- INSERM U_1116, Université de Lorraine, Vandoeuvre les Nancy, France
This mini-review develops the hypothesis that increased hydration leads to body weight loss, mainly through a decrease in feeding, and a loss of fat, through increased lipolysis. The publications cited come from animal, mainly rodent, studies where manipulations of the central and/or the peripheral renin–angiotensin system lead to an increased drinking response and a decrease in body weight. This hypothesis derives from a broader association between chronic hypohydration (extracellular dehydration) and raised levels of the hormone angiotensin II (AngII) associated with many chronic diseases, such as obesity, diabetes, cancer, and cardiovascular disease. Proposed mechanisms to explain these effects involve an increase in metabolism due to hydration expanding cell volume. The results of these animal studies often can be applied to the humans. Human studies are consistent with this hypothesis for weight loss and for reducing the risk factors in the development of obesity and type 2 diabetes.
Introduction
Increased water intake is associated with loss of body weight produced via two mechanisms, decreased feeding and increased lipolysis. The obverse also appears to be true. Mild, but chronic, hypohydration is correlated with increased body weight and its attendant dysfunctions (1). The common denominator likely is angiotensin II (AngII), the principal hormone of body fluid regulation. In what follows, this hypothesis will be tested against the available evidence (2).
AngII acts on two, seven transmembrane domain peptide receptors, AT1 and AT2. Working through the AT1 receptor AngII stimulates thirst (the act of seeking out and drinking fluids, mainly water), an appetite for sodium, the release of anti-diuretic hormone (ADH or vasopressin) to conserve water via the kidneys, and vasoconstriction (conserving perfusion pressure to all organs and cells). The principal physiological signal for an increase in plasma AngII is extracellular dehydration (hypovolemia) (3). The responses listed above enable the rapid return of plasma volume to normal levels, thus reducing the signal for AngII generation. This is the physiological response to hypovolemia displayed by rodents.
However, chronically elevated AngII appears to be involved in several chronic human diseases (2). Antagonists of the renin–angiotensin system (RAS) are prescribed in 85% of cases to treat cardiovascular disease (4, 5). The same antagonists are used to treat obesity (6), diabetes (7, 8), cancer (9), and Alzheimer’s disease (10). These effects could result if a subsection of the population was chronically, but mildly, hypohydrated [e.g., Ref. (11)], i.e., chronically, but mildly, hypovolemic.
These chronic diseases also involve metabolic dysfunctions (12, 13). This has been observed for cardiovascular disease (14, 15), obesity (16), diabetes (17–19), cancer (20), and Alzheimer’s disease (21). In other words, chronic hypohydration may be driving the continuous release of AngII and the metabolic dysfunction found in the chronic human diseases.
Given that in animals AngII stimulates appropriate drinking responses, why is that some humans appear not to respond appropriately to the same AngII signal? The influence of other, perhaps cognitive, factors on appropriate drinking responses has been noted in kidney stone formation, where increased water intake is recommended as a preventative measure, but compliance is difficult (22, 23). The authors noted that “not knowing the benefits of water drinking,” “not liking the taste,” and “the need to urinate frequently” influenced patient’s behavior.
Methods
This mini-review concentrates on angiotensin and metabolic function by looking at the effect of central and peripheral manipulations of the RAS that increase drinking, reduce food intake, decrease body weight, and produce fat loss through increased lipolysis. Literature searches used keywords: angiotensin, drinking, water intake, body weight loss, obesity, diabetes, RAS antagonists, metabolism, hydration, atrial peptides, UCP1, insulin resistance, and mitochondria. Research and clinical articles are cited where there is an associated increase in water intake, a decrease in body weight, a decrease in body fat, and/or a decrease in the markers of the risk of developing obesity and type 2 diabetes. There is a large literature on the RAS and body weight regulation as well as metabolism but not all articles measured water intake and thus are not cited.
Central AngII, Drinking, and Weight Loss
Administration of AngII into the brain of behaving animals increases drinking. Rats can consume over 2 h up to 15 ml of water in response after a single injection of AngII, depending on the dose and the site of injection (24–30). A decrease in feeding following drinking stimulated by intracranial AngII was noted early on, but this appeared to fade as the drinking response waned (31). Furthermore, in rats, chronically administered AngII over several days or weeks increased drinking (at least a doubling in daily intake), which was associated with a small decrease in food intake and a decrease in body weight, mainly through loss of fat (32–35). The decrease in body weight following the AngII infusion was greater than that in pair-fed rats.
Several mechanisms not necessarily related to the increased drinking have been suggested for this, AngII produces an increase in uncoupling protein I (33, 35). Others have suggested an increased thermic effect of food, an increased feeding hormone effect, or even an increased in stress hormone release (35). Both mechanisms imply a change in metabolic activity.
RAS Antagonists Drinking and Weight Loss
In other rodent models of obesity, using either angiotensin-converting enzyme (ACE) inhibitors or AT1-specific antagonists increased drinking significantly with an associated decrease in food intake and body weight mainly through loss of fat. In some cases, the fat loss was specifically linked to increased lipolysis (36–41). The drinking responses ranged from a 30% increase to up to a doubling of normal intake in both rats and mice. With two AT1-specific antagonists, candesartan and losartan, this effect is observed in obese, rather than lean, rats (42, 43). Use of the renin inhibitor aliskiren in mice on both low-fat and high-fat diets demonstrated a significantly increased drinking response with a lower body weight gain and loss of body fat over a 43-day treatment period (44).
Increased drinking to RAS blockade may appear paradoxical, but it could be in response to blockade-induced increased urine flow (45, 46) or to peripheral blockade-induced increase in AI passing through the blood–brain barrier, converting to AngII in the brain, and activating hypothalamic AT1 receptors (47–49). It could also be in response to the hypovolemia produced by the RAS blockade, but no data were found to support this.
The same RAS inhibitors have been reported to be renoprotective, reduce obesity, and improve insulin sensitivity in rodents, but without recording water intakes (50–53). Similar results occurred in one human study (54), yet not in another (55), both without recorded fluid intakes. Hypohydration has been shown to lead to hyperglycemia (56), which is linked with the major problems of obesity and type 2 diabetes.
RAS “Knockout” Mice Drinking and Weight Loss
Similar “paradoxical” results are found when the renin gene is knocked out, mice drink copiously (2.4 ± 0.1 compared with 9.2 ± 0.7 ml/day), are hyperactive, thin, have low body fat, and do not develop obesity (57). A decrease in body weight and % fat with an increase in activity was observed in renin-deficient mice on a high-fat diet, but no water intakes were given (58). Similar results occur in mice lacking the AT1 receptor (59, 60); however, no decrease in body weight was observed, despite a nearly threefold increase in drinking in these AT1-receptor KO mice (61). Furthermore, angiotensinogen-deficient mice exhibit a decrease in body weight and % body fat with an increase in activity. Water intakes were not reported in this study (62), but have been noted by others (63, 64). Similarly, in ACE gene knockout mice, water intake was doubled (from 4.2 ± 0.2 to 9.8 ± 0.5 ml/day), food intake was slightly decreased, whereas body weight and body fat were significantly decreased (fat by 10%) compared with intact controls (65).
