- 1Laboratory of Physiology and Sport Performance (LAFIDE), Bauru, Brazil
- 2Post-Graduate Program in Movement Sciences, Sao Paulo State University–UNESP, School of Science, Bauru, Brazil
- 3School of Physical Education and Sport, University of São Paulo, São Paulo, Brazil
- 4Department of Medicine and Aging Sciences, “G. d'Annunzio” University of Chieti-Pescara, Pescara, Italy
The aim of this study was to verify the effects of caffeine on anaerobic capacity estimated by the sum of the estimated glycolytic [E[La]] and phosphagen [EPCr] metabolism based on blood lactate and excess post-oxygen consumption responses (AC[La−]+EPOCfast). Fourteen male cyclists were submitted to a graded exercise test to determine the maximal oxygen uptake (V°O2max) and intensity associated with V°O2max (iV°O2max). Subsequently, the participants performed two supramaximal efforts at 115% of iV°O2max to determine the AC[La−]+EPOCfast, after previous supplementation with caffeine (6 mg·kg−1) or a placebo (dextrose), in a cross over, randomized, double blind, and placebo-controlled design. The time to exhaustion was higher in the caffeine (186.6 ± 29.8 s) than in the placebo condition (173.3 ± 25.3 s) (p = 0.006) and a significant correlation was found between them (r = 0.86; P = 0.00008). Significant differences were not found between AC[La−]+EPOCfast values from the placebo (4.06 ± 0.83 L and 55.2 ± 5.7 mL·kg−1) and caffeine condition (4.00 ± 0.76 L and 54.6 ± 5.4 mL·kg−1); however, a significant correlation was observed only for AC[La−]+EPOCfast expressed in absolute values (r = 0.74; p < 0.002). The E[La] and EPCr also presented no significant differences and they were significantly correlated (r = 0.82 and r = 0.55, respectively; p < 0.05). We conclude based on the overall comparison of mean values between two treatments that acute caffeine ingestion improves the time to exhaustion but does not affect anaerobic capacity estimation.
Introduction
Acute caffeine ingestion has been shown to cause an increase in the excessive post-exercise oxygen consumption (EPOC) after resistance training (1), time to exhaustion during supramaximal effort (2) and other performance parameters. Moreover, the improvement in time to exhaustion during a supramaximal effort after caffeine intake caused by changes in oxygen consumption ()at the exhaustion moment, possibly affecting the phosphagen energy system estimation (2). In addition, some studies have shown that acute ingestion of caffeine increased time to exhaustion during a supramaximal test and anaerobic capacity estimated by the maximal accumulated oxygen deficit (MAOD) (3–5). Bell et al. (3) found a consequent increase in peak lactate concentration after a supramaximal effort to determine MAOD with caffeine supplementation. However, other studies have not shown significant effects of caffeine ingestion on exercise blood lactate concentration after supramaximal efforts to determine MAOD (4, 5). The possible changes in lactate concentrations could cause changes in the estimation of glycolytic system and consequently alter the estimation of capacity anaerobic when estimated by blood lactate concentration and EPOC responses (6–8), and consequently can alters the anaerobic capacity.
MAOD is one of the most accepted methods for evaluating an individual's maximal capacity of ATP resynthesis by means of the non-mitochondrial metabolism, which is determined by means of calculations involving measurements during several submaximal exercise sessions and one supramaximal exhaustive exercise session (9). However, the time-consuming nature of MAOD determination can discourage its use in routine athlete training and, for this reason, the anaerobic capacity has been alternatively estimated using only a single supramaximal effort (AC[La−]+EPOCfast) (6–8). AC[La−]+EPOCfast determination is based on an estimation of the oxygen equivalent from the glycolytic (E[La−]) and phosphagen (EPCr) pathways, considering the accumulated blood lactate (10) and the fast component of the excessive post-exercise oxygen consumption (EPOCfast) (11), respectively. The AC[La−]+EPOCfast is an advantageous method for anaerobic capacity assessment and its validity has been demonstrated by the absence of significant differences with the conventional MAOD determined in cycling (6, 7), running (8), and table tennis (12, 13). Furthermore, AC[La−]+EPOCfast method has shown high reliability (intraclass correlation coefficient [ICC] = 0.87 and typical error = 0.27 L) and significant associations with mechanical variables assessed in a 30 s maximal effort (14), besides to be sensitive in distinguishing individuals with different physical conditioning status (15).
The E[La−] is estimated by the values of blood lactate elevation from baseline during exercise, which could be influenced by factors related to the efflux of this metabolite from the muscle (16). On the other hand, the EPCr is estimated by computing the EPOCfast, which could be affected by any factor that causes alterations in the parameters related to this metabolic pathway calculation (e.g., oxygen uptake attained at exhaustion) (17). Thus, any intervention capable of modifying these responses (i.e., blood lactate and post-exercise oxygen consumption) could compromise the reliability of the AC[La−]+EPOCfast. For example, the AC[La−]+EPOCfast was increased after acute supplementation with sodium bicarbonate due to E[La] alteration (16). However, it is not yet clear whether AC[La−]+EPOCfast is sensitive to possible changes caused in the EPOCfast, induced for example by caffeine ingestion (1, 2).
