- 1Karlsruhe Institute of Technology, Institute for Nuclear Waste Disposal, Karlsruhe, Germany
- 2Svensk Kärnbränslehantering AB, Solna, Sweden
The uptake of 63Ni(II), 152Eu(III) and 242Pu(III/IV) by hardened cement paste (HCP, CEM I) in the degradation stage II (pH ≈ 12.5 [Ca] ≈ 0.02 M) was investigated in the absence and presence of α-hydroxyisobutyric, 3-hydroxybutyric and glutaric acids. These organic ligands were previously identified as proxies for the degradation products of UP2W (a polyacrylonitrile-based material used as filter aid in nuclear power plants) under repository conditions. Sorption experiments were conducted with various ligand concentrations (10−4 M ≤ [L]tot ≤ 0.1 M) and solid-to-liquid ratios (0.5 g⋅dm–3 ≤ S:L ≤ 20 g⋅dm–3). Redox conditions in the Pu systems were buffered with either hydroquinone (HQ, pe + pH ≈ 10) or Sn(II) (pe + pH ≈ 2). Strong sorption is observed for 152Eu(III) and 242Pu(III/IV) in the absence of proxy ligands, with distribution coefficients (log Rd ≈ 2.2–4, with Rd in m3⋅kg–1) in line with data reported in the literature. No differences are observed for sorption experiments with Pu in HQ and Sn(II) systems. Lower Rd values are determined for 63Ni(II) (log
1 Introduction
UP2W is a polyacrylonitrile-based polymer (PAN) used as filter aid in nuclear power plants (Dario et al., 2004; Keith-Roach et al., 2021). Significant amounts of used UP2W are disposed of in SFR, the repository for short-lived low and intermediate level wastes (L/ILW) in Sweden (Dario et al., 2004; Duro et al., 2012; Keith-Roach et al., 2014; Keith-Roach et al., 2021).
Cement is extensively used in L/ILW repositories for the conditioning of the waste and for construction purposes. The porewater in cementitious materials is characterized by hyperalkaline pH conditions (10 ≤ pH ≤ 13.3) and Ca concentrations ranging from ∼10−4 M to ∼0.02 M (Taylor, 1997; Hewlett and Liska, 2019). Cementitious materials, in particular calcium silicate hydrate (C-S-H) phases, are known to sorb radionuclides strongly, primarily metal ions and cationic species (Wieland, 2014; Ochs et al., 2016).
In the context of an on-going collaboration between Karlsruhe Institute for Technology—Institute for Nuclear Waste Disposal (KIT-INE) and the Swedish Nuclear Fuel and Waste Management Co. (SKB), and within Work Package 3 CORI of the EURAD EU project (CORI-SOTA, 2021), the organic compounds glutaric acid (GTA), α-hydroxyisobutyric acid (HIBA) and 3-hydroxybutyric acid (HBA) were proposed as proxies for the degradation products of UP2W (Figure 1). The structure of these proxy ligands is consistent with 13C-NMR data obtained for the degradation leachates of UP2W under hyperalkaline conditions (Tasi et al., 2021b). Considering the conversion of nitrile into carboxylate groups in the alkaline hydrolysis of PAN (Ermakov et al., 2000; Litmanovich and Plate, 2000), GTA represents the bulk chain of the PAN fragments, whereas HIBA and HBA correspond to the possible end-groups forming in the course of the degradation process.

FIGURE 1. Structural formulae of the proxy ligands investigated in this work: α-hydroxyisobutyric acid (HIBA, left), 3-hydroxybutyric acid (HBA, centre) and glutaric acid (GTA, right).
The presence of organic ligands disposed with the waste in L/ILW or generated through the degradation of more complex organic materials may result in the formation of stable complexes with radionuclides, eventually affecting the retention properties of the latter in repository systems. Previous studies have investigated the impact of the UP2W degradation products on the sorption of radionuclides in cementitious systems (Dario et al., 2004; Holgersson et al., 2011; Duro et al., 2012). These studies focused on the leachates resulting from the degradation of UP2W material, and did not attempt to assess the effect of individual degradation products on the uptake of radionuclides by cement. Isosaccharinic (ISA) and gluconic (GLU) acids are polyhydroxycarboxylic acids expected in L/ILW repositories as main degradation product of cellulose and cement additive, respectively. They share some structural similarities with HIBA and HBA, with alcohol groups in α- and β-positions with respect to the carboxylic group. Several studies in the literature highlight the strong complexes formed by ISA and GLU with radionuclides, including Ni(II), Ln(III) and An(III/IV/V/VI), among others (Vercammen et al., 2001; Tits et al., 2005; Gaona et al., 2008; Rojo et al., 2013; Rai and Kitamura, 2017; González-Siso et al., 2018; Tasi et al., 2018a; Tasi et al., 2018b; Rojo et al., 2021). The formation of these complexes can potentially affect the retention of radionuclides in cement-based repositories (Wieland and Van Loon, 2003; Wieland, 2014; Tasi et al., 2021a).
In this context, this study aims at investigating the uptake of 63Ni(II), 152Eu(III) and 242Pu(III/IV) by HCP in the absence and presence of the proxy ligands HIBA, HBA and GTA. 59,63Ni and 239Pu are important contributors to the radiotoxicity of the wastes in SFR, whereas Eu(III) is often considered as inactive analogue of Am(III) and Pu(III). Experiments with plutonium are conducted under mildly and strongly reducing conditions (pe + pH ≈ 10 and 2, respectively) to capture the possible interplay between Pu(IV) and Pu(III) oxidation states in the redox boundary conditions expected in SFR (Duro et al., 2014). The Pu(IV) system in HQ-buffered solutions provides also insight on the behaviour of other tetravalent actinides expected in SFR, e.g., U(IV). The results obtained for the sorption of these radionuclides in the presence of proxy ligands are compared with analogous studies in the presence of ISA, which shares some structural similarities with HIBA and HBA. The present work complements and further expands the experimental studies by Szabo et al. dedicated to the proxy ligands of UP2W, i.e., evaluating their effect on the solubility of selected radionuclides (Szabo et al., 2022b) and assessing their uptake by HCP (Szabo et al., 2022a).
2 Experimental
2.1 Chemicals and radiotracers
All experiments were conducted at T = (22 ± 2) °C in Ar gloveboxes with O2 < 2 ppm. Solutions were prepared with purified water (Millipore Milli-Q Advantage A10 (18.2 MΩ·cm at 22°C, 4 ppb TOC) with Millipore Millipak® 40 0.22 µm), purged with Ar for >1 h to remove traces of O2 and CO2.
