- 1Department of Radiology, Mayo Clinic, Rochester, MN, United States
- 2Department of Laboratory Medicine and Pathology, Mayo Clinic, Rochester, MN, United States
- 3Division of Medical Oncology, Mayo Clinic, Rochester, MN, United States
- 4Division of Gastroenterology and Hepatology, Mayo Clinic, Rochester, MN, United States
- 5Division of Hepatobiliary and Pancreas Surgery, Mayo Clinic, Rochester, MN, United States
Purpose: The aims of this study were to evaluate and compare fibroblast activation protein (FAP) expression and localization in surgically resected cholangiocarcinoma (CCA), primary and metastatic hepatocellular carcinoma (HCC), hepatocellular adenoma (HCA), and focal nodular hyperplasia (FNH), and to identify any association between CCA clinical or pathologic features and FAP expression.
Materials and methods: FAP immunostaining from surgically resected CCA (N = 58), primary intrahepatic and extrahepatic metastatic HCC (N = 148), HCA (N26), and FNH (N = 19) was scored (negative, weak positive, moderate positive or strong positive) from tissue microarrays. FAP expression was compared between groups. CCA FAP expression was compared to clinical and tumor pathology features.
Results: Moderate-strong FAP expression in the tumor stroma was present in 93.1% of CCA, 60.7% of extrahepatic metastatic HCC, 29.6% of primary HCC, 21.1% of FNH, and 11.6% of HCA. Moderate-strong FAP expression in tumor stroma was significantly more prevalent in CCA than HCC (p < 0.001), metastatic HCC (p = 0.005), HCA (p < 0.001) and FNH (p < 0.001). FAP was expressed in the stroma of all but one CCA (1.7%), and FAP expression in CCA tumor stroma was not associated with any clinical or tumor pathology features (p > 0.05, all).
Conclusion: FAP is expressed in the stroma of a high proportion (93%) of primary CCA independent of patient clinical or tumor pathology features. As such, these data provide the tissue basis for systematically evaluating FAP as a theranostic target across a broad range of CCA subtypes.
Introduction
Cholangiocarcinomas (CCAs) and gallbladder cancer are known as biliary tract cancers and account for approximately 3% of all gastrointestinal malignancies (1). There are approximately 6,100 cases of intrahepatic and 12,000 cases of extrahepatic cholangiocarcinomas diagnosed annually in the United States (2, 3). There are several risk factors for CCAs, mainly primary sclerosing cholangitis, fibropolycystic liver disease, recurrent pyogenic cholangitis, chronic liver disease, obesity, and metabolic syndrome; however, a specific risk factor is often not identified for many patients. The prognosis for advanced CCA is poor with or without treatment, with the majority of patients having an overall median survival of less than 12 months (4, 5), depending on the disease stage and subtype.
Perihilar tumors comprise about 50% of disease, extrahepatic 40%, and intrahepatic 10% (6). CCAs are adenocarcinomas and often have a dense desmoplastic reaction. Resectability rates are low (7). Importantly, the tumor microenvironment has relative genetic stability and enhances tumor growth, which makes it an important potential target for therapy, given that it cannot develop resistance to therapies through mutation as easily compared to epithelial components (8, 9).
In recent years, fibroblast activation protein (FAP), a cell membrane bound type II serine protease, has emerged as a marker for identifying several tumor types due to its presence in the tumor stroma and has been shown to be associated with worse outcomes in many tumors (10, 11). FAP is overexpressed in tumors with robust desmoplastic reactions and has been validated as a target for molecular diagnostics (12–14). Such desmoplastic reaction is typical of a majority of CCAs. In fact, prior studies using FAP-inhibitor positron emission tomography (PET) radiotracers have shown higher 68Ga-FAPI vs. 18F-FDG uptake in CCA compared to hepatocellular carcinoma (HCC) (15, 16). This has opened the door for FAP-inhibitor development for tumor-specific targeting small molecule inhibitors and radiopharmaceuticals (17–19). Taken together, FAP may be a candidate theranostic target in CCA with FAP-targeted PET imaging and theranostics.
The aims of this study were to evaluate and compare the protein expression and localization of FAP in surgically resected CCA, primary intrahepatic and extrahepatic metastatic HCC, hepatocellular adenoma (HCA), and focal nodular hyperplasia (FNH), and to identify any association between CCA clinical or pathologic features and FAP expression.
Materials and methods
This HIPAA-compliant study was conducted following institutional review board (IRB) approval. Tissue Microarrays (TMAs) were constructed from patients who had authorized use of their medical record for research purposes. A waiver of written informed consent was obtained from the IRB.
Tissue microarrays
Previously constructed TMAs were utilized for the current study and were created using methods previously described (20). TMAs were constructed using the ISEnet Galileo CK4500 instrument (Integrated Systems Engineering srl, Milan, Italy) with 1.0 mm cores from formalin-fixed paraffin-embedded donor blocks stored in the institutional pathology tissue archives selected by a hepatobiliary pathologist (>22 years of experience). Control tissues were included within the array and for orientation including normal prostate, normal liver, ovarian cancer, normal tonsil, and cervical cancer tissues. TMAs from surgically resected CCA (n = 58), primary intrahepatic HCC (n = 134), extrahepatic metastatic HCC (n = 28), HCA (n = 26), and FNH (n = 19) were used.
