- 1Applied Physiology and Nutrition Research Group, University of São Paulo, São Paulo, Brazil
- 2Laboratory of Nutritional Biochemistry, University of Brasília, Brasília, Brazil
- 3São Camilo University Centre, São Paulo, Brazil
- 4School of Physical Education and Sport, University of São Paulo, São Paulo, Brazil
- 5Sports Nutrition and Performance Group, Department of Sport and Physical Activity, Edge Hill University, Ormskirk, United Kingdom
- 6Department of Sport and Movement Studies, Faculty of Health Science, University of Johannesburg, Johannesburg, South Africa
- 7Rheumatology Division, Faculty of Medicine, University of São Paulo, São Paulo, Brazil
- 8Institute of Orthopaedics and Traumatology, Faculty of Medicine, University of São Paulo, São Paulo, Brazil
Blood alkalosis, as indicated by an increased blood bicarbonate concentration and pH, has been shown to be beneficial for exercise performance. Sodium bicarbonate, sodium citrate, and sodium or calcium lactate, can all result in increased circulating bicarbonate and have all independently been shown to improve exercise capacity and performance under various circumstances. Although there is considerable evidence demonstrating the efficacy of these supplements in several sports-specific situations, it is commonly acknowledged that their efficacy is equivocal, due to contrasting evidence. Herein, we discuss the physiological and environmental factors that may modify the effectiveness of these supplements including, (i) absolute changes in circulating bicarbonate; (ii) supplement timing, (iii) the exercise task performed, (iv) monocarboxylate transporter (MCT) activity; (v) training status, and (vi) associated side-effects. The aim of this narrative review is to highlight the factors which may modify the response to these supplements, so that individuals can use this information to attempt to optimize supplementation and allow the greatest possibility of an ergogenic effect.
Introduction
Substances capable of increasing extracellular buffering capacity to combat exercise induced acidosis have been researched for almost a century. Sodium bicarbonate, sodium citrate, and sodium/calcium lactate can all result in alkalosis, indicated by an increase in blood pH and circulating bicarbonate, and all have independently been shown to improve exercise capacity and performance under various circumstances (1). Despite considerable evidence demonstrating these supplements to be effective in specific situations (2, 3), it is commonly acknowledged that their efficacy is equivocal due to contrasting evidence. Contradictory results from different investigations are commonly cited to highlight that the ergogenic effect from increased bicarbonate concentration is highly variable and is due to a variability in the individual responses. However, this may be oversimplifying the complex nature of individual responses (i.e., physiological and genetic differences), whilst also overlooking experimental differences (loading and exercise protocols), sample populations (i.e., untrained; physically active; trained individuals), and design flaws (e.g., exercise unlikely to be affected by increased buffering capacity).
Recent developments in the way in which researchers analyze and interpret data is moving away from group means and toward more in-depth analysis of individuals responses (4). This is because we now know that there are several contributing factors that determine how individuals respond to supplementation, including their genetic composition, training status, habitual diet, and other circumstantial factors. Identification of any modifying factors that may alter the individual response to supplementation with extracellular buffering agents would provide vital information to clinicians, coaches, and athletes about the likelihood of gaining a positive or negative response. This would aid them in making fully informed decisions and optimize each individual's personal supplementation protocol. The aim of this review is to summarize the current evidence on the potential modifying factors underlying the individual response to supplementation with extracellular buffering agents.
The Role of pH in Skeletal Muscle Fatigue
The ability of skeletal muscle tissue to maintain energy production and generate mechanical work is closely linked to performance in a variety of sports. The high turnover rate of skeletal muscle ATP that is seen during high-intensity exercise increases hydrogen ion (H+) production, leading to muscle acidosis that is associated with performance loss (5). This is because an exercise-induced metabolic acidosis, characterized by a higher H+ production rather than removal rate, can decrease energy substrate generation via glycolytic pathways by reducing the activity of key enzymes such as glycogen phosphorylase and phosphofructokinase (6). The H+ also compete with calcium ions for the troponin binding site, directly hindering the muscle's contraction capacity (7). Oxidative phosphorylation can also be inhibited by acidosis (6) while resynthesis of phosphorylcreatine may also be compromised at low pH (8). Thus, a myoplasmic drop in pH drives an inability to produce the desired or required power output with a subsequent loss of exercise performance (9), although not all agree (10).
The body has several endogenous systems to control pH homeostasis; this balance is maintained by intracellular and extracellular buffers which can accept or release H+ to prevent dramatic pH changes. In muscle, intracellular physicochemical buffers such as organic and inorganic phosphates, bicarbonate anions, and histidine containing dipeptides, are the primary mediators of pH homeostasis. There is also an active and passive transport of H+ out of the muscle into the blood mediated by transport systems; during intense activity, H+ efflux is mainly mediated by the lactate-proton transporters, namely monocarboxylate transporter 1 (MCT1) and 4 (MCT4) (11). Thereafter, the H+ are buffered by the circulating anion bicarbonate (), which forms carbonic acid, a weak acid. These endogenous buffering systems are well-regulated and highly efficient under normal physiological conditions (12). However, these systems can be quickly overwhelmed by the accumulation of H+ during exercise, particularly when the intensity is high. Thus, increasing the buffering contribution of one or more of these systems is a feasible way to improve control of systemic pH changes and maintain exercise performance.
Extracellular Buffering Agents
Several buffering agents are employed as ergogenic supplements, including sodium bicarbonate (SB), sodium citrate (SC), sodium lactate (SL), and calcium lactate (CL); the independent mechanisms through which they increase circulating bicarbonate will not be discussed here (for review see Lancha Junior et al. (1)). Nonetheless, all substances are ingested with the same focus: to increase the extracellular concentration of bicarbonate, increasing H+ efflux out of the working muscle, thereby contributing to muscle acid-base balance during exercise, which may lead to an improved performance.
The ability of increased circulating bicarbonate to improve exercise capacity and performance has been extensively studied. It is widely acknowledged that the most effective extracellular buffer is SB, with numerous narrative reviews (13, 14) and meta-analyses (2, 3, 15, 16) demonstrating its efficacy. Meta-analytic data have suggested SC supplementation to be ineffective for performance (2), while limited data on SL and CL mean that no meta-analytical data on their efficacy currently exists. Despite this, supplementation with SC, SL, and CL has been shown to improve exercise capacity and performance on numerous independent occasions. Contrasting results in study outcomes following ingestion of these supplements is often cited as evidence that the response to these buffering supplements is variable. Such generalized statements do not consider the numerous contributing and modifying factors that influence the response to supplementation and do not allow any single individual to identify whether they are likely to benefit from supplementation or not. Thus, it is of importance to determine the factors that may contribute to an individual's response to these supplements.
Potential Influencing Factors on the Efficacy of Extracellular Buffers to Improve Exercise Capacity and Performance
Increases in Circulating Bicarbonate
To gain a competitive advantage from increased extracellular buffering capacity, there needs to be an increase in circulating bicarbonate following supplementation. Theoretically, any increase in bicarbonate would lead to a corresponding increase in buffering capacity; what the minimal increase necessary is to elicit performance gains is currently unknown. Carr et al. (17) have suggested that a +5 mmol·L−1 increase from baseline levels is required to have a potential ergogenic benefit in exercise performance, while a +6 mmol·L−1 increase leads to almost certain ergogenic benefits. Despite this, no study to date has directly investigated the minimal increase necessary for performance gains or linked individual increases to change in performance. Saunders et al. (18) determined whether there were any correlations between blood values (bicarbonate, pH, base excess) and exercise capacity. Surprisingly, we did not show any relationship between the magnitude of change in circulating bicarbonate (or any other measure) and subsequent changes in exercise capacity.
Jones et al. (19) showed that no individual's maximal increases in circulating bicarbonate with a 0.1 g·kg−1 BM dose reached a +5 mmol·L−1 increase, which could explain why this dose appears to be ineffective for exercise performance (20). A 0.2 g·kg−1 BM dose, however, was effective at reaching this threshold in all individuals (19), although the mean increase 60 min post-ingestion was < +5 mmol·L−1. Nonetheless, it is possible that 60 min post-ingestion could coincide with a sufficient increase (i.e., > +5 mmol·L−1) in bicarbonate for some individuals. This could partially explain why a 0.2 g·kg−1 dose appears to be effective in some (20, 21), but not all (22–24), studies when exercise was performed 1 h post-supplementation. The time to reach this suggested minimal threshold varies between individuals (19), meaning it is possible that a higher proportion of individuals had not attained sufficient alkalosis in the studies showing no effect than those showing an effect, contributing to the group effects shown.