De Poli et al. (2) found no effect of caffeine on AC[La−]+EPOCfast values determined in running, but suggested possible alterations in the relative contributions of the glycolytic (+9.3% with ~68% possibly positive effect) and phosphagen metabolic (−5.4% with ~76% possibly negative effect) pathways. Nevertheless, anaerobic evaluations are widely used in cycling (18) and investigations into the effects of caffeine on cycling AC[La−]+EPOCfast are missing. Importantly, different physiological responses can be observed in cycling compared to running (19) and MAOD seems to be affected by the exercise mode (20). Thus, considering the possible “fluctuations” in determining AC[La−]+EPOCfast that could be caused by these changes in EPCr and E[La], it is necessary to investigate the effects of acute caffeine intake on the estimation of “anaerobic” capacity using this protocol in cycling.
Therefore, the aim of the present study was to verify the effects of acute caffeine ingestion on “anaerobic” capacity estimated by the AC[La−]+EPOCfast in cycling. We hypothesized that caffeine intake would improve performance in the supramaximal effort and change the relative energetic contribution of E[La−] and EPCr on AC[La−]+EPOCfast method, as observed in running (2).
Materials and Methods
Subjects
Fifteen male mountain bikers were considered eligible to participate in the study. Participants were recruited from regional cycling groups. To be included, they should be healthy, without any vascular disease, metabolic disorders, recent muscle-skeletal, or joint injuries and should not have used nutritional supplements as beta-alanine and creatine or pharmacological substances for at least 3 months. One participant was excluded from the study due to the inclusion criteria and therefore the final sample size was composed of 14 bikers. Five subjects had been competing at the regional level for at least 10 years. The other nine subjects reported at least 1 year of regular training and competition experience. The average weekly training volume reported by these individuals was 203 ± 122 km per week with a training frequency of 3–6 times.
One individual was excluded from the study due to high self-reported habitual daily caffeine ingestion (~780 mg·day−1). The participants' daily intake of caffeine was estimated for 3 days prior to the commencement of the study and reported to be 53.4 ± 39.8 mg·day−1. All subjects were prohibited to consume any food or drink containing caffeine (i.e., tea, coffee, soft drinks, energy drinks, chocolate, and others) and alcohol, as well as were instructed to refrain to perform vigorous physical activity for at least 24 h before each test session. In addition, all subjects were instructed to consume their habitual meal. The characteristics of the subjects are presented in Table 1.
The subjects were informed about the risks and benefits of the procedures and signed a written consent prior to commencing study participation. All procedures were approved by the local Ethics Committee (Protocol 645 784/2014) and were conducted in accordance with the Helsinki Declaration.
Experimental Design
The study design was a placebo-controlled double-blind crossover randomized trial. Figure 1 presents a flow diagram of the study. Initially, the subjects were submitted to a graded exercise test (GXT) to determine the maximal oxygen uptake () and intensity associated with (i). Next, they performed two supramaximal efforts at 115% of i to determine the AC[La−]+EPOCfast, with or without (placebo condition) caffeine supplementation. The three sessions were separated by a minimum of 48 h. In all tests, the warm-up was standardized at 100 W for 5-min and was carried out 5-min before the tests.
All exercise tests were performed on an electromagnetic cycle ergometer (Lode-Excalibur, Lode, Netherlands). The subjects were instructed to adopt a preferred cadence between 70 and 90 rpm and to maintain the chosen cadence with a maximum variation of ± 5 rpm throughout the tests. The procedures for each study session were applied in an environment with controlled temperature and humidity (20 ± 1°C and 61 ± 8%, respectively).
For all laboratory visits, the subjects were informed to maintain the normal diet during the day and to make a meal between 2 and 4 h before to start the exercise procedures.
Physiological and Metabolic Data Collection
The respiratory responses were measured breath-by-breath by a stationary gas analyzer (Quark CPET, COSMED, Rome, Italy). The gas analyzer was calibrated before each test session using gas samples with known concentrations (5.00% CO2 and 16.02% O2, White Martins®, Osasco, Brazil) and room air, while the turbine was calibrated through a 3-L syringe (Hans-Rudolf, USA). The obtained during the tests was smoothed each 5 points and interpolated at 1 s intervals through OriginPro 9.0 software (OriginLab Corporation, Microcal, Massachusetts, USA). The heart rate (HR) was measured by a transmitter belt with wireless connection to the gas analyzer (Wireless HR 138 Monitor, COSMED, Rome, Italy),while the rating of perceived exertion (RPE) was assessed using the 6–20 Borg scale (21).
