- Department of Endocrinology and Metabolism, The First Hospital of Jilin University, Changchun, China
Plurihormonal pituitary adenoma (PPA) is a type of pituitary tumor capable of producing two or more hormones and usually presents as an aggressive, large adenoma. As yet, its pathogenesis remains unclear. This is the first study to systematically summarize the underlying pathogenesis of PPA. The pathogenesis is related to plurihormonal primordial stem cells, co-transcription factors, hormone co-expression, differential gene expression, and cell transdifferentiation. We conducted a literature review of PPA and analyzed its clinical characteristics. We found that the average age of patients with PPA was approximately 40 years, and most showed only one clinical symptom. The most common manifestation was acromegaly. Currently, PPA is treated with surgical resection. However, recent studies suggest that immunotherapy may be a potentially effective treatment.
Introduction
Pituitary neuroendocrine tumors, formerly known as pituitary adenomas, are usually benign monoclonal tumors (Neou et al., 2020; Cui et al., 2021). The incidence is approximately 0.07–0.15% and accounts for 15% of all intracranial tumors (Ho et al., 2001; Fernandez et al., 2010; Raappana et al., 2010; Mete and Lopes, 2017). Most pituitary adenomas produce only one hormone, and the production of multiple hormones is rare (Daly et al., 2006; Ostrom et al., 2014; Melmed, 2020; Asa et al., 2022; Graffeo et al., 2022; Yao et al., 2022). Plurihormonal pituitary adenoma (PPA) is defined as the immunopositivity of two or more hormones within the same cell (haplomorphism), or two or more distinct cell populations with different hormones (pleomorphism) (Thapar et al., 1993; Ho et al., 2001; Liu et al., 2018; Asa et al., 2022). Initially thought to be an atypical variant, an increasing number of immunohistochemical, ultrastructural, and biochemical analyses have confirmed PPA as a type of pituitary tumor. According to the 2017 classification of pituitary tumors by the World Health Organization (WHO), PPA is divided into two subtypes: pituitary transcription factor 1 (PIT-1)-positive plurihormonal pituitary adenomas (PIT-1 + PPA) and plurihormonal adenomas with unusual immunohistochemical combinations (PAwUIC). Notably, WHO suggested that growth hormone-prolactin (GH-PRL) and follicle-stimulating hormone-luteinizing hormone (FSH-LH) adenomas are not PPA (Rasul et al., 2014; Villa et al., 2014; Lopes, 2017; Mete and Lopes, 2017; Nf and Ai, 2023).
Plurihormonal pituitary adenomas were previously considered rare. Recent application of immunohistochemistry to pituitary tumors has shown that many pituitary adenomas, including clinically nonfunctional pituitary adenomas, are actually plurihormonal (Salehi et al., 2006; Chinezu et al., 2017; Kobalka et al., 2021). Recent studies have also found that PPA accounts for 10–15% of all pituitary tumors (Shi et al., 2022). PPA can be more aggressive than pituitary tumors that secrete a single hormone, with more than 50% of the tumors being locally aggressive (Pawlikowski et al., 2010; Andrews et al., 2021). PPA is usually a large adenoma with a high recurrence rate (Pawlikowski et al., 2010). Marek et al. analyzed 155 excised pituitary adenomas and found that more than one-third of the pituitary adenomas were pluripotent, and that the recurrence rate of plurihormonal adenomas was twice that of single-hormone adenomas (17.8% vs. 9.4%). They also found that adrenocorticotropic hormone (ACTH)-expressing adenomas in plurihormonal nonfunctional pituitary tumors had a higher recurrence rate than ACTH-negative adenomas (35.3% vs. 14.2%) (Pawlikowski et al., 2010). Furthermore, several studies have also shown that the Ki-67 index of PPA is significantly higher than that of single-hormone adenomas (Thapar et al., 1993; Pawlikowski et al., 2010; Luk et al., 2012).
Ultrastructural studies have shown that PPA can be classified as monomorphic and pleomorphic adenomas. Monomorphic adenomas consist of single cells capable of producing two or more hormones. Pleomorphic adenomas are composed of a variety of cell types, and different cells produce different hormones. The immunoelectron microscopy colloidal gold double-labeling technique has shown that multiple hormonal profiles can co-exist within a single cell, even in pleomorphic adenomas. Chiang et al. immunostained 167 cases of PPA and observed the presence of multiple hormone mixtures in individual adenoma cells. Therefore, they concluded that most PPAs are probably monomorphic adenomas (Ho et al., 2001).
In 2022, the WHO proposed that the adenohypophyseal is composed of at least six cell types: somatotrophs, lactotrophs, mammosomatotrophs, and thyrotrophs of the PIT1 lineage; corticotrophs of the T-box family member TBX19 (T-PIT) lineage; and gonadotrophs of the steroidogenic factor-1 (SF1) lineage (Mariniello et al., 2019; Alatzoglou et al., 2020; Asa et al., 2021; Kobalka et al., 2021; Asa et al., 2022; Villa et al., 2023). Studies have shown that the pathogenesis of pituitary adenoma may be related to gene mutations, chromosome number variations, DNA methylation, microRNA regulation, and transcription factor regulation (Asa and Ezzat, 2009; Salomon et al., 2018; Vandeva et al., 2019; Chang et al., 2020; Sabatino et al., 2022). However, the pathogenesis of PPA is still unclear. This study aimed to describe the potential pathogenesis of PPA and analyze its clinical characteristics.
Potential mechanisms
The pathogenesis of PPA is unclear (Srirangam Nadhamuni and Korbonits, 2020). The underlying mechanism may be as follows.
Plurihormonal primordial stem cell
The anterior pituitary gland originates from the Rathke sac. Stem cells in the anterior pituitary gland proliferate in the embryo, and stem cells in the embryo differentiate into different pituitary cells under the regulation of various transcription factors (Mete et al., 2018; Alatzoglou et al., 2020; Matsumoto et al., 2020; Zhang et al., 2020; Matsumoto and Takahashi, 2021). Over the past decade, evidence for the existence of adult pituitary stem cells (PSC) has been provided using in vitro clonogenic assays, flow cytometry side-population analyses, immunohistochemical analyses, and genetic methods. These cells can self-regenerate, and undergo pluripotent differentiation to produce all the hormone lineages in the anterior pituitary (Carreno et al., 2017; Cox et al., 2017; Haston et al., 2018; Tordjman et al., 2019; Laporte et al., 2020; Würth et al., 2020; Willis et al., 2022; Winningham and Camper, 2023). PSC is thought to be present in the intermediate lobe (IL) and the dorsal anterior lobe (AL), in the marginal zone (MZ), and is dispersed throughout the AL parenchyma (Garcia-Lavandeira et al., 2012; Haston et al., 2018). Garcia et al. found that cell populations located in MZ co-express GFRα2, RET, and PROP1, and that these cells contribute to the stem cell niche in MZ and may play a role in regulating structural guidance and the survival of PSC (Garcia-Lavandeira et al., 2009). Chen et al. found that PSC expressed SOX2, SOX9, CD4, CD133, and SCA1 (Chen et al., 2005). Previous in vitro clonal assay studies have demonstrated the expression of PROP1, PRX1/2, GFRα2, CXCR4, and NESTIN in PSC (Horiguchi et al., 2012; Rizzoti et al., 2013; Higuchi et al., 2014). Notably, cancer cells with stem cell properties are called cancer stem cells (CSC), and these cells can self-regenerate and differentiate into all cell types within the tumor (Batlle and Clevers, 2017; Steinbichler et al., 2018; Lan and Behrens, 2023). More recent studies have identified these cells in pituitary adenomas (Carreno et al., 2017; Cox et al., 2017; Lodge et al., 2019).
Xu et al. first discovered the presence of stem cells in pituitary adenomas that expressed pituitary-specific markers, such as PIT-1 and stem cell markers OCT4, NOTCH4, CD133, and NESTIN (Xu M. et al., 2009). Wurth et al. isolated CSC-like cell populations from 38 human pituitary adenomas that expressed CD133, OCT4, SOX2, and NESTIN, with the potential to differentiate into hormone-producing cells (Würth et al., 2017). These findings support a potential role for adult pituitary stem cells in pituitary adenomas. Almeida et al. suggested that the occurrence of plurihormonal and null cell-type adenomas (no distinguishable ultrastructural, immunohistochemical, or histologic characteristics of recognized adenohypophyseal cell types) supports the hypothesis that pituitary stem cells may be a potential source of pituitary adenomas (De Ameida et al., 2010). Radian et al. found that GH-and thyroid-stimulating hormone (TSH)-secreting cells share common progenitor cell expression. This suggests that TSH adenomas originate from pituitary stem cells that differentiate into GH, PRL, or TSH cells, which may play a role in the pathogenesis of TSH-secreting PPA (Teramoto et al., 2004; Vankelecom and Roose, 2017). Matsuno et al. studied the expression of pituitary hormones including glycoprotein hormone mRNA in GH and PRL adenomas using non-isotope in situ hybridization. They found that some cells in GH adenomas expressed multiple hormone genes, mainly ACTH, FSH, and LH. These results suggest that some GH adenomas may originate from adult pituitary stem cells, which are precursors of various hormone-expressing cells (Matsuno et al., 1995, 1996). For example, Kovacs et al. reported a case of PPA-producing GH and ACTH. Through immunohistochemical analysis, fluoroscopic electron microscopy, immunoelectron microscopy, and in situ hybridization, the tumor was found to be composed of two independent cell types. Moreover, one cell population synthesized GH, and the other synthesized ACTH. The authors proposed that PPA may originate from undifferentiated stem cells that can differentiate into many different cell types and that this multidirectional differentiation could explain the pathogenesis of PPA (Kovacs et al., 1998).
In this study, we summarized the underlying pathogenesis (Figure 1). A growing number of studies have identified the presence of PSC in the pituitary gland. PSC can grow into adenomas under conditions such as activation of oncogenes and inactivation of oncogenes (Carreno et al., 2017; Laporte et al., 2020; Würth et al., 2020; Winningham and Camper, 2023). Under the regulation of different transcription factors, they differentiate into different cells and secrete different hormones, which ultimately lead to PPA. In addition, we found that the WNT, TGFβ, NOTCH, HIPPO and SHH pathways may be associated with adult pituitary stem cell tumorigenesis (Gaston-Massuet et al., 2011; Lodge et al., 2019; Würth et al., 2020; Winningham and Camper, 2023). (Figure 2) Further basic research on adult pituitary stem cells is needed to explore their role in the development, progression, and recurrence of PPA.
