- 1Department of Biological and Medical Sciences, Oxford Brookes University, Oxford, United Kingdom
- 2Department of Clinical Neurosciences, John Radcliffe Hospital, Oxford, United Kingdom
- 3Nuffield Department of Surgical Sciences, University of Oxford, Oxford, United Kingdom
Better understanding of breathlessness perception addresses an unmet clinical need for more effective treatments for intractable dyspnoea, a prevalent symptom of multiple medical conditions. The insular-cortex is predominantly activated in brain-imaging studies of dyspnoea, but its precise role remains unclear. We measured experimentally-induced hypercapnic air-hunger in three insular-glioma patients before and after surgical resection. Tests involved one-minute increments in inspired CO2, raising end-tidal PCO2 to 7.5 mmHg above baseline (38.5 ± 5.7 mmHg), whilst ventilation was constrained (10.7 ± 2.3 L/min). Patients rated air-hunger on a visual analogue scale (VAS). Patients had lower stimulus–response (2.8 ± 2 vs. 11 ± 4 %VAS/mmHg; p = 0.004), but similar threshold (40.5 ± 3.9 vs. 43.2 ± 5.1 mmHg), compared to healthy individuals. Volunteered comments implicated diminished affective valence. After surgical resection; sensitivity increased in one patient, decreased in another, and other was unable to tolerate the ventilatory limit before any increase in inspired CO2.We suggest that functional insular-cortex is essential to register breathlessness unpleasantness and could be targeted with neuromodulation in chronically-breathless patients. Neurological patients with insula involvement should be monitored for blunted breathlessness to inform clinical management.
Introduction
James Parkinson stated in his seminal paper on the ‘shaking palsy’ in 1817 that the patient ‘seemed to catch their breath rather hard’ (Parkinson, 2002) yet monitoring of breathlessness in management of neurological patients is rare. Dyspnoea is a complaint of seriously ill hospitalised patients, especially those with cardiopulmonary conditions (Edmonds et al., 2001; Hutchinson et al., 2018). Within the Nuffield department of surgical sciences at Oxford, we noticed cases of new-onset breathlessness, or relief of existing breathlessness, before and after brain surgery. The insular-cortex of the limbic system has been universally reported to be predominantly activated in functional brain-imaging studies of experimentally-induced breathlessness in healthy individuals, insinuating a principal role in breathlessness perception (Banzett et al., 2000; Evans et al., 2002). To progress our understanding of the neural mechanisms of breathlessness, we applied established techniques (Grogono et al., 2018) to test breathlessness sensitivity in patients with low-grade insula-gliomas, before and after surgical resection of the gliomas. We focused on air-hunger (AH) defined as an uncomfortable urge to breathe which is a particularly unpleasant component of clinical dyspnoea (Banzett et al., 2008).
Materials and methods
Three consecutive cases of low-grade insula-glioma (two males, 41 ± 4 years., 181 ± 13 cm height, and 82 ± 40 kg weight at pre-surgery testing) awaiting surgery, were selected. Patients attended a single test session before and at least 5 months after surgical resection of their glioma. NRES South Central-Oxford C provided ethics approval (REC ref.: 11/SC/0229), and patients gave written informed consent.
Experimentally induced air hunger
Hypercapnia was used with constrained ventilation to induce AH, an established experimental model for inducing the AH component of clinical dyspnoea (O’donnell et al., 2013; Banzett et al., 2021). Participants breathed via a circuit that separated inspiration from expiration (Figure 1). An inspiratory reservoir provided the only source of inspiration (3-litre anaesthetic bag). A fixed flow of humidified air was fed into the bag to match resting ventilation, and patients breathed in time with a metronome to match respiratory frequency, thus fixing tidal volume. To induce AH, up to 7% CO2 was added to the bag using an air-oxygen blender supplied by gas cylinders containing 10%CO2 in air, and medical air. Participants rated AH using a 10cm visual analogue scale (VAS), cued by a LED every 15s.