The blood lactate concentration ([La−]) was measured from blood samples collected from the ear lobe (25 μl) at rest ([La−]Rest) (prior to warm-up) and 3, 5, and 7 min after each maximal test to determine the peak lactate concentration ([La−]Peak). The blood samples were collected and stored in Eppendorf tubes containing 50 μL of 1% sodium fluoride and then analyzed using a YSI 2300 STAT (Yellow Spring Instruments, Ohio, USA) (typical error of ± 2%).
The subjects remained seated for 10 min to determine the baseline and [La−]Rest. Baseline was considered as the mean of the final 2 min, while the exhaustion was considered the mean of the final 30 s of the supramaximal test.
Body Composition Analysis
Body composition was measured by dual-energy X-ray absorptiometry (DXA) using the Discovery corporal scanner (Hologic, Sunnyvale, USA). The body segmentation analysis was carried out with the horizontal line positioned above the bowl slightly above iliac crest. The angular lines that define the pelvic triangle were sectioned at the femur, and the vertical line positioned between the legs dividing the two feet. The lean mass of the lower limbs (LM-LL) was considered the sum of the right and left legs, not considering the bone mass values (7).
Graded Exercise Test (GXT)
The GXT was designed to induce exhaustion in ~8–12 min (22). The initial power output was set at 100–150 W with increments of 25 W every 2 min until voluntary exhaustion or the inability to maintain the pre-defined cadence (23). In each test stage, measured during the final 30 s was averaged. The highest 30 s average obtained during the test was considered as the , considering the verification of a plateau in (variation in < 2.1 mL·kg−1·min−1 between the final and penultimate stage of exercise). Secondary criteria were: maximal HR ≥ 90% of predicted maximal value, respiratory exchange ratio (RER) ≥ 1.10, and peak lactate ≥ 8.0 mmol·L−1 (22). The i was assumed as the lowest intensity at which the was attained (24).
Caffeine Supplementation and Supramaximal Efforts
The subjects ingested 6 mg·kg−1 of caffeine or a placebo (dextrose) (Neonutri, Minas Gerais, Brazil) 1 h before each supramaximal effort (Gemini Pharmaceutical Ingredients Industry Ltda, Anápolis, GO, Brazil), in a double blind and randomized fashion. The caffeine and placebo were contained in identical gel capsules, which were produced in our laboratory using a manual capsule filling machine. The caffeine dosage used was chosen as it has been proved to cause changes in the excessive post-exercise oxygen consumption (1).
The individuals performed the supramaximal efforts at 115% of i to determine the time to exhaustion at this intensity and the corresponding AC[La−]+EPOCfast.. The EPCr was estimated by the EPOCFAST, analyzed using a bi-exponential model (Equation 1) (Origin PRO 9.0, OriginLab Corporation, Micrical, Massachusetts, USA) and corresponded to the product between A1 and τ1 (Equation 2) (6–8). The E[La] was estimated by the difference between the [La−]Peak and [La−]Rest, considering each 1.0 mmol·L−1 of accumulated lactate equivalent to 3 mL·kg−1 of oxygen (10). The AC[La−]+EPOCfast was determined from the sum of oxygen equivalents of E[La−] and EPCr pathways (Equation 3)
Where corresponds to the oxygen uptake at time t, is the rest oxygen uptake, A1 is the amplitude, and τ1 is the constant time.
In addition, the oxidative metabolism contribution (EOXID) was estimated considering the accumulated during the supramaximal effort using the trapezoidal method, excluding the rest values.
Statistical Analysis
The sample size was calculated (software G*Power) based on power analysis, taking into consideration the statistical power of 90%, α error probability of 0.05, and the effect size estimated from AC[La−]+EPOCfast mean and standard deviation differences in placebo and caffeine supplemented conditions (2), resulting in a minimum sample size of 13 participants. The results are presented as mean ± SD and 95% confidence interval (CI95%). Initially, the data were submitted to the Shapiro-Wilk test to verify data normality. The variables related to AC[La−]+EPOCfast in the caffeine and placebo conditions were compared using the paired t-test. The association was analyzed using the Pearson's product-moment correlation test. In all tests a level of significance of 5% was assumed. In addition, the data were analyzed qualitatively by magnitude-based inference and expressed as raw mean differences. The threshold values for Cohen's d statistical power were considered as >0.2 (small), >0.5 (moderate), and >0.8 (large). The chances of a possible substantial benefit or harm were calculated [assuming the value of 0.2 multiplied by the between-subject deviation as the smallest worthwhile change (SWC)].
Results
Table 2 displays the physiological responses at exhaustion in the GXT. All subjects reached the criteria to confirm determination.

Table 2. Values of heart rate (HR), respiratory exchange ratio (RER), peak blood lactate concentration ([La−]Peak), maximal oxygen uptake (), intensity associated with (i), and total time obtained in graded exercise test (n = 14).
