- Department of Laboratory Medicine, West China Hospital of Sichuan University, Chengdu, China
Background and aim: Invasion of the central nervous system by Treponema pallidum can occur at any stage of syphilis. In the event that T. pallidum is not cleared promptly, certain individuals may experience progression to neurosyphilis, which manifests as cognitive and behavioral abnormalities, limb paralysis, and potentially fatal outcomes. Early identification or prevention of neurosyphilis is therefore crucial. The aim of this paper is to conduct a critical and narrative review of the latest information focusing exclusively to the pathogenesis and clinical management of neurosyphilis.
Methodology: To compile this review, we have conducted electronic literature searches from the PubMed database relating to neurosyphilis. Priority was given to studies published from the past 10 years (from 2013 to 2023) and other studies if they were of significant importance (from 1985 to 2012), including whole genome sequencing results, cell structure of T. pallidum, history of genotyping, and other related topics. These studies are classic or reflect a developmental process.
Results: Neurosyphilis has garnered global attention, yet susceptibility to and the pathogenesis of this condition remain under investigation. Cerebrospinal fluid examination plays an important role in the diagnosis of neurosyphilis, but lacks the gold standard. Intravenous aqueous crystalline penicillin G continues to be the recommended therapeutic approach for neurosyphilis. Considering its sustained prominence, it is imperative to develop novel public health tactics in order to manage the resurgence of neurosyphilis.
Conclusion: This review gives an updated narrative description of neurosyphilis with special emphasis on its pathogenesis, susceptibility, diagnosis, treatment, and prevention.
Introduction
Neurosyphilis (NS) is a neurological infection caused by the spirochete Treponema pallidum. T. pallidum can affect the central nervous system (CNS) during any stage of syphilis (1). Estimates from the WHO indicate that approximately 22.3 million individuals worldwide had T. pallidum infection, with 7.1 million new cases, in 2020 (2). However, most regions have not monitored neurosyphilis at the national level, and there are few studies reporting the incidence of neurosyphilis (3). As 1.2% to 1.8% of patients with early syphilis will develop neurosyphilis, there is persistent prevalence of neurosyphilis worldwide (4). In Europe, the estimated annual incidence of neurosyphilis varies from 0.16 to 2.1 per 100 000 adults; in Australia, the annual incidence of neurosyphilis from 2007 to 2016 was 2.47 cases per 10,0000 people; in the Canadian province of British Columbia, the incidence of neurosyphilis increased from 0.03 cases per 10,0000 people in 1992 to 0.8 cases per 10,0000 people in 2012; in Guangdong province of China, the incidence of neurosyphilis increased from 0.21 cases per 10,0000 people in 2009 to 0.31 cases per 10,0000 people in 2014 (5–8). In high-income countries, the prevalence of syphilis is extremely high among men; in low-income and middle-income countries, syphilis is prevalent among the general population; WHO estimates that the prevalence of syphilis in Africa was the highest in 2016, which may be related to insufficient health education, human and infrastructural resources (9–11). It is speculated that similar patterns also exist in patients with neurosyphilis (10). Neurosyphilis not only imposes a great economic burden on patients but also causes a decline in quality of life due to the stigma associated with the disease (12).
As pathogenic treponemes, yaws and syphilis treponemes exhibit genetic similarity of more than 99.8% in their genome (13). However, yaws are unlikely to affect the CNS, suggesting that certain small genetic changes might be responsible for the differences in pathogenesis among these organisms (13). Exploring the genomes, neuroinvasion properties, and transmission routes of T. pallidum is essential for comprehending the pathogenesis of neurosyphilis and developing effective clinical strategies. Benzathine penicillin G, the preferred drug for treating primary syphilis, secondary syphilis, and tertiary syphilis patients with normal cerebrospinal fluid (CSF) examination, cannot reach an effective concentration in the CSF (14). Thus, identifying syphilis patients at a high risk of developing neurosyphilis would allow for targeted intervention. While the exact pathogenesis is not completely understood, certain factors, such as genetic susceptibility, for neurosyphilis have been identified (15). Despite this, numerous clinical and preclinical questions remain unanswered due to the complexities of real-world practice. This review describes the strain factors and mechanisms behind the neuroinvasion property of T. pallidum, as well as risk factors, predictors, diagnosis, treatment and prevention of neurosyphilis. Additionally, it addresses existing controversies and provides a discussion on future prospects.
To compile this review, we have conducted electronic literature searches from the PubMed database relating to neurosyphilis. Priority was given to studies published from the past 10 years (from 2013 to 2023) and other studies if they were of significant importance (from 1985 to 2012), including whole genome sequencing results, cell structure of T. pallidum, history of genotyping, and other related topics. These studies are classic or reflect a developmental process.
The biological basis of T. pallidum
In contrast to gram-negative bacteria, T. pallidum has a helical shape with a fragile dual-membrane structure and peptidoglycan layer, but lacks lipopolysaccharides (LPS) (Figure 1) (16). The flagella motors are fixed in the cytoplasmic membrane and arranged in a row, allowing the flagella filaments to extend into the periplasmic space between the two membranes (17). T. pallidum can swim by rolling or undulation of the cell body driven by the rotation of periplasmic flagella and it is the existence of periplasmic flagella maintains its helical shape (18). The cytoplasmic filaments anchored to the inner surface of the cytoplasmic membrane are arranged in a ribbon configuration (17). The cone-shaped structure locates at the end of spirochetes outside of the peptidoglycan layer (17). However, the exact role of cytoplasmic filaments and cone-shaped structure remains unknown (17). The defining characteristic of T. pallidum is the low density of membrane-spanning proteins and sufficient lipoproteins, which may reflect its capacity for host immune response evasion (19).