Immunohistochemical staining: anti-fibroblast activation protein
Immunohistochemical (IHC) staining was performed at the Pathology Research Core (Mayo Clinic, Rochester, MN) using the Leica Bond RX stainer (Leica). TMA sections were cut at 5 microns, mounted on charged slides, and dried overnight. For staining, slides were retrieved for 20 min using Epitope Retrieval 1 (Citrate; Leica) and incubated in Protein Block (Dako) for 5 min. The FAP primary antibody (Clone: EPR20021; Abcam ab207178) was diluted to 1:300 in Background Reducing Diluent (Dako) and incubated for 15 min (21, 22).
The detection system used was Polymer Refine Detection System (Leica). This system includes a hydrogen peroxidase block, post primary and polymer reagents, and DAB. Immunostaining visualization was achieved by incubating slides for 10 min in DAB and DAB buffer (1:19 mixture) from the Bond Polymer Refine Detection System. Slides were counterstained for five minutes using a 1:1 mixture of Schmidt hematoxylin (Mayo DLMP Preparation and Processing Laboratory) and molecular biology grade water. Once the immunochemistry process was completed, slides were removed from the stainer and rinsed in tap water for three minutes. Slides were dehydrated in increasing concentrations of ethyl alcohol and cleared in 3 changes of xylene prior to permanent coverslipping in xylene-based medium. Separate slides were stained for hematoxylin and eosin (H&E).
Pathology review
All H&E and FAP-stained sections were evaluated in a blinded and random fashion by a hepatobiliary pathologist (MST). Histopathology features were analyzed by H&E for tumor grade (well-differentiated, moderately differentiated, poorly differentiated) and histological subtype (small, large, mixed). Both FAP staining intensity and percentage area tumor stroma involved were scored for the FAP-stained sections. The score was a 2-digit number: one for intensity and one for tumor stroma percentage staining. A similar scoring method was previously validated for assessing prostate-specific membrane agent (PSMA) expression by IHC in hepatobiliary tumors (20). The intensity was scored as follows: 0 = none, 1 = weak, 2 = moderate, 3 = strong. The FAP-stained score for percentage staining by area was as follows: 0% staining = 0, 1%–5% staining = 1, 6%–33% staining = 2, 34%–66% staining = 3, and 67%–100% staining = 4. The final interpretation of these two digits was obtained by combining the intensity score and tumor stroma percentage score as follows: 11–12 = negative; 13, 14, 21 = weak positive; 22, 23 = moderate positive; 24, 31–34 = strong positive.
CCA demographic and clinical data
Demographic and clinical data for the subset of 58 CCA patients were extracted from the electronic medical record.
Statistical analyses
Study data were collected and managed using REDCap electronic data capture tools (23, 24). Data were analyzed using JMP 16.2.0 (Raleigh, NC). FAP expression by IHC (moderate/strong vs. weak/none and present vs. absent) was compared between CCA, HCC, metastatic HCC, HCA, and FNA groups using Fisher's exact test. Sub-group analysis of the CCA subjects was performed. Categorical variables were compared using the chi-square test or Fisher's exact test. Continuous variables were compared using the one-way ANOVA test or Kruskal-Wallis test. Differences in CCA FAP expression were compared by CCA pathology features (anatomic class, morphology, cellularity type, tumor grade, and size of the involved ducts) using Fisher's exact test. Differences in CCA tumor size were compared by fibroblast activation protein inhibitor (FAPI) expression using a Kruskal-Wallis test. Univariate logistic regression was performed to assess association between FAPI expression with clinical characteristics including absence or presence of choledocholithiasis, cholelithiasis, primary sclerosing cholangitis, cirrhosis, hepatitis B virus, hepatitis C virus, hemochromatosis, inflammatory bowel disease, primary biliary cirrhosis, diabetes, hypertension, alcohol use, cigarette smoking, hyperlipidemia, and asbestos exposure. A p < 0.05 was considered statistically significant.
Results
FAP protein expression and localization by tumor type
FAP IHC data by tumor type are summarized in Table 1. Moderate-strong FAP expression in the tumor stroma was present in 93.1% (54/58) of CCA (Figures 1–3), 60.7% (17/28) of metastatic HCC (Supplementary Figure S1), 29.6% (42/142) of primary HCC (Supplementary Figure S2), 21.1% (4/19) of FNH (Supplementary Figure S3) and 11.6% (3/26) of HCA (Supplementary Figure S4). FAP expression was not localized to the tumor cells and there was no significant FAP expression in normal background biliary tissue. There was no detectable FAP expression in only one CCA case (Figure 1A, 1.7%), whereas the majority of primary HCC, HCA, and FNH had no detectable FAP expression (57%, 76.9%, and 78.9%, respectively). When comparing moderate/strong vs. negative/weak staining between groups, moderate-strong FAP staining was significantly more prevalent in CCA vs. primary HCC (93.1% v. 29.6%; p < 0.0001), metastatic HCC lesions (93.1% v. 60.7%; p = 0.0005), FNH (93.1% v. 21.1% p < 0.0001), and HCA (93.1% v. 11.6%; p < 0.0001), respectively. Moderate-strong FAP staining was significantly more prevalent in metastatic HCC compared to primary HCC (60.7% v. 29.6%; p = 0.0023), FNH (60.7% vs. 21.1%; p = 0.009) and HCA (60.7% v. 11.6%; p = 0.0002), respectively, but there was no difference in FAP staining between primary HCC and FNH or HCA (p = 0.59 and p = 0.089, respectively). There was no significant difference in FAP expression between FNH and HCA (p = 0.43). Similar results were found when comparing present vs. absent FAP staining between groups.
