflammatory effects, and thus contribute to an altered immune set point in smokers (78). Current or former smoking is a risk factor for severe disease in patients with COVID-19. Smokers appear to have a 40% higher chance of developing severe symptoms, and a 140% higher chance of being admitted to an ICU, need mechanical ventilation or die as compared to non-smokers (79). A similar observation was made in patients with influenza (80). Smokers were over 5 times more likely to develop laboratory-confirmed influenza, and 34% more likely to develop influenza-like illness than non-smokers. Importantly, smoking cessation recovers airway ciliary clearance and immune function as early as 1 month after the intervention (81). Thus, smoking cessation is strongly encouraged as a public health measure to limit the global impact of COVID-19 (81).
Alcohol
Alcohol consumption affects the number, survival and function of both innate and adaptive immune cells, thereby interfering with immune responses (82). Consumption of high doses can directly suppress a wide range of immune responses, and alcohol abuse is associated with an increased incidence of infectious disease (83). For instance, alcoholics are highly susceptible to respiratory pathogens and lung injury, and have a 2–4 fold increased risk of ARDS (84). Furthermore, alcohol consumption increases the risk of community-acquired pneumonia in adults (85). A dose-response relationship is observed, indicating that the risk for pneumonia increases by 8% for every 10–20 g (one unit of alcohol) higher daily alcohol intake. Surprisingly, even a single episode of binge drinking can have measurable effects on the immune system, inducing a transient pro-inflammatory state within the first 20 min after alcohol ingestion, followed by an anti-inflammatory state 2–5 h after alcohol ingestion (82). Also in animal models of pulmonary infections, alcohol administration is associated with adverse clinical parameters and increased lung damage (82). In many cultures, drinking alcohol is socially accepted and integrated in daily life. Increased awareness of the negative effects of alcohol and making moderation or abstinence the default choice are of paramount importance for public health.
Sleep
It is suggested that both circadian rhythm and sleep significantly modify the susceptibility to SARS-CoV-2 infection as well as the overall clinical manifestations of COVID-19 (86). This relates to an interdependent balance maintained between the circadian clock, the intestinal microbiota and immune system activity (87). Naturally occurring sleep acutely enhances the expression of cytokines such as IL-12 and IFN-γ that regulate anti-viral immune defense mechanisms (88). Also, sleep might improve immunological memory formation by boosting the number of antigen presenting cells and CD4+ T-cells (89). The central nervous system seems to use the metabolically quiescent period of sleep to regulate both innate and antiviral immune responses (88). Sleep deprivation leads to decreased lymphocyte blastogenesis and natural killer cell activity, and upregulates IL-1 and IL-2 (90). However, sleep also increases IL-2 production by T-cells (88). Short habitual sleep (<6 h) is associated in humans with reduced life span, increased vulnerability to viral infection and reduced antibody titers after vaccination (91). In addition, short-term sleep deprivation prior to vaccination appears to negatively impact antibody titers after influenza vaccination (92). In rodents, sleep disturbances reduces influenza vaccine efficacy (93). When attempting to improve on sleep quality to boost the immune system, paucity of evidence prevents strong recommendations. However, a key point is that in current times circadian rhythm and sleep disruption frequently occur through shift work, nighttime light exposure and social jet lag (91). Morin et al. provide a condense overview of recommendations to improve sleep quality, especially during the COVID-19 pandemic (Table 2) (94). Further research could explore the effects of these recommendations on immunity and susceptibility to infections.
An Upstream Environmental Approach Toward Healthier Lifestyles is Needed
Thus, we know that obesity, chronic disease and their underlying causes impair immune function and leave individuals more vulnerable to infection. These underlying causes include behavioral and modifiable factors such as an unhealthy diet, inadequate physical activity, chronic stress, use of alcohol and tobacco and suboptimal sleep quality (Table 3). To improve on all these aspects of health, multiple methods can be used. We make a case for health education, choice architecture, and regulation on local, national, and international levels.
Undeniably, widespread health education is an important aspect of reaching these lifestyle goals. This challenge is a shared responsibility of healthcare professionals, governments, the private sector, and civil society. Healthcare organizations and professionals should advocate the use of lifestyle as therapy in its own right, of equal importance to medication and interventions. Lifestyle modification should be an integral part of chronic disease management. National and local policies should address social determinants of health and health inequalities (95).