The 115% of i was 335.2 ± 49.7 W (CI95% = 306.5 to 363.9 W). Figure 2 shows in mean ± SD and individual values that the time to exhaustion (tlim) was higher (Δ% = +7.8%) in the caffeine condition (CI95% = 169.4 to 203.9 s) than in the placebo condition (CI95% = 158.7 to 187.9 s) (P = 0.006) (Figure 2A), with 10 out of 14 subjects with performance higher than SWC boundary (Figure 2B). Significant correlations were found between the conditions in tlim (r = 0.86; CI95% = 0.60 to 0.95; p = 0.00008).
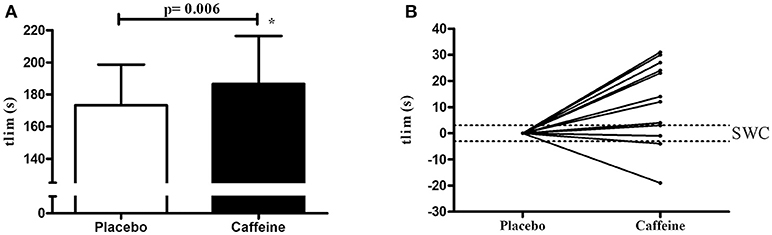
Figure 2. Time to exhaustion (tlim) measured during supramaximal effort at 115% of i under placebo and caffeine conditions. (A) shows the values in mean and standard deviation, while the figure (B) shows individual changes relative to placebo values and in relation to boundaries of smallest worthwhile change (SWC).
(Figures 3A,C,E) shows in mean ± SD the individual values of the AC[La−]+EPOCfast values determined in the caffeine (CI95% = 3.56 to 4.44 L; CI95% = 51.5 to 57.7 mL·kg−1; CI95% = 180.3 to 202.8 mL·kg−1LM-LL) and placebo (CI95% = 3.57 to 4.54 L; CI95% = 51.9 to 58.5 mL·kg−1; CI95% = 180.3 to 202.8 mL·kg−1 LM-LL) conditions. There were no differences between the AC[La−]+EPOCfast values expressed in absolute or relative terms (p > 0.708). In addition, when the data are analyzed individually (Figures 3B,D,F), 10 subjects from 14 participants modified the anaerobic capacity beyond upper/lower SWC limits (i.e., ~4 subjects were “positive” responders and ~7 subjects were “negative” responders), evidencing high inter-individual variability, while ~3 subjects were “non-responders.” Significant correlation was found only for the AC[La−]+EPOCfast expressed in absolute values (r = 0.74 and p = 0.002), but not when expressed in relative values (r = 0.12 and p = 0.68 for relative to body mass and r = 0.40 and p = 0.16 for relative to lean mass of lower limbs).
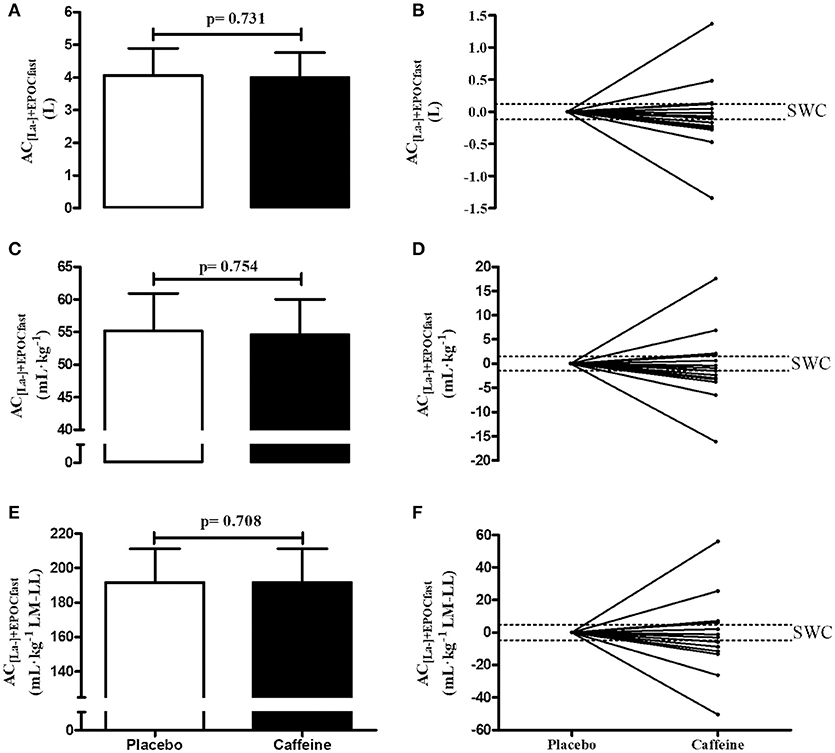
Figure 3. AC[La−]+EPOCfast values in the caffeine and placebo conditions expressed in values absolute (A,B), relative to body mass (C,D) and relative to lean mass of lower limbs (E,F) (n = 14). (A,C,F) show the values in mean and standard deviation, while the figures (B,D,F) show individual changes relative to placebo values and in relation to boundaries of smallest worthwhile change (SWC).