A 0.3 g·kg−1 BM dose of SB is the most commonly employed in the literature and appears to stem from the work of McNaughton who showed this to be most effective compared to lower and higher doses (20). Mean increases in circulating bicarbonate prior to exercise following a 0.3 g·kg−1 BM dose of SB is approximately +5–6 mmol·L−1 (25–27), and thus should be sufficient to improve exercise capacity and performance (19). Indeed, numerous studies have shown exercise gains with SB at this dose (28–38), though similarly, substantial data exist showing no effect following supplementation (26, 27, 34, 39–43). This may, in part, be explained by the absolute increases in blood bicarbonate shown prior to exercise, which appears to differ substantially between studies (Figure 1). Certainly, this visual demonstrates the importance in mean increases in circulating bicarbonate prior to subsequent exercise performance, although it must be acknowledged that several further contributing factors may account for this variation including exercise models not limited by H+ accumulation, genotype, associated-side effects, and individual variation in the response to supplementation.
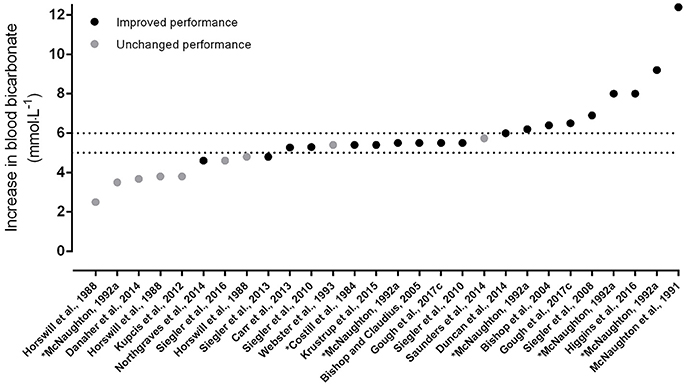
Figure 1. Increases in blood bicarbonate from baseline following acute supplementation with sodium bicarbonate, in order of magnitude of change. Data points indicate whether exercise performance was improved with supplementation (dark circles) or not (light circles). The dotted lines indicate the thresholds for the zone of a potential ergogenic effect (+5 mmol·L−1) and the zone of an almost certain ergogenic effect (+6 mmol·L−1); taken from Carr et al. (2) and Jones et al. (19). *Denotes data estimated from graphs using specialized software (18, 20, 21, 23, 26, 29, 30, 33, 38, 42–53).
Few studies have investigated the effect of repeated supplementation in the same individuals using the same exercise protocol. We previously showed that SB supplementation in the same individual produced consistent blood bicarbonate responses, but this only translated into a mean improvement in one of the four sessions when SB was ingested (27). Interestingly, only one individual improved on all four occasions and nine others on at least one occasion, suggesting that subsequent exercise performance is more variable than blood responses. Nonetheless, although mean increases in circulating bicarbonate were approximately +6 mmol·L−1 during each session, not all individuals may have achieved a sufficient increase in circulating bicarbonate on every occasion. Additionally, inconsistencies may also be due to the training status of the participants who were recreationally active; trained individuals are likely to perform more consistently with lower variation between tests and thus may be better able to consistently take advantage of increased buffering capacity. Indeed, Carr et al. (41) showed 2,000 m rowing performance was reliable following repeated acute (0.3 g·kg−1 BM) and chronic (0.5 g·kg−1·day−1 BM for 3 days) supplementation in well-trained rowers, although performance was not improved. Trained cyclists and triathletes did consistently improve their cycling tolerance when administered SB on repeated occasions (35). Thus, it is possible that a proportion of the inconsistency in the response to SB (and potentially SC, CL, and SL) may be attributed to the training status of the individual and it would be of interest to determine the within-participant repeatability following supplementation using trained individuals.
Like the 0.3 g·kg−1 BM dose of SB, the most commonly employed dose of SC is 0.5 g·kg−1 BM and can be attributed to the pioneering work of McNaughton (54). Significant increases in bicarbonate were shown 90 min following a dose as low as 0.1 g·kg−1 BM, but since circulating bicarbonate increased in a linear fashion, and the greatest amount of work completed during a 60-s maximal exercise test was following a 0.5 g·kg−1 BM dose, this was considered the most effective. And yet, despite impressive increases in blood bicarbonate following SC supplementation, numerous studies have shown no effect on exercise capacity or performance (55–57). In fact, meta-analytic data showed an unclear effect of SC on exercise, with pre-exercise alkalosis associated with a small but unclear effect on performance (2). Indeed, despite clear increases in blood bicarbonate, several studies have shown no positive effect on subsequent exercise capacity or performance theoretically limited by acidosis (Figure 2). Aside from other confounding factors, discussed herein that may have modified the ergogenic effect of supplementation with SC, these increases may be counteracted by some other physiological response which inhibits the ergogenic effect of increased buffering capacity.
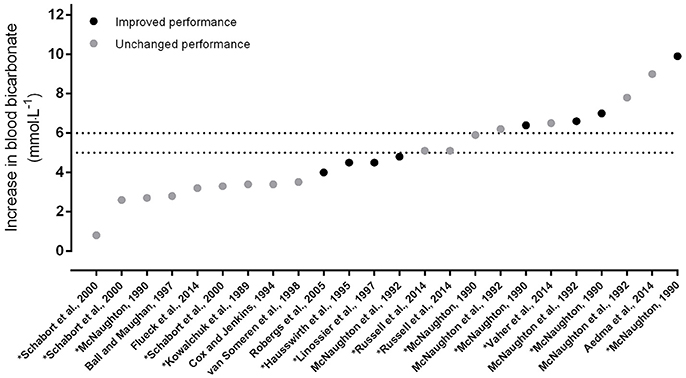
Figure 2. Increases in blood bicarbonate from baseline following acute supplementation with sodium citrate, in order of magnitude of change. Data points indicate whether exercise performance was improved with supplementation (dark circles) or not (light circles). The dotted lines indicate the thresholds for the zone of a potential ergogenic effect (+5 mmol·L−1) and the zone of an almost certain ergogenic effect (+6 mmol·L−1); taken from Carr et al. (2) and Jones et al. (19). *Denotes data estimated from graphs using specialized software (24), (54–66).
Increases in blood bicarbonate following ingestion of calcium or sodium lactate appear less obvious. Several studies have shown increases of the magnitude of ~+3 mmol·L−1 (67, 68) while further studies showed no increase in circulating bicarbonate following acute (26) and chronic (69) supplementation, which explains the lack of an ergogenic effect in these studies. The +3 mmol·L−1 increase shown by Morris et al. (68) was sufficient to improve exercise tolerance by 17% during a cycling capacity test. Similarly, Morris et al. (70) showed improved high-intensity exercise capacity with mean bicarbonate increases of only +2.5 and +2.6 mmol·L−1 following supplementation of 120 and 300 mg·kg−1 BM of CL. Lactate supplementation improved running performance by a modest 1.7% (71), although changes in bicarbonate in this study cannot be determined since blood was only sampled at a solitary timepoint immediately prior to exercise. Low (150 mg·kg−1 BM) and high (300 mg·kg−1 BM) doses of CL lead to only moderate increases of +2 mmol·L−1 of bicarbonate, with no subsequent effect on repeated-bout high-intensity exercise (72). Certainly, the modest increases in blood bicarbonate with lactate supplementation appear to be the primary factor behind the lack of an ergogenic effect shown in some, but not all, studies with lactate supplementation (Figure 3). Certainly, the individual range of bicarbonate increases could have contributed to this variability as, for example, the range in increases with low and high doses of calcium was between +0.4 and +6.7 mmol·L−1 and changes in bicarbonate were positively associated with the improvements in exercise capacity (70). More studies should relate the individual increases in circulating bicarbonate to changes in performance to provide insight into this relationship.
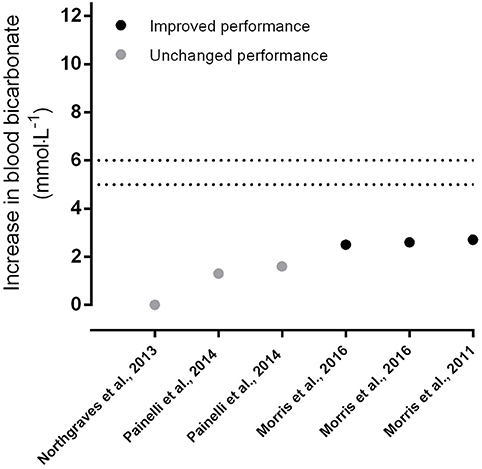
Figure 3. Increases in blood bicarbonate from baseline following acute supplementation with calcium or sodium lactate, in order of magnitude of change. Data points indicate whether exercise performance was improved with supplementation (dark circles) or not (light circles). The dotted lines indicate the thresholds for the zone of a potential ergogenic effect (+5 mmol·L−1) and the zone of an almost certain ergogenic effect (+6 mmol·L−1); taken from Carr et al. (2) and Jones et al. (19). *Denotes data estimated from graphs using specialized software (26, 68, 70, 72).