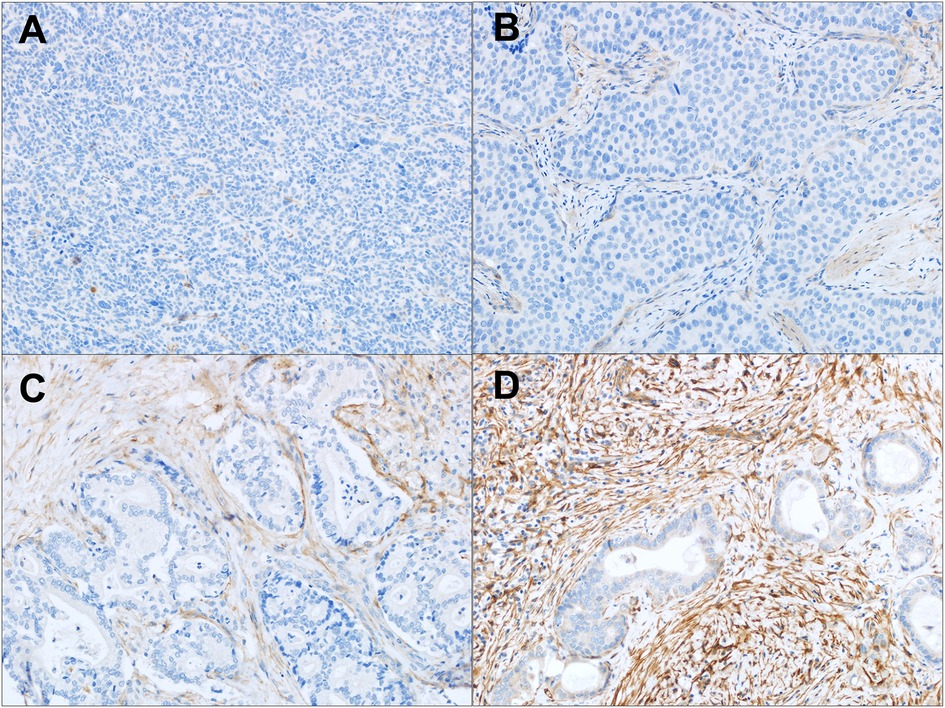
Figure 1. FAP expression in intrahepatic CCA. (A–D) Tumor associated stroma FAP staining: (A) absent staining in a poorly differentiated CCA; (B) weak staining in a poorly differentiated CCA; (C) moderate staining in a moderately differentiated CCA; and (D) strong staining in a moderately differentiated CCA. Original magnification (20x).
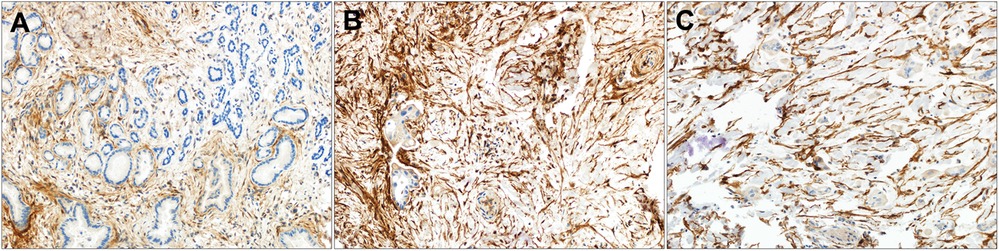
Figure 2. Strong FAP expression in perihilar CCA by tumor grade. (A–C) Tumor associated stroma FAP staining in (A) well-differentiated, (B) moderately differentiated and (C) poorly differentiated perihilar CCA. Original magnification (20x).
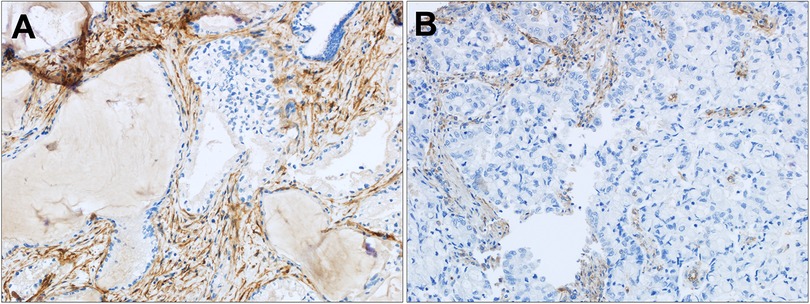
Figure 3. FAP expression in distal and mixed CCA. (A) Strong tumor associated stroma FAP staining in a moderately differentiated distal CCA and (B) moderate tumor associated stroma FAP staining in a poorly differentiated mixed CCA. Original magnification (20x).
