- Department of Critical Care Medicine, Affiliated Hangzhou First People’s Hospital, Zhejiang University School of Medicine, Hangzhou, China
Sepsis-associated encephalopathy (SAE) is an acute neurological deficit caused by severe sepsis without signs of direct brain infection, characterized by the systemic inflammation and disturbance of the blood–brain barrier. SAE is associated with a poor prognosis and high mortality in patients with sepsis. Survivors may exhibit long-term or permanent sequelae, including behavioral changes, cognitive impairment, and decreased quality of life. Early detection of SAE can help ameliorate long-term sequelae and reduce mortality. Half of the patients with sepsis suffer from SAE in the intensive care unit, but its physiopathological mechanism remains unknown. Therefore, the diagnosis of SAE remains a challenge. The current clinical diagnosis of SAE is a diagnosis of exclusion; this makes the process complex and time-consuming and delays early intervention by clinicians. Furthermore, the scoring scales and laboratory indicators involved have many problems, including insufficient specificity or sensitivity. Thus, a new biomarker with excellent sensitivity and specificity is urgently needed to guide the diagnosis of SAE. MicroRNAs have attracted attention as putative diagnostic and therapeutic targets for neurodegenerative diseases. They exist in various body fluids and are highly stable. Based on the outstanding performance of microRNAs as biomarkers for other neurodegenerative diseases, it is reasonable to infer that microRNAs will be excellent biomarkers for SAE. This review explores the current diagnostic methods for sepsis-associated encephalopathy (SAE). We also explore the role that microRNAs could play in SAE diagnosis and if they can be used to make the SAE diagnosis faster and more specific. We believe that our review makes a significant contribution to the literature because it summarizes some of the important diagnostic methods for SAE, highlighting their advantages and disadvantages in clinical use, and could benefit the field as it highlights the potential of miRNAs as SAE diagnostic markers.
1. Introduction
Sepsis is defined as life-threatening organ dysfunction caused by a dysregulated host response to infection (Singer et al., 2016). Sepsis is a critical healthcare problem, affecting tens of millions of people worldwide each year, with one in three to one in six deaths (Fleischmann et al., 2016; Reinhart et al., 2017; Rhee et al., 2017; Fleischmann-Struzek et al., 2020; Rudd et al., 2020). According to the National Institute of Health, sepsis-associated encephalopathy (SAE) is an acute diffuse neurological deficit caused by severe sepsis without signs of direct infection of the brain. It is characterized by systemic inflammation, disturbance of the blood-brain barrier (BBB), and changes in consciousness that can range from confusion to delirium or even lead to coma induction (Ebersoldt et al., 2007). SAE is one of the main manifestations of sepsis, which can manifest as the first organ dysfunction and contribute to a worse prognosis in patients with sepsis. The mortality rate increases with increasing severity (Gofton and Young, 2012) and affects up to 70% of patients with sepsis (Widmann and Heneka, 2014). Patients who survive SAE may exhibit long-term or permanent sequelae, including behavioral changes, cognitive impairment, decreased quality of life, or premature death (Feng et al., 2019). Data suggest that SAE occurs in 9 to 71% of cases (Sprung et al., 1990; Iacobone et al., 2009; Zhang et al., 2012; Molnár et al., 2018) and is one of the most common causes of encephalopathy in intensive care units (ICU) all over the world (Bleck et al., 1993; Eidelman et al., 1996; Ely et al., 2004). Differences in the incidence of SAE suggest that current diagnostic criteria are limited or not easily enforceable clinically. Given the enormous health impact of SAE, specific, accurate, and easy-to-use new diagnostic criteria for this condition are urgently needed.
2. Current diagnostic methods
Currently, SAE is still a diagnosis of exclusion. Based on the daily neurological assessment, when sepsis-related encephalopathy is suspected, it is first necessary to judge the patient’s mental status according to the rating scale and determine the manifestations of encephalopathy, which may be mild in the early stages. Secondly, an unabridged neurological examination is completed and necessary auxiliary tests are conducted to assist in the diagnosis or rule out other diagnoses. At the same time, it is essential to exclude the interference of sedatives, direct infection of the central nervous system, cerebrovascular disease, traumatic brain injury, brain tumors, metabolic encephalopathy, and drug side effects. It is also necessary to check the source of infection, obtain appropriate culture and drug sensitivity test results, actively treat sepsis, and give symptomatic and supportive treatment (Figure 1; Iacobone et al., 2009).
The rest of this paper describes the universal rating scales, auxiliary tests, and biomarkers used to diagnose SAE.
3. Rating scales
The general ward confusion assessment method (CAM) or the confusion assessment method in the intensive care unit (CAM-ICU) is used to assess a patient’s mental status. In their respective environments, multiple studies have demonstrated that CAM and CAM-ICU exhibit excellent sensitivity and specificity (CAM: 94–100%, 89–95%; CAM-ICU: 97–100%, 89–100%) (Inouye et al., 1990; Ely et al., 2001a,b; Vreeswijk et al., 2008; Wei et al., 2008; Toro et al., 2010). However, studies have shown that CAM is most likely to miss patients with quiet delirium (Khan et al., 2012), whereas CAM-ICU, although research has shown that it is a good ICU delirium screening scale, only has about 41–47% sensitivity when ICU nurses use it (van Eijk et al., 2011).
In ICU, sedatives are frequently used to improve the comfort and safety of patient, aid in the synchronization of patients with mechanical ventilation, and prevent accidental extubation (Devlin et al., 2001; Chen et al., 2022). However, the use of sedatives may have some side effects, such as excessive sedation leading to inability to awaken the patient even if the sedative is stopped or awakening accompanied by agitation, both of which will interfere with the judgment of the patient’s mental status (Ebersoldt et al., 2007; Van Rompaey et al., 2009; Lahariya et al., 2014; Junior et al., 2022). Therefore, before making a judgment, the sedation state should be evaluated through the Richmond Restlessness and Sedation Scale (RASS), which demonstrates excellent interrater reliability and criterion, construct, and face validity (Ely et al., 2003). A score of 0 means that the patient is in a conscious and natural state (Ely et al., 2003). It is generally recommended that a scale ≥ −1 be used for neurological examination or CAM-ICU evaluation (Ely et al., 2003; Van Rompaey et al., 2009; Lahariya et al., 2014; Junior et al., 2022).
The Glasgow Coma Scale (GCS) is a commonly used non-specific method to assess the degree of a coma and has been associated with the prognosis of sepsis-related encephalopathy (Sonneville et al., 2017). However, this study is based on the premise that GCS is one of the diagnostic criteria, and it cannot explain the advantages of GCS as a diagnostic method. The main limitations of GCS are that verbal responses are not assessable in mechanically ventilated patients and that brainstem examination is not directly considered (Sharshar et al., 2014).