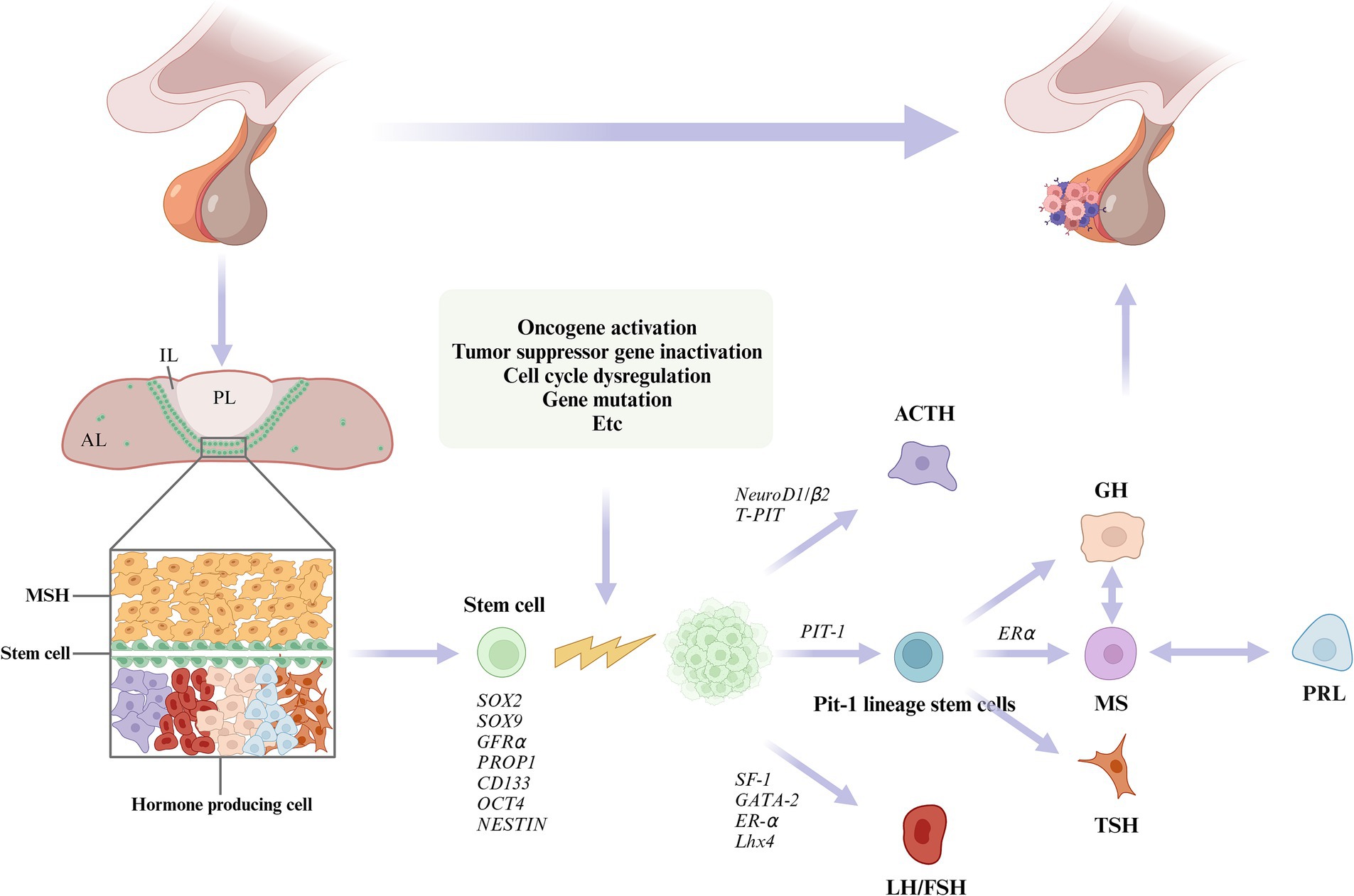
Figure 1. The pituitary gland consists of the anterior lobe (AL), intermediate lobe (IL), and posterior lobe (PL). Plurihormonal primordial stem cells (PSC) are thought to reside in the intermediate lobe (IL) and the dorsal anterior lobe (AL), and are dispersed throughout the AL parenchyma. PSCs proliferate into adenomas under conditions that activate oncogenes, inactive tumor suppressor genes, dysregulate cell cycles, or cause mutations. Under the regulation of different transcription factors, the PSCs differentiate into different cells and secrete a variety of hormones.
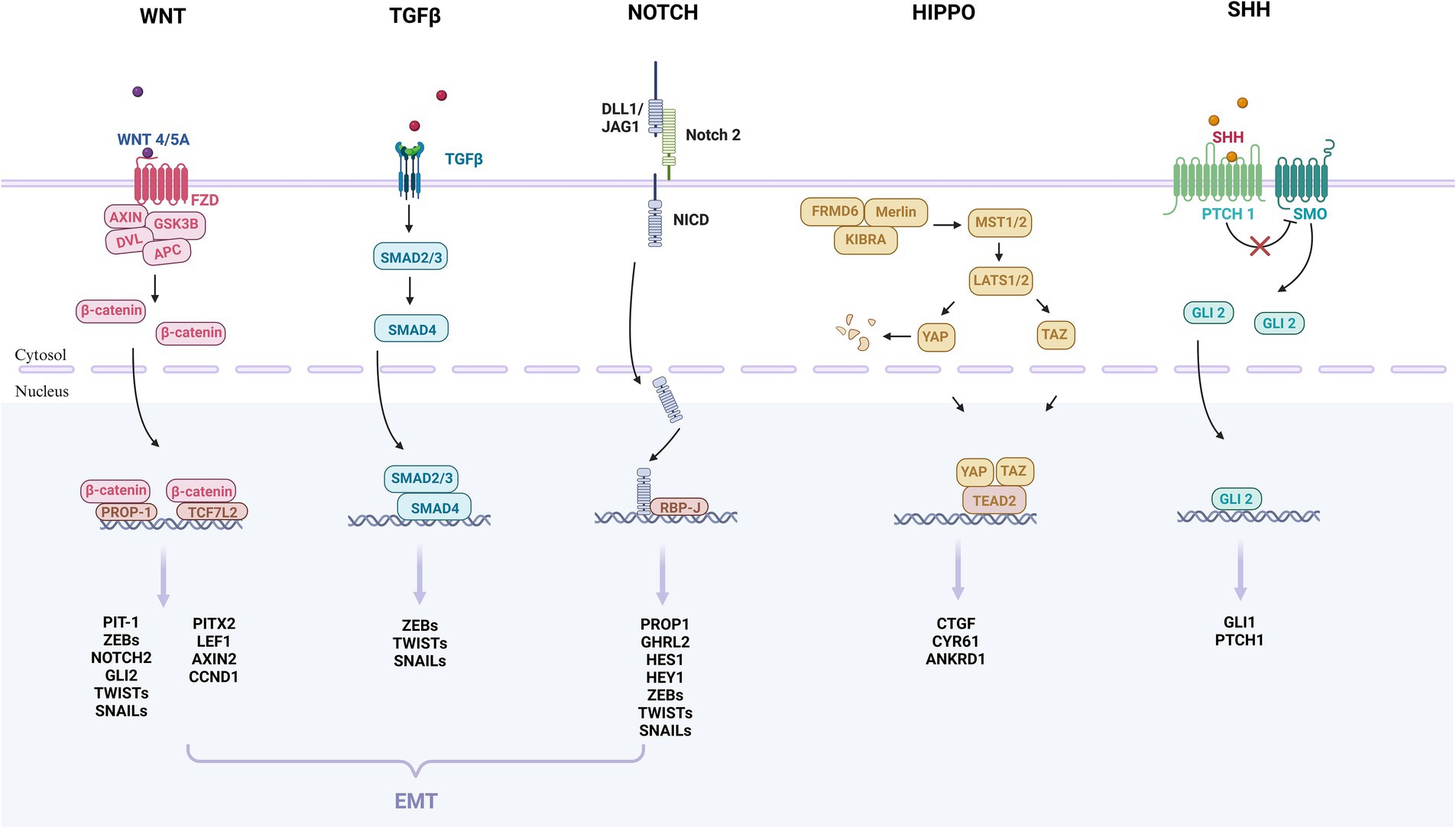
Figure 2. These are the key signaling pathways of pituitary adenomas that may be involved in the initiation and progression of adult pituitary stem cell tumors. The pathways include WNT, TGFβ, NOTCH, HIPPO and SHH. The WNT, TGFβ, and NOTCH pathways may further participate in epithelium-mesenchymal transition (EMT), and promote tumor growth and spread. The WNT pathway: The WNT protein binds to the FZD receptor on the cell membrane, leading to the stabilization and accumulation of β-catenin protein, which enters the nucleus and binds to the transcription factor PROP-1, TGF7L2, regulating the expression of the target gene. The TGFβ pathway: TGFβ binds to the receptor complex, phosphorylates to SMAD2/3, and binds to SMAD4 to form a complex that enters the nucleus and binds to transcription factors to regulate the expression of target genes. The NOTCH pathway: After the NOTCH receptor binds to ligand DLL1/JAG1, NICD is released, which enters the nucleus and binds to transcription factor RBP-J to regulate gene expression. The HIPPO pathway: The protein encoded by the Hippo gene interacts with MST to activate the LATS protein, so that YAP and TAZ are phosphorylated and fixed in the nucleus, unable to enter the nucleus to regulate gene expression. When the signaling pathway is suppressed, YAP and TAZ enter the nucleus, bind to the transcription factor TEAD, and regulate gene expression. SHH pathway: In the absence of SHH, the PTCH receptor inhibits the activity of the protein SMO, and when SHH binds to PTCH, PTCH no longer inhibits SMO, thus SMO is activated and GLI protein is regulated. These proteins enter the nucleus to regulate the expression of target genes. EMT: Three families of transcription factors, ZEB, SNAIL, and TWIST, play a central role in EMT. For pituitary stem cell tumors, WNT, TGFβ, and NOTCH pathways may be involved in the regulation of EMT.