FAP by CCA clinical and tumor pathology characteristics
CCA patient clinical and tumor pathology data are summarized in Tables 2 and 3. There was no significant association between FAPI expression and patient age (p = 0.33), gender (p = 0.76), race (p = 0.99), ethnicity (p = 0.91), presence of choledocholithiasis (p = 0.088), cholelithiasis (p = 0.66), primary sclerosing cholangitis (p = 0.75), cirrhosis (p = 0.49), hepatitis B virus (p = 0.85), hepatitis C virus (p = 0.053), hereditary hemochromatosis (p = 0.94), inflammatory bowel disease (p = 0.93), primary biliary cirrhosis (p = 0.86), diabetes (p = 0.72), obesity (p = 0.41), hypertension (p = 0.13), alcohol use (p = 0.41), cigarette smoking (p = 0.52), hyperlipidemia (p = 0.58), or asbestos exposure (p = 0.83). There was no significant association between FAP expression and CCA tumor size (p = 0.20), anatomic location (p = 0.15), morphologic subtype (p = 0.99), cellularity type (p = 0.32), tumor grade (p = 0.56), or duct size (p = 0.86).
Discussion
The current FAP IHC data confirmed moderate to strong FAP expression in a significantly higher proportion of CCA (93.1%, 54 of 58 patients) compared to other malignant or benign liver tumors. Moreover, FAP was expressed in the tumor stroma of the majority (98.3%, 57 of 58 patients) of CCA, independent of patient clinical or tumor characteristics. Taken together, these data provide the tissue basis for systematically evaluating FAP as a diagnostic and therapeutic target across a broad range of CCA subtypes using FAP-targeted theranostic radiotracers.
These data add to the growing body of evidence supporting current and future clinical trials investigating FAP as both a diagnostic and therapeutic target across multiple tumor types, including biliary tract cancers (16, 25–33). Several studies have shown that CCA cancer-associated fibroblasts induce a robust desmoplastic reaction (34–38). This has in turn supported the development of FAP-targeted PET tracers, which have been used to image at least 28 different cancer types in humans, including CCA (13, 15, 16). When compared to 18F-FDG PET, 68Ga-FAPI based PET agent performance has been shown to be superior at discrimination of benign vs. malignant lesions, locating primary tumors in metastatic disease (15, 16, 31), and has resulted in restaging in up to 33% of cases (39). Several FAP-targeted PET agents have been used in clinical trials, including 68Ga-FAPI-02 (18), 68Ga-FAPI-04 (17), 68Ga-FAPI-46 (40, 41), and 68Ga-FAPI-34 (42). 18F-FDG is limited in its ability to stage CCA due to suboptimal liver imaging kinetics (43). Despite high glucose uptake in bile duct epithelial cells, aggressive CCAs are typically not FDG-avid (29, 30, 33, 44, 45). Thus, there is a strong unmet need for improved molecular imaging in CCA.
While a minority of primary HCCs demonstrated moderate to strong FAP expression, a majority of metastatic HCC lesions had moderate to strong FAP expression, suggesting FAP may be a consideration as a theranostic target in metastatic HCC but less so in primary HCC (15, 28, 43). Alternatively, PSMA has been shown to be expressed in a high percentage (>90%) of primary HCCs by IHC, and subsequently confirmed in patients with 68Ga-PSMA PET imaging with greater than 70% of HCC demonstrating grade 3 or 4 PSMA uptake at PET (20). Furthermore, a small percentage of FNH and HCA demonstrated moderate to strong FAP expression, suggesting the FAP-expressing cancer-associated fibroblasts are not entirely specific to malignant lesions. As such, molecular imaging findings will need to be correlated with contrast-enhanced CT or MRI findings for these benign liver tumors.
There are limitations to this study. The IHC was evaluated by a single reader. The correlative data between tissue CCA FAP expression, demographic data, and tumor characteristics are based on TMA and not full tissue sections. This study does not compare FAP expression with CCA genetic mutations or molecular alterations such as clinically relevant, targetable mutations in isocitrate dehydrogenase-1 mutations or fibroblast growth factor receptor-2 fusions or immunotherapy-related indicators such as PD-L1 expression and effector T cell infiltration (46, 47). Additionally, only 1.7% of CCAs were distal in location, and 5% were well-differentiated CCA tumors, thereby limiting the generalizability to these subtypes. Furthermore, only one CCA case was completely negative for FAP expression. This case was a 52-year-old white male with history of cholelithiasis and hepatitis C virus infection, obesity and smoking with a 4.4 cm moderately differentiated, mass forming intrahepatic CCA with small duct size. Given the lack of clinical and tumor features associated with FAP expression in this cohort, the absence of FAP expression in this case cannot be hypothesized. Taken together, while the CCA cases herein are representative of a broad range of CCA subtypes including intrahepatic and perihilar location, moderately and poorly differentiated grades and small and large duct size, further work in larger cohorts of CCA patients will be needed to better understand such outlier cases. Furthermore, due to the retrospective nature, our study does not include any FAP-targeted PET imaging to correlate the degree of FAP expression in the IHC staining data with diagnostic and potentially prognostic significance. Prior studies assessing IHC-PET correlation in HCC with PSMA PET imaging showed that while >90% of HCC were positive for PSMA expression by IHC, >70% of HCC tumors showed high (i.e., grade 3 or 4) uptake at PSMA PET imaging (20). As such, further work is needed to assess FAP expression by IHC and tumor uptake at FAPI PET imaging across a range of benign and malignant liver lesions. Lastly, moderate-strong FAP staining was significantly more prevalent in metastatic HCC compared to primary HCC (60.7% v. 29.6%, respectively). One hypothesis is that there is a greater desmoplastic reaction in the tumor microenvironment with metastatic extrahepatic HCC compared to primary HCC in the liver. Nonetheless, while FAP may be a potential theranostic target in extrahepatic metastatic HCC, further research is needed across both primary intrahepatic and extrahepatic metastatic HCC.