Glutaric acid (C5H8O4, 99%, solid), α-hydroxyisobutyric acid (C4H8O3, 99%, solid), 3-hydroxybutyric acid (C4H8O3, 99%, liquid), portlandite (Ca(OH)2 (cr)) and tin (II) chloride (SnCl2, p.a) were purchased from Sigma-Aldrich. NaOH, HCl (both Titrisol), hydroquinone (C6H6O2, HQ, p.a.), concentrated HNO3 (Supra- and Ultrapure) were obtained from Merck.
63Ni and 152Eu radiotracers were purchased from Eckert and Ziegler, with total activities of 100 μCi/3.7 MBq in 1 mL of 0.5 and 0.1 M HCl, respectively. Although both radiotracers were commercialized as “carrier-free”, the concentrations of stable Ni in the 63Ni stock solution and stable Eu in the 152Eu stock solutions were quantified as (3.0 ± 0.3)∙10−5 M and (1.1 ± 0.1)∙10−5 M. The isotopic composition of the Pu(VI) stock used in this work was 99.4 wt% 242Pu, 0.58 wt% 239Pu, 0.005 wt% 238Pu and 0.005 wt% 241Pu, as characterized in a previous study (Altmaier et al., 2008). The original Pu(VI) stock solution ([Pu]tot = 5.63·10−2 M) in 3 M HClO4 was diluted to [Pu]tot = 2.64·10−6 M in 0.2 M HCl. The latter solution was used for the preparation of the sorption samples containing plutonium. Pu(IV) and eventually Pu(III) are expected to prevail in the reducing conditions of the experiments (see Tasi et al., 2018a), but the choice of the +VI oxidation state for the spike of Pu aimed at avoiding high initial oversaturation conditions that may result in the formation of Pu(IV) colloids (see Tasi et al., 2021a).
2.2 Cement and cement porewater
The hardened cement paste used in this work was provided by SKB as a monolith, which was prepared from a mixture of Swedish structural Portland cement for civil engineering (CEM I 42.5N BV/SR/LA) and deionized water at a water-to-cement weight ratio of 0.5. In a previous study (Tasi et al., 2021a), the HCP monolith was milled, sieved to a particle size of <100 μm, extensively characterized and stored under Ar atmosphere until use in the sorption experiments. Tasi and co-workers reported a (Brunauer–Emmett–Teller, BET) surface area of 79.2 m2⋅g−1 for the HCP powder material used in the present study.
The cement porewater was prepared using the protocol described in Tasi et al. (2021a). The HCP was weighted in a 2 L Kautex flask and contacted with Milli-Q water to reach a solid-to-liquid ratio (S:L) of 25 g·dm−3. After a contact time of 1 week, the initial leachate was separated by decantation from the HCP, and the solid material was equilibrated again with fresh Milli-Q water during one additional week. The same HCP material and cement pore water were used in the solubility and sorption studies with proxy ligands for UP2W degradation products (Szabo et al., 2022a; Szabo et al., 2022b). The composition of the cement porewater is buffered by portlandite at pH ≈ 12.5 and [Ca] ≈ 0.02 M, and is characterized by a low content of Na and K (≤3⋅10−4 M). A detailed description of the impact of the proxy ligands investigated in this work on the HCP is provided in our previous study dedicated to this binary system (Szabo et al., 2022a).
2.3 pH and Eh measurements
The pH was measured using a ROSS combination pH electrode (Thermo Scientific, OrionTM) calibrated against standard buffer solutions (pH = 2 to 12, Merck).
Redox potentials of plutonium systems containing either HQ or Sn(II) were measured using Pt combination electrodes (Metrohm) with Ag/AgCl as a reference system. Measurements were performed following the protocol described in the literature (Altmaier et al., 2010). The measured potentials were converted to Eh values (referred to the standard hydrogen electrode, SHE) by correcting for the potential of the Ag/AgCl reference in 3.0 M KCl at T = 22°C, i.e. +207 mV. Eh values were converted to pe (with pe = −log ae−) as Eh = −RT ln (10) F−1 log ae−, where R is the ideal gas constant (8.3144 J⋅mol−1⋅K−1) and F is the Faraday constant (96,485.3 C mol−1). Uncertainties of pH and Eh measurements (calculated as 2σ of averaged measurements) ranged between ±0.05/±0.10 and ±15/± 40 mV, respectively.
2.4 Sorption experiments with 63Ni, 152Eu and 242Pu in the absence and presence of proxy ligands
All batch sorption samples were prepared using the HCP and cement porewater described in Section 2.2. Stock solutions of the proxy ligands were titrated with 1.0 M NaOH to pH ≈ 12.5 in order to avoid any impact on the pH of the cement porewater.
Suspensions of HCP and cement porewater (10 mL) at the targeted S:L ratio were equilibrated for 2 days before the addition of the corresponding radionuclide. In the experiments with plutonium, the HCP was mixed with cement porewater containing 2 mM of either HQ or Sn(II), and the suspension was equilibrated for 2 days, after which pH and Eh were determined as described in Section 2.3. Small volumes (14–351 μL) of the diluted radionuclide stock solutions were pipetted into these systems, aiming at log[63Ni(II)]0 = −8/−9, log[152Eu(III)]0 = −8/−8.67 and log[242Pu(IV)]0 ≈ −8. After the addition of the corresponding radionuclides, the values of pH, Eh (only in the case of Pu) and total radionuclide concentration [RN]aq, in the aqueous phase were monitored for ≤4 months [RN]aq was measured after phase separation, i.e., ultrafiltration with 10 kD filters at 3,400–3,900 g (Nanosep®, Pall Life Sciences; pore size ≈ 2-3 nm) (Ni, Eu, Pu) or ultracentrifugation (694,000 g, 1 h; Beckman XL-90, rotor type 90Ti) (Eu). After each sampling, the volume of the original sample was restored with cement pore water. The aliquots obtained after phase separation were diluted with 2% HNO3 (Suprapur). In the samples containing nickel, the acidified aliquot was mixed with 10 mL of liquid scintillation counting (LSC) cocktail (Ultima Gold XR, Perkin Elmer) and measured for 30 min in a LSC Tri-Carb 3110 TR (Perkin Elmer) equipment considering an energy window between 0 and 100 keV. Detection limits achieved with this counting time and sample characteristics were ≈6⋅10−12 M, calculated as three times the standard deviation of blank solutions. The concentration of Eu was determined by gamma counting (Wizard2 10—Detector Gamma Counter, Perkin Elmer) for an energy window between 15 and 2000 keV and a counting time of 30 min. Detection limits calculated for these conditions were ≈10−13 M. Pu was quantified by sector field inductively coupled plasma mass spectrometry (SF-ICP-MS; type Element XR, Thermo Fisher), with a detection limit of ≈10−13 M in the conditions of the present study. The solutions in the HCP-Ni system were additionally quantified for total nickel concentration by ICP-MS.