Co-transcription factor
Previous studies identified several transcription factors that regulate the transformation of cellular precursors into mature secretory cells, including T-pit, Pitx1, Pitx2, Prop-1, Pit-1, SF-1, NeuroD1, and GATA-2 (Parvin et al., 2017; Sjöstedt et al., 2017; Ellsworth and Stallings, 2018; Mete et al., 2018; Cheung and Camper, 2020; Hickman et al., 2021). Pitx1 is a universal transcription factor found in all types of pituitary cells and all types of pituitary adenomas. Pitx1 acts synergistically with NeuroD1/2, SF-1, and Pit-1 to activate POMC/ACTH, FSH-LH, and GH-PRL-TSH transcription, respectively (Szeto et al., 1999; Lamolet et al., 2001). Matsuno et al. described a GH-PRL-ACTH pituitary tumor and demonstrated the use of immunohistochemical staining, in situ hybridization, and cell culture techniques to generate GH, PRL, ACTH, and Pit-1 expression. They believed that in addition to Pit-1, there may be unknown transcription factors that simultaneously express GH, PRL, and ACTH (Matsuno et al., 1996). Shigeyuki et al. found that GH and ACTH are expressed in the same cell in one GH-ACTH adenoma, suggesting that GH is produced in ACTH-derived adenoma cells and that Pit-1 and NeuroD1 are expressed in the nucleus of the same adenoma cells. This suggests that the production of multiple cell lineage hormones in the same cell may be due to abnormal expression patterns associated with the transcription factors in pituitary tumors. The synergistic mechanism of NeuroD1 and Pit-1 is of great scientific significance and requires further investigation (Tahara et al., 2002).
Co-expression of hormones
Normally, a cell synthesizes and secretes specific hormones for the regulation of physiological functions and metabolic processes. However, some normal cells and adenoma cells express and secrete many different types of hormones, a phenomenon known as “hormone co-expression” (Radian et al., 2003; Tong et al., 2013; Allensworth-James et al., 2020). Osamura et al. observed the co-expression of GH, α subunits, and gonadotropin in some cells of the anterior pituitary in normal adults (Osamura et al., 2009). This evidence suggests the presence of a certain percentage of plurihormonal cells in the normal anterior pituitary, which refutes the notion of “one cell type, one hormone.” Lubov et al. found that the same cells in normal adenohypophysis could co-express PRL with ACTH, TSH, FSH, and LH; GH with ACTH, TSH, FSH, and LH; and TSH with ACTH, FSH, and LH. After comparing 10 scanning chemical sections of the pituitary gland, they found a significant overlap in the GH, PRL, LH, and ACTH expression regions of the distal pituitary gland. Moreover, the co-expression of TSH and PRL was highly prominent in 50% of the anterior pituitary cells using confocal scanning microscopy, with co-expression coefficients ranging from 3.4 to 22%. The co-expression coefficients of PRL and LH were between 4 to15%. The coexpression coefficients of GH and LH ranged from 0.4 to 24%. The coexpression coefficients of ACTH/PRL and ACTH/GH were 34 and 55%, respectively (Mitrofanova et al., 2017). Therefore, this hormone co-expression phenomenon may also be the cause of PPA. Evidence from immunohistochemistry studies has shown that GH and PRL are co-expressed in the same cell (Kurotani et al., 2001; Tong et al., 2013). Likewise, the cells in pituitary adenomas may display hormone co-expression. Electron microscopy, immunocytochemistry, and laser scanning confocal microscopy revealed that GH and TSH were co-expressed in the anterior pituitary cells of adult rats with methimazole-induced hypothyroidism (Radian et al., 2003). Tong et al. performed immunohistochemical tests on a patient with TSH-GH-PRL multihormone adenoma and found that TSH and GH were colocalized in most tumor cells, indicating that the two hormones were mainly expressed by the same cells. The ultrasonic structure presented a simplex cell appearance and a single type of secretory particle, similar to the secretory particles in normal TSH cells. This suggests that TSH and GH originated from the same secretory particles (Tong et al., 2013).
Differential gene expression
PPA pathogenesis may involve several abnormally expressed genes and pathways. Zhiquan et al. examined the gene expression profiles of seven PPA using a magnetic bead-based optical fiber array, and compared them with those of three normal pituitary glands. A pathway analysis of all differentially expressed genes using the Kyoto Encyclopedia of Genes and Genomes (Jiang et al., 2012) demonstrated that the expression of six genes in PPA increased significantly, and the expression of 334 genes and 15 expression sequence tags decreased significantly. Bioinformatics analysis indicated that HIGD1B, EPS8, ECT2, and BTG2 may play important roles in the tumorigenesis and progression of PPA. Pathway analysis showed that the Notch and p53 signaling pathways may play an important role in the development of PPA tumors. Extracellular matrix (ECM) receptor interactions may play a role in inhibiting tumor invasion and metastasis (Table 1).
Cell transdifferentiation
In the past few decades, the “one cell, one hormone” theory has been widely accepted, and it has been proposed that the phenotype of adenopituitary cells is immutable, and that they are irreversibly committed to producing a single hormone (Kovacs et al., 1989). In recent years, new technologies have emerged to disprove this. Evidence has shown that polyhormone cells may exist in some differentiated cellular pathways (Stefaneanu et al., 1992; Mitrofanova et al., 2017; Asa et al., 2023). Normal gonadotrophs produce gonadotropins, FSH, and LH. Mammosomatotrophs are cells that produce both GH and PRL, and represent a fluid cell type that can transdifferentiate into somatotrophs during growth and lactotrophs during pregnancy (Stefaneanu et al., 1992; Asa et al., 2023). Childs et al. found that a single cell of the pituitary gland in rodents can contain both ACTH and FSH, and explained this observation as “transdifferentiation.” One cell type may be transformed into another owing to the genetic and epigenetic factors at play during tumor progression, and are often accompanied by different morphological changes (Vidal et al., 2000). Kovacs et al. reported a case of an ACTH-GH pituitary tumor and identified ACTH in several GH-secreting particles using immunoelectron microscopy. This result suggests that the tumor was originally a GH adenoma that started producing ACTH because of mutations that occurred during tumor progression (Kovacs et al., 1998). Mark et al. reported a case of adenohypophyseal hyperplasia in a patient with primary hypothyroidism, in which TSH and PRL cells proliferated, and GH cells transdifferentiated into cells that secreted both GH and TSH (Jentoft et al., 2012). Sergio et al. found GH-and TSH-labeled double-hormone particles in the cells of TSH-GH adenomas and double-hormone particles in the adenomas of two patients with acromegaly labeled with PRL and TSH, respectively. Using double immunogold labeling in GH-PRL adenomas, they observed that most tumor cells contained dual hormone particles, and some cells contained only GH or PRL particles. These findings strongly suggest that the transdifferentiation of one cell type into another may be the cause of PPA (Vidal et al., 1999). It has been suggested that the interconversion of one cell type into another is not a direct process but occurs through a bihormonal transition cell that contains functional components common to both cells (Frawley and Boockfor, 1991).
Clinical features
Studies have found that approximately 70% of patients with PPA have hormonal symptoms, with acromegaly being the most common (accounting for 50% of cases). Hyperprolactinemia-related symptoms account for 20% of all the cases, whereas gonadotropin-and thyrotropin-related symptoms are rare (Ho et al., 2001).
Ruoyu et al. analyzed the clinical characteristics of 70 patients with PPA and found that these adenomas accounted for 13% of all pituitary adenomas recorded during the same period. 53% of the patients had one clinical symptom, 1% had two endocrine symptoms, and none of the patients showed three or more endocrine symptoms. Of the patients, 74.3% had an elevated level of one hormone, 7.1% had an elevated level of two hormones, and only one had an elevated level of all three hormones (Shi et al., 2022). Seckin et al. analyzed the clinical features of 27 patients with PPA, 10 of whom were diagnosed with PAwUIC and 9 with PIT-1 + PPA. The median age was 44.7 years (range, 19–75 years) and 59.3% were males and 40.7% were females. Large adenomas accounted for 88.8%, giant adenomas ≥4 cm accounted for 22.2, 44.4% of patients were accompanied by cavernous sinus infiltration, and nearly half of the patients were highly invasive. 77.8 of the patients with PAwUIC, 77.8% showed non-functional pituitary adenomas, and only four patients had hormonal secretory characteristics (Aydin et al., 2019). Mete et al. reported 31 patients with monomorphic multihormone Pit-1 lineage tumors with a mean age of 44.3 years, 11 of whom had hormone secretion symptoms. Most patients have large adenomas, and 30% have tumors that invade the cavernous sinus (Mete et al., 2016). Horvath et al. reported 29 multihormone Pit-1 spectrum tumors in patients with a mean age of 37.5 years, predominantly female (25:4), all of which were macroadenomas, and approximately half of the tumors were aggressive (Horvath et al., 2005). Erickson et al. reported 27 patients with plurihormonal Pit-1 spectrum tumors (mean age, 36.4 years). All tumors were macroadenomas, and 60% of the tumors were aggressive (Erickson et al., 2009). Sylvia et al. reported that PPA co-expressed with Pit-1 and SF-1 in 38 patients (mean age, 53 years; range, 12–79 years). Most tumors had lamellar or nested adherent epithelioid cells that were eosinophilic throughout the intestine. The most commonly secreted hormone is the growth hormone (GH), which causes acromegaly. However, central hyperthyroidism is rare. Furthermore, GATA3 expression was detected in a subset of PPA that expresses both Pit-1 and SF-1 (Asa et al., 2023).
Although PPA immunohistochemistry usually shows a positive expression of multiple hormones, it does not clinically show an increased secretion of multiple hormones. Most patients only show one clinical symptom (Ho et al., 2001), We analyze the possible reasons as follows. (1) Some immunohistochemically positive hormones have fewer positive particles and the hormone content is insufficient (Elhadd et al., 2009; Vora and Karunakaran, 2017). (2) Although tumor cells can synthesize certain hormones, the secretion mechanism may be obstructed, and when the secretion of one hormone is dominant, the secretion of another may be suppressed (Lamberts and Macleod, 1990; Müller et al., 1999). (3) Tumors may originate from primitive cells that are not biologically active. Tumor cells can only synthesize hormonal precursors. Although they can cross-react with the corresponding antibody and appear immunopositive, they do not exhibit biological activity (Tordjman et al., 2019).
We have summarized 31 cases of PPA reported in the literature (Table 2). Most patients had aggressive macroadenomas, and the mean age of the patients was 46.51 years, ranging from 13 to 72 years, with 42% men and 58% women. The most common symptom was acromegaly, and most patients opt for surgical tumor removal.
