- The Second Affiliated Hospital of Harbin Medical University, Harbin, China
Background: Earlier researches have demonstrated that ischemic stroke, metabolic factors, and associated medications may influence the risk of epilepsy. Nevertheless, the causality between these elements and epilepsy remains inconclusive. This study aims to examine whether ischemic stroke, metabolic factors, and related medications affect the overall risk of epilepsy.
Methods: We used single nucleotide polymorphisms associated with ischemic stroke, hypothyroidism, hypertension, blood glucose levels, high cholesterol, serum 25-Hydroxyvitamin D levels, testosterone, HMG CoA reductase inhibitors, and beta-blocking agents as instrumental variables in a Mendelian randomization technique to investigate causality with epilepsy. Multiple sensitivity methods were performed to evaluate pleiotropy and heterogeneity.
Results: The IVW analysis revealed positive associations between ischemic stroke (OR = 1.29; p = 0.020), hypothyroidism (OR = 1.05; p = 0.048), high blood pressure (OR = 1.10; p = 0.028), high cholesterol (OR = 1.10; p = 0.024), HMG CoA reductase inhibitors (OR = 1.19; p = 0.003), beta-blocking agents (OR = 1.20; p = 0.006), and the risk of epilepsy. Conversely, blood glucose levels (OR = 0.79; p = 0.009), serum 25-Hydroxyvitamin D levels (OR = 0.75; p = 0.020), and testosterone (OR = 0.62; p = 0.019) exhibited negative associations with the risk of epilepsy. Sensitivity analyses confirmed the robustness of these findings (p > 0.05).
Conclusion: Our research suggests that ischemic stroke, hypothyroidism, high blood pressure, high cholesterol, HMG CoA reductase inhibitors, and beta-blockers may increase the risk of epilepsy, whereas serum 25-Hydroxyvitamin D levels and blood glucose levels may reduce the risk.
Introduction
Epilepsy is a common neurological condition caused by highly synchronized neuronal discharges (1). Its etiology is complex, encompassing structural, genetic, infectious, metabolic, immunological, and other unknown factors (2, 3). The disorder significantly impacts global physical health, particularly among infants and the elderly, leading to 13 million injuries and disabilities annually (4). Therefore, identifying the underlying causes is crucial for the effective management of epilepsy.
Numerous studies have shown that ischemic stroke (5), and metabolic factors such as hypothyroidism (6), hypertension (7, 8), blood glucose levels (9), high cholesterol (10), serum 25-Hydroxyvitamin D levels(25(OH)D) (11), and testosterone (12) are associated with the development of epilepsy. Additionally, certain medications, including HMG CoA reductase inhibitors(HMGCR) (13) and β-blockers (14) have been discovered to raise the risk of epilepsy. However, the causal relationship between these hazard elements and epilepsy remains unclear, as most previous studies were observational and yielded inconsistent findings.
Mendelian randomization (MR) is an epidemiological technique that uses genome-wide association study (GWAS) data to investigate the causality between different phenotypes and diseases (15). Consequently, in our research, we employed MR to assess the underlying association between these hazard elements and epilepsy. Additionally, reverse MR analyses were performed to determine the causality of epilepsy on the association between these risk factors.
Methods
MR analysis
The flowchart of the MR analyses in this research is described in Figure 1. This research rigorously adheres to the STROBE-MR guidelines, incorporating the following three key assumptions (16): (A) single nucleotide polymorphism (SNP) is associated with ischemic stroke, hypothyroidism, hypertension, blood glucose levels, high cholesterol, 25(OH)D, testosterone, HMGCR, and β-blockers; (B) SNP influences epilepsy solely through ischemic stroke, hypothyroidism, hypertension, blood glucose levels, high cholesterol, 25(OH)D, testosterone, HMGCR, and β-blockers; (C) SNP is not linked to confounding factors.
Data source
The data analyzed in the research were sourced from publicly available GWAS datasets, eliminating the need for additional moral permission. The GWAS pooled statistics encompassed various conditions, including epilepsy (n = 407,746), ischemic stroke (34,217 cases/ 406,111 controls), hypothyroidism (n = 405,357), high blood pressure (n = 407,746), blood glucose levels (n = 400,458), high cholesterol (n = 407,746), 25(OH)D (n = 417,580), high cholesterol (n = 407,746), HMGCR (73,475 cases/ 216,910 controls), beta blocking agents (n = 31,700 cases/ 192,324 controls) from the IEU Open GWAS project (17).1 The GWAS dataset for Testosterone is sourced from the UK Biobank (Table 1).
Selection of instrumental variables
We employed the following guidelines for selecting appropriate SNPs as IVs (18). Initially, a p-value significance threshold of 5 × 10–8 was set for SNPs. Subsequently, SNPs were pruned for linkage disequilibrium based on criteria of r2 < 0.001 and kb = 10,000. Next, the F-statistic was computed to evaluate the strength of the IVs, with SNPs having F-statistic values below 10 considered weak IVs and thus eliminated (Supplementary Table S1). Lastly, palindromic SNPs were excluded. Additionally, PhenoScanner was utilized to eliminate SNPs associated with confounding factors (19).
Statistical analysis
We principal employed the inverse variance weighting (IVW) approach to analyze the causality between exposure risk and epilepsy. Significance was determined when p < 0.05. Additionally, supplementary techniques comprising the simple mode, MR-Egger, weighted median, and weighted mode were utilized. Heterogeneity was evaluated using Cochrane’s Q-test, while multiple validity analyses were performed utilizing MR-Egger and MR-PRESSO, with significance set at p < 0.05 for indicating heterogeneity and horizontal pleiotropy. All statistical analyses were performed utilizing the ‘TwoSampleMR’ package in R 4.3.2.