Extra attention should be given to personal health and lifestyle factors in periods of complete or partial lockdown. Lockdown periods lead to increases in sedentary behavior (unhealthy) food consumption, alcohol consumption, tobacco use, stress, and decreased well-being (96–98). Distressingly, increased food consumption and snacking are more prevalent in people with overweight or obesity. Even 2 weeks of physical inactivity and a positive energy balance can lead to reduced insulin sensitivity, higher total body fat, and a proinflammatory state (99). People should be aware of the influence of lockdown on their daily habits and be guided to eat healthy, exercise adequately, and find help for psychosocial adversities. We conclude that advocating home-confinement should be accompanied by stressing the need to maintain a healthy lifestyle.
Besides health education, we need to realize that our environment influences our choices substantially. Choices are made by means of two cognitive processes: the reflective and the impulsive system. The former is rational and conscious, the latter is quick, automatic, and subconscious (100). In the context of the current environment, we make approximately 219 decisions in regard to food per day, of which 90% subconsciously (101). Default options such as easy availability of unhealthy foods significantly influence our choices in a negative way (102). Thus, in order to promote healthy choices we need to create an environment in which the default option is a healthy option.
In many parts of the world people currently live in an obesogenic environment (103). In recent decades most societies increasingly offer an overabundance of cheap and convenient energy-dense and nutrient-poor foods and drinks, which contribute to adverse dietary choices. Intensive marketing of ultra-processed foods only makes matters worse. Moreover, many of the current environments promote sedentary behavior and discourage physical activity (104). As a society, we need to promote a healthy lifestyle, and thereby decrease rates of obesity and improve immune function. To do so, we need to reconstruct our living environment so as to facilitate and promote healthy dietary choices, physical activity, stress relieve, and regular sleep (105). Changing choice architecture can be done by changing food positioning in supermarkets, schools, or workplaces (106, 107). Another example is using built environment to increase physical activity and active transport (108).
In addition to choice architecture, creating healthy communities requires regulation by governments aimed at protecting society from the commercial determinants of chronic non-communicable diseases (109). This includes regulating the sale and promotion of unhealthy commodities such as tobacco, alcohol, and ultra-processed food and drinks (109). Strong leadership is essential to resist attempts by powerful organizations with vested interests (e.g., the tobacco, food, and alcohol industries) to undermine the development and implementation of effective policies and laws (110). Evidence-based approaches such as legislation, regulation, taxation, pricing, ban, and restriction of advertising and sponsorship should be introduced to reduce consumption of unhealthy commodities (109). All in all, the campaign for healthier lifestyle includes, but is not limited to, aspects on health education, environmental changes, and regulatory actions (Table 4).
Conclusions
COVID-19 has significant impact on public health. Obesity, chronic disease, and various lifestyle components increase the severity of the disease course, the appeal to health care, and the mortality rate of SARS-CoV-2 infections. The harmful impact of these factors on the immune system probably explains the worse outcome to a large extent. Conditions associated with low-grade systemic inflammation, such as obesity, metabolic syndrome, and old age, hamper an optimal and timely response by the immune system to an infectious challenge like SARS-CoV-2. Several Western diet components train innate immune cells so as to adopt a more pro-inflammatory set point, which enigmatically diminishes their capacity to activate adequate adaptive immunity required for an effective battle against pathogens.
Adapting lifestyle on a global scale can most likely enhance population resistance to viral and other infections. However, making healthy choices is not as easy as knowing what to choose. In this context it is important to be aware of the existing obesity stigma and to counteract it. Obesity and related cardiometabolic diseases are not only a problem of the individual, but a burden for society as well. These metabolic disorders are primarily caused by environmental and societal factors, in concert with genetic and other individual (biological) characteristics. Reconstruction of our living environment so as to promote healthier lifestyles is of paramount importance to both prevent and treat obesity and related disorders. Obviously, a collective effort is required to get this done. Healthcare institutions, governments, the private sector, and (creative) citizens have to put their heads together to map out the route to a healthy future. Together we can change our lifestyle-choice architecture to make healthy living the norm, and thereby improve immune function to be better prepared for the next viral pandemic.
Author Contributions
DF wrote and performed the literature searches, analyzed the results, selected relevant articles for this manuscript, and drafted the concept version of the manuscript. ER took the lead in supervising the creation process, provided content related to obesity, and gave feedback on every draft. DA, PL, WD, HP, and JS provided feedback during the creation process. Specifically on their fields of expertise but also on the manuscript as a whole. WD and PL designed both figures adopted in the manuscript. All authors provided them with feedback. All in all, DA, PL, WD, HP, and JS contributed roughly equally to the formation of the manuscript.
Conflict of Interest
The authors declare that the research was conducted in the absence of any commercial or financial relationships that could be construed as a potential conflict of interest.
