- 1University of Ulsan College of Medicine, Seoul, Republic of Korea
- 2Department of Radiology, Seoul National University Bundang Hospital, Seoul National University College of Medicine, Seongnam, Republic of Korea
- 3Department of Radiology and Research Institute of Radiology, Asan Medical Center, University of Ulsan College of Medicine, Seoul, Republic of Korea
- 4Department of Imaging, Dana-Farber Cancer Institute, Harvard Medical School, Boston, MA, United States
- 5Division of Neuroradiology, Brigham and Women's Hospital, Dana-Farber Cancer Institute, Harvard Medical School, Boston, MA, United States
- 6Department of Oncology, Asan Medical Center, University of Ulsan College of Medicine, Seoul, Republic of Korea
Objectives: We aim to assess the pooled incidence of immune effector cell-associated neurotoxicity syndrome (ICANS) in clinical trials and real-world studies of chimeric antigen receptor (CAR) T-cell therapy for hematologic malignancy and compare the incidences among different agents.
Methods: The PubMed, Embase, and Web of Science databases were searched for clinical trials and real-world studies. An inverse-variance weighting model was used to calculate pooled incidences and subgroup analyses. Multivariable analysis was conducted using binomial-normal modeling.
Results: Seventy-five trials comprising 3,184 patients were included. The overall pooled incidence was 26.9% (95% CI, 21.7–32.7%) for all-grade and 10.5% (95% CI, 8.1–13.6%) for high-grade ICANS. In subgroup analysis, cohorts with anti-CD19 drugs had significantly higher ICANS incidences than cohorts with other agents. The multivariable analysis demonstrated higher odds of ICANS in anti-CD19 drug studies for high-grade (OR, 4.6) compared to anti-BCMA drug studies. In 12 real-world studies, studies used axicabtagene ciloleucel with CD28 (54.0% all-grade, 26.4% high-grade) exhibited significantly higher rates of all-grade and high-grade ICANS than studies using tisagenlecleucel with 4-1BB (17.2% all-grade, 6.1% high-grade).
Conclusions: The overall incidences of ICANS with CAR T-cell therapy were 26.9% for all-grade and 10.5% for high-grade. Compared with other agents, patients with anti-CD19 drugs had a significantly increased risk of developing high-grade ICANS. Therefore, careful monitoring of ICANS should be considered for patients undergoing CAR T-cell therapy.
Highlights
• The pooled incidence of ICANS with CAR T-cell therapy was 26.9% (95% CI, 21.7–32.7%) for all-grade and 10.5% (95% CI, 8.1–13.6%) for high-grade.
• Univariable meta-regression analysis showed that leukemia patients (OR, 4.7; 95% CI, 1.5–14.2; P = 0.007) and lymphoma patients (OR, 3.1; 95% CI, 1.1–9.1; P = 0.036) had higher odds of all-grade ICANS compared with patients with multiple myeloma.
• Multivariable meta-regression analysis showed that patients treated with anti-CD19 drugs had higher odds for all-grade (OR, 2.7; 95% CI, 1.0–7.7; P = 0.057) and high-grade (OR, 4.6; 95% CI, 1.5–13.7; P = 0.008) ICANS compared with patients treated with anti-BCMA drugs.
Introduction
Chimeric antigen receptor (CAR) T-cell therapy is promising immunotherapy for hematologic malignancies (1–3). Studies have shown a high response rate to CAR T-cell therapy with remission rates of up to 80% or more in patients with relapsed or refractory hematologic malignancies (4–6). To date, six CAR T-cell therapies targeting the CD19 antigen or B-cell maturation antigen (BCMA) have received approval from the United States Food and Drug Administration (FDA): tisagenlecleucel, axicabtagene ciloleucel, brexucabtagene autoleucel, lisocabtagene maraleucel, idecabtagene vicleucel, and ciltacabtagene autoleucel (7–9). Toxicities associated with CAR T-cell therapy, including cytokine-release syndrome (CRS) and neurotoxicity, have been reported as adverse events in almost all clinical trials (10–13).
Neurotoxicity, what is termed “immune effector cell-associated neurotoxicity syndrome (ICANS),” is the second most common adverse event following CRS. The incidence of ICANS has been reported to range widely, from 5 to 42%, in clinical trials (1, 14–20), but the exact incidence of ICANS among patients undergoing CAR T-cell therapy has not been systematically investigated in large-scale datasets. Furthermore, although it has been assumed that incidences of ICANS differ according to the type of agent used or other factors such as the co-stimulatory domain or number of agents, there is no concrete evidence to support this hypothesis or demonstrate the magnitude of the differences.
We, therefore, performed a systematic review and meta-analysis of the incidence of ICANS in clinical trials and real-world studies of CAR T-cell therapies for hematologic malignancies. Our aim is to determine if there are differences in the incidence of ICANS among cohorts with various underlying diseases, treated with agents targeting different antigens, and utilizing different co-stimulatory domains. The null hypothesis was that there would be no differences in the incidence of ICANS across these cohorts.
Clinical trials of CAR T-cell therapies for hematologic malignancies, until May 28, 2022 were included in the study. The pooled incidence of ICANS was calculated using the inverse-variance weighting method.
Materials and methods
We followed the Preferred Reporting Items for Systematic Reviews and Meta-Analysis (PRISMA) guidelines (21, 22); the completed checklist is provided in Supplementary Table 1.
Search methods and study selection
The PubMed, Embase, and Web of Science databases were searched for published clinical trials of CAR T-cell therapies administered to patients with hematologic malignancies until May 28, 2022. The search terms were formulated using “car t cell therapy” and “neurotoxicity” as keywords. Details and specific search queries are described in the Supplementary Tables 2–4. This study was registered in the international prospective register of systematic reviews (PROSPERO; CRD4202233390960).
The inclusion criteria were as follows: (1) clinical trials with patients treated with CAR T-cell therapies for hematologic malignancies and (2) detailed data sufficient to assess the incidence of ICANS. The exclusion criteria were as follows: (1) conference abstracts, review articles, letters, editorials, comments, notes, short surveys, or chapters; (2) studies other than clinical trials; (3) studies not reporting on CAR T-cell therapy; (4) studies not discussing ICANS; (5) studies with patient numbers below 10; (6) study protocols; (7) studies with patient cohorts overlapping with those of other studies; and (8) summaries of other studies.
For additional analysis of the incidence of ICANS in real-world studies, an additional computerized search of the literature was performed using the PubMed and Embase databases for published real-world studies of CAR T-cell therapies until August 13, 2022. The search terms and inclusion/exclusion criteria were identical to the analysis for previous clinical trials. The added inclusion criterion was (1) studies in the real-world clinical setting, and the added exclusion criterion was (1) studies with patient numbers below 100.
The 75 eligible studies of clinical trials comprised of 3,184 patients were included in our analysis (Figure 1), and 12 eligible studies of real-world studies comprised of 3,403 patients were included for additional analysis for real-world clinical settings.
Data extraction
From the each eligible article, we extracted data indicating the numbers of patients who developed ICANS of all grade and high-grade (grade 3 or higher), and the numbers of ICANS-related deaths (grade 5). Different articles referred to ICANS in varying ways; we included reports on “neurotoxicity,” “neurologic events,” or “neurologic symptoms.” In addition, the phase of each clinical trial, NCT numbers, types of specific CAR T-cells used, and the included patients' diseases were recorded. Specifically, the CAR T-cell types included all landmark treatments with FDA approval (axicabtagene ciloleucel, brexucabtagene autoleucel, lisocabtagene maraleucel, tisagenlecleucel, idecabtagene vicleucel, and ciltacabtagene autoleucel); we also included various types of CAR T-cells currently waiting for FDA approval. We focused on the targets of CAR T-cells rather than the brand of each drug. Two reviewers (M.W.H and S.Y.J) performed the data extraction, with an independent review by an additional reviewer (C.H.S); when discrepancies were identified between the data extracted by the two reviewers, they first engaged in a discussion to understand the source of the disagreement and to try to resolve it collaboratively. If the discussion did not lead to a consensus, they referred to the predefined data extraction protocol to ensure alignment with the study's objectives and criteria. Throughout this process, all discrepancies and their resolutions were thoroughly documented to maintain transparency and ensure the integrity of the review.
Quality assessment
We assessed the risk of bias for each study using the Cochrane risk of bias tool for randomized controlled trials (23). The tool evaluates seven domains including random sequence generation, allocation concealment, blinding of participants and personnel, blinding of outcome assessment, incomplete outcome data, selective reporting, and others to assess selection, performance, detection, attrition, and reporting biases. Based on available information, two independent reviewers (M.W.H and S.Y.J) scored each domain as high or low risk or unclear. Disagreements were resolved by discussion.
In addition, the quality of evidence from the pooled results were evaluated using the Grading of Recommendations Assessment, Development, and Evaluation (GRADE) system (24).
Statistical methods
The pooled incidence of all-grade and high-grade ICANS was obtained using an inverse-variance weighting model (25). Heterogeneity was evaluated with Cochran's Q test and Higgins inconsistency index (I2) test, with values >50% indicating substantial heterogeneity. Publication bias was evaluated and recorded using Egger's test and funnel plots (26).
The pooled incidence of ICANS was also obtained for each subgroup classified according to CAR T-cell agent, patient disease, trial phase, number of used drugs (monotherapy vs. combination therapy), co-stimulatory domain, lymphodepletion strategy, and involvement or non-involvement of the CNS. Univariable meta-regression analyses were conducted to assess the associations between each of the study-level covariates and the incidence of ICANS. Multivariable analysis was performed using binomial-normal modeling (27, 28). To test if study-level covariates as moderators had statistical effects in the meta-regression, the regression coefficient was obtained to estimate the intervention effect on each subgroup from a reference group. All statistical analyses were performed using the “meta” package of R version 4.0.4 (R Foundation for Statistical Computing, Vienna, Austria) (29).