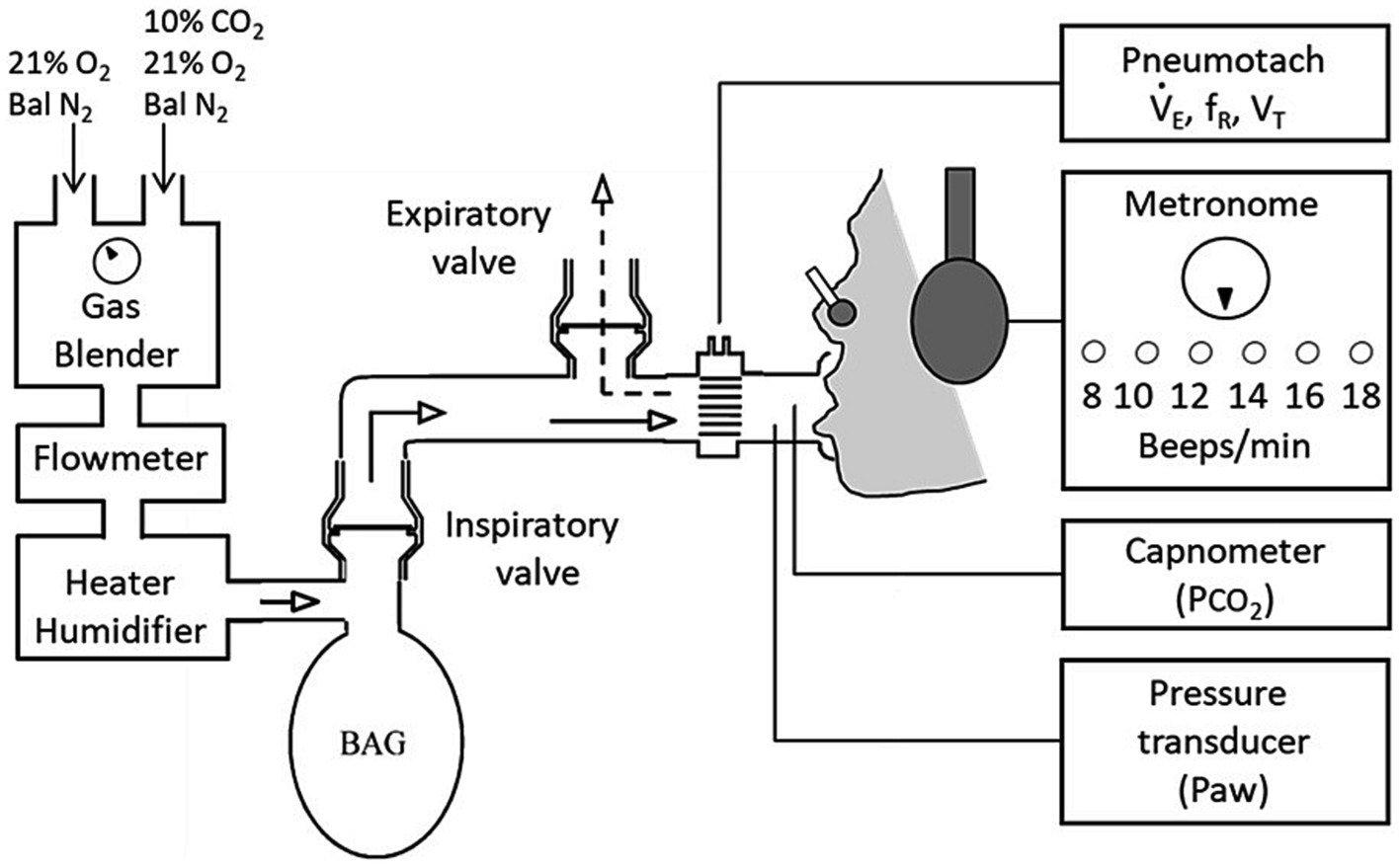
Figure 1. Experimental setup and protocol. Experimental setup: Participants breathed via a mouthpiece from a 3 L anaesthetic bag into which the flow of fresh gas was set to the participants’ baseline minute ventilation (VE). A Metronome was used to set breathing frequency (fR) to the participants’ spontaneous rate at baseline.
Study design
An initial familiarisation hypercapnic ‘ramp’ test involved 1-min increments of 1.3% inspired CO2 that raised end-tidal PCO2 (PETCO2) by between 2 and 3 mmHg/min whilst participants rated any breathing discomfort. Extremes of the VAS were labelled ‘none’ (no breathing discomfort) and ‘extreme’ (the tolerable limit). End-point was when tolerance was reached and participants came off the mouthpiece or PETCO2 reached 60 mmHg. Debriefs involved; (i) asking patients to describe their breathing when they had rated high, (ii) presenting a list of respiratory descriptors for participants to select the three most applicable to ensure that they could distinguish AH (i.e., urge to breathe, shortness of breath, feeling of suffocation/smothering). Following debrief, the test was repeated with participants instructed to exclusively rate AH.
Data processing and analysis
Analogue signals were digitised and recorded for offline analysis (Spike 2, Cambridge Electronic Design, United Kingdom). Breath-by-breath PETCO2 was processed with a 60s moving average to account for delay in AH response dynamics (Banzett et al., 1996). AH ratings were plotted against change in PETCO2 from that at AH-threshold determined by visual inspection of individual scatter plots.
The pooled scatter for the stimulus response data from 10 healthy individuals (mean ± sd age 25 ± 3 years, height was 169 ± 12 cm, and weight was 70 ± 23 kg) was extracted from a previous study using the same protocol (Grogono et al., 2018) and plotted alongside the stimulus response data for the patients to determine the overlap in scatter. The x-axis was normalised for the PCO2 at individual AH thresholds to align the individual stimulus response slopes. The AH threshold was determined by visual inspection of individual stimulus response curves (see Supplementary Figure 2). As no AH threshold was evident for case 1, the average AH threshold in the other two patients was assumed in order to include the data points for case 1 in this plot.