Further details on studies on the role of the RAS in food intake and metabolic parameters are in the excellent reviews by Mathai et al. (37) and by de Kloet et al. (66). In nearly all human and animal studies, pharmacological blockade of the RAS decreases body weight, food intake, and body fat. Unfortunately, most, if not all, studies did not report measurements of water intake. This argues for clinical studies on the effects of hydration on body weight regulation.
Mechanisms
What Physiological Link Exists between Increased Drinking and Lipolysis?
Work in humans with administration of hypoosmotic solutions showed that there was an increase in lipolysis (67–69). The studies also show an increase in lipolysis with increased drinking indicating and, by inference, an increase in metabolism. This produced the hypothesis that increased hydration leads to an increase in cell volume and from that to increased insulin sensitivity (70–72). Furthermore, the RAS has been linked also with mitochondrial dysfunction (73–75), and treatments with RAS antagonists improved mitochondrial function (76–80). Because the same treatment induces increased water intake, this suggests that an increased hydration may enhance mitochondrial function and thus metabolism. These mechanisms are illustrated in Figures 1 and 2.
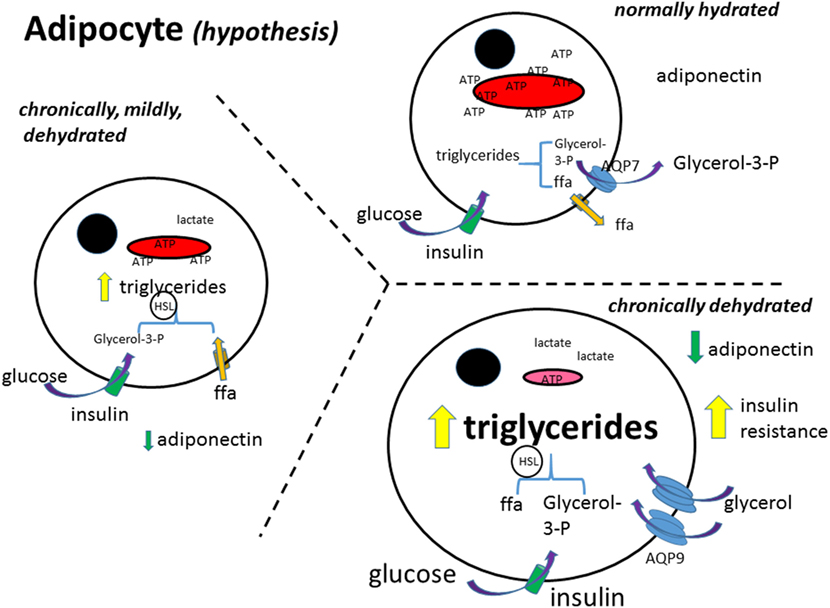
Figure 1. Adipocyte metabolism (hypothesis). In the normally hydrated (euhydrated) adipocyte, triglycerides are formed from glucose and free fatty acid uptake, and as well broken down (lipolysis); the rate depending on the needs of the cell for ATP. Glycerol in excess is exported out of the cell. Free fatty acids (ffa) are either metabolized or exported (81). As the adipocyte gets more and more dehydrated, formation of triglycerides increases and the ffa are not able to be transformed into pyruvate and thence metabolized in the mitochondria. The glycerol transporter, aquaporin 9 (AQP9), increases, bringing in more glycerol to make more triglycerides. Glucose uptake is further stimulated by insulin, increasing also triglyceride synthesis. Black circle, cell nucleus; red structure, mitochondria; ffa, free fatty acid; aa, amino acid; AQP7 + 9, aquaporin 7 and 9; HSL, hormone-sensitive lipase.
Some studies report an increase in activity with increased hydration, but the authors did not look at activity alone in the overall effects on body weight decrease.
Another plausible mechanism is that increased water intake drives thermogenesis (83–87) that would lead also to a decrease in weight gain.
Physiologically, increased water intake leads to an increase in blood volume with an attendant increase in right atrium pressure. This would release atrial natriuretic peptide (ANP), which was one of the first identified natriuretic peptides (88). This family of cardiac natriuretic peptides activates uncoupling protein 1 (UCP1) that increases fat metabolism and leads to a loss of body weight (89–95). A significant increase in UCP1 was observed in renin knockout mice fed a high-fat diet (58), and these animals drink copious amounts of water (57). Furthermore, receptors for atrial peptides have been demonstrated in brown adipose tissue (96, 97).
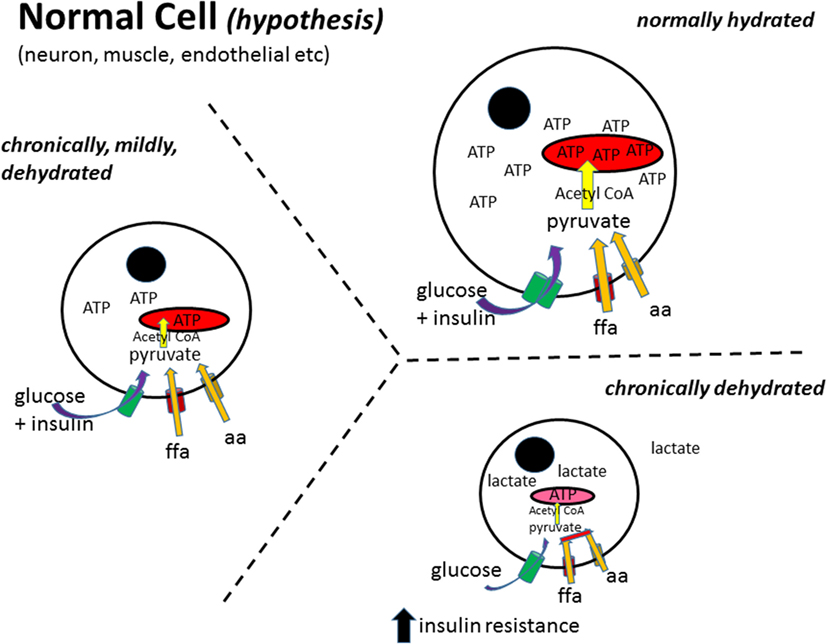
Figure 2. Normal cell metabolism (hypothesis). In a normally hydrated (euhydrated) cell, all substrates are taken up by their appropriate transporter mechanisms and enzymatically converted to pyruvate, transported into the mitochondria, converted to acetyl-CoA, which then enters the tricarboxylic acid cycle to generate ATP (82). As the cell gets more and more dehydrated, the metabolism of free fatty acids (ffa) and amino acids (aa) to pyruvate and/or acetyl-CoA decreases producing a dependence on glucose as the main fuel source [as has been reported for obesity (16)]. Furthermore, as the cell decreases in size, the ability of insulin to stimulate glucose uptake decreases, leading to insulin resistance. Black circle, cell nucleus; red structure, mitochondria; ffa, free fatty acid; aa, amino acid.