The above scoring scales are non-specific methods and can therefore only be used for screening rather than diagnosis.
4. Neurological examination
Once the patient has altered cognition or consciousness, a detailed neurological examination is performed. This examination examines verbal stimuli, painful stimuli, brainstem reflexes, motor responses, and breathing patterns. Painful stimuli can elicit focal movements, withdrawal, postural reflexes, or unresponsiveness. Brainstem examination includes assessment of pupils and pupillary reactivity, spontaneous eye position and movements, vestibular-eyelid reflex, corneal reflexes, and cough and gag reflexes (Stevens and Bhardwaj, 2006; Sharshar et al., 2011). In deeply sedated patients, examination of pupil size, pupillary light reflex, corneal reflex, pain upon stimulation with a finger, ocular head response, and cough reflex may be more beneficial (Iacobone et al., 2009).
5. Metabolic analysis
Performing a comprehensive metabolic analysis includes a complete blood count and analyses of the blood glucose, blood gases, electrolytes, creatinine, ammonia, and alanine aminotransferase levels to look for other known causes of altered mental status, such as hypovolemia, hypoglycemia, diabetic ketoacidosis, uremic encephalopathy, hepatic encephalopathy, hypokalemia, hypoxemia, hypercapnia, and hyperemia (Iacobone et al., 2009; Mazeraud et al., 2020).
6. Electrophysiological examination
An electroencephalogram (EEG) is very sensitive in diagnosing sepsis-related encephalopathy. Young et al. (1992) studied 69 sepsis patients, 49 of whom had some degree of encephalopathy, classified as mild or severe. They identified five classes of gradually progressive EEG patterns: class 1 normal EEG, class 2: excessive θ rhythms, class 3: increased δ rhythm, class 4: triphasic, and class 5: suppression or burst suppression. Mortality in SAE is associated with the severity of EEG abnormalities: 0% for normal EEG, 19% for excessive θ rhythms, 36% for increased δ rhythm, 50% for triphasic waves, and 67% for suppression or burst suppression (Young et al., 1992). However, EEG is not highly specific, and EEG abnormalities are also common in other neurological injuries (Young et al., 1992; Hosokawa et al., 2014), and the use of sedatives can also lead to abnormal EEG signals (Young et al., 1992; Purdon et al., 2013; Akeju et al., 2014; Hosokawa et al., 2014).
Abnormal somatosensory evoked potentials (SEP) may also be beneficial electrophysiological markers of SAE (Zauner et al., 2002; Rosengarten et al., 2012; Hosokawa et al., 2014). Studies by Zauner et al. (2002) revealed that subcortical and cortical SEP pathways were impaired in 84 and 34% of all patients, respectively. In addition, subclinical cerebral focal signs were present in 24% of the subcortical SEP pathways and 6% of the cortical SEP pathways, but the results were not significantly different (Zauner et al., 2002). The advantage of SEP over EEG is that it is not affected by continuous sedation; however, the assessment of SEP is too cumbersome and may not be used routinely in the ICU (Zauner et al., 2002).
Despite more than 20 years of research, the role of electrophysiological examination in the diagnosis of SAE remains unclear, and most studies have significant limitations (Hosokawa et al., 2014).
7. Neuroimaging
Sharshar et al. (2007) showed that an “MRI of SAE included multiple ischemic strokes and white matter lesions at the level of the centrum semiovale, mainly surrounding Virchow–Robin spaces, ranging from small multiple areas to diffuse lesions, and characterized by hyperintensity on FLAIR images. The major lesions were also characterized by reduced signal on diffusion isotropic images and increased apparent diffusion coefficient” (Sharshar et al., 2007). Some patients with SAE exhibit vasogenic edema, which may suggest an increased BBB permeability or decreased brain autoregulation (Sharshar et al., 2007; Piazza et al., 2009; Polito et al., 2013).
A transcranial doppler ultrasound (TCD) has been used to measure cerebral blood flow velocity in SAE, where the impairment of cerebrovascular autoregulation can be founded, especially in the preclinical phase of SAE. During the first 2 days, most patients with severe sepsis have impaired cerebrovascular autoregulation (AR), and impaired AR is associated with SAE. In some cases, however, TCD did not find differences in cerebral perfusion (Schramm et al., 2012; Pierrakos et al., 2014).
Although neuroimaging in patients with SAE has a few defining characteristics and can be beneficial for diagnosis, its benefits have not been confirmed by multi-center clinical studies; therefore it is mostly used to rule out abscesses, tumors, trauma, and cerebrovascular diseases. Neuroimaging should be as perfect as possible in the case of focal neurological symptoms and changes in mental status.
8. Biomarkers
NT-proCNP, S100B, NSE, and NF are biomarkers of endothelial dysfunction, microglial activation, and brain injury with concomitant axonal damage. Although their enhancements help diagnose SAE (Yao et al., 2014; Ehler et al., 2017; Ehler et al., 2019a,b; Khan et al., 2020), their sensitivity and specificity are not enough. Moreover they have not been clinically validated by multi centers, which limits their clinical use (Table 1).
9. Diagnostic value of microRNAs in SAE
MicroRNAs non-coding RNAs that are about 22-nt long; they induce mRNA degradation or repress protein translation by binding to target messenger RNAs (mRNAs), thus playing a role in gene silencing (Bartel, 2009; Jonas and Izaurralde, 2015; O’Brien et al., 2018). microRNAs play significant roles in various neurobiological processes, such as differentiation, cell proliferation, metabolism, cell cycle regulation, and apoptosis (Nowakowski et al., 2018).
The high degree of conservation of microRNAs determines that a single miRNA can target multiple genes and regulate different biological pathways (Valinezhad Orang et al., 2014). Under pathological conditions, such as neurodegenerative diseases, network-based patterns of gene regulation converge on distinct biological pathways to drive disease phenotypes (Salta et al., 2016; Wilk and Braun, 2018; Juźwik et al., 2019).
In addition, microRNAs have other advantages as biomarkers. For example, they are present in various bodily fluids, including blood, urine, and saliva, thus allowing relatively non-invasive sample collection (Ciesla et al., 2011). In addition to accessibility, microRNAs are highly stable in various body fluids and different biological specimens and can be efficiently detected and amplified by means of molecular biology tools such as real-time PCR or small RNA sequencing (Mitchell et al., 2008; Ciesla et al., 2011; Enuka et al., 2016; Beltrán-García et al., 2020). Moreover, current evidence suggests that the BBB does not prevent the passage of microRNAs between CSF and blood (Stoicea et al., 2016). microRNAs can enter the blood from brain tissue through the BBB under pathological conditions, making them potential biomarkers for central nervous system diseases (Dalkara and Alarcon-Martinez, 2015).