For decades, it was widely believed that pituitary adenomas were monoclonal (Beck-Peccoz et al., 1996; Dahia and Grossman, 1999; Levy and Lightman, 2003). There is much evidence to support the view that pituitary tumors are clonal lesions caused by defects in intrinsic pituitary cells, most of which are based on X chromosome inactivation (Chu et al., 2008; Sav et al., 2012). However, an increasing number of scholars have proposed that multiple synchronous adenomas are of polyclonal origin and that some sporadic tumors may also be polyclonal (Clayton and Farrell, 2001; Asa and Ezzat, 2002; Buch et al., 2002). PPA can potentially be explained also by heteroclonality (Herman et al., 1990; Levy and Lightman, 2003). In an earlier study based on nuclear DNA analysis by flow cytofluorimetry, biclonal cell lines were isolated in 10% of pituitary adenomas (Anniko and Tribukait, 1985). In more than half of the “recurrent” pituitary tumors with repeated analysis of loss of heterozygosity after further surgery, the loci involved in the original pattern of loss of heterozygosity were heterozygous again (Clayton et al., 2000). It also suggests that these pituitary tumors may be polyclonal. In addition, a growing number of studies have found that pituitary tumors can display intrinsic heterogeneity and cellular subpopulations with different biological, genetic and epigenetic properties (Sabatino et al., 2022). Tissue structure and microenvironment play a crucial role in tumors. Different cellular processes alter the structure and interactions within the gland, thereby shaping tumor growth. Tumor heterogeneity exists in many forms, ranging from somatic coding and non-coding alterations to epigenetic, transcriptomic and post-translational modifications (Caswell and Swanton, 2017; Mcgranahan and Swanton, 2017).
Treatment
PPA is characterized by its aggressiveness and high postoperative recurrence rate (Steinbichler et al., 2018; Lodge et al., 2019; Lan and Behrens, 2023). Transsphenoidal microsurgical resection of mixed pituitary adenomas remains the preferred treatment for PPA (Swearingen, 2012; Ammirati et al., 2013). Surgical removal of tumors can reduce tissue pressure and hormone levels. However, it is not ideal for controlling tumor development for long-term remission. Radiotherapy is used only in patients whose tumor size or hormone levels are not sufficiently reduced by surgery or medication. Radiotherapy can be used as a postoperative adjuvant therapy. Importantly, the monitoring of pituitary function indefinitely after radiotherapy to detect anterior pituitary hypofunction is recommended. Approximately 50% of patients develop hypopituitarism within five years of radiation therapy (Loeffler and Shih, 2011; Ding et al., 2014; Molitch, 2017; Tritos and Miller, 2023). Wang et al. reported a case of GH-PRL adenoma in which programmed death ligand 1 (PD-L1) protein and CD8+ lymphocyte infiltrations were detected in tumor tissue. The tumor was aggressive and did not respond to bromocriptine therapy. GH and PRL levels did not improve after surgical resection and recurred postoperatively. The authors proposed the use of PD-1 inhibitors in combination with radiotherapy or temozolomide for the treatment of aggressive PPA (Wang et al., 2017) John et al. also found a higher incidence of significant PD-L1 in PIT-1-positive multihormone tumors than in other pituitary adenomas, and PD-L1 overexpression was very rare in ACTH and gonadotropin cells. The authors suggested that immunosuppressants may be a reasonable treatment for PIT-1 positive plurihormonal adenoma (Turchini et al., 2021).
Conclusion
PPA is a relatively rare tumor that can secrete more than two hormones that differ in their biochemical composition, immunohistochemistry, and biological effects. We conducted a review of the literature to elucidate the clinical features of PPA and suggested the potential pathogenesis. We hypothesized that the pathogenesis of PPA is related to plurihormonal primordial stem cells, co-transcription factors, hormone co-expression, differential gene expression, and cell transdifferentiation. In the future, more basic and clinical studies are required to verify the pathogenesis and clinical features of PPA.
Author contributions
YC: Conceptualization, Data curation, Formal analysis, Writing – original draft. SL: Investigation, Writing – review & editing. XZ: Funding acquisition, Project administration, Writing – review & editing. LR: Conceptualization, Methodology, Writing – review & editing. XL: Investigation, Writing – review & editing. XG: Resources, Validation, Visualization, Writing – review & editing. GW: Conceptualization, Funding acquisition, Project administration, Resources, Validation, Visualization, Writing – review & editing.
Funding
The author(s) declare financial support was received for the research, authorship, and/or publication of this article. This work was supported by grants from the National Natural Science Foundation of China (81970687 and 82270850 to GW), the Department of Science and Technology of Jilin Province (20210303001SF, 20190901006JC and YDZJ202202CXJD042 to GW, 20210101439JC to XZ), and the first hospital of Jilin University (JDYYZH-2102030 to GW, JDYYCB-2023010 and 2021-zl-01 to XZ).
Acknowledgments
The authors would like to thank Editage (www.editage.cn) for English language editing.
Conflict of interest
The authors declare that the research was conducted in the absence of any commercial or financial relationships that could be construed as a potential conflict of interest.
Publisher’s note
All claims expressed in this article are solely those of the authors and do not necessarily represent those of their affiliated organizations, or those of the publisher, the editors and the reviewers. Any product that may be evaluated in this article, or claim that may be made by its manufacturer, is not guaranteed or endorsed by the publisher.
Abbreviations
PPA, plurihormonal pituitary adenoma; PIT-1, Pituitary transcription factor 1; PAwUIC, plurihormonal adenomas with unusual immunohistochemical combinations; GH, growth hormone; PRL, prolactin releasing hormone; FSH, follicle-stimulating hormone; LH, luteinizing hormone; ACTH, adrenocorticotropic hormone; PSC, pituitary stem cells; IL, intermediate lobe; AL, anterior lobe; MZ, marginal zone; CSC, cancer stem cells; TSH, thyroid stimulating hormone; T-pit, T-box family member TBX19; SF-1, steroidogenic factor-1; EMT, epithelium-mesenchymal transition; ECM, Extracellular matrix; EPS8, epidermal growth factor receptor pathway substrate 8; ECT2, epithelial cell transforming sequence 2; BTG2, B cell translocation gene 2; COL11A2, alpha2 chain of type XI collagen; ITGB4, integrin beta4; THBS4, thrombospondin 4; COL4A6, alpha 6 chain of type IV collagen; COL11A1, alpha1 chain of type XI collagen; COL6A2, alpha2 chain of type IV collagen; COL6A1, alpha1 chain of type IV collagen; ECM, extracellular matrix; DLK1, the Notch1 inhibitor delta-like 1 homolog; CtBP2, transcriptional corepressor C-terminal binding protein 2; HES1, the hairy enhancer of the split 1; Ep300, histone acetyltransferase p300; GADD45, growth arrest and DNA damage-inducible gene 45; IGFBP3, insulin-like growth factor binding protein 3; PMAIP1, phorbol-12-myristate-13-acetate-induced protein 1; PD-L1, programmed death ligand 1.
References
Alatzoglou, K. S., Gregory, L. C., and Dattani, M. T. (2020). Development of the pituitary gland. Compr. Physiol. 10, 389–413. doi: 10.1002/cphy.c150043
Allehaibi, E., Almalki, M. H., and Brema, I. (2021). Plurihormonal pituitary macroadenoma: a case report. J. Med. Case Rep. 15:407. doi: 10.1186/s13256-021-02948-6
Allensworth-James, M. L., Odle, A. K., Lim, J., Lagasse, A. N., Miles, T. K., Hardy, L. L., et al. (2020). Metabolic signalling to somatotrophs: transcriptional and post-transcriptional mediators. J. Neuroendocrinol. 32:e12883. doi: 10.1111/jne.12883
Al-Shraim, M., Scheithauer, B. W., Horvath, E., Kovacs, K., Smyth, H., Coire, C., et al. (2009). Plurihormonal gonadotroph cell pituitary adenoma: report of a unique case. Clin. Neuropathol. 28, 182–187. doi: 10.5414/npp28182
Amir, J., Guiot, M. C., and Garfield, N. (2022). Plurihormonal pituitary adenoma cosecreting ACTH and GH: a rare cause of Cushing's disease. BMJ Case Rep. 15:e251451. doi: 10.1136/bcr-2022-251451
