- Shobhaben Pratapbhai School of Pharmacy and Technology Management, SVKM'S Narsee Monjee Institute of Management Studies-NMIMS, Mumbai, India
There is a high occurrence of obesity worldwide without many new medications being approved for its treatment. Therefore, there is an urgent need to introduce new approaches for treating obesity. Bioactive peptides have been used to treat metabolic disorders- such as type-2 diabetes and obesity; while also possessing anti-oxidant, anti-inflammatory, anti-microbial, and anti-viral properties. However, the development of these peptides has taken backstage due to their size, reduced stability, poor delivery and bioavailability, fast rate of degradation etc. But with the emergence of newer techniques for multifunctional peptides, mimetics, peptide analogs, and aptamers, there is a sudden revival in this therapeutic field. An increased attention is required for development of the natural peptides from food and marine sources which can mimic the function of mediators involved in weight management to avoid obesity. Herein, the search for the structures of anti-obesity peptides was carried out in order to establish their potential for drug development in future. An extensive search for the current status of endogenous, food and marine peptides, with reference to novel and interesting experimental approaches based on peptidomimetics for controlling obesity, was performed. Apolipoprotein A-I (apoA-I), melanocortin-4 receptor (MC4R)-specific agonist, GLP-1 dual and triple agonists, neuropeptides and prolactin-releasing peptide mimetics were specifically examined for their anti-obesity role. Novel peptides, mimetics, and synthesis interventions are transpiring and might offer safer alternatives for otherwise scarcely available safe antiobesity drug. A deeper understanding of peptides and their chemistry through the use of peptide engineering can be useful to overcome the disadvantages and select best mimetics and analogs for treatment in future.
Introduction
Obesity is an abnormal condition which involves accumulation of excessive body fat and increases the risk of associated health problems. The worldwide prevalence of obesity has almost tripled since 1975, which is an alarming and dreadful sign of impairment of human health. It increases the likelihood of diseases like type-2 diabetes, cardiovascular diseases, obstructive sleep apnea, osteoarthritis and depression (1, 2). Recently, Reilly et al. (3) pointed out at the low sensitivity of body-mass index (BMI) for classifying obesity and suggests to consider large biases for the condition. The undesirable side effects of drugs like Orlistat, phentermine/topiramate, lorcaserin, bupropion/naltrexone, liraglutide 3.0, phentermine, and diethylpropion restrict their uses and thus limit the availability of safe anti-obesity drugs (4).
Endogenous as well as bioactive peptides from other sources having twenty amino acid residues exhibit anti-obesity property (5–7). Analogs for novel targets such as amylin, leptin, GLP-1 MC4R, oxyntomodulin, neuropeptide Y antagonists, cannabinoid type-1 receptor blockers, MetAP2 inhibitors, lipase inhibitors and anti-obesity vaccines are currently being studied and it is predicted that the combined use of two or more classes of drugs involving various pathways might be beneficial (8). In Figure 1 the role of major endogenous peptides, which help in regulating obesity by different pathways and exhibit combined effect is composed. α- melanocyte stimulating hormone (α-MSH) is a melanocortin 4 receptor (MC4R) agonist and is stimulated by leptin. The MC4R likewise initiates brain-derived neurotrophic factor (BDNF) through tyrosine receptor kinase B (TrkB) receptors in the ventromedial (VMH) area of the hypothalamus. BDNF, when administered centrally in db/db mice, decreased food allowance and expanded vitality consumption, exhibiting its role in controlling sustenance balance which is possibly intervened through MC4R (14, 15). α- MSH actuates MC4R at the paraventricular nucleus, which leads to a suppression in food intake and an increase in energy expenditure (16). Leptin increases BDNF production by causing an increase in the release of α-MSH from the Arcuate nucleus (Arc). It also acts on MC4R which is expressed by BDNF-producing neurons; these events demonstrate the involvement of BDNF in the control of energy expenditure and as a sequential target of leptin. The secretion of BDNF leads to its interaction with trkB situated in the paraventricular nucleus (PVN), dorsomedial hypothalamus (DMH), Arc and ventromedial hypothalamus (VMH), causing reduced food intake by stimulating the feedback mechanism. Interestingly, leptin is also responsible for causing direct activation of phosphoinositide 3-kinase (PI3K) in pro-opiomelanocortin (POMC) neurons while generating an inactivation in Agouti-related protein (AgRP) neurons (17). Neuropeptide Y (NPY)/(AgRP) neurons are inhibited by insulin and leptin and are activated in negative energy conditions. NPY/AgRP neurons estrange the melanocortin signaling while γ-amino butyric acid (GABA) discharged from NPY/AgRP neurons inhibit the POMC neurons (18, 19). Ghrelin increases food intake by actuating NPY/AgRP neurons, which results to express the ghrelin receptors and consequently adjusts the inhibitory signs from peptide YY, leptin and insulin (20).
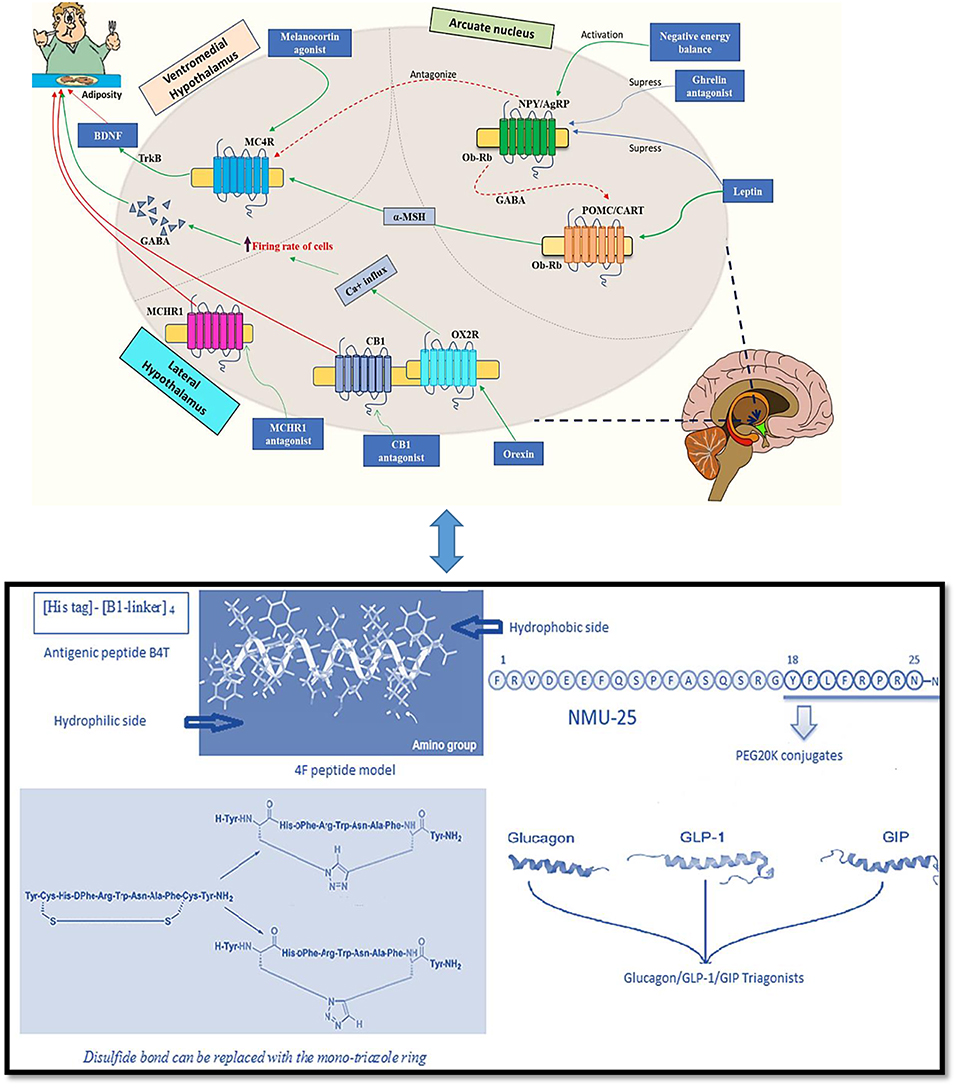
Figure 1. Endogenous peptides and peptidomimetics approaches for treating obesity [Adapted from Navab et al. (9), Tschop et al. (10), and Inooka et al. (11); Kim et al. (12); Tala et al. (13)] (Indications- Green solid arrow-activation, blue solid arrow-suppression, red dashed arrow-antagonism, and red solid arrow-block).