In summary, FAP is expressed in a high proportion (93%) of primary CCA independent of patient clinical or tumor pathology features. As such, these data provide the tissue basis for future studies systematically evaluating FAP as a theranostic target across a broad range of CCA subtypes using paired FAP-targeted PET. FAP-targeted radioligand therapy represents a novel therapeutic approach to targeting the CCA tumor microenvironment in FAP expressing CCA and may be useful in combination with other CCA molecular-targeted or immunotherapies. Such novel diagnostic and therapeutic approaches may help improve outcomes for patients with unresectable CCA.
Data availability statement
The raw data supporting the conclusions of this article will be made available by the authors, without undue reservation.
Ethics statement
The studies involving humans were approved by Mayo Clinic Institutional Review Board IRB #19-001786. The studies were conducted in accordance with the local legislation and institutional requirements. The Ethics Committee/institutional review board waived the requirement of written informed consent for participation from the participants or the participants' legal guardians/next of kin because study was conducted using tissue microarrays previously created under IRB #19-001786 using tissue from surgical resection specimens from patients that had agreed to use of their clinically obtained tissue for research purposes; as such, the IRB granted a waiver of written informed consent.
Author contributions
LJ: Conceptualization, Data curation, Formal Analysis, Investigation, Writing – original draft, Writing – review & editing. MT: Conceptualization, Data curation, Formal Analysis, Investigation, Methodology, Project administration, Visualization, Writing – original draft, Writing – review & editing. TH: Writing – original draft, Writing – review & editing. LK: Writing – review & editing. NT: Writing – original draft, Writing – review & editing. LR: Writing – review & editing. RS: Writing – original draft, Writing – review & editing. AG: Conceptualization, Formal Analysis, Writing – original draft, Writing – review & editing. ST: Conceptualization, Data curation, Formal Analysis, Investigation, Methodology, Project administration, Supervision, Writing – original draft, Writing – review & editing.
Funding
The author(s) declare that no financial support was received for the research, authorship, and/or publication of this article.
Acknowledgments
The authors thank Desiree Lanzino, PhD, and Sonia Watson, PhD, for their assistance in editing the manuscript.
Conflict of interest
ST is a research consultant for Boston Scientific and Terumo Corporation, unrelated to the content of this manuscript. AG is the PI on an institutional research grant from Sofie Biosciences and Novartis; Goenka is a paid consultant to Bayer Healthcare, LLC; Candel Therapeutics; and UWorld. AG research is supported by NIH National Cancer Institute grants [R01CA256969] and [R01CA272628-01], Mayo Clinic Comprehensive Cancer Center (MCCCC) and grants from the Champions for Hope Pancreatic Cancer Research Program of the Funk Zitiello Foundation, the Centene Charitable Foundation, and the Hoveida Family Foundation.
The remaining authors declare that the research was conducted in the absence of any commercial or financial relationships that could be construed as a potential conflict of interest.
Publisher's note
All claims expressed in this article are solely those of the authors and do not necessarily represent those of their affiliated organizations, or those of the publisher, the editors and the reviewers. Any product that may be evaluated in this article, or claim that may be made by its manufacturer, is not guaranteed or endorsed by the publisher.
Supplementary material
The Supplementary Material for this article can be found online at: https://www.frontiersin.org/articles/10.3389/fnume.2024.1480471/full#supplementary-material
Supplemental Figure S1 | FAP expression in metastatic hepatocellular carcinoma (mHCC) (A-C). Negative staining (A), weak positive staining (B), strong positive staining (C). Original magnification (20x).
Supplemental Figure S2 | FAP expression in hepatocellular carcinoma (HCC) (A-C). Negative staining (A), weak positive staining (B), strong positive staining (C). Original magnification (20x).
Supplemental Figure S3 | FAP expression in fibronodular hyperplasia (A, B). Negative staining (A), moderate positive staining (B). Original magnification (20x).
Supplemental Figure S4 | FAP expression in hepatic adenoma (HCA) (A-C). Negative staining (A), weak positive staining (B), strong positive staining (C). Original magnification (20x).