Individual air hunger sensitivities were also determined by linear regression through individual stimulus response scatter normalised for AH threshold. The average AH sensitivity (slope) between patients (n = 3) and healthy controls (n = 10) was compared using an unpaired t-test. The same t-test was also applied to the comparison of the group mean AH threshold for each group.
Brain imaging processing and analysis
T2-weighted FLAIR MRIs were imported into 3D-Slicer (Fedorov et al., 2012). A semi-automated segmentation editor within 3D-slicer was used to generate 3D images of the glioma and generate reports on its volume, shown to be an accurate alternative to manual segmentation by experienced consultants (Egger et al., 2013). The insular cortex was manually segmented on the healthy hemisphere and subsequently flipped to generate bilateral-segmented insular, assuming symmetry (Figure 2). Glioma details were obtained from clinical notes. Pre-surgery and post-surgery FLAIR scans were acquired within 1 year and 1 month of surgery, respectively. Clinical notes specified there was no change in size of glioma from the between pre-surgery scan and surgery.
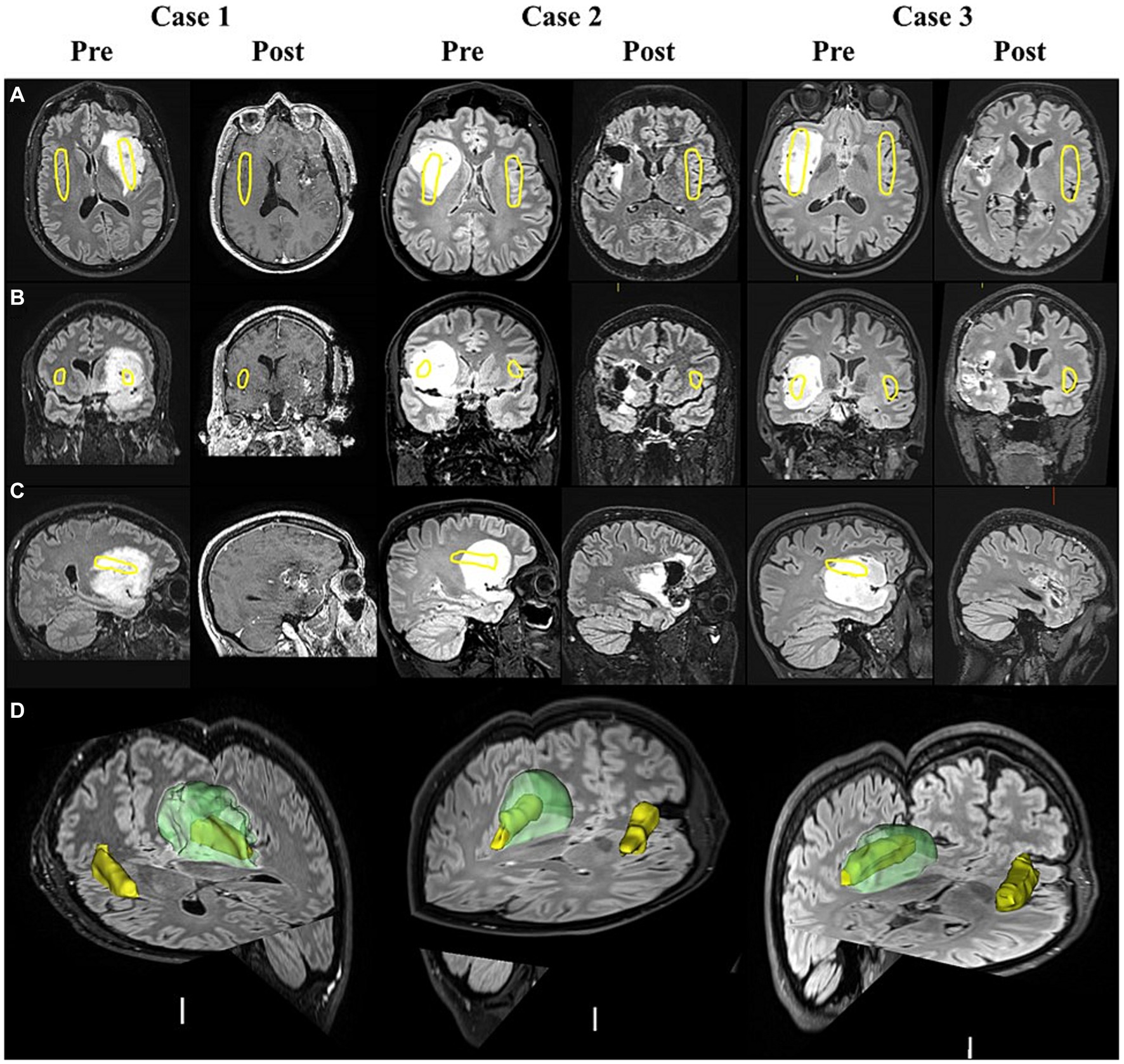
Figure 2. MRI scans of insula-glioma pre- and post-surgery. T2 weighted FLAIR MRI images displayed in the axial (A), coronal (B), and sagittal (C) planes before surgery (Pre) and after surgery (Post) for cases 1 to 3. Case-1 did not have a post-surgery FLAIR scan as they were isolating abroad during the COVID-19 pandemic and therefore available post-surgery T1 weighted MRI scans were used (48 months before post-surgery testing). Yellow outlines represent the positioning of the insular cortex. Panel (D) displays the segmented insula (yellow) and glioma (Green) generated using pre-surgery FLAIR scans in 3D slicer for each case.