Results
The IVW analysis indicated that ischemic stroke (OR = 1.29, 95% CI 1.04–1.60; p = 0.020), hypothyroidism (OR = 1.05, 95% CI 1.00–1.11; p = 0.048), high blood pressure (OR = 1.10, 95% CI 1.01–1.19; p = 0.028), high cholesterol (OR = 1.10, 95% CI 1.01–1.20; p = 0.024), HMGCR (OR = 1.19, 95% CI 1.06–1.33; p = 0.003), and beta-blocking agents (OR = 1.20, 95% CI 1.06–1.38; p = 0.006) are associated with an increased risk of epilepsy. Conversely, blood glucose levels (OR = 0.79, 95% CI 0.66–0.94; p = 0.009), 25(OH)D (OR = 0.75, 95% CI 0.59–0.95; p = 0.020), and testosterone (OR = 0.62, 95% CI 0.42–0.92; p = 0.019) exhibited negatively related to the risk of epilepsy (Figure 2). However, the IVW analysis indicated that no causal association between age (p = 0.737), sex (p = 0.231), and epilepsy. Sensitivity analyses confirmed the robustness of these results. The results of the MR sensitivity analysis are presented in Table 2. During the heterogeneity test, all p-values derived from Cochrane’s Q statistic were found to be greater than 0.05, indicating a lack of heterogeneity among the SNPs. Additionally, the MR-Egger regression intercept, used to assess horizontal pleiotropy, did not indicate the presence of pleiotropy. The MR-PRESSO results also confirmed the absence of significant horizontal pleiotropy outliers (p > 0.05). Leave-one-out analysis did not suggest that any individual SNPs had a significant impact on the overall results. Detailed results of the leave-one-out analysis and single forest plots are shown in Supplementary Figures S1–S2. As depicted in the scatter plot in Figure 3, no evidence of horizontal pleiotropy was detected in the MR-Egger regression (p > 0.05). The funnel plot in Figure 4 showed no apparent bias, further confirming the robustness of our findings. However, according to Supplementary Table S2, the p value for IVW was >0.05 or each method showed an inconsistent direction, thus reverse MR analysis showed no causal relationship between epilepsy and these several risk factors. Additionally, sensitivity analyses confirmed the robustness of our findings (Supplementary Table S3).
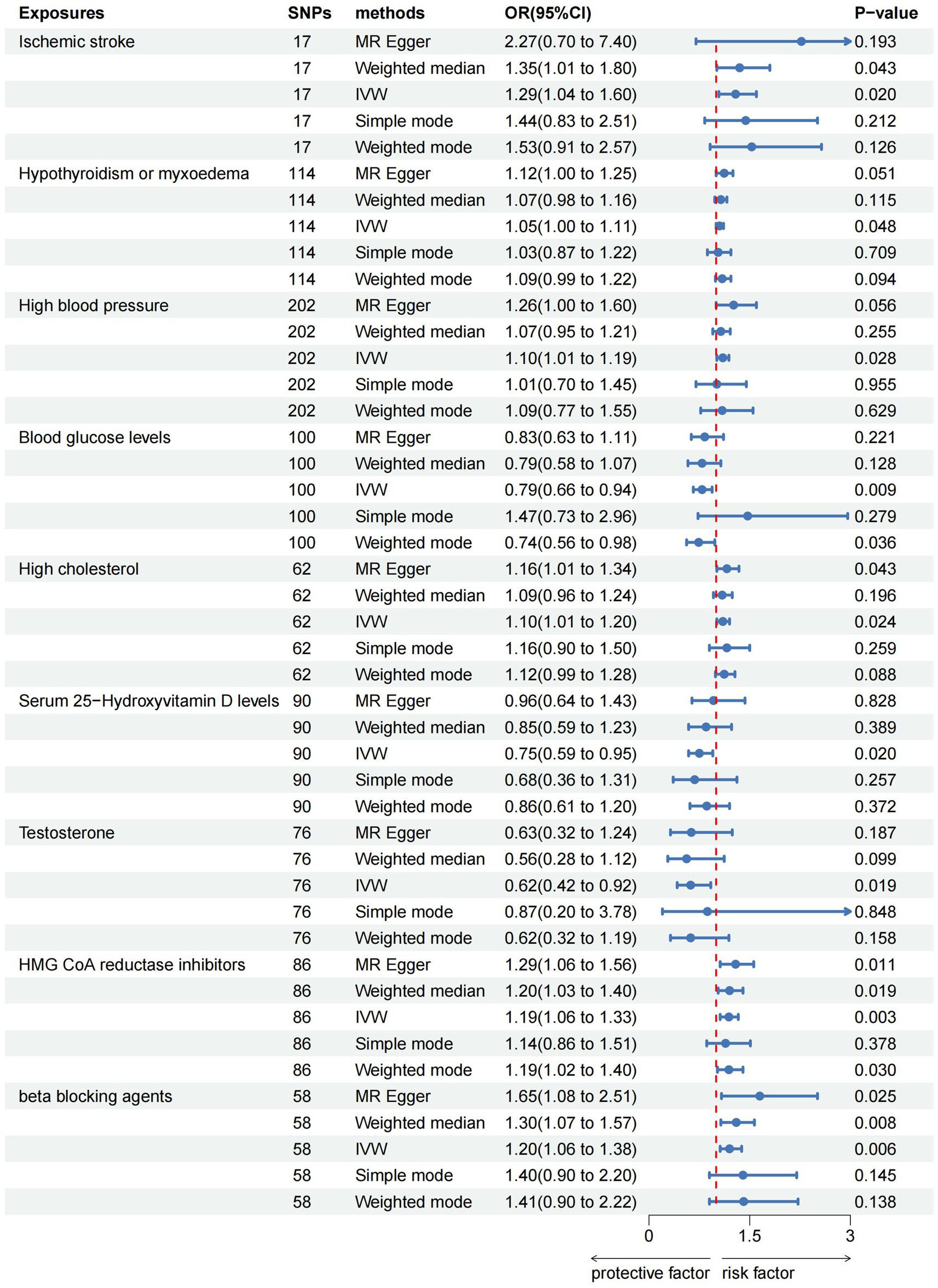
Figure 2. Forest plots of the causal relationship of ischemic stroke, metabolic factors, and related medications with epilepsy in the result of IVW in the MR analysis.
