- 1Cell Biology and Immunology Group, Department of Animal Sciences, Wageningen University and Research, Wageningen, Netherlands
- 2Host-Microbe Interactomics Group, Department of Animal Sciences, Wageningen University and Research, Wageningen, Netherlands
- 3Laboratory of Animal Products Chemistry, Graduate School of Agricultural Science, Tohoku University, Sendai, Japan
- 4Livestock Immunology Unit, International Education and Research Center for Food Agricultural Immunology, Graduate School of Agricultural Science, Tohoku University, Sendai, Japan
- 5South Product Co., Ltd., Uruma, Japan
Fucoidan represents fucose-rich sulfated polysaccharides derived from brown seaweeds, which exerts various biological activities applicable for functional foods and therapeutic agents. The objective of the present study was to investigate in vivo effects of fucoidan extracted from Okinawa mozuku (Cladosiphon okamuranus), common edible seaweed in Japan, on immune responses and microbiota composition in zebrafish. We treated larvae and adult zebrafish with Okinawa mozuku (OM) fucoidan by immersion (100 and 500 μg/mL, 3 days) and by feeding (3 weeks), respectively. The effect of OM fucoidan on immune responses in zebrafish larvae was evaluated by live imaging of neutrophils and macrophages as well as quantitative polymerase chain reaction of pro- and anti-inflammatory cytokine genes. Whole microbiota of zebrafish larvae and intestinal microbiota of adult zebrafish treated with OM fucoidan were analyzed by Illumina MiSeq pair-end sequencing of the V3–V4 region of 16S rRNA genes. Fucoidan treatment only slightly affected the composition of the larvae microbiota and the number of neutrophils and macrophages, while pro- and anti-inflammatory cytokine gene expression levels were upregulated in the larvae treated with 500 μg/mL OM fucoidan. In contrast, feeding of OM fucoidan clearly altered the intestinal microbiota composition of adult zebrafish, which was characterized by the emergence and predominance of multiple bacterial operational taxonomic units (OTUs) affiliated with Rhizobiaceae and Comamonadaceae at the expense of E. coli-related Enterobacteriaceae, the dominant OTUs throughout the studied samples. These changes were accompanied by decreased expression levels of pro-inflammatory cytokine il1b in the intestines of the adult zebrafish. Our current study provides the first insights into in vivo modulatory effects of fucoidan on microbiota and immune responses of unchallenged zebrafish, which underscores the potential of fucoidan to play a modulatory role in the diet–microbiota–host interplay.
Introduction
Fucoidan represents polysaccharides consisting of α-(1 → 3) or α-(1 → 4) -linked L-fucose residues with sulfate substitutions, which occasionally contain acetate, glucuronic acid, and monosaccharides such as mannose and galactose (1). Fucoidan from different algal origins has been reported to exhibit unique properties such as antiinflammatory, antiallergic, antitumor, or antiviral effects (2, 3) and is therefore recognized as a prospective ingredient for functional foods and for therapeutic agents (3, 4). Although beneficial effects of fucoidan have been well-studied and described, daily intake of fucoidan from brown seaweed is still not common in Western countries. In Japan, daily seaweed consumption can exceed ~5 g/day (5) and the brown seaweed mozuku represents one of the most common edible seaweeds, which is usually consumed raw. Okinawa mozuku (Cladosiphon okamuranus) is exclusively cultivated and used in the traditional cuisine on the Okinawan Islands in Japan, a region that is well-known for its high prevalence of centenarians and the general healthy states of its elderly population (6). Fucoidan extracted from Okinawa mozuku (OM fucoidan) has a simple structure with a backbone of α-(1 → 3) fucopyranose, substituted with sulfate and α-glucuronic acid at ~50 and 17% of its residues, respectively (7). Similar to what has been shown for fucoidan derived from other origins, OM fucoidan has been reported to exert antitumor and antiviral effects. In a murine model, antitumor activity has been attributed to the fucoidan-mediated stimulation of macrophages and natural killer cells (8, 9), while antiviral activities seem to be more complex and may involve both host–virus and virus–fucoidan interactions. Previous studies have reported antiviral activities of OM fucoidan against human T-cell leukemia virus type 1 (HTLV-1) (10, 11), dengue virus type 2 (12), hepatitis C (13), Newcastle disease virus (DSV) in poultry (14, 15), and canine distemper virus (CDV) (16). Collectively, these studies support the high potential of OM fucoidan as a therapeutic agent in viral infections.
Meanwhile, effects of OM fucoidan on the intestinal microbiota remain poorly understood. Polysaccharides such as fucoidan have a potential to not only mechanistically interfere with host–microbiota interactions but also to serve as nutrition for bacteria constituting the microbiota (17, 18). Since no enzymes digesting fucoidan have been found in animal intestinal tracts, fucoidan can reach the lower intestinal tract intact and may confer beneficial effects on microbiota as prebiotics (19–21). Importantly, some studies have suggested that bioactivities of fucoidan may be attributable to its modulatory effects on gut microbiota. A recent study has shown that fucoidan from Undaria pinnatifida can affect host lipid metabolism by modulating the gut microbiota composition (22), which may also explain the effect of OM fucoidan to ameliorate dyslipidemia in rodents (23). Other studies have reported that fucoidan from sea cucumber (Acaudina molpadioides) and hijiki seaweed (Sargassum fusiforme) can relieve symptoms of diabetes by modulating gut microbiota (24, 25).
Considering possible interactions between microbiota and host immune responses, it is crucial to evaluate host immunity and microbiota simultaneously to elucidate the prebiotic potential OM fucoidan (18). Zebrafish offer an ideal in vivo model to investigate how fucoidan affects host immunity and microbiota under normal (unchallenged) conditions because of their compatibility with live visualization (26). Using a double-transgenic zebrafish model combined with next-generation sequencing of 16S rRNA genes, we have recently shown that microbiota modulation by antibiotics can significantly affect host inflammatory immune responses in zebrafish larvae immersed in saponin (27). In this study, we exploited this approach to investigate how OM fucoidan can affect immune response and microbiota composition of zebrafish larvae. We also investigated the effect of OM fucoidan on immune responses and intestinal microbiota of adult zebrafish, which were fed with OM fucoidan for 3 weeks.
Materials and Methods
Ethics Statement
The present study was approved by the Dutch Committee on Animal Welfare and the Animal Welfare Body (IvD) of Wageningen University, The Netherlands. Furthermore, we adhere to our standard biosecurity and institutional safety procedures at Wageningen University and Research.
Zebrafish and Fucoidan
Tg (mpeg1:mCherry/mpx:eGFPi114) (28, 29) and wild-type zebrafish were maintained in Zebtec family tanks (Tecniplast, Buguggiate, Italy) under continuous flow-through at 28°C (14/10-h light/dark cycle) and fed daily with Tetramin Flakes (Tetra, Melle, Germany). For the experiments using zebrafish larvae, embryos were obtained from the adult transgenic zebrafish by natural spawning and raised with embryo medium (E3) water as described previously (27). OM fucoidan powder (>95% pure fucoidan) extracted from C. okamuranus as described previously (30) was provided by South Product Co., Ltd., Okinawa, Japan. The characteristics of this fucoidan were as follows: average molecular weight of 49.8 kDa, L-fucose content of 52.7%, uronic acid content of 18.0%, and sulfate ion content of 17.6%. The OM fucoidan powder was stored at room temperature until use. Fucoidan treatment of larvae and adult zebrafish was performed as follows: the zebrafish larvae (3 days post fertilization; dpf) were randomly distributed in six-well plates (n = 8 fish/well) and kept in different concentrations (0, 100, and 500 μg/mL of E3 water) of OM fucoidan (immersion) until 6 dpf. Ten adult zebrafish were maintained in two separate tanks in a continuous flow and temperature-controlled (28°C) system and fed once daily with Tetramin Flakes (control group) or a combination of the flakes and OM fucoidan at the ratio of 1:1 (fucoidan group) over 3 weeks.