The same sequence was used in the preparation of the sorption samples in the ternary systems HCP-RN-proxy ligands. Two days after the addition of the corresponding radionuclide, the suspensions were spiked with appropriate volumes of GTA, HIBA and HBA stock solutions (0.2, 5·10−2 and 5·10−3 M) targeting the range of initial ligand concentrations −4 ≤ log([L]0/M) ≤ −1. Note that the sorption of the proxy ligands on HCP at the S:L ratio used in the ternary system HCP-RN-proxy ligands is very minor (Szabo et al., 2022a), and thus [L]0 is considered equal to the concentration of the ligand in equilibrium conditions [L]aq. After the addition of the proxy ligands, the values of pH, Eh (only in the case of Pu) and [RN]aq were monitored for ≤3 months. The sampling procedure was the same as described above for the binary system HCP-RN. After each sampling, the volume of the original sample was restored with cement pore water containing the corresponding proxy ligand and ligand concentration. The sorption of Ni(II) and Eu(III) on the walls of the vessels was evaluated after terminating the sorption experiments. Hence, the suspension remaining in the vessel was discarded, the vessel was washed three times with MQ-water, filled in with 2% HNO3 and left overnight. The concentration of Ni and Eu in the resulting solution was quantified by LSC and gamma counting, respectively. From these results, the fraction of 63Ni and 152Eu sorbed on the vessel’s walls was determined as 30% and 4%, respectively. Distribution ratios determined in this work are corrected accordingly. A negligible sorption of Pu on the vessel’s walls under analogous experimental conditions was previously reported by Tasi et al. (2021a). The main experimental conditions considered in this work for the binary HCP-RN and ternary HCP-RN-proxy ligands systems are summarized in Table 1.
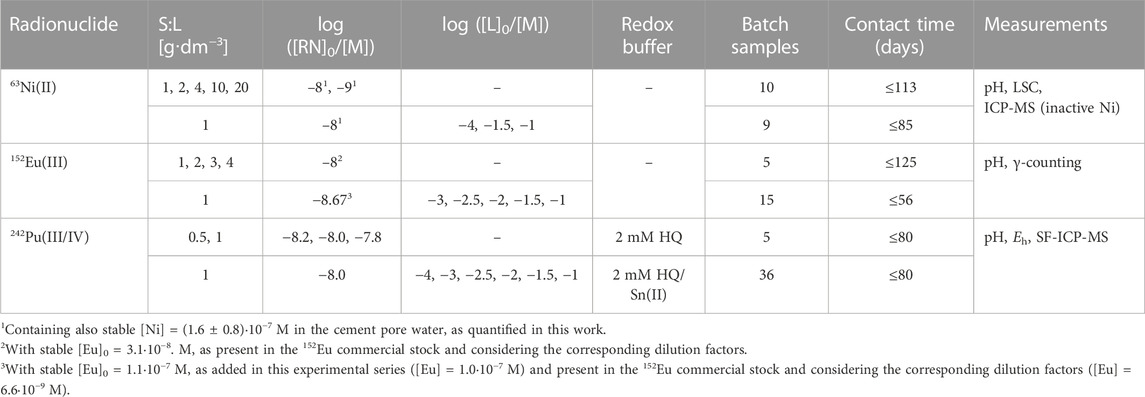
TABLE 1. Summary of the experimental conditions considered for the sorption studies with the binary and ternary systems HCP-RN and HCP-RN-proxy ligands, with L = HIBA, HBA and GTA.
Sorption was evaluated in terms of distribution ratios, Rd (in m3∙kg−1), calculated as the ratio of radionuclide concentration in the solid ([RN]solid, in mol∙kg−1) and aqueous ([RN]aq, in M) phases:
where [M]0 is the initial radionuclide concentration in the aqueous phase (M), V is the volume of sample (m3) and m is the mass of HCP (kg).
3 Results and discussion
3.1 Uptake of Ni(II) by HCP in the absence and presence of proxy ligands
Figure 2 shows the uptake of 63Ni(II) by HCP in the absence of proxy ligands, as determined in this work for the degradation stage II of cement (pH ≈ 12.5). The figure shows also sorption data reported by Wieland and co-workers for the sorption of 63Ni(II) by HCP in the degradation stage I (pH = 13.3), both in the absence and presence of added stable Ni(II) (Wieland et al., 2000; Wieland and Van Loon, 2003; Wieland et al., 2006).
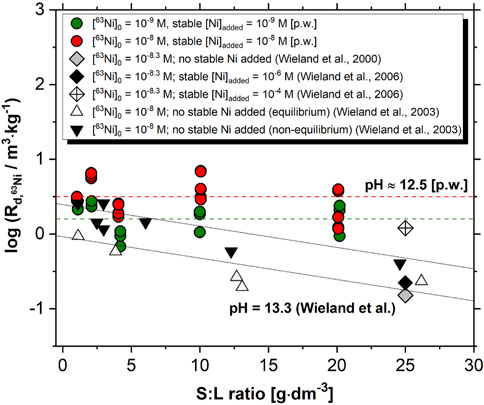
FIGURE 2. Uptake of 63Ni(II) by HCP in the absence of proxy ligands as determined in this present work (p.w., red and green, pH ≈ 12.5) or reported by Wieland and co-workers (triangles and inverted triangles) (Wieland et al., 2000; Wieland and Van Loon, 2003; Wieland et al., 2006). Dashed and solid lines show the trend of Rd values with increasing S:L ratios as determined in this work and reported in Wieland and Van Loon, (2003), respectively.
Rd values determined in this work remain mostly constant within the investigated range of S:L ratios (1–20 g⋅dm–3). Slight differences in the quantified Rd values are identified between sorption experiments with [63Ni]0 = 10−8 and 10−9 M, i.e. (3.6 ± 1.8) m3⋅kg–1 and (1.8 ± 0.7) m3⋅kg–1, although both values agree within the respective uncertainties. In contrast to this, Wieland and co-workers observed a systematic decrease of the measured Rd values with increasing S:L ratio (see Figure 2). The authors proposed isotopic exchange with stable nickel present in pristine HCP as main mechanism for the uptake of 63Ni, and used the partition coefficient (α) to describe the isotopic distribution of 63Ni between the HCP and the cement pore water:
where
Wieland et al. reported α = (3.3 ± 1.7)⋅10−2 (absence of added stable Ni), α = (2.9 ± 0.6)⋅10−2 (with stable [Ni]added = 10−6 M) and α = (4.5 ± 1.0)⋅10−2 (with stable [Ni]added = 10−4 M) for the uptake of 63Ni by HCP (in the degradation stage I) (Wieland et al., 2006). On the basis of these results, Wieland and co-workers argued that only a small fraction of stable Ni present in pristine HCP is available for isotopic exchange with 63Ni.