Supplementary Material
The Supplementary Material for this article can be found online at: https://www.frontiersin.org/articles/10.3389/fnut.2020.597600/full#supplementary-material
References
1. Harapan H, Itoh N, Yufika A, Winardi W, Keam S, Te H, et al. Coronavirus disease 2019 (COVID-19): a literature review. J Infect Public Health. (2020) 13:667–73. doi: 10.1016/j.jiph.2020.03.019
2. Worldometers.info. Worldometer Coronavirus Dover, Delaware, U.S.A. (2020). Available online at: https://www.worldometers.info/coronavirus/ (accessed August 20, 2020).
3. Kushner RF, Sorensen KW. Lifestyle medicine: the future of chronic disease management. Curr Opin Endocrinol Diabetes Obes. (2013) 20:389–95. doi: 10.1097/01.med.0000433056.76699.5d
4. Wu Z, McGoogan JM. Characteristics of and important lessons from the coronavirus disease 2019 (COVID-19) outbreak in China: summary of a report of 72 314 cases from the Chinese Center for Disease Control and Prevention. JAMA. (2020) 323:1239–42. doi: 10.1001/jama.2020.2648
5. Garg S, Kim L, Whitaker M, O'Halloran A, Cummings C, Holstein R, et al. Hospitalization rates and characteristics of patients hospitalized with laboratory-confirmed coronavirus disease 2019. COVID-NET, 14 states. Morb Mortal Wkly Rep. (2020) 69:458–64. doi: 10.15585/mmwr.mm6915e3
6. Petrilli CM, Jones SA, Yang J, Rajagopalan H, O'Donnell LF, Chernyak Y, et al. Factors associated with hospitalization and critical illness among 4,103 patients with COVID-19 disease in New York City. BMJ. (2020) 369:m1966. doi: 10.1136/bmj.m1966
7. Gao F, Zheng KI, Wang XB, Sun QF, Pan KH, Wang TY, et al. Obesity is a risk factor for greater COVID-19 severity. Diabetes Care. (2020) 43:e72–4. doi: 10.2337/dc20-0682
8. Petrakis D, Margină D, Tsarouhas K, Tekos F, Stan M, Nikitovic D, et al. Obesity—a risk factor for increased COVID-19 prevalence, severity and lethality (Review). Mol Med Rep. (2020) 22:9–19. doi: 10.3892/mmr.2020.11127
9. Lighter J, Phillips M, Hochman S, Sterling S, Johnson D, Francois F, et al. Obesity in patients younger than 60 years is a risk factor for COVID-19 hospital admission. Clin Infect Dis. (2020) 71:896–7. doi: 10.1093/cid/ciaa415
10. Tsatsanis C, Margioris AN, Kontoyiannis DP. Association between H1N1 infection severity and obesity-adiponectin as a potential etiologic factor. J Infect Dis. (2010) 202:459–60. doi: 10.1086/653842
11. Ryan PM, Caplice NM. Is adipose tissue a reservoir for viral spread, immune activation, and cytokine amplification in coronavirus disease 2019? Obesity (Silver Spring, Md). (2020) 28:1191–4. doi: 10.1002/oby.22843
12. Yatim KM, Lakkis FG. A brief journey through the immune system. Clin J Am Soc Nephrol. (2015) 10:1274–81. doi: 10.2215/CJN.10031014
13. Kane H, Lynch L. Innate immune control of adipose tissue homeostasis. Trends Immunol. (2019) 40:857–72. doi: 10.1016/j.it.2019.07.006
14. Zhao R, Tang D, Yi S, Li W, Wu C, Lu Y, et al. Elevated peripheral frequencies of Th22 cells: a novel potent participant in obesity and type 2 diabetes. PLoS ONE. (2014) 9:e85770. doi: 10.1371/journal.pone.0085770
15. Touch S, Clément K, André S. T cell populations and functions are altered in human obesity and type 2 diabetes. Curr Diab Rep. (2017) 17:81. doi: 10.1007/s11892-017-0900-5
16. Terán-Cabanillas E, Hernández J. Role of leptin and SOCS3 in inhibiting the type i interferon response during obesity. Inflammation. (2017) 40:58–67. doi: 10.1007/s10753-016-0452-x
