- 1Department of Neurology, Psychosomatic Medicine and Psychiatry, Institute of Health Promotion and Clinical Movement Science, German Sport University (GSU) Cologne, Cologne, Germany
- 2Department of Motor Behavior in Sports, Institute of Health Promotion and Clinical Movement Science, German Sport University (GSU) Cologne, Cologne, Germany
Introduction: Each individual touches the own body several 100 times a day. While some researchers propose a self-regulatory function of self-touch, others report that self-touching increases nervousness. This controversy appears to be caused by the fact that researchers did not define the kind of self-touch they examined and actually, referred to different types of self-touch. Thus, kinematically defining different types of self-touch, such as phasic (discrete), repetitive, and irregular, and exploring the neural correlates of the different types will provide insight into the neuropsychological function of self-touching behavior.
Methods: To this aim, we assessed hemodynamic responses in prefrontal brain areas using functional near-infrared spectroscopy (fNIRS) and behavioral responses with NEUROGES®. Fifty-two participants were recorded during three specific kinematically types of self-touch (phasic, irregular, repetitive) that were to be performed on command. The recently developed toolbox Satori was used for the visualization of neuronal processes.
Results: Behaviorally, the participants did not perform irregular self-touch reliably. Neurally, the comparison of phasic, irregular and repetitive self-touch revealed different activation patterns. Repetitive self-touch is associated with stronger hemodynamic responses in the left Orbitofrontal Cortex and the Dorsolateral Prefrontal Cortex than phasic self-touch.
Discussion: These brain areas have been reported to be associated with self-regulatory processes. Furthermore, irregular self-touch appears to be primarily generated by implicit neural control. Thus, by distinguishing kinematically different types of self-touch, our findings shed light on the controverse discussion on the neuropsychological function of self-touch.
1 Introduction
Self-touching is ubiquitous in everyday life (Grunwald et al., 2014; Kreyenbrink et al., 2017; Lausberg, 2022). According to observational studies, self-touch occurs in emotional, aroused, and stressful situations (Lausberg, 2013; Grunwald et al., 2014; Heubach, 2016; Kreyenbrink et al., 2017; Densing et al., 2018; Reinecke et al., 2020, 2022; Furley, 2021; Neumann et al., 2022). Some researchers propose a self-regulatory function of self-touch (Freedman et al., 1972; Grunwald et al., 2014; Helmich et al., 2014; Densing et al., 2018). Others report that self-touching represents stress. This controversy appears to be caused by the fact that researchers did not define the kind of self-touch they examined and actually referred to different types of self-touch (Reinecke et al., 2020). This leads to the fact that self-touch and its neuropsychological correlates are still poorly understood. Thus, kinematically defining different types of self-touch, such as phasic (discrete), repetitive, and irregular, and exploring the neural correlates of the different types will provide insights into the neuropsychological function of self-touching behavior.
Self-touch is defined as the dynamic physical contact between two parts of the body, typically the hand acting on a part of the body (Lausberg, 2022). Self-touch varies from scratching, rubbing, and kneading to stroking. Kinematically, based on the movement trajectory, three types of self-touch can be observed in everyday life and therefore distinguished as follows: phasic self-touches are characterized by a phase structure. They contain a transport phase, in which the hand is transported to the location of touching, and a concept phase, with a one-way movement path in which the hand acts on the body, which is directly followed by a retraction phase in which the hand is moved back, for example, a single stroke. Repetitive self-touches, such as phasic touches, consist of a transport phase, a concept phase, and a retraction phase. However, in the concept phase, the same movement path is used repetitively without a rest, for example, scratching. Only when a movement has been performed several times in the same direction does the retraction phase follow. In contrast, irregular self-touches have no phase structure. They are characterized by short movement paths in various directions and practically no displacement of the hand. Since they have no concept phase, they are not based on any motor plan (Lausberg, 2019). Repetitive vs. phasic touch represents two distinct phenomenological entities. It is not the quantity of a touch that is important but the quality of the contact (Spencer et al., 2003; Schaal et al., 2004; van Mourik and Beek, 2004; Huys et al., 2008; Lausberg, 2013). The different self-touch types occur in different contexts in daily life (Heubach, 2016; Mueller et al., 2019; Neumann et al., 2022). Repetitive self-touch is associated with better psychological wellbeing, in contrast to irregular self-touch (Reinecke et al., 2020). irregular self-touch probably serves to shield from other negative stimuli via strong somatosensory stimulation. Furthermore, opposite effects are found for phasic vs. irregular self-touch (Lausberg, 2022). phasic self-touch is also associated with the regulation process during acute stress and thereby enhances the cognitive process (Freedman and Bucci, 1981; Grunwald et al., 2014; Heubach, 2016). The higher the time proportion of phasic self-touching, the lower the subjective stress experience (Heubach, 2016). All three types of touch are to be distinguished in terms of their emotional, cognitive, and physical functions. In this case, it is not the quantity of a touch that is important but the quality of the contact (Lausberg, 2013). The differential effects of repetitive, irregular, and phasic self-touch explain the controversy debated by current researchers and show the importance of a fine-grained analysis of self-touch.
To our knowledge, there has never been any attempt to investigate brain activation during the three specific types of self-touch. Previous studies investigated self-touch without kinematically defining and distinguishing different types of self-touch. The self-touch was described as more “repetitive-like” or more “phasic-like,” but no specific movement criteria were used to classify self-touch. Different methods such as functional magnetic resonance imaging (fMRI) or electroencephalogram (EEG) were used to measure brain activity. These previous studies revealed a deactivation in the prefrontal areas, such as the ventrolateral prefrontal cortex, the orbitofrontal cortex (OFC), the dorsomedial prefrontal cortex, and the amygdala, the right striatum, the superior temporal gyrus, and the posterior cingulate for instructed explicit self-touch (Grunwald et al., 2014; Kikuchi et al., 2018; Boehme et al., 2019). These results are attributed to the principle of reafference. The reafference principle attenuates the effects of explicit and therefore conscious self-stimulation through predictive mechanisms (Weiskrantz et al., 1971; Blakemore et al., 1998; Synofzik, 2008; Boehme et al., 2019).
Studies have shown an association between repetitive movements in general and an activation in the prefrontal cortex (PFC). Brain activation of the PFC is considered to reduce arousal (Kinsbourne, 2011). Moreover, repetitive movements can lead to flow and trance-like states, where persons merge action and awareness and experience a loss of the sense of space and time (Hove and Stelzer, 2018; Sudeck and Thiel, 2020). The brain activation in the PFC and the OFC reflects the merging of action and awareness during flow (Nagai et al., 2004; Kinsbourne, 2011). The potential of repetitive movements to reach an extraordinary mental state such as flow implies that repetitive self-touch can have a strong self-regulatory effect. Considering this effect, it is important to differentiate between specific types of touch.
Research on social touch may further help with understanding the potential self-regulatory effect of self-touch. Social touches include all tactile touches that are not self-performed, regardless of whether these touches are performed directly skin-to-skin or not (Olausson et al., 2016). The neuropsychological effects of pleasantly perceived social touch, skin-to-skin and brush-to-skin, have been widely studied, and their positive effects on the recipient's wellbeing have been documented (Field, 2019; Li et al., 2019; Portnova et al., 2020; Uvnäs-Moberg et al., 2020). Unmyelinated C-tactile (CT) afferents in hairy skin are associated with these effects. These unmyelinated CT afferents are optimally activated at a repetitive gentle stroking frequency (1–10 cm/s; Field, 2019; Uvnäs-Moberg et al., 2020). Repeated activation of CT afferents is associated with better health by reducing sympathetic nervous system activity, increasing parasympathetic nervous system activity, and reducing stress, pain, and anxiety via oxytocin (Heinrichs and Domes, 2008; Ishak et al., 2011; Quirin et al., 2011; Love, 2014; Pfeifer et al., 2016; Walker et al., 2017; Hurlemann and Grinevich, 2018; Field, 2019; Uvnäs-Moberg et al., 2020; Uvnäs-Moberg and Petersson, 2022). At the neural level, correlates of social touch are found with the cortical brain regions in the dorsolateral prefrontal cortex (dlPFC) and the OFC, which are also associated with oxytocin projections (Rolls et al., 2003; Croy et al., 2016; Morita et al., 2018; Boehme et al., 2019; Field, 2019; Chen et al., 2020; Uvnäs-Moberg et al., 2020). Motor aspects of touch (discriminative touch) significantly predict the activation in the sensorimotor cortex (Rolls et al., 2003; Case et al., 2016). The higher the intensity of discriminative touch, the greater the change in signal (Kashou and Giacherio, 2016). However, research on affective touch indicates that brain activity shows a stronger association with pleasantness than with intensity in the context of affective touch (Case et al., 2016). Since these effects take place via tactile stimulation by hand or brush, it can be assumed that self-touch also may achieve stimulation of the CT afferents.