References
1. Vauthey JN, Blumgart LH. Recent advances in the management of cholangiocarcinomas. Semin Liver Dis. (1994) 14(2):109–14. doi: 10.1055/s-2007-1007302
2. Patel T. Increasing incidence and mortality of primary intrahepatic cholangiocarcinoma in the United States. Hepatology. (2001) 33(6):1353–7. doi: 10.1053/jhep.2001.25087
3. Siegel RL, Miller KD, Wagle NS, Jemal A. Cancer statistics, 2023. CA Cancer J Clin. (2023) 73(1):17–48. doi: 10.3322/caac.21763
4. Finn RS, Qin S, Ikeda M, Galle PR, Ducreux M, Kim TY, et al. Atezolizumab plus bevacizumab in unresectable hepatocellular carcinoma. N Engl J Med. (2020) 382(20):1894–905. doi: 10.1056/NEJMoa1915745
5. Park J, Kim MH, Kim KP, Park DH, Moon SH, Song TJ, et al. Natural history and prognostic factors of advanced cholangiocarcinoma without surgery, chemotherapy, or radiotherapy: a large-scale observational study. Gut Liver. (2009) 3(4):298–305. doi: 10.5009/gnl.2009.3.4.298
6. DeOliveira ML, Cunningham SC, Cameron JL, Kamangar F, Winter JM, Lillemoe KD, et al. Cholangiocarcinoma: thirty-one-year experience with 564 patients at a single institution. Ann Surg. (2007) 245(5):755–62. doi: 10.1097/01.sla.0000251366.62632.d3
7. Nakeeb A, Pitt HA, Sohn TA, Coleman J, Abrams RA, Piantadosi S, et al. Cholangiocarcinoma. A spectrum of intrahepatic, perihilar, and distal tumors. Ann Surg. (1996) 224(4):463–73; discussion 73–5. doi: 10.1097/00000658-199610000-00005
8. Fabris L, Sato K, Alpini G, Strazzabosco M. The tumor microenvironment in cholangiocarcinoma progression. Hepatology. (2021) 73 Suppl 1(1):75–85. doi: 10.1002/hep.31410
9. Ramamonjisoa N, Ackerstaff E. Characterization of the tumor microenvironment and tumor-stroma interaction by non-invasive preclinical imaging. Front Oncol. (2017) 7:3. doi: 10.3389/fonc.2017.00003
10. Brennen WN, Rosen DM, Wang H, Isaacs JT, Denmeade SR. Targeting carcinoma-associated fibroblasts within the tumor stroma with a fibroblast activation protein-activated prodrug. J Natl Cancer Inst. (2012) 104(17):1320–34. doi: 10.1093/jnci/djs336
11. Saadi A, Shannon NB, Lao-Sirieix P, O'Donovan M, Walker E, Clemons NJ, et al. Stromal genes discriminate preinvasive from invasive disease, predict outcome, and highlight inflammatory pathways in digestive cancers. Proc Natl Acad Sci U S A. (2010) 107(5):2177–82. doi: 10.1073/pnas.0909797107
12. Dendl K, Finck R, Giesel FL, Kratochwil C, Lindner T, Mier W, et al. FAP Imaging in rare cancer entities-first clinical experience in a broad spectrum of malignancies. Eur J Nucl Med Mol Imaging. (2022) 49(2):721–31. doi: 10.1007/s00259-021-05488-9
13. Kratochwil C, Flechsig P, Lindner T, Abderrahim L, Altmann A, Mier W, et al. (68)Ga-FAPI PET/CT: tracer uptake in 28 different kinds of cancer. J Nucl Med. (2019) 60(6):801–5. doi: 10.2967/jnumed.119.227967
14. Sirica AE, Campbell DJ, Dumur CI. Cancer-associated fibroblasts in intrahepatic cholangiocarcinoma. Curr Opin Gastroenterol. (2011) 27(3):276–84. doi: 10.1097/MOG.0b013e32834405c3
15. Shi X, Xing H, Yang X, Li F, Yao S, Congwei J, et al. Comparison of PET imaging of activated fibroblasts and (18)F-FDG for diagnosis of primary hepatic tumours: a prospective pilot study. Eur J Nucl Med Mol Imaging. (2021) 48(5):1593–603. doi: 10.1007/s00259-020-05070-9
16. Siripongsatian D, Promteangtrong C, Kunawudhi A, Kiatkittikul P, Boonkawin N, Chinnanthachai C, et al. Comparisons of quantitative parameters of Ga-68-labelled fibroblast activating protein inhibitor (FAPI) PET/CT and [(18)F]F-FDG PET/CT in patients with liver malignancies. Mol Imaging Biol. (2022) 24(5):818–29. doi: 10.1007/s11307-022-01732-2
17. Lindner T, Loktev A, Altmann A, Giesel F, Kratochwil C, Debus J, et al. Development of quinoline-based theranostic ligands for the targeting of fibroblast activation protein. J Nucl Med. (2018) 59(9):1415–22. doi: 10.2967/jnumed.118.210443
18. Loktev A, Lindner T, Mier W, Debus J, Altmann A, Jäger D, et al. A tumor-imaging method targeting cancer-associated fibroblasts. J Nucl Med. (2018) 59(9):1423–9. doi: 10.2967/jnumed.118.210435