Physiologically, the presence of AngII is linked almost exclusively to extracellular dehydration (or extracellular thirst). The physiological stimuli for thirst are known (3) and can be broken down to intracellular and extracellular deficits. Intracellular dehydration involves an increase in plasma osmolality (normal levels between 295 and 300 mosmol/kg water), leading to activation of hypothalamic osmoreceptors that stimulate drinking and the release of ADH that in turn conserves hydration by increasing renal water reabsorption. This action should return plasma osmolality to normal levels, reduce the motivation to drink, and stop the release of ADH. Extracellular dehydration, or a decrease in blood (plasma) volume (hypovolemia), leads to renin release from the kidney, which acts enzymatically on angiotensinogen in the blood making angiotensin I (AngI). AngI is transformed by ACE into AngII. As mentioned in Section “Introduction,” AngII stimulates the seeking out and drinking of fluids (mainly water), an appetite for sodium, the release of ADH, and vasoconstriction. These actions should return plasma volume to normal levels while reducing blood AngII levels, the motivation to drink, to eat salt (mainly sodium), and the release of ADH. Most hypohydration leads to a mixture of intracellular and extracellular stimuli that should stimulate the behavioral acts of drinking and sodium intake, as well as the release of ADH, thus allowing correct regulation of body (and cellular) hydration.
Although thirst is an effective motivation in most animal studies, it may not be a sufficient or adequate stimulus for drinking in many humans, including the ill, the elderly, and infants (98). The increased blood levels of AngII indicate that part of the human population may be chronically, but mildly, hypohydrated. As suggested earlier, chronic hypohydration is driving continuous release of AngII and, by extension, the metabolic dysfunction found in cardiovascular disease, obesity, diabetes, cancer, and Alzheimer’s disease.
Rodent and Human Hydration
In its homozygous form, the Brattleboro rat figures prominently in studies of metabolism. This animal does not produce ADH and thus urinates copiously and consequently drinks considerably, up to 200 ml/day. These animals grow more slowly than their littermate controls with ADH for the same amount of food ingested (99–101). In the Brattleboro rat, this could be due to a significantly increased metabolism as observed in neurons when measuring fluorine 18-labeled fluorodeoxyglucose uptake with a PET scanner (102).
Human studies suggest a similar effect as an increase in water intake has been associated with a decrease in body weight in obese, overweight, and normal children, and adults (103–111). Furthermore, addition of 500 ml of water before eating breakfast or a hypocaloric meal reduces energy intake (112) or increases weight loss (113). In a recent random controlled trial, there was a significant weight loss between a group eating meals with a pre-meal water load compared with the controls without a pre-meal water load (114).
Diets, Drinking, and Weight Loss
To take this further, in rodents, a high-protein diet is associated with weight loss (115, 116) and with increased drinking (117, 118). This increased drinking may reflect the increased urine output (119, 120) needed to excrete the added urea resulting from the additional dietary protein metabolism (121). Nevertheless, based on the evidence reviewed above, the weight loss observed while on not in a high-protein diet also could be a direct result of the increased water intake. Furthermore, an increased protein diet is also associated with an increase in size and number of functionally normal liver cell mitochondria (122, 123). This would correlate with an increase in cell size following an increase in hydration as mentioned above. Finally, weight loss produced using a hypocaloric diet induces a significant (30%) increase in water intake in both young (4 months old) and old (9 months old) female mice (124).
Discussion and Conclusion
This brief review highlights the considerable evidence that an increase in water intake, i.e., increased hydration, leads to loss of body weight. In rodent studies, the effect is clear and consistent. At the least, this requires that measurement of water intake must be included in an experiment concerning rodents and all aspects of body weight regulation, from ingestive behavior to metabolic function. An increase in metabolism is one likely mechanism for the weight loss effect (125) because this can lead to increased mitochondrial function. In adipocytes, ramping up mitochondrial activity increases lipolysis. Human studies should also address the question of hydration with the increased use of RAS antagonists in the treatment of insulin resistance (126). Body weight regulation is a complex process, and increased water intake should be part of the measures required to reduce the overall risk factors.
As mentioned in Section “Introduction,” the effects of chronic mild hypohydration extend beyond fostering obesity. Extracellular dehydration-induced AngII, and the attendant possible mitochondrial dysfunction, may contribute not only to obesity and diabetes but also to cardiovascular disease, cancer, and Alzheimer’s disease. Furthermore, there could be other “symptoms” linking these major health problems to hypohydration such as a decrease in brain volume that is also associated with Alzheimer’s disease, obesity, and diabetes and could be (127). A simple solution for reducing these modern chronic diseases would be to increase water intake across the general population. Given that hypohydration is a chronic circumstance, the effects of increased water intake would likely appear as younger groups age, as seen in schools to ameliorate childhood obesity (107, 110) and where dehydration is an issue at the start of the day (128, 129). Hypohydration occurs in France in that water intake is less than the National Nutrition Program recommendation of at least 1.5 l/day (130). The precise amounts of additional water needed and the relative importance of the different possible pathways and mechanisms remain to be specified. The implementation of such a policy would then require a public health initiative.
A limitation of this mini-review is that it concentrates mainly on papers dealing with the hypovolemia (or hypohydration)-related hormone AngII and the stimulated water intake that has effects on body weight, lipolysis, and food intake. There are a large number of studies in both animals and humans looking at the effects of RAS antagonist treatments for reducing the risk of cardiovascular disease, obesity, diabetes, cancer, and even Alzheimer’s disease where water intake, or even thirst responses, is not reported.
Author Contributions
The author confirms being the sole contributor of this work and approved it for publication. The author would like to thank Dr. R. Norgren for his very helpful editing suggestions following detailed discussions.
Conflict of Interest Statement
The author declares that the research was conducted in the absence of any commercial or financial relationships that could be construed as a potential conflict of interest.