Research on non-verbal behavior and recent advances in neuroimaging research have shown that it may be of particular interest to incorporate more naturalistic conditions (Dehais and Ayaz, 2019; Mueller et al., 2019; von Lühmann et al., 2021). In the present study, this was taken into account by considering the three specific kinematic types of self-touch, which can be observed in everyday life. Furthermore, functional near-infrared spectroscopy (fNIRS), as a wearable neuroimaging system, enables data from freely moving participants. This allows participants to behave in a more naturalistic way during the self-touch (von Lühmann et al., 2021). This study leads to a more complex and innovative experimental paradigm.
Taken together, the main purpose of this study is to provide profound insights into how the healthy brain works. The aim of this study is to better understand the neural correlates of self-touch as a non-verbal behavior and to be able to solve the controversy through research. Our main hypothesis postulates that the three specific types of self-touch differ in their cerebral activity in the dlPFC and the OFC. Furthermore, we expect to observe higher brain activation for repetitive and phasic compared to irregular self-touch in the OFC and the dlPFC as an effect of repetitive and phasic self-touch being more involved in positive self-regulation. Owing to the fact that repetitive self-touch seems to have the highest impact on wellbeing, we further expect to observe higher brain activation for repetitive self-touch compared to phasic self-touch in the OFC and the dlPFC.
2 Materials and methods
To achieve high standards, this publication followed best-practice recommendations for fNIRS articles (Yücel et al., 2021).
2.1 Ethical approval
The study was approved by the Local Ethics Committee of the German Sport University (Nr. 162/2022). Written informed consent was obtained from each participant.
2.2 Participants
Fifty-two healthy individuals (mean age: 26.73 ± 5.23 years; 36 women, 16 men; note that a diverse sample was not achieved) participated in the study. According to a power analysis with G*Power, data for 43 participants were needed for a moderate effect size, a power of 0.8, and a low correlation (f = 0.25; α error probability = 0.05; power = 0.8; r = 0.2). All participants had no known history of neurological or psychiatric disorders. Handedness was examined using the Montreal Handiness Questionnaire version that Crovitz and Zener (1962) used at the Montreal Neurological Institute. Thirty-one participants were right-handed, and 21 were ambidextrous.
2.3 Experimental procedure
In a room with lowered blinds, participants were seated in a comfortable upright position on a chair without armrests to allow unrestricted arm and hand movements. To achieve familiarization with the experimental procedure, the participants practiced phasic, repetitive, and irregular self-touch. First, the three types of self-touch were presented in a tutorial video. After watching the video tutorial, the participants were instructed to perform the three types of self-touch on the upper side of the forearm as naturally as possible and as pleasantly as possible. Since research about affective touch indicates that brain activity shows a stronger association with pleasantness than with the intensity in the context of affective touch, the study focuses on the participants' natural and pleasant touch behaviors (Case et al., 2016). The participants immediately received feedback from the experimenter if their performance was in line with the definition of the values from NEUROGES®.
To test our hypotheses, we used three conditions on command in a block design: (1) phasic: a single movement with no repetition of the same trajectory, (2) repetitive: same trajectory is executed at least twice; and (3) irregular: small irregular and unrhythmic movements (see the Introduction section). Each stimulus was performed six times with the right hand and six stimuli were performed with the left hand (left phasic–right phasic–left irregular–right irregular–left repetitive–right repetitive). This resulted in a total duration of 106 s per condition. The entire duration of the experiment was 16 min (Figure 1). phasic self-touch was performed first because this type of self-touch is expected to have the least sustained effects on cerebral activity (Lausberg, 2019). Since phasic self-touch and irregular self-touch hypothetically have the least cerebral similarity they followed sequentially (Schaal et al., 2004; Lausberg, 2013; Konczak and Winter, 2020). Repetitive self-touch seems to have the greatest and most lasting impact on cerebral activity in the regions of interest (ROIs). Therefore, repetitive self-touch was performed last. To ensure that the activation of the PFC can be attributed as little as possible to motor behavior, our design took into account two aspects. First, we presented familiar motor behavior stimuli (Lausberg, 2013; Heubach, 2016; Kreyenbrink et al., 2017; Densing et al., 2018; Reinecke et al., 2020; Neumann et al., 2022). Second, we presented each stimulus six times in a row. Thus, the stimuli did not come unexpectedly. Both aspects result in less involvement of the PFC (Miller and Cohen, 2001; Corbetta and Shulman, 2002; Lin et al., 2022).
The experiment started and ended with a 3-min resting phase in which the participant watched a neutral landscape presented on the screen. Participants began to move immediately after a peep sound, and the stimuli were given by written instructions (e.g., left phasic). They continued for 9 s until the next peep sounded and the word pause appeared on the screen. Pause means a resting phase without movement for 7 s (interstimulus interval). All instructions were developed with PsychoPy Version 3 (Peirce et al., 2019).
2.4 fNIRS acquisition and analyses
2.4.1 fNIRS acquisition and montage
Cerebral activity was recorded using a portable continuous wave fNIRS system (NIRSport 2, NIRx, Medical Technologies LLC, Berlin, Germany; wavelengths of 760 and 850 nm; sampling rate 10.2 Hz). The montage contains eight light sources, seven detectors, and a bundle of eight short-distance detectors. The optodes were placed according to the 10–20 system on a standardized cap (EasyCap GmbH, Herrsching, Germany; Jasper, 1958). An fNIRS Optoden Location Decider was used to determine the most sensitive placement for each optode, transcribe the Montreal Neurological Institute and Hospital (MNI) coordinates, and determine the overlap of each channel with the corresponding brain region (>80% overlap; Morais et al., 2018; Table 1; Figure 2). The position of optodes allowed the coverage of brain areas in the prefrontal cortex. These areas included two ROIs in the frontal lobe: the OFC and the dlPFC (McGlone et al., 2012; Gordon et al., 2013; Bennett et al., 2014; Scheele et al., 2014; Morais et al., 2018; Table 1).
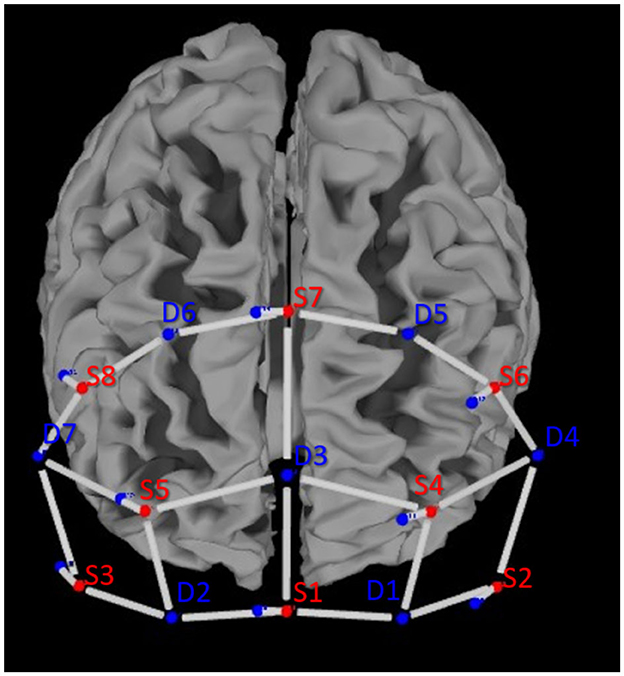
Figure 2. Optode placement according to the 10–20 system above the prefrontal cortex of the left and right hemispheres.
