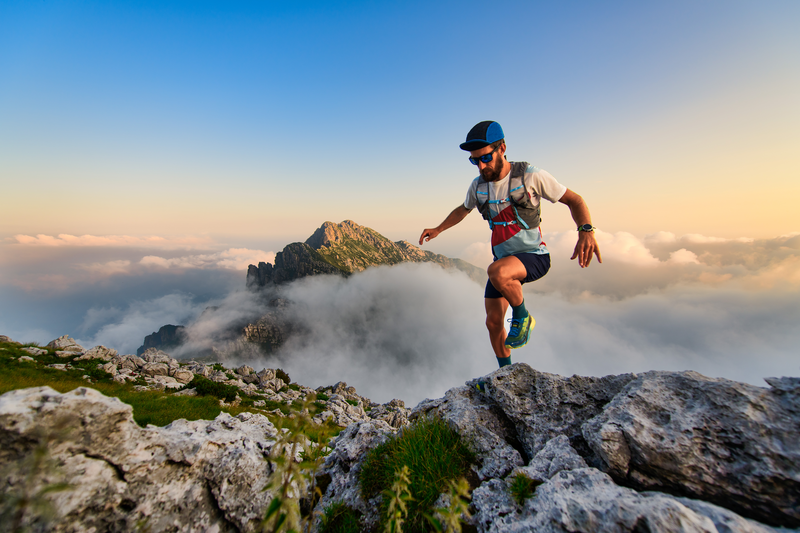
95% of researchers rate our articles as excellent or good
Learn more about the work of our research integrity team to safeguard the quality of each article we publish.
Find out more
ORIGINAL RESEARCH article
Front. Nutr. , 31 July 2020
Sec. Clinical Nutrition
Volume 7 - 2020 | https://doi.org/10.3389/fnut.2020.00115
This article is part of the Research Topic Ageing-Related Symptoms, Kampo Medicine and Treatment View all 16 articles
Shinkiku (Massa Medicata Fermentata) is a traditional crude drug used to treat anorexia and dyspepsia of elder patients in east Asia. Shinkiku is generally prepared by the microbial fermentation of wheat and herbs. Shinkiku is also used in Japanese Kampo medicine as a component of 半夏白朮天麻湯 (Hangebyakujutsutemmato). However, the quality of shinkiku varies by manufacture because there are no reference standards to control the quality of medicinal shinkiku. Thus, we aim to characterize the quality of various commercially available shinkiku by chemical and microbial analysis. We collected 13 shinkiku products manufactured in China and Korea and investigated the microbial structure and chemical constituents. Amplicon sequence analysis revealed that Aspergillus sp. was common microorganism in shinkiku products. Digestive enzymes (α-amylase, protease, and lipase), organic acids (ferulic acid, citric acid, lactic acid, and acetic acid), and 39 volatile compounds were commonly found in shinkiku products. Although there were some commonalities in shinkiku products, microbial and chemical characteristic considerably differed as per the manufacturer. Aspergillus sp. was predominant in Korean products, and Korean products showed higher enzyme activities than Chinese products. Meanwhile, Bacillus sp. was commonly detected in Chinese shinkiku, and ferulic acid was higher in Chinese products. Principal component analysis based on the GC-MS peak area of the volatiles also clearly distinguished shinkiku products manufactured in China from those in Korea. Chinese products contained higher amounts of benzaldehyde and anethole than Korean ones. Korean products were further separated into two groups: one with relatively higher linalool and terpinen-4-ol and another with higher hexanoic acid and 1-octen-3-ol. Thus, our study revealed the commonality and diversity of commercial shinkiku products, in which the commonalities can possibly be the reference standard for quality control of shinkiku, and the diversity suggested the importance of microbial management to stabilize the quality of shinkiku.
Massa Medicata Fermentata is a traditional crude drug used for treatment of anorexia and dyspepsia in elders in East Asia, called shinkiku in Japan, shenqu in China, and singug in Korea. Shinkiku is prepared from wheat (Triticum sativum), apricot kernel (Prunus armeniaca), red beans (Phaseolus angularis), polygonum (Polygonum hydropiper), sweet wormwood (Artemisia apiacea), and cocklebur (Xanthium strumarium). After mixing these materials, the mixture is fermented for a few days and subsequently dried to obtain shinkiku (1). Although shinkiku is manufactured in only China or Korea, it is also used in Japanese Kampo medicine as a component of “Hangebyakujutsutemmato (半夏白朮天麻湯),” which is used to treat dizziness and nausea (2, 3). Along with the increase in the consumption of Hangebyakujutsutemmato, the demand for shinkiku has also increased in recent years in Japan (4). However, the quality of shinkiku considerably differs with respect to the manufacture (5). This is because there are no reference standards for quality control of shinkiku. The voluntary standard in Japan stated that shinkiku should contain appreciable starch and reducing sugar, while that in Korea or China focused on the maximum ash or water content (1, 6, 7). The quality instability of shinkiku is a serious problem among the distributers of crude drugs in Japan. Thus, the characterization and standardization of commercial shinkiku are strongly needed to stabilize its medicinal grade quality.