Previous studies have shown that microRNAs, principal gene regulators in different biological pathways, are closely related to disease progression and play crucial roles in the initiation and progression of neurodegenerative diseases as well as in neuronal differentiation and synaptic plasticity (Lehmann et al., 2012; Pasquinelli, 2012; Sohel, 2016; Nowakowski et al., 2018). microRNAs, have been widely studied and can be used in the diagnosis of Alzheimer’s Disease, Parkinson’s Disease, multiple sclerosis, Huntington’s Disease, epilepsy, and other diseases (Junker et al., 2011; Henshall et al., 2016; Miniarikova et al., 2018; Sadlon et al., 2019; Doxakis, 2020; Singh and Yadav, 2020; Bazrgar et al., 2021). Therefore, we infer that microRNAs have the potential to be SAE biomarkers (Table 2).
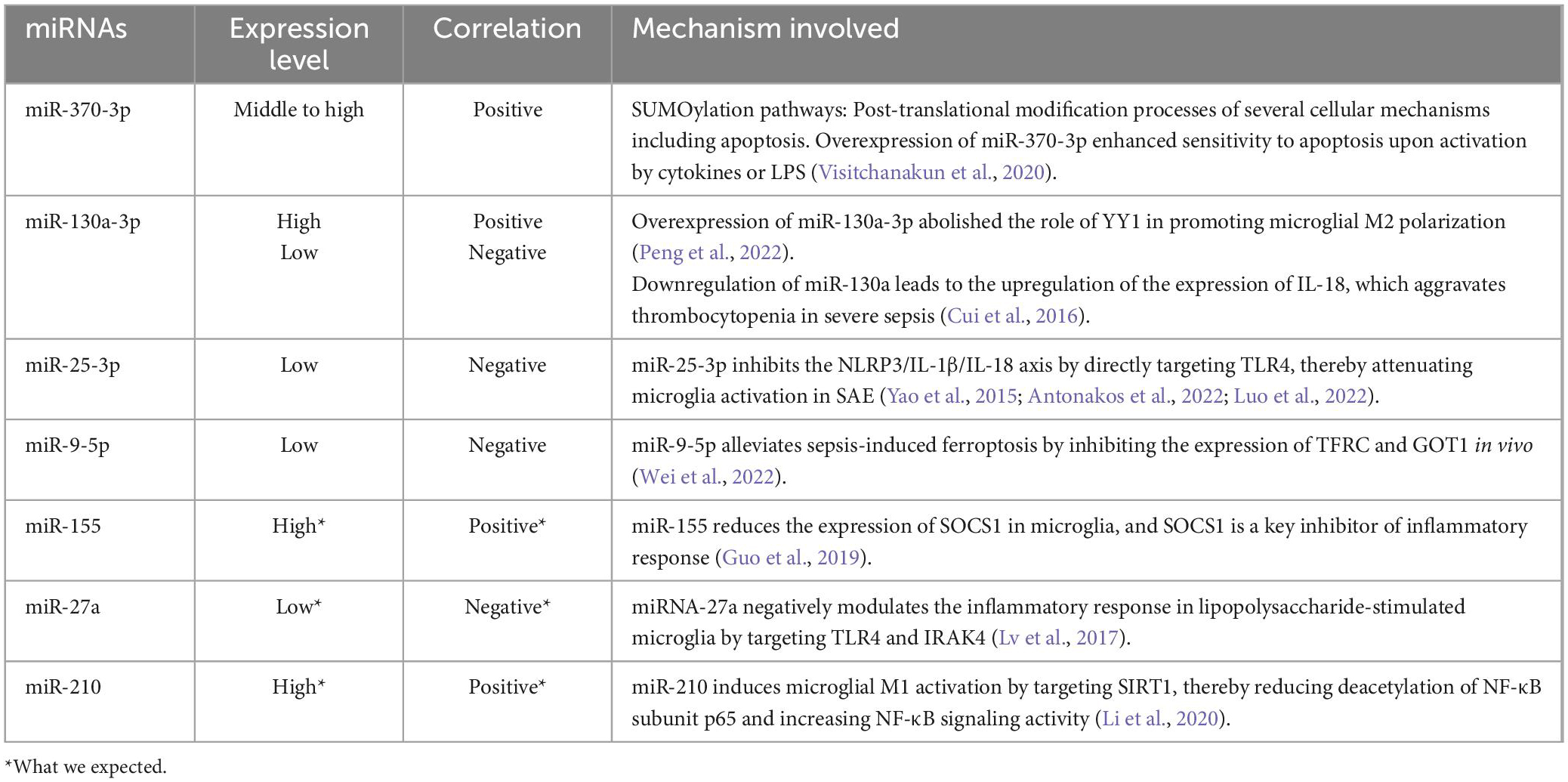
Table 2. Systematic table of all miRNAs and their expression levels, their correlations with SAE progression, and the mechanisms underlying their action.
Research shows that miR-370-3p levels increase in the brain and plasma in SAE, but not in uremic encephalopathy. Overexpression of miR-370-3p enhances sensitivity to apoptosis upon activation by cytokines or LPS. TNF-α induces apoptosis of PC-12 neurons by activating the expression of miR-370-3p in PC-12 neurons (Visitchanakun et al., 2020). miR-130a-3p is upregulated in SAE. YY1 can promote microglial M2 polarization by inhibiting miR-130a-3p promoter activity, thereby improving SAE (Peng et al., 2022). miR-25-3p is expressed at low levels in the cerebral cortex of SAE mice, while microglia are overactivated. In vitro experiments demonstrated that increasing miR-25-3p expression can attenuate microglial activation in SAE by directly targeting TLR4 (toll-like receptor 4) to inhibit the NLRP3/IL-1β/IL-18 axis, suggesting that miR-25-3p plays a protective role against SAE (Luo et al., 2022). Sepsis induced high expression of serous exosome-derived NEAT1, which might exacerbate SAE by regulating the miR-9-5p/TFRC and GOT1 axis to promote siderosis (Wei et al., 2022). Although there are few microRNAs studied in SAE, it is expected that some microRNAs in sepsis may be related to SAE, especially some microRNAs related to inflammation.