Ammirati, M., Wei, L., and Ciric, I. (2013). Short-term outcome of endoscopic versus microscopic pituitary adenoma surgery: a systematic review and meta-analysis. J. Neurol. Neurosurg. Psychiatry 84, 843–849. doi: 10.1136/jnnp-2012-303194
Andrews, J. P., Joshi, R. S., Pereira, M. P., Oh, T., Haddad, A. F., Pereira, K. M., et al. (2021). Plurihormonal PIT-1-positive pituitary adenomas: a systematic review and single-center series. World Neurosurg. 151, e185–e191. doi: 10.1016/j.wneu.2021.04.003
Anniko, M., and Tribukait, B. (1985). DNA pattern of human pituitary tumors. Am. J. Otolaryngol. 6, 103–110. doi: 10.1016/s0196-0709(85)80047-8
Asa, S. L., and Ezzat, S. (2002). The pathogenesis of pituitary tumours. Nat. Rev. Cancer 2, 836–849. doi: 10.1038/nrc926
Asa, S. L., and Ezzat, S. (2009). The pathogenesis of pituitary tumors. Annu. Rev. Pathol. 4, 97–126. doi: 10.1146/annurev.pathol.4.110807.092259
Asa, S. L., Mete, O., Cusimano, M. D., Mccutcheon, I. E., Perry, A., Yamada, S., et al. (2021). Pituitary neuroendocrine tumors: a model for neuroendocrine tumor classification. Modern Pathol. Off. J. U. S. Can. Acad. Pathol. 34, 1634–1650. doi: 10.1038/s41379-021-00820-y
Asa, S. L., Mete, O., Perry, A., and Osamura, R. Y. (2022). Overview of the 2022 WHO classification of pituitary tumors. Endocr. Pathol. 33, 6–26. doi: 10.1007/s12022-022-09703-7
Asa, S. L., Mete, O., Riddle, N. D., and Perry, A. (2023). Multilineage pituitary neuroendocrine tumors (PitNETs) expressing PIT1 and SF1. Endocr. Pathol. 34, 273–278. doi: 10.1007/s12022-023-09777-x
Aydin, S., Comunoglu, N., Ahmedov, M. L., Korkmaz, O. P., Oz, B., Kadioglu, P., et al. (2019). Clinicopathologic characteristics and surgical treatment of Plurihormonal pituitary adenomas. World Neurosurg. 130, e765–e774. doi: 10.1016/j.wneu.2019.06.217
Barausse, M., Attanasio, R., Dallabonzana, D., Oppizzi, G., Veronese, S., Lasio, G., et al. (2000). From macroprolactinoma to concomitant ACTH-PRL hypersecretion with Cushing's disease. J. Endocrinol. Investig. 23, 107–111. doi: 10.1007/bf03343688
Batlle, E., and Clevers, H. (2017). Cancer stem cells revisited. Nat. Med. 23, 1124–1134. doi: 10.1038/nm.4409
Beck-Peccoz, P., Brucker-Davis, F., Persani, L., Smallridge, R. C., and Weintraub, B. D. (1996). Thyrotropin-secreting pituitary tumors. Endocr. Rev. 17, 610–638. doi: 10.1210/edrv-17-6-610
Bergman, L. M., Birts, C. N., Darley, M., Gabrielli, B., and Blaydes, J. P. (2009). CtBPs promote cell survival through the maintenance of mitotic fidelity. Mol. Cell. Biol. 29, 4539–4551. doi: 10.1128/mcb.00439-09
Buch, H., El-Hadd, T., Bicknell, J., Simpson, D. J., Farrell, W. E., and Clayton, R. N. (2002). Pituitary tumours are multiclonal from the outset: evidence from a case with dural metastases. Clin. Endocrinol. 56, 817–822. doi: 10.1046/j.1365-2265.2002.01524.x
Carreno, G., Gonzalez-Meljem, J. M., Haston, S., and Martinez-Barbera, J. P. (2017). Stem cells and their role in pituitary tumorigenesis. Mol. Cell. Endocrinol. 445, 27–34. doi: 10.1016/j.mce.2016.10.005
Caswell, D. R., and Swanton, C. (2017). The role of tumour heterogeneity and clonal cooperativity in metastasis, immune evasion and clinical outcome. BMC Med. 15:133. doi: 10.1186/s12916-017-0900-y
Chang, M., Yang, C., Bao, X., and Wang, R. (2020). Genetic and epigenetic causes of pituitary adenomas. Front. Endocrinol. 11:596554. doi: 10.3389/fendo.2020.596554
Chen, J., Hersmus, N., Van Duppen, V., Caesens, P., Denef, C., and Vankelecom, H. (2005). The adult pituitary contains a cell population displaying stem/progenitor cell and early embryonic characteristics. Endocrinology 146, 3985–3998. doi: 10.1210/en.2005-0185
Cheung, L. Y. M., and Camper, S. A. (2020). PROP1-dependent retinoic acid signaling regulates developmental pituitary morphogenesis and hormone expression. Endocrinology 61:bqaa002. doi: 10.1210/endocr/bqaa002
Chinezu, L., Vasiljevic, A., Trouillas, J., Lapoirie, M., Jouanneau, E., and Raverot, G. (2017). Silent somatotroph tumour revisited from a study of 80 patients with and without acromegaly and a review of the literature. Eur. J. Endocrinol. 176, 195–201. doi: 10.1530/eje-16-0738
Chu, I. M., Hengst, L., and Slingerland, J. M. (2008). The Cdk inhibitor p27 in human cancer: prognostic potential and relevance to anticancer therapy. Nat. Rev. Cancer 8, 253–267. doi: 10.1038/nrc2347
Clayton, R. N., and Farrell, W. E. (2001). Clonality of pituitary tumours: more complicated than initially envisaged? Brain Pathol. 11, 313–327. doi: 10.1111/j.1750-3639.2001.tb00402.x
Clayton, R. N., Pfeifer, M., Atkinson, A. B., Belchetz, P., Wass, J. A., Kyrodimou, E., et al. (2000). Different patterns of allelic loss (loss of heterozygosity) in recurrent human pituitary tumors provide evidence for multiclonal origins. Clin. Cancer Res. 6, 3973–3982.
Cox, B., Roose, H., Vennekens, A., and Vankelecom, H. (2017). Pituitary stem cell regulation: who is pulling the strings? J. Endocrinol. 234, R135–r158. doi: 10.1530/joe-17-0083
Cui, Y., Li, C., Jiang, Z., Zhang, S., Li, Q., Liu, X., et al. (2021). Single-cell transcriptome and genome analyses of pituitary neuroendocrine tumors. Neuro-Oncology 23, 1859–1871. doi: 10.1093/neuonc/noab102
D'addio, F., Maestroni, A., Assi, E., Ben Nasr, M., Amabile, G., Usuelli, V., et al. (2022). The IGFBP3/TMEM219 pathway regulates beta cell homeostasis. Nat. Commun. 13:684. doi: 10.1038/s41467-022-28360-2
Dahia, P. L., and Grossman, A. B. (1999). The molecular pathogenesis of corticotroph tumors. Endocr. Rev. 20, 136–155. doi: 10.1210/edrv.20.2.0358
Daly, A. F., Rixhon, M., Adam, C., Dempegioti, A., Tichomirowa, M. A., and Beckers, A. (2006). High prevalence of pituitary adenomas: a cross-sectional study in the province of Liege, Belgium. J. Clin. Endocrinol. Metab. 91, 4769–4775. doi: 10.1210/jc.2006-1668
De Ameida, J. P., Sherman, J. H., Salvatori, R., and Quiñones-Hinojosa, A. (2010). Pituitary stem cells: review of the literature and current understanding. Neurosurgery 67, 770–780. doi: 10.1227/01.Neu.0000373013.75994.Cd
Denko, N., Schindler, C., Koong, A., Laderoute, K., Green, C., and Giaccia, A. (2000). Epigenetic regulation of gene expression in cervical cancer cells by the tumor microenvironment. Clin. Cancer Res. 6, 480–487.
Ding, D., Starke, R. M., and Sheehan, J. P. (2014). Treatment paradigms for pituitary adenomas: defining the roles of radiosurgery and radiation therapy. J. Neuro-Oncol. 117, 445–457. doi: 10.1007/s11060-013-1262-8
Duello, T. M., and Halmi, N. S. (1977). Pituitary adenoma producing thyrotropin and prolactin. An immunocytochemical and electron microscopic study. Virchows Arch. A Pathol. Anat. Histol. 376, 255–265. doi: 10.1007/bf00432401
Durbin, A. D., Wang, T., Wimalasena, V. K., Zimmerman, M. W., Li, D., Dharia, N. V., et al. (2022). EP300 selectively controls the enhancer landscape of MYCN-amplified neuroblastoma. Cancer Discov. 12, 730–751. doi: 10.1158/2159-8290.Cd-21-0385
Egensperger, R., Scheithauer, B. W., Horvath, E., Kovacs, K., Giannini, C., Young, W. F., et al. (2001). Cushing's disease due to plurihormonal adrenocorticotropic hormone and gonadotropin-producing pituitary adenoma. Acta Neuropathol. 102, 398–403. doi: 10.1007/s004010100376
Elhadd, T. A., Ghosh, S., Teoh, W. L., Trevethick, K. A., Hanzely, Z., Dunn, L. T., et al. (2009). A patient with thyrotropinoma cosecreting growth hormone and follicle-stimulating hormone with low alpha-glycoprotein: a new subentity? Thyr. Off. J. Am. Thyr. Assoc. 19, 899–903. doi: 10.1089/thy.2008.0384
Ellsworth, B. S., and Stallings, C. E. (2018). Molecular mechanisms governing embryonic differentiation of pituitary Somatotropes. Trends Endocrinol Metab 29, 510–523. doi: 10.1016/j.tem.2018.04.009
Erickson, D., Scheithauer, B., Atkinson, J., Horvath, E., Kovacs, K., Lloyd, R. V., et al. (2009). Silent subtype 3 pituitary adenoma: a clinicopathologic analysis of the Mayo Clinic experience. Clin. Endocrinol. 71, 92–99. doi: 10.1111/j.1365-2265.2008.03514.x
Felix, I., Asa, S. L., Kovacs, K., Horvath, E., and Smyth, H. S. (1994). Recurrent plurihormonal bimorphous pituitary adenoma producing growth hormone, thyrotropin, and prolactin. Arch. Pathol. Lab. Med. 118, 66–70.