A characteristic of shinkiku is the unique microbial fermentation process during its manufacture. “Qu” is sometimes mixed as a fermentative starter in China. Qu is the same as “Koji” in Japan, which is a solid-state culture of filamentous fungi (Aspergillus sp., Rhizopus sp., or Mucor sp.) on cereal grains, and is used to manufacture soy sauce, vinegar, and liquor. As these filamentous fungi produce various enzymes during growth (8–10), qu serves as an exogenous enzyme source during the production of fermented foods. Enzymes purified from Aspergillus sp. are also applied for medical industries. α-Amylase and lipase produced by Aspergillus oryzae have been used as digestive agents to treat dyspepsia (11). Furthermore, Aspergillus sp. produces ferulic acid esterase and releases free ferulic acid from the cell wall of plants (12, 13). Ferulic acid has been reported to show anti-inflammatory effects and accelerate gastrointestinal motility (14, 15). It has also been reported that free ferulic acid increased by fermentation with qu (16). Thus, the fermentation process by those filamentous fungi would add the stomachic function to the unfermented materials.
Chen et al. (17) has reported that Aspergillus sp. and Rhizopus sp. also exist in Chinese shinkiku products. Our previous study showed that shinkiku obtained from local markets in China and Korea contained digestive enzymes and ferulic acid (5). Therefore, digestive enzymes and ferulic acid derived by filamentous fungi possibly contribute to the stomachic property of shinkiku. However, in the previous study, neither Aspergillus sp. nor Rhizopus sp. could be detected using denaturing gradient gel electrophoresis (DGGE). It seems that DGGE is not appropriate for investigating the microbial structures of shinkiku. In addition, it was possible that shinkiku was stored in unsuitable environments in local markets, which altered the microbial conditions during storage. As shinkiku is prepared by microbial fermentation, characterization of microorganisms is required for quality control. In the present study, we obtained new commercial shinkiku products from a Japanese trading company specializing in crude drugs and investigated their chemical constituents and microbial structures using amplicon sequencing to establish reference standards for quality control of shinkiku in Japan.
Chemicals used for the analysis were obtained from Nacalai Tesque Inc. (Kyoto, Japan) and Wako Pure Industries Ltd. (Osaka, Japan). Folin and Ciocalteu's phenol reagent were purchased from MP Biomedicals (CA, USA), and ethylenediaminetetraacetic acid (EDTA) was obtained from DOJINDO (Kumamoto, Japan).
Thirteen commercial shinkiku products, imported from Korea or China, were kindly provided by Tochimoto Tenkaido Co., Ltd. (Osaka, Japan), Kotaro pharmaceutical Co., Ltd. (Osaka, Japan), and UCHIDAWAKANYAKU Ltd. (Tokyo, Japan). Shinkiku products used in this study are shown in Figure 1, and their production country and year of import are shown in Table 1. All the samples were maintained at −20°C until analysis.
Figure 1. Commercial shinkiku products used in this study. Chinese products for C1–C7, and Korean products for K8–K13.
DNA extraction was performed using a ZR Fecal DNA Miniprep kit (Zymo Research, CA, USA) according to the manufacturer's instructions. Mechanical disruption was carried out for each 100 mg of shinkiku at 6.0 m/s for 30 s by bead beating using a FastPrep 120 Cell Disrupter System (Thermo Savant; Carlsbad, CA, USA) and subjected to DNA extraction. The extracted DNA was quantified using a NanoDrop-8000 Spectrophotometer (Thermo Fisher Scientific K.K., Japan) and stored at −20°C until amplicon sequencing.
Amplicon sequencing analysis was performed at Bioengineering Lab. Co., Ltd. (Kanagawa, Japan). PCR amplification was performed using primer sets V3V4f and V3V4r for bacterial 16S rDNA V3/V4 regions and ITS1F_KYO1 and ITS2_KYO2 for the fungal ITS1 region (18, 19). For each sample, a library was prepared using the two-step tailed PCR method. The obtained amplicons were subjected to a 2 × 300 bp paired end run using a MiSeq system (Illumina K.K., Tokyo, Japan). The data were deposited to the DNA data Bank of Japan (DDBJ) under the accession numbers DRR205793-DRR205818. After quality filtering and chimera check of sequencing reads, the operational taxonomic units (OTU) were predicted using QIIME (Quantitative Insight Into Microbial Ecology) (20). The OTUs that accounted for more than 1% of the total sequence number were classified, whereas taxa with abundance <1% were summarized as “others.”
Crude enzyme extract was prepared according to the Official Analysis Method of National Tax Agency of Japan. Shinkiku (2 g) was suspended in 10 mL of 0.1 M acetate buffer solution (pH 5.0) containing 0.5% NaCl and homogenized at 11,000 rpm for 2 min on ice. Then, the homogenate was centrifuged at 22,300 × g at 4°C for 3 min. α-Amylase activity was measured using an α-amylase assay kit (Kikkoman Biochemifa, Tokyo, Japan) following the manufacturer's instructions, and 2-chloro-4-nitrophenyl-65-azido-65-deoxy-β-maltopentaoside (N3-G5-β-CNP) was used as a substrate, which was cleaved to 2-chloro-4-nitrophenol (CNP) on action of α-amylase. The increment of CNP was measured at 400 nm using a spectrophotometer. One unit of α-amylase activity was referred to the quantity of α-amylase required to release 1 μmol of CNP from N3-G5-β-CNP per minute at 37°C.