Table 3 shows the , RPE, EOXID, E[La], and EPCr variables at exhaustion during the supramaximal efforts after caffeine or placebo supplementation. The exhaustion and RPE in both conditions were not statistically different and demonstrated significant correlations. Furthermore, the E[La] and EPCr were not different and presented significant correlations (between conditions), while the EOXID was higher in the caffeine than in the placebo condition.
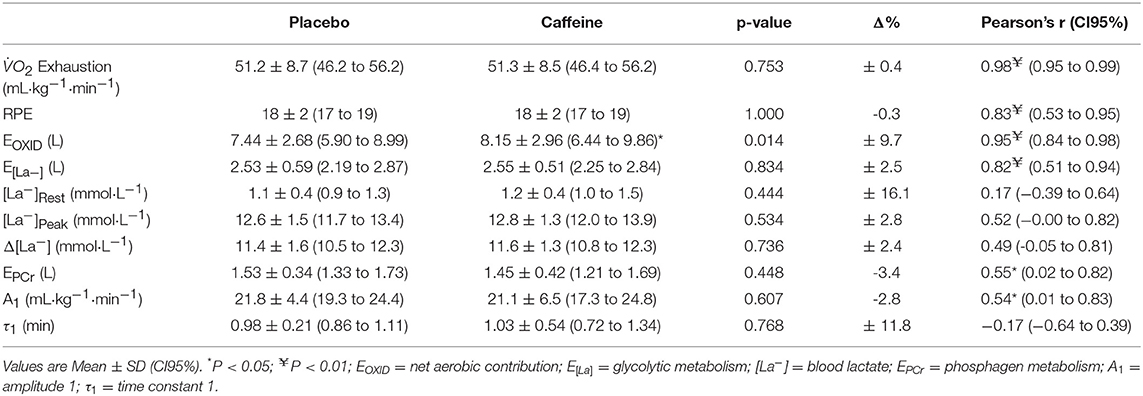
Table 3. Values of oxygen uptake () at exhaustion, rate of perceived exertion (RPE), and energetic contribution measured at 115% of i after caffeine and placebo supplementation (n = 14).
Discussion
The aim of the study was to verify the effects of acute caffeine supplementation on AC[La−]+EPOCfast in cycling. The main finding of this study was that acute caffeine supplementation improved the time to exhaustion during the supramaximal effort at 115% of i, but the AC[La−]+EPOCfast remained unaltered.
It has been shown that acute caffeine supplementation affects performance and the conventional MAOD estimate (3, 4). Bell et al. (3) found an improvement in the time to exhaustion at 125% of peak intensity and ~7% increase in the MAOD value after supplementation with 5 mg·kg−1 of caffeine in untrained subjects. Corroborating these findings, Doherty (4) found an improvement in the MAOD values (~10%) and time to exhaustion (~14%) in untrained subjects with the same caffeine dosage. The explanation of these authors for the improvement in MAOD values was the greater mobilization of the glycolytic metabolism, leading to high production and accumulation of lactate. However, Simmonds et al. (25) found that the iso-time accumulated and oxygen deficit during supramaximal efforts were similar with caffeine (5 mg·kg−1) and placebo supplementation. In addition, these authors found no differences in the kinetics during the supramaximal efforts, demonstrating that the greater MAOD values in the caffeine than placebo condition were not related to changes in the relative contribution of the aerobic and anaerobic metabolisms, but were more related to increased time to exhaustion and a consequently greater deficit. In contrast to the results of these authors, in the present study we observed an improvement in time to exhaustion (Table 3), but not in AC[La−]+EPOCfast values after caffeine ingestion.
These differences between the results found in the literature and in the present study can possibly be explained by the methodological issues involved in determining the MAOD and AC[La−]+EPOCfast. Medbø et al. (9) suggested that the supramaximal test to determine the MAOD should cause exhaustion in a minimum of 2 min due to the need to achieve the maximum capacity of energy production through anaerobic pathways. These authors demonstrated that MAOD increased with increasing time to exhaustion, reaching a plateau from 2 min on. In both the studies of Bell et al. (3) and Simmonds et al. (25) the mean values of time to exhaustion in the placebo condition (108.2 ± 8.9 s and 93.5 ± 24.1 s, respectively) were lower than the recommended time (i.e., 2–3 min) (26). Thus, this could have caused underestimation of MAOD values in the placebo condition and demonstrated a value closer to the “real” MAOD in the condition supplemented with caffeine due to the increased time to exhaustion. Considering the Simmonds et al. (25) findings that the on kinetics of are not altered with caffeine supplementation, the higher time to exhaustion causes an increase in the oxygen deficit. It is worth emphasizing that the AC[La−]+EPOCfast does not present this “limitation” as it is based on blood lactate and EPOC responses, and not directly on time to exhaustion. In addition, it has been shown that AC[La−]+EPOCfast remains unaltered at different supramaximal intensities (8).