It is currently unclear what the minimal increase in circulating bicarbonate necessary is to result in an improvement in exercise performance. This has been suggested to be of the order of +5 mmol·L−1 (17), although studies have shown mean increases as little as +2.5 mmol·L−1 are sufficient to improve exercise tolerance (70), while +2 mmol·L−1 generally do not (72). Further research should investigate the smallest worthwhile change in blood bicarbonate that can consistently lead to exercise gains.
Ingestion Timing
Siegler et al. (25) showed that increases in circulating bicarbonate and absolute concentration are similar at 60, 120, and 180 min post-ingestion of 0.3 g·kg−1 BM SB. Since performance during their repeated sprint protocol was not different when performed at these different post-ingestion timepoints, it could be assumed that precise timing of supplementation is of little importance to obtain performance gains. Nonetheless, recent interest has focused on the peak changes in circulating bicarbonate, with theory suggesting that maximal increases in blood bicarbonate will lead to maximal performance benefits, or at least the greatest chance of improvements. Most studies have employed a standardized supplementation protocol at a uniform timepoint. Although the data of Siegler et al. (25) showed that mean changes in circulating bicarbonate did not differ between timepoints, it is unlikely that all individuals performed exercise at a point which coincided with peak alkalosis.
A recent spate of investigations reporting the time course changes in blood markers following acute supplementation with SB (19, 73, 74), SC (75), and lactate (26, 72) suggest that a uniform supplementation timepoint is unlikely to be optimal for all individuals. The overwhelming conclusion that can be drawn from these independent investigations is that the time-to-peak bicarbonate concentration differs drastically between individuals, as does the absolute maximal change. Jones et al. (19) showed that peak bicarbonate concentration occurred between 30 and 150, 40 and 165, and 75 and 180 min following 0.1, 0.2, and 0.3 g·kg−1 BM of SB. Another study showed one individual who peaked only 10 min following supplementation of 0.3 g·kg−1 BM of SB (76). Absolute maximal changes in bicarbonate following SB ingestion varies greatly between individuals (Table 1), which could be a contributing factor to why some individuals respond more than others. Urwin et al. (75) showed that peak bicarbonate occurred much later than the commonly employed time given for supplementation with SC; indeed, it has recently been suggested that this may be a key factor contributing to the unclear effect shown with SC (77). Thus, if the greatest likelihood of an ergogenic effect occurs at the moment of peak circulating bicarbonate, then it is of great importance to determine individual time-to-peak bicarbonate following supplementation.
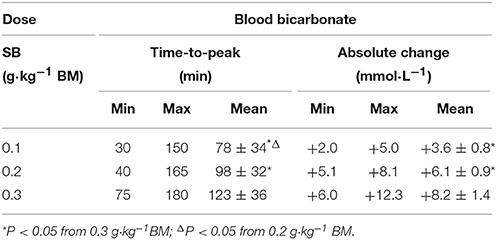
Table 1. Minimum (Min), maximum (Max), and mean time-to-peak and absolute maximal increases in blood bicarbonate concentration following supplementation with 0.1, 0.2, and 0.3 g·kg−1 BM sodium bicarbonate (SB) in Jones et al. (19).
Determination of the time-to-peak bicarbonate concentration is only worthwhile for repeat ingestion if subsequent blood responses are reproducible. The consistency in blood responses following supplementation with extracellular buffers has only been determined with SB. Although both blood pH and bicarbonate responses showed good reliability following repeated supplementation with both 0.2 and 0.3 g·kg−1 BM doses of SB, blood bicarbonate responses were more reproducible, suggesting any individualized ingestion strategy should be based upon the time-to-peak bicarbonate (73). Nonetheless, although no statistical differences were shown in the time-to-peak, or the absolute maximal changes, it currently remains unclear whether minor changes in circulating bicarbonate significantly influence changes in exercise performance.
Two studies have investigated the effect of peak circulating bicarbonate concentration on exercise performance, both following SB supplementation. Overall repeated sprint performance was significantly improved with 0.3 g·kg−1 BM of SB compared to placebo and control (76). More recently it has been shown that 4-km cycling time-trial performance could be improved following both 0.2 and 0.3 g·kg−1 BM of SB when individualized to the time-to-peak blood bicarbonate (48). These findings are interesting considering two previous investigations showed no effect of SB supplementation on 4-km time-trial performance following a 0.3 g·kg−1 BM dose. Discrepancies could be due to timing and subsequent increases in circulating bicarbonate. Mean bicarbonate increases in Gough et al. (48) were +6.5 ± 1.3 mmol·L−1, which is higher than the approximate +3 mmol·L−1 (78) and +5 mmol·L−1 (79) shown 150 and 100 min following supplement ingestion in the other studies. Despite these initial positive results, no data exist directly comparing exercise performed at the moment of peak circulating bicarbonate to a standardized time (e.g., 60 min) following supplementation in the same participants. As it stands, it cannot currently be concluded that exercise performed at peak bicarbonate concentrations elicits greater exercise improvements than normally seen with standardized ingestion prior to exercise.
Interestingly, recent data with SB show that determining time-to-peak blood bicarbonate concentration may allow a lower dose to be employed since maximal increases with 0.2 g·kg−1 BM were similar to those seen with 0.3 g·kg−1 BM, while 4-km time-trial performance was not different between these two doses (80). The absolute difference in maximal bicarbonate increases between these doses was ~1 mmol·L−1, suggesting that such a difference may not be meaningful. Since peak bicarbonate is only assessed by the absolute maximal value, and not via some statistical inference, it is possible that minor fluctuations in the actual bicarbonate concentration following large increases may not significantly influence subsequent exercise further, although work remains to be done to determine the smallest worthwhile change in blood bicarbonate that would influence exercise performance.
Exercise Task
An increase in buffering capacity via increases in circulating bicarbonate can lead to improvements in exercise capacity and performance, and the magnitude of this increase in buffering capacity appears to influence the likelihood of an effect, although even following substantial increases not all research is unanimous. These equivocal findings may also be due to the exercise protocols employed. If improvements are to be gained from increases in buffering capacity, exercise must be limited by increases in H+ accumulation. Certainly, it has been suggested that short-duration exercise (in this case a 30 s cycle sprint) is unaffected by changes in muscle pH (81), while more endurance based activities rely increasingly on aerobic energy sources with no further increases in muscle acidosis. Therefore, improvements following supplementation with SB, SC, and CL and SL, are likely confined to specific exercise tasks which are significantly influenced by changes in acid-base balance. In fact, beta-alanine supplementation, which increases intracellular buffering capacity via increased muscle carnosine content, has been shown to be most effective during high-intensity exercise of 30 s to 10 min in duration, while longer and shorter duration exercise was unaffected (82). Since the mechanism of action of increased circulating bicarbonate is like that of beta-alanine supplementation (i.e., increased buffering capacity), comparable results would be expected.
Indeed, research appears to suggest exactly this. Despite similar increases in circulating bicarbonate following a 0.3 g·kg−1 BM dose of SB, exercise lasting 10 and 30 s in duration was unaffected, while exercise 120 and 240 s in duration was significantly improved (83). Identical results were shown following supplementation with 0.5 g·kg−1 BM of SC (63). Although some evidence suggests that buffering agents may be of benefit to longer duration exercise (36, 84), most studies report no improvements in continuous endurance exercise following supplementation (26, 57, 58, 85, 86). Multiple-bout high-intensity exercise, which has been shown to result in greater muscle acidosis than continuous supra-maximal exercise (87, 88), appears particularly susceptible to improvements with these supplements (21, 28–30, 38, 76, 89). Interestingly, Higgins et al. (34) showed that SB improved exercise capacity at 100% peak mean minute power, but not at 110 or 120%, despite the duration of all these exercise tasks being within the timeframe where an effect may be most likely expected (82).
Taken collectively, these results suggest that the likelihood of gaining a competitive edge from increased bicarbonate may be, in part, dependent on the exercise performed; the duration and intensity of the exercise appear to be key factors in this. Thus, the exercise task performed will be a determining factor in whether supplementation will be beneficial and cannot be overlooked; exercise gains with these substances should only be expected during exercise limited by the accumulation of H+. Individuals should base their decision to supplement on their own exercise demands and the likelihood of gaining a worthwhile improvement therein. These are likely to be continuous high-intensity exercise tasks, such as 4 km cycling, 100 and 200 m swimming, and 2,000 m rowing, or repeated high-intensity activities such as those performed during team sports (e.g., football, hockey, basketball, etc).