Crude enzyme extract (10 mL) was dialyzed in a cellulose tube (Dialysis membrane 27/32, Viskase Companies Inc., USA) for 15 h in 1 L of 10 mM of acetate buffer (pH 5.0) and then filled up to 20 mL with water for the protease activity assay. A casein solution (2%, w/v) was used as a substrate, and the derived tyrosin by protease was quantified by a chromogenic reaction which used Folin and Ciocalteu's phenol reagent. Tyrosine was quantified using the standard curve obtained from absorbance of the known concentration of tyrosine standard. One unit of protease activity was defined as the quantity of protease required to release 1 μg of tyrosine from casein per hour at 40°C.
Lipase solution from shinkiku was prepared according to the method of Rahayu et al. (21) with slight modification. Shinkiku (5 g) was added to 15 mL of 0.1 M acetate buffer solution (pH 5.0) containing 0.5% NaCl and homogenized at 11,000 rpm for 1 min, and then, 10 mL of ethanol (99.5%) was added to the mixture and held for 1 h to extract. The supernatant obtained by centrifugation at 890 × g for 5 min was used as a lipase solution. The lipase activity was determined by Lipase Activity Assay Kit (Cayman Chemical, MI, USA) following the assay protocol. An arachidonoyl-1-thioglycerol was used as substrate, and thioglycerol derived by lipase was quantified using a fluorescence detector (ex. 380 nm, em. 510 nm) (Mithras LB940, Berthold, GmbH & co. KG., Germany) after reaction with the thiol detector. The thioglycerol concentration was determined using the calibration curve obtained from the fluorescence intensity of the known concentration of thioglycerol standard. One unit was defined as the amount of lipase that was required to release 1 nmol of thioglycerol from arachidonoyl-1-thioglycerol per minute at 37°C.
Ferulic acid (4-hydroxy-methoxycinnamic acid) was quantified according to the method of Okutsu et al. (5) with slight modifications. Shinkiku (2 g) was added to 10 mL of a water/methanol mixture (3:2) and was homogenized at 11,000 rpm for 1 min. The mixture was then centrifuged at 4,800 × g for 5 min. The supernatant was filtered through a 0.45-μm cellulose acetate membrane filter (Toyo Roshi Kaisha Ltd., Tokyo, Japan), and 10 μL of the sample was injected into the High Performance Liquid Chromatography (HPLC) system (Shimadzu LC system 20A, Shimadzu Corp., Kyoto, Japan). The ferulic acid concentration was determined using the calibration curve obtained from the peak area of the known concentration of ferulic acid standard.
Organic acids except for ferulic acid were determined using a Prominence HPLC system and an electroconductivity detection (Shimadzu CDD-10AVP). Each shinkiku (2 g) sample was mixed with 20 mL of deionized water, homogenized at 11,000 rpm for 1 min, and then, centrifuged at 4,800 × g for 5 min to obtain a supernatant. The supernatant was filtered through a 0.45 μm pore-size cellulose acetate membrane filter. The separation of organic acids was performed using an ion-exclusion chromatography column (Shim-pack SCR-102H, 8 mm I.D. × 300 mm × 2) equipped with a Guard column (SCR-102H, 6 mm I.D. × 50 mm) at 50°C using 4 mM p-toluenesulfonic acid as the mobile phase at a flow rate of 0.8 mL/min. The buffer solution containing 16 mM Bis-Tris, 4 mM p-toluenesulfonic acid, and 80 μM EDTA was pumped into the column at 0.8 mL/min flow rate.
The supernatant used for organic acid analysis was also used to analyze the volatile compounds in shinkiku products. The volatile compounds were evaluated with the method described by Rahayu et al. (21). In brief, 10 mL supernatant, described in the quantification of organic acid, was transferred to a 10 mL sample vial with a 15-mm stir bar coated with 0.5 mm polydimethylsiloxane (Twister, GERSTEL K.K., Japan). The sample was stirred using a stir bar at 1,200 rpm for 60 min at 25–30°C to adsorb volatile components. Then, the stir bar was removed from the sample, washed with deionized water, and placed into a glass insert. The volatile compounds were desorbed from the SBSE stir bar using the temperature program of thermal desorption system (GERSTEL TDS 3, GERSTEL CIS 4, GERSTEL K.K., Japan) and transferred to a gas chromatograph (6890N Network GC System) equipped with a mass spectrometer (5975B insert MSD) (Agilent Technologies, Palo Alto, CA). The volatile compounds were separated on a Pure-WAX column (0.25 mm I.D. × 60 m length × 0.25 μm film thickness, J&W Scientific, Folsom, CA), and the oven temperature was held at 50°C for 5 min at 3°C/min till the temperature reached 240°C (hold for 5 min). The carrier gas was helium at a flow rate of 2 mL/min. The detected compounds were identified by comparison of their mass spectra with the National Institute of Standards and Technology (Gaithersburg, MD, USA) mass spectral library (NIST 05) and by comparison of their retention index (RI) with the database of Aroma Office software (Nishikawa Keisoku/Gerstel K.K., Japan).
Preliminary, we investigated the viable fungi in shinkiku products using a plating assay to detect Aspergillus sp., Rhizopus sp., or Mucor sp.; however, no filamentous fungus like colonies were detected except for C1, K11, and K13 (Table S1). Thus, amplicon sequencing analysis was applied to investigate the community structure of microorganism that included filamentous fungi in shinkiku products.