In vivo Imaging of Neutrophils and Macrophages in Zebrafish Larvae
Tg (mpeg1:mCherry/mpx:eGFPi114) zebrafish larvae were anesthetized with MS-222 (tricaine methane sulfonate) solution and embedded in 1% low melting point agarose (Thermo Fisher Scientific, Waltham, MA, USA), as previously described (27). Larvae were imaged as whole mounts with a Leica M205 FA Fluorescence Stereo Microscope. Neutrophils and macrophages in the intestinal region of each specimen were quantified by counting the total number of cells per defined area using the cell counter plugin available in ImageJ® software (31).
Relative Gene Expression by Quantitative Polymerase Chain Reaction
Zebrafish were euthanized with MS-222 and the whole larvae (five or six fish per 1.5-mL tube) were preserved in RNA later™ at −20°C. Adult zebrafish were anesthetized with MS-222 and intestines were isolated by dissection and were preserved in RNA later™ at −20°C. Total RNA was isolated from larvae or intestinal samples from adult zebrafish using the RNeasy® Micro Kit (QIAGEN, Venlo, The Netherlands) according to the manufacturer's instructions. After quantifying RNA by a NanoDrop 1000 Spectrophotometer (Thermo Fisher Scientific, Waltham, MA, USA), cDNA was generated from 1 μg of RNA using Superscript™ III First Strand Synthesis Systems (Invitrogen, Carlsbad, CA, USA) according to the manufacturer's instructions. The diluted cDNA corresponding to 125 ng of RNA was used as a template for each reaction of quantitative polymerase chain reaction (qPCR) using the ABsoluteTM qPCR SYBR® Green Mix (Thermo Fisher Scientific, Waltham, MA, USA) as previously described (27). The sequences of the primer used in this study can be found in Table S1. The amplification data of each sample were normalized to the reference gene elf1α and calculated using the Pfaffl quantification method with efficiency correction (32), as described by Forlenza et al. (33). The statistical significance of differences between the control and fucoidan-treated groups was assessed by a one-way analysis of variance (ANOVA) test using R version 3.5.3 (34) where <0.05 was regarded as significant.
16S rRNA-Based Analyses of Zebrafish Microbiota
Zebrafish were euthanized with MS-222 and the whole larvae (four fish per 1.5-mL tube) were washed with sterilized phosphate-buffered saline and preserved at −20°C. Adult zebrafish were euthanized with MS-222 and the intestines were isolated by dissection. The intestinal contents were preserved in InhibitEX Buffer supplied in the QIAamp® DNA Fast Stool Mini Kit (QIAGEN, Venlo, The Netherlands) at −20°C. Total DNA was isolated from the whole larvae or intestines of adult zebrafish using the QIAamp® DNA Fast Stool Mini Kit according to the manufacturer's instructions. Pair-end sequencing was performed using Illumina MiSeq (BaseClear, Leiden, The Netherlands) using amplicons generated with the primer pair 341F−785R that target the V3–V4 variable region of the 16S rRNA gene of most bacteria (35). Raw Illumina sequencing reads were pair-ended, end-trimmed, filtered, and clustered into operational taxonomic units (OTUs) using the microbial genomic module 3.0 implemented in the CLC Bio Genomics Workbench v7.5.1 (Qiagen, Venlo, The Netherlands), 16S Microbiome Pipeline in the EZBioCloud web server (36), or the MICCA pipeline (37), for which OTU assignment was performed using the SILVA ribosomal RNA reference database [release 128, 97% similarity threshold, (38)], the EZBioCloud database (36), and the Ribosomal Database Project (RDP) classifier [version 2.11, 97% identity threshold, (39)], respectively. After confirming the reproducibility of the core microbiota composition of each sample, OTU tables in BIOM format generated by the CLC Bio Genomics Workbench was used for statistical analyses of the diversity and richness (alpha- and beta-diversity) implemented in MicrobiomeAnalyst© using the default filtering parameter settings (40). Significantly different taxa between control and fucoidan-treated group were identified by differential abundance (DESeq2) analysis by R version 3.5.3 (34) and by Linear Discriminant Analysis Effect Size (LEfSe) analysis implemented with EZBioCloud (36, 41).
Statistical Analysis
The quantified data collected from the fluorescent in vivo imaging of the zebrafish larvae and qPCR was analyzed using Student's t-test assuming unequal variation as well as one-way analysis of variance (ANOVA) test using Microsoft Excel® and R version 3.5.3 (34), where <0.05 was regarded as significant. The indices of α-diversity and β-diversity for comparing compositional structure of microbiota of each larva and adult zebrafish group were calculated on a species-level summarization of the rarefied OTU tables generated as described in the preceding text. Chao1 and Abundance-based Coverage Estimator (ACE) as well as Shannon and Simpson indices were used to measure the species-level community richness and species level community evenness, respectively, and each index was calculated using the online module of Microbiomeanalyst© (40). The plots of β-diversity indicating dissimilarities between samples were produced by principal coordinates analyses (PCoA) calculated using the Bray–Curtis dissimilarity index implemented in Microbiomeanalyst© (40).
Results
Effect of OM Fucoidan on Innate Immunity of Zebrafish Larvae
Zebrafish larvae (3−6 dpf) treated with OM fucoidan (0, 100, and 500 μg/mL of E3 water) by immersion showed normal development without visible signs of damage relative to untreated controls (data not shown). To investigate whether the treatment with OM fucoidan affected cellular immunity of zebrafish larvae, the numbers of neutrophils (mpx:GFP) and macrophages (mpeg1:mCherry) in the intestinal area of the control and the fucoidan-treated larvae were compared. There was no observable difference between the live-imaged control and the fucoidan-treated larvae (Figure 1A). The cell counts of neutrophils and macrophages in fucoidan-treated zebrafish larvae tended to be reduced compared to the control, but the difference was not significant (P > 0.1, Figure 1B).
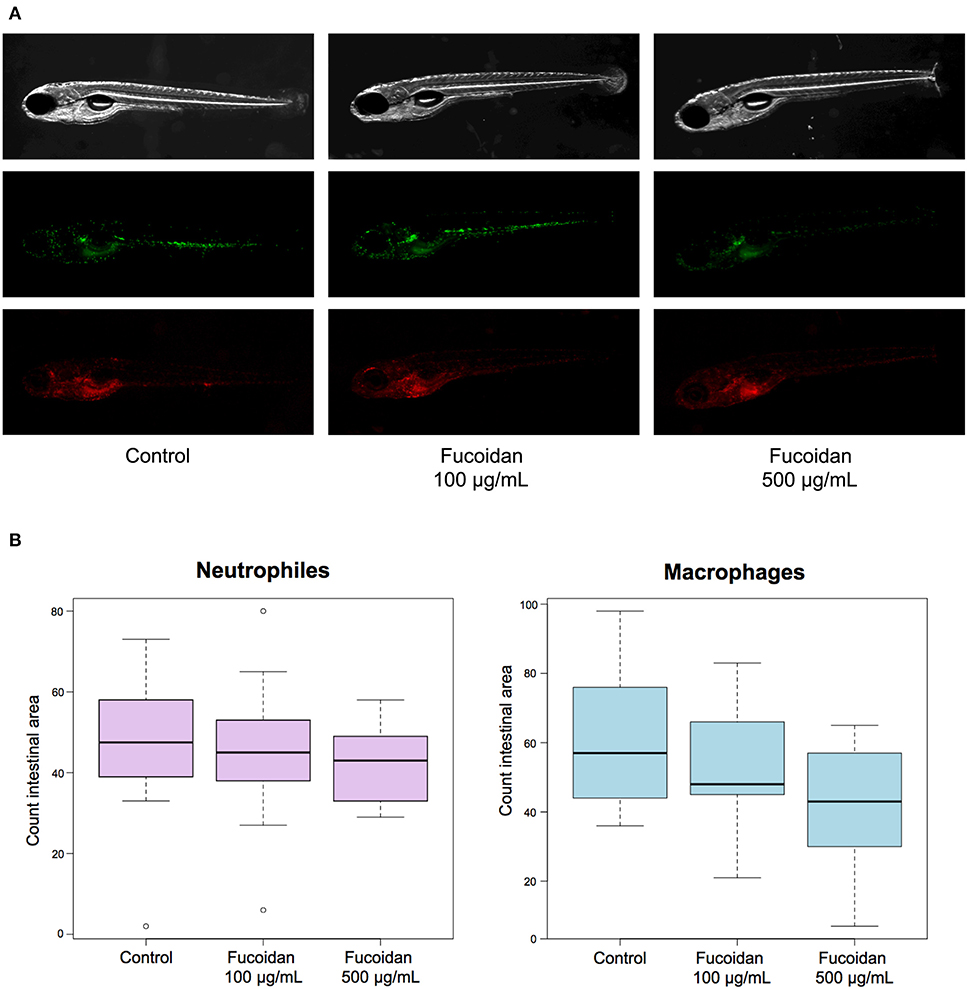
Figure 1. Effect of OM fucoidan on lymphocyte recruitment to the intestinal region of zebrafish larvae. (A) Representative pictures of 6 dpf zebrafish larvae (mpeg:mCherry, mpx:GFP) displaying neutrophils (green) and macrophages (red). F100, the zebrafish larvae treated with 100 μg/mL OM fucoidan from 3 dpf for 3 days; F500, the zebrafish larvae treated with 500 μg/mL OM fucoidan from 3 dpf for 3 days. (B) Quantification of neutrophils and macrophages in the intestinal area of larval zebrafish. Control, no treatment (n = 14); F100, treated with 100 μg/mL (n = 13); F500, treated with 500 μg/mL (n = 17).