In severe sepsis with thrombocytopenia, miR-130a is downregulated. The mechanism is that the downregulation of miR-130a leads to upregulation of the expression of IL-18t, which aggravates thrombocytopenia in severe sepsis (Cui et al., 2016). Notably, this is contrary to what Peng et al. (2022) observed in SAE, arguably both SAE and severe sepsis with thrombocytopenia are a more severe subtype of sepsis, and the expression of miR-130a should be consistent, which deserves further study by future researchers. In the study by Yao et al. (2015) miR-25 was the most significantly altered RNA in sepsis, and the decrease of miR-25 levels correlated with the severity of sepsis, SOFA score, CRP, and PCT levels, which is similar to the results observed by Luo et al. (2022), Antonakos et al. (2022). Dysregulation of circulating miR-25 has also been described in other inflammatory settings such as periodontitis and vascular endothelial cell inflammation, further highlighting the potential of miR-25 as a biomarker.
Microglia are generally considered as macrophages in the central nervous system. After activation, microglia can cause neuronal damage and even apoptosis by releasing inflammatory mediators, reactive oxygen species, neurotransmitters, and other substances. Therefore, some microRNAs that play a central role in microglia are also expected to affect the progression of SAE. miR-155, miR-27a, and miR-210 are widely recognized as biomarkers in sepsis, and recent studies have shown that they also play a central role in microglial function (Huang et al., 2014; Szilágyi et al., 2019; Osca-Verdegal et al., 2021).
miR-155 mediates LPS-induced neuroinflammation by modulating microglia, and inhibition of miR-155 contributes to the establishment of endotoxin tolerance. The mechanism is that inhibition of miR-155 enhances the expression of SOCS1 in microglia, and SOCS1 is a key inhibitor of inflammatory response (Guo et al., 2019). Therefore, it is expected that high levels of miR-155 will be found in SAE.
miR-27a levels rapidly decrease in microglia after LPS stimulation, and overexpression of miR-27a significantly reduces the production of inflammatory cytokines, such as IL-6, IL-1β, TNF-α, and NO. miR-27a directly inhibits the expression of TLR4 and IRAK4—key adapter kinases in the TLR4/MyD88 signaling pathway (Lv et al., 2017).
miR-210 inhibitor can effectively suppress microglia-mediated neuroinflammation and significantly reduce HIE-induced brain damage. miR-210 induces microglial M1 activation by targeting SIRT1, thereby reducing deacetylation of NF-κB subunit p65 and increasing NF-κB signaling activity (Li et al., 2020). Low levels of miR-210 exhibit neuroprotective effects in mice with hypoxic-ischemic encephalopathy (Ma et al., 2016; Li et al., 2020); its effect in SAE, however, is yet to be studies.
Although, in theory, microRNAs are excellent biomarkers, they have their disadvantages. A prominent issue is how microRNAs recognize partially complementary sequences. Smaller microRNAs provide a limited amount of sequence information for specificity. Furthermore, since partial pairing between microRNAs and target sites is usually sufficient, the range of genes that can be regulated is relatively broad. This implies that a single miRNA can regulate multiple mRNAs, and predicting these targets is not easy (Bartel, 2009). However, given the challenges of matching microRNAs to specific target sequences, several methods have been developed to identify miRNA targets, ranging from small-scale genetic studies to biochemical approaches such as algorithmic prediction and high-throughput sequencing to isolate target mRNAs or sequences (Thomson et al., 2011).
10. Conclusion
In conclusion, sepsis-related encephalopathy is a complex central nervous system injury causing long-term sequelae in patients, resulting in a poor prognosis and lower quality of life. SAE is still diagnosed by exclusion. Some biomarkers have been used to assess brain dysfunction caused by sepsis, but the evidence is insufficient, resulting in invalid clinical measurements.
As chief gene regulators, microRNAs are closely related to disease progression. Because of their stable structure, presence in various body fluids, and ability to pass through the BBB, they are theoretically excellent biomarkers for diagnosis and prognosis of central nervous system diseases. However, they have some disadvantages. Due to the wide range of downstream targets of microRNAs, a single miRNA can interfere with the immune response in multiple ways and simultaneously manifest the multi-organ damage of sepsis; therefore, some differential microRNAs may not be attributed to SAE. If they are used for diagnosis, they will lead to misdiagnosis, and any treatment guided by them will bring immeasurable harm to patients. The complexity of miRNA interactions underscores the challenge of having to take a holistic view of the impact of miRNAs on the immune response. Thus, we must be rigorous and cautious when identifying biomarkers, especially with potential molecules with a wide range of targets. Additionally, the combined use of multiple miRNAs and/or other biomarkers associated with sepsis may be an effective method for diagnosing SAE because the miRNA expression profile is not SAE-specific. Undoubtedly, the postulation of microRNAs as biomarkers of SAE requires more research in the future, but it will be worthwhile.
Author contributions
ZY, XS, and HW contributed to the conception of the review and revised the manuscript. ZY was in charge of collating the literature and wrote the first draft of the manuscript. ZY, XS, ZZ, and GF wrote the sections of the manuscript. All authors contributed to the manuscript and approved the submitted version.
Funding
This work was supported by the Zhejiang Provincial Medical and Health Technology Project (Grant No. 2022KY252) and the Construction Fund of Medical Key Disciplines of Hangzhou (OO20200485).
Acknowledgments
We thank all members of the Department of Critical Care Medicine of Hangzhou First People’s Hospital for their helpful suggestions about the manuscript. We also thank Editage (www.editage.com) for English language editing.
Conflict of interest
The authors declare that the research was conducted in the absence of any commercial or financial relationships that could be construed as a potential conflict of interest.
Publisher’s note
All claims expressed in this article are solely those of the authors and do not necessarily represent those of their affiliated organizations, or those of the publisher, the editors and the reviewers. Any product that may be evaluated in this article, or claim that may be made by its manufacturer, is not guaranteed or endorsed by the publisher.