Data were recorded from 20 long-distance channels of measurement and 8 short-distance channels to account for changes in the extracerebral blood flow (see Figure 2). The mean source–detector distance (long-distance channels) was 34.4 ± 4.6 mm. The short-distance channels were 8.0 ± 0.0 mm (Brigadoi and Cooper, 2015).
2.4.2 Data quality check
To assess the quality of the fNIRS signal, data were visually inspected for each participant. To complete the visual inspection, the scalp coupling index (SCI) was computed during preprocessing (Pollonini et al., 2016). All channels with a value of <0.6 were rejected. Using this quality check, seven participants were excluded from further analysis. Of the remaining 52 participants, 46 had 0 rejected channels, 6 had 1 rejected channel, and 1 had 2 rejected channels.
2.4.3 Preprocessing
The fNIRS data were analyzed using the Satori (v.1.8) toolbox (Lührs and Goebel, 2017). The preprocessing was performed on the whole signal for each participant. First, fNIRS raw data were transformed into optical density. Second, the SCI channel rejection was computed. Then, optical density was converted via the modified Beer–Lambert law (MBLL) into the concentration changes of oxygenated hemoglobin (Δoxy-Hb) and deoxygenated hemoglobin (Δdeoxy-Hb). Motion artifacts were corrected by applying the motion correction functions of Satori [spike removal; temporal derivative distribution repair (TDDR) according to Fishburn et al. (2019)]. Because the use of short-separation detector measurements as a regressor in the general linear model (GLM) has been previously shown to statistically improve hemodynamic response function (HRF) estimation (Gagnon et al., 2011; Yücel et al., 2015; Tachtsidis and Scholkmann, 2016), we used short-distance signals to regress out signals of extra-cerebral layers from the long-distance channels. To account for cardiac oscillations and Mayer waves, we used a 0.5 Hz low-pass filter, a high-pass filter (Butterworth) of 0.01 Hz, and the linear detrending function of Satori. The data were z-transformed.
2.4.4 Statistical analyses
Since this study focused on naturalistic behavior, there was no pre-established number of repetitions or speed. Thus, we decided that the fNIRS response amplitude is better explained using a regressor based on duration compared to a regressor modulated by stimulus intensity.
The beta values were estimated for each channel and participant. The beta weights represent the strength of each regressor on the amplitude of the hemodynamic response (Plichta et al., 2007; Pinti et al., 2018). The beta values of the oxygenated hemodynamic and deoxygenated response were estimated by a general linear model and were statistically analyzed by the mixed-model analysis of variance (ANOVA) using R studio software (Allaire, 2012; R Core Team, 2022). The mixed ANOVA included the within-subjects factors CONDITION (phasic vs. repetitive vs. irregular) and CHANNEL (CH1:CH28). While the between-subjects factors GENDER (male vs. female) and HANDEDNESS (right-handed vs. ambidextrous) were not subjects of our research question, they were controlled for since previous studies had shown their effect on non-verbal behavior and cerebral blood flow (Skomroch et al., 2013; Helmich and Lausberg, 2014; Zhang et al., 2020; Helmich et al., 2022). The significance level was set to a p-value of < 0.05. We applied corrected significance according to Greenhouse and Geisser (1959). First, an analysis was performed which averaged over all channels and then for each single channel. Whenever the interaction between the factors reached significance, post-hoc t-tests were performed by applying Bonferroni correction.
2.5 Behavioral analysis (NEUROGES®)
The participants' behavioral responses, i.e., the execution of phasic, repetitive, and irregular self-touch on command, were assessed with the NEUROGES system for non-verbal behavior and gesture (Lausberg, 2019). The complete research coding manual has recently been accepted for open-access publication. The interrater agreement of two independent, certified raters as measured with the modified Cohen's kappa EasyDiag (Holle and Rein, 2015) was for phasic (kappa = 0.67), repetitive (kappa = 0.67), and irregular (kappa = 0.77) self-touch.
3 Results
3.1 fNIRS
3.1.1 GENDER and HANDEDNESS
The analysis revealed a significant effect for the between-subjects factor GENDER, F(1,49) = 5.18, p = 0.027 but no effects for the interactions of GENDER* CONDITION, GENDER* CHANNEL, and GENDER*CONDITION*CHANNEL. Furthermore, there was no significant effect for the between-subjects factor HANDEDNESS or of any interaction with CONDITION or CHANNEL. Since GENDER and HANDEDNESS had no effect on the within-subjects factors CONDITION and CHANNEL, which were the subjects of our research question, in the following, we only report the results for the latter factors.
3.1.2 CONDITION
Averaged over all channels, there was a significant effect of CONDITION on the Δoxy-Hb (p = 0.031). The highest Δoxy-Hb was found for irregular self-touch [emmean = 0.072, SE = 0.034, 95% CI = (0.004– 0.14)], followed by repetitive [emmean = 0.066, SE = 0.034, 95% CI = (−0.002–0.14)] and phasic [emmean = −0.040, SE = 0.032, 95% CI = (−0.02–0.10)] self-touches. However, none of the post-hoc analyses' results showed significant effects (phasic compared to irregular, p = 0.07; phasic compared to repetitive, p = 0.12; and irregular compared to repetitive, p = 1.00).
There were no significant effects for beta weights on Δdeoxy-Hb.
3.1.3 CHANNEL*CONDITION
There was a significant effect of the interaction CONDITION*CHANNEL on the Δoxy-Hb, F(54, 2, 646) = 1.33, p = 0.05. Post-hoc analyses resolved by CHANNEL indicated a significant increase of activation in four channels, namely, 5, 6, 11, and 13, in the left prefrontal cortex (see Figure 3).
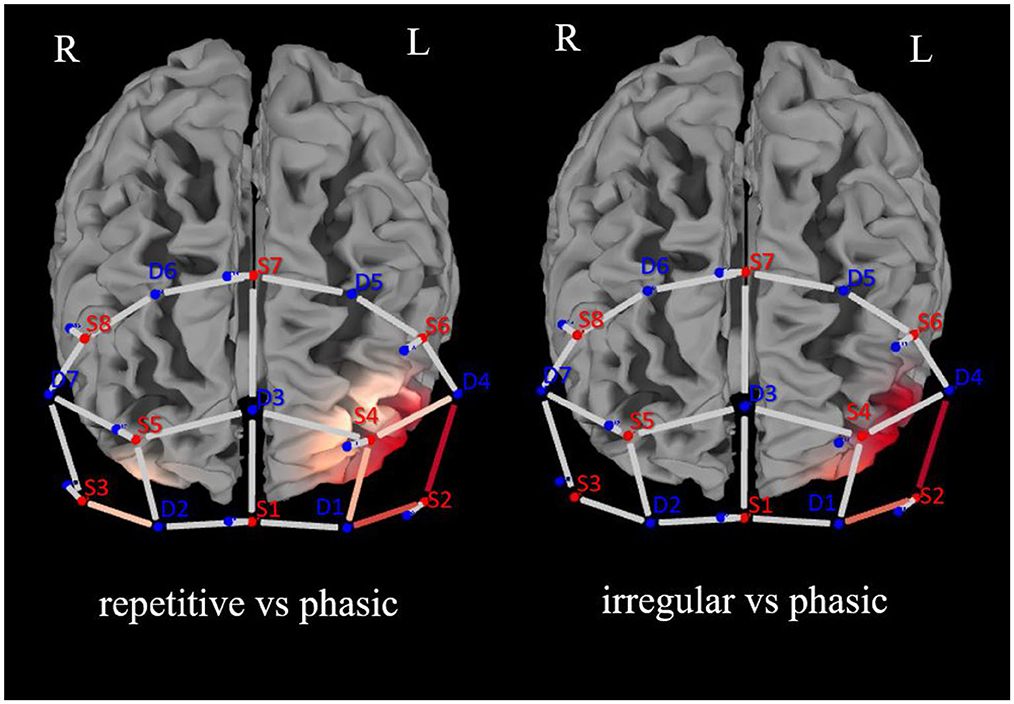
Figure 3. Brain activation (oxygenated hemoglobin) for the analysis of repetitive vs. phasic and irregular vs. phasic self-touch conditions for each single channel in the prefrontal cortex (red represents contrast: the darker the red, the greater the contrast described).