Astorino et al. (1) found that acute supplementation with caffeine changed the magnitude of EPOC after a resistance training session. However, these authors analyzed the area under the EPOC curve, whereas in the present study we analyzed kinetics using a mathematical fitting to derive EPOCFAST, which is thought to estimate the phosphagen metabolism pathway (6–8). Regardless of the EPOC method of analysis, the variables from the mathematical adjustment (i.e., A1 and τ1) used to determine the EPCr in the present study were not statistically different. These findings do not corroborate Poli et al. (2) who found no significant differences in AC[La−]+EPOCfast determined in running after caffeine and placebo supplementation, but reported a lower τ1 in the caffeine compared to placebo condition. The main argument of the authors for these differences in τ1 is related to the greater exhaustion that allows a faster drop in after the effort. Nevertheless, in both studies the EPCr was not significantly different in the caffeine and placebo conditions. Thus, considering that the effect of caffeine does not seem to be related to increased anaerobic energy supply and a possible effect on EPCr in the caffeine condition would not be “wise,” the EPCr estimated by the EPOCFAST does not seem to be influenced by this ergogenic.
Mechanisms explaining the improvement in time to exhaustion after caffeine supplementation have been inconclusive. Possible explanations could be related to the effects of caffeine on stimulation of the central nervous system, an improvement in neuromuscular transmission, and in the contractility of muscle fiber (4). Simmonds et al. (25) suggested that caffeine can alleviate fatigue by maintaining electrolyte homeostasis at the beginning of the effort, maintaining the extracellular K+ concentration and improving the action potential of the membrane, allowing muscle contraction and performance for a longer period before the onset of fatigue. However, in the present study the factors that could explain these mechanisms were not addressed.
However, the ergogenic effect of caffeine on performance is variable and seems to be associated to CYP1A2 polymorphism (27, 28), explaining the variable individuals effects with caffeine ingestion. In a recent study, Guest et al. (27) reported that some individuals are more responsible to caffeine ingestion than other 10 km cycling time-trial performance. The caffeine is metabolized by the CYP1A2 enzyme and these authors found that individual with CC genotype (i.e., homozygous slow metabolizers) decreased the performance compared to individuals with AA (fast metabolizers) and AC (heterozygous slow metabolizers) genotypes after 4 mg/kg intake of caffeine. These authors reported that in 101 participants 49% were AA, 43% AC, and 8% CC, and these range distributions can probably explain the inter-individual variations on performance and mainly on anaerobic capacity in the current study, with inter-individual range for anaerobic capacity change with caffeine compared with placebo was −24.5 to 34.2%. In addition, the Figure 2B shows that 10 of 14 subjects were positive responders to caffeine for performance while 2 were “non-responders” and 2 “negative” responders, evidencing that caffeine ingestion does not affect the anaerobic estimation.
A possible limitation of the study was the absence ofkinetics analysis, which could partly explain the improved performance in the supramaximal effort and the unaltered AC[La−]+EPOCfast values. In addition, no analyses were performed related to the effects of caffeine on metabolite accumulation, or intracellular concentrations of K+, or any analysis to explain the mechanisms related to the effects of caffeine on the stimulation of the central nervous system, which could contribute to clarifying the issues raised. Finally, it is important to report that the current study did not investigate genetic variation and its potential effects on the relationship between caffeine and the outcomes, and therefore this highlighted the individual responses with caffeine ingestion.
Therefore, we concluded that based on the overall comparison of mean values the acute caffeine supplementation improves the time to exhaustion in a supramaximal effort, although the EPCr, E[La], and AC[La−]+EPOCfast remain unaltered. However, the effect of caffeine on the performance and anaerobic capacity depend on the individual.
Perspective
The AC[La−]+EPOCfast method has been considered an advantageous method due to the reduced time taken to estimate anaerobic capacity (6–8). In addition, this method allows discrimination of the energetic equivalent of both the glycolytic and phosphagen pathways. For this reason, the effects of different ergogenic resources specifically on metabolic pathways have been investigated (16). Caffeine intake increases the time to exhaustion in the supramaximal effort and therefore possibly influences the determination of MAOD by the conventional method (25). However, AC[La−]+EPOCfast does not appear to be time-to-exhaustion dependent (8) and the present study investigated the possible effects of caffeine due to evidence of an impact on lactate concentrations (3) and on EPOC (1). This could influence the AC[La−]+EPOCfast values due to changes in the energy equivalents of the glycolytic and phosphagen pathways. However, the Figures 2, 3 which show individual data strongly suggest that individual responses to the caffeine vary quite markedly. Therefore, the findings of the present study showed that acute caffeine intake increases the time to exhaustion in the supramaximal effort, however does not alter the components of the EPCr (i.e., amplitude and constant time of the mathematical adjustment), E[La] (i.e., lactate concentrations), and consequently the AC[La−]+EPOCfast
Author Contributions
WM participated in data acquisition, analyses and writing the manuscript. RB participated in manuscript writing. FN participated in the study design. RdP participated in data acquisition and analyses. AZ participated in the study design and writing the manuscript. All authors read and approved the final manuscript.