References
Akeju, O., Pavone, K., Westover, M., Vazquez, R., Prerau, M., Harrell, P., et al. (2014). A comparison of propofol- and dexmedetomidine-induced electroencephalogram dynamics using spectral and coherence analysis. Anesthesiology 121, 978–989. doi: 10.1097/ALN.0000000000000419
Antonakos, N., Gilbert, C., Théroude, C., Schrijver, I., and Roger, T. (2022). Modes of action and diagnostic value of miRNAs in sepsis. Front. Immunol. 13:951798. doi: 10.3389/fimmu.2022.951798
Bartel, D. (2009). MicroRNAs: Target recognition and regulatory functions. Cell 136, 215–233. doi: 10.1016/j.cell.2009.01.002
Bazrgar, M., Khodabakhsh, P., Prudencio, M., Mohagheghi, F., and Ahmadiani, A. (2021). The role of microRNA-34 family in Alzheimer’s disease: A potential molecular link between neurodegeneration and metabolic disorders. Pharmacol. Res. 172:105805. doi: 10.1016/j.phrs.2021.105805
Beltrán-García, J., Osca-Verdegal, R., Romá-Mateo, C., Carbonell, N., Ferreres, J., Rodríguez, M., et al. (2020). Epigenetic biomarkers for human sepsis and septic shock: Insights from immunosuppression. Epigenomics 12, 617–646. doi: 10.2217/epi-2019-0329
Bleck, T., Smith, M., Pierre-Louis, S., Jares, J., Murray, J., and Hansen, C. (1993). Neurologic complications of critical medical illnesses. Crit. Care Med. 21, 98–103. doi: 10.1097/00003246-199301000-00019
Chen, T., Chung, Y., Chen, P., Hu, S., Chang, C., Hsieh, S., et al. (2022). Effects of daily sedation interruption in intensive care unit patients undergoing mechanical ventilation: A meta-analysis of randomized controlled trials. Int. J. Nurs. Pract. 28:e12948. doi: 10.1111/ijn.12948
Ciesla, M., Skrzypek, K., Kozakowska, M., Loboda, A., Jozkowicz, A., and Dulak, J. (2011). MicroRNAs as biomarkers of disease onset. Anal. Bioanal. Chem. 401, 2051–2061. doi: 10.1007/s00216-011-5001-8
Cui, Y., Wang, B., Gao, H., Xing, Y., Li, J., Li, H., et al. (2016). Interleukin-18 and miR-130a in severe sepsis patients with thrombocytopenia. Patient Prefer. Adherence 10, 313–319. doi: 10.2147/PPA.S95588
Dalkara, T., and Alarcon-Martinez, L. (2015). Cerebral microvascular pericytes and neurogliovascular signaling in health and disease. Brain Res. 1623, 3–17. doi: 10.1016/j.brainres.2015.03.047
Devlin, J., Fraser, G., Kanji, S., and Riker, R. (2001). Sedation assessment in critically ill adults. Ann. Pharmacother. 35, 1624–1632. doi: 10.1345/aph.1A117
Doxakis, E. (2020). Cell-free microRNAs in Parkinson’s disease: Potential biomarkers that provide new insights into disease pathogenesis. Ageing Res. Rev. 58:101023. doi: 10.1016/j.arr.2020.101023
Ebersoldt, M., Sharshar, T., and Annane, D. (2007). Sepsis-associated delirium. Intensive Care Med. 33, 941–950. doi: 10.1007/s00134-007-0622-2
Ehler, J., Barrett, L., Taylor, V., Groves, M., Scaravilli, F., Wittstock, M., et al. (2017). Translational evidence for two distinct patterns of neuroaxonal injury in sepsis: A longitudinal, prospective translational study. Crit. Care 21:262. doi: 10.1186/s13054-017-1850-7
Ehler, J., Petzold, A., Wittstock, M., Kolbaske, S., Gloger, M., Henschel, J., et al. (2019a). The prognostic value of neurofilament levels in patients with sepsis-associated encephalopathy – A prospective, pilot observational study. PLoS One 14:e0211184. doi: 10.1371/journal.pone.0211184
Ehler, J., Saller, T., Wittstock, M., Rommer, P., Chappell, D., Zwissler, B., et al. (2019b). Diagnostic value of NT-proCNP compared to NSE and S100B in cerebrospinal fluid and plasma of patients with sepsis-associated encephalopathy. Neurosci. Lett. 692, 167–173. doi: 10.1016/j.neulet.2018.11.014
Eidelman, L., Putterman, D., Putterman, C., and Sprung, C. (1996). The spectrum of septic encephalopathy. Definitions, etiologies, and mortalities. JAMA 275, 470–473.
Ely, E., Inouye, S., Bernard, G., Gordon, S., Francis, J., May, L., et al. (2001a). Delirium in mechanically ventilated patients: Validity and reliability of the confusion assessment method for the intensive care unit (CAM-ICU). JAMA 286, 2703–2710. doi: 10.1001/jama.286.21.2703
Ely, E., Margolin, R., Francis, J., May, L., Truman, B., Dittus, R., et al. (2001b). Evaluation of delirium in critically ill patients: Validation of the confusion assessment method for the intensive care unit (CAM-ICU). Crit. Care Med. 29, 1370–1379. doi: 10.1097/00003246-200107000-00012
Ely, E., Shintani, A., Truman, B., Speroff, T., Gordon, S., Harrell, F., et al. (2004). Delirium as a predictor of mortality in mechanically ventilated patients in the intensive care unit. JAMA 291, 1753–1762. doi: 10.1001/jama.291.14.1753
Ely, E., Truman, B., Shintani, A., Thomason, J., Wheeler, A., Gordon, S., et al. (2003). Monitoring sedation status over time in ICU patients: Reliability and validity of the Richmond Agitation-Sedation Scale (RASS). JAMA 289, 2983–2991. doi: 10.1001/jama.289.22.2983
Enuka, Y., Lauriola, M., Feldman, M., Sas-Chen, A., Ulitsky, I., and Yarden, Y. (2016). Circular RNAs are long-lived and display only minimal early alterations in response to a growth factor. Nucleic Acids Res. 44, 1370–1383. doi: 10.1093/nar/gkv1367
Feng, Q., Ai, Y., Gong, H., Wu, L., Ai, M., Deng, S., et al. (2019). Characterization of sepsis and sepsis-associated encephalopathy. J. Intensive Care Med. 34, 938–945. doi: 10.1177/0885066617719750
Fleischmann, C., Scherag, A., Adhikari, N., Hartog, C., Tsaganos, T., Schlattmann, P., et al. (2016). Assessment of global incidence and mortality of hospital-treated sepsis. Current estimates and limitations. Am. J. Respir. Crit. Care Med. 193, 259–272. doi: 10.1164/rccm.201504-0781OC