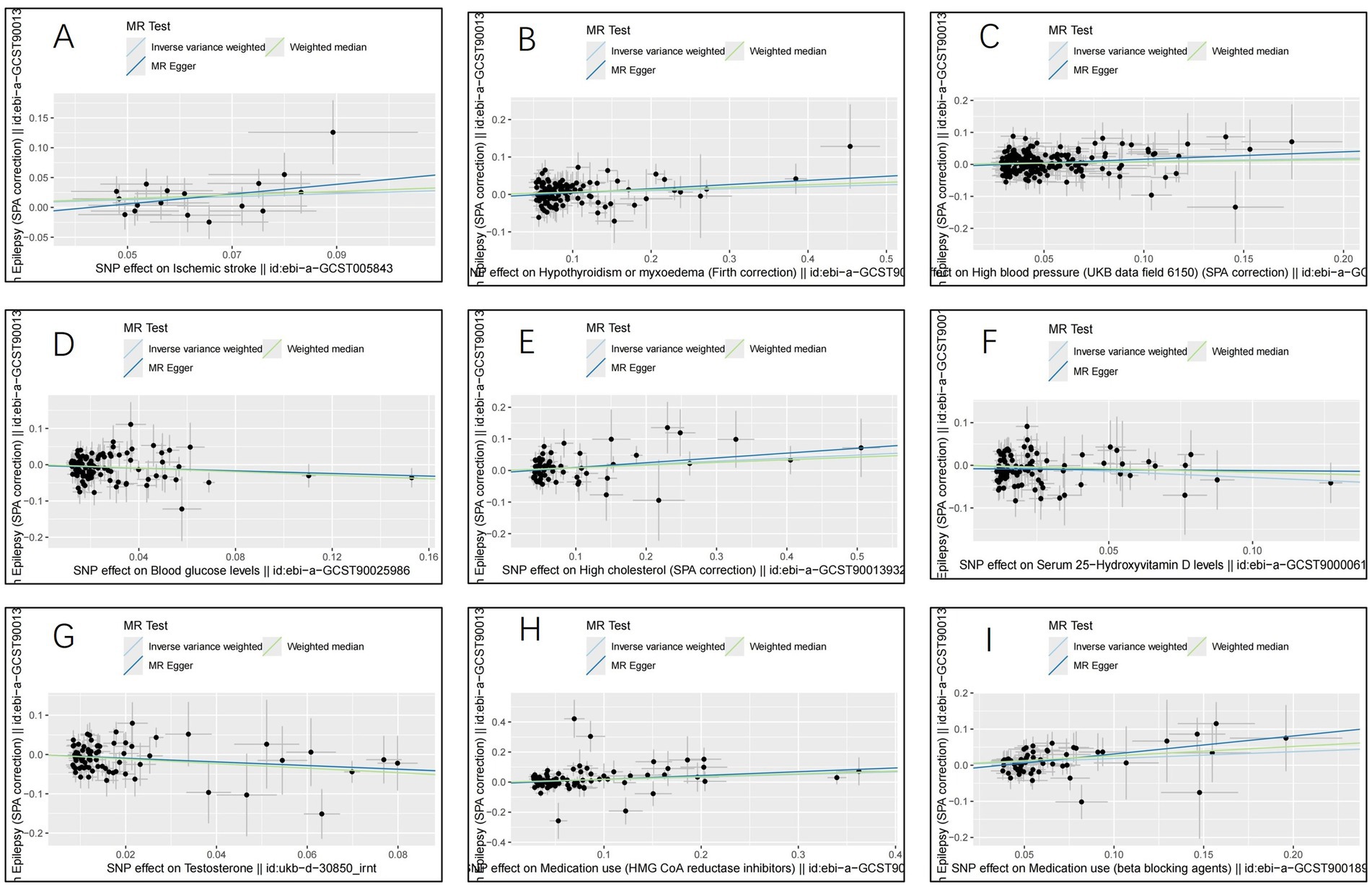
Figure 3. Scatter plots for the causal relationship of ischemic stroke, metabolic factors, and related medications with epilepsy. (A) ischemic stroke; (B) Hypothyroidism; (C) High blood pressure; (D) Blood glucose levels; (E) High cholesterol; (F) Serum 25 − Hydroxyvitamin D levels; (G) Testosterone; (H) HMG CoA reductase inhibitors; (I) beta blocking agents.
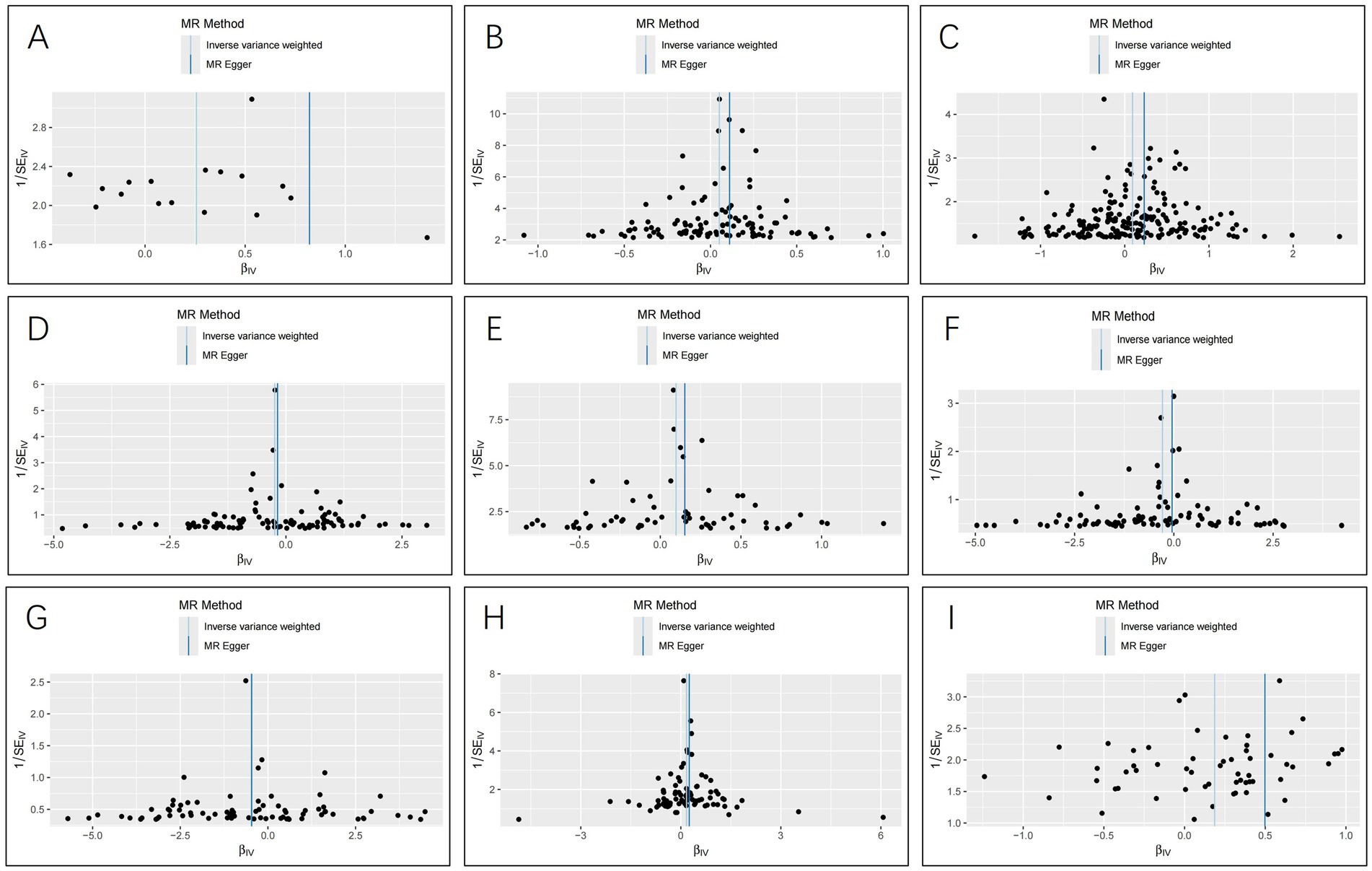
Figure 4. Funnel plots for the causal relationship of ischemic stroke, metabolic factors, and related medications with epilepsy. (A) ischemic stroke; (B) Hypothyroidism; (C) High blood pressure; (D) Blood glucose levels; (E) High cholesterol; (F) Serum 25 − Hydroxyvitamin D levels; (G) Testosterone; (H) HMG CoA reductase inhibitors; (I) beta blocking agents.