References
1. Fetissov SO, Thornton SN. Hypovolaemia-induced obesity and diabetes. Metabolism (2009) 58:1678. doi:10.1016/j.metabol.2009.06.022
2. Thornton SN. Angiotensin, the hypovolaemia hormone, aggravates hypertension, obesity, diabetes and cancer. J Intern Med (2009) 265:616–7. doi:10.1111/j.1365-2796.2008.02037.x
3. Thornton SN. Thirst and hydration: physiology and consequences of dysfunction. Physiol Behav (2010) 100:15–21. doi:10.1016/j.physbeh.2010.02.026
4. Hargovan M, Ferro A. Aldosterone synthase inhibitors in hypertension: current status and future possibilities. JRSM Cardiovasc Dis (2014) 5:2048004014522440. doi:10.1177/2048004014522440
5. Taddei S. Combination therapy in hypertension: what are the best options according to clinical pharmacology principles and controlled clinical trial evidence? Am J Cardiovasc Drugs (2015) 15:185–94. doi:10.1007/s40256-015-0116-5
6. Lteif AA, Chisholm RL, Gilbert K, Considine RV, Mather KJ. Effects of losartan on whole body, skeletal muscle and vascular insulin responses in obesity/insulin resistance without hypertension. Diabetes Obes Metab (2012) 14:254–61. doi:10.1111/j.1463-1326.2011.01522.x
7. Nakamura A, Shikata K, Nakatou T, Kitamura T, Kajitani N, Ogawa D, et al. Combination therapy with an angiotensin-converting-enzyme inhibitor and an angiotensin II receptor antagonist ameliorates microinflammation and oxidative stress in patients with diabetic nephropathy. J Diabetes Investig (2013) 4:195–201. doi:10.1111/jdi.12004
8. van Hateren KJ, Landman GW, Groenier KH, Bilo HJ, Kleefstra N. Effectiveness of angiotensin II receptor antagonists in a cohort of Dutch patients with type 2 diabetes mellitus (ZODIAC-14). Can J Diabetes (2015) 39:157–61. doi:10.1016/j.jcjd.2014.10.012
9. Hosseinimehr SJ. The use of angiotensin II receptor antagonists to increase the efficacy of radiotherapy in cancer treatment. Future Oncol (2014) 10:2381–90. doi:10.2217/fon.14.177
10. O’Caoimh R, Kehoe PG, Molloy DW. Renin angiotensin aldosterone system inhibition in controlling dementia-related cognitive decline. J Alzheimers Dis (2014) 42:S575–86. doi:10.3233/JAD-141284
11. Ritz P; Investigators of the Source Study and of the Human Nutrition Research Centre-Auvergne. Chronic cellular dehydration in the aged patient. J Gerontol A Biol Sci Med Sci (2001) 56:M349–52. doi:10.1093/gerona/56.6.M349
12. de Cavanagh EM, Inserra F, Ferder M, Ferder L. From mitochondria to disease: role of the renin-angiotensin system. Am J Nephrol (2007) 27:545–53. doi:10.1159/000107757
13. Archer SL. Mitochondrial dynamics – mitochondrial fission and fusion in human diseases. N Engl J Med (2013) 369:2236–51. doi:10.1056/NEJMra1215233
14. Tang X, Luo YX, Chen HZ, Liu DP. Mitochondria, endothelial cell function, and vascular diseases. Front Physiol (2014) 5:175. doi:10.3389/fphys.2014.00175
15. Sharp WW, Archer SL. Mitochondrial dynamics in cardiovascular disease: fission and fusion foretell form and function. J Mol Med (Berl) (2015) 93:225–8. doi:10.1007/s00109-015-1258-2
16. Rogge MM. The role of impaired mitochondrial lipid oxidation in obesity. Biol Res Nurs (2009) 10:356–73. doi:10.1177/1099800408329408
17. Aroor AR, Mandavia CH, Sowers JR. Insulin resistance and heart failure: molecular mechanisms. Heart Fail Clin (2012) 8:609–17. doi:10.1016/j.hfc.2012.06.005
18. Blake R, Trounce IA. Mitochondrial dysfunction and complications associated with diabetes. Biochim Biophys Acta (2014) 1840:1404–12. doi:10.1016/j.bbagen.2013.11.007
19. Hwang MH, Kim S. Type 2 diabetes: endothelial dysfunction and exercise. J Exerc Nutr Biochem (2014) 18:239–47. doi:10.5717/jenb.2014.18.3.239
21. Picone P, Nuzzo D, Caruana L, Scafidi V, Di Carlo M. Mitochondrial dysfunction: different routes to Alzheimer’s disease therapy. Oxid Med Cell Longev (2014) 2014:780179. doi:10.1155/2014/780179
22. McCauley LR, Dyer AJ, Stern K, Hicks T, Nguyen MM. Factors influencing fluid intake behavior among kidney stone formers. J Urol (2012) 187:1282–6. doi:10.1016/j.juro.2011.11.111
23. Lotan Y, Buendia Jiménez I, Lenoir-Wijnkoop I, Daudon M, Molinier L, Tack I, et al. Increased water intake as a prevention strategy for recurrent urolithiasis: major impact of compliance on cost-effectiveness. J Urol (2013) 189:935–9. doi:10.1016/j.juro.2012.08.254
24. Epstein AN, Fitzsimons JT, Rolls BJ. Drinking induced by injection of angiotensin into the rain of the rat. J Physiol (1970) 210:457–74. doi:10.1113/jphysiol.1970.sp009220
25. Radio GJ, Summy-Long J, Daniels-Severs A, Severs WB. Hydration changes produced by central infusion of angiotensin II. Am J Physiol (1972) 223:1221–6.