Fleischmann-Struzek, C., Mellhammar, L., Rose, N., Cassini, A., Rudd, K., Schlattmann, P., et al. (2020). Incidence and mortality of hospital- and ICU-treated sepsis: Results from an updated and expanded systematic review and meta-analysis. Intensive Care Med. 46, 1552–1562. doi: 10.1007/s00134-020-06151-x
Gofton, T., and Young, G. (2012). Sepsis-associated encephalopathy. Nat. Rev. Neurol. 8, 557–566. doi: 10.1038/nrneurol.2012.183
Guo, Y., Hong, W., Wang, X., Zhang, P., Körner, H., Tu, J., et al. (2019). MicroRNAs in microglia: How do micrornas affect activation, inflammation, polarization of microglia and mediate the interaction between microglia and glioma? Front. Mol. Neurosci. 12:125. doi: 10.3389/fnmol.2019.00125
Henshall, D., Hamer, H., Pasterkamp, R., Goldstein, D., Kjems, J., Prehn, J., et al. (2016). MicroRNAs in epilepsy: Pathophysiology and clinical utility. Lancet Neurol. 15, 1368–1376. doi: 10.1016/S1474-4422(16)30246-0
Hosokawa, K., Gaspard, N., Su, F., Oddo, M., Vincent, J., and Taccone, F. (2014). Clinical neurophysiological assessment of sepsis-associated brain dysfunction: A systematic review. Crit. Care 18:674. doi: 10.1186/s13054-014-0674-y
Huang, J., Sun, Z., Yan, W., Zhu, Y., Lin, Y., Chen, J., et al. (2014). Identification of microRNA as sepsis biomarker based on miRNAs regulatory network analysis. Biomed Res. Int. 2014:594350. doi: 10.1155/2014/594350
Iacobone, E., Bailly-Salin, J., Polito, A., Friedman, D., Stevens, R., and Sharshar, T. (2009). Sepsis-associated encephalopathy and its differential diagnosis. Crit. Care Med. 37(Suppl. 10) S331–S336. doi: 10.1097/CCM.0b013e3181b6ed58
Inouye, S., van Dyck, C., Alessi, C., Balkin, S., Siegal, A., and Horwitz, R. (1990). Clarifying confusion: The confusion assessment method. A new method for detection of delirium. Ann. Intern. Med. 113, 941–948. doi: 10.7326/0003-4819-113-12-941
Jonas, S., and Izaurralde, E. (2015). Towards a molecular understanding of microRNA-mediated gene silencing. Nat. Rev. Genet. 16, 421–433. doi: 10.1038/nrg3965
Junior, M., Kumar, A., Kumar, P., and Gupta, P. (2022). Assessment of delirium as an independent predictor of outcome among critically ill patients in intensive care unit: A prospective study. Indian J. Crit. Care Med. 26, 676–681. doi: 10.5005/jp-journals-10071-23907
Junker, A., Hohlfeld, R., and Meinl, E. (2011). The emerging role of microRNAs in multiple sclerosis. Nat. Rev. Neurol. 7, 56–59. doi: 10.1038/nrneurol.2010.179
Juźwik, C., Drake, S., Zhang, Y., Paradis-Isler, N., Sylvester, A., Amar-Zifkin, A., et al. (2019). microRNA dysregulation in neurodegenerative diseases: A systematic review. Prog. Neurobiol. 182:101664. doi: 10.1016/j.pneurobio.2019.101664
Khan, B., Perkins, A., Prasad, N., Shekhar, A., Campbell, N., Gao, S., et al. (2020). Biomarkers of delirium duration and delirium severity in the ICU. Crit. Care Med. 48, 353–361. doi: 10.1097/CCM.0000000000004139
Khan, B., Zawahiri, M., Campbell, N., Fox, G., Weinstein, E., Nazir, A., et al. (2012). Delirium in hospitalized patients: Implications of current evidence on clinical practice and future avenues for research–A systematic evidence review. J. Hosp. Med. 7, 580–589. doi: 10.1002/jhm.1949
Lahariya, S., Grover, S., Bagga, S., and Sharma, A. (2014). Delirium in patients admitted to a cardiac intensive care unit with cardiac emergencies in a developing country: Incidence, prevalence, risk factor and outcome. Gen. Hosp. Psychiatry 36, 156–164. doi: 10.1016/j.genhosppsych.2013.10.010
Lehmann, S., Krüger, C., Park, B., Derkow, K., Rosenberger, K., Baumgart, J., et al. (2012). An unconventional role for miRNA: Let-7 activates Toll-like receptor 7 and causes neurodegeneration. Nat. Neurosci. 15, 827–835. doi: 10.1038/nn.3113
Li, B., Dasgupta, C., Huang, L., Meng, X., and Zhang, L. (2020). MiRNA-210 induces microglial activation and regulates microglia-mediated neuroinflammation in neonatal hypoxic-ischemic encephalopathy. Cell Mol. Immunol. 17, 976–991. doi: 10.1038/s41423-019-0257-6
Luo, X., Ying, J., and Wang, Q. (2022). miR-25-3p ameliorates SAE by targeting the TLR4/NLRP3 axis. Metab. Brain Dis. 37, 1803–1813. doi: 10.1007/s11011-022-01017-1
Lv, Y., Ou-Yang, A., and Fu, L. (2017). MicroRNA-27a negatively modulates the inflammatory response in lipopolysaccharide-stimulated microglia by targeting TLR4 and IRAK4. Cell Mol. Neurobiol. 37, 195–210. doi: 10.1007/s10571-016-0361-4
Ma, Q., Dasgupta, C., Li, Y., Bajwa, N., Xiong, F., Harding, B., et al. (2016). Inhibition of microRNA-210 provides neuroprotection in hypoxic-ischemic brain injury in neonatal rats. Neurobiol. Dis. 89, 202–212. doi: 10.1016/j.nbd.2016.02.011
Mazeraud, A., Righy, C., Bouchereau, E., Benghanem, S., Bozza, F., and Sharshar, T. (2020). Septic-associated encephalopathy: A comprehensive review. Neurotherapeutics 17, 392–403. doi: 10.1007/s13311-020-00862-1
Miniarikova, J., Evers, M., and Konstantinova, P. (2018). Translation of MicroRNA-based huntingtin-lowering therapies from preclinical studies to the clinic. Mol. Ther. 26, 947–962. doi: 10.1016/j.ymthe.2018.02.002
Mitchell, P., Parkin, R., Kroh, E., Fritz, B., Wyman, S., Pogosova-Agadjanyan, E., et al. (2008). Circulating microRNAs as stable blood-based markers for cancer detection. Proc. Natl. Acad. Sci. U.S.A. 105, 10513–10518. doi: 10.1073/pnas.0804549105
Molnár, L., Fülesdi, B., Németh, N., and Molnár, C. (2018). Sepsis-associated encephalopathy: A review of literature. Neurol. India 66, 352–361. doi: 10.4103/0028-3886.227299
Nowakowski, T., Rani, N., Golkaram, M., Zhou, H., Alvarado, B., Huch, K., et al. (2018). Regulation of cell-type-specific transcriptomes by microRNA networks during human brain development. Nat. Neurosci. 21, 1784–1792. doi: 10.1038/s41593-018-0265-3