Aspergillus sp. was detected in all shinkiku products, and Rhizopus sp. was found in more than half of shinkiku products (7/13) (Figure 2A). Aspergillus sp. and Rhizopus sp. were totally accounted for more than 50% in C3, K11, K12, and K13. Rhizopus sp. was predominant in C1, C3, and C4 compared with Aspergillus sp., but Aspergillus sp. was predominant in C2, K11, K12, and K13. In particular, Aspergillus sp. accounted for more than 70% in K11, K12, and K13. Meanwhile, Mucor sp. was detected only in K11 at 3%, and Saccharomyces sp. was detected in K11 and K12 at 4–7%. Xeromyces sp. was detected in C5, K8, and K9, and accounted for more than 40%. Wallemia sp. was detected in K10 and accounted for more than 80%. In addition, Fusarium sp. was detected in Chinese products such as C1, C2, C3, C4, C6, and C7, and accounted for 36% in sample C4. Botrytis sp. was characteristically detected in C7 with an abundance rate of 55%. Wickerhamomyces sp. was detected in C1, C3, C6, C7, K9, and K11 and accounted for 52% in C6.
Figure 2. Relative abundance of bacterial and fungal sequence reads at the genus level in shinkiku products. (A) Fungi detected by ITS1F_KYO1-ITS2_KYO2 primer set; (B) Bacteria detected by V3/V4f-V3/V4r primer set.
For bacterial community structure, Bacillus sp. accounted for more than 60% in all Chinese products, but chloroplast and mitochondria DNA accounted for more than 50% in Korean products such as K11, K12, and K13 (Figure 2B). Multiple lactic acid bacteria were detected in Korean products. Pediococcus sp. and Lactobacillus sp. were detected in K12, and Weissella sp. was detected in K9 and K10. In contrast, Erwinia sp. was detected in K8.
As shinkiku is used to treat anorexia and dyspepsia, digestive enzymes derived by microorganisms in shinkiku work as a digestive promotor (22). Thus, we measured the digestive enzyme activities in shinkiku products. All shinkiku products showed α-amylase (8.3–51.3 U/g), protease (86.0–7892.8 U/g), and lipase (0.1–5805.5 U/g) activity, and Korean products tended to have higher enzyme activity than Chinese products (Table 2). K8 and K10 showed higher amylase activity compared to other shinkiku products. K13 showed the highest protease activity, and K11 showed the highest lipase activity among all shinkiku products.
Ferulic acid could contribute to efficacies of shinkiku because it accelerates gastrointestinal motility (15). Because some microorganisms secrete ferulic acid esterase (13, 23), free ferulic acid could be released from wheat materials during shinkiku fermentation. All shinkiku products were confirmed to contain free ferulic acid, and the content ranged from 25.8 to 91.4 nmol/g in Korean products and from 99.9 to 554.6 nmol/g in Chinese products (Table 3).
Organic acids are important metabolites of fermentative microorganisms. As each microorganism secretes different organic acids, we quantified the organic acids to investigate the relationship with microbial structure in shinkiku products. Citric acid, pyruvic acid, lactic acid, formic acid, and acetic acid were detected in shinkiku products (Table 3). Although K11, K12, and K13 contained remarkably high citric acid (71.9–88.8 μmol/g), the total organic acid contents tended to be higher in Chinese products compared to Korean products. Especially C2, C4, and C5 contained higher lactic acid compared to other shinkiku products.
Herb materials in shinkiku contain various essential oils, and they act as appetizers (stomachic) (24). We measured the volatile content in shinkiku products exhaustively using GC-MS. A total of 146 compounds were detected, and among them, 39 compounds were common in all Chinese and Korean products: 7 acids, 5 alcohols, 8 aldehydes, 7 esters, 5 ketones, 3 lactones, 3 phenols, and 1 polycyclic aromatic hydrocarbon (Table 4). Principal component analysis (PCA) were used to analyze datasets of peak area of 39 common volatiles to differentiate shinkiku products. The PCA score plot indicated a clear variance for different volatiles based on different manufacture across PC1 (42.98%) and PC2 (16.91%) (Figure 3).
Figure 3. Principal component analysis based on peak area of common volatile components in shinkiku products. (A) is for Score plot, and (B) is for Loading plot.
A clustered pattern was observed in Chinese products with a higher content of some benzenoid aromatic hydrocarbons such as naphthalene, ethyl benzoate, benzaldehyde, and benzyl alcohol. The Korean products were further separated into two groups. Samples K8, K9, and K10 were classified into the same group (Korea A) because of the higher content of terpenoids such as linalool, paeonol, and terpinen-4-ol. Meanwhile, samples K11, K12, and K13 were differentiated (Korea B) from other groups with higher contents of fatty acid (hexanoic acid, capric acid, and caprylic acid), aldehydes (decanal and hexanal), and alcohols (β-phenylethyl alcohol and 1-octen-3-ol). In addition to the common volatiles, 2,3-butanediol and pyrazines were characteristically detected in all Chinese products. Vanillin and 4-vinylguaiacol, which are metabolites of ferulic acid, were also detected in all Chinese products. Meanwhile, d-limonene, myristic acid, toluene, 2-pentylfuran, 2-heptanone, hinesol, and methylguaiacol were only detected in all Korean products (data not shown).