Measurements of stable Ni in the HCP and corresponding pore water used in this work resulted in (3.7 ± 1.0)⋅10−4 mol⋅kg–1 and (1.6 ± 0.8)⋅10−7 M, 1 respectively, which allow the calculation of
The concentration of stable Ni in the cement porewater is by a factor of 10 or 100, respectively, higher than the added 63Ni. At the relatively high stable Ni concentration which is most likely determined by solubility of a Ni-bearing solid phase (LDH phases or Ni(OH)2 (am)) we can expect that Ni species are not only abundant in a solid phase and as dissolved Ni(OH)2(aq), but also as surface sorbed onto or incorporated into cement phases such as e.g., C-S-H. Spiked 63Ni will undergo the same reactions as stable Ni so that isotope exchange is assumed to be the dominant retention mechanism under our experimental conditions. Note further that the partition coefficients measured in this work are consistent (within the uncertainties) with α = 1, which suggests the full availability of stable Ni in pristine HCP (in the degradation stage II) for isotopic exchange with 63Ni.
Figure 3 shows the effect of proxy ligands (with 10−4 M ≤ [L]tot ≤ 0.1 M) on the uptake of 63Ni by HCP in degradation stage II. The figure includes also data reported by Bruno and co-workers for the uptake of 63Ni under the same conditions but in the presence of ISA (Bruno et al., 2018). HIBA, HBA and GTA have a minor impact on the retention of 63Ni(II) by HCP, and a slight decrease in the
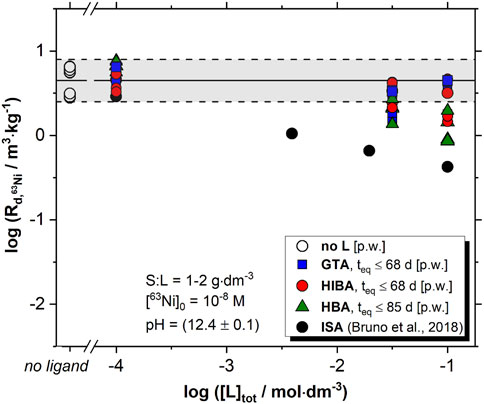
FIGURE 3. Uptake of 63Ni(II) by HCP under increasing concentrations of proxy ligands, with 10−4 M ≤ [L]tot ≤ 0.1 M and S:L = 1 g⋅dm−3. White circles and grey-shaded area correspond to the uptake of 63Ni(II) by HCP in the absence of proxy ligands, as reported in Figure 2 for S:L = 1-2 g⋅dm−3. Black symbols show the effect of ISA on the uptake of 63Ni(II) by HCP, as reported by Bruno et al. (2018). All data correspond to degradation stage II of cement, with pH = (12.4 ± 0.1). [p.w.] stands for present work.
3.2 Uptake of Eu(III) by HCP in the absence and presence of proxy ligands
Figure 4 shows the uptake of 152Eu(III) by HCP in degradation stage II, both in the absence and presence of increasing concentrations of proxy ligands. For comparative purposes, the figure shows also the sorption of Eu(III) by HCP in degradation stage I in the absence and presence of ISA and GLU, as reported by Wieland and co-workers (Wieland et al., 1998; Wieland and Van Loon, 2003; Wieland, 2014).
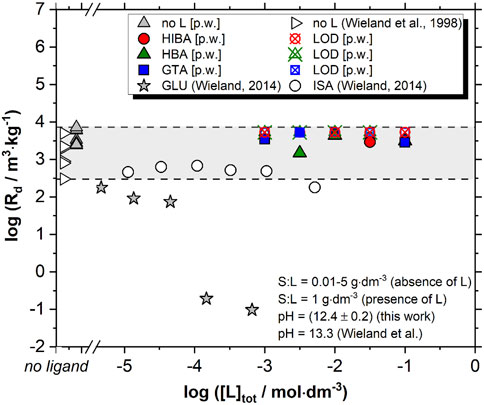
FIGURE 4. Uptake of 152Eu(III) by HCP in the absence and presence of proxy ligands (with 10−3 M ≤ [L]tot ≤ 0.1 M) as determined in this work. Data reported by Wieland and co-workers for the uptake of 152Eu(III) by HCP (degradation stage I) in the absence and presence of ISA and GLU provided for comparison (Wieland et al., 1998; Wieland, 2014). Grey-shaded area covers the range of Rd values determined in this work (S:L = 1–4 g⋅dm−3) or reported by Wieland and co-workers (S:L = 0.01–0.1 g⋅dm−3) in the absence of organic ligands. Experiments in the presence of organic ligands were conducted at S:L = 1 g⋅dm−3. LOD and [p.w.] stand for limit of detection and present work, respectively.
A very strong sorption is observed in the absence of proxy ligands, with Rd values ranging from ≈3⋅103 m3⋅kg−1 and ≈1⋅104 m3⋅kg−1 at S:L = 1–4 g⋅dm−3. These values are slightly higher but in line with sorption studies available in the literature. Wieland and co-workers reported Rd values ≥ 103 m3⋅kg−1 at S:L = 0.01 g⋅dm−3 and > 102 m3⋅kg−1 at S:L = 0.1 g⋅dm−3 (Wieland et al., 1998; Wieland and Van Loon, 2003). Our results are also consistent with the uptake observed for Eu(III) in the presence of C-S-H phases (Tits et al., 2000; Pointeau et al., 2001), thus strongly supporting that the latter phases are the main sink of Eu(III) (as well as An (III)) in cementitious systems. The uptake mechanism investigated by time-resolved laser fluorescence spectroscopy and X-ray absorption spectroscopy for Eu(III), Nd(III) and Cm(III) supports a fast adsorption with the formation of surface complexes, followed by the slower incorporation of the trivalent lanthanide or actinide in the C-S-H structure (Pointeau et al., 2001; Tits et al., 2003; Stumpf et al., 2004; Mandaliev et al., 2010a; Mandaliev et al., 2010b). Note that stable Eu(III) is also present in HCP (quantified as 2.7⋅10−6 mol⋅kg−1 in the CEM I material used in this work), although the inventory is significantly lower than in the case of stable Ni(II) (3.7⋅10−4 mol⋅kg−1) and has a negligible effect in the retention of 152Eu(III) in the current laboratory study.2
The uptake of Eu(III) by HCP is not affected by the presence of the proxy ligands up to ligand concentrations of [L]tot = 0.1 M, which is consistent with solubility data previously reported for Nd(OH)3(s) in the presence of the same proxy ligands (Szabo et al., 2022b). Although these ligands are known to form stable complexes with Ln(III)/An(III) in acidic to near-neutral pH conditions (Deelstra and Verbeek, 1964; Wang et al., 2000; Giroux et al., 2002), they cannot compete against hydrolysis in hyperalkaline systems or against the strong sorption occurring in HCP systems. This observation is further supported by thermodynamic calculations conducted by Keith-Roach and Shahkarami (Keith-Roach and Shahkarami, 2021). In contrast to the proxy ligands investigated in this work, the uptake of Eu(III) is strongly decreased in the presence of GLU and, to a lesser extent, ISA (Wieland, 2014) (see Figure 4). Even though HIBA and HBA contain a carboxylic group with an alcohol group in α- or β-position, this configuration is insufficient to form stable complexes with Eu(III) in hyperalkaline systems. As discussed in Szabo et al. (2022a), a greater number of alcohol groups (as occurs in ISA or GLU) is required to form stable chelates in hyperalkaline conditions and thus affect aqueous complexation and sorption phenomena.