In CH5 and CH6, significant differences between phasic and repetitive (CH5: p = 0.004; CH6: p = 0.001) and between phasic and irregular (CH5: p = < 0.001; CH6: p = 0.004) self-touch conditions were revealed, and in CH11 and CH13, there were also significant differences between phasic and repetitive (CH11: p = 0.037; CH13: p = 0.025) self-touch conditions. The contrast of repetitive vs. irregular self-touch conditions did not result in significantly different brain activation for oxygenated hemoglobin. The post-hoc analyses resolved by CONDITION showed no significant effects. The full details of analysis outputs are described in Table 2 and Figure 4.
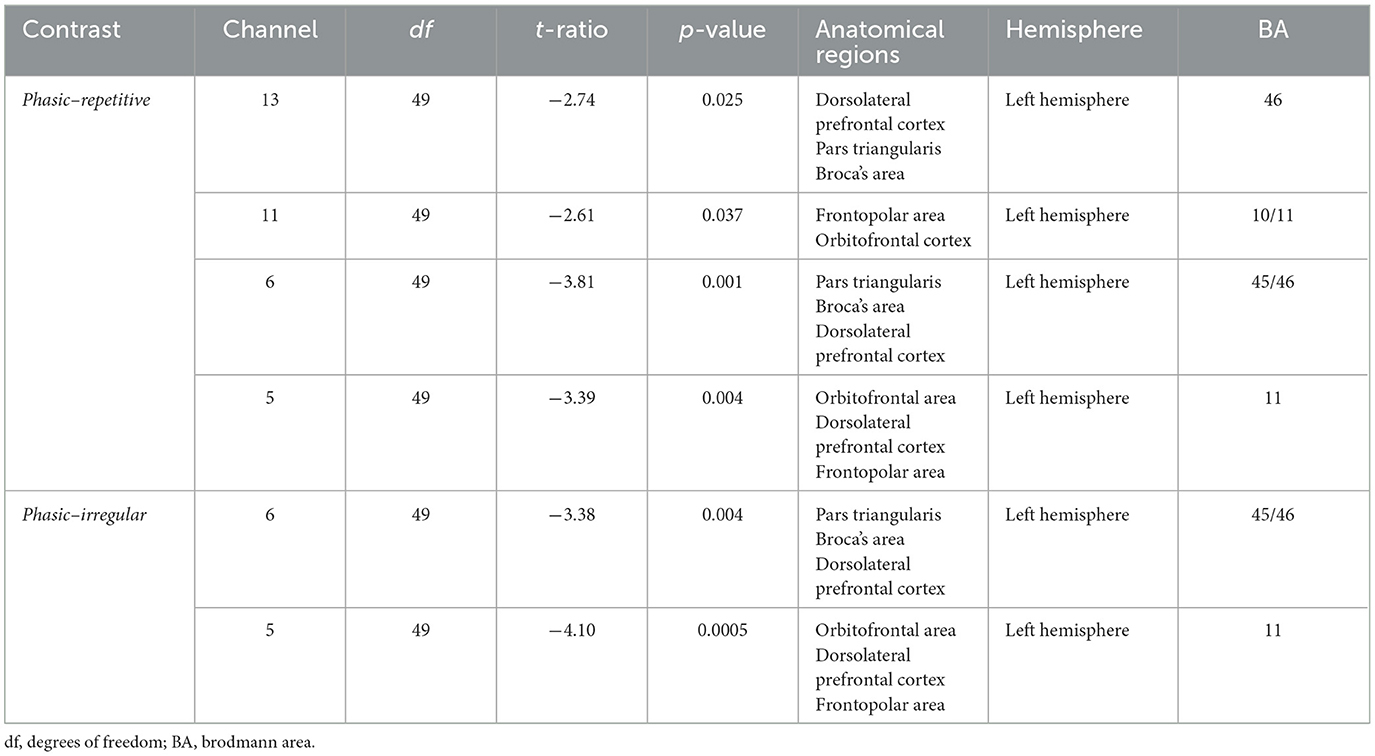
Table 2. Post-hoc analysis of the CONDITIONS repetitive vs. phasic and irregular vs. phasic in the prefrontal cortex.
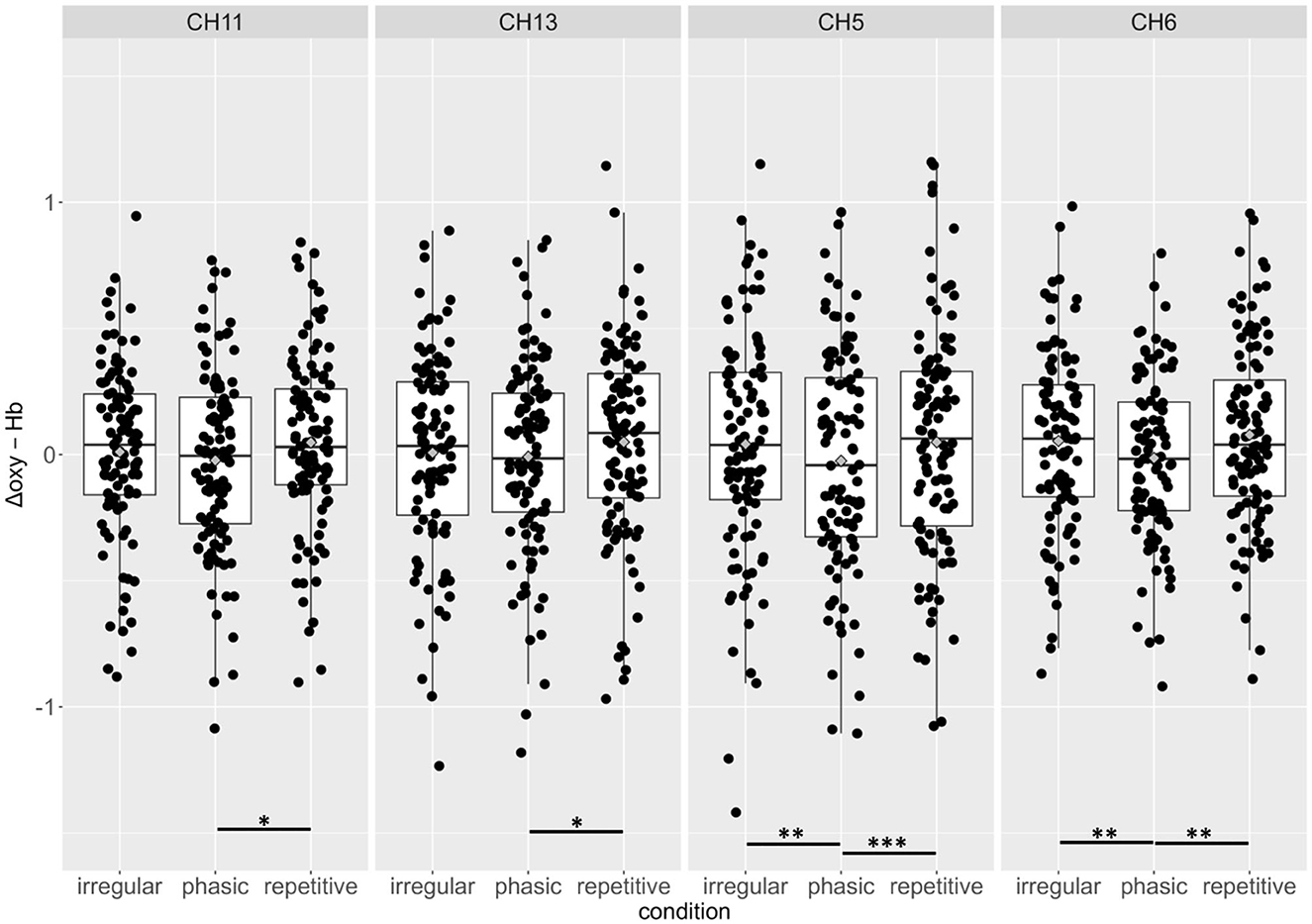
Figure 4. Group means and individual scores for oxygenated hemoglobin of the irregular, phasic, and repetitive conditions in channels 11, 13, 5, and 6. There were no significant effects for beta weights of Δdeoxy-Hb. *p < 0.05; **p < 0.01; ***p < 0.001.