26. Simpson JB, Routtenberg A. Subfornical organ: site of drinking elicitation by angiotensin II. Science (1973) 181:1172–5. doi:10.1126/science.181.4105.1172
27. Buggy J, Fisher AE. Evidence for a dual central role for angiotensin in water and sodium intake. Nature (1974) 250:733–5. doi:10.1038/250733a0
28. Elghozi JL, Fitzsimons JT, Meyer P, Nicolaïdis S. Central angiotensin in the control of water intake and blood pressure. Prog Brain Res (1977) 47:137–49. doi:10.1016/S0079-6123(08)62720-X
29. Sirett NE, Thornton SN, Hubbard JI. Brain angiotensin II binding and central [Sar1,Ala8]angiotensin responses in normal rats and the New Zealand strain of genetically hypertensive rats. Clin Sci (Lond) (1979) 56:607–11. doi:10.1042/cs0560607
30. Fitzsimons JT. Angiotensin and other peptides in the control of water and sodium intake. Proc R Soc Lond B Biol Sci (1980) 210:165–82. doi:10.1098/rspb.1980.0126
31. McFarland DJ, Rolls B. Suppression of feeding by intracranial injections of angiotensin. Nature (1972) 236:172–3. doi:10.1038/236172a0
32. Porter JP, Anderson JM, Robison RJ, Phillips AC. Effect of central angiotensin II on body weight gain in young rats. Brain Res (2003) 959:20–8. doi:10.1016/S0006-8993(02)03676-4
33. Porter JP, Potratz KR. Effect of intracerebroventricular angiotensin II on body weight and food intake in adult rats. Am J Physiol Regul Integr Comp Physiol (2004) 287:R422–8. doi:10.1152/ajpregu.00537.2003
34. Ortiz RM, Kobori H, Conte D, Navar LG. Angiotensin II-induced reduction in body mass is Ang II receptor mediated in association with elevated corticosterone. Growth Horm IGF Res (2010) 20:282–8. doi:10.1016/j.ghir.2010.03.003
35. de Kloet AD, Krause EG, Scott KA, Foster MT, Herman JP, Sakai RR, et al. Central angiotensin II has catabolic action at white and brown adipose tissue. Am J Physiol Endocrinol Metab (2011) 301:E1081–91. doi:10.1152/ajpendo.00307.2011
36. Zorad S, Dou JT, Benicky J, Hutanu D, Tybitanclova K, Zhou J, et al. Long-term angiotensin II AT1 receptor inhibition produces adipose tissue hypotrophy accompanied by increased expression of adiponectin and PPARgamma. Eur J Pharmacol (2006) 552:112–22. doi:10.1016/j.ejphar.2006.08.062
37. Mathai ML, Naik S, Sinclair AJ, Weisinger HS, Weisinger RS. Selective reduction in body fat mass and plasma leptin induced by angiotensin-converting enzyme inhibition in rats. Int J Obes (Lond) (2008) 32:1576–84. doi:10.1038/ijo.2008.126
38. Weisinger HS, Begg DP, Egan GF, Jayasooriya AP, Lie F, Mathai ML, et al. Angiotensin converting enzyme inhibition from birth reduces body weight and body fat in Sprague-Dawley rats. Physiol Behav (2008) 93:820–5. doi:10.1016/j.physbeh.2007.11.046
39. de Kloet AD, Krause EG, Kim DH, Sakai RR, Seeley RJ, Woods SC. The effect of angiotensin-converting enzyme inhibition using captopril on energy balance and glucose homeostasis. Endocrinology (2009) 150:4114–23. doi:10.1210/en.2009-0065
40. Weisinger RS, Stanley TK, Begg DP, Weisinger HS, Spark KJ, Jois M. Angiotensin converting enzyme inhibition lowers body weight and improves glucose tolerance in C57BL/6J mice maintained on a high fat diet. Physiol Behav (2009) 98:192–7. doi:10.1016/j.physbeh.2009.05.009
41. Aubert G, Burnier M, Dulloo A, Perregaux C, Mazzolai L, Pralong F, et al. Neuroendocrine characterization and anorexigenic effects of telmisartan in diet- and glitazone-induced weight gain. Metabolism (2010) 59:25–32. doi:10.1016/j.metabol.2009.07.002
42. Alonso-Galicia M, Brands MW, Zappe DH, Hall JE. Hypertension in obese Zucker rats. Role of angiotensin II and adrenergic activity. Hypertension (1996) 28:1047–54. doi:10.1161/01.HYP.28.6.1047
43. Ecelbarger CM, Rash A, Sinha RK, Tiwari S. The effect of chronic candesartan therapy on the metabolic profile and renal tissue cytokine levels in the obese Zucker rat. Mediators Inflamm (2010) 2010:841343. doi:10.1155/2010/841343
44. Stucchi P, Cano V, Ruiz-Gayo M, Fernández-Alfonso MS. Aliskiren reduces body-weight gain, adiposity and plasma leptin during diet-induced obesity. Br J Pharmacol (2009) 158:771–8. doi:10.1111/j.1476-5381.2009.00355.x
45. Tallam LS, Jandhyala BS. Significance of exaggerated natriuresis after angiotensin AT1 receptor blockade or angiotensin-converting enzyme inhibition in obese Zucker rats. Clin Exp Pharmacol Physiol (2001) 28:433–40. doi:10.1046/j.1440-1681.2001.3457.x
46. Sebeková K, Lill M, Boor P, Heidland A, Amann K. Functional and partial morphological regression of established renal injury in the obese Zucker rat by blockade of the renin-angiotensin system. Am J Nephrol (2009) 29:164–70. doi:10.1159/000151771
47. Lopez-Sela P, Brime JI, Diaz F, Marin B, Costales M, Vijande M. Effects of inhibitors of the renin-angiotensin system on water intake after insulin administration. Appetite (1989) 13:143–54. doi:10.1016/0195-6663(89)90111-6
48. Thunhorst RL, Fitts DA, Simpson JB. Angiotensin-converting enzyme in subfornical organ mediates captopril-induced drinking. Behav Neurosci (1989) 103:1302–10. doi:10.1037/0735-7044.103.6.1302
49. Robinson MM, McLennan GP, Thunhorst RL, Johnson AK. Interactions of the systemic and brain renin-angiotensin systems in the control of drinking and the central mediation of pressor responses. Brain Res (1999) 842:55–61. doi:10.1016/S0006-8993(99)01807-7
50. Araki K, Masaki T, Katsuragi I, Tanaka K, Kakuma T, Yoshimatsu H. Telmisartan prevents obesity and increases the expression of uncoupling protein 1 in diet-induced obese mice. Hypertension (2006) 48:51–7. doi:10.1161/01.HYP.0000225402.69580.1d
51. Henriksen EJ. Improvement of insulin sensitivity by antagonism of the renin-angiotensin system. Am J Physiol Regul Integr Comp Physiol (2007) 293:R974–80. doi:10.1152/ajpregu.00147.2007