3.3 Uptake of Pu(III/IV) by HCP in the absence and presence of proxy ligands
The measurements of pH and Eh in the Pu systems containing HQ and Sn(II) resulted in (pe + pH) = (10.0 ± 0.5) and (2 ± 1), respectively. These values are consistent with previous studies available in the literature using the same reducing chemicals (Tasi et al., 2018a; Tasi et al., 2018c). Considering the redox distribution of plutonium in alkaline reducing conditions, Pu(IV) is expected to prevail in the aqueous phase of HQ-buffered systems, whereas both Pu(III) and Pu(IV) may coexist in the strongly reducing conditions imposed by Sn(II) (Fellhauer, 2013; Tasi et al., 2018c; Grenthe et al., 2020). Figures 5A, B (HQ-system) and (Sn(II)-system) show the uptake of Pu (in terms of log Rd values) by HCP in the degradation stage II, both in the absence and presence of increasing concentrations of proxy ligands. The figure includes also sorption data reported for Pu in the presence of ISA under analogous experimental conditions (Tasi et al., 2021a).
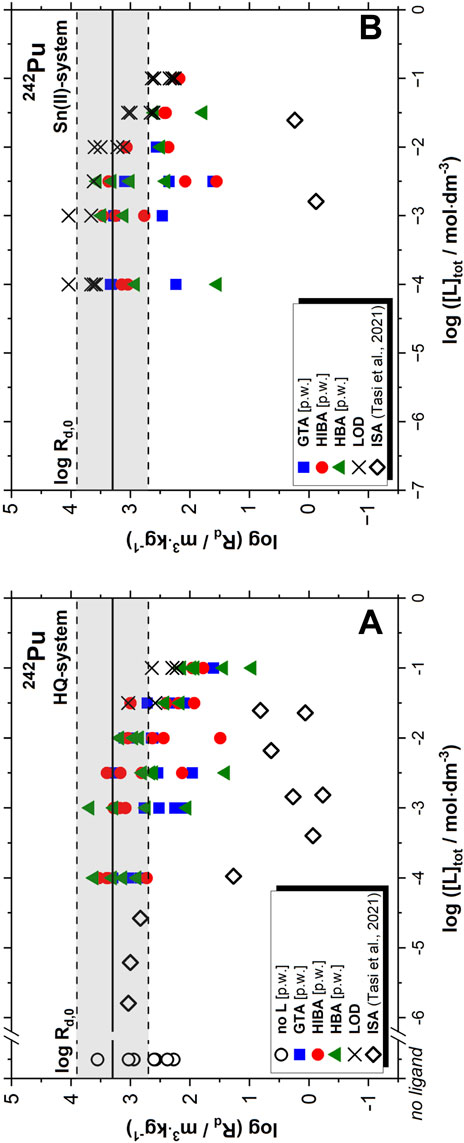
FIGURE 5. Uptake of 242Pu(IV/III) by HCP (degradation stage II) in the absence and presence of proxy ligands (with 10−3 M ≤ [L]tot ≤ 0.1 M), with L = GTA, HIBA and HBA, in HQ (A) and Sn(II) (B) systems. Data reported by Tasi et al. for the uptake of 242Pu(IV) by HCP (degradation stage II) in the presence of ISA provided for comparison (Tasi et al., 2021a). Experiments in the absence of organic ligands were conducted at S:L = 0.5–2 g⋅dm−3 (this work). The grey-shaded area indicates the range of log Rd values reported by Tasi and co-workers in the absence of organic ligands at S:L = 1 g⋅dm−3 (Tasi et al., 2021a). Experiments in the presence of organic ligands were conducted at S:L = 1 g⋅dm−3, both in this work and in (Tasi et al., 2021a). LOD and [p.w.] stand for limit of detection and present work, respectively.
Sorption data in the investigated Pu samples are characterized by a large dispersion. This is a common feature of An(IV) systems in pH-conditions where the neutral species An(OH)4(aq) prevails, and it is caused by difficulties in the phase separation and the possible presence of intrinsic colloids. A very strong sorption of Pu is observed in HQ-systems and absence of proxy ligands, with log Rd values ranging from 2.2 to 3.5 (with Rd in m3∙kg−1). These results are consistent with sorption data reported by Tasi and co-workers under analogous experimental conditions (Tasi et al., 2021a). The reference book by Ochs et al. recommends a log Rd of 1.5 for the uptake of Pu(IV) by HCP in the degradation stage II, with log Rd = 3 defined as upper limit (Ochs et al., 2016), also in line with our experimental observations.
Strong sorption is observed also in the presence of proxy ligands, with analogous log Rd values determined in HQ- and Sn(II)-systems. Considering the strong sorption described also in Section 3.2 for Eu(III), the experimental observations for Pu in Sn(II)-systems do not allow the unequivocal assignment of the oxidation state prevailing under these very reducing conditions. A slight decrease in the log Rd values can be speculated at [L]tot > 10−2 M, especially in the case of HQ-systems. However, this observation is uncertain due to the large dispersion of the data, and by the increase of the detection limit with increasing ligand concentration caused by the steady increase of the dilution factors required for ICP-MS. In spite of this, Rd values remain high in all the investigated systems containing proxy ligands, i.e. log Rd > 1. The weak impact of the proxy ligands on the retention of Pu by HCP in degradation stage II is in line with the results of solubility experiments in the presence of analogous concentrations of proxy ligands, in which the solubility of PuO2(ncr, hyd) remains unaffected up to [L]tot = 0.1 M, both in HQ- and Sn(II)-buffered systems (Szabo et al., 2022b). Although HIBA, HBA and GTA are known to form stable binary complexes with An (III/IV) under acidic conditions, these complexes can neither outcompete hydrolysis nor the strong sorption on HCP under the hyperalkaline pH conditions characteristic of the degradation stage II of cement.