Hydrogen Ion Transporters
The H+ that accumulate in muscle throughout high-intensity exercise are predominantly removed by MCT1 and MCT4, through co-transport with lactate in a 1:1 ratio (11, 90). The sodium/hydrogen transport system (NHE) may also contribute to this process (91), although its relative contribution during exercise has been suggested to be minimal (90, 92). It is widely acknowledged that increased blood bicarbonate increases the activity of these MCT transporters, increasing the efflux of H+ out of the muscle and reducing muscle acidosis (93). Surprisingly, no study to date has directly measured the effect of increased circulating bicarbonate via supplementation on the activity of these transporters in humans. It is currently unclear whether the relationship between the increased bicarbonate and the activity of these transporters is intrinsically associated.
Lactate transport has been shown to be elevated in athletes (94). This makes sense since training interventions have been shown to increase the abundance and activity of MCT transporters (95, 96). Furthermore, lactate transport capacity (and subsequently H+ transport) was related to the occurrence of type I muscle fibers (97). MCT1 and MCT4 are both expressed in human skeletal muscle, although MCT1 is more prevalent in type I fibers and MCT4 in type II fibers (98). The higher affinity of MCT4 (Km = 28–34 mM) compared to MCT1 (Km = 4–6 mM) for lactate, in addition to its higher prevalence in glycolytic fibers, suggests that MCT4 may be more important for efflux out of the working muscle (99). Hence, it could be suggested that any differences in training status or muscle fiber type predominance (i.e., trained sprinters vs. untrained individuals) may modify the response to supplementation with extracellular buffering agents. Indeed, this could, in part, explain some of the variation in responses between trained and non-trained individuals; a meta-analysis showed that the effect of SB supplementation on exercise was significantly lower for specifically trained compared to recreationally trained individuals (16). However, the exact mechanisms for this reduced effect has not been investigated and thus, suggestion that this may be due to differences in MCT transporter activity is currently highly speculative and warrants further investigation.
Polymorphisms in the MCT transporters may also influence an individual's response to supplementation. A single-nucleotide polymorphism in the gene coding for MCT1, for example, can influence the lactate/H+ co-transport across the sarcolemma (100). The T allele carriers have been shown to have a reduced MCT1 lactate transport (101, 102), TT homozygotes reach the ventilatory threshold at higher speeds (103), while T allele frequency has been shown to differ between athletes of different modalities (100, 104). Despite this, the effect of this polymorphism on high-intensity exercise performance, with or without supplementation, is currently unclear. Although genetic variations of the MCT4 gene exist (105), to our knowledge, no study has investigated the relevance of any polymorphisms on lactate/H+ flux and its effect on exercise performance. It is apparent that polymorphisms in the genes encoding for MCT1 and MCT4 could alter their activity during high-intensity activity; it could thus be implied that genetic differences may also modify the individual response to supplementation with buffering agents, although no data currently exist. It is essential that more research on genotypic differences in MCT transporters and response to supplementation is undertaken before any recommendations can be made.
Associated Side-Effects
An evident moderator of the efficacy of buffering agents to improve exercise capacity and performance, is their associated side-effects. The occurrence of gastrointestinal (GI) discomfort with these supplements is common, with stomach cramps, nausea, vomiting, and diarrhea among some of the most frequent complaints with SB and SC (17, 75), although the only reported side-effects with lactate supplementation was increased incidence of belching and flatulence (72). This has obvious implications for athletes considering supplementing during competition and is likely a contributing factor to why incidence of supplementation with such agents is low (106).
Following ingestion of SB, it is dissociated in the stomach acid to form sodium (Na+) and bicarbonate, much of the latter of which is swiftly neutralized by H+, thereby producing carbon dioxide [CO2] (107). The production of CO2 in the stomach may cause gastric discomfort with symptoms including bloating and abdominal pain; nausea and vomiting are other commonly reported side effects (17). A contributing factor to the intensity of any side-effects with SB is certainly dose, as McNaughton et al. (20) reported increasing discomfort with increasing doses above 0.3 g·kg−1 BM, with no concomitant increases in performance. The typical 0.3 g·kg−1 BM often results in reported side-effects which may moderate performance (2, 16), although whether they directly influence performance may be disputed. Indeed, Price and Simons (40) reported no association between GI discomfort and performance while data from our laboratory has shown on several occasions that exercise capacity may be modified by GI discomfort (18, 27). Specifically, all individuals reporting discomfort worsened performance, and reanalyzing data following the removal of these individuals modified the group level statistics, turning a non-significant result into a significant result. Thus, it is apparent that minimizing the discomfort associated with SB supplementation could increase the likelihood of a positive response, both at the individual and group level. A potential solution is to ingest SB in gastro-resistant capsules, avoiding neutralization in the stomach and the associated side-effects; this theory is currently being tested in our laboratory.
The timing of supplement ingestion may be a key modifiable component that can increase or decrease the likelihood of an ergogenic effect not only due to increased circulating bicarbonate, but also due to the associated side-effects. Siegler et al. (25) reported lower symptoms of GI discomfort 180 min following SB supplementation (compared to 60 and 120 min). The most intense symptoms with SC were 60–120 min following supplementation (75). Since supplements are commonly ingested at a standardized time, often 60 min prior to the commencement of exercise as in the case of SB, this suggests that most studies may have forced individuals to exercise at a time when they experience sufficient discomfort to negatively impact their performance. Thus, adopting an individual time-to-peak bicarbonate supplementation protocol would likely avoid performing exercise when associated side-effects are most intense. Although we have shown that side-effects with these supplements could modify their ergogenic effect, it cannot explain the lack of an effect in all individuals. Nonetheless, it is apparent that offsetting any discomfort with supplementation will avoid any possibility that side-effects will affect the exercise response.
Optimizing Supplementation
It is becoming apparent that timing of ingestion, may be one of the key moderating factors to the ergogenic response to increased circulating bicarbonate. Coinciding exercise with the point at which bicarbonate peaks is likely to maximize the chance of exercise improvements since buffering capacity will be maximized. Importantly, early reports suggest that the time at which peak bicarbonate occurs with SB is repeatable within individuals (73); more information is needed regarding consistency in blood bicarbonate responses to other buffering agents (SC, SL, and CL). Furthermore, peak GI discomfort has been shown to occur earlier than maximal increases in blood bicarbonate following SB (73, 80) and SC (75) supplementation. Reported side-effects with lactate supplementation are minimal and mean peak bicarbonate concentration occurred after 90 min although further information is necessary regarding the individual time course response. Altogether, data suggest that individualizing supplementation timing with these agents may provide the best opportunity of gaining an ergogenic effect by coinciding the moment of exercise with peak bicarbonate concentration following the selected dose.
In fact, supplement dose is another highly modifiable factor that could be adapted to optimize supplementation. Increasing doses of SB above 0.3 g·kg−1 BM results in increased incidence of GI discomfort, while 0.1 g·kg−1 BM appears insufficient to result in exercise improvements (20). Although 0.3 g·kg−1 BM has long been considered the optimal dose, recent evidence suggests that 0.2 g·kg−1 BM may be equally efficient at improving performance (80). Considering the lower incidence of GI discomfort with similar gains in bicarbonate, a 0.2 g·kg−1 BM ingested at a timepoint specific to the individual may be the most effective way of maximizing the chances of an ergogenic effect with SB. SC increased blood bicarbonate in a linear manner in doses increasing from 0.1 to 0.5 g·kg−1 BM (54); doses above 0.5 g·kg−1 BM of SC showed no further gains in blood bicarbonate but increased the incidence and severity of side-effects (75) suggesting 0.5 g·kg−1 BM of SC to be the optimal dose. Lactate supplementation results in modest increases in bicarbonate regardless of a 0.15 or a 0.3 g·kg−1 BM dose although no significant GI discomfort. Nonetheless, current doses of up to 0.3 g·kg−1 BM with CL or SL may be insufficient to improve exercise though further work is necessary to ascertain whether higher doses may be more beneficial.