3.2 NEUROGES
The NEUROGES analysis of the participants' behavioral responses showed that phasic and repetitive self-touches were performed correctly, while only 108 of 1,872 irregular self-touches were performed correctly. The participants instead combined the specific types of self-touch. The participants performed irregular self-touches quite similarly to repetitive self-touches.
4 Discussion
The present study investigated the hemodynamic responses during the execution of different types of self-touch. The comparison of phasic, irregular, and repetitive self-touch conditions revealed different neural activation patterns, as measured by oxygenated hemoglobin, averaged over all channels. Furthermore, the analysis of the single channels revealed significant effects of repetitive self-touch within the frontopolar area, the orbitofrontal area, the dlPFC, and the pars triangularis Broca's area. In these brain regions, the execution of repetitive self-touch leads to stronger hemodynamic responses compared to phasic self-touch. The behavioral analysis, however, revealed that participants performed phasic and repetitive self-touch correctly but not irregular self-touch.
Despite practicing the different types of self-touch before the experiment, the participants, in the irregular condition, performed irregular self-touches quite similar to repetitive self-touches. This could be due to irregular self-touch being performed unconsciously, i.e., beyond the individual's awareness (Lausberg, 2022), in everyday life. The attempt to consciously perform this behavior, as requested in the present experiment, results in a more structured and regular performance. The more structured performance leads to self-touch that is more similar to that of repetitive self-touch. Therefore, in the present study, we cannot draw any conclusion from the fNIRS findings for the irregular condition about the neural correlates of irregular self-touch. The results of the irregular condition show that it is essential to distinguish between an implicit (naturalistic) self-touch paradigm and an explicit (on command) self-touch paradigm, which was performed in the present study (Grunwald et al., 2014). Furthermore, the results of the irregular condition show that the type of self-touch plays a crucial role in brain activity. Mostly, the factor time is considered with an increase in brain activation (Sailer et al., 2016). In terms of time, a difference between the irregular and the phasic condition would be expected. Therefore, the non-significant difference between these two conditions is due to the type of performance rather than due to the time factor. However, the following discussion concentrates on the fNIRS findings for the phasic and repetitive conditions.
The findings of the present study seem to be in contrast with those of previous studies about self-touch, which show a deactivation of or no effect on the brain during self-touch (Grunwald et al., 2014; Kikuchi et al., 2018; Boehme et al., 2019). Regarding our definition of the specific types of self-touch, Grunwald et al. (2014) investigated more “phasic-like” self-touch on command and Kikuchi et al. (2018) and Boehme et al. (2019) investigated more “repetitive-like” self-touch on command. Considering specific types of touch, our results are similar to Grunwald et al.'s (2014) but in contrast to those of Kikuchi et al.'s (2018) and Boehme et al.'s (2019) because the instructed phasic self-touch reveals no significant effects. However, for a clear understanding of previous research, a specific definition of self-touch and control of the participants' performance with objective and reliable behavioral methods would be necessary to enhance the reliability and interpretation of reported self-touch studies. Nevertheless, the present study is in line with the assumption that repetitive self-touch is able to have a stronger neuropsychological effect than phasic self-touch (Hove and Stelzer, 2018; Sudeck and Thiel, 2020).
Additionally, these results support the assumption that unmyelinated CT afferents are optimally activated with repetitive stroking (Field, 2019; Uvnäs-Moberg et al., 2020). Moreover, the execution of repetitive self-touch activated the left hemispheric pars triangularis Broca's area, the left hemispheric dlPFC, and the left hemispheric frontopolar and left hemispheric orbitofrontal areas. Concerning the field of social touch, the OFC and the dlPFC play important roles in self-regulation, and an association with oxytocin is likely (Heinrichs and Domes, 2008; Ishak et al., 2011; Kinsbourne, 2011; Love, 2014; Pfeifer et al., 2016; Walker et al., 2017; Hurlemann and Grinevich, 2018; Field, 2019; Portnova et al., 2020; Uvnäs-Moberg et al., 2020; Uvnäs-Moberg and Petersson, 2022). In particular, the activation of the left hemispheric dlPFC indicates an important structure for emotional processing and regulation (Herrington et al., 2005; Nejati et al., 2021). Hence, we reject the theory that self-touch via the reafference principle cannot have any effects (Weiskrantz et al., 1971; Blakemore et al., 1998). Instead, we underline the argument that self-touch is of behavioral relevance (Synofzik, 2008; Boehme and Olausson, 2022). We, therefore, assume that repetitive self-touch is similarly involved in self-regulation as social touch via the OFC and the dlPFC.
Given that our findings shed light on the controversial discussion about the neuropsychological function of self-touch, it is necessary to define specific types of self-touch conditions to achieve a deeper understanding of the neuropsychological correlates of human behavior and enhance the reliability and interpretation of reported self-touch studies. Thus, repetitive self-touch on command appears to indicate self-regulation processes, while phasic self-touch does not. Contrary to this finding, Grunwald et al. (2014) revealed that “phasic-self-touch” under an implicit paradigm is involved in self-regulation. Therefore, further studies should distinguish between an implicit and an explicit self-touch paradigm, and for reliable data, behavioral analysis methods should be used. Furthermore, additional physiological data such as heart rate variability or respiration measurements could help quantify the self-regulative processes more precisely.
Besides the above-discussed limitations, the sample size (n = 52) constitutes a particular strength of this study. In fact, most studies investigated smaller samples (Grunwald et al., 2014; Herold et al., 2018; Kikuchi et al., 2018; Boehme et al., 2019). Additionally, we revealed the first study that controls the participants' performance with objective and reliable behavioral methods. Thus, the large sample size and the in-depth study of self-touch combined with behavioral analyses are strengths of the present study. Furthermore, our innovative experimental paradigm includes more naturalistic stimuli. The more naturalistic stimuli provide information about the natural movement behavior.
4.1 Conclusion
To summarize, the present study conducted an innovative and complex experimental paradigm using fNIRS in combination with motor–behavioral analyses. For the first time, hemodynamic responses during specific non-verbal human behaviors were investigated in a large sample size. The experiment provided information on how the brain works in more realistic environments. We quantified neural correlates in the OFC and dlPFC during repetitive and phasic self-touch conditions. These brain regions, which have been described as being associated with self-regulatory processes, were activated to be significantly stronger during repetitive than during phasic self-touch. Thus, the present findings indicate that repetitive self-touch has a stronger self-regulatory function than phasic self-touch.