In contrast to the proxy ligands, Figure 5 shows the remarkable impact that ISA on the uptake of Pu, which results in a decrease of up to 3 orders of magnitude in the Rd values (Tasi et al., 2021a). This is attributed to the strong ternary and quaternary complexes (Ca-)Pu-OH-ISA forming in hyperalkaline conditions (Tasi et al., 2018a; Tasi et al., 2018b). Moreover, distinct trends are observed at high ISA concentrations, involving the formation of stable surface complexes than increase sorption. Tasi and co-workers explained this observation with the strong uptake of ISA by cement, which modifies the surface properties at [ISA]tot ≥ 10−3 M. This again highlights the significantly different behaviour of proxy ligands and ISA, not only with respect to the interaction with radionuclides but also concerning the interaction with cement surfaces. Similar observations have been reported for the uptake of Pu(IV) and Th(IV) by HCP and C-S-H phases in the presence gluconate, a polyhydroxocarboxylic acid analogous to ISA (Moreton, 1993; Moreton et al., 2000; Wieland, 2014; Guidone et al., 2022).
4 Summary and conclusion
A comprehensive sorption study was conducted with the aim of quantitatively assessing the impact of α-hydroxyisobutyric acid, 3-hydroxybutyric acid and glutaric acid on the retention of Ni(II), Eu(III) and Pu(III/IV) by HCP in degradation stage II of cement. These low molecular weight organic ligands were identified within Work Package 3 CORI of the EURAD EU project as possible degradation products of UP2W, a polyacrylonitrile-based filter aid used in nuclear power plants.
Sorption experiments conducted in the absence of proxy ligands are consistent with literature data, with log
The retention of 63Ni(II) and 242Pu(III/IV) is slightly decreased in the presence of proxy ligands at [L]tot > 10−2 M. The effect is less evident for Pu due to the dispersion of the data and to the increased detection limits with increasing ligand concentration. On the contrary, the uptake of 152Eu(III) is not affected by the presence of proxy ligands up to concentrations of [L]tot = 0.1 M. These results are consistent with solubility studies conducted with β-Ni(OH)2(cr), Nd(OH)3(s) and PuO2(hyd, ncr) under analogous conditions and in the presence of proxy ligands. Although HIBA and HBA share some functional groups with ISA and GLU, the investigated proxy ligands show a minor capacity for the mobilization of radionuclides in cementitious systems. These observations support that mono- and dicarboxylic acids can hardly outcompete the hydrolysis and sorption of Ni(II), Eu(III) and Pu(III/IV) in cement systems in the degradation stage II, except if several alcohol groups are also present in the organic ligand. With the aim to approaching systems of even higher complexity, the last step of this project will target the impact of the complex degradation leachates of UP2W on the retention of 63Ni(II), 152Eu(III) and 242Pu(III/IV) by hardened cement paste in the degradation stage II.
Data availability statement
The original contributions presented in the study are included in the article/Supplementary Material, further inquiries can be directed to the corresponding authors.
Author contributions
SH, AM, XG, MA, and HG defined the initial concept of the project. AT and XG contributed to conception and design of the experimental study. PS and AT performed the experiments. AT and PS conducted data evaluation. XG wrote the first draft of the manuscript. PS and AT wrote sections of the manuscript. All authors contributed to manuscript revision, read, and approved the submitted version.
Funding
The EURAD project leading to this application has received funding from the European Union’s Horizon 2020 research and innovation programme under grant agreement No 847593. The work was performed in EURAD as part of the WP CORI and was partially funded by SKB. We acknowledge support by the KIT-Publication Fund of the Karlsruhe Institute of Technology.
Acknowledgments
Klas Källström (SKB) is kindly acknowledged for his strong involvement in conceiving and conceptualising the study, as well as in the selection of the proxy ligands. Jonas Rentmeister, Darlyn Rehhorn and Melanie Böttle (all KIT–INE) are gratefully acknowledged for the NPOC measurements and technical support.
Conflict of interest
AM and SH were employed by Svensk Kärnbränslehantering AB.
The remaining authors declare that the research was conducted in the absence of any commercial or financial relationships that could be construed as a potential conflict of interest.
Publisher’s note
All claims expressed in this article are solely those of the authors and do not necessarily represent those of their affiliated organizations, or those of the publisher, the editors and the reviewers. Any product that may be evaluated in this article, or claim that may be made by its manufacturer, is not guaranteed or endorsed by the publisher.
Supplementary material
The Supplementary Material for this article can be found online at: https://www.frontiersin.org/articles/10.3389/fnuen.2023.1117413/full#supplementary-material
Footnotes
1This concentration is in line with previous data reported in the literature (Ochs et al., 2016) but higher than the solubility limit of β-Ni(OH)2(cr) determined under analogous boundary conditions (González-Siso et al., 2018; Szabo et al., 2022b). This observation suggests that this solid phase is neither present in the cement not forming within the timeframe of our experiments. Solubility equilibrium involving other solid phases (e.g., Ni-bearing LDH phases or Ni(OH)2(am)) may instead be responsible of the measured concentrations of stable Ni in the cement pore water.
2The ratio 152Eu (total inventory): Stable Eu (inventory in HCP) ranges from 4:1 (at S:L = 1 g⋅L−1) to 1:1 (at S:L = 4 g⋅L−1). A much larger inventory of stable Eu in HCP would be required in order to make isotopic exchange a relevant retention mechanism in our experimental study, especially considering the very high log Rd values determined for this system (log Rd = 3-4, with Rd in m3⋅kg−1).
References
Altmaier, M., Gaona, X., Fellhauer, D., and Buckau, G. (2010) Intercomparison of redox determination methods on designed and near-neutral aqueous systems. KIT-SR 7572.
Altmaier, M., Neck, V., and Fanghanel, T. (2008). Solubility of Zr(IV), Th(IV) and Pu(IV) hydrous oxides in CaCl2 solutions and the formation of ternary Ca-M(IV)-OH complexes. Radiochim. Acta 96, 541–550. doi:10.1524/ract.2008.1535
Bruno, J., González-Siso, M. R., Duro, L., Gaona, X., and Altmaier, M. (2018) Key master variables affecting the mobility of Ni, Pu, Tc and U in the near field of the SFR repository. Main experimental findings and PA implications of the PhD thesis. SKB TR-18-01.
Cori-Sota, (2021) State of the art report on cement-organic-radionuclide interactions. Final version as of 19.05.2021 of deliverable D3.1 of the HORIZON 2020 project EURAD. EC Grant agreement no: 847593.
Dario, M., Molera, M., and Allard, B. (2004) Effect of organic ligands on the sorption of europium on TiO2 and cement at high pH. SKB TR-04-04.
Deelstra, H., and Verbeek, F. (1964). The determination of the stability constants of the lanthanide α-hydroxyisobutyrate and lactate complexes by potentiometric titration. Anal. Chim. Acta 31, 251–257. doi:10.1016/s0003-2670(00)88816-9
Duro, L., Domenech, C., Grive, M., Roman-Ross, G., Bruno, J., and Kallstrom, K. (2014). Assessment of the evolution of the redox conditions in a low and intermediate level nuclear waste repository (SFR1, Sweden). Appl. Geochem. 49, 192–205. doi:10.1016/j.apgeochem.2014.04.015
Duro, L., Grive, M., Gaona, X., Bruno, J., Andersson, T., Boren, H., et al. (2012) Study of the effect of the fibre mass UP2 degradation products on radionuclide mobilisation. SKB R-12-15.