Using the same experimental setup, we performed qPCR to measure the relative expression levels of selected genes representing host immune cell responses in the fucoidan (500 μg/mL) -treated zebrafish larvae and the untreated control. The relative gene expression levels of pro- (il1b, tnfa) and antiinflammatory (il10) cytokines as well as mmp9 were moderately (1.7–2.2 fold) upregulated in the larvae treated with 500 μg/mL of fucoidan (Figure 2A). No significant difference was observed for cxcl-8a (Figure 2A) and gene transcripts for il-17f , il-22, and tnfb were not detected in our samples (data not shown).
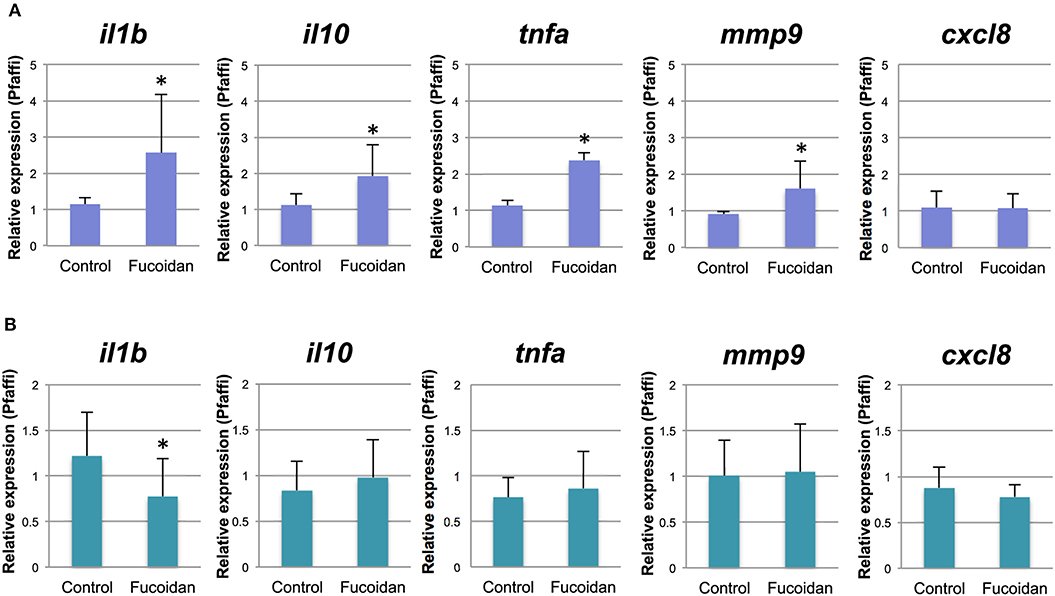
Figure 2. Relative gene expression of immune genes of zebrafish treated with OM fucoidan. (A) Control, 6 dpf zebrafish larvae; Fucoidan, 6 dpf zebrafish larvae immersed in 500 μg/mL fucoidan from 3 dpf for 3 days. (B) Control, intestinal samples of adult zebrafish fed with standard fish meal for 3 weeks; Fucoidan, intestinal samples of adult zebrafish fed with OM fucoidan meal (Tetramin fish flakes: OM fucoidan = 1:1). The asterisk denotes significant differences from the control samples (P < 0.05, 1-way ANOVA).
Adult zebrafish fed with OM fucoidan for 3 weeks did not show visible changes in fitness and behavior compared to controls fed the Tetramin Flakes only (data not shown). Immune responses of the adult zebrafish intestines were examined by quantitative PCR using primers specific for il1b, il-10, cxcl-8a, tnfa, and mmp9. Overall, there was no significant difference between the fucoidan-fed zebrafish and the control, except for il1b, which was expressed at slightly decreased levels (0.63-fold) in the fucoidan-fed zebrafish compared to the control (Figure 2B).
Effect of OM Fucoidan on Microbiota Diversity and Composition of Larval and Adult Zebrafish
The effects of OM fucoidan on diversity of larval and adult zebrafish microbiota were analyzed by 16S rRNA gene amplicon sequencing. Whole-body DNA samples of pools of 6 dpf zebrafish larvae were obtained from the three groups (n = 4 per group; immersion in 0, 100, and 500 μg/mL of OM fucoidan in E3 water) and used for Illumina MiSeq sequencing of 16S rRNA genes. For adult zebrafish, intestinal DNA from each of the two groups (n = 5 per group; control vs. OM-fucoidan fed) was used. A summary of the sequencing results is shown in Table S2 and the rarefaction curves of all samples, except one sample (WO3, a control sample of zebrafish larvae), reached saturation (Figure S1).
In microbiota of zebrafish larvae, no significant differences were observed in the species richness (Chao1, P = 0.47265; ACE; P = 0.74339; Figure 3A) or the species evenness (Shannon, P = 0.96621; Simpson, P = 0.96058; Figure 3B) between the control and fucoidan-treated fish, regardless of the fucoidan concentrations. In intestinal microbiota of adult zebrafish, the species richness was also not affected (Chao1, P = 0.88482; ACE; P = 0.90299; Figure 3C), while the species evenness tended to be moderately increased in the fucoidan-fed zebrafish (Shannon, P = 0.079088; Simpson, P = 0.078456; Figure 3D). β-Diversity analyses showed no significant association between fucoidan treatment and microbiota composition of zebrafish larvae (P < 0.49845; Figure 3E), which was in contrast to adult zebrafish, in which fucoidan-feeding was moderately associated with changes in the species composition of intestinal microbiota (P < 0.023, Figure 3F). Collectively, these results indicate that the treatments with OM fucoidan affected the diversity and composition of intestinal microbiota of adult zebrafish, but not the larvae zebrafish microbiota.