17. Wilk AJ, Rustagi A, Zhao NQ, Roque J, Martínez-Colón GJ, McKechnie JL, et al. A single-cell atlas of the peripheral immune response in patients with severe COVID-19. Nat Med. (2020) 26:1070–6. doi: 10.1038/s41591-020-0944-y
18. Brufsky A, Lotze MT. DC/L-SIGNs of hope in the COVID-19 pandemic. J Med Virol. (2020) 92:1396–8. doi: 10.1002/jmv.25980
19. Zheng H-Y, Zhang M, Yang C-X, Zhang N, Wang X-C, Yang X-P, et al. Elevated exhaustion levels and reduced functional diversity of T cells in peripheral blood may predict severe progression in COVID-19 patients. Cell Mol Immunol. (2020) 17:541–3. doi: 10.1038/s41423-020-0401-3
20. O'Shea D, Corrigan M, Dunne MR, Jackson R, Woods C, Gaoatswe G, et al. Changes in human dendritic cell number and function in severe obesity may contribute to increased susceptibility to viral infection. Int J Obes (Lond). (2013) 37:1510–3. doi: 10.1038/ijo.2013.16
21. de Heredia FP, Gómez-Martínez S, Marcos A. Obesity, inflammation and the immune system. Proc Nutr Soc. (2012) 71:332–8. doi: 10.1017/S0029665112000092
22. Larabee CM, Neely OC, Domingos AI. Obesity: a neuroimmunometabolic perspective. Nat Rev Endocrinol. (2020) 16:30–43. doi: 10.1038/s41574-019-0283-6
23. Dixon AE, Peters U. The effect of obesity on lung function. Expert Rev Respir Med. (2018) 12:755–67. doi: 10.1080/17476348.2018.1506331
24. Darvall KA, Sam RC, Silverman SH, Bradbury AW, Adam DJ. Obesity and thrombosis. Eur J Vasc Endovasc Surg. (2007) 33:223–33. doi: 10.1016/j.ejvs.2006.10.006
25. Pineton de Chambrun M, Frere C, Miyara M, Amoura Z, Martin-Toutain I, Mathian A, et al. High frequency of antiphospholipid antibodies in critically-ill COVID-19 patients: a link with hypercoagulability? J Intern Med. (2020). doi: 10.1111/joim.13126. [Epub ahead of print].
26. Andersen CJ, Murphy KE, Fernandez ML. Impact of obesity and metabolic syndrome on immunity. Adv Nutr. (2016) 7:66–75. doi: 10.3945/an.115.010207
27. Yan Y, Yang Y, Wang F, Ren H, Zhang S, Shi X, et al. Clinical characteristics and outcomes of patients with severe COVID-19 with diabetes. BMJ Open Diabetes Res Care. (2020) 8:e001343. doi: 10.1136/bmjdrc-2020-001343
28. Targher G, Mantovani A, Wang XB, Yan HD, Sun QF, Pan KH, et al. Patients with diabetes are at higher risk for severe illness from COVID-19. Diabetes Metab. (2020) 46:335–7. doi: 10.1016/j.diabet.2020.05.001
29. Roncon L, Zuin M, Rigatelli G, Zuliani G. Diabetic patients with COVID-19 infection are at higher risk of ICU admission and poor short-term outcome. J Clin Virol. (2020) 127:104354. doi: 10.1016/j.jcv.2020.104354
30. Cook TM. The importance of hypertension as a risk factor for severe illness and mortality in COVID-19. Anaesthesia. (2020) 75:976–7. doi: 10.1111/anae.15103
31. Li G, Li H, Lu J. No adequate evidence indicating hypertension as an independent risk factor for COVID-19 severity. Clin Res Cardiol. (2020) 109:1–2. doi: 10.1007/s00392-020-01653-6
32. Norlander AE, Madhur MS, Harrison DG. The immunology of hypertension. J Exp Med. (2018) 215:21–33. doi: 10.1084/jem.20171773
33. Tufik S, Gozal D, Ishikura IA, Pires GN, Andersen ML. Does obstructive sleep apnea lead to increased risk of COVID-19 infection and severity? J Clin Sleep Med. (2020) 16:1425–6. doi: 10.5664/jcsm.8596
34. Scheen AJ, Marre M, Thivolet C. Prognostic factors in patients with diabetes hospitalized for COVID-19: Findings from the CORONADO study and other recent reports. Diabetes Metab. (2020) 46:265–71. doi: 10.1016/j.diabet.2020.05.008
35. Memtsoudis SG, Ivascu NS, Pryor KO, Goldstein PA. Obesity as a risk factor for poor outcome in COVID-19-induced lung injury: the potential role of undiagnosed obstructive sleep apnoea. Br J Anaesth. (2020) 125:e262–3. doi: 10.1016/j.bja.2020.04.078