A therapeutic focus on the treatment of obesity includes development of ghrelin receptor antagonist which can cause multiple fold increase in appetite stimulation from the gut to the mind; ghrelin receptor inverse agonists would stop essential function of the ghrelin receptor, accordingly expanding the reaction to inhibitory signals to obstruct “between-suppers” food consumption (21). Reports show that use of recombinant adiponectin in fat mice enhances fatty acid digestion along with improvement in the condition of insulin resistance. Both leptin and adiponectin are adipocytokines from adipose tissue and are noteworthy insulin-sensitizing hormones (22). Adiponectin seems to act by enacting and phosphorylating AMP-activated protein kinase (AMPK), which at that point actuates and phosphorylates acetyl CoA-carboxylase, an enzyme downstream of AMPK in adipose tissue (23). MCHR1-deficient mice are impervious to the orexigenic activities of MCH and maintains leanness with increased vitality. Administration of MCH diminishes adrenocorticotropic hormone (ACTH) level, which controls the production of glucocorticoid. An increase in glucocorticoid levels are observed in MCH-deficient mice, demonstrating the importance of MCHR1 in adrenal functions (24). Orexins perform their actions through the ventral posterior Arc where actuation of OX2R orexin receptors empowers a Na+/Ca2+ trade current in GABAergic neurons and accordingly causing depolarization and enhancing the firing rate in the cells (25). As GABA works for a powerful upgrade in feeding behavior, orexins may use this system to control craving. Another peptide oxyntomodulin reduces appetite and increases body temperature; it aids weight loss and can be administered for a longer period. A renewed interest in this peptide has led to a new and stable self-assembling nanofibril formulation development, which subsequently releases pharmacologically active peptide, having anti-obesity and anti-diabetic potential (26).
Protein Data Bank (PDB) was accessed for the type of available obesity receptors from Homo sapiens, which yielded 103 results as listed in Table 1 and the receptors are shown in Figure 2.
Herein the status of natural anti-obesity peptides from food and marine sources is updated and experimental mimetic approaches for controlling obesity is discussed in further sections.
Peptidomimetic-Based Therapy in Obesity
Bioactive peptides face several challenges affecting their prolonged use and development, mainly due to their- chemical instability, hydrolysis, and aggregation, due to misfolding, short half-life, elimination, less permeability to cell membrane etc. However, a peptidomimetic approach by editing the naturally occurring peptides is currently being used for the development of promising drugs. They include various types of chemical modifications, L to D form isomerization, synthetic amino acid substitution, cyclization etc. (34). Researchers are targeting dipeptidyl peptidase-IV, STAT signaling, protein-protein interactions, arthritis, cardiovascular diseases, antimicrobial, immunomodulators with peptides and petidomimetics (35–41). Due to a limited number of antiobesity pharmacological drugs, chemists are now looking beyond traditional peptides and working on multifunctional peptides, peptide engineering, peptide aptamers, and peptidomimetics as modern alternatives involving newer design strategies. Aditpotide, is a peptidomimetic designed for weight loss and consists of an amino acid sequence of CysLysGlyGlyArgAlaLysAspCysGlyGlyAsp(LysLeuAlaLysLeuAlaLys)2. It reduced the body weight of the treated animals in the study by Kolonin et al. (42) and Barnhart et al. (43). In the first study, there was a rapid reduction in obesity without any adverse effects; while in the second study a remarkable reduction in white adipose tissue was observed by magnetic resonance imaging and X-ray absorptiometry. Barnhart et al. (43) showed that at experimentally determined optimal doses, monkeys from three different species displayed predictable and reversible changes in renal proximal tubule function. When engineering a new “Two-in-one” peptide, Day et al. (44) introduced a cyclic amide function in the peptide chain to make the complex more stable, and also locked a huge polyethylene glycol group to prevent its filtration from the kidney. These prudent modifications in the peptide exhibited full potency at both the glucagon and GLP receptor and caused dramatic weight loss in obese mice. Davalintide (AC2307), an amylinomimetic reduced food intake, thus decreasing body weight (45). It was also later discovered that combined administration of davalintide, an amylinomimetic, and a peptide YY (PYY) is advantageous in treating obesity (46). ValGlyPhe peptide profile was checked in obese mice and in type-2 diabetic patients. D'Amato et al. (47) conducted an experiment in a small group of patients and could conclude that the response of ValGlyPhe peptide was down regulated to glucose in both euglycemic obese patients and T2D subjects. Another combination of a synthetic peptide and a GHS-R1a antagonist compound led to the development of JMV 2959 with peptidomimetic approach using 1, 2, 4-triazole for designing ghrelin receptor ligands and investigating it for food intake and obesity (48). While it is reported that the metabolic instability can be overcome by development of d-amino acids containing small cyclic peptides, low oral bioavailability still remains a serious limitation for their pharmaceutical applications (49). Peptides and their mimetic approaches are further discussed in detail.
Apolipoprotein Mimetic Peptides
Apolipoprotein A-I (apoA-I) is highly abundant and one of the major protein components of high-density lipoprotein (HDL). Its role of having an inverse relation in the development of obesity is well-established (50). The relation between HDL/apoA-I and autophagy has also advanced the knowledge surroudning its anti-obesity results (51). ApoA-I mimetics are reported to decrease adiposity. The anti-obesity effect of apoA-I and an apoA-I mimetic peptide D-4F were studied. The overexpression of apoA-I and treatment with the mimetic D-4F remarkably reduced white fat mass and improved insulin sensitivity moderately. ApoA-I and mimetic D-4F when used for treatment, increased uncoupling protein mRNA and proteins along with stimulated phosphorylation of AMP-activated protein kinase (AMPK) in brown adipocytes (52). Administration of another apo A-I mimetic peptide L4F in low-density lipoprotein receptor (LDLR)-null mice also influenced weight gain and total plasma cholesterol (53). ApoA-I mimetic peptide L-4F improved the metabolic profile of male ob and female mice. L4F caused a significant reduction in the visceral fat to whole body weight ratio in male mice, while causing an opposite effect in female mice; a small and significant increase in the ratio of visceral fat to body weight compared with controls, suggesting sexually dimorphic effects of L-4F (54). 4F peptide (a hydrophobic analog of 18A) is highly hydrophobic in nature due to increased phenylalanine substitutions. The increased oxidized lipid association may be due to their ATP-binding cassette transporter (ABCA1)-dependent cellular cholesterol acceptance (55). Apolipoprotein A-I mimetic peptides, synthesized from D-amino acids, were found to be stable in the circulation when administered orally to LDL receptor-null mice. The lesions reduced without any change in HDL-cholesterol level. It was partially available in circulation during the study when given by oral route to reduce atherosclerosis (9). A peptide vaccine (B4T) (an apolipoprotein B100 mimotope) has also been developed which prevents obesity condition induced by high fat diet (HFD) in wild type mice as well as in liver steatosis (Figure 1). It targeted an epitope present in ApoB100 leading to weight reduction (56).