Results
Literature search
Initially, 222 articles from PubMed, 290 articles from Embase, and 143 articles from Web of Science were screened using keywords. Of the 655 articles, 249 were excluded by an automation tool and by a review of the article titles to eliminate duplication, conference abstracts, reviews, letters, editorials, comments, notes, short surveys, and chapters. The complete texts of the remaining 406 articles were retrieved, and 331 studies were further excluded after reviewing the full texts (188 articles were not clinical trials, 27 were summaries of other studies, six had partially overlapping patient cohorts, 43 did not discuss ICANS, 24 enrolled fewer than 10 patients, 20 were not about CAR T-cell therapy, 16 presented secondary analyses, and seven were about study protocols). The remaining 75 eligible studies comprised of 3,184 patients were included (1, 4, 5, 14–20, 30–94).
For an additional search for analysis of the incidence of ICANS in real-world clinical settings, 12 studies comprised of 3,403 patients were included in the analysis for real-world studies (95–106). The detailed literature search for analysis of real-world studies is provided in Supplementary material.
Risk of bias
A risk of bias assessment was performed for each study (Supplementary Figure 1). Based on available information, each criterion was scored by two independent reviewers as high risk, low risk, or unclear. Disagreements were resolved by discussion. Details of trial evaluations are detailed in Supplementary Table 5.
Characteristics of the included studies
Table 1 summarizes the characteristics of the included studies. Among the 75 eligible trial cohorts, 42 cohorts (1, 14, 16, 18–20, 30, 31, 33–36, 39, 40, 45, 47, 49, 50, 52, 53, 55, 56, 58, 60–64, 66, 69–81) (56.0%) were phase 1 trials, 21 (4, 5, 15, 17, 42, 43, 51, 54, 57, 59, 65, 67, 68, 82–86, 88, 89, 91) (28.0%) were phase 2 trials, and one (92) (1.3%) was a phase 3 trial. Nine studies (32, 37, 38, 41, 44, 46, 48, 87, 93) employed combined designs for phase 1/2 trials; these studies (combined phase 1/2 or 1b/2 trials) were included in the analysis as phase 2 trials. The remaining two studies (90, 94) (2.7%) did not mention the trial phase. Different studies used different types of CAR T-cells, and we classified each drug according to its specific target. The FDA-approved agents all target CD19, except idecabtagene vicleucel and ciltacabtagene autoleucel, which target BCMA. Among the 75 cohorts, 47 (62.7%) (1, 4, 5, 14, 16, 17, 31, 33, 35–38, 40, 41, 43, 47–50, 53–55, 57–63, 66, 68, 69, 72, 74, 75, 77, 78, 82–86, 88, 91–94) used agents that target CD19, nine (12.0%) (20, 32, 34, 39, 42, 45, 52, 56, 71) used agents that target BCMA, two (2.7%) (18, 44) used agents that target CD22, and 17 others (22.7%) (15, 19, 30, 46, 51, 64, 65, 67, 70, 73, 76, 79–81, 87, 89, 90) used agents that target various proteins, such as CD7, CD20, CD28, CD30, or NKG2D. Moreover, 61 of the 75 cohorts (81.3%) used single agents (anti-CD19, anti-BCMA, anti-CD22, anti-CD30, anti-CD7, or anti-NKG2D), 14 (18.7%) used combinations of agents (anti-CD19+anti-BCMA, anti-CD19+anti-20, anti-CD19+anti-22, anti-CD19+anti-28, or anti-BCMA+anti-38). Additional details about agents, doses and CD4:CD8 ratios for each cohort are listed in Supplementary Table 6.
Among the 75 trial cohorts, 24 (32.0%) included treatment for leukemia, 32 (42.7%) for lymphoma, and 12 (16.0%) for multiple myeloma. In seven articles (9.3%), patients with various diseases were pooled or the diseases treated were not stated.
Four kinds of grading schemes of ICANS were used across studies: the National Cancer Institute Common Terminology Criteria for Adverse Events, the American Society for Transplantation and Cellular Therapy scale, the American Society for Blood and Marrow Transplantation consensus, and the MD Anderson Cancer Center Scale CAR-T-cell-related encephalopathy syndrome grading system. Additional details about the ICANS grading schemes for each cohort are listed in Supplementary Table 6.
Among the 12 included studies for additional analysis of the real-world studies, seven studies included patients used agents of axicabtagene ciloleucel and tisagenlecleucel, respectively (95, 96, 98, 99, 102, 105, 106). Three studies included patients who used axicabtagene ciloleucel (100, 103, 104), and two had those who used tisagenlecleucel (97, 101). All patients treated with axicabtagene ciloleucel used CD28 as a co-stimulatory domain, and all patients treated with tisagenlecleucel used 4-1BB as a co-stimulatory domain.
Pooled incidence of neurotoxicity among patients undergoing CAR T-cell therapy
We evaluated the incidence of all-grade and high-grade ICANS after CAR T-cell infusion in a total of 75 cohorts of patients. The overall pooled incidence, evaluated with a random effects model, was 26.9% (95% CI, 21.7–32.7%; Figure 2) for all grades and 10.5% (95% CI, 8.1–13.6%; Figure 3) for high-grade ICANS (Table 2). Heterogeneity was observed in both all-grade and high-grade ICANS (I2 = 84.1% and I2 = 73.3%, respectively). Publication bias likely occurred in both all-grade and high-grade ICANS analyses (P < 0.01; Supplementary Figures 2, 3). Three cases of grade 5 ICANS were reported across cohorts, one in a study using a brexucabtagene autoleucel agent in leukemia patients, one in a cohort of multiple myeloma patients using a ciltacabtagene autoleucel agent, and one in leukemia patients using an anti-CD19 agent.
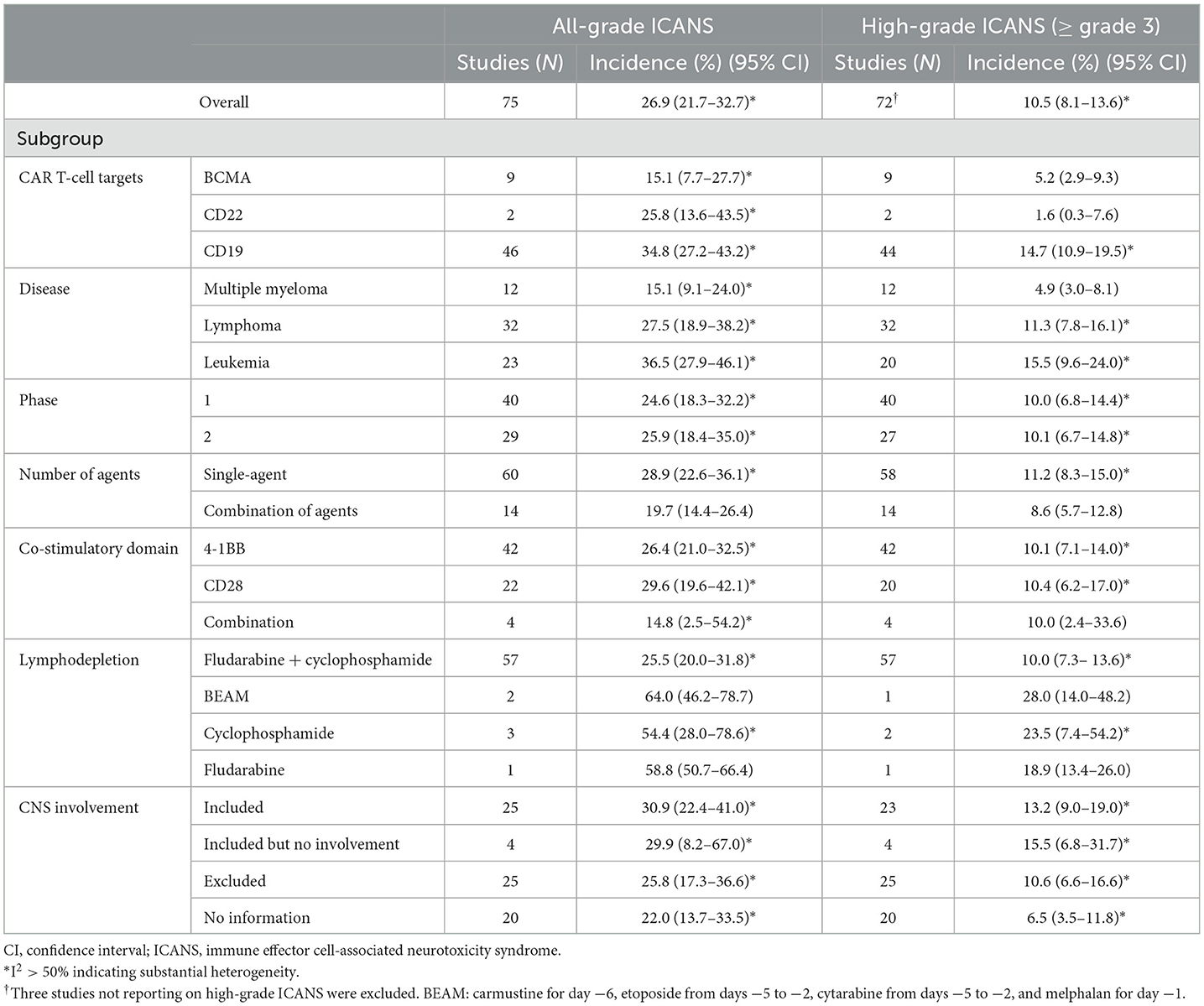
Table 2. Results of the multiple subgroup analysis on the incidence of ICANS after CAR T-cell therapy.
To address the high heterogeneity observed in the incidence of all-grade and high-grade ICANS, we conducted a sensitivity analysis by removing ~15% of the outlier studies (11 out of 75 studies). This adjustment resulted in a substantial reduction in heterogeneity, with the I2 for all-grade ICANS decreasing from 84 to 67%, and for high-grade ICANS from 73 to 43%, while maintaining the overall trend of our results (Supplementary Figure 4).
Subgroup analyses
We evaluated the incidence of ICANS classified according to target agent (Table 2). The pooled incidences associated with anti-BCMA agents were 15.1% (95% CI, 7.7–27.7%) for all-grade and 5.2% (95% CI, 2.9–9.3%) for high-grade ICANS. The pooled incidences associated with anti-CD22 agents were 25.8% (95% CI, 13.6–43.5%) for all-grade and 1.6% (95% CI, 0.3–7.6%) for high-grade ICANS. The pooled incidences associated with anti-CD19 agents were 34.8% (95% CI, 27.2–43.2%) for all-grade and 14.7% (95% CI, 10.9–19.5%) for high-grade ICANS. Agents targeting CD19 showed significantly higher rates of both all-grade and high-grade ICANS than agents targeting CD22 or BCMA (P < 0.05 for all-grade and high-grade).