Amplicon sequencing analysis revealed that Aspergillus sp. was common microorganism in all shinkiku products, and no common bacterial types was detected in all products. Thus, shinkiku was manufactured with microbial fermentation with such filamentous fungi. As these fungi were difficult to be detected as viable cells, they possibly died during the production or storage process of shinkiku. Thus, amplicon sequencing analysis seems appropriate for clarifying the microbial structure in shinkiku. In addition, digestive enzymes (α-amylase, protease, and lipase), organic acids (ferulic acid, citric acid, lactic acid, and acetic acid), and 39 volatiles were commonly found in shinkiku products. These microbial and chemical characteristics of shinkiku is fundamental data for the quality control of shinkiku in the market, and the commonalities would contribute to normalize the quality of shinkiku.
However, microbial and chemical characteristics of shinkiku were considerably different among the shinkiku products. Various fungi were detected in Chinese products. Not only Aspergillus sp. and Rhizopus sp. but also Mucor sp. and Saccharomyces sp. were detected with a small abundance. These microorganisms have been reported to exist in Chinese qu (25, 26). Qu is mixed as a fermentation starter for shinkiku manufacture in China. The microbial community of qu was reported to be different among the products because it was prepared by natural fermentation (8, 27). Thus, the microbial diversity of qu possibly affects the microbial structures of shinkiku in China.
For bacteria communities, Bacillus sp. was predominant in all Chinese products. As Bacillus sp. is generally found in soil and grass hay, it possibly comes from wheat bran or herb materials in shinkiku. Bacillus sp. is reported to secret considerable ferulic acid esterase (23). It is expected that the higher ferulic acid in Chinese products was caused by ferulic acid esterase secreted by Bacillus sp. Higher levels of benzenoid compounds such as benzaldehyde, and anethole in Chinese products were also derived by Bacillus sp. because these volatiles have been reported to be synthesized by Bacillus sp. (28, 29).
In the case of Korean products, Aspergillus sp. was more predominant than Rhizopus sp., and microbial composition was simpler compared to Chinese products. It suggests that pure cultivated Aspergillus sp. might be inoculated in the materials to ferment shinkiku in Korea. In addition, a relatively high abundance of chloroplast DNA from herb materials and that from mitochondria were detected in Korean products. This result suggests that the amount of DNA derived from bacteria was extremely low in Korean products. The number of viable bacteria in Korean products was also considerably lower than that in Chinese products (Table S1). It was reported that the number of viable bacteria in qu was increased at the late stage of fermentation (30). Therefore, it was expected that Korean shinkiku was short-fermented with simple microorganisms.
Lactic acid bacteria such as Weissella sp., Lactobacillus sp., and Pediococcus were detected in Korean products. As these lactic acid bacteria are known to have potential to promote digestion for humans (31, 32), they possibly contribute to the efficacies of Korean shinkiku for anorexia and dyspepsia. However, the relative abundances of lactic acid bacteria were inconsistent with lactic acid contents in shinkiku. In addition, Rhizopus sp. in qu was also reported to secrete lactic acid (33), but the relative abundance of Rhizopus sp. was inconsistent with lactic acid content in shinkiku products. It possible that the microbial structure changed during fermentation, and the microorganisms in the early stage of the fermentation process affect the lactic acid contents in shinkiku products. Although our study revealed the microbial structure only in the final product of shinkiku, further studies are needed to investigate the changes in microbes and their metabolites during shinkiku fermentation.
PCA based on the volatile components differentiated shinkiku products into three groups, among them two groups were composed of Korean shinkiku (Korea A and Korea B). The relative abundance of Aspergillus sp. in Korea B was considerably higher than that in Korea A. High levels of fatty acids in Korea B were possibly caused by lipase because of their higher lipase activity. The higher citric acid content in Korea B was also caused by its high abundance of Aspergillus sp. because Aspergillus sp. is known to secrete considerable digestive enzymes and citric acid (34). Therefore, the microbial characteristics were found to affect the chemical composition of shinkiku.
In contrast, some contaminating microorganisms were also detected in several shinkiku products. Xeromyces sp. and Wallemia sp. are known as food-contaminating fungi, and they were reported to have drought-resistance (35, 36). Fusarium sp. is a type of plant pathogenic fungi that produces mycotoxin (37). Erwinia sp. is known as a pathogenic bacteria of plants (38), and thus, Erwinia sp. detected in shinkiku products was possibly derived from infected herb materials. This result suggests that some samples in the market were possibly contaminated with poisonous microorganisms, and thus, microbial control would be critically important in shinkiku production.
In addition to the microbial structure, manufacturing conditions seemed to be different among manufacturers. Bacillus sp., which is predominant in Chinese products, forms heat-resistant spores (39), and thermal treatment caused the selective survival of Bacillus sp. (40). The lower enzyme activities in Chinese products indicated the possibility of enzyme deactivation by heat treatment. In addition, pyrazines, which are produced during heating (41), were also detected in Chinese products. Thus, Chinese products should be thermally treated for dryness after fermentation. In contrast, heat treatment should not be carried out for Korean products because enzyme activities in Korean products are higher than those in Chinese products. Korean products, especially Korea A, contained higher terpenoids such as linalool and terpinen-4-ol. They were derived from essential oils in herbal materials in shinkiku: wormwood (42), cocklebur (43), and polygonum (44). As these terpenoids are volatile, Korean shinkiku is possibly dried at lower temperature than Chinese shinkiku. In addition, ferulic acid content was relatively lower in Korean products in spite of the higher abundance of Aspergillus sp., which is also reported to secret ferulic acid esterase (12, 13). As ferulic acid is an enzymatic product, the production temperature and duration possibly affected the ferulic acid content in shinkiku. Further studies are needed to investigate the relationships between manufacturing processes and chemical constituents in shinkiku.