Melanocortin-4 Receptor Agonists
Peptide analogs of melanin concentrating hormone (MCH) with Kb of 0.1–0.2 μM and 4.3 nM were reported by Audinot et al. (57, 58) after the studies were carried out in rats. Bednarek et al. (59) reported another peptide as a MCH-R1 antagonist with IC50 value 14 nM and Kb of 0.9 nM. HisPheArgTrp, a tetrapeptide sequence from α-MSH and analogs were used for an antiobesity effect, due to their binding to the melanocortin-4 receptor (MC4R). It showed an increase in the metabolic stability and intestinal permeability when studies were carried out with a synthesized library of backbone cyclic peptidomimetic derivatives. Cepoi et al. (60) tested another peptide mimetic (1, 2, 3R, 4-tetrahydroisoquinoline-3-carboxylic acid) MC4R-specific agonist and its effect on inhibiting feeding behavior in mice. It decreased food intake after 4 h of intracerebroventricular (ICV) injection in mice. Peptide 1 (BL3020-1) was selective toward MC4R, had better cellular permeability in enterocytes and also increased intestinal metabolic stability. This peptide reduced food intake in mice due to the changes in backbone cyclization (61). Another antagonist ALB-127158(a) reduced food intake in DIO mice in preclinical testing and its Phase I clinical trials have been completed (62). Tala et al. (13) designed a library of 1, 2, 3-triazole bridged peptidomimetics of chimeric AGRP-melanocortin peptide. Their study concluded that the disulfide bond can be interchanged with the mono-triazole ring without affecting their functional ability at the melanocortin receptors.
GLP-1 Dual and Triple Agonists
A glucagon-like peptide 1 (GLP-1) analog named liraglutide maintained weight loss in obese individuals at a dose of 3 mg/day (63). It is licensed by the FDA and EMA for the management of obese patients. In another approach, the synergistic effect of GLP-1 and CCK pathways were tried with a fusion peptide C2816. It was constructed with AC3174 (GLP-1R agonist) and AC170222 (CCKR1-selective agonist); C2816 reduced more body weight in obese mice as compared to the GLP-1R and CCKR1-selective agonist co-administration at a higher dose (64). Zhou et al. (65) also synthesized 24 GLP-1/glucagon receptor dual agonists. A fatty acid laurate maleimide conjugate showed good results in lowering body weight in obese mice. A triple incretin agonist GLP-1-GIP-GCG has been described as potent anti-diabetic and anti-obesity agents (66) (Figure 1).
Neuropeptide
A short peglyated analog of a neuropeptide Neuromedin-U (Figure 1) facing short half-life led to its PEG20k conjugate which exhibited a good anti-obesity effect. At a dose of 10 and 30 nmol/kg, it reduced the body weight by 10 and 22%, respectively, in an obese mice model (11). In literature reports, a gut hormone fragment peptide YY3–36 reduced the hunger and also the intake of food in normal weight subjects (67). Catestatin (CST), a natural peptide occurring in the body also reduced body weight (68). Subsequently, a Y2R agonist was synthesized, PYY-1119(4-imidazolecarbonyl-[d Hyp24,Iva25,Pya(4)26,Cha27,36,γMeLeu28,Lys30,Aib31] ProTyrTyr (23–36) with better pharmacokinetic properties to alleviate emesis (69). Another study, in an attempt to extend the half-life, four apelin-13 analogs, namely, (Lys8GluPAL)apelin-13 amide, pGlu(Lys8GluPAL)apelin-13 amide, Lys8GluPAL(Tyr13)apelin-13, and Lys8GluPAL(Val13)apelin-13 were modified. It prolonged the half-life of native apelin-13 upto more than 24 h (70). A shorter peptide analog of gastrointestinal peptide, ProTyrTyr3–36, reduced appetite by activating the neuropeptide Y2 receptor (Y2R) thus controlling obesity and other metabolic diseases. A 14-amino acid ProTyrTyr analog, Ac-[d-Pro24, Pya (4)26, Cha27, 36, Aib28, 31, Lys30] ProTyrTyr (23–36) was administered continuously for a 2-week long study in mice, which showed high agonist and binding affinity for the Y2R. It showed a strong antiobesity effect resulting in more than 10% weight loss (71, 72).
Prolactin-Releasing Peptide Mimetics
Prolactin-releasing peptide (PrRP) analogs have been used for development of antiobesity agents. In a recent study, PrRP showed anorectic effects when the N terminus of PrRP was attached with palmitic acid. Later palmitoylation of PrRP31 improved its bioavailability by using two linkers (γ-glutamic acid and a modified polyethylene glycol). Two-week treatment with palmitoylated analogs reduced the body weight in a diet-induced obese mouse model. It also reduced the liver weight along with leptin, triglyceride and insulin levels (73).
Food Peptides
Soybean peptides have been used in food items extensively for their body fat-decreasing property. LeuProTyrProArg, a peptide from soybean glycinin A5A4B3 subunit and ProGlyPro are reported to have anorectic properties (74, 75). It decreased serum glycerides and cholesterol without decreasing body proteins in humans (76). Isolates from soy protein reduce the triglycerides and mRNA level of fatty acid synthase in adipose tissue in Wistar rats (77). Novel black soy peptides activated leptin-like signaling and AMP-activated protein kinase in a study for acute effects of black soy peptides on food intake and body weight in rats (78). Recently, Ashokan et al. (79) reported a peptide (ValHisValVal) from soybean responsible for stimulating lipolysis in apoptotic skeletal muscles caused by high fat diet. ValHisValVal peptide also regulated TNF-α expression which was elevated due to a high fat diet and suppressed apoptosis-related proteins. Soy isolate hydrolysate decreased fat accumulation and blood lipid profile by increasing excretion of fat in Sprague-Dawley rats by Aoyama et al. (80). Protease and pepsin prepared egg white hydrolysates reduced lipid content in liver and muscle along with total body fat in rats (81–83). Egg hydrolysate and black soy hydrolysate effects on diabetes and obesity markers in human individuals need more focussed study for their mechanism of absorption and the molecular targets (84, 85). Anti-appetizing peptides from milk suppress appetite and thus prevent weight gain and obesity. High-protein diets with whey proteins decrease appetite, resulting in less fat deposition and an improvement in insulin sensitivity. Zhang and Beynen (86) reported the effect of whey protein in the diet on lowering the level of LDL cholesterol, and increasing the release of cholecystokinin which is also known as appetite-suppressing hormone. Whey protein was isolated with peptide fractions κ-casein f, a glycomacropeptide that is manufactured by Davisco, USA (87). These peptides aid weight management due to the satiety inducing glycomarcopeptide and opioid peptides (7). The bioactivity of total whey protein may be dependent on the sequences of the peptides and its combination with active whey protein fractions (88). A peptide ValProPro derived from milk inhibited obesity-induced adipose tissue inflammation under the cascading effect of angiotensin-converting enzyme (ACE). Thus, ValProPro has been suggested as a viable therapeutic choice for obesity-associated adipose tissue inflammation and insulin resistance (89). Camel milk peptides displayed novel antidiabetic and anti-obesity peptides (90). A pentapeptide GluGlnArgProArg from rice bran < 5 kDa fraction showed around 70% adipocyte viability and a protective role against obesity (91). The antiobesity food peptides with their mechanism of action are listed in Table 2.