Methodologically, the present study further demonstrates that, when investigating the neural correlates of behaviors, it is important to control the participants' performance with objective and reliable behavioral methods. Regarding exploring the neural correlates of irregular movements, future studies should investigate irregular self-touch in an implicit paradigm such as real-world environments.
Data availability statement
The original contributions presented in the study are included in the article/supplementary material, further inquiries can be directed to the corresponding author.
Ethics statement
The studies involving humans were approved by Local Ethics Committee of the German Sport University (Nr. 162/2022). The studies were conducted in accordance with the local legislation and institutional requirements. The participants provided their written informed consent to participate in this study. Written informed consent was obtained from the individual(s) for the publication of any potentially identifiable images or data included in this article.
Author contributions
SA: Conceptualization, Data curation, Formal analysis, Writing—original draft, Project administration. IH: Writing—review & editing, Formal analysis, Visualization. SK: Formal analysis, Writing—review & editing, Methodology. HL: Conceptualization, Supervision, Writing—review & editing.
Funding
The author(s) declare no financial support was received for the research, authorship, and/or publication of this article.
Acknowledgments
The authors would like to thank Armin Heinecke for useful support with running Satori. We would like to thank Robert Rein for his help running the R code. We would like to thank Valerie Akkaya for useful support with data acquisition. We acknowledge all participants for their contribution.
Conflict of interest
The authors declare that the research was conducted in the absence of any commercial or financial relationships that could be construed as a potential conflict of interest.
Publisher's note
All claims expressed in this article are solely those of the authors and do not necessarily represent those of their affiliated organizations, or those of the publisher, the editors and the reviewers. Any product that may be evaluated in this article, or claim that may be made by its manufacturer, is not guaranteed or endorsed by the publisher.
References
Bennett, R. H., Bolling, D. Z., Anderson, L. C., Pelphrey, K. A., and Kaiser, M. D. (2014). Fnirs detects temporal lobe response to affective touch. Soc. Cogn. Affect. Neurosci. 9, 470–476. doi: 10.1093/scan/nst008
Blakemore, S. J., Wolpert, D. M., and Frith, C. D. (1998). Central cancellation of self-produced tickle sensation. Nat. Neurosci. 1, 635–640. doi: 10.1038/2870
Boehme, R., Hauser, S., Gerling, G. J., Heilig, M., and Olausson, H. (2019). Distinction of self-produced touch and social touch at cortical and spinal cord levels. Proc. Natl. Acad. Sci. USA 116, 2290–2299. doi: 10.1073/pnas.1816278116
Boehme, R., and Olausson, H. (2022). Differentiating self-touch from social touch. Curr. Opin. Behav. 43, 27–33. doi: 10.1016/j.cobeha.2021.06.012
Brigadoi, S., and Cooper, R. J. (2015). How short is short? optimum source–detector distance for short-separation channels in functional near-infrared spectroscopy. Neurophotonics 2:025005. doi: 10.1117/1.NPh.2.2.025005
Case, L. K., Laubacher, C. M., Olausson, H., Wang, B., Spagnolo, P. A., and Bushnell, M. C. (2016). Encoding of touch intensity but not pleasantness in human primary somatosensory cortex. J. Neurosci. 36, 5850–5860. doi: 10.1523/JNEUROSCI.1130-15.2016
Chen, Y., Becker, B., Zhang, Y., Cui, H., Du, J., Wernicke, J., et al. (2020). Oxytocin increases the pleasantness of affective touch and orbitofrontal cortex activity independent of valence. Eur. Neuropsychopharmacol. 39, 99–110. doi: 10.1016/j.euroneuro.2020.08.003
Corbetta, M., and Shulman, G. L. (2002). Control of goal-directed and stimulus-driven attention in the brain. Nat. Rev. Neurosci. 3, 201–215. doi: 10.1038/nrn755
Crovitz, H. F., and Zener, K. (1962). A group-test for assessing hand- and eye-dominance. Am. J. Psychol. 75, 271–276. doi: 10.2307/1419611
Croy, I., Luong, A., Triscoli, C., Hofmann, E., Olausson, H., and Sailer, U. (2016). Interpersonal stroking touch is targeted to C tactile afferent activation. Behav. Brain Res. 297, 37–40. doi: 10.1016/j.bbr.2015.09.038
Densing, K., Konstantinidis, H., and Seiler, M. (2018). Effect of stress level on different forms of self-touch in pre- and postadolescent girls. J. Mot. Behav. 50, 475–485. doi: 10.1080/00222895.2017.1367640
Field, T. (2019). Social touch, CT touch and massage therapy: a narrative review. Dev. Rev. 51, 123–145. doi: 10.1016/j.dr.2019.01.002
Fishburn, F. A., Ludlum, R. S., Vaidya, C. J., and Medvedev, A. V. (2019). Temporal Derivative Distribution Repair (TDDR): a motion correction method for fNIRS. Neuroimage 184, 171–179. doi: 10.1016/j.neuroimage.2018.09.025
Freedman, N., and Bucci, W. (1981). On kinetic filtering in associative monologue. Semiotica 34, 225–250. doi: 10.1515/semi.1981.34.3-4.225
Freedman, N., O'Hanlon, J., Oltman, P., and Witkin, H. A. (1972). The imprint of psychological differentiation on kinetic behavior in varying communicative contexts. J. Abnorm. Psychol. 79, 239. doi: 10.1037/h0033162
Furley, P. (2021). The nature and culture of nonverbal behavior in sports: theory, methodology, and a review of the literature. Int. Rev. Sport Exerc. 1–26. doi: 10.1080/1750984X.2021.1894594
Gagnon, L., Perdue, K., Greve, D., Goldenholz, D., Kaskhedikar, G., and Boas, D. (2011). Improved recovery of the hemodynamic response in diffuse optical imaging using short optode separations and state-space modeling. Neuroimage 56, 1362–1371. doi: 10.1016/j.neuroimage.2011.03.001
Gordon, I., Voos, A. C., Bennett, R. H., Bolling, D. Z., Pelphrey, K. A., and Kaiser, M. D. (2013). Brain mechanisms for processing affective touch. Hum. Brain Mapp. 34, 914–922. doi: 10.1002/hbm.21480
Greenhouse, S. W., and Geisser, S. (1959). On methods in the analysis of profile data. Psychometrika 24, 95–112. doi: 10.1007/BF02289823
Grunwald, M., Weiss, T., Mueller, S., and Rall, L. (2014). EEG changes caused by spontaneous facial self-touch may represent emotion regulating processes and working memory maintenance. Brain Res. 1557, 111–126. doi: 10.1016/j.brainres.2014.02.002
Heinrichs, M., and Domes, G. (2008). Neuropeptides and social behaviour: effects of oxytocin and vasopressin in humans. Progress Brain Res. 170, 337–350. doi: 10.1016/S0079-6123(08)00428-7
Helmich, I., and Lausberg, H. (2014). Hand movements with a phase structure and gestures that depict action stem from a left hemispheric system of conceptualization. Exp. Brain Res. 232, 3159–3173. doi: 10.1007/s00221-014-4006-x
Helmich, I., Meyer, C., Voelk, M., Coenen, J., Mueller, S., Schepmann, J., et al. (2022). The pantomime of mental rotation: left-handers are less lateralized. Neuropsychologia 176:108385. doi: 10.1016/j.neuropsychologia.2022.108385
Helmich, I., Skomroch, H., and Lausberg, H. (2014). Neuropsychological functions of hand movements and gestures change in the presence or absence of speech. Cogn. Psychol. 26, 740–753. doi: 10.1080/20445911.2014.961925
Herold, F., Wiegel, P., Scholkmann, F., and Müller, N. G. (2018). Applications of functional near-infrared spectroscopy (fNIRS) neuroimaging in exercise–cognition science: a systematic, methodology-focused review. J. Clin. Med. 7:466. doi: 10.3390/jcm7120466
Herrington, J. D., Mohanty, A., Koven, N. S., Fisher, J. E., Stewart, J. L., Banich, M. T., et al. (2005). Emotion-modulated performance and activity in left dorsolateral prefrontal cortex. Emotion 5:200. doi: 10.1037/1528-3542.5.2.200
Heubach, T. (2016). Effects of cognitive stress on hand movement behavior – can we improve our performance by hand movements during cognitive testing? (master's thesis). Cologne, German Sport University Cologne.