Discussion
Our MR study investigated the causal association between nine previously identified risk factors and epilepsy. The study revealed that ischemic stroke, hypothyroidism, high cholesterol, hypoglycemia, high blood pressure, HMGCR usage, and beta-blockers usage are related to an increased risk of epilepsy. Conversely, higher levels of 25(OH)D and testosterone were found to be associated with a decreased risk of epilepsy. Future attention should be given to screening for epileptogenesis risk in the management of patients with ischemic stroke, hypothyroidism, high cholesterol, hypoglycemia, hypertension, low levels of 25(OH)D and testosterone, and those on long-term HMGCR inhibitors and β-blockers. Regular screening and monitoring may be necessary to facilitate early detection and intervention, potentially improving the overall prognosis for these patients.
The heightened risk of epilepsy following ischemic stroke has been documented in numerous prior cohort studies (20, 21). Nevertheless, establishing a causality between ischemic stroke and epilepsy still challenging due to the susceptibility of observational studies to other confounding factors and reverse causation. Our MR study revealed that each standard unit increase in ischemic stroke elevated the risk of epileptogenesis by 29%, aligning with findings from previous research. The onset of seizures shortly after ischemic stroke may be attributed to localized ionic displacement and the release of elevated levels of excitotoxic neurotransmitters in ischemic injury sites. Conversely, epilepsy that emerges gradually during later stages may stem from potentially permanent lesions resulting from sustained neuronal excitability seizures (22–24).
Metabolic disorders have been identified as a significant factor in epileptogenesis (25). Thyroid hormones not only regulate energy metabolism but also play roles in neuronal survival, differentiation, and central nervous system energy expenditure. Several studies have demonstrated links between thyroid function and neurological disorders like dementia and depression (26). Moreover, thyroid hormones play an essential role in the pathophysiology of epilepsy (6). Our research indicates that hypothyroidism elevates epilepsy risk. Primarily, insufficient thyroid hormones due to hypothyroidism can slow neuronal metabolism and disrupt neurotransmitter synthesis and release (27, 28). Secondly, hypothyroidism can cause electrolyte imbalances like hyponatremia, which affect brain electrical activity and raise seizure risk (29–31). Prolonged hypothyroidism may also induce structural changes in specific brain regions, such as white matter damage, further increasing seizure susceptibility (32, 33).
A longitudinal study indicates that high blood pressure escalates the risk of late-onset epilepsy by 2 to 2.5 times, consistent with our MR analysis findings (7). Hypertension can trigger seizures through both direct and indirect pathways. The renin-angiotensin system may serve as a pivotal link between hypertension and epilepsy (34). Elevated blood pressure might contribute to cerebrovascular diseases such as cerebral infarction and hemorrhage, leading to brain damage, ischemic and hypoxic dysfunction, white matter lesions, and disruption of normal neuronal function, consequently heightening seizure susceptibility (35, 36). Furthermore, hypertension-induced systemic and localized inflammatory responses, along with oxidative stress products, can damage neurons and alter neuronal excitability, potentially inducing seizures (37, 38).
Prior studies consistently indicate that chronic glucose metabolism disorders frequently correlate with long-term epileptogenesis (39). Our research suggests that hypoglycemia increases the risk of epilepsy. The human brain heavily depends on glucose for energy, extracting sufficient amounts from the bloodstream to sustain normal functions. Persistent hypoglycemia triggers neuronal necrosis due to oxygen deprivation, subsequently leading to abnormal discharges capable of inducing seizures (40–43).
Disorders in brain cholesterol metabolism have been related to various neurological conditions like Parkinson’s disease, Alzheimer’s disease, and epilepsy (44–46). Our research indicates that each standard unit increase in cholesterol elevates epilepsy risk by 10 percent. Cholesterol might indirectly trigger epilepsy by impacting blood vessels, leading to cerebrovascular diseases and subsequently epilepsy. Excessive cholesterol accumulation, a crucial brain component, can not only increase membrane viscosity and decrease extrasynaptic neurotransmitter receptor mobility but also directly regulate numerous voltage-dependent and ligand-gated ion channels, thus heightening excitotoxicity and focal neuronal death (47). This process may contribute to neurological complications following prolonged epilepsy. Additionally, neurosteroids have been proposed to influence the clinical course of epileptic disorders by modulating neurotransmission (48, 49).
The relationship between vitamin D deficiency and epilepsy has been extensively researched (50). Researches have indicated that correcting vitamin D deficiency can lead to improvements in seizures (51). While previous MR analyses have not identified a causality between 25(OH)D and epilepsy, our MR analysis suggests that each standard unit increase in 25(OH)D reduces seizure risk by 25% (52). Vitamin D, a steroid hormone, plays an important role in regulating calcium homeostasis, neuroprotection, and brain function and development. The activated form of vitamin D, 25(OH)D, is primarily implicated in seizures. The predominant mechanism in current seizure research involves an unbalance between GABAergic inhibitory signaling and glutamatergic excitatory signaling at the synapse. Activation of voltage-gated calcium channels is vital for neuronal processes like neurotransmitter release, excitation, and synaptic transmission. Deficiency in 25(OH)D decreases the expression of these channels, leading to increased calcium ions in neurons and subsequent production of nitric oxide (NO)-dependent neuronal nitric oxide synthase, resulting in oxidative stress damage to the neuronal endoplasmic reticulum (53). Therefore, 25(OH)D may confer neuroprotection by reducing hyperexcitability in epileptic patients through improvements in calcium and magnesium levels and by attenuating oxidative damage to cells via NO inhibition (54–56).