O’Brien, J., Hayder, H., Zayed, Y., and Peng, C. (2018). Overview of MicroRNA biogenesis, mechanisms of actions, and circulation. Front. Endocrinol. (Lausanne) 9:402. doi: 10.3389/fendo.2018.00402
Osca-Verdegal, R., Beltrán-García, J., Pallardó, F., and García-Giménez, J. (2021). Role of microRNAs As biomarkers in sepsis-associated encephalopathy. Mol. Neurobiol. 58, 4682–4693. doi: 10.1007/s12035-021-02445-3
Pasquinelli, A. (2012). MicroRNAs and their targets: Recognition, regulation and an emerging reciprocal relationship. Nat. Rev. Genet. 13, 271–282. doi: 10.1038/nrg3162
Peng, L., Xu, Y., and Wang, Q. (2022). YY1 promotes microglia M2 polarization through the Mir-130a-3p/Trem-2 axis to alleviate sepsis-associated encephalopathy. Shock 58, 128–136. doi: 10.1097/SHK.0000000000001914
Piazza, O., Cotena, S., De Robertis, E., Caranci, F., and Tufano, R. (2009). Sepsis associated encephalopathy studied by MRI and cerebral spinal fluid S100B measurement. Neurochem. Res. 34, 1289–1292. doi: 10.1007/s11064-008-9907-2
Pierrakos, C., Attou, R., Decorte, L., Kolyviras, A., Malinverni, S., Gottignies, P., et al. (2014). Transcranial Doppler to assess sepsis-associated encephalopathy in critically ill patients. BMC Anesthesiol. 14:45. doi: 10.1186/1471-2253-14-45
Polito, A., Eischwald, F., Maho, A., Polito, A., Azabou, E., Annane, D., et al. (2013). Pattern of brain injury in the acute setting of human septic shock. Crit. Care 17:R204. doi: 10.1186/cc12899
Purdon, P., Pierce, E., Mukamel, E., Prerau, M., Walsh, J., Wong, K., et al. (2013). Electroencephalogram signatures of loss and recovery of consciousness from propofol. Proc. Natl. Acad. Sci. U.S.A. 110, E1142–E1151. doi: 10.1073/pnas.1221180110
Reinhart, K., Daniels, R., Kissoon, N., Machado, F., Schachter, R., and Finfer, S. (2017). Recognizing sepsis as a global health priority – A WHO resolution. N. Engl. J. Med. 377, 414–417. doi: 10.1056/NEJMp1707170
Rhee, C., Dantes, R., Epstein, L., Murphy, D., Seymour, C., Iwashyna, T., et al. (2017). Incidence and trends of sepsis in US hospitals using clinical vs claims data, 2009-2014. JAMA 318, 1241–1249. doi: 10.1001/jama.2017.13836
Rosengarten, B., Krekel, D., Kuhnert, S., and Schulz, R. (2012). Early neurovascular uncoupling in the brain during community acquired pneumonia. Crit. Care 16:R64. doi: 10.1186/cc11310
Rudd, K., Johnson, S., Agesa, K., Shackelford, K., Tsoi, D., Kievlan, D., et al. (2020). Global, regional, and national sepsis incidence and mortality, 1990-2017: Analysis for the Global Burden of Disease Study. Lancet 395, 200–211. doi: 10.1016/S0140-6736(19)32989-7
Sadlon, A., Takousis, P., Alexopoulos, P., Evangelou, E., Prokopenko, I., and Perneczky, R. (2019). miRNAs identify shared pathways in Alzheimer’s and Parkinson’s diseases. Trends Mol Med. 25, 662–672. doi: 10.1016/j.molmed.2019.05.006
Salta, E., Sierksma, A., Vanden Eynden, E., and De Strooper, B. (2016). miR-132 loss de-represses ITPKB and aggravates amyloid and TAU pathology in Alzheimer’s brain. EMBO Mol. Med. 8, 1005–1018. doi: 10.15252/emmm.201606520
Schramm, P., Klein, K., Falkenberg, L., Berres, M., Closhen, D., Werhahn, K., et al. (2012). Impaired cerebrovascular autoregulation in patients with severe sepsis and sepsis-associated delirium. Crit. Care 16:R181. doi: 10.1186/cc11665
Sharshar, T., Carlier, R., Bernard, F., Guidoux, C., Brouland, J., Nardi, O., et al. (2007). Brain lesions in septic shock: A magnetic resonance imaging study. Intensive Care Med. 33, 798–806. doi: 10.1007/s00134-007-0598-y
Sharshar, T., Citerio, G., Andrews, P., Chieregato, A., Latronico, N., Menon, D., et al. (2014). Neurological examination of critically ill patients: A pragmatic approach. Report of an ESICM expert panel. Intensive Care Med. 40, 484–495. doi: 10.1007/s00134-014-3214-y
Sharshar, T., Porcher, R., Siami, S., Rohaut, B., Bailly-Salin, J., Hopkinson, N., et al. (2011). Brainstem responses can predict death and delirium in sedated patients in intensive care unit. Crit. Care Med. 39, 1960–1967. doi: 10.1097/CCM.0b013e31821b843b
Singer, M., Deutschman, C., Seymour, C., Shankar-Hari, M., Annane, D., Bauer, M., et al. (2016). The third international consensus definitions for sepsis and septic shock (Sepsis-3). JAMA 315, 801–810. doi: 10.1001/jama.2016.0287
Singh, T., and Yadav, S. (2020). Role of microRNAs in neurodegeneration induced by environmental neurotoxicants and aging. Ageing Res. Rev. 60:101068. doi: 10.1016/j.arr.2020.101068
Sohel, M. (2016). Extracellular/circulating MicroRNAs: Release mechanisms, functions and challenges. Achiev. Life Sci. 10, 175–186. doi: 10.1016/j.als.2016.11.007
Sonneville, R., de Montmollin, E., Poujade, J., Garrouste-Orgeas, M., Souweine, B., Darmon, M., et al. (2017). Potentially modifiable factors contributing to sepsis-associated encephalopathy. Intensive Care Med. 43, 1075–1084. doi: 10.1007/s00134-017-4807-z
Sprung, C., Peduzzi, P., Shatney, C., Schein, R., Wilson, M., Sheagren, J., et al. (1990). Impact of encephalopathy on mortality in the sepsis syndrome. The veterans administration systemic sepsis cooperative study group. Crit. Care Med. 18, 801–806. doi: 10.1097/00003246-199008000-00001
Stevens, R., and Bhardwaj, A. (2006). Approach to the comatose patient. Crit. Care Med. 34, 31–41. doi: 10.1097/01.ccm.0000194534.42661.9f
Stoicea, N., Du, A., Lakis, D., Tipton, C., Arias-Morales, C., and Bergese, S. (2016). The MiRNA journey from theory to practice as a CNS biomarker. Front. Genet. 7:11. doi: 10.3389/fgene.2016.00011
Szilágyi, B., Fejes, Z., Pócsi, M., Kappelmayer, J., and Nagy, B. (2019). Role of sepsis modulated circulating microRNAs. EJIFCC 30, 128–145.