In subgroups classified by patient disease, the pooled incidences for patients with multiple myeloma were 15.1% (95% CI, 9.1–24.0%) for all-grade and 4.9% (95% CI, 3.0–8.1%) for high-grade ICANS. For patients with lymphoma, the pooled incidences were 27.5% (95% CI, 18.9–38.2%) for all-grade and 11.3% (95% CI, 7.8–16.1%) for high-grade ICANS. Finally, for patients with leukemia, the pooled incidences were 36.5% (95% CI, 27.9–46.1%) for all-grade and 15.5% (95% CI, 9.6–24.0%) for high-grade ICANS. Patients with leukemia had significantly higher rates of both all-grade and high-grade ICANS than patients with lymphoma or multiple myeloma (P < 0.05 for all-grade and high-grade).
In subgroups classified according to the number of agents, cohorts using a single agent exhibited higher rates of all-grade ICANS (P = 0.05) than cohorts using combinations of agents. In subgroups classified by co-stimulatory domain, the pooled incidences for 4-1BB, evaluated with a random effects model, were 26.4% (95% CI, 21.0–32.5%) for all-grade and 10.1% (95% CI, 7.1–14.0%) for high-grade ICANS. For CD28, the pooled incidences were 29.6% (95% CI, 19.6–42.1%) for all-grade and 10.4% (95% CI, 6.2–17.0%) for high-grade ICANS.
Univariable and multivariable meta-regression analyses
The univariable meta-regression analyses yielded significantly higher odds for all-grade [odds ratio [OR], 3.7; 95% CI, 1.2–11.9; P = 0.029] and high-grade (OR, 4.9; 95% CI, 1.6–14.7; P = 0.006) ICANS for the cohorts treated with anti-CD19 drugs than for those treated with anti-BCMA drugs (Tables 3, 4). The cohorts consisting of leukemia patients had significantly higher odds for all-grade (OR, 4.7; 95% CI, 1.5–14.2; P = 0.007) and high-grade (OR, 5.9; 95% CI, 1.8–19.0; P = 0.003) ICANS than those consisting of multiple myeloma patients. The cohorts of lymphoma patients also had significantly higher odds for all-grade (OR, 3.1; 95% CI, 1.1–9.1; P = 0.036) and high-grade (OR, 3.9; 95% CI, 1.3–11.8; P = 0.017) ICANS than the cohorts of multiple myeloma patients. No significant differences in ICANS incidences were observed between cohorts with different trial phases, therapy types, co-stimulatory domains, and CNS involvement or non-involvement.
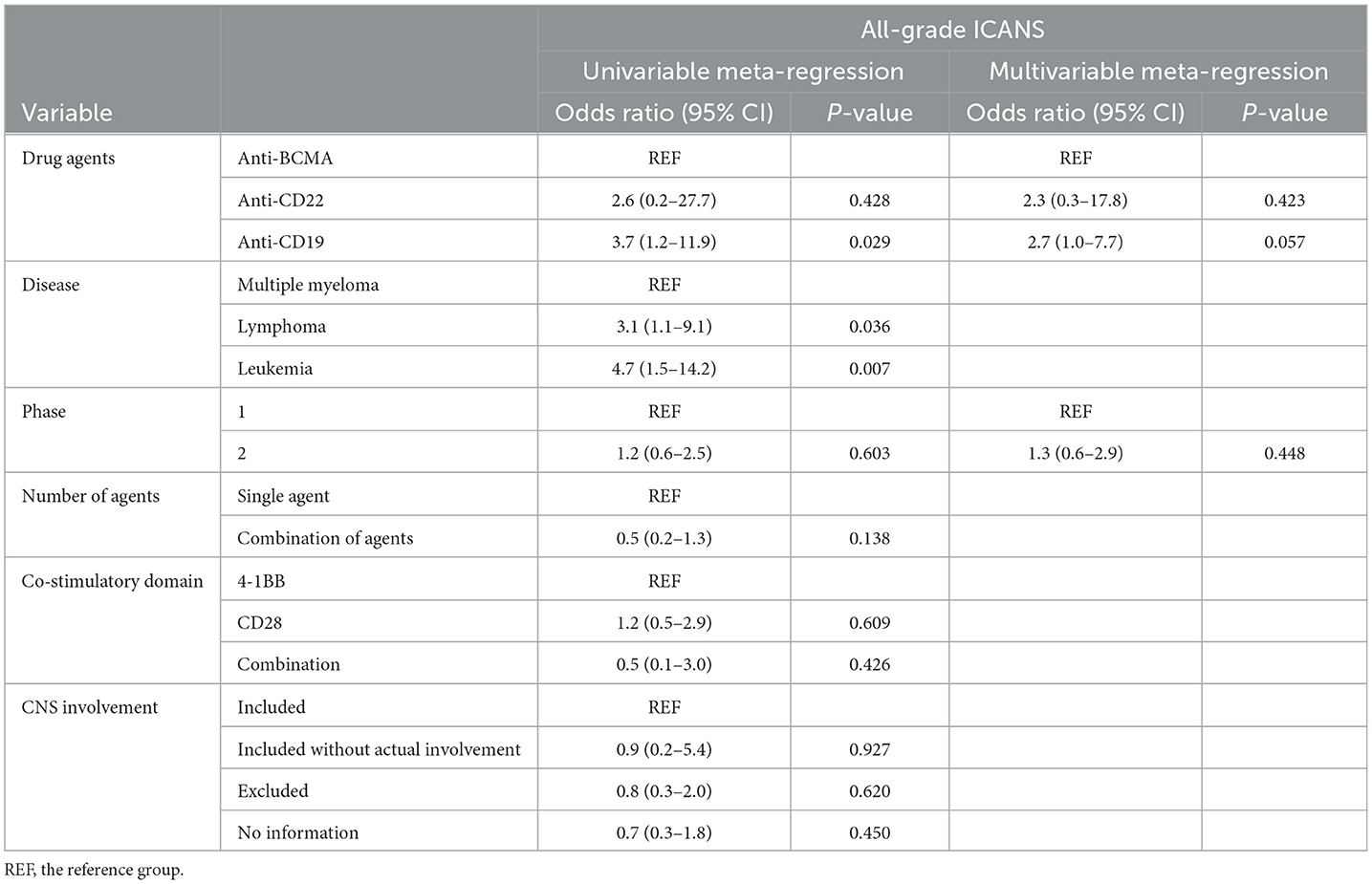
Table 3. Results of the univariable and multivariable meta-regression on the incidence of all-grade ICANS.
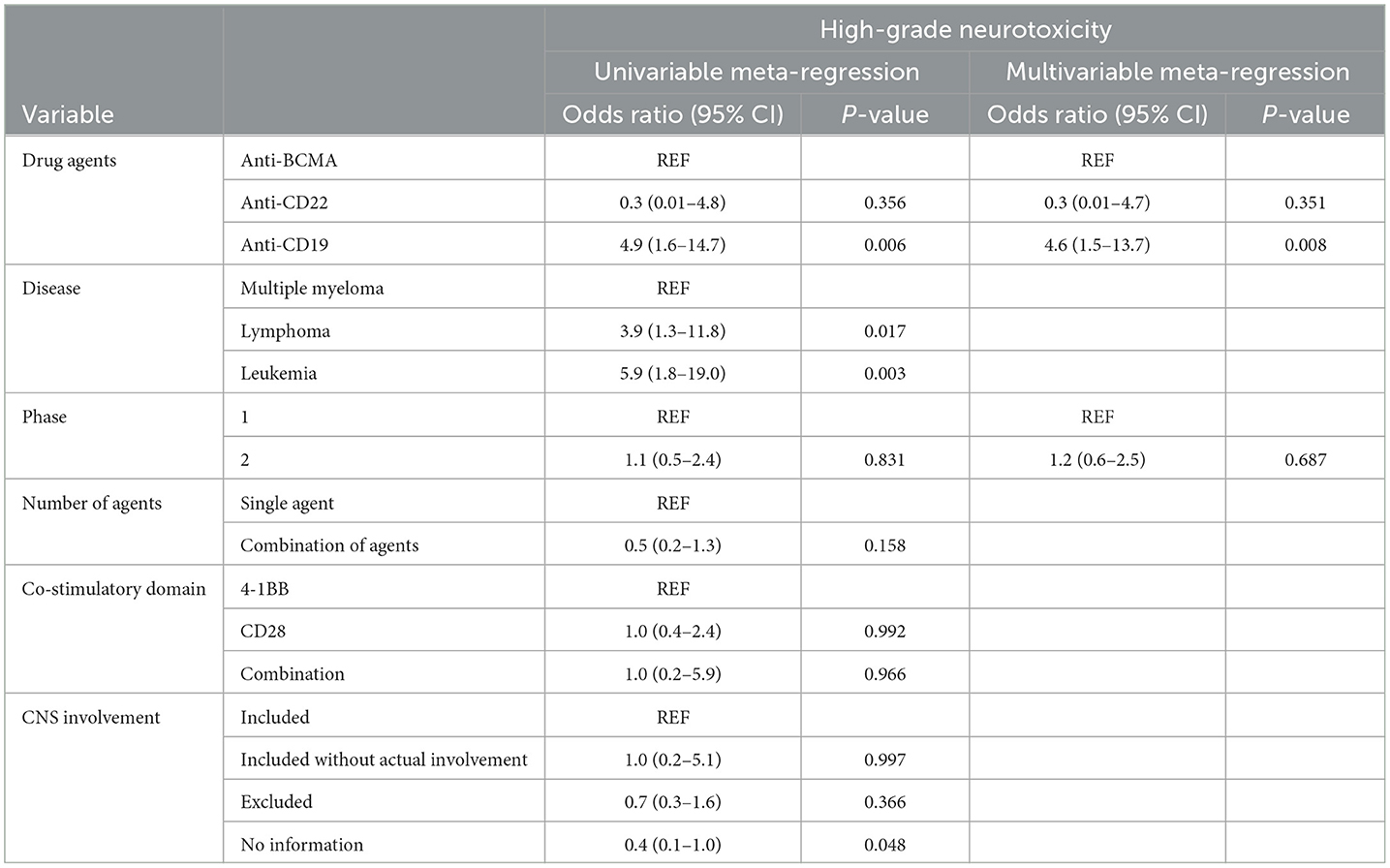
Table 4. Results of the univariable and multivariable meta-regression on the incidence of high-grade ICANS.