Although there was no relationship between the import year and quality of shinkiku (Table 1), some shinkiku products contained vanillin, which is possibly formed during storage. Vanillin is derived from 4-vinylguaiacol by the maturation of liquors such as awamori (45). As vanillin formation is affected by temperature, the storage condition of shinkiku would affect the vanillin content. The effects of storage and transportation were also confirmed by comparing the effects observed in the previous study. Digestive enzyme activities and ferulic acid contents of some shinkiku obtained from local markets were significantly lower than those in the present study (5). As shinkiku seems to be stored for a long time in unsuitable environment in the local markets, the enzyme activities or ferulic acid content were possibly altered depending on the storage condition. Further studies are required to investigate the aging effects on the quality of shinkiku.
In conclusion, our study revealed that the commonality and diversity of commercial shinkiku products. The commonalities were possibly the reference standard for quality control of shinkiku. Furthermore, amplicon sequence analysis showed a clearly different microbial characteristic among the products. As a result of chemical analysis partially corresponding to microbial structure, the microorganisms in shinkiku were found to affect its chemical composition. Thus, microbial management was suggested to be important to stabilize the quality of shinkiku products. In addition, the results indicated that manufacturing conditions such as heating temperature seemed to be different among manufacturers. To standardize the shinkiku quality, further studies are needed to elucidate the effects of microbes or manufacturing conditions on chemical constituents of shinkiku.
The datasets generated for this study can be found in the DNA data Bank of Japan (DDBJ)/DRR205793-DRR205818.
ZW and KO performed all chemical analyses and wrote the original draft. YY, KK, FH, and KTa contributed to the conception and design of the experiment and paper preparation. TF and HT supervised microbial analysis and reviewed the drafts of the paper. TM and KTo performed sample collection and reviewed drafts of the paper. All authors contributed to the article and approved the submitted version.
This work was supported by a Grant-in-Aid for the Cooperative research Project from Institute of Natural Medicine, University of Toyama in 2017 and Grant No. (19K02374) of JSPS KAKENHI.
The authors declare that the research was conducted in the absence of any commercial or financial relationships that could be construed as a potential conflict of interest.
The institute of Natural Medicine, University of Toyama, is gratefully acknowledged for having provided the financial support for this study. We appreciate Dr. Yohei Shiraishi in Bio'c Co., Ltd. for viable cell analysis of shinkiku products. We wish to thank Kotaro pharmaceutical Co., Ltd., Tochimoto Tenkaido Co., Ltd. and UCHIDAWAKANYAKU Ltd. for kindly providing us the shinkiku products. We would like to thank Editage (www.editage.jp) for English language editing.
The Supplementary Material for this article can be found online at: https://www.frontiersin.org/articles/10.3389/fnut.2020.00115/full#supplementary-material
1. Ministry of Health, Labour, and Welfare Evaluation, and Licensing Division of Pharmaceutical and Food Safety Bureau. Self-Imposed Standards for the Crude Drugs Without Official Standards (Provisional translation). (2012).
2. Watanabe K, Mori K, Tokumine J, Yorozu T. Effect of hangebyakujutsutemmato on pregabalin-induced dizziness in a rat model of neuropathic pain. Tradit Kampo Med. (2019) 6:88–95. doi: 10.1002/tkm2.1218
3. Suzuki H, Sone H, Watanabe K. Japanese kampo medicines for diabetes mellitus. In: Arumugam S, Watanabe K, editors. Japanese Kampo Medicines for the Treatment of Common Diseases: Focus on Inflammation. Elsevier: Academic Press (2017). p. 69–73. doi: 10.1016/B978-0-12-809398-6.00008-1
4. Yamamoto Y, Ko H, Sasaki H, Takeda O, Higuchi Y, Mukaida Y, et al. Survey on crude drug in Japan. Japan J Pharmacog. (2019) 73:16–35. doi: 10.24684/jspharm.73.1_16
5. Okutsu K, Kadooka C, Kojo A, Yoshizaki Y, Futagami T, Tamaki H, et al. Microbial diversity and chemical constituents in “Shinkiku”, a fermented crude drug. Japan J Pharmacog. (2017) 71:41–8. doi: 10.24684/jspharm.71.1_41
6. National Health Commission of the People's Republic China. National Standard for Chinese Patent Drugs (Provisional Translation). (1989).
7. Korea Food and Drug Administration. The Korean Herbal Pharmacopoeia Fourth edition (KHP IV). (2002). p. 162.
8. Huang FX, Cai TD, Nip W. Chinese wines: jiu. In: Hui YY, editor. Handbook of Food Science, Technology, and Engineering. Boca Raton, FL: CRC press (2006). p. 3–15.
9. Ushijima S. Improvement of industrial Aspergillus fungi. In: Smith JE, editor. Aspergillus. New York, NY: Springer Science & Business Media, LLC (2012). p. 41–64.