Results
Interval between diagnosis and surgical resection averaged 7 ± 1 months, and that between pre and post-surgery testing was 48, 9 and 7 months, respectively (Table 1). Demographics and pre- and post-MRI scans are provided in Table 1 for each case. Post-surgery weight and medications for each case were as follows: Case 1; 111 kg, Keppra. Case 2; 65 kg, Keppra. Case 3; 47 kg, Lacosamide.
Figure 3A shows that there is little overlap between the scatter of the pooled stimulus–response data from healthy controls and that of the 3 patients. Mean ± sd individual sensitivity was significantly lower in patients pre surgery (2.8 ± 2 vs. 11.0 ± 4 %VAS/mmHg; p = 0.004, students unpaired t-test; Figure 3B, left). However, the group difference in AH thresholds did not achieve statistical significance (Figure 3B, right). After surgery, Case-2 recovered some AH sensitivity (3.9 to 8.0%VAS/mmHg), returning to within the 95% predicted normal range (Figure 3C, middle). Case-3 had a substantial further reduction in AH sensitivity (4.3 to 0.9%VAS/mmHg; Figure 3C, right). Since Case-1 did not rate any AH during the pre-surgery test, we assumed an AH threshold as the average of that seen in Case-2 and Case-3 in order to plot Case-1 in Figure 3C (left). We could not do the same for the post-surgery data in Case-1 because they terminated the test before any change in PETCO2.
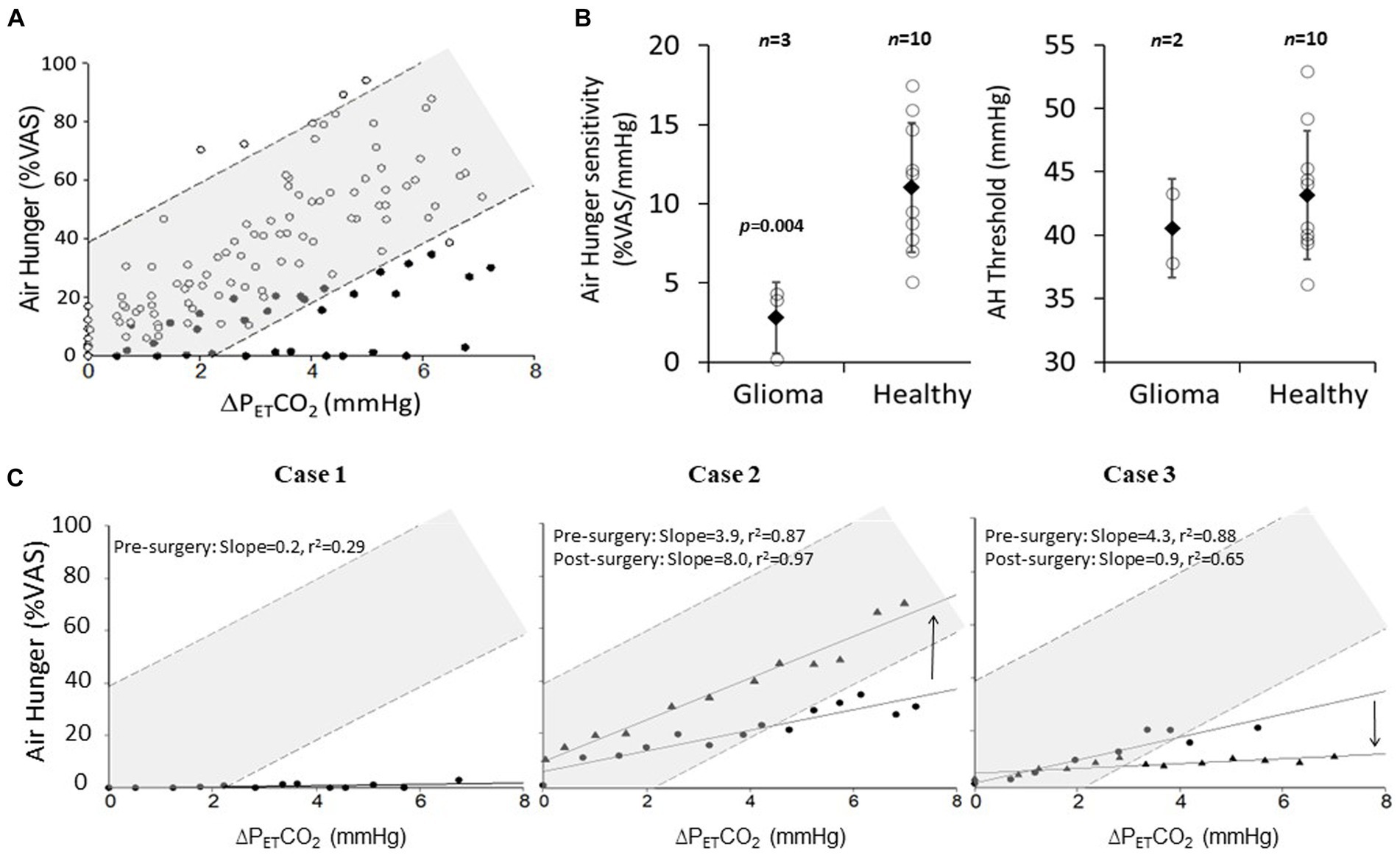
Figure 3. The effect of low-grade insula-glioma and its removal on air hunger (AH) sensitivity. (A) Pooled AH VAS ratings plotted against change in PETCO2 above threshold AH for 3 patients with insula-glioma (closed circles) and 10 healthy-controls (open circles). Breath-by-breath PETCO2 was processed with a 60 s moving average to account for delay in AH response dynamics (5). AH threshold was determined visually from individual slopes. Light shading shows 95% prediction intervals. Normative data was extracted from a previous publication using the same protocol (3). (B) Mean ± sd (closed diamonds) of individual AH sensitivities (left) and thresholds (right) for pre-surgery glioma patients (n = 3 for left and n = 2 for right) and healthy controls (n = 10). Individual scatter around these means are also shown (open circles). Average AH sensitivity was lower in patients (*p = 0.004; unpaired Student’s t-test). (C) AH plotted against change in PETCO2 above AH threshold for pre-surgery (circles) and post-surgery (triangles). Case-1 (left) did not rate any AH pre-surgery, the threshold was assumed to be the average of Cases-2 and 3 for inclusion in this figure. Case 1 could not tolerate the post-surgery test. 95% prediction and 95% confidence intervals for the pooled healthy control data is represented on these graphs by the darker and lighter shaded regions. Direction of arrows for case 2 and 3 represent whether AH sensitivity has increased or decreased.
Discussion
Low-grade insula-glioma appears to blunt AH sensitivity without rightward shift in threshold, and its resection can increase or reverse this effect. Gliomas can compress, displace, and infiltrate brain tissue; generally, without destroying grey or white matter or eliciting neurological deficits (Whittle, 2004). However, blunted AH implies damage to the insula’s neural fabric with a hidden functional deficit only manifested if breathlessness pre-exists or is measured.