36. Tham KW, Lee PC, Lim CH. Weight management in obstructive sleep apnea: medical and surgical options. Sleep Med Clin. (2019) 14:143–53. doi: 10.1016/j.jsmc.2018.10.002
37. van der Valk ES, van den Akker ELT, Savas M, Kleinendorst L, Visser JA, Van Haelst MM, et al. A comprehensive diagnostic approach to detect underlying causes of obesity in adults. Obes Rev. (2019) 20:795–804. doi: 10.1111/obr.12836
38. Tomiyama AJ, Carr D, Granberg EM, Major B, Robinson E, Sutin AR, et al. How and why weight stigma drives the obesity “epidemic” and harms health. BMC Med. (2018) 16:123. doi: 10.1186/s12916-018-1116-5
39. Alberga AS, Russell-Mayhew S, von Ranson KM, McLaren L. Weight bias: a call to action. Journal of eating disorders. (2016) 4:34. doi: 10.1186/s40337-016-0112-4
40. Kahan S, Manson JE. Obesity treatment, beyond the guidelines: practical suggestions for clinical practice. JAMA. (2019) 321:1349–50. doi: 10.1001/jama.2019.2352
41. Yumuk V, Tsigos C, Fried M, Schindler K, Busetto L, Micic D, et al. European guidelines for obesity management in adults. Obes Facts. (2015) 8:402–24. doi: 10.1159/000442721
42. Apovian CM, Aronne LJ, Bessesen DH, McDonnell ME, Murad MH, Pagotto U, et al. Pharmacological management of obesity: an endocrine society clinical practice guideline. J Clin Endocrinol Metabol. (2015) 100:342–62. doi: 10.1210/jc.2014-3415
43. Jongbloed F, Meijers RWJ, IJzermans JNM, Klaassen RA, Dollé MET, van den Berg S, et al. Effects of bariatric surgery on telomere length and T-cell aging. Int J Obes (Lond). (2019) 43:2189–99. doi: 10.1038/s41366-019-0351-y
44. Moulin CM, Rizzo LV, Halpern A. Effect of surgery-induced weight loss on immune function. Expert Rev Gastroenterol Hepatol. (2008) 2:617–9. doi: 10.1586/17474124.2.5.617
45. Jing Y, Wu F, Li D, Yang L, Li Q, Li R. Metformin improves obesity-associated inflammation by altering macrophages polarization. Mol Cell Endocrinol. (2018) 461:256–64. doi: 10.1016/j.mce.2017.09.025
46. Drucker DJ. The cardiovascular biology of glucagon-like peptide-1. Cell Metab. (2016) 24:15–30. doi: 10.1016/j.cmet.2016.06.009
47. van der Zalm IJB, van der Valk ES, Wester VL, Nagtzaam NMA, van Rossum EFC, Leenen PJM, et al. Obesity-associated T-cell and macrophage activation improve partly after a lifestyle intervention. Int J Obes (Lond). (2020) 44:1838–50. doi: 10.1038/s41366-020-0615-6
48. Arthur SS, Nyide B, Soura AB, Kahn K, Weston M, Sankoh O. Tackling malnutrition: a systematic review of 15-year research evidence from INDEPTH health and demographic surveillance systems. Glob Health Action. (2015) 8:28298. doi: 10.3402/gha.v8.28298
49. Collins N. Dietary regulation of memory T cells. Int J Mol Sci. (2020) 21(12). doi: 10.3390/ijms21124363
50. Ahmad S, Fatima SS, Rukh G, Smith CE. Gene Lifestyle interactions with relation to obesity, cardiometabolic, and cardiovascular traits among South Asians. Front Endocrinol (Lausanne). (2019) 10:221. doi: 10.3389/fendo.2019.00221
51. Gupta SC, Kunnumakkara AB, Aggarwal S, Aggarwal BB. Inflammation, a double-edge sword for cancer and other age-related diseases. Front Immunol. (2018) 9:2160. doi: 10.3389/fimmu.2018.02160
52. Christ A, Lauterbach M, Latz E. Western diet and the immune system: an inflammatory connection. Immunity. (2019) 51:794–811. doi: 10.1016/j.immuni.2019.09.020
53. Bekkering S, Saner C, Riksen NP, Netea MG, Sabin MA, Saffery R, et al. trained immunity: linking obesity and cardiovascular disease across the life-course? Trends Endocrinol Metab. (2020) 31:378–89. doi: 10.1016/j.tem.2020.01.008