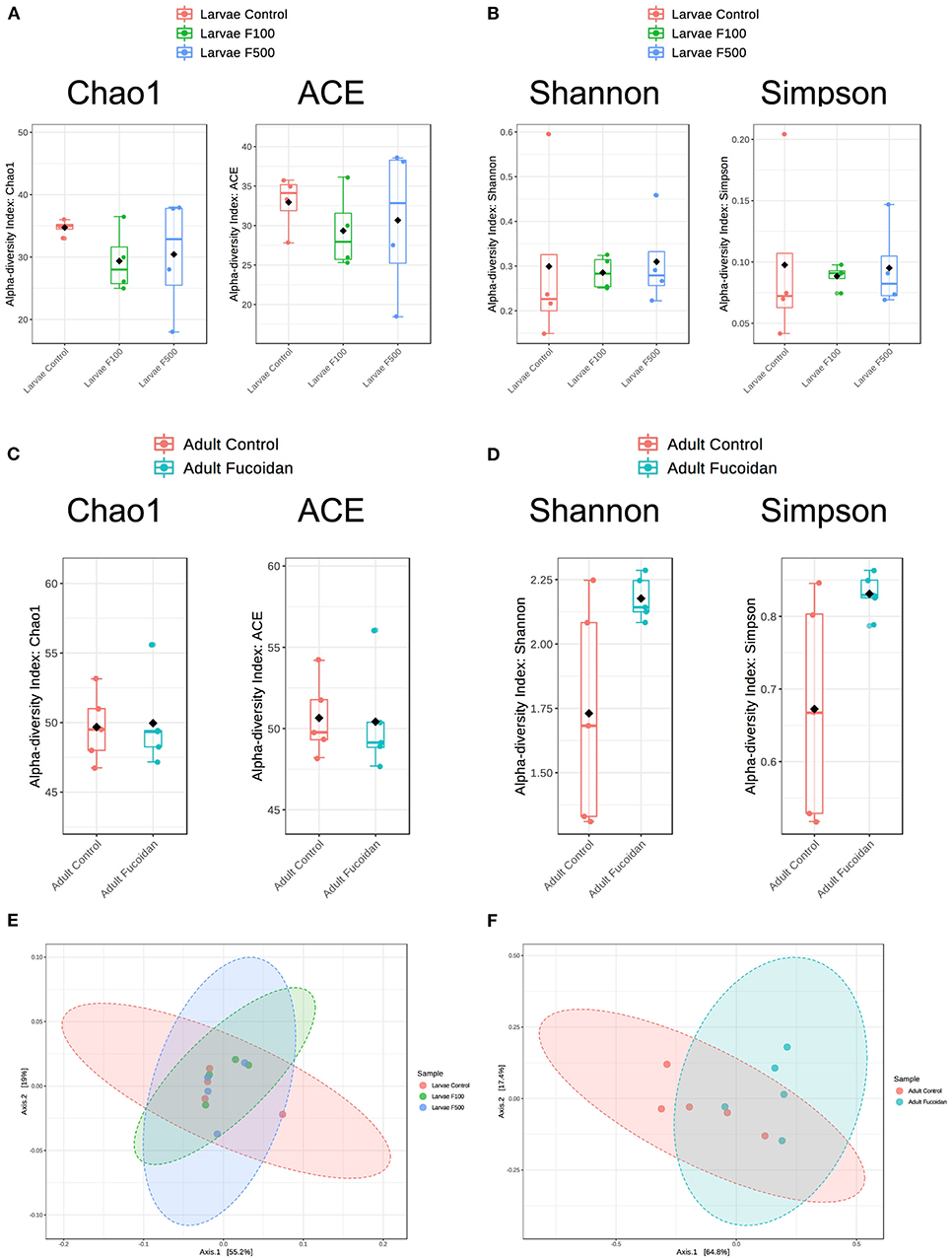
Figure 3. Effect of OM fucoidan on the diversity of larvae zebrafish microbiota and adult zebrafish intestinal microbiota. Species-level community richness (A,C) and species level community evenness (B,D) were compared between larvae zebrafish samples (A, B, E; F100, the zebrafish larvae treated with 100 μg/mL OM fucoidan from 3 dpf for 3 days; F500, the zebrafish larvae treated with 500 μg/mL OM fucoidan from 3 dpf for 3 days) and adult zebrafish intestinal samples (C, D, F; Fucoidan [Tetramin fish flakes: OM fucoidan = 1:1] for 3 weeks). Beta-diversity of larval (E) and adult intestinal (F) microbiota compared by the principal coordinates analyses (PCoA) based on Bray–Curtis dissimilarity index.
Taxonomic assignment of OTUs generated from each sample was performed using EzBioCloud database (https://www.ezbiocloud.net), which offers a high genus and species-level resolution (36). Consistent with our previous work (27), microbiota of zebrafish larvae was predominated (>95%) by Enterobacteriaceae (Figure 4A), which were affiliated with the Escherichia coli group (Figure S2, Table S3). Consistent with the diversity analyses (Figure 3), the relative abundance of each bacterial species and OTUs were similar between the larvae samples and did not reflect an effect of OM fucoidan (Figure 4A, Figure S2). In addition, DESeq2 and LEfSe analyses failed to identify significantly different taxa between the control and fucoidan-treated zebrafish larvae.
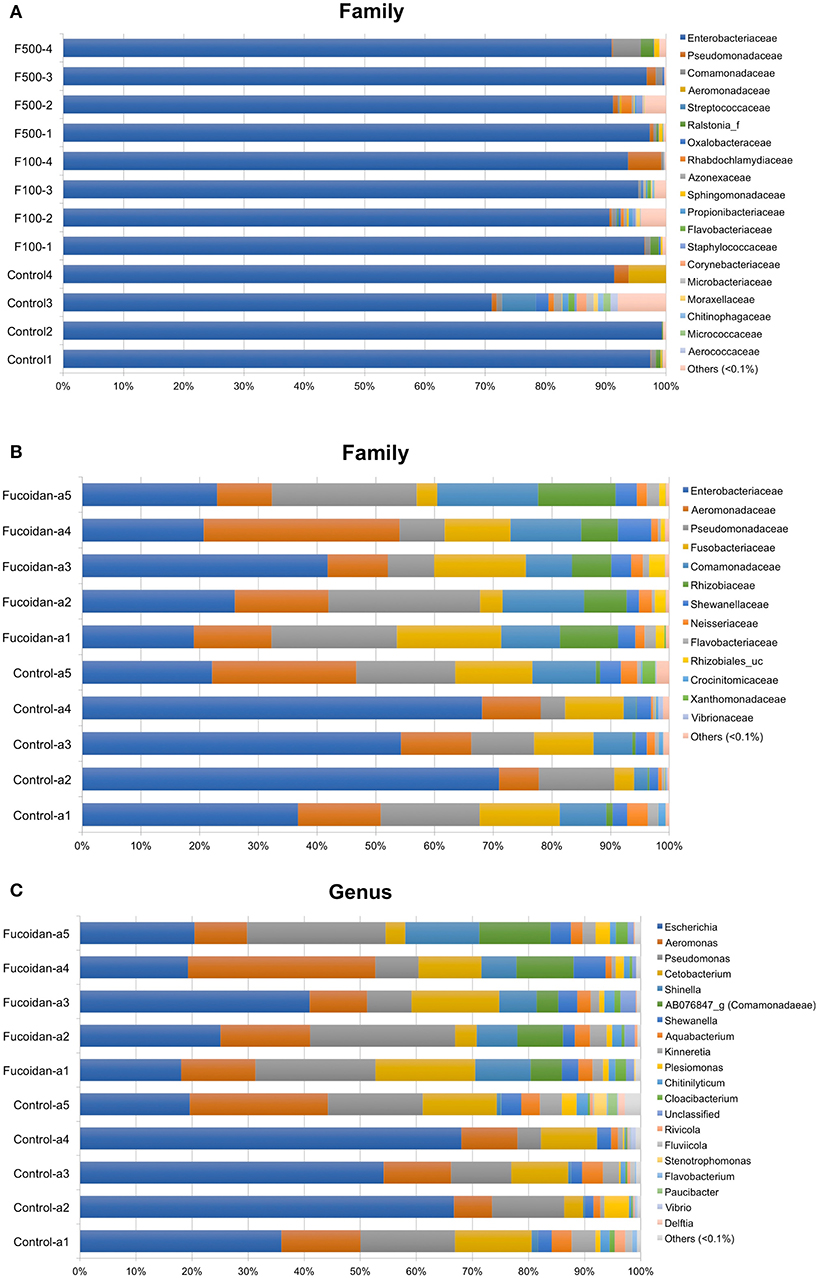
Figure 4. Effects of OM fucoidan on the bacterial community structure of larvae zebrafish microbiota and adult zebrafish intestinal microbiota. (A) Composition of family-level bacterial groups in the larvae zebrafish microbiota (F100, the zebrafish larvae treated with 100 μg/mL OM fucoidan from 3 dpf for 3 days; F500, the zebrafish larvae treated with 500 μg/mL OM fucoidan from 3 dpf for 3 days.). (B) Composition of family-level bacterial groups in the adult zebrafish intestinal microbiota. (C) Composition of genus-level bacterial groups in adult zebrafish intestinal microbiota. (B,C) Fucoidan [Tetramin fish flakes: OM fucoidan = 1:1] for 3 weeks). The taxonomic assignment is based on the latest EZbioCloud database (36). Taxa representing <0.1% of the total community are not visualized.