Marine Peptides
Marine peptides help aid weight maintenance by reducing the post-prandial blood sugar levels (95, 96). Recently, Fan et al. (97) reported the anti-obesity properties of Spirulina platensis-derived peptides which has attracted the focus once again to marine originated peptides. Chitosan, a polysaccharide is already known to modulate the level of serum leptin, C- reactive protein and inhibits the adipocyte differentiation in obese rats (98, 99). Fucoxanthin, alginates, fucoidans, and phlorotannins from seaweeds have been covered well and reviewed for their anti-obesity potential (100). Some marine peptides resemble gastrin, cholecystokinin and/or stimulate their release resulting in appetite control. Small peptides extracted from shrimp stimulate the release of cholecystokinin in STC-1 cells (101). Biofunctional peptides and their mining can be done from fish and shellfish waste components (102). Seaweed Plocamium telfairiae and Spirulina platensis exhibit the highest lipogenesis inhibitory effect in 3T3-L1 cells (97, 103). Four novel peptides AsnAlaLeuLysCysCysHisSerCysProAla, LeuAsnAsnProSerValCysAspCysAspCysMetMetLysAlaAlaArg, AsnProValTrpLysArgLys, and CysAlaAsnProHisGluLeuProAsnLys slowed down 3T3-L1 cells proliferation (32.29–60.08%). Intake of cod decreased the adipose tissue mass and hepatic lipids in mice (104). AspIleValAspLysIleGluIle peptide from boiled tuna inhibited CCAAT/enhancer-binding proteins (C/EBPs) and expression of peroxisome proliferator-activated receptor gamma (PPAR-γ). It also caused activation of the Wnt/β-catenin pathway, which inhibited pre-adipocytes differentiation into fat globule cells (105). In another work, Henda et al. (106) reported small peptides AlaPro, ValAlaPro, and AlaLysLys, and studied them for their effect on the viability of adipocytes during the proliferation period, among many LysTrp and ValTrp affected the viability during the differentiation stage. Peptides GlyProLeu and IleTyr decreased the final lipid content, glycerol-3-phosphate dehydrogenase activity and the mRNA level of adipocyte markers along with down regulation of two key regulators of adipogenesis—PPARγ and C/EBPα expression. Marine collagen peptide (MCPs) reduced the levels of total triglycerides, total cholesterol, low-density lipoprotein, free-fatty acids along with fasting blood glucose, fasting blood insulin and human glycated hemoglobin A1C in Chinese diabetic patients (107). MCPs in the size range of 2–26 kDa decreased body weight in animal models (108). Fish collagen peptide from a water-hydrolyzed fraction decreased the accumulation of lipid and also decreased expression of C/EBPα, PPARγ, and adipocyte protein 2 (aP2) genes during the 3T3-L1 preadipocytes differentiation (109). Oral supplementation of collagen fragments decreased body fat and weight with an improved cytokine (110). Recent studies have shown anti-oxidant potential of food peptides. Hu et al. (111) isolated novel peptides from Pecan protein hydrolysate and a peptide with amino acid sequence LeuAlaTyrLeuGlnTyrThrAspPheGluThrArg (mol. wt. < 3 kDa) which exhibited strongest antioxidant activity. This novel peptide is suggested to be further evaluated for the possibility of its development as a functional food with pharmaceutical properties. A smaller molecular weight peptide from carrot seed protein also showed strong antioxidant activity (112). Marine functional bioactives and ingredients in bakery and pasta products are also currently projected for future applications (113). All other antiobesity marine peptides are listed in Table 3.
Conclusion and Future directions
Peptides have been known for several decades from different sources with promising activity but very few studies have been carried out in vivo. Initially they were isolated from live animals and now they hold a specific therapeutic potential in the pharmaceutical field. They have been widely tested in new regimens due to rapid development in molecular biology in vitro assays. Newer approaches in chemistry are helping to improve the peptide stability and its pharmaceutical property. Due to their various limitations, continued study of natural peptides from bioresources with newer strategies of peptide engineering and their mimetics is suggested to be explored extensively. The number of therapeutic peptides and mimetics will increase substantially if advances in peptide engineering, combined uses of peptides, dual or triple agonists, aptamers, cell-penetrating, and multifunctional peptides are utilized to their highest potential. The current shortcomings faced by peptide drugs can be addressed by developing a deeper understanding of natural peptides from food and marine sources. Computational biology currently is a great support for peptide drug discovery and omics approaches uses genomics, proteomics, transcriptomics, and metabolomics as modern age platforms for finding bioactive peptides with unique structural features. Any novel finding pertaining to peptides are capable of shifting all the concepts toward obesity targets, they supported by evidence based studies and hence hold more promising future to control body weight. A new peptide, mimetic, and analog can be further taken for a development of suitable drug delivery system, stable formulation, with increased half-life to be used as an antiobesity drug. Though there is an increased interest in peptides and their mimetics, more efforts are required to meet the criteria to develop them as a therapeutic drug. Further studies of peptide's structural scaffold, their functions and mechanism of action, and other mediators involved in obesity can help discovery and development of novel peptides.
Author Contributions
MK performed the initial literature research and wrote the structured manuscript, corrected and prepared the final version of the manuscript.
Conflict of Interest Statement
The author declares that the research was conducted in the absence of any commercial or financial relationships that could be construed as a potential conflict of interest.
Acknowledgments
MK acknowledges SPP-School of Pharmacy and Technology Management, SVKM'S NMIMS, Mumbai for providing facility to carry out the work. MK also acknowledges the extended help by Ms. Sayli Chaudhary for the artwork.
References
1. Luppino FS, de Wit LM, Bouvy PF, Stijnen T, Cuijpers P, Penninx BW, et al. Overweight, obesity, and depression: a systematic review and meta-analysis of longitudinal studies. Arc Gen Psych. (2010) 67:220–9. doi: 10.1001/archgenpsychiatry.2010.2
3. Reilly JJ, El-Hamdouchi A, Diouf A, Monyeki A, Somda SA. Determining the worldwide prevalence of obesity. Lancet (2018) 391:1773–4. doi: 10.1016/S0140-6736(18)30794-3
4. Kumar RB, Aronne LJ. Pharmacologic Treatment of Obesity. In: De Groot LJ, Chrousos G, Dungan K, Feingold KR, Grossman A, Hershman JM, Koch C, Korbonits M, McLachlan R, New M, Purnell J, Rebar R, Singer F, Vinik A, editors. Available online at: https://www.ncbi.nlm.nih.gov/books/NBK279038/ (2017).
5. Meisel H, FitzGerald RJ. Biofunctional peptides from milk proteins: mineral binding and cytomodulatory effects. Curr Pharm Des. (2003) 9:1289–95. doi: 10.2174/1381612033454847
6. Delgado TC. Glutamate and GABA in appetite regulation. Front Endocrinol. (2013) 4:103. doi: 10.3389/fendo.2013.00103
7. Mohanty DP, Mohapatra S, Misra S, Sahu PS. Milk derived bioactive peptides and their impact on human health – a review. Saudi J Biol Sci. (2016) 23:577–83. doi: 10.1016/j.sjbs.2015.06.005
8. Bhat SP, Sharma A. Current drug targets in obesity pharmacotherapy - a review. Curr Drug Targets (2017) 18:983–93. doi: 10.2174/1389450118666170227153940
9. Navab M, Anantharamaiah GM, Hama S, Garber DW, Chaddha M, Hough G, et al. Oral administration of an Apo A-I mimetic peptide synthesized from D-amino acids dramatically reduces atherosclerosis in mice independent of plasma cholesterol. Circulation (2002) 105:290–2. doi: 10.1161/hc0302.103711
10. Tschop MH, Finan B, Clemmensen C, Gelfanov V, Perez-Tilve D, Muller TD, et al. Unimolecular polypharmacy for treatment of diabetes and obesity. Cell Metabol. (2016) 24:51–62. doi: 10.1016/j.cmet.2016.06.021