54. Salminen A. Activation of immunosuppressive network in the aging process. Ageing Res Rev. (2020) 57:100998. doi: 10.1016/j.arr.2019.100998
55. Levine ME, Lu AT, Quach A, Chen BH, Assimes TL, Bandinelli S, et al. An epigenetic biomarker of aging for lifespan and healthspan. Aging. (2018) 10:573–91. doi: 10.18632/aging.101414
56. Horvath S, Raj K. DNA methylation-based biomarkers and the epigenetic clock theory of ageing. Nat Rev Genet. (2018) 19:371–84. doi: 10.1038/s41576-018-0004-3
57. Yang H, Youm YH, Vandanmagsar B, Rood J, Kumar KG, Butler AA, et al. Obesity accelerates thymic aging. Blood. (2009) 114:3803–12. doi: 10.1182/blood-2009-03-213595
58. Lapuente M, Estruch R, Shahbaz M, Casas R. Relation of fruits and vegetables with major cardiometabolic risk factors, markers of oxidation, and inflammation. Nutrients. (2019) 11:2381. doi: 10.3390/nu11102381
59. Calder PC, Carr AC, Gombart AF, Eggersdorfer M. Optimal nutritional status for a well-functioning immune system is an important factor to protect against viral infections. Nutrients. (2020) 12(4). doi: 10.3390/nu12041181
61. Childs CE, Calder PC, Miles EA. Diet and immune function. Nutrients. (2019) 11(8). doi: 10.3390/nu11081933
62. Wu D, Lewis ED, Pae M, Meydani SN. Nutritional modulation of immune function: analysis of evidence, mechanisms, and clinical relevance. Front Immunol. (2018) 9:3160. doi: 10.3389/fimmu.2018.03160
63. Myles IA. Fast food fever: reviewing the impacts of the Western diet on immunity. Nutr J. (2014) 13:61. doi: 10.1186/1475-2891-13-61
64. Hall KD, Ayuketah A, Brychta R, Cai H, Cassimatis T, Chen KY, et al. Ultra-processed diets cause excess calorie intake and weight gain: an inpatient randomized controlled trial of ad libitum food intake. Cell Metab. (2019) 30:67–77.e3. doi: 10.1016/j.cmet.2019.05.008
65. da Luz Scheffer D, Latini A. Exercise-induced immune system response: anti-inflammatory status on peripheral and central organs. Biochim Biophys Acta. (2020) 1866:165823. doi: 10.1016/j.bbadis.2020.165823
66. Campbell JP, Turner JE. Debunking the Myth of exercise-induced immune suppression: redefining the impact of exercise on immunological health across the lifespan. Front Immunol. (2018) 9:648. doi: 10.3389/fimmu.2018.00648
67. Nieman DC, Wentz LM. The compelling link between physical activity and the body's defense system. J Sport Health Sci. (2019) 8:201–17. doi: 10.1016/j.jshs.2018.09.009
68. Wang M, Baker JS, Quan W, Shen S, Fekete G, Gu Y. A preventive role of exercise across the coronavirus 2 (SARS-CoV-2) pandemic. Front Physiol. (2020) 11:572718. doi: 10.3389/fphys.2020.572718
69. de Matos MA, Garcia BCC, Vieira DV, de Oliveira MFA, Costa KB, Aguiar PF, et al. High-intensity interval training reduces monocyte activation in obese adults. Brain Behav Immun. (2019) 80:818–24. doi: 10.1016/j.bbi.2019.05.030
70. Barrett B, Hayney MS, Muller D, Rakel D, Ward A, Obasi CN, et al. Meditation or exercise for preventing acute respiratory infection: a randomized controlled trial. Ann Fam Med. (2012) 10:337–46. doi: 10.1370/afm.1376
72. Bailey MT. psychological stress, immunity, and the effects on indigenous microflora. Adv Exp Med Biol. (2016) 874:225–46. doi: 10.1007/978-3-319-20215-0_11
73. Thau L, Jayashree G, Sharma S. Physiology, Cortisol. Treasure Island, FL: StatPearls Publishing (2020). Available online at: https://www.ncbi.nlm.nih.gov/books/NBK538239/ (accessed June 12, 2020).