Holle, H., and Rein, R. (2015). EasyDIAg: a tool for easy determination of interrater agreement. Behav. Res. Methods 47, 837–847. doi: 10.3758/s13428-014-0506-7
Hove, M. J., and Stelzer, J. (2018). Biological foundations and beneficial effects of trance. Behav. Brain Sci. 41:e76. doi: 10.1017/S0140525X17002072
Hurlemann, R., and Grinevich, V. (2018). Behavioral Pharmacology of Neuropeptides: Oxytocin. Current Topics in Behavioral Neurosciences. Cham: Springer International Publishing AG. doi: 10.1007/978-3-319-63739-6
Huys, R., Studenka, B. E., Rheaume, N. L., Zelaznik, H. N., and Jirsa, V. K. (2008). Distinct timing mechanisms produce discrete and continuous movements. PLoS Comput. Biol. 4:e1000061. doi: 10.1371/journal.pcbi.1000061
Ishak, W. W., Kahloon, M., and Fakhry, H. (2011). Oxytocin role in enhancing well-being: a literature review. J. Affect. Disord. 130, 1–9. doi: 10.1016/j.jad.2010.06.001
Jasper, H. H. (1958). The ten-twenty electrode system of the international federation. Electroencephalogr. Clin. Neurophysiol. 10, 371–375.
Kashou, N. H., and Giacherio, B. M. (2016). Stimulus and optode placement effects on functional near-infrared spectroscopy of visual cortex. Neuropho. 3:e025005. doi: 10.1117/1.NPh.3.2.025005
Kikuchi, Y., Shirato, M., Machida, A., Inoue, T., and Noriuchi, M. (2018). The neural basis of self-touch in a pain-free situation. Neuropsychiatry 8, 186–196. doi: 10.4172/Neuropsychiatry.1000340
Kinsbourne, M. (2011). “Repetitive movements and arousal,” in The Neuropsychology of Autism, ed D. A. Fein (New York, NY: Oxford University Press), 367–394.
Konczak, J., and Winter, L. (2020). “Grundlagen zur Neurophysiologie der Motorik,” in Bewegung, Training, Leistung und Gesundheit, eds A. Güllich and M. Krüger (Berlin, Heidelberg: Springer). doi: 10.1007/978-3-662-53386-4_57-1
Kreyenbrink, I., Joraschky, P., Konstantinidis, I., Neumann, N., and Lausberg, H. (2017). Nonverbales Verhalten von Patienten mit sozialen Phobien und ihren Therapeuten in psychodynamischen Psychotherapien (Teilprojekt SOPHO-NET). Z. Psychosom. Med. Psychother. 63, 297–313. doi: 10.13109/zptm.2017.63.3.297
Lausberg, H. (2013). Understanding Body Movement: A Guide to Empirical Research on Nonverbal Behaviour: With An Introduction to the NEUROGES Coding System. Berlin: Peter Lang GmbH.
Lausberg, H. (2019). The NEUROGES® Analysis System for Nonverbal Behavior and Gesture. Berlin: Peter Lang GmbH. doi: 10.3726/b15103
Lausberg, H. (2022). Der Körper in der Psychotherapie. Stuttgart: Verlag W. Kohlhammer. doi: 10.17433/978-3-17-030148-1
Li, Q., Becker, B., Wernicke, J., Chen, Y., Zhang, Y., Li, R., et al. (2019). Foot massage evokes oxytocin release and activation of orbitofrontal cortex and superior temporal sulcus. Psychoneuroendocrinology 101, 193–203. doi: 10.1016/j.psyneuen.2018.11.016
Lin, Y., Pi, Y., Wang, Y., Xia, X., Qiu, F., Cao, N., et al. (2022). Dorsolateral prefrontal cortex mediates working memory processes in motor skill learning. Psychol. Sport Exerc. 59:102129. doi: 10.1016/j.psychsport.2021.102129
Love, T. M. (2014). Oxytocin, motivation and the role of dopamine. Pharmacol. Biochem. Behav. 119, 49–60. doi: 10.1016/j.pbb.2013.06.011
Lührs, M., and Goebel, R. (2017). Turbo-Satori: a neurofeedback and brain-computer interface toolbox for real-time functional near-infrared spectroscopy. Neurophotonics 4:041504. doi: 10.1117/1.NPh.4.4.041504
McGlone, F., Olausson, H., Boyle, J. A., Jones-Gotman, M., Dancer, C., Guest, S., et al. (2012). Touching and feeling: differences in pleasant touch processing between glabrous and hairy skin in humans. Eur. J. Neurosci. 35, 1782–1788. doi: 10.1111/j.1460-9568.2012.08092.x
Miller, E. K., and Cohen, J. D. (2001). An integrative theory of prefrontal cortex function. Annu. Rev. Neurosci. 24, 167–202. doi: 10.1146/annurev.neuro.24.1.167
Morais, G. A. Z., Balardin, J. B., and Sato, J. R. (2018). Fnirs Optodes' Location Decider (fOLD): a toolbox for probe arrangement guided by brain regions-of-interest. Sci. Rep. 8, 3341. doi: 10.1038/s41598-018-21716-z
Morita, I., Sakuma, S., Shimomura, J., Hayashi, N., and Toda, S. (2018). Brain activity in response to the touch of a hand on the center of the back. PLoS ONE 13:e0206451. doi: 10.1371/journal.pone.0206451
Mueller, S. M., Martin, S., and Grunwald, M. (2019). Self-touch: contact durations and point of touch of spontaneous facial self-touches differ depending on cognitive and emotional load. PLoS ONE 14:e0213677. doi: 10.1371/journal.pone.0213677
Nagai, Y., Critchley, H. D., Featherstone, E., Trimble, M. R., and Dolan, R. J. (2004). Activity in ventromedial prefrontal cortex covaries with sympathetic skin conductance level: a physiological account of a “default mode” of brain function. Neuroimage 22, 243–251. doi: 10.1016/j.neuroimage.2004.01.019
Nejati, V., Khalaji, S., Goodarzi, H., and Nitsche, M. (2021). The role of ventromedial and dorsolateral prefrontal cortex in attention and interpretation biases in individuals with general anxiety disorder (GAD): a tDCS study. J. Psychiatr. Res. 144, 269–277. doi: 10.1016/j.jpsychires.2021.10.034
Neumann, N., Drewes, V., Konstantinidis, I., Reinecke, K. H. C., Lausberg, H., and Helmich, I. (2022). Neuropsychological functions of nonverbal hand movements and gestures during sports. J. Cogn. Psychol. 34, 362–374. doi: 10.1080/20445911.2021.1998075
Olausson, H., Wessberg, J., Morrison, I., and McGlone, F. (2016). Affective Touch and the Neurophysiology of CT Afferents. New York, NY: Springer New York. doi: 10.1007/978-1-4939-6418-5
Peirce, J., Gray, J. R., Simpson, S., MacAskill, M., Höchenberger, R., Sogo, H., et al. (2019). PsychoPy2: experiments in behavior made easy. Behav. Res. Methods. 51, 195–203. doi: 10.3758/s13428-018-01193-y
Pfeifer, A.-C., Ditzen, B., Neubauer, E., and Schiltenwolf, M. (2016). Wirkung von oxytocin auf das menschliche Schmerzerleben. Schmerz. 30, 457–469. doi: 10.1007/s00482-016-0164-z
Pinti, P., Aichelburg, C., Gilbert, S., Hamilton, A., Hirsch, J., Burgess, P., et al. (2018). A review on the use of wearable functional near-infrared spectroscopy in naturalistic environments. Jpn. Psychol. Res. 60, 347–373. doi: 10.1111/jpr.12206
Plichta, M. M., Herrmann, M. J., Baehne, C. G., Ehlis, A. C., Richter, M. M., Pauli, P., et al. (2007). Event-related functional near-infrared spectroscopy (fNIRS) based on craniocerebral correlations: reproducibility of activation?. Hum. Brain Mapp. 28, 733–741. doi: 10.1002/hbm.20303