The association between sex steroid hormones and epilepsy is a topic of significant interest and has undergone extensive study (12). Nevertheless, the causality between hormones and epilepsy still somewhat ambiguous. Our MR analysis revealed that each standard deviation decrease in testosterone levels decreased the risk of epilepsy by 38%, lending crucial theoretical support to previous studies exploring testosterone therapy for epilepsy (57). There are several mechanisms through which testosterone may exert its antiepileptic effects. Firstly, testosterone exhibits neuroprotective properties and can modulate neural activity by reducing glutamate release and enhancing GABA function (58). Secondly, testosterone has been shown to mitigate neuroinflammation, thereby reducing the frequency and severity of seizures (59). Additionally, testosterone can influence neuronal excitability by modulating ion channel function, enhancing neuronal membrane stability, and decreasing the likelihood of neuronal discharge (60). Reduction of oxidative stress may also facilitate to the antiepileptogenic effects of testosterone (61). Finally, testosterone may reduce seizure risk by affecting neurotransmitter balance in the brain, thereby preserving normal neuronal function (62).
Many drugs have the potential to induce epilepsy, yet this type of epilepsy is often overlooked by clinicians. The mechanisms behind drug-induced seizures are diverse and may include direct effects on the central nervous system, electrolyte imbalances, metabolic disturbances, and more (63). HMGCR, an important statin, has primarily been associated with anticonvulsant effects in previous studies. However, our research revealed that HMGCR may actually increase the risk of epilepsy, possibly due to its ability to induce hypokalemia, a known seizure trigger (64). Additionally, HMGCR might interact with other antiepileptic medications, affecting their efficacy (65). HMGCR inhibitors may influence the plasma concentration of certain antiepileptic drugs by competing for their metabolic pathways, potentially altering their efficacy or increasing the risk of adverse effects. Additionally, HMGCR inhibitors might indirectly affect neuronal membrane stability by altering cholesterol metabolism, which could influence the seizure threshold. Moreover, the anti-inflammatory effects of statins may interact synergistically or antagonistically with the actions of specific antiepileptic drugs. Moreover, HMGCR may cross the blood–brain barrier and directly influence the central nervous system, potentially leading to seizures (66, 67). Past reports have also documented cases of seizures induced by beta receptor antagonists (68). Hypoglycemic seizures are a commonly reported serious adverse effect of propranolol (69). Our MR analysis demonstrated that each standard unit increase in beta-blocking agent administration raised the risk of epileptogenesis by 20%. Consequently, we propose that beta-blocking agents can induce epilepsy by inducing hypoglycemia and interfering with the efficacy of other antiepileptic drugs. Beta-blockers may potentially impact the efficacy of antiepileptic drugs by reducing sympathetic nervous system activity and altering neurotransmitter balance in the brain. In certain cases, beta-blockers may indirectly influence the metabolism and clearance of antiepileptic drugs by affecting blood flow to the liver or kidneys. These changes could lead to lower plasma concentrations of the antiepileptic drugs, thereby reducing their effectiveness. Beta-blockers cause hypoglycemia probably through direct inhibition of hepatic production of glucose and release of glucagon. In addition, by attenuating the counter-regulatory effects of adrenaline, thereby promoting sympathetic-induced glycogenolysis and reducing gluconeogenesis. However, an analysis showed differences between short- and long-half-life beta blockers (particularly nadolol) and non-selective and selective beta blockers in terms of the risk of hypoglycemia occurring (70). Studies have shown that the risk of hypoglycemia appears to be higher with the use of non-selective beta blockers and long-acting beta blockers.
While our MR study offers a thorough examination of the causal relationship between various risk factors, including ischemic stroke, metabolic factors, and associated medications, with epilepsy, it is vital to admit some limitations. Firstly, the predominance of GWAS data from European ethnic populations calls for caution when generalizing our findings to other ethnic groups. Secondly, due to the aggregated nature of our data, we lacked access to detailed individual-level information for further stratified analyses. Additionally, although MR minimizes concerns regarding reverse causality and confounding factors, there may still exist some residual biases that could potentially impact the reliability of our results.
Conclusion
In summary, our study indicates that ischemic stroke, hypothyroidism, hypertension, high cholesterol, hypoglycemia, HMGCR inhibitors, and β-blockers may elevate the risk of epilepsy, whereas high levels of 25(OH)D may decrease the risk. These findings offer valuable insights for the tertiary prevention of epilepsy in clinical settings and suggest potential avenues for further research into the mechanisms underlying epilepsy.
Data availability statement
The original contributions presented in the study are included in the article/Supplementary material, further inquiries can be directed to the corresponding author.
Ethics statement
Ethical review and approval were not required for the study on human participants in accordance with the local legislation and institutional requirements. Written informed consent from the patients/participants or patients/participants' legal guardian/next of kin was not required to participate in this study in accordance with the national legislation and the institutional requirements.
Author contributions
WW: Conceptualization, Data curation, Formal analysis, Investigation, Methodology, Project administration, Resources, Software, Supervision, Validation, Visualization, Writing – original draft, Writing – review & editing. ML: Conceptualization, Data curation, Software, Visualization, Writing – review & editing. FL: Conceptualization, Formal analysis, Supervision, Writing – review & editing. ZW: Conceptualization, Data curation, Writing – review & editing. WY: Conceptualization, Writing – review & editing. XL: Conceptualization, Funding acquisition, Project administration, Resources, Writing – review & editing.
Funding
The author(s) declare that financial support was received for the research, authorship, and/or publication of this article. This work was supported in part by grants from Neurosurgery First-class Discipline Funding Project of the Second Affiliated Hospital of Harbin Medical University.
Acknowledgments
We thank the investigators and participants of the original GWAS. We are grateful for all GWAS sharing summary data used in this study.
Conflict of interest
The authors declare that the research was conducted in the absence of any commercial or financial relationships that could be construed as a potential conflict of interest.
Publisher’s note
All claims expressed in this article are solely those of the authors and do not necessarily represent those of their affiliated organizations, or those of the publisher, the editors and the reviewers. Any product that may be evaluated in this article, or claim that may be made by its manufacturer, is not guaranteed or endorsed by the publisher.
Supplementary material
The Supplementary material for this article can be found online at: https://www.frontiersin.org/articles/10.3389/fneur.2024.1464984/full#supplementary-material
Abbreviations
25(OH)D, serum 25-Hydroxyvitamin D levels; HMGCR, HMG CoA reductase inhibitors; MR, Mendelian randomization; GWAS, genome-wide association study; IVs, instrumental variables; IVW, inverse variance weighting.