The insular receives a multitude of visceral afferents (Gogolla, 2017), is activated in brain-imaging studies of unpleasant sensations including pain (Duerden and Albanese, 2013) and breathlessness (Banzett et al., 2000; Evans et al., 2002), and if lesioned, results in alterations in pain (Starr et al., 2009). One previous study compared the dyspnoea associated with inspiratory resistive loading between four patients with ischaemic stroke of the insula, and healthy controls (Schon et al., 2008). A reduction in intensity and unpleasantness of dyspnoea was reported in the patients. However, this conclusion was unconvincing as it was based upon change from baseline dyspnoea to the dyspnoea with applied respiratory stimulus, and the patients had elevated baseline levels of dyspnoea (Adams et al., 2009). In contrast none of our patients rated any AH at normocapnia which might suggest that glioma and ischaemia of the insular may have different impact on its functionality. Our data on the other hand, provides clear evidence that a more unpleasant form of dyspnoea (air hunger) is reduced in patients with insular glioma.
Possible mechanism of blunted perception
The fact that we did not observe a significant difference in AH threshold may imply that there is no impact of the insular glioma on the gating of the AH signal which is thought to take place in the thalamus or brainstem. However, the blunted sensitivity may imply that the neural processing of this signal at the insular has been affected. We discuss below several possible explanations for complete absence of AH sensitivity pre-surgery in Case-1, compared to diminished sensitivity in Cases 2 and 3:
i. Case-1 glioma engulfed the entire right insular whereas the tip of the left posterior insular extended beyond the glioma in Cases 2 and 3 (Table 1). This could account for some preserved AH sensitivity in cases 2 and 3. Pain research suggests that sensory-intensity is processed in posterior, and sensory-affect in anterior insular (Gogolla, 2017). By this token, AH with high affective valence is more likely to associate with anterior insular function consistent with brain imaging studies. However, involvement of anterior insular was not different between case 1 and the others.
ii. A more likely explanation for the complete absence of AH sensitivity in Case-1 is that they had right-sided glioma. Brain-imaging of dyspnoea showed greater activity in the right insular (Banzett et al., 2000). We cannot discount that Case-1 had naturally elevated AH threshold, but most healthy individuals rate close to full-scale at the highest hypercapnia we imposed (49 mmHg; Banzett et al., 1996).
iii. The glioma in Case-1 extended into the amygdala, which is linked to fear, anxiety, and breathlessness in COPD (Esser et al., 2017). After the pre-surgery test, Case-1 stated; ‘I knew my breathing was not right, but it did not bother me at all’, suggesting specific impact on the affective domain. This is consistent with absent hypercapnia induced dyspnoea in patients where epileptic seizures extending to the amygdala and in two cases of focal electrical stimulation (50 Hz) of the amygdala (Dlouhy et al., 2015).
iv. Glioma in Case-1 was an oligodendroglioma and an astrocytoma in the others. We speculate whether disruption of neuronal insulation by the oligodendroglioma has greater impact on sensitivity than disruption of the supply of nutrients and structural support by astrocytomas.