Ermakov, I. V., Rebrov, A. I., Litmanovich, A. D., and Plate, N. A. (2000). Alkaline hydrolysis of polyacrylonitrile, 1 - structure of the reaction products. Macromol. Chem. Phys. 201, 1415–1418. doi:10.1002/1521-3935(20000801)201:13<1415::aid-macp1415>3.0.co;2-r
Fellhauer, D. (2013). Untersuchungen zur Löslichkeit und Redoxchemie von Plutonium und Neptunium. PhD thesis. Universität Heidelberg.
Gaona, X., Montoya, V., Colàs, E., Grivé, M., and Duro, L. (2008). Review of the complexation of tetravalent actinides by ISA and gluconate under alkaline to hyperalkaline conditions. J. Contam. Hydrol. 102, 217–227. doi:10.1016/j.jconhyd.2008.09.017
Giroux, S., Aury, S., Henry, B., and Rubini, P. (2002). Complexation of lanthanide(III) ions with polyhydroxy carboxylic acids in aqueous solutions. Eur. J. Inorg. Chem. 2002, 1162–1168. doi:10.1002/1099-0682(200205)2002:5<1162::aid-ejic1162>3.0.co;2-o
González-Siso, M. R., Gaona, X., Duro, L., Altmaier, M., and Bruno, J. (2018). Thermodynamic model of Ni(II) solubility, hydrolysis and complex formation with ISA. Radiochim. Acta 106, 31–45. doi:10.1515/ract-2017-2762
Grenthe, I., Gaona, X., Plyasunov, A. V., Rao, L., Runde, W. H., Grambow, B., et al. (2020). Second update on the chemical thermodynymics of uranium, neptunium, plutonium, americium and technetium. Paris, France: OECD Publications, Vol. 14.
Guidone, R. E., Lothenbach, B., Tasi, A., Gaona, X., Altmaier, M., and Geckeis, H. (2022). “Impact of formate, citrate and gluconate on the uptake of radionuclides by cement: Study of the binary and ternary systems cement-L and cement,” in Proceedings NUWCEM Conference, Avignon, France.
Holgersson, S., Dubois, I., and Börstell, L. (2011) Batch experiments of Cs, Co and Eu sorption onto cement with dissolved fibre mass UP2 in the liquid phase. SKB P-11-24.
Keith-Roach, M. J., Lindgren, M., and Källström, K. (2014) Assessment of complexing agent concentrations in SFR. SKB R-14-03.
Keith-Roach, M., Lindgren, M., and Källström, K. (2021) Assessment of complexing agent concentrations for the post-closure safety assessment in PSAR SFR. SKB R-20-04.
Keith-Roach, M., and Shahkarami, P. (2021) Organic materials with the potential for complexation in SFR, the final repository for short-lived radioactive waste. SKB R-21-03.
Litmanovich, A. D., and Plate, N. A. (2000). Alkaline hydrolysis of polyacrylonitrile. On the reaction mechanism. Macromol. Chem. Phys. 201, 2176–2180. doi:10.1002/1521-3935(20001101)201:16<2176::aid-macp2176>3.0.co;2-5
Mandaliev, P., Dahn, R., Tits, J., Wehrli, B., and Wieland, E. (2010a). EXAFS study of Nd(III) uptake by amorphous calcium silicate hydrates (C-S-H). J. Colloid Interf. Sci. 342, 1–7. doi:10.1016/j.jcis.2009.06.011
Mandaliev, P., Wieland, E., Dahn, R., Tits, J., Churakov, S. V., and Zaharko, O. (2010b). Mechanisms of Nd(III) uptake by 11Å tobermorite and xonotlite. Appl. Geochem. 25, 763–777. doi:10.1016/j.apgeochem.2010.01.002
Missana, T., Garcia-Gutierrez, M., Alonso, U., and Almendros-Ginesta, O. (2022). Nickel retention by calcium silicate hydrate phases: Evaluation of the role of the Ca/Si ratio on adsorption and precipitation processes. Appl. Geochem. 137, 105197. doi:10.1016/j.apgeochem.2022.105197
Moreton, A. D., Pilkington, N. J., and Tweed, C. J. (2000) Thermodynamic modelling of the effect of hydroxycarboxylic acids on the solubility of plutonium at high pH. NIREX NSS/R339.
Moreton, A. D. (1993). Thermodynamic modelling of the effect of hydroxycarboxylic acids on the solubility of plutonium at high pH. Mater. Res. Soc. Symposium Proc. 294, 753–758. doi:10.1557/proc-294-753
Ochs, M., Mallants, D., and Wang, L. (2016). Topics in safety, risk, reliability and quality. 1st ed. Cham: Springer International Publishing: Imprint: Springer, 1. online resource (XXX, 301 pages).Radionuclide and metal sorption on cement and concrete
Pointeau, I., Coreau, N., and Reiller, P. (2008). Uptake of anionic radionuclides onto degraded cement pastes and competing effect of organic ligands. Radiochim. Acta 96, 367–374. doi:10.1524/ract.2008.1503
Pointeau, I., Piriou, B., Fedoroff, M., Barthes, M. G., Marmier, N., and Fromage, F. (2001). Sorption mechanisms of Eu3+ on CSH phases of hydrated cements. J. Colloid Interf. Sci. 236, 252–259. doi:10.1006/jcis.2000.7411
Pointeau, I., Reiller, P., Mace, N., Landesman, C., and Coreau, N. (2006). Measurement and modeling of the surface potential evolution of hydrated cement pastes as a function of degradation. J. Colloid Interf. Sci. 300, 33–44. doi:10.1016/j.jcis.2006.03.018
Rai, D., and Kitamura, A. (2017). Thermodynamic equilibrium constants for important isosaccharinate reactions: A review. J. Chem. Thermodyn. 114, 135–143. doi:10.1016/j.jct.2017.02.012
Rojo, H., Gaona, X., Rabung, T., Polly, R., Garcia-Gutierrez, M., Missana, T., et al. (2021). Complexation of Nd(III)/Cm(III) with gluconate in alkaline NaCl and CaCl2 solutions: Solubility, TRLFS and DFT studies. Appl. Geochem. 126, 104864. doi:10.1016/j.apgeochem.2020.104864
Rojo, H., Tits, J., Gaona, X., Garcia-Gutierrez, M., Missana, T., and Wieland, E. (2013). Thermodynamics of Np(IV) complexes with gluconic acid under alkaline conditions: Sorption studies. Radiochim. Acta 101, 133–138. doi:10.1524/ract.2013.2022
Stumpf, T., Tits, J., Walther, C., Wieland, E., and Fanghanel, T. (2004). Uptake of trivalent actinides (curium(III)) by hardened cement paste: A time-resolved laser fluorescence spectroscopy study. J. Colloid Interf. Sci. 276, 118–124. doi:10.1016/j.jcis.2004.03.014