10. Londoño-Hernándeza L, Ramírez-Torob C, Ruiza AH, Ascacio-Valdésa AJ, Aguilar-Gonzalezc AM, Rodríguez-Herreraa R, et al. Rhizopus oryzae – ancient microbial resource with importance in modern food industry. Int J Food Microbiol. (2017) 257:110–27. doi: 10.1016/j.ijfoodmicro.2017.06.012
11. Zhu Y, Tramper J. Koji - where East meets West in fermentation. Biotechnol Adv. (2013) 31:1448–57. doi: 10.1016/j.biotechadv.2013.07.001
12. Faulds CB, Williamson G. Release of ferulic acid from wheat bran by a ferulic acid esterase (FAE-III) from Aspergillus niger. Appl Microbiol Biotechnol. (1995) 43:1082–7. doi: 10.1007/BF00166929
13. Koseki T, Hori A, Seki S, Murayama T, Shiono Y. Characterization of two distinct feruloyl esterases, AoFaeB d AoFaeC, from Aspergillus oryzae. Appl Microbiol Biotechnol. (2009) 83:689–96. doi: 10.1007/s00253-009-1913-z
14. Ou SY, Kwok KC. Ferulic acid: pharmaceutical functions, preparation and applications in foods. J Sci Food Agric. (2004) 84:1261–9. doi: 10.1002/jsfa.1873
15. Badary OA, Awad AS, Sherief MA, Hamada FM. In vitro and vivo effects of ferulic acid on gastrointestinal motility: inhibition of cisplatin-induced delay in gastric emptying in rats. World J Gastroenterol. (2006) 12:5363–7. doi: 10.3748/wjg.v12.i33.5363
16. Mo X, Xu Y. Ferulic acid release and 4-vinylguaiacol formation during Chinese rice wine brewing and fermentation. J Inst Brew. (2012) 116:304–11. doi: 10.1002/j.2050-0416.2010.tb00435.x
17. Chen J, Jiao XL, Yang CY, Song MF, Gao WW. Fungal composition in massa medicata fermentata based on culture dependent method and independent PCR-SSCP technique. Zhongguo Zhong Yao Za Zhi. (2014) 39:4169–73. doi: 10.4268/cjcmm20142117
18. Toju H, Tanabe AS, Yamamoto S, Sato H. High-coverage ITS primers for the DNA-based identification of ascomycetes and basidiomycetes in environmental samples. PLoS ONE. (2012) 7:e40863. doi: 10.1371/journal.pone.0040863
19. Herlemann D, Labrenz M, Jürgens K, Bertilsson S, Waniek J, Anderssonet A. Transitions in bacterial communities along the 2000 km salinity gradient of the Baltic Sea. ISME J. (2011) 5:1571–9. doi: 10.1038/ismej.2011.41
20. Caporaso J, Kuczynski J, Stombaugh J, Bittinger K, Bushman F, Costello E, et al. QIIME allows analysis of high-throughput community sequencing data. Nat Methods. (2010) 7:335–6. doi: 10.1038/nmeth.f.303
21. Rahayu YYS, Yoshizaki Y, Yamaguchi K, Okutsu K, Futagami T, Tamaki H, et al. Key volatile compounds in red koji-shochu, a Monascus-fermented product, and their formation steps during fermentation. Food Chem. (2017) 224:398–406. doi: 10.1016/j.foodchem.2016.12.005
22. Whitcomb DC, Lowe ME. Human pancreatic digestive enzymes. Dig Dis Sci. (2007) 52:1. doi: 10.1007/s10620-006-9589-z
23. Donaghy J, Kelly PF, McKay AM. Detection of ferulic acid esterase production by Bacillus spp and lactobacilli. Appl Microbiol Biotechnol. (1998) 50:257–60. doi: 10.1007/s002530051286
24. Skalicka-Wozniak K, Walasek M, Ludwiczuk A, Glowniak K. Isolation of terpenoids from Pimpinella anisum essential oil by high-performance counter-current chromatography. J Sep Sci. (2013) 36:2611–4. doi: 10.1002/jssc.201300407
25. Gou M, Wang H, Yuan H, Zhang W, Tang Y, Kida K. Characterization of the microbial community in three types of fermentation starters used for Chinese liquor production. J. Inst. Brew. (2015) 121:620–7. doi: 10.1002/jib.272
26. Wu H, Zhang S, Ma Y, Zhou J, Luo H, Yang J. Comparison of microbial communities in the fermentation starter used to brew Xiaoqu liquor. J Inst Brew. (2017) 123:113–20. doi: 10.1002/jib.388
27. Tang Q, He G, Huang J, Wu C, Jin Y, Zhou R. Characterizing relationship of microbial diversity and metabolite in Sichuan Xiaoqu. Front Microbiol. (2019) 10:696. doi: 10.3389/fmicb.2019.00696
28. Fujita K, Fujita T, Kubo I. Anethole, a potential antimicrobial synergist, converts a fungistatic dodecanol to a fungicidal agent. Phytother. Res. (2007) 21:47–51. doi: 10.1002/ptr.2016
29. Chukeatirote E, Eungwanichayapant PD, Kanghae A. Determination of volatile components in fermented soybean prepared by a co-culture of Bacillus subtilis and Rhizopus oligosporus. Food Res. (2017) 1:225–33. doi: 10.26656/fr.2017.6.066
30. Hu Y, Dun Y, Li S, Fu B, Xiong X, Peng N, et al. Changes in microbial community during fermentation of high-temperature Daqu used in the production of Chinese ‘Baiyunbian' liquor. J Inst Brew. (2017) 123:594–9. doi: 10.1002/jib.455