In contrast, a significant difference was observed in the intestinal microbiota between the control and fucoidan-fed adult zebrafish (Figures 4B,C, Figure S3). The class to species-level composition of intestinal microbiota of fucoidan-fed zebrafish was clearly different from the control (Figure S3), and this difference is characterized by the emergence and increase of relative abundance of several bacterial groups affiliated with Comamonadaceae and Rhizobiaceae (Figure 4B, Figure S3). At the genus and species level, these families were represented by unclassified genus of Comamonadaceae [AB076847, (42)] and the genus Shinella granuli group (Rhizobiaceae) (Figure 4C, Figure S3). The predominant families Comamonadaceae and Rhizobiaceae were also identified as the significantly different taxa represented in the fucoidan-fed zebrafish by the DESeq2 and LEfSe analyses (Figures 5A,B). Interestingly, both analyses revealed that the increase of Comamonadaceae and Rhizobiaceae were concomitant with the decrease of Enterobacteriaceae (Figures 5A,B, Figure S3). LEfSe analysis also found that unclassified groups of Rhizobiales and Betaproteobacteria were significantly associated with the fucoidan-feeding, while Flavobacteriia (phylum Bacteroides) were negatively affected (Figure 5B).
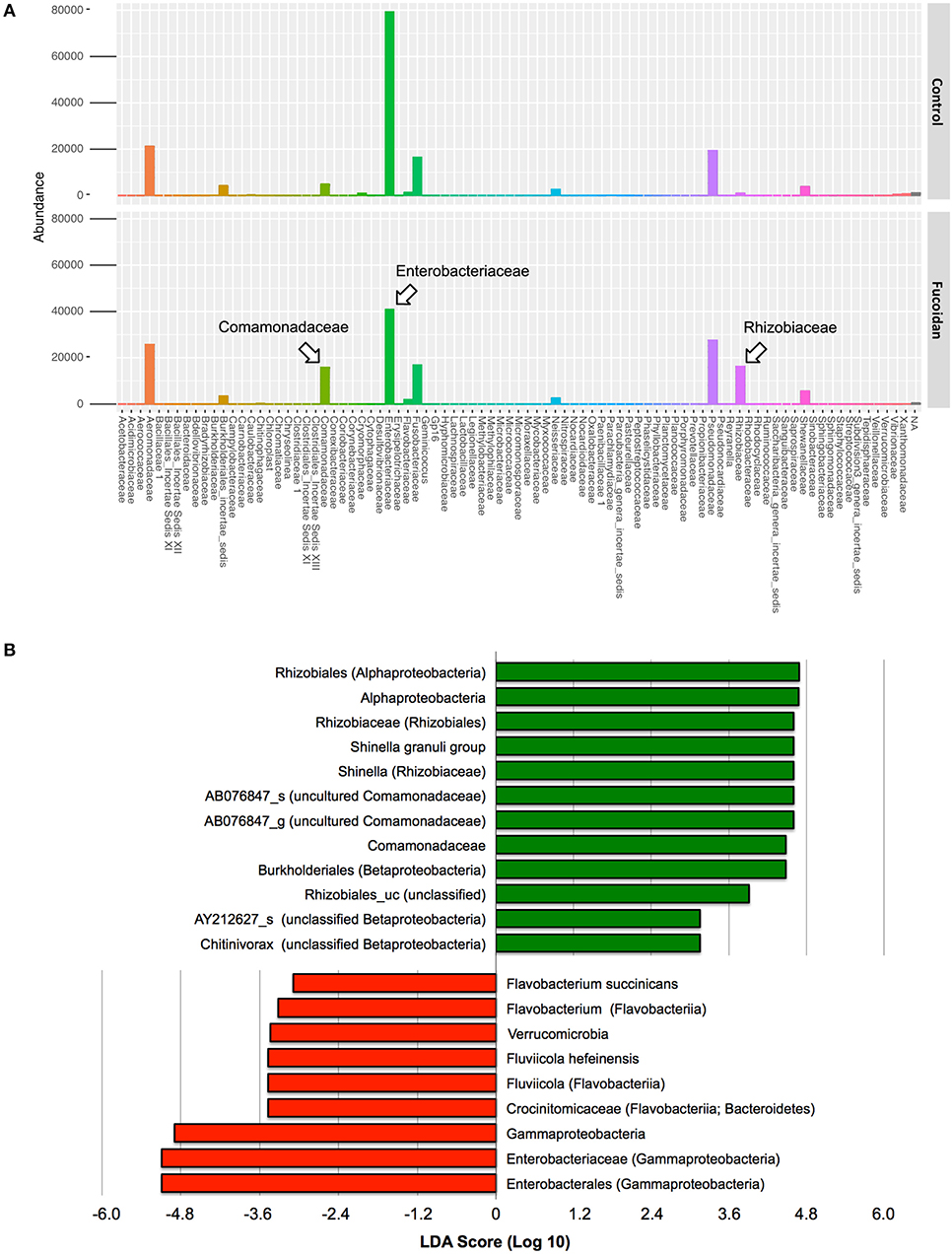
Figure 5. Significantly different taxa in the adult zebrafish intestinal microbiota associated with the OM fucoidan treatment. (A) Differently abundant bacterial families between the intestinal microbiota of the control and OM fucoidan-fed adult zebrafish, identified by differential abundance analysis using DESeq2 in R. The taxonomy assignment of the OTU dataset used is based on the RDP classifier (version 2.11, 97% identity threshold, 39). (B) Specific bacterial groups positively (green)- and negatively (red)- associated with OM fucoidan treatment. Identification of the significantly different taxa and LDA score calculation were performed by Linear discriminant analysis effect size (LEfSe) tool implemented with EZBioCloud (36). The taxonomic assignment is based on the latest EZbioCloud database (36).
Discussion
Our current study showed that fucoidan derived from Japanese brown seaweed C. okamuranus has the potential to modulate the intestinal microbiota of adult zebrafish. The profound compositional change associated with the fucoidan-feeding in adult zebrafish can be characterized by the increased abundance of bacterial groups affiliated with Comamonadaceae and Rhizobiaceae and a decreased abundance of Enterobacteriaceae. Although non-pathogenic bacteria of the E. coli species have been proposed to confer a protective effect on zebrafish larvae via lipopolysaccharide (LPS) tolerance and acid production (43, 44), numerous studies have reported proinflammatory effects of Enterobacteriaceae in fish (45, 46). In addition, Enterobacteriaceae are thought to be responsible for the spread of antimicrobial resistance in aquatic environments (47). In this context, it is intriguing that the relative expression of il1b, a proinflammatory cytokine, was moderately downregulated in the fucoidan- fed adult zebrafish (Figure 2B), which suggests that OM fucoidan may have directly or indirectly suppressed the dominance of Enterobacteriaceae that can induce proinflammatory responses. This type of diet–microbiota–host interplay is likely to play a crucial role in pro- and antiinflammatory states in animal intestines [(48) for review], and it is therefore of great interest further investigate in future studies on the mechanism that OM fucoidan decreases the relative abundance of Enterobacteriaceae in the fish intestine with regard to how it may impact the health of the fish population.