Funding
This work was supported by the São Paulo Research Fundation (FAPESP) under Grants [numbers 2013/12940-8, 2014/02829-5 and 2016/17836-2].
Conflict of Interest Statement
The authors declare that the research was conducted in the absence of any commercial or financial relationships that could be construed as a potential conflict of interest.
The reviewer BG and handling Editor declared their shared affiliation
Abbreviations
[La−], blood lactate concentration; [La−]Peak, peak of blood lactate concentration; [La−]Rest, rest blood lactate concentration; AC[La−]+EPOCfast, anaerobic capacity alternative estimation; E[La−], glycolytic pathway; EOXID, oxidative metabolism contribution; EPCr, phosphagen pathway; EPOCfast, fast component of the excessive post-exercise oxygen consumption; GXT, graded exercise test; HR, heart rate; i, intensity associated with maximal oxygen uptake; MAOD, Maximal accumulated oxygen deficit; RER, Respiratory exchange ratio; RPE, ating of perceived exertion; VO2, oxygen consumption; , maximal oxygen uptake; SWC, Smallest worthwhile change
References
1. Astorino TA, Martin K, Wong L, and Schachtsiek L Effect of acute caffeine ingestion on EPOC after intense resistance training. J. Sports Med. Phys. Fitness (2011) 51:11–7.
2. de Poli RAB, Miyagi, WE, Nakamura, FY, and Zagatto AM Caffeine improved time to exhaustion but did not change alternative maximal accumulated oxygen deficit estimated during a single supramaximal running bout. Int J Sport Nutr Exerc Metab. (2016) 26:549–57. doi: 10.1123/ijsnem.2016-0038
3. Bell DG, Jacobs I, and Ellerington K Effect of caffeine and ephedrine ingestion on anaerobic exercise performance. Med Sci Sports Exerc. (2001) 33:1399–403. doi: 10.1097/00005768-200108000-00024
4. Doherty M The effects of caffeine on the maximal accumulated oxygen deficit and short-term running performance. Int J Sport Nutr. (1998) 8:95–104. doi: 10.1123/ijsn.8.2.95
5. Doherty M, Smith PM, Davison RCR, and Hughes MG Caffeine is ergogenic after supplementation of oral creatine monohydrate. Med Sci Sports Exerc. (2002) 34:1785–92. doi: 10.1097/00005768-200211000-00015
6. Bertuzzi RCM, Franchini E, Ugrinowitsch C, Kokubun E, Lima-Silva AE, Pires FO, et al. Predicting MAOD using only a supramaximal exhaustive test. Int J Sports Med. (2010) 31:477–81. doi: 10.1055/s-0030-1253375
7. Miyagi WE, de Poli RAB, Papoti, M, Bertuzzi, R, and Zagatto AM Anaerobic capacityestimated in a single supramaximal test in cycling: validity and reliability analysis. Sci Rep. (2017) 7:42485. doi: 10.1038/srep42485
8. Zagatto AM, Bertuzzi R, Miyagi WE, Padulo J, and Papoti M MAOD determined in a single supramaximal test: a study on the reliability and effects of supramaximal intensities. Int J Sports Med. (2016) 37:700–7. doi: 10.1055/s-0042-104413
9. Medbø, JI Mohna, C, Tabata, I, Bahr, R, Vaage, O, and Sejersted OM Anaerobic capacity determined by maximal accumulated O2 deficit. J. Appl. Physiol. (1988) 64:50–60. doi: 10.1152/jappl.1988.64.1.50
10. Di Prampero PE, and Ferretti G The energetics of anaerobic muscle metabolism: a reappraisal of older and recent concepts. Respir Physiol. (1999) 118:103–15. doi: 10.1016/S0034-5687(99)00083-3
11. Margaria R, Edwards H, and Dill D The possible mechanisms of contracting and paying the oxygen debt and the role of lactic acid in muscular contraction. Am J Physiol. (1933) 106:689–715. doi: 10.1152/ajplegacy.1933.106.3.689
12. Zagatto AM, and Gobatto CA Relationship between anaerobic parameters provided from Maod and critical power model in specific table tennis test. Int J Sports Med. (2012) 33:613–20. doi: 10.1055/s-0032-1304648
13. Zagatto AM, De Mello Leite JV, Papoti, M, and Beneke R Energetics of table tennis and table tennis-specific exercise testing. Int J Sports Physiol Perform. (2016) 11:1012–7. doi: 10.1123/ijspp.2015-0746