The multivariable meta-regression analyses showed high levels of coexistence and concurrence for types of drug agents and diseases. Diseases were excluded from the multivariable analysis to avoid multi-collinearity. The cohorts treated with anti-CD19 drugs had higher odds for all-grade (OR, 2.7; 95% CI, 1.0–7.7; P = 0.057) ICANS than cohorts treated with anti-BCMA drugs, with borderline significance. The cohorts treated with anti-CD19 drugs had significantly higher odds for high-grade (OR, 4.6; 95% CI, 1.5–13.7; P = 0.008) ICANS than cohorts treated with anti-BCMA drugs.
Evidence of the critical outcomes
The GRADE system was used to assess the certainty of evidence for the pooled incidence outcome (Table 5). Due to a high risk of bias in the included studies and a strongly suspected publication bias, the overall quality of evidence regarding the incidence of ICANS in patients receiving CAR-T cell therapy for hematologic malignancies was rated as moderate.
Analysis of real-world studies
We evaluated the incidence of ICANS in real-world clinical data of 12 studies classified according to target agent (axicabtagene ciloleucel and tisagenlecleucel) (95–106). The pooled incidences for axicabtagene ciloleucel with CD28 were 54.0% (95% CI, 46.5–61.4%) for all-grade and 26.4% (95% CI, 21.3–32.2%) for high-grade ICANS. The pooled incidences for tisagenlecleucel with 4-1BB were 17.2% (95% CI, 13.7–21.4%) for all-grade and 6.1% (95% CI, 4.5–8.1%) for high-grade ICANS. Studies using axicabtagene ciloleucel with CD28 exhibited higher rates of all-grade and high-grade ICANS than studies using tisagenlecleucel with 4-1BB (P < 0.001 for all-grade and high-grade ICANS; Supplementary Figure 5). There were six deaths from ICANS (grade 5), and all cases were treated with axicabtagene ciloleucel with CD28 (95, 100, 102).
Discussion
In the present meta-analyses, the overall ICANS incidence among patients undergoing CAR T-cell therapy for hematologic malignancies was 26.9% for all-grade and 10.5% for high-grade ICANS. In the subgroup analysis according to the type of agent, the cohorts with anti-CD19 drugs had significantly higher ICANS incidence than those with anti-BCMA drugs. The multivariable analysis also demonstrated significant higher odds of ICANS in anti-CD19 drug studies for high-grade (OR, 4.6; P = 0.008) ICANS compared with studies on anti-BCMA drugs. In real-world studies, studies using axicabtagene ciloleucel with CD28 (54.0% for all-grades, 26.4% for high-grades) exhibited significantly higher rates of ICANS than studies using tisagenlecleucel with 4-1BB (17.2% for all-grades, 6.1% for high-grades). The current study demonstrates that ICANS is not uncommon among patients undergoing CAR T-cell therapy, and our results provide further insights into risk factors for ICANS.
In terms of risk factors associated with ICANS in CAR T-cell therapies, previous studies reported that ICANS is significantly correlated with a high pretreatment disease burden and a higher peak CAR T-cell expansion (107, 108). However, it is difficult to predict which patients will develop ICANS, the timing of their symptoms, or their disease severity. Rubin et al. (103) developed a predictive scoring system for ICANS, which includes age, histologic subtype, maximum temperature, maximum C-reactive protein level, ferritin level, minimum white blood cell count, CRS severity, and CRS onset day. Our subgroup analysis by patient disease, drug agent, and the number of agents showed that patients with leukemia, and anti-CD19 drugs had significantly higher rates of ICANS than patients with other diseases, and drugs that have other targets. However, the high ICANS rate among leukemia patients may be attributable to confounding factors, given that CD19-targeting CAR T-cell drugs are more generally used to treat leukemia. The risk of ICANS in patients with preexisting neurologic comorbidities was controversial in previous studies (76, 109). However, in our study, the risk did not appear to be higher in groups with preexisting neurologic comorbidities.
On February 2022, the United States FDA approved ciltacabtagene autoleucel for treating adults with relapsed or refractory multiple myeloma, making it the sixth FDA-approved CAR T-cell agent. The ciltacabtagene autoleucel trials had reported atypical neurotoxicity (non-ICANS-related neurotoxicity) after the resolution of ICANS. Symptoms associated with non-ICANS neurotoxicity included parkinsonian symptoms, peripheral motor neuropathy, and cranial nerve palsies. Van Oekelen et al. (110) reported cases of multiple myeloma patients, enrolled in the CARTITUDE-1 trial, who developed a progressive movement disorder with features of parkinsonism 3 months after ciltacabtagene autoleucel infusion. These findings indicate that further research into non-ICANS-related neurotoxicity is needed.
Our multivariable analysis also demonstrated significantly increased odds of ICANS in the cohorts treated with anti-CD19 drugs for high-grade (OR, 4.6; P = 0.008) ICANS compared with cohorts treated with anti-BCMA drugs. These results indicate that anti-CD19 drugs are an independent risk factor for high-grade ICANS in patients undergoing CAR T-cell therapy. Multiple studies have explored the biological mechanisms of neurotoxicity in patients treated with CAR T-cells. The primary mechanism currently suggested is endothelial dysfunction and increased blood-brain barrier (BBB) permeability. Gust et al. (109) demonstrated widespread endothelial activation and increased BBB permeability, followed by leakage of systemic cytokines, including IFN-γ, into the cerebrospinal fluid. A recent study by Parker et al. (111), using single-cell RNA sequencing, revealed the expression of CD19 in brain mural cells surrounding the endothelium. This finding indicates the potential for CD19-targeted CAR T-cells to recognize CD19+ mural cells, leading to endothelial dysfunction and subsequent neurotoxicity. Anti-BCMA drugs, while associated with clinically significant incidences of ICANS, have also been linked to atypical neurotoxic events that appear distinct from ICANS in several clinical studies, including tremor and hemiparesis (32, 42). Although the mechanisms underlying these atypical neurotoxicities are not yet fully understood, the presence of BCMA expression in the basal ganglia and cerebellum could potentially explain the occurrence of atypical Parkinsonian symptoms associated with anti-BCMA drugs (112).
Both axicabtagene ciloleucel and tisagenlecleucel are anti-CD19 drugs but use different co-stimulatory domains. Axicabtagene ciloleucel uses CD28 as the co-stimulatory domain, and tisagenlecleucel uses 4-1BB as the co-stimulatory domain. In real-world studies, studies using axicabtagene ciloleucel with CD28 exhibited higher rates of both all-grade and high-grade ICANS than those using tisagenlecleucel with 4-1BB (both P < 0.001 for all-grade and high-grade ICANS). These differences in the incidence of ICANS are possibly related to its CD28 co-stimulation domain. In a previous study (113), CAR T-cells with CD28 co-stimulatory domains posed a greater risk for the development of ICANS. CD28 and 4-1BB operate via different mechanisms as co-stimulatory domains in CAR T-cells. The CD28-CD80/86 interaction enhances IL-2 activity, upregulates pro-survival gene transcription, and promotes Th1 cytokine production. CD28 co-stimulation is generally believed to preferentially expand CD4+ T cells. Conversely, 4-1BB, also known as CD137, is similar in its upregulation of anti-apoptotic proteins and IL-2, but it preferentially leads to CD8+ T cell expansion (114). Several studies have demonstrated that T cell expansion occurs more rapidly and with greater amplitude when stimulated by CD28 compared to 4-1BB in vivo. Salter et al. (115) observed significantly increased kinetics and intensity of T cell phosphorylation via mass spectrometry when stimulated by CD28/CD3ζ signaling compared to 4-1BB/CD3ζ signaling. Similarly, Sterner et al. (116) noted that CD28-stimulated CAR T-cells tend to differentiate into central memory T cells and rely on aerobic glycolysis, which may contribute to their faster onset and eventual exhaustion. These findings, altogether provide a biological basis for the higher incidence of ICANS observed in cohorts using axicabtagene ciloleucel with CD28 in our real-world study.
Imaging findings related to ICANS among patients undergoing CAR T-cell therapy have been variable and unspecific. The most common imaging findings associated with ICANS are cerebral vasogenic or cytotoxic edema, detected on T2-weighted and FLAIR as hyperintensities with or without diffusion restriction (1, 117). Reported locations of involvement include the bilateral thalami, brainstem, and splenium of the corpus callosum (113, 117). In a study by Santomasso et al. (113), brain MRI findings were normal in all five patients with grade 1 and 2 ICANS as well as in four of 14 patients with severe ICANS (113). Other abnormalities, including leptomeningeal enhancement and multifocal microhemorrhage, have also been reported (5). These imaging abnormalities may be explained by several potential pathophysiologic mechanisms: endothelial cell damage, disruption of the BBB, and systemic inflammation (117, 118).
The present study has, however, several limitations. First, the present meta-analysis was limited to cohort-level data, as patient-level data were not available. This restriction hindered us to assess individual patient-level risk factors associated with ICANS, such as disease burden, number of previous lines of treatment, age, and baseline cognitive function. Consequently, these patient-level factors, which might influence the development of ICANS, were not analyzed in our study. Second, there are a number of previous systemic reviews and articles about the adverse effects of CAR T-cell therapy. However, previous studies included only patients with specific diseases and analyzed only specific treatment agents. The advantage of this meta-analysis is that we included a total of 75 recent papers, covered the entire range of hematologic malignancies, and also performed multiple subgroups and multivariable meta-regression analyses. Third, despite our efforts to address heterogeneity through sensitivity analysis, subgroup analyses and meta-regression, substantial heterogeneity remained in some of the results. A relatively large sample size (75 studies) contributed to substantial heterogeneity in our results. Also, this heterogeneity reflects the diverse clinical settings and methodologies of the included studies, and underscores the need for cautious interpretation of our results. Lastly, the employed grading systems of neurotoxicity were heterogeneous, and we did not attempt to unify the schemes. There are, however, differences between these grading methods, and more sophisticated studies that correct for such differences are needed in the future. Overall, the results of our study should be interpreted with caution due to high levels of heterogeneity among the included studies.