52. Santos EL, de Picoli Souza K, Guimarães PB, Reis FC, Silva SM, Costa-Neto CM, et al. Effect of angiotensin converting enzyme inhibitor enalapril on body weight and composition in young rats. Int Immunopharmacol (2008) 8:247–53. doi:10.1016/j.intimp.2007.07.021
53. Ohmura T, Tsunenari I, Seidler R, Chachin M, Hayashi T, Konomi A, et al. Renoprotective effects of telmisartan on renal injury in obese Zucker rats. Acta Diabetol (2012) 49:S15–24. doi:10.1007/s00592-007-0016-5
54. Shimabukuro M, Tanaka H, Shimabukuro T. Effects of telmisartan on fat distribution in individuals with the metabolic syndrome. J Hypertens (2007) 25:841–8. doi:10.1097/HJH.0b013e3280287a83
55. Lind L, Pollare T, Berne C, Lithell H. Long-term metabolic effects of antihypertensive drugs. Am Heart J (1994) 128:1177–83. doi:10.1016/0002-8703(94)90749-8
56. Roussel R, Fezeu L, Bouby N, Balkau B, Lantieri O, Alhenc-Gelas F, et al. Low water intake and risk for new-onset hyperglycemia. Diabetes Care (2011) 34:2551–4. doi:10.2337/dc11-0652
57. Takahashi N, Lopez ML, Cowhig JE Jr, Taylor MA, Hatada T, Riggs E, et al. Ren1c homozygous null mice are hypotensive and polyuric, but heterozygotes are indistinguishable from wild-type. J Am Soc Nephrol (2005) 16:125–32. doi:10.1681/ASN.2004060490
58. Takahashi N, Li F, Hua K, Deng J, Wang CH, Bowers RR, et al. Increased energy expenditure, dietary fat wasting, and resistance to diet-induced obesity in mice lacking renin. Cell Metab (2007) 6:506–12. doi:10.1016/j.cmet.2007.10.011
59. Kouyama R, Suganami T, Nishida J, Tanaka M, Toyoda T, Kiso M, et al. Attenuation of diet-induced weight gain and adiposity through increased energy expenditure in mice lacking angiotensin II type 1a receptor. Endocrinology (2005) 146:3481–9. doi:10.1210/en.2005-0003
60. Li XC, Navar LG, Shao Y, Zhuo JL. Genetic deletion of AT1a receptors attenuates intracellular accumulation of ANG II in the kidney of AT1a receptor-deficient mice. Am J Physiol Renal Physiol (2007) 293:F586–93. doi:10.1152/ajprenal.00489.2006
61. Li XC, Shao Y, Zhuo JL. AT1a receptor knockout in mice impairs urine concentration by reducing basal vasopressin levels and its receptor signaling proteins in the inner medulla. Kidney Int (2009) 76:169–77. doi:10.1038/ki.2009.134
62. Massiera F, Seydoux J, Geloen A, Quignard-Boulange A, Turban S, Saint-Marc P, et al. Angiotensinogen-deficient mice exhibit impairment of diet-induced weight gain with alteration in adipose tissue development and increased locomotor activity. Endocrinology (2001) 142:5220–5. doi:10.1210/endo.142.12.8556
63. Taniguchi K, Sugiyama F, Kakinuma Y, Uehara S, Nishijho N, Tanimoto K, et al. Pathologic characterization of hypotensive C57BL/6J-agt: angiotensinogen-deficient C57BL/6J mice. Int J Mol Med (1998) 1:583–7.
64. Lochard N, Silversides DW, van Kats JP, Mercure C, Reudelhuber TL. Brain-specific restoration of angiotensin II corrects renal defects seen in angiotensinogen-deficient mice. J Biol Chem (2003) 278:2184–9. doi:10.1074/jbc.M209933200
65. Jayasooriya AP, Mathai ML, Walker LL, Begg DP, Denton DA, Cameron-Smith D, et al. Mice lacking angiotensin-converting enzyme have increased energy expenditure, with reduced fat mass and improved glucose clearance. Proc Natl Acad Sci U S A (2008) 105:6531–6. doi:10.1073/pnas.0802690105
66. de Kloet AD, Krause EG, Woods SC. The renin angiotensin system and the metabolic syndrome. Physiol Behav (2010) 100:525–34. doi:10.1016/j.physbeh.2010.03.018
67. Berneis K, Ninnis R, Häussinger D, Keller U. Effects of hyper- and hypoosmolality on whole body protein and glucose kinetics in humans. Am J Physiol (1999) 276:E188–95.
68. Bilz S, Ninnis R, Keller U. Effects of hypoosmolality on whole-body lipolysis in man. Metabolism (1999) 48:472–6. doi:10.1016/S0026-0495(99)90106-3
69. Keller U, Szinnai G, Bilz S, Berneis K. Effects of changes in hydration on protein, glucose and lipid metabolism in man: impact on health. Eur J Clin Nutr (2003) 57:S69–74. doi:10.1038/sj.ejcn.1601904
70. Schliess F, Häussinger D. Cell volume and insulin signaling. Int Rev Cytol (2003) 225:187–228. doi:10.1016/S0074-7696(05)25005-2
71. Schliess F, Richter L, vom Dahl S, Häussinger D. Cell hydration and mTOR-dependent signalling. Acta Physiol (Oxf) (2006) 187:223–9. doi:10.1111/j.1748-1716.2006.01547.x
72. Schliess F, Reinehr R, Häussinger D. Osmosensing and signaling in the regulation of mammalian cell function. FEBS J (2007) 274:5799–803. doi:10.1111/j.1742-4658.2007.06100.x
73. Dikalov SI, Nazarewicz RR. Angiotensin II-induced production of mitochondrial reactive oxygen species: potential mechanisms and relevance for cardiovascular disease. Antioxid Redox Signal (2013) 19:1085–94. doi:10.1089/ars.2012.4604
74. Burks TN, Marx R, Powell L, Rucker J, Bedja D, Heacock E, et al. Combined effects of aging and inflammation on renin-angiotensin system mediate mitochondrial dysfunction and phenotypic changes in cardiomyopathies. Oncotarget (2015) 6:11979–93. doi:10.18632/oncotarget.3979
75. Kadoguchi T, Kinugawa S, Takada S, Fukushima A, Furihata T, Homma T, et al. Angiotensin II can directly induce mitochondrial dysfunction, decrease oxidative fibre number and induce atrophy in mouse hindlimb skeletal muscle. Exp Physiol (2015) 100:312–22. doi:10.1113/expphysiol.2014.084095
76. Takada S, Kinugawa S, Hirabayashi K, Suga T, Yokota T, Takahashi M, et al. Angiotensin II receptor blocker improves the lowered exercise capacity and impaired mitochondrial function of the skeletal muscle in type 2 diabetic mice. J Appl Physiol (1985) 114:844–57. doi:10.1152/japplphysiol.00053.2012
77. de Cavanagh EM, Toblli JE, Ferder L, Piotrkowski B, Stella I, Fraga CG, et al. Angiotensin II blockade improves mitochondrial function in spontaneously hypertensive rats. Cell Mol Biol (Noisy-le-grand) (2005) 51:573–8.