Practical Recommendations
Individuals aiming to supplement with these substances should trial them outside of competition to see what works for them and adapt and optimize their individual supplementation strategies according to their own personal needs and responses. Since the time course response has been shown to be consistent (at least following SB ingestion), ingestion should occur at a time that means exercise begins when bicarbonate concentration has increased above this +5–6 mmol·L−1 threshold. Although there may be logistical difficulties, it would be necessary to determine the timeframe during which individuals increase their bicarbonate levels above +5–6 mmol·L−1, and side-effects are minimal, so that they can adapt their supplementation timing accordingly to optimize their chances of an effect. Individuals should be wary that any improvements in exercise are likely to be marginal, although this may be worthwhile for highly-trained or elite athletes. Furthermore, the type of exercise that individuals undertake will influence the likelihood of any benefit from increased bicarbonate. Short-duration high-intensity exercise, such as 4 km cycling, 2,000 m rowing, and 200 m swimming, are examples of activities likely to profit from increased buffering capacity and there is also evidence that individuals involved in prolonged intermittent exercise may benefit from supplementation. It appears the likelihood of obtaining an ergogenic effect is greater when SB is ingested compared to SC, SL, or CL, and it is the recommendation of these authors that 0.2–0.3 g·kg−1 BM SB is taken as the preferred supplement where possible to improve high-intensity exercise capacity and performance limited by acidosis.
Conclusions and Future Direction
Sodium bicarbonate, sodium citrate and sodium/calcium lactate are supplements to increase circulating bicarbonate and have all independently been shown to improve exercise capacity and performance under various conditions. However, several factors may modify their ergogenic effects including supplement timing and dose, absolute changes in circulating bicarbonate, the exercise task being performed, MCT activity, training status, and associated side-effects (Figure 4). Emerging evidence suggests that, to maximize the chances of an ergogenic effect, supplementation should occur at a time point that results in exercise being performed at the moment of peak bicarbonate concentration, with minimal or no side-effects. More information is required regarding each supplement independently and their interaction with the individual, genotype, and the environment; these have been detailed throughout this article.
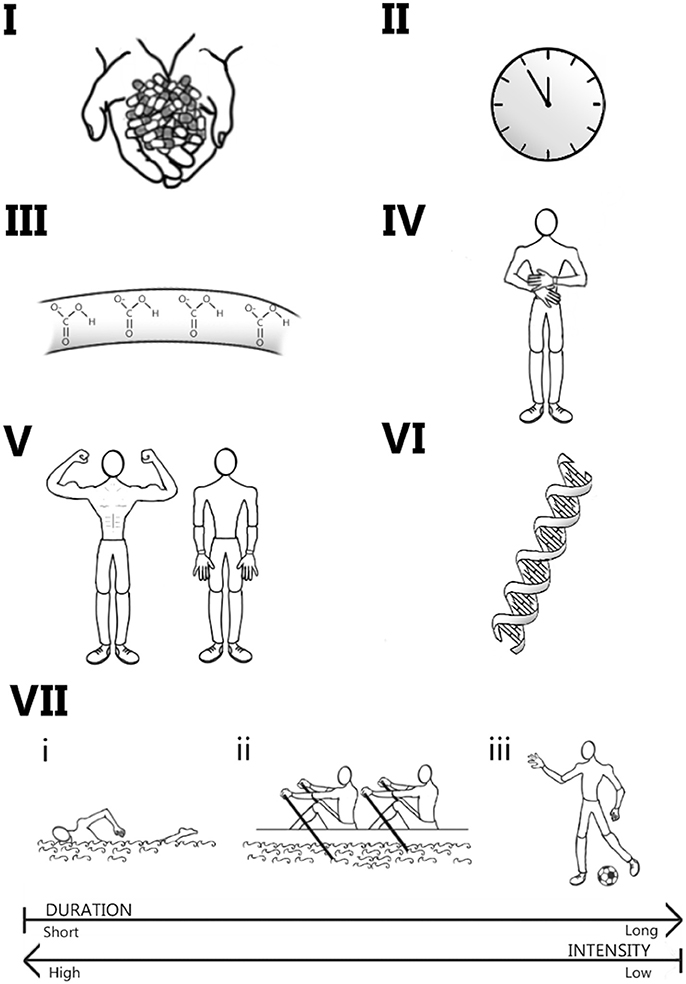
Figure 4. Overview of the factors which may modify the response to supplementation with sodium bicarbonate, sodium citrate, and calcium and sodium lactate. The dose (Panel I) and timing of ingestion (Panel II) can influence both the increases in circulating bicarbonate (Panel III) and associated side-effects (Panel IV) at the moment of exercise, impacting upon the likelihood of a performance benefit. Training status (Panel V) and genetic make-up (Panel VI) of the individual may further modify this response. The chances of a positive response may also be modified by the duration and intensity of the exercise task being performed (Panel VII): short-duration continuous exercise, such as 100 and 200 m swimming (i) and 2,000 m rowing (ii), and prolonged intermittent activity, such as football (iii), may be particularly susceptible to improvements with these supplements.
Author Contributions
BS is responsible for the conception of the work. AH, PP, LO, and BS are responsible for the writing of the manuscript. LM reviewed the manuscript.
Conflict of Interest Statement
The authors declare that the research was conducted in the absence of any commercial or financial relationships that could be construed as a potential conflict of interest.
Acknowledgments
BS (2016/50438-0 and 2017/04973-4) and PP (2016/17391-0) have been financially supported by Fundação de Amparo à Pesquisa do Estado de Sao Paulo. LO has been financially supported by Coordenação de Aperfeiçoamento de Pessoal de Nível Superior (CAPES).
References
1. Lancha Junior AH, Painelli VS, Saunders B, Artioli GG. Nutritional strategies to modulate intracellular and extracellular buffering capacity during high-intensity exercise. Sports Med. (2015) 45(Suppl. 1):S71–81. doi: 10.1007/s40279-015-0397-5
2. Carr AJ, Hopkins WG, Gore CJ. Effects of acute alkalosis and acidosis on performance: a meta-analysis. Sports Med. (2011) 41:801–14. doi: 10.2165/11591440-000000000-00000
3. Christensen PM, Shirai Y, Ritz C, Nordsborg NB. Caffeine and bicarbonate for speed. A meta-analysis of legal supplements potential for improving intense endurance exercise performance. Front Physiol. (2017) 8:240. doi: 10.3389/fphys.2017.00240
4. Hecksteden A, Kraushaar J, Scharhag-Rosenberger F, Theisen D, Senn S, Meyer T. Individual response to exercise training - a statistical perspective. J Appl Physiol. (1985) (2015) 118:1450–9. doi: 10.1152/japplphysiol.00714.2014
5. Hargreaves M, Spriet LL. Exercise metabolism: fuels for the fire. Cold Spring Harb Perspect Med. (2017) 2017:a029744. doi: 10.1101/cshperspect.a029744
6. Jubrias SA, Crowther GJ, Shankland EG, Gronka RK, Conley KE. Acidosis inhibits oxidative phosphorylation in contracting human skeletal muscle in vivo. J Physiol. (2003) 553(Pt 2):589–99. doi: 10.1113/jphysiol.2003.045872
7. Fabiato A, Fabiato F. Effects of pH on the myofilaments and the sarcoplasmic reticulum of skinned cells from cardiace and skeletal muscles. J Physiol. (1978) 276:233–55. doi: 10.1113/jphysiol.1978.sp012231
8. Sahlin K, Harris RC, Hultman E. Creatine kinase equilibrium and lactate content compared with muscle pH in tissue samples obtained after isometric exercise. Biochem J. (1975) 152:173–80. doi: 10.1042/bj1520173
9. Fitts RH. The role of acidosis in fatigue: pro perspective. Med Sci Sports Exerc. (2016) 48:2335–8. doi: 10.1249/MSS.0000000000001043
10. Westerblad H. Acidosis is not a significant cause of skeletal muscle fatigue. Med Sci Sports Exerc. (2016) 48:2339–42. doi: 10.1249/MSS.0000000000001044
11. Juel C. Lactate-proton cotransport in skeletal muscle. Physiol Rev. (1997) 77:321–58. doi: 10.1152/physrev.1997.77.2.321
12. Juel C, Klarskov C, Nielsen JJ, Krustrup P, Mohr M, Bangsbo J. Effect of high-intensity intermittent training on lactate and H+ release from human skeletal muscle. Am J Physiol Endocrinol Metab. (2004). 286:E245–51. doi: 10.1152/ajpendo.00303.2003
13. McNaughton LR, Siegler J, Midgley A. Ergogenic effects of sodium bicarbonate. Curr Sports Med Rep. (2008) 7:230–6. doi: 10.1249/JSR.0b013e31817ef530
14. McNaughton LR, Gough L, Deb S, Bentley D, Sparks SA. Recent developments in the use of sodium bicarbonate as an ergogenic aid. Curr Sports Med Rep. (2016) 15:233–44. doi: 10.1249/JSR.0000000000000283
15. Matson LG, Tran ZV. Effects of sodium bicarbonate ingestion on anaerobic performance: a meta-analytic review. Int J Sport Nutr. (1993) 3:2–28. doi: 10.1123/ijsn.3.1.2