Conclusion
In conclusion, an overall incidence of ICANS among patients undergoing CAR T-cell therapy were 26.9% for all-grade and 10.5% for high-grade ICANS. Patients with anti-CD19 drugs had a significantly increased risk of developing high-grade ICANS compared to patients with anti-BCMA drugs. These results suggest that careful monitoring of ICANS should be considered for patients undergoing CAR T-cell therapy, particularly those treated with anti-CD19 drugs and those with leukemia.
Data availability statement
The original contributions presented in the study are included in the article/Supplementary material, further inquiries can be directed to the corresponding author.
Author contributions
MH: Data curation, Formal analysis, Investigation, Methodology, Writing – original draft. SJ: Conceptualization, Data curation, Formal analysis, Investigation, Methodology, Software, Writing – original draft, Writing – review & editing, Supervision. CS: Conceptualization, Data curation, Formal analysis, Funding acquisition, Investigation, Methodology, Project administration, Supervision, Validation, Writing – original draft, Writing – review & editing. HP: Investigation, Supervision, Writing – review & editing. JG: Investigation, Supervision, Writing – review & editing. RH: Conceptualization, Formal analysis, Supervision, Writing – review & editing. KK: Conceptualization, Data curation, Formal analysis, Supervision, Writing – review & editing. DY: Conceptualization, Supervision, Writing – review & editing.
Funding
The author(s) declare financial support was received for the research, authorship, and/or publication of this article. This research was supported by a grant of the Korea Health Technology R&D Project through the Korea Health Industry Development Institute (KHIDI), funded by the Ministry of Health and Welfare, Republic of Korea (Grant Number: HR18C0016) and National Research Foundation of Korea (NRF) grants funded by the Korea Government (Grant Number: 2021R1A2B5B03001891).
Conflict of interest
RH received research support from Agios Pharmaceuticals/Bristol-Myers Squibb and consultation fees from Nuvation Bio and served on the advisory board of Vysioneer. DY received consultation fees from Abclone and GI cell and served on the advisory board of Abclone, GC cell, and GI cell.
The remaining authors declare that the research was conducted in the absence of any commercial or financial relationships that could be construed as a potential conflict of interest.
Publisher's note
All claims expressed in this article are solely those of the authors and do not necessarily represent those of their affiliated organizations, or those of the publisher, the editors and the reviewers. Any product that may be evaluated in this article, or claim that may be made by its manufacturer, is not guaranteed or endorsed by the publisher.
Supplementary material
The Supplementary Material for this article can be found online at: https://www.frontiersin.org/articles/10.3389/fneur.2024.1392831/full#supplementary-material
References
1. Abramson JS, Palomba ML, Gordon LI, Lunning MA, Wang M, Arnason J, et al. Lisocabtagene maraleucel for patients with relapsed or refractory large B-cell lymphomas (TRANSCEND NHL 001): a multicentre seamless design study. Lancet. (2020) 396:839–52. doi: 10.1016/S0140-6736(20)31366-0
2. Han D, Xu Z, Zhuang Y, Ye Z, Qian Q. Current progress in CAR-T cell therapy for hematological malignancies. J Cancer. (2021) 12:326. doi: 10.7150/jca.48976
3. Kasakovski D, Xu L, Li Y. T cell senescence and CAR-T cell exhaustion in hematological malignancies. J Hematol Oncol. (2018) 11:1–9. doi: 10.1186/s13045-018-0629-x
4. Maude SL, Laetsch TW, Buechner J, Rives S, Boyer M, Bittencourt H, et al. Tisagenlecleucel in children and young adults with B-cell lymphoblastic leukemia. N Engl J Med. (2018) 378:439–48. doi: 10.1056/NEJMoa1709866
5. Schuster SJ, Bishop MR, Tam CS, Waller EK, Borchmann P, McGuirk JP, et al. Tisagenlecleucel in adult relapsed or refractory diffuse large B-cell lymphoma. N Engl J Med. (2019) 380:45–56. doi: 10.1056/NEJMoa1804980
6. Locke FL, Ghobadi A, Jacobson CA, Miklos DB, Lekakis LJ, Oluwole OO, et al. Long-term safety and activity of axicabtagene ciloleucel in refractory large B-cell lymphoma (ZUMA-1): a single-arm, multicentre, phase 1-2 trial. Lancet Oncol. (2019) 20:31–42. doi: 10.1016/S1470-2045(18)30864-7
7. Holstein SA, Lunning MA. CAR T-cell therapy in hematologic malignancies: a voyage in progress. Clin Pharmacol Therapeut. (2020) 107:112–22. doi: 10.1002/cpt.1674
8. Zhao Z, Chen Y, Francisco NM, Zhang Y, Wu M. The application of CAR-T cell therapy in hematological malignancies: advantages and challenges. Acta Pharmaceutica Sinica B. (2018) 8:539–51. doi: 10.1016/j.apsb.2018.03.001
9. Yoon JG, Smith D, Tirumani SH, Caimi PF, Ramaiya N. CAR T-cell therapy: an update for radiologists. Am J Roentgenol. (2021) 21:26091. doi: 10.2214/AJR.21.26091
10. Brudno JN, Kochenderfer JN. Recent advances in CAR T-cell toxicity: mechanisms, manifestations and management. Blood Rev. (2019) 34:45–55. doi: 10.1016/j.blre.2018.11.002
11. Davila ML, Riviere I, Wang X, Bartido S, Park J, Curran K, et al. Efficacy and toxicity management of 19-28z CAR T cell therapy in B cell acute lymphoblastic leukemia. Sci Transl Med. (2014) 6:224ra25. doi: 10.1126/scitranslmed.3008226
12. Neelapu SS, Tummala S, Kebriaei P, Wierda W, Gutierrez C, Locke FL, et al. Chimeric antigen receptor T-cell therapy—assessment and management of toxicities. Nat Rev Clin Oncol. (2018) 15:47–62. doi: 10.1038/nrclinonc.2017.148
13. Schubert ML, Schmitt M, Wang L, Ramos CA, Jordan K, Müller-Tidow C, et al. Side-effect management of chimeric antigen receptor (CAR) T-cell therapy. Ann Oncol. (2021) 32:34–48. doi: 10.1016/j.annonc.2020.10.478
14. Park JH, Romero FA, Taur Y, Sadelain M, Brentjens RJ, Hohl TM, et al. Cytokine release syndrome grade as a predictive marker for infections in patients with relapsed or refractory B-cell acute lymphoblastic leukemia treated with chimeric antigen receptor T cells. Clin Infect Dis. (2018) 67:533–40. doi: 10.1093/cid/ciy152
15. Sang W, Shi M, Yang J, Cao J, Xu L, Yan D, et al. Phase II trial of co-administration of CD19- and CD20-targeted chimeric antigen receptor T cells for relapsed and refractory diffuse large B cell lymphoma. Cancer Med. (2020) 9:5827–38. doi: 10.1002/cam4.3259
16. Shah BD, Bishop MR, Oluwole OO, Logan AC, Baer MR, Donnellan WB, et al. KTE-X19 anti-CD19 CAR T-cell therapy in adult relapsed/refractory acute lymphoblastic leukemia: ZUMA-3 phase 1 results. Blood. (2021) 138:11–22. doi: 10.1182/blood.2020009098
17. Shah BD, Ghobadi A, Oluwole OO, Logan AC, Boissel N, Cassaday RD, et al. KTE-X19 for relapsed or refractory adult B-cell acute lymphoblastic leukaemia: phase 2 results of the single-arm, open-label, multicentre ZUMA-3 study. Lancet. (2021) 21:8. doi: 10.1016/S0140-6736(21)01222-8
18. Shah NN, Highfill SL, Shalabi H, Yates B, Jin J, Wolters PL, et al. CD4/CD8 T-cell selection affects chimeric antigen receptor (CAR) T-cell potency and toxicity: updated results from a phase I anti-CD22 CAR T-cell trial. J Clin Oncol. (2020) 38:1938–50. doi: 10.1200/JCO.19.03279
19. Shah NN, Johnson BD, Schneider D, Zhu F, Szabo A, Keever-Taylor CA, et al. Bispecific anti-CD20, anti-CD19 CAR T cells for relapsed B cell malignancies: a phase 1 dose escalation and expansion trial. Nat Med. (2020) 26:1569–75. doi: 10.1038/s41591-020-1081-3
20. Wang D, Wang J, Hu G, Wang W, Xiao Y, Cai H, et al. A phase 1 study of a novel fully human BCMA-targeting CAR (CT103A) in patients with relapsed/refractory multiple myeloma. Blood. (2021) 137:2890–901. doi: 10.1182/blood.2020008936
21. Page MJ, McKenzie JE, Bossuyt PM, Boutron I, Hoffmann TC, Mulrow CD, et al. The PRISMA 2020 statement: an updated guideline for reporting systematic reviews. Br Med J. (2021) 372:n71. doi: 10.1136/bmj.n71
22. Park HY, Suh CH, Woo S, Kim PH, Kim KW. Quality reporting of systematic review and meta-analysis according to PRISMA 2020 guidelines: results from recently published papers in the Korean Journal of Radiology. Korean J Radiol. (2022) 23:355–69. doi: 10.3348/kjr.2021.0808
23. Higgins J, Green S. Cochrane Handbook for Systematic Reviews of Interventions Version 5.1.0. The Cochrane Collaboration. Available at: http://handbookcochraneorg/chapter (accessed October 2, 2017).
24. Guyatt GH, Oxman AD, Vist GE, Kunz R, Falck-Ytter Y, Alonso-Coello P, et al. GRADE: an emerging consensus on rating quality of evidence and strength of recommendations. BMJ. (2008) 336:924–6.
25. Higgins JP, Thomas J, Chandler J, Cumpston M, Li T, Page MJ, et al. Cochrane Handbook for Systematic Reviews of Interventions. Hoboken, NJ: John Wiley & Sons (2019).