Effect of surgical resection on AH sensitivities
Post-surgery, Case-1 terminated the test before any inspired CO2 load. We suspect that during the post-surgery test ventilatory constraint acted as a cue for intolerance, consistent with their debrief comment; ‘I cannot breathe, so obviously I am breathless’. Recovery of AH-sensitivity post-surgery in Case-2 (Figure 3C, middle) may reflect cross-hemispheric neuroplasticity with heightened function of the unaffected right-insular during the 9 months between pre and post-surgery testing. We cannot exclude the possibility that some residual functioning insular may have survived resection, but since most of the left-insular was removed neuroplasticity of the right insular is more likely responsible for recovery of sensitivity. The further reduction in AH sensitivity post-surgery in Case-3 is supported by the fact that this patient, who had resting breathlessness before surgery, stated that after surgery this had ‘completely disappeared’ and ‘breathing now feels very easy, as if the air is lighter’. Although neuroplasticity of unaffected insular could occur within 5-months (Duffau, 2020), Case-3 had no improvement despite 7-months between testing. This patient had further reduction in sensitivity post-surgery, we propose that residual functioning insular tissue in the glioma was lost in resection.
Clinical implications
The belief that low-grade gliomas (whether compressive or infiltrative) are not commonly associated with neurological deficit may need to be re-visited based on our findings that the ability to sense unpleasant symptoms such as air hunger may be lost. This may otherwise be classed as ‘no neurological deficits’ unless specific testing is conducted, or disappearance of pre-existing symptoms are observed.
Insular lesions are commonly associated with dysphagia. Blunted breathlessness perception alongside this may compromise patients’ ability to detect aspiration-pneumonia, pulmonary embolism, and upper-respiratory tract infections delaying treatment and exacerbating the condition. Post-mortem records of 30 patients with pulmonary embolism after stroke (Wijdicks and Scott, 1997), revealed sudden-death had occurred in half the patients but pleuritic pain and dyspnoea were major symptoms only in the others. A hitherto unexplored possibility is that blunted dyspnoea played a role in the fatal outcome of the half who died suddenly, consistent with a suggested decrease in symptom perception in patients with a history of near fatal asthma attacks (Kikuchi et al., 1994). We also cannot comment on whether blunted AH sensitivity is specific for insular-glioma or whether other sensory modalities are affected.
Limitations
This case series is limited primarily in the small number of cases we were able to study. We compared the individual stimulus responses in these three cases with that of 10 healthy control datasets extracted from a previous study from our lab (Grogono et al., 2018). Since we only have three cases our use of an unpaired t-test to compare the mean AH sensitivity and threshold between groups (Figure 3B) may be deemed inappropriate even though the data passed the Shapiro Wilks test of normality. Putting aside the statistics that we have presented, the individual scatter for the AH sensitivities in the healthy individuals does not overlap with that of the corresponding scatter for the patients (Figure 3B, left).
The patient group was older, taller and heavier on average than the healthy controls. To check if this difference in group demographics could account for the group difference in average slope, we selected the 3 healthy controls who were closest matched with respect to sex, age, height, weight and BMI. The revised average height, weight and BMI compared well between groups: 181 vs. 181 cm, 82 vs. 90 kg, 26.8 vs. 24.3 kg/m2, respectively. To check if the AH slopes in these better-matched healthy controls remain substantially steeper than that in the patients, we re-plotted Figure 3A with just the 3 best-matched healthy controls (Supplementary Figure 1); this revised figured showed that the pooled healthy control data had an even steeper response slope (15.5 %VAS/mmHg).
Age however remained significantly younger for the healthy controls when only including the three best-matched controls (27 vs. 41 years; p = 0.009, unpaired two-tailed t-test). We cannot exclude the possibility that age could have an effect in reducing the slope of the hypercapnic air hunger response -however this is unlikely based on previous studies that have reported slopes of dyspnoea responses in older individuals using the same experimental model (hypercapnia with constrained ventilation). For example, the oldest participant in the study by Banzett et al. (2021) was 57 years old (older than our oldest patient) and yet they had the second highest response slope (9.4 mm/mmHg).
Conclusion
In conclusion, glioma of the insular cortex blunted breathlessness, resonating with reports of insular activation in brain-imaging studies. The differential effects of surgical resection on breathlessness sensitivity may be explained by compensatory increases in insular activity in the healthy hemisphere over time, and acute loss of residual functioning tissue by resection. This case-series advocates; (i) monitoring breathlessness in patients with insular-glioma, (ii) targeting insular with neuromodulation for symptomatic relief of intractable dyspnoea in cardiopulmonary conditions.