11. Inooka H, Sakamoto K, Shinohara T, Masuda Y, Terada M, Kumano S, et al. A PEGylated analog of short-length Neuromedin U with potent anorectic and anti-obesity effects. Bioorg Med Chem. (2017) 25:2307–12. doi: 10.1016/j.bmc.2017.02.023
12. Kim HJ, Lee HJ, Choi JS, Han J, Kim JY, Na HK, et al. An apolipoprotein B100 mimotope prevents obesity in mice. Clin Sci. (2015) 130:105–16. doi: 10.1042/CS20150423
13. Tala SR, Singh A, Lensing CJ, Schnell SM, Freeman KT, Rocca JR, et al. 1,2,3-Triazole rings as a disulfide bond mimetic in chimeric AGRP-melanocortin peptides: design, synthesis, and functional characterization. ACS Chem Neurosci. (2018) 9:1001–13. doi: 10.1021/acschemneuro.7b00422
14. Nakagawa T, Tsuchida A, Itakura Y, Nonomura T, Ono M, Hirota F, et al. Brain-derived neurotrophic factor regulates glucose metabolism by modulating energy balance in diabetic mice. Diabetes (2000) 49:436–44. doi: 10.2337/diabetes.49.3.436
15. Xu B, Goulding EH, Zang K, Cepoi D, Cone RD, Jones KR, et al. Brain-derived neurotrophic factor regulates energy balance downstream of melanocortin-4 receptor. Nat Neurosci. (2003) 6:736–42. doi: 10.1038/nn1073
16. Harvey J, Ashford MLJ. Leptin in the CNS: much more than a satiety signal. Neuropharmacology (2003) 44:845–54. doi: 10.1016/S0028-3908(03)00076-5
17. Yang R, Barouch LA. Leptin signaling and obesity: cardiovascular consequences. Circ Res. (2007) 101:545–59. doi: 10.1161/CIRCRESAHA.107.156596
18. Ju SH, Cho GB, Sohn JW. Understanding melanocortin-4 receptor control of neuronal circuits: toward novel therapeutics for obesity syndrome. Pharmacol Res. (2018) 129:10–9. doi: 10.1016/j.phrs.2018.01.004
19. Zagmutt S, Mera P, Soler-Vazquez MC, Herrero L, Serra D. Targeting AgRP neurons to maintain energy balance: Lessons from animal models. Biochem Pharmacol. (2018) 155:224–32. doi: 10.1016/j.bcp.2018.07.008
20. Hashiguchi H, Sheng Z, Routh V, Gerzanich V, Simard JM, Bryan J. Direct versus indirect actions of ghrelin on hypothalamic NPY neurons. PLoS ONE (2017) 12:e0184261. doi: 10.1371/journal.pone.0184261
21. Abegg K, Bernasconi L, Hutter M, Whiting L, Pietra C, Giuliano C, et al. Ghrelin receptor inverse agonists as a novel therapeutic approach against obesity-related metabolic disease. Diabetes Obes Metabol. (2017) 19:1740–50. doi: 10.1111/dom.13020
22. Kou H, Deng J, Gao D, Song A, Han Z, Wei J, et al. Relationship among adiponectin, insulin resistance and atherosclerosis in non-diabetic hypertensive patients and healthy adults. Clin Exp Hypertens. (2018) 40:656–63. doi: 10.1080/10641963.2018.1425414
23. Kadowaki T, Yamauchi T, Kubota N, Hara K, Ueki K, Tobe K. Adiponectin and adiponectin receptors in insulin resistance, diabetes, and the metabolic syndrome. J Clin Invest. (2006) 116:1784–92. doi: 10.1172/JCI29126
24. Marsh DJ, Weingarth DT, Novi DE, Chen HY, Trumbauer ME, Chen AS, et al. Melanin-concentrating hormone 1 receptor-deficient mice are lean, hyperactive, and hyperphagic and have altered metabolism. Proc Natl Acad Sci USA. (2002) 99:3240–5. doi: 10.1073/pnas.052706899
25. Urbanska A, Sokołowska P, Woldan-Tambor A, Bieganska K, Brix B, Jöhren O, et al. Orexins/hypocretins acting at Gi protein-coupled OX2 receptors inhibit cyclic AMP synthesis in the primary neuronal cultures. J Mol Neurosci. (2012) 46:10–7. doi: 10.1007/s12031-011-9526-2
26. Ouberai MM, Gomes Dos Santos AL, Kinna S, Madalli S, Hornigold DC, Baker D, et al. Controlling the bioactivity of a peptide hormone in vivo by reversible self-assembly. Nat Commun. (2017) 8:1026. doi: 10.1038/s41467-017-01114-1
27. Nolte RT, Wisely GB, Westin S, Cobb JE, Lambert MH, Kurokawa R, et al. Ligand binding and co-activator assembly of the peroxisome proliferator-activated receptor-gamma. Nature (1998) 395:137–43. doi: 10.1038/25931
28. Carpenter B, Hemsworth GR, Wu Z, Maamra M, Strasburger CJ, Ross RJ, et al. Structure of the human obesity receptor leptin-binding domain reveals the mechanism of leptin antagonism by a monoclonal antibody. Structure (2012) 20:487–97. doi: 10.1016/j.str.2012.01.019
29. Onate SA, Tsai SY, Tsai MJ, O'Malley BW. Sequence and characterization of a coactivator for the steroid hormone receptor superfamily. Science (1995) 270:1354–7. doi: 10.1126/science.270.5240.1354
30. Darimont BD, Wagner RL, Apriletti JW, Stallcup MR, Kushner PJ, Baxter JD, et al. Structure and specificity of nuclear receptor-coactivator interactions. Genes Dev. (1998) 12:3343–56. doi: 10.1101/gad.12.21.3343
31. Maurus R, Begum A, Williams LK, Fredriksen JR, Zhang R, Withers SG, et al. Alternative catalytic anions differentially modulate human alpha-amylase activity and specificity. Biochemistry (2008) 47:3332–44. doi: 10.1021/bi701652t
32. Osz J, McEwen AG, Wolf J, Poussin-Courmontagne P, Peluso-Iltis C, Chebaro Y, et al. Modulation of RXR-DNA complex assembly by DNA context. Mol Cell Endocrinol. (2018) 481:44–52. doi: 10.1016/j.mce.2018.11.008
33. Burgermeister E, Schnoebelen A, Flament A, Benz J, Stihle M, Gsell B, et al. A novel partial agonist of peroxisome proliferator-activated receptor-gamma (PPARgamma) recruits PPARgamma-coactivator-1alpha, prevents triglyceride accumulation, and potentiates insulin signaling in vitro. Mol Endocrinol. (2006) 20:809–30. doi: 10.1210/me.2005-0171
34. Qvit N, Rubin S, Urban TJ, Mochly-Rosen D, Gross ER. Peptidomimetic therapeutics: scientific approaches and opportunities. Drug Discov Today (2016) 22:454–62. doi: 10.1016/j.drudis.2016.11.003
35. Lai JH, Wu W, Zhou Y, Maw HH, Liu Y, Milo LJ Jr, et al. Synthesis and characterization of constrained peptidomimetic dipeptidyl peptidase IV inhibitors: amino-lactam boroalanines. J Med Chem. (2007) 50:2391–8. doi: 10.1021/jm061321&
36. Moradi S, Soltani S, Ansari AM, Sardari S. Peptidomimetics and their applications in antifungal Drug Design. Anti-Infec Ag Med Chem. (2009) 8:327–44. doi: 10.2174/187152109789760216
37. Akram ON, DeGraff DJ, Sheehan JH, Tilley WD, Matusik RJ, Ahn J-M, et al. Tailoring peptidomimetics for targeting protein–protein interactions. Mol Cancer Res. (2014) 12:967–78. doi: 10.1158/1541-7786.MCR-13-0611
38. Gokhale AS, Satyanarayanajois S. Peptides and peptidomimetics as immunomodulators. Immunotheraphy (2014) 6:755–74. doi: 10.2217/imt.14.37
39. Szelag M, Wesoly J, Bluyssen HA. Advances in peptidic and peptidomimetic-based approaches to inhibit STAT signaling in human diseases. Curr Protein Pept Sci. (2016) 17:135–46. doi: 10.2174/1389203716666151102103706
40. Recio C, Maione F, Iqbal AJ, Mascolo N, De Feo V. The potential therapeutic application of peptides and peptidomimetics in cardiovascular disease. Front Pharmacol. (2017) 7:526. doi: 10.3389/fphar.2016.00526
41. Molchanova N, Hansen PR, Damborg P, Nielsen HM, Franzyk H. Lysine-based α-Peptide/β-peptoid peptidomimetics: influence of hydrophobicity, fluorination, and distribution of cationic charge on antimicrobial activity and cytotoxicity. Chem Med Chem. (2017) 12:312–8. doi: 10.1002/cmdc.201600553
42. Kolonin MG, Saha PK, Chan L, Pasqualini R, Arap W. Reversal of obesity by targeted ablation of adipose tissue. Nat Med. (2004) 10:625–32. doi: 10.1038/nm1048
43. Barnhart KF, Christianson DR, Hanley PW, Driessen WH, Bernacky BJ, Baze WB, et al. A peptidomimetic targeting white fat causes weight loss and improved insulin resistance in obese monkeys. Sci Transl Med. (2011) 3:108ra112. doi: 10.1126/scitranslmed.3002621
44. Day JW, Ottaway N, Patterson JT, Gelfanov V, Smiley D, Gidda J, et al. A new glucagon and GLP-1 co-agonist eliminates obesity in rodents. Nat Chem Biol. (2009) 5:749–57. doi: 10.1038/nchembio.209
45. Mack CM, Soares CJ, Wilson JK, Athanacio JR, Turek VF, Trevaskis JL, et al. Davalintide (AC2307), a novel amylin-mimetic peptide: enhanced pharmacological properties over native amylin to reduce food intake and body weight. Int J Obes. (2010) 34:385–95. doi: 10.1038/ijo.2009.238
46. Coffey JT, Parkes DG, Roth JD. Combination Therapy Comprising Administration of an Amylinomimetic and a PYY Peptidomimetic for Effecting Weight Loss and for Treating Obesity and Related Metabolic Conditions and Disorders. WO 2011/050008. (2011).