78. Sumbalová Z, Kucharská J, Kristek F. Losartan improved respiratory function and coenzyme Q content in brain mitochondria of young spontaneously hypertensive rats. Cell Mol Neurobiol (2010) 30:751–8. doi:10.1007/s10571-010-9501-4
79. de Cavanagh EM, Inserra F, Ferder L. Angiotensin II blockade: a strategy to slow ageing by protecting mitochondria? Cardiovasc Res (2011) 89:31–40. doi:10.1093/cvr/cvq285
80. Kurokawa H, Sugiyama S, Nozaki T, Sugamura K, Toyama K, Matsubara J, et al. Telmisartan enhances mitochondrial activity and alters cellular functions in human coronary artery endothelial cells via AMP-activated protein kinase pathway. Atherosclerosis (2015) 239:375–85. doi:10.1016/j.atherosclerosis.2015.01.037
81. Méndez-Giménez L, Rodríguez A, Balaguer I, Frühbeck G. Role of aquaglyceroporins and caveolins in energy and metabolic homeostasis. Mol Cell Endocrinol (2014) 397:78–92. doi:10.1016/j.mce.2014.06.017
82. McCommis KS, Finck BN. Mitochondrial pyruvate transport: a historical perspective and future research directions. Biochem J (2015) 466:443–54. doi:10.1042/BJ20141171
83. Boschmann M, Steiniger J, Hille U, Tank J, Adams F, Sharma AM, et al. Water-induced thermogenesis. J Clin Endocrinol Metab (2003) 88:6015–9. doi:10.1210/jc.2003-030780
84. Brown CM, Dulloo AG, Montani JP. Water-induced thermogenesis reconsidered: the effects of osmolality and water temperature on energy expenditure after drinking. J Clin Endocrinol Metab (2006) 91:3598–602. doi:10.1210/jc.2006-0407
85. Boschmann M, Steiniger J, Franke G, Birkenfeld AL, Luft FC, Jordan J. Water drinking induces thermogenesis through osmosensitive mechanisms. J Clin Endocrinol Metab (2007) 92:3334–7. doi:10.1210/jc.2006-1438
86. Dubnov-Raz G, Constantini NW, Yariv H, Nice S, Shapira N. Influence of water drinking on resting energy expenditure in overweight children. Int J Obes (Lond) (2011) 35:1295–300. doi:10.1038/ijo.2011.130
87. Kocełak P, Zak-Gołab A, Rzemieniuk A, Smętek J, Sordyl R, Tyrka A, et al. The influence of oral water load on energy expenditure and sympatho-vagal balance in obese and normal weight women. Arch Med Sci (2012) 8:1003–8. doi:10.5114/aoms.2012.32406
88. Ruskoaho H, Lang RE, Toth M, Ganten D, Unger T. Release and regulation of atrial natriuretic peptide (ANP). Eur Heart J (1987) 8:B99–109. doi:10.1093/eurheartj/8.suppl_B.99
89. Inuzuka M, Tamura N, Yamada N, Katsuura G, Oyamada N, Taura D, et al. C-type natriuretic peptide as a new regulator of food intake and energy expenditure. Endocrinology (2010) 151:3633–42. doi:10.1210/en.2010-0141
90. Bordicchia M, Liu D, Amri EZ, Ailhaud G, Dessì-Fulgheri P, Zhang C, et al. Cardiac natriuretic peptides act via p38 MAPK to induce the brown fat thermogenic program in mouse and human adipocytes. J Clin Invest (2012) 122:1022–36. doi:10.1172/JCI59701
91. Engeli S, Birkenfeld AL, Badin PM, Bourlier V, Louche K, Viguerie N, et al. Natriuretic peptides enhance the oxidative capacity of human skeletal muscle. J Clin Invest (2012) 122:4675–9. doi:10.1172/JCI64526
92. Wang TJ. The natriuretic peptides and fat metabolism. N Engl J Med (2012) 367:377–8. doi:10.1056/NEJMcibr1204796
93. Whittle AJ, Vidal-Puig A. NPs – heart hormones that regulate brown fat? J Clin Invest (2012) 122:804–7. doi:10.1172/JCI62595
94. Collins S, Bordicchia M. Heart hormones fueling a fire in fat. Adipocyte (2013) 2:104–8. doi:10.4161/adip.22515
95. Moro C, Lafontan M. Natriuretic peptides and cGMP signaling control of energy homeostasis. Am J Physiol Heart Circ Physiol (2013) 304:H358–68. doi:10.1152/ajpheart.00704.2012
96. Bacay AC, Mantyh CR, Vigna SR, Mantyh PW. Receptor binding sites for atrial natriuretic factor are expressed by brown adipose tissue. Peptides (1988) 9:1021–6. doi:10.1016/0196-9781(88)90083-6
97. Okamura H, Kelly PA, Chabot JG, Morel G, Belles-Isles M, Heisler S. Atrial natriuretic peptide receptors are present in brown adipose tissue. Biochem Biophys Res Commun (1988) 156:1000–6. doi:10.1016/S0006-291X(88)80943-4
98. Campbell SM. Hydration needs throughout the lifespan. J Am Col Nutr (2007) 26:585S–7S. doi:10.1080/07315724.2007.10719662
99. Odorizzi M, Max JP, Tankosic P, Burlet C, Burlet A. Dietary preferences of Brattleboro rats correlated with an overexpression of galanin in the hypothalamus. Eur J Neurosci (1999) 11:3005–14. doi:10.1046/j.1460-9568.1999.00722.x
100. Beck B, Max JP. Hypothalamic galanin and plasma leptin and ghrelin in the maintenance of energy intake in the Brattleboro rat. Biochem Biophys Res Commun (2007) 364:60–5. doi:10.1016/j.bbrc.2007.09.092
101. Zelena D, Mergl Z, Makara GB. Postnatal development in vasopressin deficient Brattleboro rats with special attention to the hypothalamo-pituitary-adrenal axis function: the role of maternal genotype. Int J Dev Neurosci (2009) 27:175–83. doi:10.1016/j.ijdevneu.2008.11.003
102. Idbaih A, Burlet A, Adle-Biassette H, Boisgard R, Coulon C, Paris S, et al. Altered cerebral glucose metabolism in an animal model of diabetes insipidus: a micro-PET study. Brain Res (2007) 1158:164–8. doi:10.1016/j.brainres.2007.05.016
103. Stookey JD, Constant F, Gardner CD, Popkin BM. Replacing sweetened caloric beverages with drinking water is associated with lower energy intake. Obesity (Silver Spring) (2007) 15:3013–22. doi:10.1038/oby.2007.359
104. Stookey JD, Constant F, Popkin BM, Gardner CD. Drinking water is associated with weight loss in overweight dieting women independent of diet and activity. Obesity (Silver Spring) (2008) 16:2481–8. doi:10.1038/oby.2008.409
105. Daniels MC, Popkin BM. Impact of water intake on energy intake and weight status: a systematic review. Nutr Rev (2010) 68:505–21. doi:10.1111/j.1753-4887.2010.00311.x
106. Armstrong LE. Challenges of linking chronic dehydration and fluid consumption to health outcomes. Nutr Rev (2012) 70:S121–7. doi:10.1111/j.1753-4887.2012.00539.x