26. Egger M, Smith GD, Schneider M, Minder CJB. Bias in meta-analysis detected by a simple, graphical test. Br Med J. (1997) 315:629–34. doi: 10.1136/bmj.315.7109.629
27. Haugen BR, Alexander EK, Bible KC, Doherty GM, Mandel SJ, Nikiforov YE, et al. 2015 American thyroid association management guidelines for adult patients with thyroid nodules and differentiated thyroid cancer: the American thyroid association guidelines task force on thyroid nodules and differentiated thyroid cancer. Thyroid. (2016) 26:1–133. doi: 10.1089/thy.2015.0020
28. Spittal MJ, Pirkis J, Gurrin LC. Meta-analysis of incidence rate data in the presence of zero events. BMC Med Res Methodol. (2015) 15:42. doi: 10.1186/s12874-015-0031-0
29. Viechtbauer W. Conducting meta-analyses in R with the metafor package. J Stat Softw. (2010) 36:1–48. doi: 10.18637/jss.v036.i03
30. Baumeister SH, Murad J, Werner L, Daley H, Trebeden-Negre H, Gicobi JK, et al. Phase I trial of autologous CAR T cells targeting NKG2D ligands in patients with AML/MDS and multiple myeloma. Cancer Immunol Res. (2019) 7:100–12. doi: 10.1158/2326-6066.CIR-18-0307
31. Benjamin R, Graham C, Yallop D, Jozwik A, Mirci-Danicar OC, Lucchini G, et al. Genome-edited, donor-derived allogeneic anti-CD19 chimeric antigen receptor T cells in paediatric and adult B-cell acute lymphoblastic leukaemia: results of two phase 1 studies. Lancet. (2020) 396:1885–94. doi: 10.1016/S0140-6736(20)32334-5
32. Berdeja JG, Madduri D, Usmani SZ, Jakubowiak A, Agha M, Cohen AD, et al. Ciltacabtagene autoleucel, a B-cell maturation antigen-directed chimeric antigen receptor T-cell therapy in patients with relapsed or refractory multiple myeloma (CARTITUDE-1): a phase 1b/2 open-label study. Lancet. (2021) 398:314–24. doi: 10.1016/S0140-6736(21)00933-8
33. Cao J, Wang G, Cheng H, Wei C, Qi K, Sang W, et al. Potent anti-leukemia activities of humanized CD19-targeted Chimeric antigen receptor T (CAR-T) cells in patients with relapsed/refractory acute lymphoblastic leukemia. Am J Hematol. (2018) 93:851–8. doi: 10.1002/ajh.25108
34. Cohen AD, Garfall AL, Stadtmauer EA, Melenhorst JJ, Lacey SF, Lancaster E, et al. B cell maturation antigen-specific CAR T cells are clinically active in multiple myeloma. J Clin Invest. (2019) 129:2210–21. doi: 10.1172/JCI126397
35. Curran KJ, Margossian SP, Kernan NA, Silverman LB, Williams DA, Shukla N, et al. Toxicity and response after CD19-specific CAR T-cell therapy in pediatric/young adult relapsed/refractory B-ALL. Blood. (2019) 134:2361–8. doi: 10.1182/blood.2019001641
36. Geyer MB, Rivière I, Sénéchal B, Wang X, Wang Y, Purdon TJ, et al. Safety and tolerability of conditioning chemotherapy followed by CD19-targeted CAR T cells for relapsed/refractory CLL. JCI Insight. (2019) 5:122627. doi: 10.1172/jci.insight.122627
37. Heng G, Jia J, Li S, Fu G, Wang M, Qin D, et al. Sustained therapeutic efficacy of humanized anti-CD19 chimeric antigen receptor T cells in relapsed/refractory acute lymphoblastic leukemia. Clin Cancer Res. (2020) 26:1606–15. doi: 10.1158/1078-0432.CCR-19-1339
38. Jacoby E, Bielorai B, Avigdor A, Itzhaki O, Hutt D, Nussboim V, et al. Locally produced CD19 CAR T cells leading to clinical remissions in medullary and extramedullary relapsed acute lymphoblastic leukemia. Am J Hematol. (2018) 93:1485–92. doi: 10.1002/ajh.25274
39. Li C, Cao W, Que Y, Wang Q, Xiao Y, Gu C, et al. A phase I study of anti-BCMA CAR T cell therapy in relapsed/refractory multiple myeloma and plasma cell leukemia. Clin Transl Med. (2021) 11:e346. doi: 10.1002/ctm2.346
40. Ma F, Ho JY, Du H, Xuan F, Wu X, Wang Q, et al. Evidence of long-lasting anti-CD19 activity of engrafted CD19 chimeric antigen receptor-modified T cells in a phase I study targeting pediatrics with acute lymphoblastic leukemia. Hematol Oncol. (2019) 37:601–8. doi: 10.1002/hon.2672
41. Magnani CF, Gaipa G, Lussana F, Belotti D, Gritti G, Napolitano S, et al. Sleeping beauty-engineered CAR T cells achieve antileukemic activity without severe toxicities. J Clin Invest. (2020) 130:6021–33. doi: 10.1172/JCI138473
42. Munshi NC, Anderson LD Jr, Shah N, Madduri D, Berdeja J, Lonial S, et al. Idecabtagene vicleucel in relapsed and refractory multiple myeloma. N Engl J Med. (2021) 384:705–16. doi: 10.1056/NEJMoa2024850
43. Neelapu SS, Locke FL, Bartlett NL, Lekakis LJ, Miklos DB, Jacobson CA, et al. Axicabtagene ciloleucel CAR T-cell therapy in refractory large B-cell lymphoma. N Engl J Med. (2017) 377:2531–44. doi: 10.1056/NEJMoa1707447
44. Pan J, Niu Q, Deng B, Liu S, Wu T, Gao Z, et al. CD22 CAR T-cell therapy in refractory or relapsed B acute lymphoblastic leukemia. Leukemia. (2019) 33:2854–66. doi: 10.1038/s41375-019-0488-7
45. Raje N, Berdeja J, Lin Y, Siegel D, Jagannath S, Madduri D, et al. Anti-BCMA CAR T-cell therapy bb2121 in relapsed or refractory multiple myeloma. N Engl J Med. (2019) 380:1726–37. doi: 10.1056/NEJMoa1817226
46. Ramos CA, Grover NS, Beaven AW, Lulla PD, Wu MF, Ivanova A, et al. Anti-CD30 CAR-T cell therapy in relapsed and refractory hodgkin lymphoma. J Clin Oncol. (2020) 38:3794–804. doi: 10.1200/JCO.20.01342
47. Sauter CS, Senechal B, Rivière I, Ni A, Bernal Y, Wang X, et al. CD19 CAR T cells following autologous transplantation in poor-risk relapsed and refractory B-cell non-Hodgkin lymphoma. Blood. (2019) 134:626–35. doi: 10.1182/blood.2018883421
48. Turtle CJ, Hanafi LA, Berger C, Gooley TA, Cherian S, Hudecek M, et al. CD19 CAR-T cells of defined CD4+:CD8+ composition in adult B cell ALL patients. J Clin Invest. (2016) 126:2123–38. doi: 10.1172/JCI85309
49. Yan ZX, Li L, Wang W, Ou Yang BS, Cheng S, Wang L, et al. Clinical efficacy and tumor microenvironment influence in a dose-escalation study of anti-CD19 chimeric antigen receptor T cells in refractory B-cell non-Hodgkin's lymphoma. Clin Cancer Res. (2019) 25:6995–7003. doi: 10.1158/1078-0432.CCR-19-0101
50. Ying Z, Huang XF, Xiang X, Liu Y, Kang X, Song Y, et al. A safe and potent anti-CD19 CAR T cell therapy. Nat Med. (2019) 25:947–53. doi: 10.1038/s41591-019-0421-7
51. Zhang Y, Li J, Lou X, Chen X, Yu Z, Kang L, et al. A prospective investigation of bispecific CD19/22 CAR T cell therapy in patients with relapsed or refractory B cell non-Hodgkin lymphoma. Front Oncol. (2021) 11:664421. doi: 10.3389/fonc.2021.664421
52. Zhao WH, Liu J, Wang BY, Chen YX, Cao XM, Yang Y, et al. A phase 1, open-label study of LCAR-B38M, a chimeric antigen receptor T cell therapy directed against B cell maturation antigen, in patients with relapsed or refractory multiple myeloma. J Hematol Oncol. (2018) 11:141. doi: 10.1186/s13045-018-0681-6
53. Zhou X, Tu S, Wang C, Huang R, Deng L, Song C, et al. Phase I trial of fourth-generation anti-CD19 chimeric antigen receptor T cells against relapsed or refractory B cell non-Hodgkin lymphomas. Front Immunol. (2020) 11:564099. doi: 10.3389/fimmu.2020.564099
54. An F, Wang H, Liu Z, Wu F, Zhang J, Tao Q, et al. Influence of patient characteristics on chimeric antigen receptor T cell therapy in B-cell acute lymphoblastic leukemia. Nat Commun. (2020) 11:5928. doi: 10.1038/s41467-020-19774-x
55. Brudno JN, Lam N, Vanasse D, Shen YW, Rose JJ, Rossi J, et al. Safety and feasibility of anti-CD19 CAR T cells with fully human binding domains in patients with B-cell lymphoma. Nat Med. (2020) 26:270–80. doi: 10.1038/s41591-019-0737-3
56. Brudno JN, Maric I, Hartman SD, Rose JJ, Wang M, Lam N, et al. T cells genetically modified to express an anti-B-cell maturation antigen chimeric antigen receptor cause remissions of poor-prognosis relapsed multiple myeloma. J Clin Oncol. (2018) 36:2267–80. doi: 10.1200/JCO.2018.77.8084
57. Frey NV, Gill S, Hexner EO, Schuster S, Nasta S, Loren A, et al. Long-term outcomes from a randomized dose optimization study of chimeric antigen receptor modified T cells in relapsed chronic lymphocytic leukemia. J Clin Oncol. (2020) 38:2862–71. doi: 10.1200/JCO.19.03237
58. Gardner RA, Finney O, Annesley C, Brakke H, Summers C, Leger K, et al. Intent-to-treat leukemia remission by CD19 CAR T cells of defined formulation and dose in children and young adults. Blood. (2017) 129:3322–31. doi: 10.1182/blood-2017-02-769208