Data availability statement
The raw data supporting the conclusions of this article will be made available by the authors, without undue reservation.
Ethics statement
The studies involving humans were approved by NRES South Central Oxford C (REC ref.:11/SC/0229). The studies were conducted in accordance with the local legislation and institutional requirements. The participants provided their written informed consent to participate in this study. Written informed consent was obtained from the individual(s) for the publication of any potentially identifiable images or data included in this article.
Author contributions
TC: Data curation, Formal Analysis, Funding acquisition, Methodology, Project administration, Resources, Software, Writing – original draft, Writing – review & editing, Investigation. SF: Data curation, Investigation, Project administration, Supervision, Writing – original draft, Writing – review & editing, Methodology. PP: Resources, Supervision, Writing – review & editing, Conceptualization, Methodology. AG: Conceptualization, Investigation, Resources, Supervision, Writing – review & editing. SM: Conceptualization, Formal Analysis, Investigation, Methodology, Resources, Supervision, Writing – review & editing, Funding acquisition, Project administration.
Funding
The author(s) declare financial support was received for the research, authorship, and/or publication of this article. TC was funded by the Nigel Groome Studentship from Oxford Brookes University.
Acknowledgments
The authors would like to thank the patients for their willingness to participate in this research and the Neurofunctional nursing team at the John Radcliffe Hospital for their collaboration. We would like to acknowledge the Nigel Groome Studentship which funds TC.
Conflict of interest
The authors declare that the research was conducted in the absence of any commercial or financial relationships that could be construed as a potential conflict of interest.
The author(s) declared that they were an editorial board member of Frontiers, at the time of submission. This had no impact on the peer review process and the final decision.
Publisher’s note
All claims expressed in this article are solely those of the authors and do not necessarily represent those of their affiliated organizations, or those of the publisher, the editors and the reviewers. Any product that may be evaluated in this article, or claim that may be made by its manufacturer, is not guaranteed or endorsed by the publisher.
Supplementary material
The Supplementary material for this article can be found online at: https://www.frontiersin.org/articles/10.3389/fnins.2024.1339839/full#supplementary-material
SUPPLEMENTARY FIGURE 1 | Comparison of stimulus response for patients and best matched controls (n=3). Pooled AH VAS ratings plotted against change in PETCO2 above threshold AH for 3 patients with insula-glioma (closed circles) and 3 best matched healthy-controls (open circles). Solid lines are linear regression with 95% CI (dashed lines) and 95%PI (dotted lines). For the patient group the data from case 1 was not included in the linear regression because they rated close to 0%VAS AH throughout. Had we included the data for case 1 in the linear regression the average slope for the patient group would have been even more shallow compared to the best matched healthy controls.
SUPPLEMENTARY FIGURE 2 | Individual hypercapnic air hunger response slopes. For the patient group, AH responses for both pre-surgery (closed circles) and post-surgery (triangles) are included. Open circles are used for the healthy controls.
References
Adams, L, Lansing, RW, O’Donnell, CR, Paydarfar, D, and Banzett, RB. (2009). No evidence for reduction in dyspnea following lesions of the right insula. Am. J. Respir. Crit. Care Med. 179, 623–4. doi: 10.1164/ajrccm.179.7.623
Banzett, R. B., Lansing, R. W., and Binks, A. P. (2021). Air Hunger: A Primal Sensation and a Primary Element of Dyspnea. Compr. Physiol, 11, 1449–1483. doi: 10.1002/cphy.c200001
Banzett, R. B., Lansing, R. W., Evans, K. C., and Shea, S. A. (1996). Stimulus-response characteristics of Co2-induced air hunger in normal subjects. Respir. Physiol. 103, 19–31. doi: 10.1016/0034-5687(95)00050-X
Banzett, R. B., Mulnier, H. E., Murphy, K., Rosen, S. D., Wise, R. J., and Adams, L. (2000). Breathlessness in humans activates insular cortex. Neuroreport 11, 2117–2120. doi: 10.1097/00001756-200007140-00012
Banzett, R. B., Pedersen, S. H., Schwartzstein, R. M., and Lansing, R. W. (2008). The affective dimension of laboratory dyspnea: air hunger is more unpleasant than work/effort. Am. J. Respir. Crit. Care Med. 177, 1384–1390. doi: 10.1164/rccm.200711-1675OC