Pollonini, L., Bortfeld, H., and Oghalai, J. S. (2016). PHOEBE: a method for real time mapping of optodes-scalp coupling in functional near-infrared spectroscopy. Biomed. Opt. Express 7, 5104–5119. doi: 10.1364/BOE.7.005104
Portnova, G. V., Proskurnina, E. V., Sokolova, S. V., Skorokhodov, I. V., and Varlamov, A. A. (2020). Perceived pleasantness of gentle touch in healthy individuals is related to salivary oxytocin response and EEG markers of arousal. Exp. Brain Res. 238, 2257–2268. doi: 10.1007/s00221-020-05891-y
Quirin, M., Kuhl, J., and Düsing, R. (2011). Oxytocin buffers cortisol responses to stress in individuals with impaired emotion regulation abilities. Psychoneuroendocrinology 36, 898–904. doi: 10.1016/j.psyneuen.2010.12.005
R Core Team (2022). R: A Language and Environment for Statistical Computing. Vienna: R Foundation for Statistical Computing. Available online at: https://www.R-project.org/
Reinecke, K. C. H., Dvoretska, D., Joraschky, P., and Lausberg, H. (2020). Fidgeting behavior during psychotherapy: hand movement structure contains information about depressive symptoms. J. Contemp. Psychother. 50, 323–329. doi: 10.1007/s10879-020-09465-5
Reinecke, K. C. H., Joraschky, P., and Lausberg, H. (2022). Hand movements that change during psychotherapy and their relation to therapeutic outcome: an analysis of individual and simultaneous movements. Psychother. Res. 32, 117–127. doi: 10.1080/10503307.2021.1925989
Rolls, E. T., O'Doherty, J., Kringelbach, M. L., Francis, S., Bowtell, R., and McGlone, F. (2003). Representations of pleasant and painful touch in the human orbitofrontal and cingulate cortices. Cereb. Cortex. 13, 308–317. doi: 10.1093/cercor/13.3.308
Sailer, U., Triscoli, C., Häggblad, G., Hamilton, P., Olausson, H., and Croy, I. (2016). Temporal dynamics of brain activation during 40 minutes of pleasant touch. Neuroimage 139, 360–367. doi: 10.1016/j.neuroimage.2016.06.031
Schaal, S., Sternad, D., Osu, R., and Kawato, M. (2004). Rhythmic arm movement is not discrete. Nat. Neurosci. 7, 1136–1143. doi: 10.1038/nn1322
Scheele, D., Kendrick, K. M., Khouri, C., Kretzer, E., Schläpfer, T. E., Stoffel-Wagner, B., et al. (2014). An oxytocin-induced facilitation of neural and emotional responses to social touch correlates inversely with autism traits. Neuropsychopharmacol. 39, 2078–2085. doi: 10.1038/npp.2014.78
Skomroch, H., Petermann, K., Helmich, I., Dvoretska, D., Rein, R., Kim, Z. H., et al. (2013). “Gender Differences in Hand Movement Behavior,” in Poster presented at the Tilburg Gesture Research Meeting (TiGeR). Available online at: tiger.uvt.nl/pdf/papers/skomroch.pdf
Spencer, R. M., Zelaznik, H. N., Diedrichsen, J., and Ivry, R. B. (2003). Disrupted timing of discontinuous but not continuous movements by cerebellar lesions. Science 300, 437–1439. doi: 10.1126/science.1083661
Sudeck, G., and Thiel, A. (2020). “Sport, Wohlbefinden und psychische Gesundheit,” in Sportpsychologie: Grundlagen und Anwendung, eds J. Schüler, M. Wegner, and H. Plessner (Berlin, Heidelberg: Springer Verlag), 551–579. doi: 10.1007/978-3-662-56802-6_24
Synofzik, M. B. (2008). Die Rolle interner Modelle bei der Wahrnehmung von Eigenbewegungen (dissertation). Tübingen: Universität Tübingen.
Tachtsidis, I., and Scholkmann, F. (2016). False positives and false negatives in functional near-infrared spectroscopy: issues, challenges, and the way forward. Neurophotonics 3:031405. doi: 10.1117/1.NPh.3.3.031405
Uvnäs-Moberg, K., Handlin, L., and Petersson, M. (2020). Neuroendocrine mechanisms involved in the physiological effects caused by skin-to-skin contact - with a particular focus on the oxytocinergic system. Infant Behav. Dev. 61:101482. doi: 10.1016/j.infbeh.2020.101482
Uvnäs-Moberg, K., and Petersson, M. (2022). Physiological effects induced by stimulation of cutaneous sensory nerves, with a focus on oxytocin. Curr. Opin. Behav. 43, 159–166. doi: 10.1016/j.cobeha.2021.10.001
van Mourik, A. M., and Beek, P. J. (2004). Discrete and cyclical movements: unified dynamics or separate control? Acta Psychol. 117, 121–138. doi: 10.1016/j.actpsy.2004.06.001
von Lühmann, A., Zheng, Y., Ortega-Martinez, A., Kiran, S., Somers, D. C., Cronin-Golomb, A., et al. (2021). Towards Neuroscience of the Everyday World (NEW) using functional near-infrared spectroscopy. Curr. Opin. Biomed. Eng. 18:100272. doi: 10.1016/j.cobme.2021.100272
Walker, S. C., Trotter, P. D., Swaney, W. T., Marshall, A., and Mcglone, F. P. (2017). C-tactile afferents: cutaneous mediators of oxytocin release during affiliative tactile interactions? Neuropeptides 64, 27–38. doi: 10.1016/j.npep.2017.01.001
Weiskrantz, L., Elliott, J., and Darlington, C. (1971). Preliminary observations on tickling oneself. Nature 230, 598–599. doi: 10.1038/230598a0
Yücel, M., Selb, J., Aasted, C., Petkov, M., Becerra, L., Borsook, D., et al. (2015). Short separation regression improves statistical significance and better localizes the hemodynamic response obtained by near-infrared spectroscopy for tasks with differing autonomic responses. Neurophotonics 2:035005. doi: 10.1117/1.NPh.2.3.035005
Yücel, M. A., Lühmann, A. V., Scholkmann, F., Gervain, J., Dan, I., Ayaz, H., et al. (2021). Best practices for fNIRS publications. Neurophotonics 8:12101. doi: 10.1117/1.NPh.8.1.012101
Keywords: self-touch, prefrontal activity, left orbitofrontal cortex, left dorsolateral prefrontal cortex, functional near-infrared spectroscopy
Citation: von Au S, Helmich I, Kieffer S and Lausberg H (2023) Phasic and repetitive self-touch differ in hemodynamic response in the prefrontal cortex–An fNIRS study. Front. Neuroergon. 4:1266439. doi: 10.3389/fnrgo.2023.1266439
Received: 24 July 2023; Accepted: 24 October 2023;
Published: 29 November 2023.
Edited by:
Jack Adam Noah, Yale University, United StatesCopyright © 2023 von Au, Helmich, Kieffer and Lausberg. This is an open-access article distributed under the terms of the Creative Commons Attribution License (CC BY). The use, distribution or reproduction in other forums is permitted, provided the original author(s) and the copyright owner(s) are credited and that the original publication in this journal is cited, in accordance with accepted academic practice. No use, distribution or reproduction is permitted which does not comply with these terms.
*Correspondence: Sabrina von Au, cy52b25hdUBkc2hzLWtvZWxuLmRl