While metabolic and physiological properties of the intestinal bacteria of adult zebrafish that responded to the fucoidan feeding are yet to be determined, the increase of Comamonadaceae and Rhizobiaceae suggests their involvement in the degradation of OM fucoidan. Interestingly, Comamonadaceae and Rhizobiaceae have been frequently found in a nitrogen removal process in wastewater treatment systems called solid-phase denitrification (49), where solid biodegradable polymers are used as carbon sources for denitrifying bacteria (50). This system is also applicable for nitrogen removal in aquaculture, where increased nitrate concentration poses negative effects on fish (51). Indeed, the unclassified Comamonadaceae [AB076847, (42)] and Shinella spp., the intestinal abundance of which increased in response to the fucoidan feeding of adult zebrafish in our current study, have been reported to belong to denitrifying bacteria possessing biodegrading abilities of diverse compounds including biopolymers and xenobiotics (42, 52, 53). Therefore, it seems plausible that OM fucoidan may serve as a carbon source for intestinal bacteria of adult zebrafish, and identification of the degradation pathways involved awaits further investigation
Although a large body of studies has documented that the host innate immunity plays significant roles for shaping microbiota and vice versa (26, 54), our current results imply that the compositional structure of microbiota is not strongly correlated to the expression patterns of host immune genes. Feeding of OM fucoidan for 3 weeks profoundly altered gut microbiota composition in adult zebrafish; however, the analysis of a selected set of immune genes only showed a slight reduction in the expression of il1b. In contrast, the exposure of zebrafish larvae to OM fucoidan resulted in changes in expression of immune genes (il1b, il10, tnfa, and mmp9) but did not affect the microbiota composition. These results might be partly explained by the timing and duration of the exposure. Since larval feeding starts in the immersion window, it is expected that the immune system of these developing larvae is responding to novel antigens that it is exposed to. In contrast, in adult fish, the immune system has fully developed and a proper homeostasis is reached at the mucosal surfaces such as the intestines (55). Furthermore, since we only evaluated the immune response in the intestines at 3 weeks after feeding, the initial immune modulatory effect of primary exposure to fucoidan might have been missed, while the microbiota had 3 weeks to adapt to the new substrate provided. Future studies will include multiple time points to address early vs. late immune modulatory effects at different time points in life (of fish).
Furthermore, as has been shown in a study by Burns et al. using innate immune-deficient Myd88 knockout zebrafish (56), the gut microbiota composition can be better explained by the interhost dispersal effect, i.e., transmission and sharing microbiota among hosts, than immune gene expression patterns. Also, Stagaman et al. have reported that the effects of adaptive immunity on microbiota composition can be overwhelmed by other factors derived from co-housing within the same tank (57). Our study also reflected this phenomenon, since all adult zebrafish fed with OM fucoidan showed the same compositional changes in the relative abundance of specific bacterial groups (Figures 4B,C, Figure S3). The interhost dispersal of Comamonadaceae and Rhizobiaceae among adult zebrafish associated with the fucoidan-feeding suggests that these specific bacterial groups are subject to filtering by local host environments. Further studies are warranted to determine whether the interhost dispersal and OM fucoidan reciprocally affected the microbial composition.
In contrast to previous studies reporting inhibitory effects of fucoidan on inflammatory responses of injury zebrafish models (58, 59), the influence of OM fucoidan treatments on the baseline zebrafish immune responses were rather mild in our current study. In addition, while a previous study has implied a high concentration of fucoidan may be cytotoxic (60), immersion of zebrafish larvae (3–6 dpf) in OM fucoidan at concentrations of 100 and 500 μg/mL did not affect their fitness. In previous studies using LPS-challenged zebrafish models (58, 59), the antiinflammation effects of fucoidan may be rather explained by the interference of LPS–host interaction rather than direct modulation of host immunity. Another interpretation of the less profound effect on the baseline immune response of zebrafish to fucoidan is that zebrafish immunity may have evolved to become tolerant to the constituents of blown algae abundant in their original habitats (61).
Our current finding that OM fucoidan modulated the gut microbiota composition of zebrafish is in line with studies using rodents (22, 23). The improvement of diabetic symptoms attributed to the modulation of gut microbiota by fucoidan that have been shown in previous studies (24, 25) implies that fucoidan feeding and the subsequent alteration of intestinal microbiota may also affect metabolic properties of fish. Future studies toward a better understanding of the commonalities between intestinal microbial metabolism and host responses shared by fish and animals (62, 63) will help us to evaluate the potential of OM fucoidan as a new prebiotic in aquaculture (64).
Conclusions
Treatment with OM fucoidan moderately modulated the relative expression of innate immune genes in larvae zebrafish, while no change in microbiota composition was observed. In adult zebrafish, feeding OM fucoidan increased the relative abundance of Comamonadaceae and Rhizobiaceae at the expense of Enterobacteriaceae, which was accompanied by a slight decrease of relative expression of a proinflammatory gene il1b, which suggests a potential of OM fucoidan to shift the microbial composition to an antiinflammatory state by selectively suppressing populations of bacteria that are associated with proinflammatory responses. To our knowledge, this is the first study to describe in vivo modulatory effects of fucoidan on microbiota and immune responses of unchallenged zebrafish.
Data Availability Statement
The raw sequence data files were deposited in the NCBI sequence read archive (SRA) database under BioProject number PRJNA597701 (SRA accession numbers listed in Supplementary File “data sheet 3.xlsx”).
Ethics Statement
The animal study was reviewed and approved by Dutch Committee on Animal Welfare (2017.W-0034) and the Animal Welfare Body (IvD) of the Wageningen University (Netherlands).
Author Contributions
WI-O, AL, MK, and SB designed the research. WI-O, AL, and SB conducted the experiments. WI-O, AL, EZ, MK, and SB analyzed the data. WI-O and SB wrote the article. MK and SB provided the funding. All authors critically reviewed the manuscript and approved the manuscript.
Funding
This work was financially supported by the Japan Society for the Promotion of Science (JSPS) through JSPS Core-to-Core Program (Advanced Research Networks) entitled Establishment of International Agricultural Immunology Research-Core for a Quantum Improvement in Food Safety. WI-O was supported by WIAS (Wageningen Institute of Animal Sciences) fellowship (WIAS1807229) provided by Graduate School of Animal Science of Wageningen University and Research and Leading Young Researcher Overseas Visit Program of Tohoku University.
Conflict of Interest
MI is employed by the company South Product Co., Ltd.