47. D'Amato F, Noli B, Angioni L, Cossu E, Incani M, et al. VGF peptide profiles in type 2 diabetic patients' plasma and in obese mice. PLoS ONE (2015) 10:e0142333. doi: 10.1371/journal.pone.0142333
48. Moulin A, Brunel L, Boeglin D, Demange L, Ryan J, M'Kadmi C, et al. The 1:2:4–Triazole as a scaffold for the design of ghrelin receptor ligands: development of JMV 2959, A Potent Antagonist. Amino Acids (2013) 44:301–14. doi: 10.1007/s00726-012-1355-2
49. Rader AFB, Reichart F, Weinmuller M, Kessler H. Improving oral bioavailability of cyclic peptides by N-methylation. Bioorg Med Chem. (2018) 26:2766–73. doi: 10.1016/j.bmc.2017.08.031
50. Rashid S, Genest J. Effect of obesity on high-density lipoprotein metabolism. Obesity (2007) 15:2875–88. doi: 10.1038/oby.2007.342
51. Wang S, Peng D. Regulation of adipocyte autophagy — The potential anti-obesity mechanism of high density lipoprotein and ApolipoproteinA-I. Lipids Health Dis. (2012) 11:131. doi: 10.1186/1476-511X-11-131
52. Ruan X, Li Z, Zhang Y, Yang L, Pan Y, Wang Z, et al. Apolipoprotein A-I possesses an anti-obesity effect associated with increase of energy expenditure and up-regulation of UCP1 in brown fat. J Cell Mol Med. (2011) 15:763–72. doi: 10.1111/j.1582-4934.2010.01045.x
53. Averill MM, Kim EJ, Goodspeed L, Wang S, Subramanian S, Den Hartigh LJ, et al. The apolipoprotein-AI mimetic peptide L4F at a modest dose does not attenuate weight gain, inflammation, or atherosclerosis in LDLR-null mice. PLoS ONE (2014) 9:e109252. doi: 10.1371/journal.pone.0109252
54. Marino JS, Peterson SJ, Li M, Vanella L, Sodhi K, Hill JW, et al. ApoA-1 mimetic restores adiponectin expression and insulin sensitivity independent of changes in body weight in female obese mice. Nutr Diab. (2012) 2:e33. doi: 10.1038/nutd.2012.4
55. Anantharamaiah GM, Mishra VK, Garber DW, Datta G, Handattu SP, Palgunachari MN, et al. Structural requirements for antioxidative and anti-inflammatory properties of apolipoprotein A-I mimetic peptides. J Lipid Res. (2007) 48:1915–23. doi: 10.1194/jlr.R700010-JLR200
56. Kong SK, Choe MK, Kim HJ, Kim YS, Binas B, Kim HJ. An ApoB100-mimetic vaccine prevents obesity and liver steatosis in ApoE-/- mice. Pharmacol Rep. (2017) 69:1140–4. doi: 10.1016/j.pharep.2017.05.019
57. Audinot V, Lahaye C, Suply T, Beauverger P, Rodriguez M, Galizzi JP, et al. [125I]-S36057: a new and highly potent radioligand for the melanin-concentrating hormone receptor. Br J Pharmacol. (2001) 133:371. doi: 10.1038/sj.bjp.0704085
58. Audinot V, Zuana OD, Fabry N, Ouvry C, Nosjean O, Henlin JM, et al. S38151 [p-guanidinobenzoyl-[Des-Gly(10)]-MCH(7-17)] is a potent and selective antagonist at the MCH (1) receptor and has anti-feeding properties in vivo. Peptides (2009) 30:1997–2007. doi: 10.1016/j.peptides.2009.07.007
59. Bednarek MA, Hreniuk DL, Tan C, Palyha OC, MacNeil DJ, Van der Ploeg LH, et al. Synthesis and biological evaluation in vitro of selective, high affinity peptide antagonists of human melanin-concentrating hormone action at human melanin-concentrating hormone receptor 1. Biochemistry (2002) 41:6383–90. doi: 10.1021/bi0200514
60. Cepoi D, Phillips T, Cismowski M, Goodfellow VS, Ling N, Cone R.D., et al. Assessment of a small molecule melanocortin-4 receptor-specific agonist on energy homeostasis. Brain Res. (2004) 1000:64–71. doi: 10.1016/j.brainres.2003.10.041
61. Hess S, Linde Y, Ovadia O, Safrai E, Shalev DE, Swed A, et al. Backbone cyclic peptidomimetic melanocortin-4 receptor agonist as a novel orally administrated drug lead for treating obesity. J Med Chem. (2008) 514:1026–34. doi: 10.1021/jm701093y
62. Moore NA, Surman M, Guzzo P, Sargent BJ, Silverman MH, Blundell J, et al. MCH1 Receptor Antagonist, ALB-127158(a): Phase I Safety, Tolerability and Activity in Lean and Overweight Volunteers. Albany, NY: AMRI (2011).
63. Anandhakrishnan A, Korbonits M. Glucagon-like peptide 1 and human obesity. Prac Guide Obes. Med. (2018) 17–36. doi: 10.1016/B978-0-323-48559-3.00003-8
64. Hornigold DC, Roth E, Howard V, Will S, Oldham S, Coghlan MP, et al. A GLP-1: CCK fusion peptide harnesses the synergistic effects on metabolism of CCK-1 and GLP-1 receptor agonism in mice. Appetite (2018) 127:334–40. doi: 10.1016/j.appet.2018.05.131
65. Zhou J, Cai X, Huang X, Dai Y, Sun L, Zhang B, et al. A novel glucagon-like peptide-1/glucagon receptor dual agonist exhibits weight-lowering and diabetes-protective effects. Eur J Med Chem. (2017) 138:1158–69. doi: 10.1016/j.ejmech.2017.07.046
66. Gault VA, Bhat VK, Irwin N, Flatt PR. A novel glucagon-like peptide-1 (GLP-1)/Glucagon hybrid peptide with triple-acting agonist activity at glucose-dependent insulinotropic polypeptide, GLP-1, and glucagon receptors and therapeutic potential in high fat-fed mice. J Biol Chem. (2013) 288:35581–91. doi: 10.1074/jbc.M113.512046
67. Batterham RL, Cohen MA, Ellis SM, Le Roux CW, Withers DJ, Frost GS, et al. Inhibition of food intake in obese subjects by peptide YY3–36. N Engl J Med. (2003) 349:941–8. doi: 10.1056/NEJMoa030204
68. Ying W, Mahata S, Bandyopadhyay GK, Zhou Z, Wollam J, Vu J, et al. Catestatin inhibits obesity-induced macrophage infiltration and inflammation in the liver and suppresses hepatic glucose production leading to improved insulin sensitivity. Diabetes (2018) 67:841–8. doi: 10.2337/db17-0788
69. Niida A, Kanematsu-Yamaki Y, Asakawa T, Ishimura Y, Fujita H, Matsumiya K, et al. Antiobesity and emetic effects of a short-length peptide YY analog and its PEGylated and alkylated derivatives. Bioorg Med Chem. (2018) 26:566–72. doi: 10.1016/j.bmc.2017.12.014
70. O'Harte FPM, Parthsarathy V, Hogg C, Flatt PR. Acylated apelin-13 amide analogues exhibit enzyme resistance and prolonged insulin releasing, glucose lowering and anorexic properties. Biochem Pharmacol. (2017) 146:165–73. doi: 10.1016/j.bcp.2017.10.002
71. Nishizawa N, Niida A, Adachi Y, Kanematsu-Yamaki Y, Masuda Y, Kumano S, et al. Highly potent antiobesity effect of a short-length peptide YY analog in mice. Bioorg Med Chem. (2017) 25:5718–25. doi: 10.1016/j.bmc.2017.08.044
72. Nishizawa N, Niida A, Adachi Y, Masuda Y, Kumano S, Yokoyama K, et al. Potent antiobesity effect of a short-length peptide YY-analogue continuously administered in mice. Bioorg Med Chem Lett. (2017) 27:3829–32. doi: 10.1016/j.bmcl.2017.06.055
73. Prazlienkova V, Holubova M, Pelantova H, Bugaanova M, Pirnoak Z, Miroslava B, et al. Impact of novel palmitoylated prolactin-releasing peptide analogs on metabolic changes in mice with diet-induced obesity. PLoS ONE (2017) 12:e0183449. doi: 10.1371/journal.pone.0183449
74. Takenaka Y, Utsumi S, Yoshikawa M. Introduction of a low molecular weight agonist peptide for complement C3a receptor into soybean proglycinin A1aB1b subunit by site-directed mutagenesis. Biosci Biotechnol Biochem. (2001) 65:1202–5. doi: 10.1271/bbb.65.1202
75. Takenaka Y, Nakamura F, Yamamoto T, Yoshikawa M. Enterostatin (VPDPR) and its peptide fragment DPR reduce serum cholesterol levels after oral administration in mice. Biosci Biotechnol Biochem. (2003) 67:1620–2. doi: 10.1271/bbb.67.1620
76. Inaba H, Sakai K, Ota A. Body Fat-Decreasing Agents Containing Peptides and Glutamine, and Food Compositions Containing Them. Japan: Meiji Seika Kaisha, Ltd. Jpn. Kokai Tokkyo Koho JP 2002020312 A2 23. 5 p. (2002).