Footnotes
References
1. Thijs, RD, Surges, R, O'Brien, TJ, and Sander, JW. Epilepsy in adults. Lancet. (2019) 393:689–701. doi: 10.1016/S0140-6736(18)32596-0
2. Chabolla, DR. Characteristics of the epilepsies. Mayo Clin Proc. (2002) 77:981–90. doi: 10.1016/S0025-6196(11)62268-4
3. Scheffer, IE, Berkovic, S, Capovilla, G, Connolly, MB, French, J, Guilhoto, L, et al. ILAE classification of the epilepsies: position paper of the ILAE Commission for Classification and Terminology. Epilepsia. (2017) 58:512–21. doi: 10.1111/epi.13709
4. Singh, G, and Sander, JW. The global burden of epilepsy report: implications for low- and middle-income countries. Epilepsy Behav. (2020) 105:106949. doi: 10.1016/j.yebeh.2020.106949
5. Sundelin, HEK, Tomson, T, Zelano, J, Söderling, J, Bang, P, and Ludvigsson, JF. Pediatric ischemic stroke and epilepsy: a Nationwide cohort study. Stroke. (2021) 52:3532–40. doi: 10.1161/STROKEAHA.121.034796
6. Tamijani, SM, Karimi, B, Amini, E, Golpich, M, Dargahi, L, Ali, RA, et al. Thyroid hormones: possible roles in epilepsy pathology. Seizure. (2015) 31:155–64. doi: 10.1016/j.seizure.2015.07.021
7. Stefanidou, M, Himali, JJ, Devinsky, O, Romero, JR, Ikram, MA, Beiser, AS, et al. Vascular risk factors as predictors of epilepsy in older age: the Framingham heart study. Epilepsia. (2022) 63:237–43. doi: 10.1111/epi.17108
8. Trompeter, RS, Smith, RL, Hoare, RD, Neville, BG, and Chantler, C. Neurological complications of arterial hypertension. Arch Dis Child. (1982) 57:913–7. doi: 10.1136/adc.57.12.913
9. Reid, CA, Kim, TH, Berkovic, SF, and Petrou, S. Low blood glucose precipitates spike-and-wave activity in genetically predisposed animals. Epilepsia. (2011) 52:115–20. doi: 10.1111/j.1528-1167.2010.02911.x
10. Wei, H, Liu, D, Geng, L, Liu, Y, Wang, H, and Yan, F. Application value of serum metabolic markers for cognitive prediction in elderly epilepsy. Neuropsychiatr Dis Treat. (2022) 18:2133–40. doi: 10.2147/NDT.S371751
11. Tombini, M, Palermo, A, Assenza, G, Pellegrino, G, Benvenga, A, Campana, C, et al. Calcium metabolism serum markers in adult patients with epilepsy and the effect of vitamin D supplementation on seizure control. Seizure. (2018) 58:75–81. doi: 10.1016/j.seizure.2018.04.008
12. Taubøll, E, Sveberg, L, and Svalheim, S. Interactions between hormones and epilepsy. Seizure. (2015) 28:3–11. doi: 10.1016/j.seizure.2015.02.012
13. Seker, FB, Kilic, U, Caglayan, B, Ethemoglu, MS, Caglayan, AB, Ekimci, N, et al. HMG-CoA reductase inhibitor rosuvastatin improves abnormal brain electrical activity via mechanisms involving eNOS. Neuroscience. (2015) 284:349–59. doi: 10.1016/j.neuroscience.2014.10.014
14. Borowicz, KK, and Banach, M. Antiarrhythmic drugs and epilepsy. Pharmacol Rep. (2014) 66:545–51. doi: 10.1016/j.pharep.2014.03.009
15. Emdin, CA, Khera, AV, and Kathiresan, S. Mendelian Randomization. JAMA. (2017) 318:1925–6. doi: 10.1001/jama.2017.17219
16. Skrivankova, VW, Richmond, RC, Woolf, BAR, Yarmolinsky, J, Davies, NM, Swanson, SA, et al. Strengthening the reporting of observational studies in epidemiology using Mendelian randomization: the STROBE-MR statement. JAMA. (2021) 326:1614–21. doi: 10.1001/jama.2021.18236
17. Mbatchou, J, Barnard, L, Backman, J, Marcketta, A, Kosmicki, JA, Ziyatdinov, A, et al. Computationally efficient whole-genome regression for quantitative and binary traits. Nat Genet. (2021) 53:1097–103. doi: 10.1038/s41588-021-00870-7
18. Sekula, P, Del Greco, MF, Pattaro, C, and Köttgen, A. Mendelian randomization as an approach to assess causality using observational data. J Am Soc Nephrol. (2016) 27:3253–65. doi: 10.1681/ASN.2016010098
19. Staley, JR, Blackshaw, J, Kamat, MA, Ellis, S, Surendran, P, Sun, BB, et al. PhenoScanner: a database of human genotype-phenotype associations. Bioinformatics. (2016) 32:3207–9. doi: 10.1093/bioinformatics/btw373
20. Sinka, L, Abraira, L, Imbach, LL, Zieglgänsberger, D, Santamarina, E, Álvarez-Sabín, J, et al. Association of Mortality and Risk of epilepsy with type of acute symptomatic seizure after ischemic stroke and an updated prognostic model. JAMA Neurol. (2023) 80:605–13. doi: 10.1001/jamaneurol.2023.0611
21. Ferreira-Atuesta, C, Döhler, N, Erdélyi-Canavese, B, Felbecker, A, Siebel, P, Scherrer, N, et al. Seizures after ischemic stroke: a matched multicenter study. Ann Neurol. (2021) 90:808–20. doi: 10.1002/ana.26212
22. Camilo, O, and Goldstein, LB. Seizures and epilepsy after ischemic stroke. Stroke. (2004) 35:1769–75. doi: 10.1161/01.STR.0000130989.17100.96
23. Gibson, LM, Hanby, MF, Al-Bachari, SM, Parkes, LM, Allan, SM, and Emsley, HC. Late-onset epilepsy and occult cerebrovascular disease. J Cereb Blood Flow Metab. (2014) 34:564–70. doi: 10.1038/jcbfm.2014.25
24. Ferro, JM, and Pinto, F. Poststroke epilepsy: epidemiology, pathophysiology and management. Drugs Aging. (2004) 21:639–53. doi: 10.2165/00002512-200421100-00003
25. Reddy, C, and Saini, AG. Metabolic Epilepsy. Indian J Pediatr. (2021) 88:1025–32. doi: 10.1007/s12098-020-03510-w