59. Kochenderfer JN, Somerville RPT, Lu T, Shi V, Bot A, Rossi J, et al. Lymphoma remissions caused by anti-CD19 chimeric antigen receptor T cells are associated with high serum interleukin-15 levels. J Clin Oncol. (2017) 35:1803–13. doi: 10.1200/JCO.2016.71.3024
60. Liu H, Lei W, Zhang C, Yang C, Wei J, Guo Q, et al. CD19-specific CAR T cells that express a PD-1/CD28 chimeric switch-receptor are effective in patients with PD-L1-positive B-cell lymphoma. Clin Cancer Res. (2021) 27:473–84. doi: 10.1158/1078-0432.CCR-20-1457
61. Myers RM, Li Y, Barz Leahy A, Barrett DM, Teachey DT, Callahan C, et al. Humanized CD19-targeted chimeric antigen receptor (CAR) T cells in CAR-naive and CAR-exposed children and young adults with relapsed or refractory acute lymphoblastic leukemia. J Clin Oncol. (2021) 39:3044–55. doi: 10.1200/JCO.20.03458
62. Ramos CA, Rouce R, Robertson CS, Reyna A, Narala N, Vyas G, et al. In vivo fate and activity of second- versus third-generation CD19-specific CAR-T cells in B cell non-Hodgkin's lymphomas. Mol Ther. (2018) 26:2727–37. doi: 10.1016/j.ymthe.2018.09.009
63. Singh H, Srour SA, Milton DR, McCarty J, Dai C, Gaballa MR, et al. Sleeping beauty generated CD19 CAR T-cell therapy for advanced B-Cell hematological malignancies. Front Immunol. (2022) 13:1032397. doi: 10.3389/fimmu.2022.1032397
64. Spiegel JY, Patel S, Muffly L, Hossain NM, Oak J, Baird JH, et al. CAR T cells with dual targeting of CD19 and CD22 in adult patients with recurrent or refractory B cell malignancies: a phase 1 trial. Nat Med. (2021) 27:1419–31. doi: 10.1038/s41591-021-01436-0
65. Tong C, Zhang Y, Liu Y, Ji X, Zhang W, Guo Y, et al. Optimized tandem CD19/CD20 CAR-engineered T cells in refractory/relapsed B-cell lymphoma. Blood. (2020) 136:1632–44. doi: 10.1182/blood.2020005278
66. Wang J, Zhang X, Zhou Z, Liu Y, Yu L, Jia L, et al. A novel adoptive synthetic TCR and antigen receptor (STAR) T-Cell therapy for B-Cell acute lymphoblastic leukemia. Am J Hematol. (2022) 97:992–1004. doi: 10.1002/ajh.26586
67. Wang Y, Cao J, Gu W, Shi M, Lan J, Yan Z, et al. Long-term follow-up of combination of B-cell maturation antigen and CD19 chimeric antigen receptor T cells in multiple myeloma. J Clin Oncol. (2022) 40:2246–56. doi: 10.1200/JCO.21.01676
68. Ying Z, He T, Jin S, Wang X, Zheng W, Lin N, et al. A durable 4-1BB-based CD19 CAR-T cell for treatment of relapsed or refractory non-Hodgkin lymphoma. Chin J Cancer Res. (2022) 34:53–62. doi: 10.21147/j.issn.1000-9604.2022.01.05
69. Caimi PF, Pacheco Sanchez G, Sharma A, Otegbeye F, Ahmed N, Rojas P, et al. Prophylactic tocilizumab prior to anti-CD19 CAR-T cell therapy for non-Hodgkin lymphoma. Front Immunol. (2021) 12:745320. doi: 10.3389/fimmu.2021.745320
70. Cordoba S, Onuoha S, Thomas S, Pignataro DS, Hough R, Ghorashian S, et al. CAR T cells with dual targeting of CD19 and CD22 in pediatric and young adult patients with relapsed or refractory B cell acute lymphoblastic leukemia: a phase 1 trial. Nat Med. (2021) 27:1797–805. doi: 10.1038/s41591-021-01497-1
71. Cornell RF, Bishop MR, Kumar S, Giralt SA, Nooka AK, Larson SM, et al. A phase 1, multicenter study evaluating the safety and efficacy of KITE-585, an autologous anti-BCMA CAR T-cell therapy, in patients with relapsed/refractory multiple myeloma. Am J Cancer Res. (2021) 11:3285–93.
72. Fan L, Wang L, Cao L, Zhu H, Xu W, Li J. Phase I study of CBMCD19 chimeric antigen receptor T cell in the treatment of refractory diffuse large B-cell lymphoma in Chinese patients. Front Med. (2022) 16:285–94. doi: 10.1007/s11684-021-0843-8
73. Mei H, Li C, Jiang H, Zhao X, Huang Z, Jin D, et al. A bispecific CAR-T cell therapy targeting BCMA and CD38 in relapsed or refractory multiple myeloma. J Hematol Oncol. (2021) 14:161. doi: 10.1186/s13045-021-01170-7
74. Ortíz-Maldonado V, Rives S, Castellà M, Alonso-Saladrigues A, Benítez-Ribas D, Caballero-Baños M, et al. CART19-BE-01: a multicenter trial of ARI-0001 cell therapy in patients with CD19(+) relapsed/refractory malignancies. Mol Ther. (2021) 29:636–44. doi: 10.1016/j.ymthe.2020.09.027
75. Roddie C, Dias J, O'Reilly MA, Abbasian M, Cadinanos-Garai A, Vispute K, et al. Durable responses and low toxicity after fast off-rate CD19 chimeric antigen receptor-T therapy in adults with relapsed or refractory B-cell acute lymphoblastic leukemia. J Clin Oncol. (2021) 39:3352–63. doi: 10.1200/JCO.21.00917
76. Shalabi H, Martin S, Yates B, Wolters PL, Kaplan C, Smith H, et al. Neurotoxicity following CD19/CD28ζ CAR T-cells in children and young adults with B-cell malignancies. Neuro Oncol. (2022) 2022:noac034. doi: 10.1093/neuonc/noac034
77. Siddiqi T, Soumerai JD, Dorritie KA, Stephens DM, Riedell PA, Arnason J, et al. Phase 1 TRANSCEND CLL 004 study of lisocabtagene maraleucel in patients with relapsed/refractory CLL or SLL. Blood. (2022) 139:1794–806. doi: 10.1182/blood.2021011895
78. Talleur A, Qudiemat A, Métais JY, Langfitt D, Mamcarz E, Crawford JC, et al. Preferential expansion of CD8+ CD19-CAR T cells postinfusion and the role of disease burden on outcome in pediatric B-ALL. Blood Adv. (2022) 2021:6293. doi: 10.1182/bloodadvances.2021006293
79. Wei G, Zhang Y, Zhao H, Wang Y, Liu Y, Liang B, et al. CD19/CD22 dual-targeted CAR T-cell therapy for relapsed/refractory aggressive B-cell lymphoma: a safety and efficacy study. Cancer Immunol Res. (2021) 9:1061–70. doi: 10.1158/2326-6066.CIR-20-0675
80. Pan J, Tan Y, Wang G, Deng B, Ling Z, Song W, et al. Donor-derived CD7 chimeric antigen receptor T cells for T-cell acute lymphoblastic leukemia: first-in-human, phase I trial. J Clin Oncol. (2021) 39:3340–51. doi: 10.1200/JCO.21.00389
81. Liu Y, Deng B, Hu B, Zhang W, Zhu Q, Liu Y, et al. Sequential different B-cell antigen-targeted CAR T-cell therapy for pediatric refractory/relapsed Burkitt lymphoma. Blood Adv. (2022) 6:717–30. doi: 10.1182/bloodadvances.2021004557
82. Fowler NH, Dickinson M, Dreyling M, Martinez-Lopez J, Kolstad A, Butler J, et al. Tisagenlecleucel in adult relapsed or refractory follicular lymphoma: the phase 2 ELARA trial. Nat Med. (2022) 28:325–32. doi: 10.1038/s41591-021-01622-0
83. Jacobson CA, Chavez JC, Sehgal AR, William BM, Munoz J, Salles G, et al. Axicabtagene ciloleucel in relapsed or refractory indolent non-Hodgkin lymphoma (ZUMA-5): a single-arm, multicentre, phase 2 trial. Lancet Oncol. (2022) 23:91–103. doi: 10.1016/S1470-2045(21)00591-X
84. Kato K, Makita S, Goto H, Kanda J, Fujii N, Shimada K, et al. Phase 2 study of axicabtagene ciloleucel in Japanese patients with relapsed or refractory large B-cell lymphoma. Int J Clin Oncol. (2022) 27:213–23. doi: 10.1007/s10147-021-02033-4
85. Makita S, Yamamoto G, Maruyama D, Asano-Mori Y, Kaji D, Ananthakrishnan R, et al. Phase 2 results of lisocabtagene maraleucel in Japanese patients with relapsed/refractory aggressive B-cell non-Hodgkin lymphoma. Cancer Med. (2022) 2022:4820. doi: 10.1002/cam4.4820
86. Neelapu SS, Dickinson M, Munoz J, Ulrickson ML, Thieblemont C, Oluwole OO, et al. Axicabtagene ciloleucel as first-line therapy in high-risk large B-cell lymphoma: the phase 2 ZUMA-12 trial. Nat Med. (2022) 28:735–42. doi: 10.1038/s41591-022-01731-4
87. Shi X, Yan L, Shang J, Kang L, Yan Z, Jin S, et al. Anti-CD19 and anti-BCMA CAR T cell therapy followed by lenalidomide maintenance after autologous stem-cell transplantation for high-risk newly diagnosed multiple myeloma. Am J Hematol. (2022) 97:537–47. doi: 10.1002/ajh.26486
88. Ying Z, Yang H, Guo Y, Li W, Zou D, Zhou D, et al. Relmacabtagene autoleucel (relma-cel) CD19 CAR-T therapy for adults with heavily pretreated relapsed/refractory large B-cell lymphoma in China. Cancer Med. (2021) 10:999–1011. doi: 10.1002/cam4.3686
89. Zhang H, Liu M, Xiao X, Lv H, Jiang Y, Li X, et al. A combination of humanized anti-BCMA and murine anti-CD38 CAR-T cell therapy in patients with relapsed or refractory multiple myeloma. Leuk Lymphoma. (2022) 63:1418–27. doi: 10.1080/10428194.2022.2030476
90. Wu J, Meng F, Cao Y, Zhang Y, Zhu X, Wang N, et al. Sequential CD19/22 CAR T-cell immunotherapy following autologous stem cell transplantation for central nervous system lymphoma. Blood Cancer J. (2021) 11:131. doi: 10.1038/s41408-021-00523-2