77. Nagasawa A, Fukui K, Kojima M, Kishida K, Maeda N, Nagaretani H, et al. Divergent effects of soy protein diet on the expression of adipocytokines. Biochem Biophy Res. Commun. (2003) 311:909–14. doi: 10.1016/j.bbrc.2003.10.087
78. Jang EH, Moon JS, Ko JH, Ahn CW, Lee HH, Shin JK, et al. Novel black soy peptides with antiobesity effects: activation of leptin-like signaling and AMP-activated protein kinase. Int J Obes. (2008) 32:1161–70. doi: 10.1038/ijo.2008.60
79. Ashokan SM, Hung T-H, Chiang W-D, Lin WT. Lipolysis-stimulating peptide from soybean protects against high fat diet-induced apoptosis in skeletal muscles. J Med Food (2018) 21:225–32. doi: 10.1089/jmf.2017.3941
80. Aoyama T, Fukui K, Takamatsu K, Hashimoto Y, Yamamoto T. Soy protein and its hydrolysate reduce body fat of dietary obese rats and generically obese mice (yellow KK). Nutrition (2000) 16:349–54. doi: 10.1016/S0899-9007(00)00230-6
81. Ochiai M, Kuroda T, Matsuo T. Increased muscular triglyceride content and hyperglycemia in Goto-Kakizaki rat are decreased by egg white hydrolysate. Int J Food Sci Nutr. (2014) 65:495–501. doi: 10.3109/09637486.2013.879288
82. Ochiai M, Misaki K, Takeuchi T, Narumi R, Azuma Y, Matsuo T. Egg white hydrolysate can be a low-allergenic food material to suppress ectopic fat accumulation in rats fed an equicaloric diet. J Nutr Sci Vitaminol. (2017) 63:111–9. doi: 10.3177/jnsv.63.111
83. Garces-Rimon M, González C, Uranga JA, Lopez-Miranda V, Lopez-Fandino R, Miguel M. Pepsin egg white hydrolysate ameliorates obesity-related oxidative stress, inflammation and steatosis in zucker fatty rats. PLoS ONE (2016) 11:e0151193. doi: 10.1371/journal.pone.0151193
84. Kwak JH, Ahn C, Park S, Jung S, Min B, Kim OY, et al. Weight reduction effects of a black soy peptide supplement in overweight and obese subjects: double blind, randomized, controlled study. Food Funct. (2012) 3:1019–24. doi: 10.1039/c2fo10244g
85. C. de Campos Zani S, Wu J, B. Chan C. Egg and soy-derived peptides and hydrolysates: a review of their physiological actions against diabetes and obesity. Nutrients (2018) 10:549. doi: 10.3390/nu10050549
86. Zhang X, Beynen AC. Lowering effect of dietary milk-whey protein v. casein on plasma and liver cholesterol concentrations in rats. Br J Nutr. (1993) 70:139–46. doi: 10.1079/BJN19930111
87. Burton-Freeman BM. Glycomacropeptide (GMP) is not critical to whey-induced satiety, but may have a unique role in energy intake regulation through cholecystokinin (CCK). Phys Behavior. (2008) 93:379–87. doi: 10.1016/j.physbeh.2007.09.010
88. Park YW, Nam MS. Bioactive peptides in milk and dairy products: a review. Korean J Food Sci An. (2015) 35:831–40. doi: 10.5851/kosfa.2015.35.6.831
89. Sawada Y, Sakamoto Y, Toh M, Ohara N, Hatanaka Y, Naka A, et al. Milk-derived peptide Val-Pro-Pro (VPP) inhibits obesity-induced adipose inflammation via an angiotensin-converting enzyme (ACE) dependent cascade. Mol Nutr Food Res. (2015) 59:2502–10. doi: 10.1002/mnfr.201500324
90. Mudgil P, Kamal H, Yuen GC, Maqsood S. Characterization and identification of novel antidiabetic and anti-obesity peptides from camel milk protein hydrolysates. Food Chem. (2018) 259:46–54. doi: 10.1016/j.foodchem.2018.03.082
91. Kannan A, Hettiarachchy NS, Mahedevan M. Peptides derived from rice bran protect cells from obesity and Alzheimer's disease. Int J Biomed Res. (2012) 3:131–5. doi: 10.7439/ijbr.v3i3.299
92. Peterson SJ, Kim DH, Li M, Positano V, Vanella L, Rodella LF, et al. The L-4F mimetic peptide prevents insulin resistance through increased levels of HO-1, pAMPK, and pAKT in obese mice. Lipid Res. (2009) 50:1293–304. doi: 10.1194/jlr.M800610-JLR200
93. Linde Y, Ovadia O, Safrai E, Xiang Z, Portillo FP, Shalev DE, et al. Structure-activity relationship and metabolic stability studies of backbone cyclization and N-methylation of melanocortin peptides. Biopolymers (2008) 90:671–82. doi: 10.1002/bip.21057
94. Costantino L, Barlocco D. New perspectives on the development of antiobesity drugs. Future Med Chem. (2015) 7:315–36. doi: 10.4155/fmc.14.167
95. Vegge G, Ronnestad BR, Ellefsen S. Improved cycling performance with ingestion of hydrolyzed marine protein depends on performance level. J Int Soc Sports Nutr. (2012) 9:14. doi: 10.1186/1550-2783-9-14
96. Cudennec B, Ravallec R. Biological active peptides from marine sources related to gut hormones. Curr Protein Pept Sci. (2013) 14:231–4. doi: 10.2174/13892037113149990034
97. Fan X, Cui Y, Zhang R, Zhang X. Purification and identification of anti-obesity peptides derived from Spirulina platensis. J Funct Foods (2018) 47:350–60. doi: 10.1016/j.jff.2018.05.066
98. Walsh AM, Sweeney T, Bahar B, O'Doherty JV. Multi-functional roles of chitosan as a potential protective agent against obesity. PLoS ONE (2013) 8:e53828. doi: 10.1371/journal.pone.0053828
99. Huang L, Chen J, Cao P, Pan H, Ding C, Xiao T, et al. Anti-obese effect of glucosamine and chitosan oligosaccharide in high-fat diet-induced obese rats. Mar Drugs (2015) 13:2732–56. doi: 10.3390/md13052732
100. Chu W-L, Phang S-M. Marine algae as a potential source for anti-obesity agents. Mar. Drugs (2016) 14:222. doi: 10.3390/md14120222
101. Cudennec B, Ravallec-Plé R, Courois E, Fouchereau-Peron M. Peptides from fish and crustacean by-products hydrolysates stimulate cholecystokinin release in STC-1 cells. Food Chem. (2008) 111:970–5. doi: 10.1016/j.foodchem.2008.05.016
102. Harnedy PA, FitzGerald RJ. Bioactive peptides from marine processing waste and shellfish: a review. J Funct Foods (2012) 4:6–24. doi: 10.1016/j.jff.2011.09.001
103. Kang M-C, Kang N, Ko S-C, Kim Y-B, Jeon Y-J. Anti-obesity effects of seaweeds of Jeju Island on the differentiation of 3T3-L1 preadipocytes and obese mice fed a high-fat diet. Food Chem Toxicol. (2016) 90:36–44. doi: 10.1016/j.fct.2016.01.023