Fernandez, A., Karavitaki, N., and Wass, J. A. (2010). Prevalence of pituitary adenomas: a community-based, cross-sectional study in Banbury (Oxfordshire, UK). Clin. Endocrinol. 72, 377–382. doi: 10.1111/j.1365-2265.2009.03667.x
Frawley, L. S., and Boockfor, F. R. (1991). Mammosomatotropes: presence and functions in normal and neoplastic pituitary tissue. Endocr. Rev. 12, 337–355. doi: 10.1210/edrv-12-4-337
Garcia-Lavandeira, M., Quereda, V., Flores, I., Saez, C., Diaz-Rodriguez, E., Japon, M. A., et al. (2009). A GRFa2/Prop1/stem (GPS) cell niche in the pituitary. PLoS One 4:e4815. doi: 10.1371/journal.pone.0004815
Garcia-Lavandeira, M., Saez, C., Diaz-Rodriguez, E., Perez-Romero, S., Senra, A., Dieguez, C., et al. (2012). Craniopharyngiomas express embryonic stem cell markers (SOX2, OCT4, KLF4, and SOX9) as pituitary stem cells but do not coexpress RET/GFRA3 receptors. J. Clin. Endocrinol. Metab. 97, E80–E87. doi: 10.1210/jc.2011-2187
Gaston-Massuet, C., Andoniadou, C. L., Signore, M., Jayakody, S. A., Charolidi, N., Kyeyune, R., et al. (2011). Increased wingless (Wnt) signaling in pituitary progenitor/stem cells gives rise to pituitary tumors in mice and humans. Proc. Natl. Acad. Sci. U. S. A. 108, 11482–11487. doi: 10.1073/pnas.1101553108
Goh, Q., Low, Y., Abd Rani, N. H. B., and Tong, C. (2023). An aggressive Plurihormonal pituitary adenoma with thyrotropin, growth hormone, and prolactin excess. JCEM Case Rep. 1:luad016. doi: 10.1210/jcemcr/luad016
Graffeo, C. S., Yagnik, K. J., Carlstrom, L. P., Lakomkin, N., Bancos, I., Davidge-Pitts, C., et al. (2022). Pituitary adenoma incidence, management trends, and long-term outcomes: a 30-year population-based analysis. Mayo Clin. Proc. 97, 1861–1871. doi: 10.1016/j.mayocp.2022.03.017
Haston, S., Manshaei, S., and Martinez-Barbera, J. P. (2018). Stem/progenitor cells in pituitary organ homeostasis and tumourigenesis. J. Endocrinol. 236, R1–r13. doi: 10.1530/joe-17-0258
Herman, V., Fagin, J., Gonsky, R., Kovacs, K., and Melmed, S. (1990). Clonal origin of pituitary adenomas. J. Clin. Endocrinol. Metab. 71, 1427–1433. doi: 10.1210/jcem-71-6-1427
Hickman, R. A., Bruce, J. N., Otten, M., Khandji, A. G., Flowers, X. E., Siegelin, M., et al. (2021). Gonadotroph tumours with a low SF-1 labelling index are more likely to recur and are associated with enrichment of the PI3K-AKT pathway. Neuropathol. Appl. Neurobiol. 47, 415–427. doi: 10.1111/nan.12675
Higuchi, M., Yoshida, S., Ueharu, H., Chen, M., Kato, T., and Kato, Y. (2014). PRRX1 and PRRX2 distinctively participate in pituitary organogenesis and a cell-supply system. Cell Tissue Res. 357, 323–335. doi: 10.1007/s00441-014-1861-5
Ho, D. M., Hsu, C. Y., Ting, L. T., and Chiang, H. (2001). Plurihormonal pituitary adenomas: immunostaining of all pituitary hormones is mandatory for correct classification. Histopathology 39, 310–319. doi: 10.1046/j.1365-2559.2001.01204.x
Horiguchi, K., Ilmiawati, C., Fujiwara, K., Tsukada, T., Kikuchi, M., and Yashiro, T. (2012). Expression of chemokine CXCL12 and its receptor CXCR4 in folliculostellate (FS) cells of the rat anterior pituitary gland: the CXCL12/CXCR4 axis induces interconnection of FS cells. Endocrinology 153, 1717–1724. doi: 10.1210/en.2011-1937
Horvath, E., Kovacs, K., Smyth, H. S., Cusimano, M., and Singer, W. (2005). Silent adenoma subtype 3 of the pituitary--immunohistochemical and ultrastructural classification: a review of 29 cases. Ultrastruct. Pathol. 29, 511–524. doi: 10.1080/01913120500323514
Jentoft, M. E., Osamura, R. Y., Kovacs, K., Lloyd, R. V., and Scheithauer, B. W. (2012). Transdifferentiation of pituitary thyrotrophs to lactothyrotrophs in primary hypothyroidism: case report. Virchows Archiv. Int. J. Pathol. 461, 221–225. doi: 10.1007/s00428-012-1266-3
Jiang, Z., Gui, S., and Zhang, Y. (2012). Analysis of differential gene expression in plurihormonal pituitary adenomas using bead-based fiber-optic arrays. J. Neuro-Oncol. 108, 341–348. doi: 10.1007/s11060-011-0792-1
Kobalka, P. J., Huntoon, K., and Becker, A. P. (2021). Neuropathology of pituitary adenomas and Sellar lesions. Neurosurgery 88, 900–918. doi: 10.1093/neuros/nyaa548
Kovacs, K., Horvath, E., Asa, S. L., Stefaneanu, L., and Sano, T. (1989). Pituitary cells producing more than one hormone human pituitary adenomas. Trends Endocrinol Metab 1, 104–107. doi: 10.1016/1043-2760(89)90012-x
Kovacs, K., Horvath, E., Stefaneanu, L., Bilbao, J., Singer, W., Muller, P. J., et al. (1998). Pituitary adenoma producing growth hormone and adrenocorticotropin: a histological, immunocytochemical, electron microscopic, and in situ hybridization study. Case report. J. Neurosurg. 88, 1111–1115. doi: 10.3171/jns.1998.88.6.1111
Kurotani, R., Yasuda, M., Oyama, K., Egashira, N., Sugaya, M., Teramoto, A., et al. (2001). Expression of interleukin-6, interleukin-6 receptor (gp80), and the receptor's signal-transducing subunit (gp130) in human normal pituitary glands and pituitary adenomas. Mod. Pathol. Off. J. U. S. Can. Acad. Pathol. 14, 791–797. doi: 10.1038/modpathol.3880392
Kuzuya, N., Inoue, K., Ishibashi, M., Murayama, Y., Koide, Y., Ito, K., et al. (1990). Endocrine and immunohistochemical studies on thyrotropin (TSH)-secreting pituitary adenomas: responses of TSH, alpha-subunit, and growth hormone to hypothalamic releasing hormones and their distribution in adenoma cells. J. Clin. Endocrinol. Metab. 71, 1103–1111. doi: 10.1210/jcem-71-5-1103
Lamberts, S. W., and Macleod, R. M. (1990). Regulation of prolactin secretion at the level of the lactotroph. Physiol. Rev. 70, 279–318. doi: 10.1152/physrev.1990.70.2.279
Lamolet, B., Pulichino, A. M., Lamonerie, T., Gauthier, Y., Brue, T., Enjalbert, A., et al. (2001). A pituitary cell-restricted T box factor, Tpit, activates POMC transcription in cooperation with Pitx homeoproteins. Cells 104, 849–859. doi: 10.1016/s0092-8674(01)00282-3
Lan, L., and Behrens, A. (2023). Are there specific Cancer stem cell markers? Cancer Res. 83, 170–172. doi: 10.1158/0008-5472.Can-22-2053
Laporte, E., Vennekens, A., and Vankelecom, H. (2020). Pituitary remodeling throughout life: are resident stem cells involved? Front. Endocrinol. 11:604519. doi: 10.3389/fendo.2020.604519
Levy, A., and Lightman, S. (2003). Molecular defects in the pathogenesis of pituitary tumours. Front. Neuroendocrinol. 24, 94–127. doi: 10.1016/s0091-3022(03)00012-8
Li, Z., Zhou, Z., Tian, S., Zhang, K., An, G., Zhang, Y., et al. (2022). RPRM deletion preserves hematopoietic regeneration by promoting EGFR-dependent DNA repair and hematopoietic stem cell proliferation post ionizing radiation. Cell Biol. Int. 46, 2158–2172. doi: 10.1002/cbin.11900
Liu, X. H., Jiao, Y. C., and Wang, R. Z. (2018). The significance and role of the 2017 World Health Organization classification of tumors of the pituitary gland. Zhonghua Yi Xue Za Zhi 98, 641–642. doi: 10.3760/cma.j.issn.0376-2491.2018.09.001
Lodge, E. J., Santambrogio, A., Russell, J. P., Xekouki, P., Jacques, T. S., Johnson, R. L., et al. (2019). Homeostatic and tumourigenic activity of SOX2+ pituitary stem cells is controlled by the LATS/YAP/TAZ cascade. elife 8:e43996. doi: 10.7554/eLife.43996
Loeffler, J. S., and Shih, H. A. (2011). Radiation therapy in the management of pituitary adenomas. J. Clin. Endocrinol. Metab. 96, 1992–2003. doi: 10.1210/jc.2011-0251
Lopes, M. B. S. (2017). The 2017 World Health Organization classification of tumors of the pituitary gland: a summary. Acta Neuropathol. 134, 521–535. doi: 10.1007/s00401-017-1769-8
Luk, C. T., Kovacs, K., Rotondo, F., Horvath, E., Cusimano, M., and Booth, G. L. (2012). Plurihormonal pituitary adenoma immunoreactive for thyroid-stimulating hormone, growth hormone, follicle-stimulating hormone, and prolactin. Off. J. Am. Coll. Endocrinol. Am. Assoc. Clin. Endocrinol. 18, e121–e126. doi: 10.4158/ep12033.Cr
Maisnam, I., Dutta, D., Jain, R., Ghosh, S., Mukhopadhyay, S., and Chowdhury, S. (2012). Plurihormone secreting pituitary macroadenoma masquerading as thyrotoxicosis: clinical presentation and diagnostic challenges. Ind. J. Endocrinol. Metabol. 16, 315–317. doi: 10.4103/2230-8210.104073
Mariniello, K., Ruiz-Babot, G., Mcgaugh, E. C., Nicholson, J. G., Gualtieri, A., Gaston-Massuet, C., et al. (2019). Stem cells, self-renewal, and lineage commitment in the endocrine system. Front. Endocrinol. 10:772. doi: 10.3389/fendo.2019.00772
Matsumoto, R., Suga, H., Aoi, T., Bando, H., Fukuoka, H., Iguchi, G., et al. (2020). Congenital pituitary hypoplasia model demonstrates hypothalamic OTX2 regulation of pituitary progenitor cells. J. Clin. Invest. 130, 641–654. doi: 10.1172/jci127378