31. Gilliland SE. Health and nutritional benefits from lactic acid bacteria. FEMS Microbiol Rev. (1990) 7:175–88. doi: 10.1111/j.1574-6968.1990.tb04887.x
32. Fessard A, Remize F. Why are Weissella spp. not used as commercial starter cultures for food fermentation? Fermentation. (2017) 3:38. doi: 10.3390/fermentation3030038
33. Yin X, Yoshizaki Y, Ikenaga M, Han LX, Okutsu K, Futagami T, et al. Manufactural impact of the solid-state saccharification process in rice-flavor baijiu production. J Biosci Bioeng. (2019) 129:315–21. doi: 10.1016/j.jbiosc.2019.09.017
34. Hong SB, Lee M, Kim DH, Varga J, Frisvad JC, Perrone G. Aspergillus luchuensis, an industrially important black Aspergillus in East Asia. PLoS ONE. (2013) 8:e63769. doi: 10.1371/journal.pone.0063769
35. Leong SL, Pettersson OV, Rice T, Hocking AD, Schnurer J. The extreme xerophilic mould Xeromyces bisporus – growth and competition at various water activities. Int J Food Microbiol. (2011) 145:57–63. doi: 10.1016/j.ijfoodmicro.2010.11.025
36. Zajc J, Gunde-Cimerman N. The genus Wallemia—from contamination of food to health threat. Microorganisms. (2018) 6:46. doi: 10.3390/microorganisms6020046
37. Munkvold GP. Fusarium species and their associated mycotoxins. In: Moretti A, Susca A, editors. Mycotoxigenic Fungi. New York, NY: Humana Press (2017). p. 51–106.
38. Chatterjee AK, Starr MP. Genetics of Erwinia species. Ann Rev Microbiol. (1980) 34:645–76. doi: 10.1146/annurev.mi.34.100180.003241
39. Setlow P. Spores of Bacillus subtilis: their resistance to and killing by radiation, heat and chemicals. J Appl Microbiol. (2006) 101:514–25. doi: 10.1111/j.1365-2672.2005.02736.x
40. Luu S, Cruz-Mora J, Setlow B, Feeherry FE, Doona CJ, Setlow P. The effects of heat activation on Bacillus spore germination, with nutrients or under high pressure, with or without various germination proteins. Appl Environ Microbiol. (2015) 81:2927–38. doi: 10.1128/AEM.00193-15
41. Maga JA, Sizer CE, Myhre DV. Pyrazines in foods. Crit Rev Food Sci Nutr. (1973) 4:39–115. doi: 10.1080/10408397309527153
42. Zhang N, Tang L, Hu W, Wang K, Zhou Y, Li H, et al. Insecticidal, fumigant, and repellent activities of sweet wormwood oil and its individual components against red imported fire ant workers (Hymenoptera: Formicidae). J Insect Sci. (2014) 14:241. doi: 10.1093/jisesa/ieu103
43. Esmaeili A, Rustaiyan A, Akbari TM, Moazami N, Masoudi S, Amiri H. Composition of the essential oils of Xanthium strumarium L and Cetaurea solstitialis L from Iran. J Essent Oil Res. (2006) 18:427–9. doi: 10.1080/10412905.2006.9699131
44. Lee TK, Vairappan CS. Antioxidant, antibacterial and cytotoxic activities of essential oils and ethanol extracts of selected South East Asian herbs. J Med Plant Res. (2011) 5:5284–90.
Keywords: shinkiku, Hangebyakujutsutemmato, Aspergillus sp., Rhizopus sp., ferulic acid
Citation: Wang Z, Okutsu K, Futagami T, Yoshizaki Y, Tamaki H, Maruyama T, Toume K, Komatsu K, Hashimoto F and Takamine K (2020) Microbial Community Structure and Chemical Constituents in Shinkiku, a Fermented Crude Drug Used in Kampo Medicine. Front. Nutr. 7:115. doi: 10.3389/fnut.2020.00115
Received: 17 February 2020; Accepted: 19 June 2020;
Published: 31 July 2020.
Edited by:
Masahiro Ohsawa, Nagoya City University, JapanReviewed by:
Lila Oyama, Federal University of São Paulo, BrazilCopyright © 2020 Wang, Okutsu, Futagami, Yoshizaki, Tamaki, Maruyama, Toume, Komatsu, Hashimoto and Takamine. This is an open-access article distributed under the terms of the Creative Commons Attribution License (CC BY). The use, distribution or reproduction in other forums is permitted, provided the original author(s) and the copyright owner(s) are credited and that the original publication in this journal is cited, in accordance with accepted academic practice. No use, distribution or reproduction is permitted which does not comply with these terms.
*Correspondence: Kayu Okutsu, azg2NzM3MTFAa2FkYWkuanA=
Disclaimer: All claims expressed in this article are solely those of the authors and do not necessarily represent those of their affiliated organizations, or those of the publisher, the editors and the reviewers. Any product that may be evaluated in this article or claim that may be made by its manufacturer is not guaranteed or endorsed by the publisher.
Research integrity at Frontiers
Learn more about the work of our research integrity team to safeguard the quality of each article we publish.