16. Peart DJ, Siegler JC, Vince RV. Practical recommendations for coaches and athletes: a meta-analysis of sodium bicarbonate use for athletic performance. J Strength Cond Res. (2012) 26:1975–83. doi: 10.1519/JSC.0b013e3182576f3d
17. Carr AJ, Slater GJ, Gore CJ, Dawson B, Burke LM. Effect of sodium bicarbonate on [], pH, and gastrointestinal symptoms. Int J Sport Nutr Exerc Metab. (2011) 21:189–94. doi: 10.1123/ijsnem.21.3.189
18. Saunders B, Sale C, Harris RC, Sunderland C. Sodium bicarbonate and high-intensity-cycling capacity: variability in responses. Int J Sports Physiol Perform. (2014) 9:627–32. doi: 10.1123/ijspp.2013-0295
19. Jones RL, Stellingwerff T, Artioli GG, Saunders B, Cooper S, Sale, C. Dose-response of sodium bicarbonate ingestion highlights individuality in time course of blood analyte responses. Int J Sport Nutr Exerc Metab. (2016) 26:445–53. doi: 10.1123/ijsnem.2015-0286
20. McNaughton LR. Bicarbonate ingestion: effects of dosage on 60 s cycle ergometry. J. Sports Sci. (1992) 10:415–23. doi: 10.1080/02640419208729940
21. Costill DL, Verstappen F, Kuipers H, Janssen E, Fink W. Acid-base balance during repeated bouts of exercise: influence of HCO3. Int J Sports Med. (1984) 5:228–31. doi: 10.1055/s-2008-1025910
22. Katz A, Costill DL, King DS, Hargreaves M, Fink WJ. Maximal exercise tolerance after induced alkalosis. Int J Sports Med. (1984) 5:107–10. doi: 10.1055/s-2008-1025890
23. Horswill CA, Costill DL, Fink WJ, Flynn MG, Kirwan JP, Mitchell JB, et al. Influence of sodium-bicarbonate on sprint performance - relationship to dosage. Med Sci Sports Exerc. (1988) 20:566–9. doi: 10.1249/00005768-198812000-00008
24. Robergs R, Hutchinson K, Hendee S, Madden S, Siegler J. Influence of pre-exercise acidosis and alkalosis on the kinetics of acid-base recovery following intense exercise. Int J Sport Nutr Exerc Metab. (2005) 15:59–74. doi: 10.1123/ijsnem.15.1.59
25. Siegler JC, Marshall PW, Bray J, Towlson C. Sodium bicarbonate supplementation and ingestion timing: does it matter? J Strength Cond Res. (2012) 26:1953–8. doi: 10.1519/JSC.0b013e3182392960
26. Northgraves MJ, Peart DJ, Jordan CA, Vince RV. Effect of lactate supplementation and sodium bicarbonate on 40-km cycling time trial performance. J Strength Cond Res. (2014) 28:273–80. doi: 10.1519/JSC.0b013e3182986a4c
27. Froio de Araujo Dias G, da Eira Silva V, Painelli VS, Sale C, Giannini Artioli G, Gualano B, et al. (In)consistencies in responses to sodium bicarbonate supplementation: a randomised, repeated measures, counterbalanced and double-blind study. PLoS ONE (2015) 10:e0143086. doi: 10.1371/journal.pone.0143086
28. Price M, Moss P, Rance S. Effects of sodium bicarbonate ingestion on prolonged intermittent exercise. Med Sci Sports Exerc. (2003) 35:1303–8. doi: 10.1249/01.MSS.0000079067.46555.3C
29. Bishop D, Edge J, Davis C, Goodman C. Induced metabolic alkalosis affects muscle metabolism and repeated-sprint ability. Med Sci Sports Exerc. (2004) 36:807–13. doi: 10.1249/01.MSS.0000126392.20025.17
30. Bishop D, Claudius B. Effects of induced metabolic alkalosis on prolonged intermittent-sprint performance. Med Sci Sports Exerc. (2005) 37:759–67. doi: 10.1249/01.MSS.0000161803.44656.3C
31. Artioli GG, Gualano B, Coelho DF, Benatti FB, Galley AW, Lancha AH. Does sodium-bicarbonate ingestion improve simulated judo performance? Int J Sport Nutr Exerc Metab. (2007) 17:206–17. doi: 10.1123/ijsnem.17.2.206
32. Driller MW, Gregory JR, Williams AD, Fell JW. The effects of serial and acute nahco(3) loading in well-trained cyclists. J Strength Condition Res. (2012) 26:2791–7. doi: 10.1519/JSC.0b013e318241e18a
33. Carr BM, Webster MJ, Boyd JC, Hudson GM, Scheett TP. Sodium bicarbonate supplementation improves hypertrophy-type resistance exercise performance. Eur J Appl Physiol. (2013) 113:743–52. doi: 10.1007/s00421-012-2484-8
34. Higgins MF, James RS, Price MJ. The effects of sodium bicarbonate (NaHCO3) ingestion on high intensity cycling capacity. J Sports Sci. (2013) 31:972–81. doi: 10.1080/02640414.2012.758868
35. Mueller SM, Gehrig SM, Frese S, Wagner CA, Boutellier U, Toigo M. Multiday acute sodium bicarbonate intake improves endurance capacity and reduces acidosis in men. J Int Soc Sports Nutr. (2013) 10:16. doi: 10.1186/1550-2783-10-16
36. Egger F, Meyer T, Such U, Hecksteden A. Effects of sodium bicarbonate on high-intensity endurance performance in cyclists: a double-blind, randomized cross-over trial. PLoS ONE (2014) 9:e114729. doi: 10.1371/journal.pone.0114729
37. Hobson, RM, Harris RC, Martin D, Smith P, Macklin B, Elliott-Sale KJ, et al. Effect of sodium bicarbonate supplementation on 2000-m rowing performance. Int J Sports Physiol Perform. (2014) 9:139–44. doi: 10.1123/ijspp.2013-0086
38. Krustrup P, Ermidis G, Mohr, M. Sodium bicarbonate intake improves high-intensity intermittent exercise performance in trained young men. J. Int. Soc. Sports Nutr. (2015) 12:25. doi: 10.1186/s12970-015-0087-6
39. Gaitanos GC, Nevill ME, Brooks S, Williams C. Repeated bouts of sprint running after induced alkalosis. J Sports Sci. (1991) 9:355–70. doi: 10.1080/02640419108729896
40. Price MJ, Simons C. The effect of sodium bicarbonate ingestion on high-intensity intermittent running and subsequent performance. J Strength Cond Res. (2010) 24:1834–42. doi: 10.1519/JSC.0b013e3181e06e4a
41. Carr AJ, Slater GJ, Gore CJ, Dawson B, Burke LM. Reliability and effect of sodium bicarbonate: buffering and 2000-m rowing performance. Int J Sports Physiol Perform. (2012) 7:152–60. doi: 10.1123/ijspp.7.2.152
42. Kupcis PD, Slater GJ, Pruscino CL, Kemp JG. Influence of sodium bicarbonate on performance and hydration in lightweight rowing. Int J Sports Physiol Perform. (2012) 7:11–8. doi: 10.1123/ijspp.7.1.11
43. Saunders B, Sale C, Harris RC, Sunderland C. Effect of sodium bicarbonate and Beta-alanine on repeated sprints during intermittent exercise performed in hypoxia. Int J Sport Nutr Exerc Metab. (2014) 24:196–205. doi: 10.1123/ijsnem.2013-0102
44. Danaher J, Gerber T, Wellard RM, Stathis CG. The effect of beta-alanine and NaHCO3 co-ingestion on buffering capacity and exercise performance with high-intensity exercise in healthy males. Eur J Appl Physiol. (2014) 114:1715–24. doi: 10.1007/s00421-014-2895-9
45. Siegler JC, Marshall PW, Raftry S, Brooks C, Dowswell B, Romero R, et al. The differential effect of metabolic alkalosis on maximum force and rate of force development during repeated, high-intensity cycling. J Appl Physiol. (1985) (2013) 115:1634–40. doi: 10.1152/japplphysiol.00688.2013
46. Siegler JC, Gleadall-Siddall DO. Sodium bicarbonate ingestion and repeated swim sprint performance. J Strength Cond Res. (2010) 24:3105–11. doi: 10.1519/JSC.0b013e3181f55eb1
47. Webster MJ, Webster MN, Crawford RE, Gladden LB. Effect of sodium bicarbonate ingestion on exhaustive resistance exercise performance. Med Sci Sports Exerc. (1993) 25:960–5. doi: 10.1249/00005768-199308000-00012
48. Gough LA, Deb SK, Sparks SA, McNaughton LR. Sodium bicarbonate improves 4 km time trial cycling performance when individualised to time to peak blood bicarbonate in trained male cyclists. J Sports Sci. (2017) 36:1705–12. doi: 10.1080/02640414.2017.1410875