Matsumoto, R., and Takahashi, Y. (2021). Human pituitary development and application of iPSCs for pituitary disease. Cell. Mol. Life Sci. 78, 2069–2079. doi: 10.1007/s00018-020-03692-8
Matsuno, A., Sasaki, T., Mochizuki, T., Fujimaki, T., Sanno, N., Osamura, Y., et al. (1996). A case of pituitary somatotroph adenoma with concomitant secretion of growth hormone, prolactin, and adrenocorticotropic hormone — an adenoma derived from primordial stem cell, studied by immunohistochemistry, in situ hybridization, and cell culture. Acta Neurochir. 138, 1002–1007. doi: 10.1007/bf01411291
Matsuno, A., Teramoto, A., Takekoshi, S., Sanno, N., Osamura, R. Y., and Kirino, T. (1995). Expression of plurihormonal mRNAs in somatotrophic adenomas detected using a nonisotopic in situ hybridization method: comparison with lactotrophic adenomas. Hum. Pathol. 26, 272–279. doi: 10.1016/0046-8177(95)90057-8
Mccomb, D. J., Bayley, T. A., Horvath, E., Kovacs, K., and Kourides, I. A. (1984). Monomorphous plurihormonal adenoma of the human pituitary. A histologic, immunocytologic and ultrastructural study. Cancer 53, 1538–1544. doi: 10.1002/1097-0142(19840401)53:7<1538::aid-cncr2820530720>3.0.co;2-i
Mcgranahan, N., and Swanton, C. (2017). Clonal heterogeneity and tumor evolution: past, present, and the future. Cells 168, 613–628. doi: 10.1016/j.cell.2017.01.018
Melmed, S. (2020). Pituitary-tumor Endocrinopathies. N. Engl. J. Med. 382, 937–950. doi: 10.1056/NEJMra1810772
Mete, O., Cintosun, A., Pressman, I., and Asa, S. L. (2018). Epidemiology and biomarker profile of pituitary adenohypophysial tumors. Modern pathology: an official journal of the United States and Canadian academy of pathology. Inc 31, 900–909. doi: 10.1038/s41379-018-0016-8
Mete, O., Gomez-Hernandez, K., Kucharczyk, W., Ridout, R., Zadeh, G., Gentili, F., et al. (2016). Silent subtype 3 pituitary adenomas are not always silent and represent poorly differentiated monomorphous plurihormonal Pit-1 lineage adenomas. Mod. Pathol. Off. J. U. S. Can. Acad. Pathol. 29, 131–142. doi: 10.1038/modpathol.2015.151
Mete, O., and Lopes, M. B. (2017). Overview of the 2017 WHO classification of pituitary tumors. Endocr. Pathol. 28, 228–243. doi: 10.1007/s12022-017-9498-z
Mitrofanova, L. B., Konovalov, P. V., Krylova, J. S., Polyakova, V. O., and Kvetnoy, I. M. (2017). Plurihormonal cells of normal anterior pituitary: facts and conclusions. Oncotarget 8, 29282–29299. doi: 10.18632/oncotarget.16502
Molitch, M. E. (2017). Diagnosis and treatment of pituitary adenomas: a review. JAMA 317, 516–524. doi: 10.1001/jama.2016.19699
Moszczyńska, E., Grajkowska, W., Maksymowicz, M., Malicka, J., Szalecki, M., and Prokop-Piotrkowska, M. (2021). Giant plurihormonal pituitary adenoma in a child - case study. J. Pediatr. Endocrinol. Metabol. 34, 1469–1473. doi: 10.1515/jpem-2021-0094
Müller, E. E., Locatelli, V., and Cocchi, D. (1999). Neuroendocrine control of growth hormone secretion. Physiol. Rev. 79, 511–607. doi: 10.1152/physrev.1999.79.2.511
Murata, Y., Ando, H., Nagasaka, T., Takahashi, I., Saito, K., Fukugaki, H., et al. (2003). Successful pregnancy after bromocriptine therapy in an anovulatory woman complicated with ovarian hyperstimulation caused by follicle-stimulating hormone-producing plurihormonal pituitary microadenoma. J. Clin. Endocrinol. Metab. 88, 1988–1993. doi: 10.1210/jc.2002-021820
Nakakura, T., Sato, M., Suzuki, M., Hatano, O., Takemori, H., Taniguchi, Y., et al. (2009). The spatial and temporal expression of delta-like protein 1 in the rat pituitary gland during development. Histochem. Cell Biol. 131, 141–153. doi: 10.1007/s00418-008-0494-8
Neou, M., Villa, C., Armignacco, R., Jouinot, A., Raffin-Sanson, M. L., Septier, A., et al. (2020). Pangenomic classification of pituitary neuroendocrine tumors. Cancer Cell 37, 123–134.e5. doi: 10.1016/j.ccell.2019.11.002
Nf, L., and Ai, M. (2023). Refractory Pit1 plurihormonal tumours and thyrotroph adenomas. Pituitary 26, 182–186. doi: 10.1007/s11102-023-01312-9
Ohtsuka, T., Ishibashi, M., Gradwohl, G., Nakanishi, S., Guillemot, F., and Kageyama, R. (1999). Hes1 and Hes5 as notch effectors in mammalian neuronal differentiation. EMBO J. 18, 2196–2207. doi: 10.1093/emboj/18.8.2196
Oki, K., Yamane, K., Oda, Y., Kamei, N., Watanabe, H., Tominaga, A., et al. (2009). Combined acromegaly and subclinical Cushing disease related to high-molecular-weight adrenocorticotropic hormone. J. Neurosurg. 110, 369–373. doi: 10.3171/2008.8.Jns08154
Osamura, R. Y., Egashira, N., Kajiya, H., Takei, M., Tobita, M., Miyakoshi, T., et al. (2009). Pathology, pathogenesis and therapy of growth hormone (GH)-producing pituitary adenomas: technical advances in histochemistry and their contribution. Acta Histochem. Cytochem. 42, 95–104. doi: 10.1267/ahc.09004
Ostrom, Q. T., Gittleman, H., Liao, P., Rouse, C., Chen, Y., Dowling, J., et al. (2014). CBTRUS statistical report: primary brain and central nervous system tumors diagnosed in the United States in 2007-2011. Neuro-Oncology 16 Suppl 4:iv1-63. doi: 10.1093/neuonc/nou223
Paez-Pereda, M., Kuchenbauer, F., Arzt, E., and Stalla, G. K. (2005). Regulation of pituitary hormones and cell proliferation by components of the extracellular matrix. Brazilian journal of medical and biological research =. Revista brasileira de pesquisas medicas e biologicas 38, 1487–1494. doi: 10.1590/s0100-879x2005001000005
Parvin, R., Saito-Hakoda, A., Shimada, H., Shimizu, K., Noro, E., Iwasaki, Y., et al. (2017). Role of NeuroD1 on the negative regulation of Pomc expression by glucocorticoid. PLoS One 12:e0175435. doi: 10.1371/journal.pone.0175435
Pawlikowski, M., Kunert-Radek, J., and Radek, M. (2010). Plurihormonality of pituitary adenomas in light of immunohistochemical studies. Endokrynol. Pol. 61, 63–66.
Pereira, B. D., Raimundo, L., Mete, O., Oliveira, A., Portugal, J., and Asa, S. L. (2016). Monomorphous Plurihormonal pituitary adenoma of Pit-1 lineage in a Giant adolescent with central hyperthyroidism. Endocr. Pathol. 27, 25–33. doi: 10.1007/s12022-015-9395-2
Raappana, A., Koivukangas, J., Ebeling, T., and Pirilä, T. (2010). Incidence of pituitary adenomas in northern Finland in 1992-2007. J. Clin. Endocrinol. Metab. 95, 4268–4275. doi: 10.1210/jc.2010-0537
Radian, S., Coculescu, M., and Morris, J. F. (2003). Somatotroph to thyrotroph cell transdifferentiation during experimental hypothyroidism - a light and electron-microscopy study. J. Cell. Mol. Med. 7, 297–306. doi: 10.1111/j.1582-4934.2003.tb00230.x
Rasul, F. T., Jaunmuktane, Z., Khan, A. A., Phadke, R., and Powell, M. (2014). Plurihormonal pituitary adenoma with concomitant adrenocorticotropic hormone (ACTH) and growth hormone (GH) secretion: a report of two cases and review of the literature. Acta Neurochir. 156, 141–146. doi: 10.1007/s00701-013-1890-y
Rizzoti, K., Akiyama, H., and Lovell-Badge, R. (2013). Mobilized adult pituitary stem cells contribute to endocrine regeneration in response to physiological demand. Cell Stem Cell 13, 419–432. doi: 10.1016/j.stem.2013.07.006
Roca, E., Mattogno, P. P., Porcelli, T., Poliani, L., Belotti, F., Schreiber, A., et al. (2018). Plurihormonal ACTH-GH pituitary adenoma: case report and systematic literature review. World Neurosurg. 114, e158–e164. doi: 10.1016/j.wneu.2018.02.120
Rouault, J. P., Falette, N., Guéhenneux, F., Guillot, C., Rimokh, R., Wang, Q., et al. (1996). Identification of BTG2, an antiproliferative p53-dependent component of the DNA damage cellular response pathway. Nat. Genet. 14, 482–486. doi: 10.1038/ng1296-482
Sabatino, M. E., Grondona, E., and De Paul, A. L. (2022). Architects of pituitary tumour growth. Front. Endocrinol. 13:924942. doi: 10.3389/fendo.2022.924942
Salehi, F., Cohen, S., Syro, L. V., Uribe, H., Horvath, E., Kovacs, K., et al. (2006). Plurihormonality in pituitary adenomas associated with acromegaly. Endocr. Pathol. 17, 291–296. doi: 10.1385/ep:17:3:291
Salomon, M. P., Wang, X., Marzese, D. M., Hsu, S. C., Nelson, N., Zhang, X., et al. (2018). The Epigenomic landscape of pituitary adenomas reveals specific alterations and differentiates among acromegaly, Cushing’s disease and endocrine-inactive subtypes. Clin. Cancer Res. 24, 4126–4136. doi: 10.1158/1078-0432.Ccr-17-2206
Sav, A., Rotondo, F., Syro, L. V., Scheithauer, B. W., and Kovacs, K. (2012). Biomarkers of pituitary neoplasms. Anticancer Res. 32, 4639–4654.