Szabo, P., Tasi, A., Gaona, A., Maier, A., Hedström, S., Altmaier, M., et al. (2022a). Uptake of selected organic ligands by hardened cement paste: Studies on proxy ligands for the degradation of polyacrylonitrile and general considerations on the role of different functionalities in the uptake process. Front. Nucl. Eng. 1. (in review). doi:10.3389/fnuen.2022.997398
Szabo, P., Tasi, A., Gaona, A., Polly, R., Maier, A., Hedström, S., et al. (2022b). Solubility of Ca(II), Ni(II), Nd(III) and Pu(IV) in the presence of proxy ligands for the degradation of polyacrylonitrile in cementitious systems. Dalton T 51, 9432–9444. doi:10.1039/d2dt01409b
Tasi, A., Gaona, X., Fellhauer, D., Bottle, M., Rothe, J., Dardenne, K., et al. (2018a). Thermodynamic description of the plutonium - alpha-D-isosaccharinic acid system I: Solubility, complexation and redox behavior. Appl. Geochem. 98, 247–264. doi:10.1016/j.apgeochem.2018.04.014
Tasi, A., Gaona, X., Fellhauer, D., Bottle, M., Rothe, J., Dardenne, K., et al. (2018b). Thermodynamic description of the plutonium - alpha-D-isosaccharinic acid system ii: Formation of quaternary Ca(II)-Pu(IV)-OH-ISA complexes. Appl. Geochem. 98, 351–366. doi:10.1016/j.apgeochem.2018.06.014
Tasi, A., Gaona, X., Fellhauer, D., Bottle, M., Rothe, J., Dardenne, K., et al. (2018c). Redox behavior and solubility of plutonium under alkaline, reducing conditions. Radiochim. Acta 106, 259–279. doi:10.1515/ract-2017-2870
Tasi, A., Gaona, X., Rabung, T., Fellhauer, D., Rothe, J., Dardenne, K., et al. (2021a). Plutonium retention in the isosaccharinate - cement system. Appl. Geochem. 126, 104862. doi:10.1016/j.apgeochem.2020.104862
Tasi, A., Szabo, P., Gaona, X., Altmaier, M., and Geckeis, H. (2021b). “Contribution by KIT-INE to CORI Task 2 (pers. co.),” in Eurad European joint programme on radioactive waste management, milestone DMS97 – CORI technical report -task 2 hydrolytic/radiolytic degradation of organics: Description of first results on hydrolytic and radiolytic organic degradation and identification of released species. Editors D. Ricard, and J Vandenborre.
Tits, J., Stumpf, T., Rabung, T., Wieland, E., and Fanghanel, T. (2003). Uptake of Cm(III) and Eu(III) by calcium silicate hydrates: A solution chemistry and time-resolved laser fluorescence spectroscopy study. Environ. Sci. Technol. 37, 3568–3573. doi:10.1021/es030020b
Tits, J., Wieland, E., Bradbury, M. H., and Dobler, J. P. (2000). “The uptake of Eu(III) and Th(IV) by cement-type minerals in the alkaline distrubed zone of a nuclear repository,” in Applied mineralogy. Editor D. Rammlmair (Rotterdam: Balkema), 705–708.
Tits, J., Wieland, E., and Bradbury, M. H. (2005). The effect of isosaccharinic acid and gluconic acid on the retention of Eu(III), Am(III) and Th(IV) by calcite. Appl. Geochem. 20, 2082–2096. doi:10.1016/j.apgeochem.2005.07.004
Vercammen, K., Glaus, M. A., and Van Loon, L. R. (2001). Complexation of Th(IV) and Eu(III) by alpha-isosaccharinic acid under alkaline conditions. Radiochim. Acta 89, 393–402. doi:10.1524/ract.2001.89.6.393
Wang, Z. M., van de Burgt, L. J., and Choppin, G. R. (2000). Spectroscopic study of lanthanide(III) complexes with aliphatic dicarboxylic acids. Inorg. Chim. Acta 310, 248–256. doi:10.1016/s0020-1693(00)00259-0
Wieland, E. (2014) Sorption data base for the cementitious near field of L/ILW and ILW repositories for provisional safety analyses for SGT-E2. Nagra Technical Report 14-08.
Wieland, E., Tits, J., Spieler, P., and Dobler, J. P. (1998). Interaction of Eu(III) and Th(IV) with sulphate-resisting Portland cement. Mater. Res. Soc. Symposium Proc. 506, 573–578.
Wieland, E., Tits, J., Spieler, P., Dobler, J. P., and Scheidegger, A. M. (2000). “Uptake of nickel and strontium by a sulphate-resisting Portland cement,” in Applied mineralogy. Editor D. Rammlmair (Rotterdam: Balkema), 705–708.
Wieland, E., Tits, J., Ulrich, A., and Bradbury, M. H. (2006). Experimental evidence for solubility limitation of the aqueous Ni(II) concentration and isotopic exchange of63Ni in cementitious systems. Radiochim. Acta 94, 29–36. doi:10.1524/ract.2006.94.1.29
Keywords: sorption, UP2W, polyacrylonitrile, α-hydroxyisobutyric acid, 3-hydroxybutyric acid, glutaric acid, cement, radionuclide
Citation: Szabo PG, Tasi AG, Gaona X, Maier AC, Hedström S, Altmaier M and Geckeis H (2023) Uptake of Ni(II), Eu(III) and Pu(III/IV) by Hardened Cement Paste in the Presence of Proxy Ligands for the Degradation of Polyacrylonitrile. Front. Nucl. Eng. 2:1117413. doi: 10.3389/fnuen.2023.1117413
Received: 07 December 2022; Accepted: 24 January 2023;
Published: 13 February 2023.
Edited by:
Michael Ojovan, Imperial College London, United KingdomReviewed by:
Nathalie Wall, University of Florida, United StatesTiziana Missana, Centro de Investigaciones Energéticas, Medioambientales y Tecnológicas, Spain
Copyright © 2023 Szabo, Tasi, Gaona, Maier, Hedström, Altmaier and Geckeis. This is an open-access article distributed under the terms of the Creative Commons Attribution License (CC BY). The use, distribution or reproduction in other forums is permitted, provided the original author(s) and the copyright owner(s) are credited and that the original publication in this journal is cited, in accordance with accepted academic practice. No use, distribution or reproduction is permitted which does not comply with these terms.
*Correspondence: A. G. Tasi, YWdvc3QudGFzaUBraXQuZWR1; X. Gaona, eGF2aWVyLmdhb25hQGtpdC5lZHU=