19. Siveke JT. Fibroblast-Activating protein: targeting the roots of the tumor microenvironment. J Nucl Med. (2018) 59(9):1412–4. doi: 10.2967/jnumed.118.214361
20. Thompson SM, Suman G, Torbenson MS, Chen ZE, Jondal DE, Patra A, et al. PSMA As a theranostic target in hepatocellular carcinoma: immunohistochemistry and (68) Ga-PSMA-11 PET using cyclotron-produced (68) Ga. Hepatol Commun. (2022) 6(5):1172–85. doi: 10.1002/hep4.1861
21. Hotta M, Kim GHJ, Rerkpichaisuth V, Teng PY, Armstrong WR, Carlucci G, et al. Correlation of FAPI PET uptake with immunohistochemistry in explanted lungs from patients with advanced interstitial lung disease. J Nucl Med. (2024) 65(11):1789–94. doi: 10.2967/jnumed.124.268351
22. Kirienko M, Centonze G, Sabella G, Sollai M, Sollini M, Lan X, et al. FAP Expression in alpha cells of langherhans insulae-implications for FAPI radiopharmaceuticals’ use. Eur J Nucl Med Mol Imaging. (2023) 50(10):3042–9. doi: 10.1007/s00259-023-06246-9
23. Harris PA, Taylor R, Thielke R, Payne J, Gonzalez N, Conde JG. Research electronic data capture (REDCap)–a metadata-driven methodology and workflow process for providing translational research informatics support. J Biomed Inform. (2009) 42(2):377–81. doi: 10.1016/j.jbi.2008.08.010
24. Harris PA, Taylor R, Minor BL, Elliott V, Fernandez M, O'Neal L, et al. The REDCap consortium: building an international community of software platform partners. J Biomed Inform. (2019) 95:103208. doi: 10.1016/j.jbi.2019.103208
25. Al-Ibraheem A, Al-Qasem S, El Khaldi M, Amarin R. 68 Ga-FAPI PET/CT provides a clear picture of a klatskin tumor that 18 F-FDG PET/CT missed. Clin Nucl Med. (2023) 48(6):e313–e5. doi: 10.1097/RLU.0000000000004652
26. Guo W, Pang Y, Yao L, Zhao L, Fan C, Ke J, et al. Imaging fibroblast activation protein in liver cancer: a single-center post hoc retrospective analysis to compare [(68)Ga]Ga-FAPI-04 PET/CT versus MRI and [(18)F]-FDG PET/CT. Eur J Nucl Med Mol Imaging. (2021) 48(5):1604–17. doi: 10.1007/s00259-020-05095-0
27. Jinghua L, Kui X, Deliang G, Bo L, Qian Z, Haitao W, et al. Clinical prospective study of Gallium 68 ((68)Ga)-labeled fibroblast-activation protein inhibitor PET/CT in the diagnosis of biliary tract carcinoma. Eur J Nucl Med Mol Imaging. (2023) 50(7):2152–66. doi: 10.1007/s00259-023-06137-z
28. Novruzov E, Mori Y, Novruzov F. The diagnostic value of fibroblast activation protein imaging in hepatocellular carcinoma and cholangiocellular carcinoma. PET Clin. (2023) 18(3):309–14. doi: 10.1016/j.cpet.2023.02.011
29. Pabst KM, Trajkovic-Arsic M, Cheung PFY, Ballke S, Steiger K, Bartel T, et al. Superior tumor detection for (68)Ga-FAPI-46 versus (18)F-FDG PET/CT and conventional CT in patients with cholangiocarcinoma. J Nucl Med. (2023) 64(7):1049–55. doi: 10.2967/jnumed.122.265215
30. Rajaraman V, Meenakshi LA, Selvaraj AJ, Pottakkat B, Halanaik D. Role of 68Ga-FAPI PET/CT in assessing hepatobiliary malignancies: a prospective pilot study. Clin Nucl Med. (2023) 48(6):e281–e8. doi: 10.1097/RLU.0000000000004641
31. Siripongsatian D, Promteangtrong C, Kunawudhi A, Kiatkittikul P, Chotipanich C. (68)Ga-FAPI-46 PET/MR detects recurrent cholangiocarcinoma and intraductal papillary mucinous neoplasm in a patient showing increasing CEA with negative (18)F-FDG PET/CT and conventional CT. Nucl Med Mol Imaging. (2021) 55(5):257–60. doi: 10.1007/s13139-021-00712-8
32. Sun Y, Cai X, Wang Z, Sun Y, Cheng J. Elevated 18 F-AIF-FAPI-04 uptake in hepatic hemangioma. Clin Nucl Med. (2024) 49(1):e25–e7. doi: 10.1097/RLU.0000000000004965
33. Veldhuijzen van Zanten SEM, Pieterman KJ, Wijnhoven BPL, Pruis IJ, Groot Koerkamp B, van Driel L, et al. FAPI PET versus FDG PET, CT or MRI for staging pancreatic-, gastric- and cholangiocarcinoma: systematic review and head-to-head comparisons of diagnostic performances. Diagnostics. (2022) 12(8):1958. doi: 10.3390/diagnostics12081958
34. Fingas CD, Bronk SF, Werneburg NW, Mott JL, Cazanave S, Sirica AE, et al. Myofibroblast-Derived PDGF-BB promotes hedgehog signaling-dependent resistance to TRAIL cytotoxicity in cholangiocarcinoma cells. Gastroenterology. (2011) 140(5):S909-S. doi: 10.1016/S0016-5085(11)63773-6