74. Morey JN, Boggero IA, Scott AB, Segerstrom SC. Current directions in stress and human immune function. Curr Opin Psychol. (2015) 5:13–7. doi: 10.1016/j.copsyc.2015.03.007
75. Morgan N, Irwin MR, Chung M, Wang C. The effects of mind-body therapies on the immune system: meta-analysis. PLoS ONE. (2014) 9:e100903. doi: 10.1371/journal.pone.0100903
76. Schakel L, Veldhuijzen DS, Crompvoets PI, Bosch JA, Cohen S, van Middendorp H, et al. Effectiveness of stress-reducing interventions on the response to challenges to the immune system: a meta-analytic review. Psychother Psychosom. (2019) 88:274–86. doi: 10.1159/000501645
77. Ingram J, Maciejewski G, Hand CJ. Changes in diet, sleep, and physical activity are associated with differences in negative mood during COVID-19 lockdown. Front Psychol. (2020) 11:588604. doi: 10.3389/fpsyg.2020.588604
78. Qiu F, Liang CL, Liu H, Zeng YQ, Hou S, Huang S, et al. Impacts of cigarette smoking on immune responsiveness: Up and down or upside down? Oncotarget. (2017) 8:268–84. doi: 10.18632/oncotarget.13613
79. Vardavas CI, Nikitara K. COVID-19 and smoking: a systematic review of the evidence. Tob Induc Dis. (2020) 18:20. doi: 10.18332/tid/119324
80. Lawrence H, Hunter A, Murray R, Lim WS, McKeever T. Cigarette smoking and the occurrence of influenza—systematic review. J Infect. (2019) 79:401–6. doi: 10.1016/j.jinf.2019.08.014
81. Komiyama M, Hasegawa K. Smoking cessation as a public health measure to limit the coronavirus disease 2019 pandemic. Eur Cardiol. (2020) 15:e16. doi: 10.15420/ecr.2020.11
83. Romeo J, Wärnberg J, Nova E, Díaz LE, Gómez-Martinez S, Marcos A. Moderate alcohol consumption and the immune system: a review. Br J Nutr. (2007) 98(Suppl. 1):S111–5. doi: 10.1017/S0007114507838049
84. Yeligar SM, Chen MM, Kovacs EJ, Sisson JH, Burnham EL, Brown LA. Alcohol and lung injury and immunity. Alcohol. (2016) 55:51–9. doi: 10.1016/j.alcohol.2016.08.005
85. Simou E, Britton J, Leonardi-Bee J. Alcohol and the risk of pneumonia: a systematic review and meta-analysis. BMJ Open. (2018) 8:e022344. doi: 10.1136/bmjopen-2018-022344
86. Meira ECM, Miyazawa M, Gozal D. Putative contributions of circadian clock and sleep in the context of SARS-CoV-2 infection. Eur Respir J. (2020) 55:2001023. doi: 10.1183/13993003.01023-2020
87. Zheng D, Ratiner K, Elinav E. Circadian influences of diet on the microbiome and immunity. Trends Immunol. (2020) 41:512–30. doi: 10.1016/j.it.2020.04.005
88. Irwin MR. Sleep and inflammation: partners in sickness and in health. Nat Rev Immunol. (2019) 19:702–15. doi: 10.1038/s41577-019-0190-z
89. Lange T, Born J, Westermann J. Sleep matters: CD4(+) T cell memory formation and the central nervous system. Trends Immunol. (2019) 40:674–86. doi: 10.1016/j.it.2019.06.003
90. Asif N, Iqbal R, Nazir CF. Human immune system during sleep. Am J Clin Exp Immunol. (2017) 6:92–6.
91. Haspel JA, Anafi R, Brown MK, Cermakian N, Depner C, Desplats P, et al. Perfect timing: circadian rhythms, sleep, and immunity—an NIH workshop summary. JCI Insight. (2020) 5(1). doi: 10.1172/jci.insight.131487
92. Benedict C, Brytting M, Markström A, Broman JE, Schiöth HB. Acute sleep deprivation has no lasting effects on the human antibody titer response following a novel influenza A H1N1 virus vaccination. BMC Immunol. (2012) 13:1. doi: 10.1186/1471-2172-13-1
93. Brown R, Pang G, Husband AJ, King MG. Suppression of immunity to influenza virus infection in the respiratory tract following sleep disturbance. Reg Immunol. (1989) 2:321–5.