14. Bertuzzi R, Kiss MAPDM, Damasceno M, Oliveira R, and Lima-Silva AE Association between anaerobic components of the maximal accumulated oxygen deficit and 30-second wingate test. Braz J Med Biol Res. (2015) 48:261–6. doi: 10.1590/1414-431X20144043
15. Zagatto AM, Nakamura FY, Milioni F, Miyagi WE, de Poli RAB, Padulo, J, et al. The sensitivity of the alternative maximal accumulated oxygen deficit method to discriminate training status. J Sports Sci. (2017) 00:1–8. doi: 10.1080/02640414.2016.1273539
16. Brisola GMP, Miyagi WE, da Silva HS, and Zagatto AM Sodium bicarbonate supplementation improved MAOD but is not correlated with 200- and 400-m running performances: a double-blind, crossover, and placebo-controlled study. App Physiol Nutr Metab. (2015) 40:931–7. doi: 10.1139/apnm-2015-0036
17. Green S Measurement of anaerobic work capacities in humans. Sport Med. (1995) 19:32–42. doi: 10.2165/00007256-199519010-00003
18. Zupan MF, Arata AW, Dawson LH, Wile AL, Payn TL, and Hannon ME Wingate anaerobic test peak power and anaerobic capacity classifications for men and women intercollegiate athletes. J Strength Cond Res. (2009) 23:2598–604. doi: 10.1519/JSC.0b013e3181b1b21b
19. Millet GP, Vleck VE, and Bentley DJ Physiological differences between cycling and running: lessons from triathletes. Sport Med. (2009) 39:179–206. doi: 10.2165/00007256-200939030-00002
20. Hill DW, and Vingren JL Effects of exercise mode and participant sex on measures of anaerobic capacity. J Sports Med Phys Fitness (2014) 54:255–63.
21. Borg GAV Psychophysical bases of percieved exertion. Med. Sci. Sport. Exerc. (1982) 14:377–81. doi: 10.1249/00005768-198205000-00012
22. Howley ET, Bassett DR, and Welch HG Criteria for maximal oxygen uptake: review and commentary. Med Sci Sports Exerc. (1995) 27:1292–301. doi: 10.1249/00005768-199509000-00009
23. Faria EW, Parker DL, and Faria IE The science of cycling: physiology and training - part 1. Sport Med. (2005) 35:285–312. doi: 10.2165/00007256-200535040-00002
24. Billat LV, and Koralsztein JP Significance of the velocity at VO2max and time to exhaustion at this velocity. Sport Med. (1996) 22:90–108. doi: 10.2165/00007256-199622020-00004
25. Simmonds MJ, Minahan CL, and Sabapathy S Caffeine improves supramaximal cycling but not the rate of anaerobic energy release. Eur J Appl Physiol. (2010) 109:287–95. doi: 10.1007/s00421-009-1351-8
26. Noordhof DA, De Koning JJ, and Foster C The maximal accumulated oxygen deficit method: a valid and reliable measure of anaerobic capacity? Sport Med. (2010) 40:285–302. doi: 10.2165/11530390-000000000-00000
27. Guest N, Corey P, Vescovi J, and El-Sohemy A Caffeine, CYP1A2 genotype, and endurance performance in athletes. Med Sci Sport Exerc. (2018) 50:1570–8. doi: 10.1249/MSS.0000000000001596
Keywords: caffeine, anaerobic capacity, performance, running, ergogenic aids
Citation: Miyagi WE, Bertuzzi RC, Nakamura FY, de Poli RAB and Zagatto AM (2018) Effects of Caffeine Ingestion on Anaerobic Capacity in a Single Supramaximal Cycling Test. Front. Nutr. 5:86. doi: 10.3389/fnut.2018.00086
Received: 06 February 2018; Accepted: 28 August 2018;
Published: 20 September 2018.
Edited by:
Ahmed El-Sohemy, University of Toronto, CanadaReviewed by:
Bibiana Garcia-Bailo, University of Toronto, CanadaChristopher Womack, James Madison University, United States
Nathaniel David Moyer Jenkins, Oklahoma State University, United States
Copyright © 2018 Miyagi, Bertuzzi, Nakamura, de Poli and Zagatto. This is an open-access article distributed under the terms of the Creative Commons Attribution License (CC BY). The use, distribution or reproduction in other forums is permitted, provided the original author(s) and the copyright owner(s) are credited and that the original publication in this journal is cited, in accordance with accepted academic practice. No use, distribution or reproduction is permitted which does not comply with these terms.
*Correspondence: Alessandro M. Zagatto, azagatto@yahoo.com.br