49. Duncan MJ, Weldon A, Price MJ. The effect of sodium bicarbonate ingestion on back squat and bench press exercise to failure. J Strength Cond Res. (2014) 28:1358–66. doi: 10.1519/JSC.0000000000000277
50. Siegler JC, Keatley SMidgley AW, Nevill AM, McNaughton LR. Pre-exercise alkalosis and acid-base recovery. Int J Sports Med. (2008) 29:545–51. doi: 10.1055/s-2007-989261
51. Higgins MF, Wilson S, Hill C, Price MJ, Duncan M, Tallis J. Evaluating the effects of caffeine and sodium bicarbonate, ingested individually or in combination, and a taste-matched placebo on high-intensity cycling capacity in healthy males. Appl Physiol Nutr Metab. (2016) 41:354–61. doi: 10.1139/apnm-2015-0371
52. McNaughton L, Curtin R, Goodman G, Perry D, Turner B, Showell C. Anaerobic work and power output during cycle ergometer exercise: effects of bicarbonate loading. J Sports Sci. (1991) 9:151–60. doi: 10.1080/02640419108729876
53. Siegler JC, Mudie K, Marshall P. The influence of sodium bicarbonate on maximal force and rates of force development in the triceps surae and brachii during fatiguing exercise. Exp Physiol. (2016) 101:1383–91. doi: 10.1113/EP085933
54. McNaughton LR. Sodium citrate and anaerobic performance: implications of dosage. Eur J Appl Physiol Occup Physiol. (1990) 61:392–7. doi: 10.1007/BF00236058
55. Flueck JL, Mettler S, Perret C. Influence of caffeine and sodium citrate ingestion on 1,500-m exercise performance in elite wheelchair athletes: a pilot study. Int J Sport Nutr Exerc Metab. (2014) 24:296–304. doi: 10.1123/ijsnem.2013-0127
56. Aedma M, Timpmann S, Oopik V. Dietary sodium citrate supplementation does not improve upper-body anaerobic performance in trained wrestlers in simulated competition-day conditions. Eur J Appl Physiol. (2015) 115:387–96. doi: 10.1007/s00421-014-3025-4
57. Vaher I, Timpmann S, Aedma M, Oopik V. Impact of acute sodium citrate ingestion on endurance running performance in a warm environment. Eur J Appl Physiol. (2015) 115:813–23. doi: 10.1007/s00421-014-3068-6
58. Schabort EJ, Wilson G, Noakes TD. Dose-related elevations in venous pH with citrate ingestion do not alter 40-km cycling time-trial performance. Eur J Appl Physiol. (2000) 83:320–7. doi: 10.1007/s004210000264
59. Ball D, Maughan RJ. The effect of sodium citrate ingestion on the metabolic response to intense exercise following diet manipulation in man. Exp Physiol. (1997) 82:1041–56. doi: 10.1113/expphysiol.1997.sp004079
60. Kowalchuk JM, Maltais SA, Yamaji K, Hughson, RL The effect of citrate loading on exercise performance, acid-base balance and metabolism. Eur J Appl Physiol Occup Physiol. (1989) 58:858–64. doi: 10.1007/BF02332219
61. van Someren K, Fulcher K, McCarthy J, Moore J, Horgan G, Langford R. An investigation into the effects of sodium citrate ingestion on high-intensity exercise performance. Int J Sport Nutr (1998) 8:356–63. doi: 10.1123/ijsn.8.4.356
62. Linossier MT, Dormois D, Bregere P, Geyssant A, Denis, C. Effect of sodium citrate on performance and metabolism of human skeletal muscle during supramaximal cycling exercise. Eur J Appl Physiol Occup Physiol. (1997) 76:48–54. doi: 10.1007/s004210050211
63. McNaughton L, Cedaro R. Sodium citrate ingestion and its effects on maximal anaerobic exercise of different durations. Eur J Appl Physiol Occup Physiol. (1992) 64:36–41. doi: 10.1007/BF00376437
64. Cox G, Jenkins DG. The physiological and ventilatory responses to repeated 60 s sprints following sodium citrate ingestion. J Sports Sci. (1994) 12:469–75. doi: 10.1080/02640419408732197
65. Hausswirth C, Bigard AX, Lepers R, Berthelot M, Guezennec CY. Sodium citrate ingestion and muscle performance in acute hypobaric hypoxia. Eur J Appl Physiol Occup Physiol. (1995) 71:362–8. doi: 10.1007/BF00240418
66. Russell C, Papadopoulos E, Mezil Y, Wells GD, Plyley MJ, Greenway M, et al. (2014). Acute versus chronic supplementation of sodium citrate on 200 m performance in adolescent swimmers. J Int Soc Sports Nutr. 11:26. doi: 10.1186/1550-2783-11-26
67. Fahey TD, Larsen JD, Brooks GA, Colvin W, Henderson S, Lary D. The effects of ingesting polylactate or glucose polymer drinks during prolonged exercise. Int J Sport Nutr. (1991) 1:249–56. doi: 10.1123/ijsn.1.3.249
68. Morris DM, Shafer RS, Fairbrother KR, Woodall MW. Effects of lactate consumption on blood bicarbonate levels and performance during high-intensity exercise. Int J Sport Nutr Exerc Metab. (2011) 21:311–7. doi: 10.1123/ijsnem.21.4.311
69. Oliveira LF, Painelli VS, Nemezio K, Goncalves LS, Yamaguchi G, Saunders B, et al. Chronic lactate supplementation does not improve blood buffering capacity and repeated high-intensity exercise. Scand J Med Sci Sports (2016) 27:1231–9. doi: 10.1111/sms.12792
70. Morris D, Beloni R, Wofford H. Metabolic and exercise performance responses to two different oral doses of calcium lactate. Med Sci Sports Exerc (2016) 48:250. doi: 10.1249/01.mss.0000485752.44580.79
71. Van Montfoort MC, Van Dieren L, Hopkins WG, Shearman JP. Effects of ingestion of bicarbonate, citrate, lactate, and chloride on sprint running. Med Sci Sports Exerc. (2004) 36:1239–43. doi: 10.1249/01.MSS.0000132378.73975.25
72. Painelli VS, da Silva RP, de Oliveira OM Jr, de Oliveira LF, Benatti FB, Rabelo T, et al. The effects of two different doses of calcium lactate on blood pH, bicarbonate, and repeated high-intensity exercise performance. Int J Sport Nutr Exerc Metab. (2014) 24:286–95. doi: 10.1123/ijsnem.2013-0191
73. Gough, LA, Deb, SK, Sparks, AS, and McNaughton LR. The reproducibility of blood acid base responses in male collegiate athletes following individualised doses of sodium bicarbonate: a randomised controlled crossover study. Sports Med. (2017) 47:2117–27. doi: 10.1007/s40279-017-0699-x
74. Sparks, A, Williams, E, Robinson, A, Miller, P, Bentley, DJ, Bridge, C, et al. Sodium bicarbonate ingestion and individual variability in time-to-peak pH. Res Sports Med. (2017) 25:58–66. doi: 10.1080/15438627.2016.1258645
75. Urwin CS, Dwyer DB, Carr AJ. Induced alkalosis and gastrointestinal symptoms after sodium citrate ingestion: a dose-response investigation. Int J Sport Nutr Exerc Metab. (2016) 26:542–8. doi: 10.1123/ijsnem.2015-0336
76. Miller P, Robinson AL, Sparks SA, Bridge CA, Bentley DJ, McNaughton, LR. The effects of novel ingestion of sodium bicarbonate on repeated sprint ability. J Strength Cond Res. (2016) 30:561–8. doi: 10.1519/JSC.0000000000001126
77. Painelli VS, Lancha Junior AH. Thirty years of investigation on the ergogenic effects of sodium citrate: is it time for a fresh start? Br J Sports Med. (2016) 2016:bjsports-2016-096516. doi: 10.1136/bjsports-2016-096516
78. Callahan MJ, Parr EB, Hawley JA, Burke LM. Single and combined effects of beetroot crystals and sodium bicarbonate on 4-km cycling time trial performance. Int J Sport Nutr Exerc Metab. (2017) 27:271–8. doi: 10.1123/ijsnem.2016-0228
79. Correia-Oliveira CR, Lopes-Silva JP, Bertuzzi R, McConell, GK, Bishop DJ, Lima-Silva AE, et al. Acidosis, but not alkalosis, affects anaerobic metabolism and performance in a 4-km time trial. Med Sci Sports Exerc. (2017) 49:1899–1910. doi: 10.1249/MSS.0000000000001295