26. Sawicka-Gutaj, N, Zawalna, N, Gut, P, and Ruchała, M. Relationship between thyroid hormones and central nervous system metabolism in physiological and pathological conditions. Pharmacol Rep. (2022) 74:847–58. doi: 10.1007/s43440-022-00377-w
27. Richard, S, Ren, J, and Flamant, F. Thyroid hormone action during GABAergic neuron maturation: the quest for mechanisms. Front Endocrinol (Lausanne). (2023) 14:1256877. doi: 10.3389/fendo.2023.1256877
28. Krulich, L. Neurotransmitter control of thyrotropin secretion. Neuroendocrinology. (1982) 35:139–47. doi: 10.1159/000123369
29. Nardone, R, Brigo, F, and Trinka, E. Acute symptomatic seizures caused by electrolyte disturbances. J Clin Neurol. (2016) 12:21–33. doi: 10.3988/jcn.2016.12.1.21
30. Farrar, HC, Chande, VT, Fitzpatrick, DF, and Shema, SJ. Hyponatremia as the cause of seizures in infants: a retrospective analysis of incidence, severity, and clinical predictors. Ann Emerg Med. (1995) 26:42–8. doi: 10.1016/S0196-0644(95)70236-9
31. Liamis, G, Filippatos, TD, Liontos, A, and Elisaf, MS. MANAGEMENT OF ENDOCRINE DISEASE: hypothyroidism-associated hyponatremia: mechanisms, implications and treatment. Eur J Endocrinol. (2017) 176:R15–r20. doi: 10.1530/EJE-16-0493
32. Cao, J, Chen, C, Zhang, T, Han, Y, Qin, R, Su, W, et al. Segmental abnormalities of white matter microstructure in primary hypothyroidism identified by automated Fiber quantification. Neuroendocrinology. (2023) 113:589–605. doi: 10.1159/000529062
33. Sankar, T, Bernasconi, N, Kim, H, and Bernasconi, A. Temporal lobe epilepsy: differential pattern of damage in temporopolar cortex and white matter. Hum Brain Mapp. (2008) 29:931–44. doi: 10.1002/hbm.20437
34. Gasparini, S, Ferlazzo, E, Sueri, C, Cianci, V, Ascoli, M, Cavalli, SM, et al. Hypertension, seizures, and epilepsy: a review on pathophysiology and management. Neurol Sci. (2019) 40:1775–83. doi: 10.1007/s10072-019-03913-4
35. Tai, XY, Torzillo, E, Lyall, DM, Manohar, S, Husain, M, and Sen, A. Association of Dementia Risk with Focal Epilepsy and Modifiable Cardiovascular Risk Factors. JAMA Neurol. (2023) 80:445–54. doi: 10.1001/jamaneurol.2023.0339
36. Solinas, C, Briellmann, RS, Harvey, AS, Mitchell, LA, and Berkovic, SF. Hypertensive encephalopathy: antecedent to hippocampal sclerosis and temporal lobe epilepsy? Neurology. (2003) 60:1534–6. doi: 10.1212/01.WNL.0000061479.59789.10
37. Small, HY, Migliarino, S, Czesnikiewicz-Guzik, M, and Guzik, TJ. Hypertension: focus on autoimmunity and oxidative stress. Free Radic Biol Med. (2018) 125:104–15. doi: 10.1016/j.freeradbiomed.2018.05.085
38. Merelli, A, Repetto, M, Lazarowski, A, and Auzmendi, J. Hypoxia, oxidative stress, and inflammation: three faces of neurodegenerative diseases. J Alzheimers Dis. (2021) 82:S109–26. doi: 10.3233/JAD-201074
39. Bartolini, E, Ferrari, AR, Fiori, S, and Della, VS. Glycaemic imbalances in seizures and epilepsy of Paediatric age: a literature review. J Clin Med. (2023) 12:2580. doi: 10.3390/jcm12072580
40. Auer, RN. Hypoglycemic brain damage. Forensic Sci Int. (2004) 146:105–10. doi: 10.1016/j.forsciint.2004.08.001
41. Auer, RN. Hypoglycemic brain damage. Metab Brain Dis. (2004) 19:169–75. doi: 10.1023/B:MEBR.0000043967.78763.5b
42. Filan, PM, Inder, TE, Cameron, FJ, Kean, MJ, and Hunt, RW. Neonatal hypoglycemia and occipital cerebral injury. J Pediatr. (2006) 148:552–5. doi: 10.1016/j.jpeds.2005.11.015
43. Dingledine, R, Varvel, NH, and Dudek, FE. When and how do seizures kill neurons, and is cell death relevant to epileptogenesis? Adv Exp Med Biol. (2014) 813:109–22. doi: 10.1007/978-94-017-8914-1_9
44. Nunes, VS, da Silva, FG, and Quintão, ECR. Cholesterol metabolism in aging simultaneously altered in liver and nervous system. Aging (Albany NY). (2022) 14:1549–61. doi: 10.18632/aging.203880
45. Li, D, Zhang, J, and Liu, Q. Brain cell type-specific cholesterol metabolism and implications for learning and memory. Trends Neurosci. (2022) 45:401–14. doi: 10.1016/j.tins.2022.01.002
46. Zhang, J, and Liu, Q. Cholesterol metabolism and homeostasis in the brain. Protein Cell. (2015) 6:254–64. doi: 10.1007/s13238-014-0131-3
47. Hanin, A, Baudin, P, Demeret, S, Roussel, D, Lecas, S, Teyssou, E, et al. Disturbances of brain cholesterol metabolism: a new excitotoxic process associated with status epilepticus. Neurobiol Dis. (2021) 154:105346. doi: 10.1016/j.nbd.2021.105346
48. Biagini, G, Panuccio, G, and Avoli, M. Neurosteroids and epilepsy. Curr Opin Neurol. (2010) 23:170–6. doi: 10.1097/WCO.0b013e32833735cf
49. Lévesque, M, Biagini, G, and Avoli, M. Neurosteroids and focal epileptic disorders. Int J Mol Sci. (2020) 21:9391. doi: 10.3390/ijms21249391
50. Holló, A, Clemens, Z, and Lakatos, P. Epilepsy and vitamin D. Int J Neurosci. (2014) 124:387–93. doi: 10.3109/00207454.2013.847836
51. Holló, A, Clemens, Z, Kamondi, A, Lakatos, P, and Szűcs, A. Correction of vitamin D deficiency improves seizure control in epilepsy: a pilot study. Epilepsy Behav. (2012) 24:131–3. doi: 10.1016/j.yebeh.2012.03.011
52. Yuan, S, Tomson, T, and Larsson, SC. Modifiable risk factors for epilepsy: a two-sample Mendelian randomization study. Brain Behav. (2021) 11:e02098. doi: 10.1002/brb3.2098