Thomson, D., Bracken, C., and Goodall, G. (2011). Experimental strategies for microRNA target identification. Nucleic Acids Res. 39, 6845–6853. doi: 10.1093/nar/gkr330
Toro, A., Escobar, L., Franco, J., Díaz-Gómez, J., Muñoz, J., Molina, F., et al. (2010). [Spanish version of the CAM-ICU (Confusion assessment method for the intensive care unit). Pilot study of validation]. Med. Intensiva 34, 14–21. doi: 10.1016/j.medin.2009.07.002
Valinezhad Orang, A., Safaralizadeh, R., and Kazemzadeh-Bavili, M. (2014). Mechanisms of miRNA-mediated gene regulation from common downregulation to mRNA-specific upregulation. Int. J. Genomics 2014:970607. doi: 10.1155/2014/970607
van Eijk, M., van den Boogaard, M., van Marum, R., Benner, P., Eikelenboom, P., Honing, M., et al. (2011). Routine use of the confusion assessment method for the intensive care unit: A multicenter study. Am. J. Respir. Crit. Care Med. 184, 340–344. doi: 10.1164/rccm.201101-0065OC
Van Rompaey, B., Elseviers, M., Schuurmans, M., Shortridge-Baggett, L., Truijen, S., and Bossaert, L. (2009). Risk factors for delirium in intensive care patients: A prospective cohort study. Crit. Care 13:R77. doi: 10.1186/cc7892
Visitchanakun, P., Tangtanatakul, P., Trithiphen, O., Soonthornchai, W., Wongphoom, J., Tachaboon, S., et al. (2020). Plasma miR-370-3P as a biomarker of sepsis-associated encephalopathy, the transcriptomic profiling analysis of microrna-arrays from mouse brains. Shock 54, 347–357. doi: 10.1097/SHK.0000000000001473
Vreeswijk, R., Honing, M., Bakker, K., Spronk, P., De Man, T., De Jonghe, J., et al. (2008). “Translation, retranslation and validation of the dutch confusion assessment method for the intensive care unit.”. Crit. Care 12:515. doi: 10.1186/cc6736
Wei, L., Fearing, M., Sternberg, E., and Inouye, S. (2008). The confusion assessment method: A systematic review of current usage. J. Am. Geriatr. Soc. 56, 823–830. doi: 10.1111/j.1532-5415.2008.01674.x
Wei, X., Jiang, W., Zeng, J., Huang, L., Ding, H., Jing, Y., et al. (2022). Correction to: Exosome-derived lncRNA NEAT1 exacerbates sepsis-associated encephalopathy by promoting ferroptosis through regulating miR-9-5p/TFRC and GOT1 axis. Mol. Neurobiol. 59:5252. doi: 10.1007/s12035-022-02931-2
Widmann, C., and Heneka, M. (2014). Long-term cerebral consequences of sepsis. Lancet Neurol. 13, 630–636. doi: 10.1016/S1474-4422(14)70017-1
Wilk, G., and Braun, R. (2018). Integrative analysis reveals disrupted pathways regulated by microRNAs in cancer. Nucleic Acids Res. 46, 1089–1101. doi: 10.1093/nar/gkx1250
Yao, B., Zhang, L., Ai, Y., Liu, Z., and Huang, L. (2014). Serum S100β is a better biomarker than neuron-specific enolase for sepsis-associated encephalopathy and determining its prognosis: A prospective and observational study. Neurochem. Res. 39, 1263–1269. doi: 10.1007/s11064-014-1308-0
Yao, L., Liu, Z., Zhu, J., Li, B., Chai, C., and Tian, Y. (2015). Clinical evaluation of circulating microRNA-25 level change in sepsis and its potential relationship with oxidative stress. Int. J. Clin. Exp. Pathol. 8, 7675–7684.
Young, G., Bolton, C., Archibald, Y., Austin, T., and Wells, G. (1992). The electroencephalogram in sepsis-associated encephalopathy. J. Clin. Neurophysiol. 9, 145–152. doi: 10.1097/00004691-199201000-00016
Zauner, C., Gendo, A., Kramer, L., Funk, G., Bauer, E., Schenk, P., et al. (2002). Impaired subcortical and cortical sensory evoked potential pathways in septic patients. Crit. Care Med. 30, 1136–1139. doi: 10.1097/00003246-200205000-00030
Keywords: sepsis-associated encephalopathy, nervous system disease, microRNAs, sepsis, biomarker
Citation: Yuechen Z, Shaosong X, Zhouxing Z, Fuli G and Wei H (2023) A summary of the current diagnostic methods for, and exploration of the value of microRNAs as biomarkers in, sepsis-associated encephalopathy. Front. Neurosci. 17:1125888. doi: 10.3389/fnins.2023.1125888
Received: 16 December 2022; Accepted: 02 March 2023;
Published: 16 March 2023.
Edited by:
Xiaoqing Jin, Zhongnan Hospital, Wuhan University, ChinaReviewed by:
Bertrand Kaeffer, Institut National de Recherche pour l’Agriculture, l’Alimentation et l’Environnement (INRAE), FrancePing Sun, School of Medicine, University of Pittsburgh, United States
Copyright © 2023 Yuechen, Shaosong, Zhouxing, Fuli and Wei. This is an open-access article distributed under the terms of the Creative Commons Attribution License (CC BY). The use, distribution or reproduction in other forums is permitted, provided the original author(s) and the copyright owner(s) are credited and that the original publication in this journal is cited, in accordance with accepted academic practice. No use, distribution or reproduction is permitted which does not comply with these terms.
*Correspondence: Xi Shaosong, c2ltb241MkBzaW5hLmNvbQ==; Hu Wei, cGFvbG9odTkyOUB6anUuZWR1LmNu