80. Gough LA, Deb SK, Sparks A, McNaughton LR. The reproducibility of 4-km time trial (TT) performance following individualised sodium bicarbonate supplementation: a randomised controlled trial in trained cyclists. Sports Med. Open (2017) 3:34. doi: 10.1186/s40798-017-0101-4
81. Bogdanis GC, Nevill ME, Lakomy HK, Boobis LH. Power output and muscle metabolism during and following recovery from 10 and 20 s of maximal sprint exercise in humans. Acta Physiol Scand. (1998) 163:261–72. doi: 10.1046/j.1365-201x.1998.00378.x
82. Saunders B, Elliott-Sale K, Artioli GG, Swinton PA, Dolan E, Roschel H, et al. β-alanine supplementation to improve exercise capacity and performance: a systematic review and meta-analysis. Br J Sports Med. (2017) 51:658–69. doi: 10.1136/bjsports-2016-096396
83. McNaughton LR. Sodium bicarbonate ingestion and its effects on anaerobic exercise of various durations. J Sports Sci. (1992) 10:425–35. doi: 10.1080/02640419208729941
84. McNaughton L, Dalton B, Palmer G. Sodium bicarbonate can be used as an ergogenic aid in high-intensity, competitive cycle ergometry of 1 h duration. Eur J Appl Physiol Occup Physiol (1999) 80:64–9. doi: 10.1007/s004210050559
85. Stephens TJ, McKenna MJ, Canny BJ, Snow RJ, McConell GK. Effect of sodium bicarbonate on muscle metabolism during intense endurance cycling. Med Sci Sports Exerc. (2002) 34:614–21. doi: 10.1097/00005768-200204000-00009
86. Freis T, Hecksteden A, Such U, Meyer T. Effect of sodium bicarbonate on prolonged running performance: a randomized, double-blind, cross-over study. PLoS ONE (2017) 12:e0182158. doi: 10.1371/journal.pone.0182158
87. Hermansen L, Osnes JB. Blood and muscle pH after maximal exercise in man. J Appl Physiol. (1972) 32:304–8. doi: 10.1152/jappl.1972.32.3.304
88. Belfry GR, Raymer GH, Marsh GD, Paterson DH, Thompson RT, Thomas SG. Muscle metabolic status and acid-base balance during 10-s work:5-s recovery intermittent and continuous exercise. J Appl Physiol. (1985) (2012) 113:410–7. doi: 10.1152/japplphysiol.01059.2011
89. Lavender G, Bird SR. Effect of sodium bicarbonate ingestion upon repeated sprints. Br J Sports Med. (1989) 23:41–5. doi: 10.1136/bjsm.23.1.41
90. Juel C. Muscle pH regulation: role of training. Acta Physiol Scand. (1998) 162:359–66. doi: 10.1046/j.1365-201X.1998.0305f.x
91. Bangsbo J, Juel C, Hellsten Y, Saltin B. Dissociation between lactate and proton exchange in muscle during intense exercise in man. J Physiol Lond. (1997) 504:489–99. doi: 10.1111/j.1469-7793.1997.489be.x
92. Juel C. Regulation of cellular pH in skeletal muscle fiber types, studied with sarcolemmal giant vesicles obtained from rat muscles. Biochim Biophys Acta (1995) 1265:127–32. doi: 10.1016/0167-4889(94)00209-W
93. Mainwood GW, Worsley-Brown P. The effects of extracellular pH and buffer concentration on the efflux of lactate from frog sartorius muscle. J Physiol. (1975) 250:1–22. doi: 10.1113/jphysiol.1975.sp011040
94. Pilegaard H, Juel C, Wibrand F. Lactate transport studied in sarcolemmal giant vesicles from rats - effect of training. Am J Physiol. (1993) 264:E156–60. doi: 10.1152/ajpendo.1993.264.2.E156
95. McGinley C, Bishop DJ. Influence of training intensity on adaptations in acid/base transport proteins, muscle buffer capacity, and repeated-sprint ability in active men. J Appl Physiol. (1985) (2016) 121:1290–305. doi: 10.1152/japplphysiol.00630.2016
96. McGinley C, Bishop DJ. Rest interval duration does not influence adaptations in acid/base transport proteins following 10 wk of sprint-interval training in active women. Am J Physiol Regul Integr Comp Physiol. (2017) 312:R702–17. doi: 10.1152/ajpregu.00459.2016
97. Pilegaard H, Bangsbo J, Richter EA, Juel C. Lactate transport studied in sarcolemmal giant vesicles from human muscle biopsies - relation to training status. J. Appl. Physiol. (1994) 77:1858–62. doi: 10.1152/jappl.1994.77.4.1858
98. Pilegaard H, Terzis G, Halestrap A, Juel C. Distribution of the lactate/H+ transporter isoforms MCT1 and MCT4 in human skeletal muscle. Am J Physiol. (1999) 276(5 Pt 1):E843–48. doi: 10.1152/ajpendo.1999.276.5.E843
99. Maciejewski H, Bourdin M, Feasson L, Dubouchaud H, Denis C, Freund H, et al. Muscle MCT4 content is correlated with the lactate removal ability during recovery following all-out supramaximal exercise in highly-trained rowers. Front Physiol. (2016) 7:223. doi: 10.3389/fphys.2016.00223
100. Ben-Zaken S, Eliakim A, Nemet D, Rabinovich M, Kassem E, Meckel Y. Differences in MCT1 A1470T polymorphism prevalence between runners and swimmers. Scand J Med Sci Sports (2015) 25:365–71. doi: 10.1111/sms.12226
101. Cupeiro R, Benito PJ, Maffulli N, Calderon FJ, Gonzalez-Lamuno, D. (2010). MCT1 genetic polymorphism influence in high intensity circuit training: a pilot study. J Sci Med Sport 13:526–30. doi: 10.1016/j.jsams.2009.07.004
102. Cupeiro R, Gonzalez-Lamuno D, Amigo T, Peinado AB, Ruiz JR, Ortega FB, et al. Influence of the MCT1-T1470A polymorphism (rs1049434) on blood lactate accumulation during different circuit weight trainings in men and women. J Sci Med Sport (2012) 15:541–7. doi: 10.1016/j.jsams.2012.03.009
103. Pasqua LA, Damasceno MV, Bueno S, Araújo GG, Lima-Silva AE, Bertuzzi, R. Association between ventilatory thresholds related to aerobic fitness and MCT1 A1470T polymorphism. Sports Nutr. Ther. (2016) 1:1–5. doi: 10.4172/2473-6449.1000105
104. Sawczuk M, Banting LK, Cieszczyk P, Maciejewska-Karlowska A, Zarebska A, Leonska-Duniec A, et al. MCT1 A1470T: a novel polymorphism for sprint performance? J Sci Med Sport (2015) 18:114–8. doi: 10.1016/j.jsams.2013.12.008
105. Lean CB, Lee EJ. Genetic variations of the MCT4 (SLC16A3) gene in the Chinese and Indian populations of Singapore. Drug Metab Pharmacokinet. (2012) 27:456–64. doi: 10.2133/dmpk.DMPK-11-SH-104
106. Knapik JJ, Steelman RA, Hoedebecke SS, Austin KG, Farina EK, Lieberman HR. Prevalence of dietary supplement use by athletes: systematic review and meta-analysis. Sports Med. (2016) 46:103–23. doi: 10.1007/s40279-015-0387-7
Keywords: buffering agents, alkalosis, bicarbonate, citrate, lactate
Citation: Heibel AB, Perim PHL, Oliveira LF, McNaughton LR and Saunders B (2018) Time to Optimize Supplementation: Modifying Factors Influencing the Individual Responses to Extracellular Buffering Agents. Front. Nutr. 5:35. doi: 10.3389/fnut.2018.00035
Received: 09 February 2018; Accepted: 19 April 2018;
Published: 08 May 2018.
Edited by:
Stavros A. Kavouras, University of Arkansas, United StatesCopyright © 2018 Heibel, Perim, Oliveira, McNaughton and Saunders. This is an open-access article distributed under the terms of the Creative Commons Attribution License (CC BY). The use, distribution or reproduction in other forums is permitted, provided the original author(s) and the copyright owner are credited and that the original publication in this journal is cited, in accordance with accepted academic practice. No use, distribution or reproduction is permitted which does not comply with these terms.
*Correspondence: Bryan Saunders, ZHJicnlhbnNhdW5kZXJzQG91dGxvb2suY29t