94. Morin CM, Carrier J, Bastien C, Godbout R. Sleep and circadian rhythm in response to the COVID-19 pandemic. Can J Public Health. (2020) 111:654–7. doi: 10.17269/s41997-020-00382-7
95. Marmot M. Social determinants of health inequalities. Lancet. (2005) 365:1099–104. doi: 10.1016/S0140-6736(05)71146-6
96. Sidor A, Rzymski P. Dietary choices and habits during COVID-19 lockdown: experience from Poland. Nutrients. (2020) 12:1657. doi: 10.3390/nu12061657
97. Rolland B, Haesebaert F, Zante E, Benyamina A, Haesebaert J, Franck N. Global changes and factors of increase in caloric/salty food intake, screen use, and substance use during the early COVID-19 containment phase in the general population in France: survey study. JMIR Public Health Surveill. (2020) 6:e19630. doi: 10.2196/19630
98. Ruíz-Roso MB, de Carvalho Padilha P, Matilla-Escalante DC, Brun P, Ulloa N, Acevedo-Correa D, et al. Changes of physical activity and ultra-processed food consumption in adolescents from different countries during COVID-19 pandemic: an observational study. Nutrients. (2020) 12:2289. doi: 10.3390/nu12082289
99. Martinez-Ferran M, de la Guía-Galipienso F, Sanchis-Gomar F, Pareja-Galeano H. Metabolic impacts of confinement during the COVID-19 pandemic due to modified diet and physical activity habits. Nutrients. (2020) 12:1549. doi: 10.3390/nu12061549
101. Wansink B, Sobal J. Mindless eating: the 200 daily food decisions we overlook. Environ Behav. (2007) 39:106–23. doi: 10.1177/0013916506295573
102. Smith N, Goldstein D, Johnson E. Choice without awareness: ethical and policy implications of defaults. J Public Policy Mark. (2013) 32:159–72. doi: 10.1509/jppm.10.114
103. Jackson SE, Llewellyn CH, Smith L. The obesity epidemic—nature via nurture: a narrative review of high-income countries. SAGE Open Med. (2020) 8:2050312120918265. doi: 10.1177/2050312120918265
104. Guthold R, Stevens GA, Riley LM, Bull FC. Global trends in insufficient physical activity among adolescents: a pooled analysis of 298 population-based surveys with 1·6 million participants. Lancet Child Adolesc Health. (2020) 4:23–35. doi: 10.1016/S2352-4642(19)30323-2
105. WHO. Controlling the Global Obesity Epidemic. (2020). Available online at: https://www.who.int/nutrition/topics/obesity/en/ (accessed August 21, 2020).
106. Bucher T, Collins C, Rollo ME, McCaffrey TA, De Vlieger N, Van der Bend D, et al. Nudging consumers towards healthier choices: a systematic review of positional influences on food choice. Br J Nutr. (2016) 115:2252–63. doi: 10.1017/S0007114516001653
107. Broers VJV, De Breucker C, Van den Broucke S, Luminet O. A systematic review and meta-analysis of the effectiveness of nudging to increase fruit and vegetable choice. Eur J Public Health. (2017) 27:912–20. doi: 10.1093/eurpub/ckx085
108. Smith M, Hosking J, Woodward A, Witten K, MacMillan A, Field A, et al. Systematic literature review of built environment effects on physical activity and active transport—an update and new findings on health equity. Int J Behav Nutr Phys Act. (2017) 14:158. doi: 10.1186/s12966-017-0613-9
109. Moodie R, Stuckler D, Monteiro C, Sheron N, Neal B, Thamarangsi T, et al. Profits and pandemics: prevention of harmful effects of tobacco, alcohol, and ultra-processed food and drink industries. Lancet. (2013) 381:670–9. doi: 10.1016/S0140-6736(12)62089-3
Keywords: obesity, diet, COVID-19, lifestyle, immune system
Citation: de Frel DL, Atsma DE, Pijl H, Seidell JC, Leenen PJM, Dik WA and van Rossum EFC (2020) The Impact of Obesity and Lifestyle on the Immune System and Susceptibility to Infections Such as COVID-19. Front. Nutr. 7:597600. doi: 10.3389/fnut.2020.597600
Received: 21 August 2020; Accepted: 03 November 2020;
Published: 19 November 2020.
Edited by:
Ioannis Zabetakis, University of Limerick, IrelandReviewed by:
Evangeline Mantzioris, University of South Australia, AustraliaLiam O'Mahony, University College Cork, Ireland
Copyright © 2020 de Frel, Atsma, Pijl, Seidell, Leenen, Dik and van Rossum. This is an open-access article distributed under the terms of the Creative Commons Attribution License (CC BY). The use, distribution or reproduction in other forums is permitted, provided the original author(s) and the copyright owner(s) are credited and that the original publication in this journal is cited, in accordance with accepted academic practice. No use, distribution or reproduction is permitted which does not comply with these terms.
*Correspondence: Douwe E. Atsma, ZC5lLmF0c21hJiN4MDAwNDA7bHVtYy5ubA==