The remaining authors declare that the research was conducted in the absence of any commercial or financial relationships that could be construed as a potential conflict of interest.
Supplementary Material
The Supplementary Material for this article can be found online at: https://www.frontiersin.org/articles/10.3389/fnut.2020.00067/full#supplementary-material
References
1. Ale MT, Meyer AS. Fucoidans from brown seaweeds: an update on structures, extraction techniques and use of enzymes as tools for structural elucidation. RSC Adv. (2013) 3:8131–41. doi: 10.1039/C3RA23373A
2. Vo TS, Kim SK. Fucoidans as a natural bioactive ingredient for functional foods. J Funct Foods. (2012) 5:16–27. doi: 10.1016/j.jff.2012.08.007
3. Fitton JH, Stringer DS, Park AY, Karpiniec SN. Therapies from Fucoidan: new developments. Mar Drugs. (2019) 17:571. doi: 10.3390/md17100571
4. Chollet L, Saboural P, Chauvierre C, Villemin JN, Letourneur D, Chaubet F. Fucoidans in nanomedicine. Mar Drugs. (2016) 14:145. doi: 10.3390/md14080145
5. Lange KW, Hauser J, Nakamura Y, Kanaya S. Dietary seaweeds and obesity. Food Sci Hum Wellness. (2015) 4:87–96. doi: 10.1016/j.fshw.2015.08.001
6. Willcox BJ, Willcox DC, Suzuki M. Demographic, phenotypic, and genetic characteristics of centenarians in Okinawa and Japan: part 1- centenarians in Okinawa. Mech Ageing Dev. (2017) 165:75–9. doi: 10.1016/j.mad.2016.11.001
7. Nagaoka M, Shibata H, Kimura-Tagaki I, Hashimoto S, Kimura K, Makino T, et al. Structural study of fucoidan from Cladosiphon okamuranus TOKIDA. Glycoconj J. (1999) 45:325–36. doi: 10.3177/jnsv.45.325
8. Takeda K, Tomimori K, Kimura R, Ishikawa C, Nowling TK, Mori N, et al. Anti-tumor activity of fucoidan is mediated by nitric oxide released from macrophages. Int J Oncol. (2012) 40:251–60. doi: 10.3892/ijo.2011.1168
9. Nagamine T, Kadena K, Tomori M, Nakajima K, Iha M. Activation of NK cells in male cancer survivors by fucoidan extracted from Cladosiphon okamuranus. Mol Clin Oncol. (2020) 12:81–8. doi: 10.3892/mco.2019.1943
10. Haneji K, Matsuda T, Tomita M, Kawakami H, Ohshiro K, Uchihara JN, et al. Fucoidan extracted from Cladosiphon okamuranus tokida induces apoptosis of human T-cell leukemia virus type 1-infected T-cell lines and primary adult T-cell leukemia cells. Nutr Cancer. (2005) 52:189–201. doi: 10.1207/s15327914nc5202_9
11. Araya N, Takahashi K, Sato T, Nakamura T, Sawa C, Hasegawa D, et al. Fucoidan therapy decreases the proviral load in patients with human t-lymphotropic virus type-1-associated neurological disease. Antivir Ther. (2011) 16:89–98. doi: 10.3851/IMP1699
12. Hidari KI, Takahashi N, Arihara M, Nagaoka M, Morita K, Suzuki T. Structure and anti-dengue virus activity of sulfated polysaccharide from a marine alga. Biochem Biophys Res Commun. (2008) 376:91–5. doi: 10.1016/j.bbrc.2008.08.100
13. Mori N, Nakasone K, Tomimori K, Ishikawa C. Beneficial effects of fucoidan in patients with chronic hepatitis c virus infection. World J Gastroenterol. (2012) 18:2225–30. doi: 10.3748/wjg.v18.i18.2225
14. Elizondo-Gonzalez R, Cruz-Suarez LE, Ricque-Marie D, Mendoza-Gamboa E, Rodriguez-Padilla C, Trejo-Avila LM. In vitro characterization of the antiviral activity of fucoidan from Cladosiphon okamuranus against newcastle disease virus. Virol J. (2012) 9:307. doi: 10.1186/1743-422X-9-307
15. Trejo-Avila LM, Elizondo-Gonzalez R, Rodriguez-Santillan P, Aguilar-Briseno JA, Ricque-Marie D, Rodriguez-Padilla C, et al. Innocuity and anti-Newcastle-virus-activity of Cladosiphon okamuranus fucoidan in chicken embryos. Poult Sci. (2016) 95:2795–802. doi: 10.3382/ps/pew201
16. Trejo-Avila LM, Morales-Martínez ML, Ricque-Marie D, Cruz-Suarez LE, Zapata-Benavides P, Morán-Santibañez K, et al. In vitro anti-canine distemper virus activity of fucoidan extracted from the brown alga Cladosiphon okamuranus. Virus Dis. (2014) 25:474–80. doi: 10.1007/s13337-014-0228-6
17. Shang Q, Jiang H, Cai C, Hao J, Li G, Yu G. Gut microbiota fermentation of marine polysaccharides and its effects on intestinal ecology: an overview. Carbohydr Polym. (2018) 179:173–85. doi: 10.1016/j.carbpol.2017.09.059
18. Ma N, Guo P, Zhang J, He T, Kim SW, Zhang G, et al. Nutrients mediate intestinal bacteria–mucosal immune crosstalk. Front Immunol. (2018) 9:5. doi: 10.3389/fimmu.2018.00005
19. Michel C, Lahaye M, Bonnet C, Mabeau S, Barry JL. In vitro fermentation by human faecal bacteria of total and purified dietary fibers from brown seaweeds. Br J Nutr. (1996) 75:263–80. doi: 10.1079/BJN19960129
20. de Jesus Raposo MF, de Morais AMMB, de Morais RMSC. Emergent sources of prebiotics: seaweeds and microalgae. Mar Drugs. (2016) 14:27. doi: 10.3390/md14020027
21. Brugman S, Ikeda-Ohtsubo W, Braber S, Folkerts G, Pieterse CMJ, Bakker PAHM. A comparative review on microbiota manipulation: lessons from fish, plants, livestock and human research. Front Nutr. (2018) 5:80. doi: 10.3389/fnut.2018.00080
22. Chen Q, Liu M, Zhang P, Fan S, Huang J, Yu S, et al. Fucoidan and galacto-oligosaccharides ameliorate high-fat diet-induced dyslipidemia in rats by modulating the gut microbiota and bile acid metabolism. Nutrition. (2019) 65:50–9. doi: 10.1016/j.nut.2019.03.001
23. Yokota T, Nomura K, Nagashima M, Kamimura N. Fucoidan alleviates high-fat diet-induced dyslipidemia and atherosclerosis in ApoE shl mice deficient in apolipoprotein E expression. J Nutr Biochem. (2016) 32:46–54. doi: 10.1016/j.jnutbio.2016.01.011
24. Hu S, Wang J, Wang J, Yang H, Yan X, Su L. Fucoidan from Acaudina molpadioides improves insulin resistance by altering gut microbiota dysfunction. J Funct Foods. (2019) 57:59–67. doi: 10.1016/j.jff.2019.03.033
25. Cheng Y, Luthuli S, Hou LF, Jiang HJ, Chen PC, Zhang X, et al. Sargassum fusiforme fucoidan modifies the gut microbiota during alleviation of streptozotocin-induced hyperglycemia in mice. Int J Biol Macromol. (2019) 131:1162–70. doi: 10.1016/j.ijbiomac.2019.04.040
26. López Nadal A, Ikeda-Ohtsubo W, Sipkema D, Peggs D, Mcgurk C, Forlenza M, et al. Feed, microbiota and gut immunity: using the zebrafish model to understand fish health. Front Immunol. (2020) 11:114. doi: 10.3389/fimmu.2020.00114
27. López Nadal A, Peggs D, Wiegertjes GF, Brugman S. Exposure to antibiotics affects saponin immersion-induced immune stimulation and shift in microbial composition in zebrafish larvae. Front Microbiol. (2018) 9:2588. doi: 10.3389/fmicb.2018.02588
28. Renshaw SA, Loynes CA, Trushell DM, Elworthy S, Ingham PW, Whyte MK. A transgenic zebrafish model of neutrophilic inflammation. Blood. (2006) 108:3976–8. doi: 10.1182/blood-2006-05-024075
29. Bernut A, Herrmann JL, Kissa K, Dubremetz JF, Gaillard JL, Lutfalla G, et al. Mycobacterium abscessus cording prevents phagocytosis and promotes abscess formation. Proc Natl Acad Sci USA. (2014) 111:E943–52. doi: 10.1073/pnas.1321390111
30. Nagamine T, Hayakawa K, Kusakabe T, Takada H, Nakazato K, Hisanaga E, et al. Inhibitory effect of fucoidan on Huh7 hepatoma cells through downregulation of CXCL12. Nutr Cancer. (2009) 61:340–7. doi: 10.1080/01635580802567133
31. Abràmoff MD, Magalhães PJ, Ram SJ. Image processing with ImageJ. Biophotonics Int. (2004) 11:36–42.
32. Pfaffl MW. A new mathematical model for relative quantification in real-time RT-PCR. Nucleic Acids Res. (2001) 29:e45. doi: 10.1093/nar/29.9.e45
33. Forlenza M, Kaiser T, Savelkoul HF, Wiegertjes GF. The use of real-time quantitative PCR for the analysis of cytokine mRNA levels. Methods Mol Biol. (2012) 820:7–23. doi: 10.1007/978-1-61779-439-1_2
34. R Core Team. R: a language and environment for statistical computing. R Foundation for Statistical Computing, Vienna. (2019) Available online at: https://www.R-project.org/ (accessed May 22, 2020).