107. Muckelbauer R, Sarganas G, Grüneis A, Müller-Nordhorn J. Association between water consumption and body weight outcomes: a systematic review. Am J Clin Nutr (2013) 98:282–99. doi:10.3945/ajcn.112.055061
108. Vij VA, Joshi AS. Effect of ‘water induced thermogenesis’ on body weight, body mass index and body composition of overweight subjects. J Clin Diagn Res (2013) 7:1894–6. doi:10.7860/JCDR/2013/5862.3344
109. Borys JM, de Ruyter JC, Finch H, Harper P, Levy E, Mayer J, et al. Hydration and obesity prevention. Obes Facts (2014) 7:S37–48. doi:10.1159/000360748
110. Muckelbauer R, Barbosa CL, Mittag T, Burkhardt K, Mikelaishvili N, Müller-Nordhorn J. Association between water consumption and body weight outcomes in children and adolescents: a systematic review. Obesity (Silver Spring) (2014) 22:2462–75. doi:10.1002/oby.20911
111. Vij VA, Joshi AS. Effect of excessive water intake on body weight, body mass index, body fat, and appetite of overweight female participants. J Nat Sci Biol Med (2014) 5:340–4. doi:10.4103/0976-9668.136180
112. Davy BM, Dennis EA, Dengo AL, Wilson KL, Davy KP. Water consumption reduces energy intake at a breakfast meal in obese older adults. J Am Diet Assoc (2008) 108:1236–9. doi:10.1016/j.jada.2008.04.013
113. Dennis EA, Dengo AL, Comber DL, Flack KD, Savla J, Davy KP, et al. Water consumption increases weight loss during a hypocaloric diet intervention in middle-aged and older adults. Obesity (Silver Spring) (2010) 18:300–7. doi:10.1038/oby.2009.235
114. Parretti HM, Aveyard P, Blannin A, Clifford SJ, Coleman SJ, Roalfe A, et al. Efficacy of water preloading before main meals as a strategy for weight loss in primary care patients with obesity: RCT. Obesity (Silver Spring) (2015) 23:1785–91. doi:10.1002/oby.21167
115. Weigle DS, Breen PA, Matthys CC, Callahan HS, Meeuws KE, Burden VR, et al. A high-protein diet induces sustained reductions in appetite, ad libitum caloric intake, and body weight despite compensatory changes in diurnal plasma leptin and ghrelin concentrations. Am J Clin Nutr (2005) 82:41–8.
116. Pesta DH, Samuel VT. A high-protein diet for reducing body fat: mechanisms and possible caveats. Nutr Metab (Lond) (2014) 11:53–61. doi:10.1186/1743-7075-11-53
117. L’Heureux-Bouron D, Tomé D, Bensaid A, Morens C, Lacroix M, Huneau JF, et al. Preabsorptive factors are not the main determinants of intake depression induced by a high-protein diet in the rat. Physiol Behav (2004) 81:499–504. doi:10.1016/j.physbeh.2004.02.029
118. Faipoux R, Tomé D, Bensaid A, Morens C, Oriol E, Bonnano LM, et al. Yeast proteins enhance satiety in rats. J Nutr (2006) 136:2350–6.
119. Bouby N, Trinh-Trang-Tan MM, Coutaud C, Bankir L. Vasopressin is involved in renal effects of high-protein diet: study in homozygous Brattleboro rats. Am J Physiol (1991) 260:F96–100.
120. Aparicio VA, Nebot E, Porres JM, Ortega FB, Heredia JM, López-Jurado M, et al. Effects of high-whey-protein intake and resistance training on renal, bone and metabolic parameters in rats. Br J Nutr (2011) 105:836–45. doi:10.1017/S0007114510004393
121. Bankir L, Bouby N, Trinh-Trang-Tan MM, Ahloulay M, Promeneur D. Direct and indirect cost of urea excretion. Kidney Int (1996) 49:1598–607. doi:10.1038/ki.1996.232
122. Zaragozá R, Renau-Piqueras J, Portolés M, Hernández-Yago J, Jordá A, Grisolía S. Rats fed prolonged high protein diets show an increase in nitrogen metabolism and liver megamitochondria. Arch Biochem Biophys (1987) 258:426–35. doi:10.1016/0003-9861(87)90364-X
123. Jordá A, Zaragozá R, Portolés M, Báguena-Cervellera R, Renau-Piqueras J. Long-term high-protein diet induces biochemical and ultrastructural changes in rat liver mitochondria. Arch Biochem Biophys (1988) 265:241–8. doi:10.1016/0003-9861(88)90124-5
124. Mittal A, Muthukumar A, Jolly CA, Zaman K, Fernandes G. Reduced food consumption increases water intake and modulates renal aquaporin-1 and -2 expression in autoimmune prone mice. Life Sci (2000) 66:1471–9. doi:10.1016/S0024-3205(00)00464-1
125. Thornton SN, Even PC, van Dijk G. Hydration increases cell metabolism. Int J Obes (Lond) (2009) 33:385. doi:10.1038/ijo.2008.264
126. Underwood PC, Adler GK. The renin angiotensin aldosterone system and insulin resistance in humans. Curr Hypertens Rep (2013) 15:59–70. doi:10.1007/s11906-012-0323-2
127. Thornton SN. Diabetes and hypertension, as well as obesity and Alzheimer’s disease, are linked to hypohydration-induced lower brain volume. Front Aging Neurosci (2014) 6:279. doi:10.3389/fnagi.2014.00279
128. Bonnet F, Lepicard EM, Cathrin L, Letellier C, Constant F, Hawili N, et al. French children start their school day with a hydration deficit. Ann Nutr Metab (2012) 60:257–63. doi:10.1159/000337939
129. Stookey JD, Brass B, Holliday A, Arieff A. What is the cell hydration status of healthy children in the USA? Preliminary data on urine osmolality and water intake. Public Health Nutr (2012) 15:2148–56. doi:10.1017/S1368980011003648
Keywords: drinking, water, angiotensin, lipolysis, hypovolemia, hypohydration
Citation: Thornton SN (2016) Increased Hydration Can Be Associated with Weight Loss. Front. Nutr. 3:18. doi: 10.3389/fnut.2016.00018
Received: 21 December 2015; Accepted: 30 May 2016;
Published: 10 June 2016
Edited by:
Gilles Fromentin, French National Institute for Agricultural Research and AgroParisTech, FranceReviewed by:
Derek Daniels, State University of New York at Buffalo, USAJodi Dunmeyer Stookey, Children’s Hospital Oakland Research Institute, USA
Copyright: © 2016 Thornton. This is an open-access article distributed under the terms of the Creative Commons Attribution License (CC BY). The use, distribution or reproduction in other forums is permitted, provided the original author(s) or licensor are credited and that the original publication in this journal is cited, in accordance with accepted academic practice. No use, distribution or reproduction is permitted which does not comply with these terms.
*Correspondence: Simon N. Thornton, c2ltb24udGhvcm50b25AdW5pdi1sb3JyYWluZS5mcg==