104. Liisberg U, Fauske KR, Kuda O, Fjære E, Myrmel LS, Norberg N, et al. Intake of a Western diet containing cod instead of pork alters fatty acid composition in tissue phospholipids and attenuates obesity and hepatic lipid accumulation in mice. J Nutr Biochem. (2016) 33:119–127. doi: 10.1016/j.jnutbio.2016.03.014
105. Kim YM, Kim IH, Choi JW, Lee MK, Nam T-J. The anti-obesity effects of a tuna peptide on 3T3-L1 adipocytes are mediated by the inhibition of the expression of lipogenic and adipogenic genes and by the activation of the Wnt/β-catenin signaling pathway. Int J Mol Med. (2015) 36:327–34. doi: 10.3892/ijmm.2015.2231
106. Henda YB, Laamari M, Lanneluc I, Travers M-A, Agogué H, Arnaudin I, et al. Di and tripeptides from marine sources can target adipogenic process and contribute to decrease adipocyte number and functions. J Funct Foods (2015) 17:1–10. doi: 10.1016/j.jff.2015.04.050
107. Zhu C-F, Li G-Z, Peng H-B, Zhang F, Chen Y, Li Y. Treatment with marine collagen peptides modulates glucose and lipid metabolism in Chinese patients with type 2 diabetes mellitus. Appl Physiol Nutr Metab. (2010) 35:797–804. doi: 10.1139/H10-075
108. Nagirniak OY, Raksha NG, Savchuk OM, Ostapchenko LI. The influence of collagen fragments extracted from the Antarctic region fish scales on obesity development. Ukr Antarct J. (2016) 15:153–60.
109. Lee EJ, Hur J, Ham SA, Jo Y, Lee S, Choi MJ, et al. Fish collagen peptide inhibits the adipogenic differentiation of preadipocytes and ameliorates obesity in high fat diet-fed mice. Int J Biol Macromol. (2017) 104:281–6. doi: 10.1016/j.ijbiomac.2017.05.151
110. Raksha NG, Potalitsyn PY, Yurchenko AV, Halenova TI, Savchuk OM, Ostapchenko LI. Prevention of diet-induced obesity in rats by oral application of collagen fragments. Arch Biol Sci. (2018) 70:77–86. doi: 10.2298/ABS170401027R
111. Hu F, Ci A-T, Wang H, Zhang Y-Y, Zhang J-G, Thakur K, et al. Identification and hydrolysis kinetic of a novel antioxidant peptide from pecan meal using Alcalase. Food Chem. (2018) 261:301–10. doi: 10.1016/j.foodchem.2018.04.025
112. Ye N, Hu P, Xu S, Chen M, Wang S, Hong J, et al. Preparation and characterization of antioxidant peptides from carrot seed protein. J Food Qual. (2018) 2018:8579094. doi: 10.1155/2018/8579094
113. Kadam SU, Prabhasankar P. Marine foods as functional ingredients in bakery and pasta products. Food Res Int. (2010) 43:1975–80. doi: 10.1016/j.foodres.2010.06.007
114. Tsou MJ, Kao FJ, Lu HC, Kao HC, Chiang WD. Purification and identification of lipolysis-stimulating peptides derived from enzymatic hydrolysis of soy protein. Food Chem. (2013) 138:1454–60. doi: 10.1016/j.foodchem.2012.10.149
115. Kim MJ, Yang HJ, Kim JH, Ahn CW, Lee JH, Kim KS, et al. Obesity-related metabolomic analysis of human subjects in black soybean peptide intervention study by ultra-performance liquid chromatography and quadrupole-time-of-flight mass spectrometry. J Obes. (2013) 2013:874981. doi: 10.1155/2013/874981
116. Martinez-Villaluenga C, Rupasinghe SG, Schuler MA, Gonzalez de Mejia E. Peptides from purified soybean β-conglycinin inhibit fatty acid synthase by interaction with the thioesterase catalytic domain. FEBS J. (2010) 277:1481–93. doi: 10.1111/j.1742-4658.2010.07577.x
117. Nishi T, Hara H, Asano K, Tomita F. The soybean beta-conglycinin beta 51–63 fragment suppresses appetite by stimulating cholecystokinin release in rats. J Nutr. (2003) 133:2537–42. doi: 10.1093/jn/133.8.2537
118. Inoue N, Nagao K, Sakata K, Yamano N, Ranawakage GPE, Han SY, et al. Screening of soy protein-derived hypotriglyceridemic di-peptides in vitro and in vivo. Lipids Health Dis. (2011) 10:85. doi: 10.1186/1476-511X-10-85
119. Lammi C, Zanoni C, Arnoldi A. IAVPGEVA, IAVPTGVA, and LPYP, three peptides from soy glycinin, modulate cholesterol metabolism in HepG2 cells through the activation of the LDLR-SREBP2 pathway. J Funct Foods (2015) 14:469–78. doi: 10.1016/j.jff.2015.02.021
120. Pepe T, Ceruso M, Carpentieri A, Ventrone I, Amoresano A, Anastasio A, et al. Differentiation of four tuna species by two-dimensional electrophoresis and mass spectrometric analysis. In: Heazlewood J, editor. Proteomic Applications in Biology London: InTech (2012). p. 191–208. ISBN: 978-953-307-613-3.
121. Kim YM, Kim EY, Kim IH, Nam TJ. Peptide derived from desalinated boiled tuna extract inhibits adipogenesis through the down regulation of C/EBP-α and PPAR-γ in 3T3-L1 adipocytes. Intl J Mol Med. (2015) 35:2362–8. doi: 10.3892/ijmm.2015.2127
122. Zhang H, Wang J, Liu Y, Sun B. Peptides derived from oats improve insulin sensitivity and lower blood glucose in streptozotocin-induced diabetic mice. J Biomed Sci. (2015) 4:1. doi: 10.4172/2254-609X.100007
123. Jemil I, Abdelhedi O, Nasri R, Mora L, Marrekchi R, Jamoussi K, et al. Hypolipidemic, antiobesity and cardio protective effects of sardinelle meat flour and its hydrolysates in high-fat and fructose diet fed Wistar rats. Life Sci. (2017) 176:54–66. doi: 10.1016/j.lfs.2016.07.012
Keywords: antiobesity, endogenous peptides, marine peptides, food peptides, peptidomimetics
Citation: Kumar MS (2019) Peptides and Peptidomimetics as Potential Antiobesity Agents: Overview of Current Status. Front. Nutr. 6:11. doi: 10.3389/fnut.2019.00011
Received: 12 October 2018; Accepted: 22 January 2019;
Published: 18 February 2019.
Edited by:
Alessandra Durazzo, Council for Agricultural and Economics Research, ItalyReviewed by:
Steve Meikle, Independent Researcher, Eastbourne, United KingdomZhaojun Wei, Hefei University of Technology, China
Copyright © 2019 Kumar. This is an open-access article distributed under the terms of the Creative Commons Attribution License (CC BY). The use, distribution or reproduction in other forums is permitted, provided the original author(s) and the copyright owner(s) are credited and that the original publication in this journal is cited, in accordance with accepted academic practice. No use, distribution or reproduction is permitted which does not comply with these terms.
*Correspondence: Maushmi S. Kumar, bWF1c2htaXNrdW1hckBnbWFpbC5jb20=