35. Klindworth A, Pruesse E, Schweer T, Peplies J, Quast C, Horn M, et al. Evaluation of general 16S ribosomal RNA gene PCR primers for classical and next-generation sequencing-based diversity studies. Nucleic Acids Res. (2013) 41:e1. doi: 10.1093/nar/gks808
36. Yoon SH, Ha SM, Kwon S, Lim J, Kim Y, Seo H, et al. Introducing EzBioCloud: a taxonomically united database of 16S rRNA and whole genome assemblies. Int J Syst Evol Microbiol. (2017) 67:1613–7. doi: 10.1099/ijsem.0.001755
37. Albanese D, Fontana P, de Filippo C, Cavalieri D, Donati C. MICCA: a complete and accurate software for taxonomic profiling of metagenomic data. Sci Rep. (2015) 5:9743. doi: 10.1038/srep09743
38. Quast C, Pruesse E, Yilmaz P, Gerken J, Schweer T, Yarza P, et al. The SILVA ribosomal RNA gene database project: improved data processing and web-based tools. Nucleic Acids Res. (2013) 41:D590–6. doi: 10.1093/nar/gks1219
39. Wang Q, Garrity GM, Tiedje JM, Cole JR. Naive Bayesian classifier for rapid assignment of rRNA sequences into the new bacterial taxonomy. Appl Environ Microbiol. (2007) 73:5261–7. doi: 10.1128/AEM.00062-07
40. Dhariwal A, Chong J, Habib S, King IL, Agellon LB, Xia J. MicrobiomeAnalyst: a web-based tool for comprehensive statistical, visual and meta-analysis of microbiome data. Nucleic Acids Res. (2017) 45:W180–8. doi: 10.1093/nar/gkx295
41. Segata N, Izard J, Waldron L, Gevers D, Miropolsky L, Garrett WS, et al. Metagenomic biomarker discovery and explanation. Genome Biol. (2011) 12:R60. doi: 10.1186/gb-2011-12-6-r60
42. Khan ST, Horiba Y, Yamamoto M, Hiraishi A. Members of the family Comamonadaceae as primary poly (3-hydroxybutyrate-co-3-hydroxyvalerate) -degrading denitrifiers in activated sludge as revealed by a polyphasic approach. Appl Environ Microbiol. (2002) 68:3206–14. doi: 10.1128/AEM.68.7.3206-3214.2002
43. Novoa B, Figueras A. Zebrafish: model for the study of inflammation and the innate immune response to infectious diseases. Adv Exp Med Biol. (2012) 946:253–75. doi: 10.1007/978-1-4614-0106-3_15
44. Nag D, Breen P, Raychaudhuri S, Withey JH. Glucose metabolism by Escherichia coli inhibits Vibrio cholerae intestinal colonization of zebrafish. Infect Immun. (2018) 86:e00486–18. doi: 10.1128/IAI.00486-18
45. Swain P, Nayak SK, Nanda PK, Dash S. Biological effects of bacterial lipopolysaccharide (endotoxin) in fish: a review. Fish Shellfish Immunol. (2008) 25:191–201. doi: 10.1016/j.fsi.2008.04.009
46. Pressley M, Phelan P, Witten P, Mellon M, Kim C. Pathogenesis and inflammatory response to Edwardsiella tarda infection in the zebrafish. Dev Comp Immunol. (2005) 29:501–13. doi: 10.1016/j.dci.2004.10.007
47. Dib AL, Agabou A, Chahed A, Kurekci C, Moreno E, Espigares M, et al. Isolation, molecular characterization and antimicrobial resistance of enterobacteriaceae isolated from fish and seafood. Food Control. (2018) 88:54–60. doi: 10.1016/j.foodcont.2018.01.005
48. Makki K, Deehan EC, Walter J, Bäckhed F. The impact of dietary fiber on gut microbiota in host health and disease. Cell Host Microbe. (2018) 23:705–15. doi: 10.1016/j.chom.2018.05.012
49. Hiraishi A, Khan ST. Application of polyhydroxyalkanoates for denitrification in water and wastewater treatment. Appl Microbiol Biotechnol. (2003) 61:103–9. doi: 10.1007/s00253-002-1198-y
50. Chu L, Wang J. Denitrification performance and biofilm characteristics using biodegradable polymers PCL as carriers and carbon source. Chemosphere. (2013) 91:1310–6. doi: 10.1016/j.chemosphere.2013.02.064
51. Luo GZ, Hou ZW, Tian LQ, Tan HX. Comparison of nitrate removal efficiency and bacterial properties using PCL and PHBV polymers as a carbon source to treat aquaculture water. Aquaculture Fish. (2020) 5:92-8. doi: 10.1016/j.aaf.2019.04.002
52. Wu H, Shen J, Wu R, Sun X, Li J, Han W, et al. Biodegradation mechanism of 1H-1,2,4-triazole by a newly isolated strain Shinella sp. NJUST26. Sci Rep. (2016) 6:29675. doi: 10.1038/srep29675
53. Ntougias S, Melidis P, Navrozidou E, Tzegkas F. Diversity and efficiency of anthracene-degrading bacteria isolated from a denitrifying activated sludge system treating municipal wastewater. Int Biodeterior Biodegradation. (2015) 97:151–8. doi: 10.1016/j.ibiod.2014.11.009
54. Hooper LV, Littman DR, Macpherson AJ. Interactions between the microbiota and the immune system. Science. (2012) 336:1268–73. doi: 10.1126/science.1223490
55. Brugman S. The zebrafish as a model to study intestinal inflammation. Dev Comp Immunol. (2016) 64:82–92. doi: 10.1016/j.dci.2016.02.020
56. Burns AR, Miller E, Agarwal M, Rolig AS, Milligan-Myhre K, Seredick S, et al. Interhost dispersal alters microbiome assembly and can overwhelm host innate immunity in an experimental zebrafish model. Proc Natl Acad Sci. (2017) 114:11181–6. doi: 10.1073/pnas.1702511114
57. Stagaman K, Burns AR, Guillemin K, Bohannan BJ. The role of adaptive immunity as an ecological filter on the gut microbiota in zebrafish. ISME J. (2017) 11:1630–9. doi: 10.1038/ismej.2017.28
58. Jeong JW, Hwang SJ, Han MH, Lee DS, Yoo JS, Choi IW, et al. Fucoidan inhibits lipopolysaccharide-induced inflammatory responses in RAW 264.7 macrophages and zebrafish larvae. Mol Cell Toxicol. (2017) 13:405–17. doi: 10.1007/s13273-017-0045-2
59. Lee SH, Ko CI, Jee Y, Jeong Y, Kim M, Kim JS. Anti-inflammatory effect of fucoidan extracted from Ecklonia cava in zebrafish model. Carbohydr Polym. (2013) 92:84–9. doi: 10.1016/j.carbpol.2012.09.066
60. Kim MH, Joo HG. Immunostimulatory effects of fucoidan on bone marrow-derived dendritic cells. Immunol Lett. (2008) 115:138–43. doi: 10.1016/j.imlet.2007.10.016
61. Spence R, Gerlach G, Lawrence C, Smith C. The behaviour and ecology of the zebrafish, Danio rerio. Biol Rev Camb Philos Soc. (2008) 83:13–34. doi: 10.1111/j.1469-185X.2007.00030.x
62. Ikeda-Ohtsubo W, Brugman S, Warden CH, Rebel JMJ, Folkerts G, Pieterse CMJ. How can we define “optimal microbiota?”: a comparative review of structure and functions of microbiota of animals, fish, and plants in agriculture. Front Nutr. (2018) 5:90. doi: 10.3389/fnut.2018.00113
63. Rawls JF, Mahowald MA, Ley RE, Gordon JI. Reciprocal gut microbiota transplants from zebrafish and mice to germ-free recipients reveal host habitat selection. Cell. (2006) 127:423–33. doi: 10.1016/j.cell.2006.08.043
Keywords: fucoidan, immunomodulation, microbiota, seaweed polysaccharides, zebrafish
Citation: Ikeda-Ohtsubo W, López Nadal A, Zaccaria E, Iha M, Kitazawa H, Kleerebezem M and Brugman S (2020) Intestinal Microbiota and Immune Modulation in Zebrafish by Fucoidan From Okinawa Mozuku (Cladosiphon okamuranus). Front. Nutr. 7:67. doi: 10.3389/fnut.2020.00067
Received: 20 December 2019; Accepted: 20 April 2020;
Published: 24 June 2020.
Edited by:
Jia Sun, Jiangnan University, ChinaReviewed by:
Hailong Song, University of Pennsylvania, United StatesXi Ma, China Agricultural University, China
Copyright © 2020 Ikeda-Ohtsubo, López Nadal, Zaccaria, Iha, Kitazawa, Kleerebezem and Brugman. This is an open-access article distributed under the terms of the Creative Commons Attribution License (CC BY). The use, distribution or reproduction in other forums is permitted, provided the original author(s) and the copyright owner(s) are credited and that the original publication in this journal is cited, in accordance with accepted academic practice. No use, distribution or reproduction is permitted which does not comply with these terms.
*Correspondence: Wakako Ikeda-Ohtsubo, wakako.ohtsubo@tohoku.ac.jp; Sylvia Brugman, sylvia.brugman@wur.nl