Dlouhy, B. J., Gehlbach, B. K., Kreple, C. J., Kawasaki, H., Oya, H., Buzza, C., et al. (2015). Breathing inhibited when seizures spread to the amygdala and upon amygdala stimulation. J. Neurosci. 35, 10281–10289. doi: 10.1523/JNEUROSCI.0888-15.2015
Duerden, E. G., and Albanese, M. C. (2013). Localization of pain-related brain activation: a meta-analysis of neuroimaging data. Hum. Brain Mapp. 34, 109–149. doi: 10.1002/hbm.21416
Duffau, H. (2020). Functional mapping before and after low-grade glioma surgery: a new way to decipher various spatiotemporal patterns of individual Neuroplastic potential in brain tumor patients. Cancers 12:2611. doi: 10.3390/cancers12092611
Edmonds, P., Karlsen, S., Khan, S., and Addington-Hall, J. (2001). A comparison of the palliative care needs of patients dying from chronic respiratory diseases and lung cancer. Palliat. Med. 15, 287–295. doi: 10.1191/026921601678320278
Egger, J., Kapur, T., Fedorov, A., Pieper, S., Miller, J. V., Veeraraghavan, H., et al. (2013). Gbm volumetry using the 3D slicer medical image computing platform. Sci. Rep. 3:1364. doi: 10.1038/srep01364
Esser, R. W., Stoeckel, M. C., Kirsten, A., Watz, H., Taube, K., Lehmann, K., et al. (2017). Brain activation during perception and anticipation of dyspnea in chronic obstructive pulmonary disease. Front. Physiol. 8:617. doi: 10.3389/fphys.2017.00617
Evans, K. C., Banzett, R. B., Adams, L., Mckay, L., Frackowiak, R. S., and Corfield, D. R. (2002). Bold fmri identifies limbic, paralimbic, and cerebellar activation during air hunger. J. Neurophysiol. 88, 1500–1511. doi: 10.1152/jn.2002.88.3.1500
Fedorov, A., Beichel, R., Kalpathy-Cramer, J., Finet, J., Fillion-Robin, J. C., Pujol, S., et al. (2012). 3D slicer as an image computing platform for the quantitative imaging network. Magn. Reson. Imaging 30, 1323–1341. doi: 10.1016/j.mri.2012.05.001
Grogono, J. C., Butler, C., Izadi, H., and Moosavi, S. H. (2018). Inhaled furosemide for relief of air hunger versus sense of breathing effort: a randomized controlled trial. Respir. Res. 19:181. doi: 10.1186/s12931-018-0886-9
Hutchinson, A., Barclay-Klingle, N., Galvin, K., and Johnson, M. J. (2018). Living with breathlessness: a systematic literature review and qualitative synthesis. Eur. Respir. J. 51:1701477. doi: 10.1183/13993003.01477-2017
Kikuchi, Y., Okabe, S., Tamura, G., Hida, W., Homma, M., Shirato, K., et al. (1994). Chemosensitivity and perception of dyspnea in patients with a history of near-fatal asthma. N. Engl. J. Med. 330, 1329–1334. doi: 10.1056/NEJM199405123301901
O’Donnell, C. R., Schwartzstein, R. M., Lansing, R. W., Guilfoyle, T., Elkin, D., and Banzett, R. B. (2013). Dyspnea affective response: comparing COPD patients with healthy volunteers and laboratory model with activities of daily living. BMC. Pulm Med, 13, 27. doi: 10.1186/1471-2466-13-27
Parkinson, J. (2002). An essay on the shaking palsy. 1817. J. Neuropsychiatry Clin. Neurosci. 14, 223–236. doi: 10.1176/jnp.14.2.223
Schon, D., Rosenkranz, M., Regelsberger, J., Dahme, B., Buchel, C., and Von Leupoldt, A. (2008). Reduced perception of dyspnea and pain after right insular cortex lesions. Am. J. Respir. Crit. Care Med, 178, 1173–9. doi: 10.1164/rccm.200805-731OC
Starr, C. J., Sawaki, L., Wittenberg, G. F., Burdette, J. H., Oshiro, Y., Quevedo, A. S., et al. (2009). Roles of the insular cortex in the modulation of pain: insights from brain lesions. J. Neurosci. 29, 2684–2694. doi: 10.1523/JNEUROSCI.5173-08.2009
Whittle, I. R. (2004). The dilemma of low grade glioma. J. Neurol. Neurosurg. Psychiatry 75:ii31. doi: 10.1136/jnnp.2004.040501
Keywords: dyspnoea, hypercapnia, air hunger, insular cortex, neurosurgery, brain tumour, short of breath
Citation: Chapman TP, Farrell SM, Plaha P, Green AL and Moosavi SH (2024) Blunted perception of breathlessness in three cases of low grade insular-glioma. Front. Neurosci. 18:1339839. doi: 10.3389/fnins.2024.1339839
Edited by:
Ana Paula Abdala Sheikh, University of Bristol, United KingdomReviewed by:
Andreas Von Leupoldt, KU Leuven, BelgiumHazel Blythe, University of Bristol, United Kingdom
Zoe Adams, Cardiff Metropolitan University, United Kingdom
Copyright © 2024 Chapman, Farrell, Plaha, Green and Moosavi. This is an open-access article distributed under the terms of the Creative Commons Attribution License (CC BY). The use, distribution or reproduction in other forums is permitted, provided the original author(s) and the copyright owner(s) are credited and that the original publication in this journal is cited, in accordance with accepted academic practice. No use, distribution or reproduction is permitted which does not comply with these terms.
*Correspondence: Shakeeb H. Moosavi, c21vb3NhdmlAYnJvb2tlcy5hYy51aw==
†These authors share first authorship