53. Anwar, MJ, Alenezi, SK, and Alhowail, AH. Molecular insights into the pathogenic impact of vitamin D deficiency in neurological disorders. Biomed Pharmacother. (2023) 162:114718. doi: 10.1016/j.biopha.2023.114718
54. Dursun, E, Gezen-Ak, D, and Yilmazer, S. The influence of vitamin D treatment on the inducible nitric oxide synthase (INOS) expression in primary hippocampal neurons. Noro Psikiyatr Ars. (2014) 51:163–8. doi: 10.4274/npa.y7089
55. Brewer, LD, Thibault, V, Chen, KC, Langub, MC, Landfield, PW, and Porter, NM. Vitamin D hormone confers neuroprotection in parallel with downregulation of L-type calcium channel expression in hippocampal neurons. J Neurosci. (2001) 21:98–108. doi: 10.1523/JNEUROSCI.21-01-00098.2001
56. Zanatta, L, Goulart, PB, Gonçalves, R, Pierozan, P, Winkelmann-Duarte, EC, Woehl, VM, et al. 1α,25-dihydroxyvitamin D(3) mechanism of action: modulation of L-type calcium channels leading to calcium uptake and intermediate filament phosphorylation in cerebral cortex of young rats. Biochim Biophys Acta. (2012) 1823:1708–19. doi: 10.1016/j.bbamcr.2012.06.023
57. Heiry, M, Afra, P, Matsuo, F, Greenlee, JE, and Clardy, SL. Improvement of GAD65-associated autoimmune epilepsy with testosterone replacement therapy. Neurol Neuroimmunol Neuroinflamm. (2015) 2:e142. doi: 10.1212/NXI.0000000000000142
58. Białek, M, Zaremba, P, Borowicz, KK, and Czuczwar, SJ. Neuroprotective role of testosterone in the nervous system. Pol J Pharmacol. (2004) 56:509–18.
59. Yang, L, Zhou, R, Tong, Y, Chen, P, Shen, Y, Miao, S, et al. Neuroprotection by dihydrotestosterone in LPS-induced neuroinflammation. Neurobiol Dis. (2020) 140:104814. doi: 10.1016/j.nbd.2020.104814
60. Reddy, DS, and Jian, K. The testosterone-derived neurosteroid androstanediol is a positive allosteric modulator of GABAA receptors. J Pharmacol Exp Ther. (2010) 334:1031–41. doi: 10.1124/jpet.110.169854
61. Wang, L, Pei, JH, Jia, JX, Wang, J, Song, W, Fang, X, et al. Inhibition of oxidative stress by testosterone improves synaptic plasticity in senescence accelerated mice. J Toxicol Environ Health A. (2019) 82:1061–8. doi: 10.1080/15287394.2019.1683988
62. Rupprecht, R. Neuroactive steroids: mechanisms of action and neuropsychopharmacological properties. Psychoneuroendocrinology. (2003) 28:139–68. doi: 10.1016/S0306-4530(02)00064-1
63. Larson, EA, Accardi, MV, Zhong, Y, Paquette, D, and Authier, S. Drug-induced seizures: considerations for underlying molecular mechanisms. Int J Toxicol. (2021) 40:403–12. doi: 10.1177/10915818211040483
64. Liu, BC, Yang, LL, Lu, XY, Song, X, Li, XC, Chen, G, et al. Lovastatin-induced Phosphatidylinositol-4-phosphate 5-kinase diffusion from microvilli stimulates ROMK channels. J Am Soc Nephrol. (2015) 26:1576–87. doi: 10.1681/ASN.2013121326
65. Scicchitano, F, Constanti, A, Citraro, R, De Sarro, G, and Russo, E. Statins and epilepsy: preclinical studies, clinical trials and statin-anticonvulsant drug interactions. Curr Drug Targets. (2015) 16:747–56. doi: 10.2174/1389450116666150330114850
66. Bösel, J, and Endres, M. Direct neuronal effects of statins. Nervenarzt. (2006) 77:289–93. doi: 10.1007/s00115-005-1963-0
67. Sodero, AO, and Barrantes, FJ. Pleiotropic effects of statins on brain cells. Biochim Biophys Acta Biomembr. (2020) 1862:183340. doi: 10.1016/j.bbamem.2020.183340
68. Mayer, T, and Specht, U. Propranolol in startle induced epileptic seizures. J Neurol Neurosurg Psychiatry. (1995) 58:382–3. doi: 10.1136/jnnp.58.3.382
69. Reno, CM, Skinner, A, Bayles, J, Chen, YS, Daphna-Iken, D, and Fisher, SJ. Severe hypoglycemia-induced sudden death is mediated by both cardiac arrhythmias and seizures. Am J Physiol Endocrinol Metab. (2018) 315:E240–9. doi: 10.1152/ajpendo.00442.2017
Keywords: epilepsy, Mendelian randomization, ischemic stroke, metabolic factors, medications
Citation: Wang W, Liu M, Liu F, Wang Z, Ye W and Li X (2024) Causal associations of ischemic stroke, metabolic factors, and related medications with epilepsy: a Mendelian randomization study. Front. Neurol. 15:1464984. doi: 10.3389/fneur.2024.1464984
Edited by:
Yun Ping Lim, China Medical University, TaiwanReviewed by:
Carmen Rubio, Manuel Velasco Suárez National Institute of Neurology and Neurosurgery, MexicoGaetano Gorgone, Neurology Unit Jazzolino Hospital Vibo Valentia Italy, Italy
Copyright © 2024 Wang, Liu, Liu, Wang, Ye and Li. This is an open-access article distributed under the terms of the Creative Commons Attribution License (CC BY). The use, distribution or reproduction in other forums is permitted, provided the original author(s) and the copyright owner(s) are credited and that the original publication in this journal is cited, in accordance with accepted academic practice. No use, distribution or reproduction is permitted which does not comply with these terms.
*Correspondence: Xianfeng Li, bGl4aWFuZmVuZzIwMDBAMTYzLmNvbQ==
†ORCID: Wencai Wang, https://orcid.org/0009-0004-5673-1643
Xianfeng Li, https://orcid.org/0000-0001-8549-537X