Saveanu, A., Morange-Ramos, I., Gunz, G., Dufour, H., Enjalbert, A., and Jaquet, P. (2001). A luteinizing hormone-, alpha-subunit-and prolactin-secreting pituitary adenoma responsive to somatostatin analogs: in vivo and in vitro studies. Eur. J. Endocrinol. 145, 35–41. doi: 10.1530/eje.0.1450035
Shi, R., Wan, X., Yan, Z., Tan, Z., Liu, X., and Lei, T. (2022). Clinicopathological characteristics of Plurihormonal pituitary adenoma. Front. Surg. 9:826720. doi: 10.3389/fsurg.2022.826720
Shimatsu, A., Murabe, H., Nakamura, Y., Mizuta, H., Ihara, C., and Nakao, K. (1999). Long-term treatment with bromocriptine of a plurihormonal pituitary adenoma secreting thyrotropin, growth hormone and prolactin. Endocr. J. 46, 159–165. doi: 10.1507/endocrj.46.159
Sjöstedt, E., Bollerslev, J., Mulder, J., Lindskog, C., Pontén, F., and Casar-Borota, O. (2017). A specific antibody to detect transcription factor T-pit: a reliable marker of corticotroph cell differentiation and a tool to improve the classification of pituitary neuroendocrine tumours. Acta Neuropathol. 134, 675–677. doi: 10.1007/s00401-017-1768-9
Sommergruber, M., Yaman, C., Ebner, T., Hartl, J., Moser, M., and Tews, G. (2000). A case of ovarian hyperstimulation during pituitary down-regulation caused by plurihormonal macroadenoma. Fertil. Steril. 73, 1059–1060. doi: 10.1016/s0015-0282(00)00473-8
Srirangam Nadhamuni, V., and Korbonits, M. (2020). Novel insights into pituitary tumorigenesis: genetic and epigenetic mechanisms. Endocr. Rev. 41, 821–846. doi: 10.1210/endrev/bnaa006
Stefaneanu, L., Kovacs, K., Lloyd, R. V., Scheithauer, B. W., Young, W. F., Sano, T., et al. (1992). Pituitary lactotrophs and somatotrophs in pregnancy: a correlative in situ hybridization and immunocytochemical study. Virchows Arch. B Cell Pathol. Incl. Mol. Pathol. 62, 291–296. doi: 10.1007/bf02899695
Steinbichler, T. B., Dudás, J., Skvortsov, S., Ganswindt, U., Riechelmann, H., and Skvortsova, I. I. (2018). Therapy resistance mediated by cancer stem cells. Semin. Cancer Biol. 53, 156–167. doi: 10.1016/j.semcancer.2018.11.006
Swearingen, B. (2012). Update on pituitary surgery. J. Clin. Endocrinol. Metab. 97, 1073–1081. doi: 10.1210/jc.2011-3237
Szeto, D. P., Rodriguez-Esteban, C., Ryan, A. K., O'connell, S. M., Liu, F., Kioussi, C., et al. (1999). Role of the Bicoid-related homeodomain factor Pitx1 in specifying hindlimb morphogenesis and pituitary development. Genes Dev. 13, 484–494. doi: 10.1101/gad.13.4.484
Tahara, S., Kurotani, R., Ishii, Y., Sanno, N., Teramoto, A., and Osamura, R. Y. (2002). A case of Cushing's disease caused by pituitary adenoma producing adrenocorticotropic hormone and growth hormone concomitantly: aberrant expression of transcription factors NeuroD1 and Pit-1 as a proposed mechanism. Mod. Pathol. Off. J. U. S. Can. Acad. Pathol. 15, 1102–1105. doi: 10.1097/01.Mp.0000030451.28828.00
Takiguchi, T., Koide, H., Nagano, H., Nakayama, A., Fujimoto, M., Tamura, A., et al. (2017). Multihormonal pituitary adenoma concomitant with Pit-1 and Tpit lineage cells causing acromegaly associated with subclinical Cushing's disease: a case report. BMC Endocr. Disord. 17:54. doi: 10.1186/s12902-017-0203-5
Tatsumoto, T., Xie, X., Blumenthal, R., Okamoto, I., and Miki, T. (1999). Human ECT2 is an exchange factor for rho GTPases, phosphorylated in G2/M phases, and involved in cytokinesis. J. Cell Biol. 147, 921–928. doi: 10.1083/jcb.147.5.921
Teramoto, A., Sanno, N., Tahara, S., and Osamura, Y. R. (2004). Pathological study of thyrotropin-secreting pituitary adenoma: plurihormonality and medical treatment. Acta Neuropathol. 108, 147–153. doi: 10.1007/s00401-004-0863-x
Thapar, K., Stefaneanu, L., Kovacs, K., Horvath, E., and Asa, S. L. (1993). Plurihormonal pituitary tumors: beyond the one cell-one hormone theory. Endocr. Pathol. 4, 1–3. doi: 10.1007/bf02914482
Tomita, T., Kuziez, M., and Watanabe, I. (1981). Double tumors of anterior and posterior pituitary gland. Acta Neuropathol. 54, 161–164. doi: 10.1007/bf00689411
Tong, A., Xia, W., Qi, F., Jin, Z., Yang, D., Zhang, Z., et al. (2013). Hyperthyroidism caused by an ectopic thyrotropin-secreting tumor of the nasopharynx: a case report and review of the literature. Thyr. Off. J. Am. Thyr. Assoc. 23, 1172–1177. doi: 10.1089/thy.2012.0574
Tordjman, K. M., Greenman, Y., Ram, Z., Hershkovitz, D., Aizenstein, O., Ariel, O., et al. (2019). Plurihormonal pituitary tumor of Pit-1 and SF-1 lineages, with synchronous collision Corticotroph tumor: a possible stem cell phenomenon. Endocr. Pathol. 30, 74–80. doi: 10.1007/s12022-018-9562-3
Tritos, N. A., and Miller, K. K. (2023). Diagnosis and Management of Pituitary Adenomas: a review. JAMA 329, 1386–1398. doi: 10.1001/jama.2023.5444
T'sjoen, G., Defeyter, I., Van De Saffele, J., Rubens, R., and Vandeweghe, M. (2002). Macroprolactinoma associated with Cushing's disease, successfully treated with cabergoline. J. Endocrinol. Investig. 25, 172–175. doi: 10.1007/bf03343983
Turchini, J., Sioson, L., Clarkson, A., Sheen, A., and Gill, A. J. (2021). PD-L1 is preferentially expressed in PIT-1 positive pituitary neuroendocrine tumours. Endocr. Pathol. 32, 408–414. doi: 10.1007/s12022-021-09673-2
Vandeva, S., Daly, A. F., Petrossians, P., Zacharieva, S., and Beckers, A. (2019). Somatic and germline mutations in the pathogenesis of pituitary adenomas. Eur. J. Endocrinol. 181, R235–r254. doi: 10.1530/eje-19-0602
Vankelecom, H., and Roose, H. (2017). The stem cell connection of pituitary tumors. Front. Endocrinol. 8:339. doi: 10.3389/fendo.2017.00339
Vidal, S., Horvath, E., Kovacs, K., Cohen, S. M., Lloyd, R. V., and Scheithauer, B. W. (2000). Transdifferentiation of somatotrophs to thyrotrophs in the pituitary of patients with protracted primary hypothyroidism. Virchows Archiv. Int. J. Pathol. 436, 43–51. doi: 10.1007/pl00008197
Vidal, S., Syro, L., Horvath, E., Uribe, H., and Kovacs, K. (1999). Ultrastructural and immunoelectron microscopic study of three unusual plurihormonal pituitary adenomas. Ultrastruct. Pathol. 23, 141–148. doi: 10.1080/019131299281635
Villa, C., Baussart, B., Assié, G., Raverot, G., and Roncaroli, F. (2023). The World Health Organization classifications of pituitary neuroendocrine tumours: a clinico-pathological appraisal. Endocr. Relat. Cancer 30:e230021. doi: 10.1530/erc-23-0021
Villa, A., Cervasio, M., De Caro, D. B., and Cavallo, L. M. (2014). A rare case of ACTH-LH plurihormonal pituitary adenoma: letter to the editor. Acta Neurochir. 156, 1389–1391. doi: 10.1007/s00701-014-2027-7
Vora, T. K., and Karunakaran, S. (2017). Thyrotropic pituitary adenoma with plurihormonal immunoreactivity. Neurol. India 65, 1162–1164. doi: 10.4103/neuroindia.NI_577_16
Wang, J., Thomas, H. R., Li, Z., Yeo, N. C. F., Scott, H. E., Dang, N., et al. (2021). Puma, noxa, p53, and p63 differentially mediate stress pathway induced apoptosis. Cell Death Dis. 12:659. doi: 10.1038/s41419-021-03902-6
Wang, P., Wang, T., Yang, Y., Yu, C., Liu, N., and Yan, C. (2017). Detection of programmed death ligand 1 protein and CD8+ lymphocyte infiltration in plurihormonal pituitary adenomas: a case report and review of the literatures. Medicine 96:e9056. doi: 10.1097/md.0000000000009056
Willis, T. L., Lodge, E. J., Andoniadou, C. L., and Yianni, V. (2022). Cellular interactions in the pituitary stem cell niche. Cell. Mol. Life Sci. 79:612. doi: 10.1007/s00018-022-04612-8
Winningham, A. H., and Camper, S. A. (2023). Pituitary stem cell regulation by Zeb2 and BMP signaling. Endocrinology 164:bqad016. doi: 10.1210/endocr/bqad016
Würth, R., Barbieri, F., Pattarozzi, A., Gaudenzi, G., Gatto, F., Fiaschi, P., et al. (2017). Phenotypical and pharmacological characterization of stem-like cells in human pituitary adenomas. Mol. Neurobiol. 54, 4879–4895. doi: 10.1007/s12035-016-0025-x
Würth, R., Thellung, S., Corsaro, A., Barbieri, F., and Florio, T. (2020). Experimental evidence and clinical implications of pituitary adenoma stem cells. Front. Endocrinol. 11:54. doi: 10.3389/fendo.2020.00054
Xu, M., Shorts-Cary, L., Knox, A. J., Kleinsmidt-Demasters, B., Lillehei, K., and Wierman, M. E. (2009). Epidermal growth factor receptor pathway substrate 8 is overexpressed in human pituitary tumors: role in proliferation and survival. Endocrinology 150, 2064–2071. doi: 10.1210/en.2008-1265
Xu, Q., Yuan, X., Tunici, P., Liu, G., Fan, X., Xu, M., et al. (2009). Isolation of tumour stem-like cells from benign tumours. Br. J. Cancer 101, 303–311. doi: 10.1038/sj.bjc.6605142
Yao, H., Xie, W., Dai, Y., Liu, Y., Gu, W., Li, J., et al. (2022). TRIM65 determines the fate of a novel subtype of pituitary neuroendocrine tumors via ubiquitination and degradation of TPIT. Neuro-Oncology 24, 1286–1297. doi: 10.1093/neuonc/noac053
Yevtodiyenko, A., and Schmidt, J. V. (2006). Dlk1 expression marks developing endothelium and sites of branching morphogenesis in the mouse embryo and placenta. Dev. Dynam. Off. Publ. Am. Assoc. Anatom. 235, 1115–1123. doi: 10.1002/dvdy.20705
Ying, J., Srivastava, G., Hsieh, W. S., Gao, Z., Murray, P., Liao, S. K., et al. (2005). The stress-responsive gene GADD45G is a functional tumor suppressor, with its response to environmental stresses frequently disrupted epigenetically in multiple tumors. Clin. Cancer Res. 11, 6442–6449. doi: 10.1158/1078-0432.Ccr-05-0267
Yoshida, A., Sen, C., Asa, S. L., and Rosenblum, M. K. (2008). Composite pituitary adenoma and craniopharyngioma?: an unusual sellar neoplasm with divergent differentiation. Am. J. Surg. Pathol. 32, 1736–1741. doi: 10.1097/PAS.0b013e3181753abd
Keywords: pituitary adenoma, plurihormonal, stem cell, immunohistochemistry, pathogenesis
Citation: Cai Y, Liu S, Zhao X, Ren L, Liu X, Gang X and Wang G (2024) Pathogenesis, clinical features, and treatment of plurihormonal pituitary adenoma. Front. Neurosci. 17:1323883. doi: 10.3389/fnins.2023.1323883
Edited by:
Vadim Byvaltsev, Irkutsk State Medical University, RussiaReviewed by:
Yuan Shan, Baylor Scott and White Health, United StatesMaria Eugenia Sabatino, Instituto de Ciencia y Tecnología de Alimentos Córdoba (ICyTAC-CONICET), Argentina
Copyright © 2024 Cai, Liu, Zhao, Ren, Liu, Gang and Wang. This is an open-access article distributed under the terms of the Creative Commons Attribution License (CC BY). The use, distribution or reproduction in other forums is permitted, provided the original author(s) and the copyright owner(s) are credited and that the original publication in this journal is cited, in accordance with accepted academic practice. No use, distribution or reproduction is permitted which does not comply with these terms.
*Correspondence: Xiaokun Gang, gangxk@jlu.edu.cn; Guixia Wang, gwang168@jlu.edu.cn