91. Wang M, Munoz J, Goy A, Locke FL, Jacobson CA, Hill BT, et al. KTE-X19 CAR T-cell therapy in relapsed or refractory mantle-cell lymphoma. N Engl J Med. (2020) 382:1331–42. doi: 10.1056/NEJMoa1914347
92. Locke FL, Miklos DB, Jacobson CA, Perales MA, Kersten MJ, Oluwole OO, et al. Axicabtagene ciloleucel as second-line therapy for large B-cell lymphoma. N Engl J Med. (2022) 386:640–54. doi: 10.1056/NEJMoa2116133
93. Zhang X, Lu XA, Yang J, Zhang G, Li J, Song L, et al. Efficacy and safety of anti-CD19 CAR T-cell therapy in 110 patients with B-cell acute lymphoblastic leukemia with high-risk features. Blood Adv. (2020) 4:2325–38. doi: 10.1182/bloodadvances.2020001466
94. Lamure S, Van Laethem F, De Verbizier D, Lozano C, Gehlkopf E, Tudesq JJ, et al. Clinical and product features associated with outcome of DLBCL patients to CD19-targeted CAR T-cell therapy. Cancers. (2021) 13:174279. doi: 10.3390/cancers13174279
95. Bethge WA, Martus P, Schmitt M, Holtick U, Subklewe M, von Tresckow B, et al. GLA/DRST real-world outcome analysis of CAR T-cell therapies for large B-cell lymphoma in Germany. Blood. (2022) 140:349–58. doi: 10.1182/blood.2021015209
96. Gauthier J, Gazeau N, Hirayama AV, Hill JA, Wu V, Cearley A, et al. Impact of CD19 CAR T-cell product type on outcomes in relapsed or refractory aggressive B-NHL. Blood. (2022) 139:3722–31. doi: 10.1182/blood.2021014497
97. Ghilardi G, Chong EA, Svoboda J, Wohlfarth P, Nasta SD, Williamson S, et al. Bendamustine is safe and effective for lymphodepletion before tisagenlecleucel in patients with refractory or relapsed large B-cell lymphomas. Ann Oncol. (2022) 5:521. doi: 10.1016/j.annonc.2022.05.521
98. Kuhnl A, Roddie C, Kirkwood AA, Tholouli E, Menne T, Patel A, et al. A national service for delivering CD19 CAR-Tin large B-cell lymphoma—the UK real-world experience. Br J Haematol. (2022) 198:492–502. doi: 10.1111/bjh.18209
99. Kwon M, Iacoboni G, Reguera JL, Corral LL, Morales RH, Ortiz-Maldonado V, et al. Axicabtagene ciloleucel compared to tisagenlecleucel for the treatment of aggressive B-cell lymphoma. Haematologica. (2022) 2022:280805. doi: 10.3324/haematol.2022.280805
100. Nastoupil LJ, Jain MD, Feng L, Spiegel JY, Ghobadi A, Lin Y, et al. Standard-of-care axicabtagene ciloleucel for relapsed or refractory large B-cell lymphoma: results from the US lymphoma CAR T consortium. J Clin Oncol. (2020) 38:3119–28. doi: 10.1200/JCO.19.02104
101. Pasquini MC, Hu ZH, Curran K, Laetsch T, Locke F, Rouce R, et al. Real-world evidence of tisagenlecleucel for pediatric acute lymphoblastic leukemia and non-Hodgkin lymphoma. Blood Adv. (2020) 4:5414–24. doi: 10.1182/bloodadvances.2020003092
102. Riedell PA, Hwang WT, Nastoupil LJ, Pennisi M, McGuirk JP, Maziarz RT, et al. Patterns of use, outcomes, and resource utilization among recipients of commercial axicabtagene ciloleucel and tisagenlecleucel for relapsed/refractory aggressive B cell lymphomas. Transplant Cell Ther. (2022) 7:11. doi: 10.1016/j.jtct.2022.07.011
103. Rubin DB, Al Jarrah A, Li K, LaRose S, Monk AD, Ali AB, et al. Clinical predictors of neurotoxicity after chimeric antigen receptor T-cell therapy. J Am Med Assoc Neurol. (2020) 77:1536–42. doi: 10.1001/jamaneurol.2020.2703
104. Strati P, Nastoupil LJ, Westin J, Fayad LE, Ahmed S, Fowler NH, et al. Clinical and radiologic correlates of neurotoxicity after axicabtagene ciloleucel in large B-cell lymphoma. Blood Adv. (2020) 4:3943–51. doi: 10.1182/bloodadvances.2020002228
105. Zettler ME, Feinberg BA, Phillips EG Jr, Klink AJ, Mehta S, Gajra A. Real-world adverse events associated with CAR T-cell therapy among adults age ≥ 65 years. J Geriatr Oncol. (2021) 12:239–42. doi: 10.1016/j.jgo.2020.07.006
106. Bastos-Oreiro M, Gutierrez A, Reguera JL, Iacoboni G, López-Corral L, Terol MJ, et al. Best treatment option for patients with refractory aggressive B-cell lymphoma in the CAR-T cell era: real-world evidence from GELTAMO/GETH Spanish groups. Front Immunol. (2022) 13:855730. doi: 10.3389/fimmu.2022.855730
107. Gauthier J, Turtle CJ. Insights into cytokine release syndrome and neurotoxicity after CD19-specific CAR-T cell therapy. Curr Res Transl Med. (2018) 66:50–2. doi: 10.1016/j.retram.2018.03.003
108. Hunter BD, Jacobson CA. CAR T-cell associated neurotoxicity: mechanisms, clinicopathologic correlates, and future directions. J Natl Cancer Inst. (2019) 111:646–54. doi: 10.1093/jnci/djz017
109. Gust J, Hay KA, Hanafi LA, Li D, Myerson D, Gonzalez-Cuyar LF, et al. Endothelial activation and blood-brain barrier disruption in neurotoxicity after adoptive immunotherapy with CD19 CAR-T cells. Cancer Discov. (2017) 7:1404–19. doi: 10.1158/2159-8290.CD-17-0698
110. Van Oekelen O, Aleman A, Upadhyaya B, Schnakenberg S, Madduri D, Gavane S, et al. Neurocognitive and hypokinetic movement disorder with features of parkinsonism after BCMA-targeting CAR-T cell therapy. Nat Med. (2021) 27:2099–103. doi: 10.1038/s41591-021-01564-7
111. Parker KR, Migliorini D, Perkey E, Yost KE, Bhaduri A, Bagga P, et al. Single-cell analyses identify brain mural cells expressing CD19 as potential off-tumor targets for CAR-T immunotherapies. Cell. (2020) 183:126–42.e17. doi: 10.1016/j.cell.2020.08.022
112. Mohyuddin GR, Banerjee R, Alam Z, Berger KE, Chakraborty R. Rethinking mechanisms of neurotoxicity with BCMA directed therapy. Crit Rev Oncol Hematol. (2021) 166:103453. doi: 10.1016/j.critrevonc.2021.103453
113. Santomasso BD, Park JH, Salloum D, Riviere I, Flynn J, Mead E, et al. Clinical and biological correlates of neurotoxicity associated with CAR T-cell therapy in patients with B-cell acute lymphoblastic leukemia. Cancer Discov. (2018) 8:958–71. doi: 10.1158/2159-8290.CD-17-1319
114. Weinkove R, George P, Dasyam N, McLellan AD. Selecting costimulatory domains for chimeric antigen receptors: functional and clinical considerations. Clin Transl Immunol. (2019) 8:e1049. doi: 10.1002/cti2.1049
115. Salter AI, Ivey RG, Kennedy JJ, Voillet V, Rajan A, Alderman EJ, et al. Phosphoproteomic analysis of chimeric antigen receptor signaling reveals kinetic and quantitative differences that affect cell function. Sci Signal. (2018) 11:aat6753. doi: 10.1126/scisignal.aat6753
116. Sterner RC, Sterner RM. Immune effector cell associated neurotoxicity syndrome in chimeric antigen receptor-T cell therapy. Front Immunol. (2022) 13:879608. doi: 10.3389/fimmu.2022.879608
117. Belin C, Devic P, Ayrignac X, Dos Santos A, Paix A, Sirven-Villaros L, et al. Description of neurotoxicity in a series of patients treated with CAR T-cell therapy. Sci Rep. (2020) 10:1–9. doi: 10.1038/s41598-020-76055-9
Keywords: CAR T-cell, immunotherapy, immune effector cell-associated neurotoxicity syndrome, neurotoxicity, hematologic malignancies
Citation: Han MW, Jeong SY, Suh CH, Park H, Guenette JP, Huang RY, Kim KW and Yoon DH (2024) Incidence of immune effector cell-associated neurotoxicity among patients treated with CAR T-cell therapy for hematologic malignancies: systematic review and meta-analysis. Front. Neurol. 15:1392831. doi: 10.3389/fneur.2024.1392831
Received: 28 February 2024; Accepted: 23 September 2024;
Published: 15 October 2024.
Edited by:
Stella Bouziana, King's College Hospital NHS Foundation Trust, United KingdomReviewed by:
Tao Liu, Tianjin Medical University General Hospital, ChinaJakrawadee Julamanee, Prince of Songkla University, Thailand
Copyright © 2024 Han, Jeong, Suh, Park, Guenette, Huang, Kim and Yoon. This is an open-access article distributed under the terms of the Creative Commons Attribution License (CC BY). The use, distribution or reproduction in other forums is permitted, provided the original author(s) and the copyright owner(s) are credited and that the original publication in this journal is cited, in accordance with accepted academic practice. No use, distribution or reproduction is permitted which does not comply with these terms.
*Correspondence: Chong Hyun Suh, Y2hvbmdoeXVuc3VoJiN4MDAwNDA7YW1jLnNlb3VsLmty
†These authors have contributed equally to this work and share first authorship