35. Lin Y, Li B, Yang X, Cai Q, Liu W, Tian M, et al. Fibroblastic FAP promotes intrahepatic cholangiocarcinoma growth via MDSCs recruitment. Neoplasia. (2019) 21(12):1133–42. doi: 10.1016/j.neo.2019.10.005
36. Puré E, Blomberg R. Pro-tumorigenic roles of fibroblast activation protein in cancer: back to the basics. Oncogene. (2018) 37(32):4343–57. doi: 10.1038/s41388-018-0275-3
37. Sirica AE, Campbell DJ, DeWitt JL. Organotypic cell culture modeling of desmoplastic cholangiocarcinoma progression. Hepatology. (2012) 56:616a-a. doi: 10.1111/j.1872-034X.2012.01026.x
38. Sirica AE, Gores GJ. Desmoplastic stroma and cholangiocarcinoma: clinical implications and therapeutic targeting. Hepatology. (2014) 59(6):2397–402. doi: 10.1002/hep.26762
39. Chen H, Zhao L, Ruan D, Pang Y, Hao B, Dai Y, et al. Usefulness of [(68)Ga]Ga-DOTA-FAPI-04 PET/CT in patients presenting with inconclusive [(18)F]FDG PET/CT findings. Eur J Nucl Med Mol Imaging. (2021) 48(1):73–86. doi: 10.1007/s00259-020-04940-6
40. Karbhari A, Mosessian S, Trivedi KH, Valla F Jr., Jacobson M, Truty MJ, et al. Gallium-68-labeled fibroblast activation protein inhibitor-46 PET in patients with resectable or borderline resectable pancreatic ductal adenocarcinoma: a phase 2, multicenter, single arm, open label non-randomized study protocol. PLoS One. (2023) 18(11):e0294564. doi: 10.1371/journal.pone.0294564
41. Loktev A, Lindner T, Burger EM, Altmann A, Giesel F, Kratochwil C, et al. Development of fibroblast activation protein-targeted radiotracers with improved tumor retention. J Nucl Med. (2019) 60(10):1421–9. doi: 10.2967/jnumed.118.224469
42. Lindner T, Altmann A, Krämer S, Kleist C, Loktev A, Kratochwil C, et al. Design and development of (99m)Tc-Labeled FAPI tracers for SPECT imaging and (188)Re therapy. J Nucl Med. (2020) 61(10):1507–13. doi: 10.2967/jnumed.119.239731
43. Ronot M, Clift AK, Vilgrain V, Frilling A. Functional imaging in liver tumours. J Hepatol. (2016) 65(5):1017–30. doi: 10.1016/j.jhep.2016.06.024
44. Anderson CD, Rice MH, Pinson CW, Chapman WC, Chari RS, Delbeke D. Fluorodeoxyglucose PET imaging in the evaluation of gallbladder carcinoma and cholangiocarcinoma. J Gastrointest Surg. (2004) 8(1):90–7. doi: 10.1016/j.gassur.2003.10.003
45. Kim YJ, Yun M, Lee WJ, Kim KS, Lee JD. Usefulness of 18F-FDG PET in intrahepatic cholangiocarcinoma. Eur J Nucl Med Mol Imaging. (2003) 30(11):1467–72. doi: 10.1007/s00259-003-1297-8
46. Du J, Lv X, Zhang Z, Huang Z, Zhang E. Revisiting targeted therapy and immunotherapy for advanced cholangiocarcinoma. Front Immunol. (2023) 14:1142690. doi: 10.3389/fimmu.2023.1142690
Keywords: cholangiocarcinoma (CCA), fibroblast activation protein (FAP), liver neoplasms, precision medicine, theranostics
Citation: Jorgenson LC, Torbenson MS, Halfdanarson TR, Kankeu Fonkoua LA, Tran NH, Roberts LR, Smoot RL, Goenka AH and Thompson SM (2024) Immunohistochemical basis for FAP as a candidate theranostic target across a broad range of cholangiocarcinoma subtypes. Front. Nucl. Med. 4:1480471. doi: 10.3389/fnume.2024.1480471
Received: 14 August 2024; Accepted: 14 November 2024;
Published: 27 November 2024.
Edited by:
Miao Mingsan, National Research Council (CNR), ItalyReviewed by:
Linqi Zhang, Guangzhou Medical University Cancer Hospital, ChinaAmeya Puranik, Tata Memorial Hospital, India
Copyright: © 2024 Jorgenson, Torbenson, Halfdanarson, Kankeu Fonkoua, Tran, Roberts, Smoot, Goenka and Thompson. This is an open-access article distributed under the terms of the Creative Commons Attribution License (CC BY). The use, distribution or reproduction in other forums is permitted, provided the original author(s) and the copyright owner(s) are credited and that the original publication in this journal is cited, in accordance with accepted academic practice. No use, distribution or reproduction is permitted which does not comply with these terms.
*Correspondence: Scott M. Thompson, dGhvbXBzb24uc2NvdHRAbWF5by5lZHU=