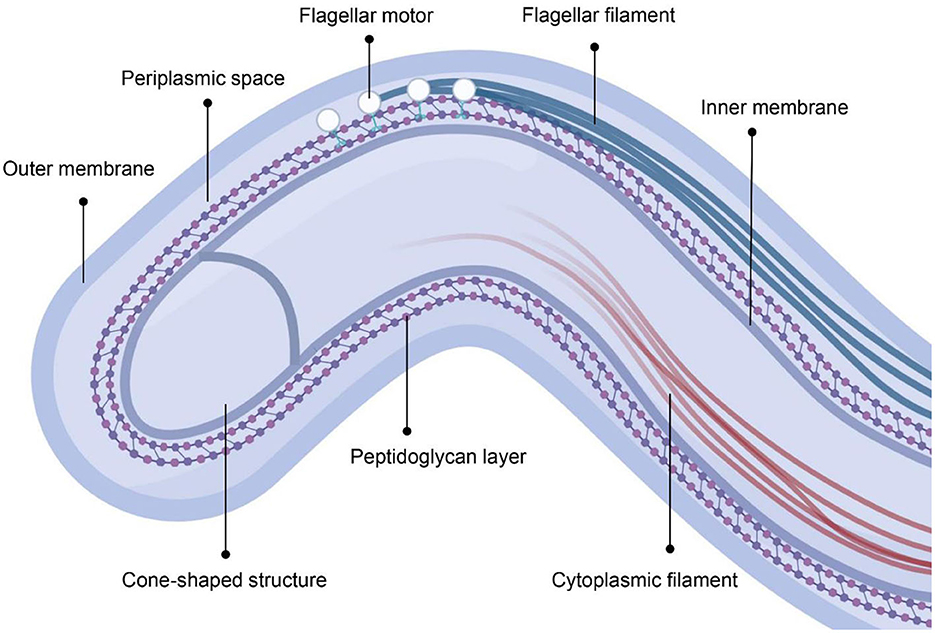
Figure 1. The cellular architecture of T. pallidum. The figure was created in BioRender.com.
The mechanisms of invasion of the CNS by T. pallidum
Current research suggests that T. pallidum does not release toxins into the host, but is one of the most invasive spirochetes (20). It has been demonstrated in rabbit models, mouse models, and human subjects that T. pallidum is capable of invading the CNS early in the disease (21). Pham et al. found T. pallidum in brain tissue, which was confirmed by 16S ribosomal RNA sequencing (22). Additionally, metagenomic next-generation sequencing (mNGS) detected T. pallidum nucleic acids in the CSF at low levels (23). These studies provide substantive evidence for the neuroinvasive capacity of T. pallidum.
The presence of T. pallidum in the CNS suggests its ability to evade the immune system and actively invade the CNS, leading to sustained damage. Macrophages are critical immune cells, especially microglia in the CNS, acting as the first line of immune defense (24). A case report of neurosyphilis observed severe proliferation of microglia in the cerebral cortex (25). CSF sTREM2 (a biomarker of microglial activation) was observed to be at significantly higher levels in neurosyphilis patients (26). Further study revealed that T. pallidum promotes microglial apoptosis and inhibits microglial migration as a means of evading clearance (27, 28). The balance between phagocytic uptake and T. pallidum evasion is influenced by production of opsonic antibodies, with TP0326 (BamA) and TP0751 (pallilysin) being identified as opsonic targets (29, 30). In addition, Tp47 can activate the NLPR2 inflammasomes in macrophages through PKM3 dependent glycolysis, thereby mediating the infection of T. pallidum (31).
T. pallidum exhibits various effects on host vascular endothelial cells, potentially linked to its invasion of the CNS (32) (shown in Figure 2). Scanning electron microscopy revealed that T. pallidum can directly adhere to human brain microvascular endothelial cells in vitro (36). TP0751 shares the same endothelial receptor with other neurotropic pathogens, and thus mediates interactions with endothelial cells (37); TP0751 alters expression of tight junction proteins, influencing the permeability of the blood-brain barrier (BBB) by promoting bEnd3 cell apoptosis and IL-6 secretion (38). Similarly, recombinant TP0965 shows a higher level of endothelial permeability induction (39). Both intercellular junction pathways and lipid raft-mediated endocytosis mechanisms for traversing endothelial barriers were observed in T. pallidum, but without disruption of barrier permeability (35). The latter view seems more reliable due to the normal or only slightly increased quotient (Qalb) level in neurosyphilis patients in clinical practice (40). In addition to interacting with macrophages and endothelial cells, the biochemical system, antigenic variation system, and matrix metalloproteinases (MMPs)/tissue inhibitors of metalloproteinases (TIMPs) imbalance are involved in the pathogenesis of T. pallidum (41–43).
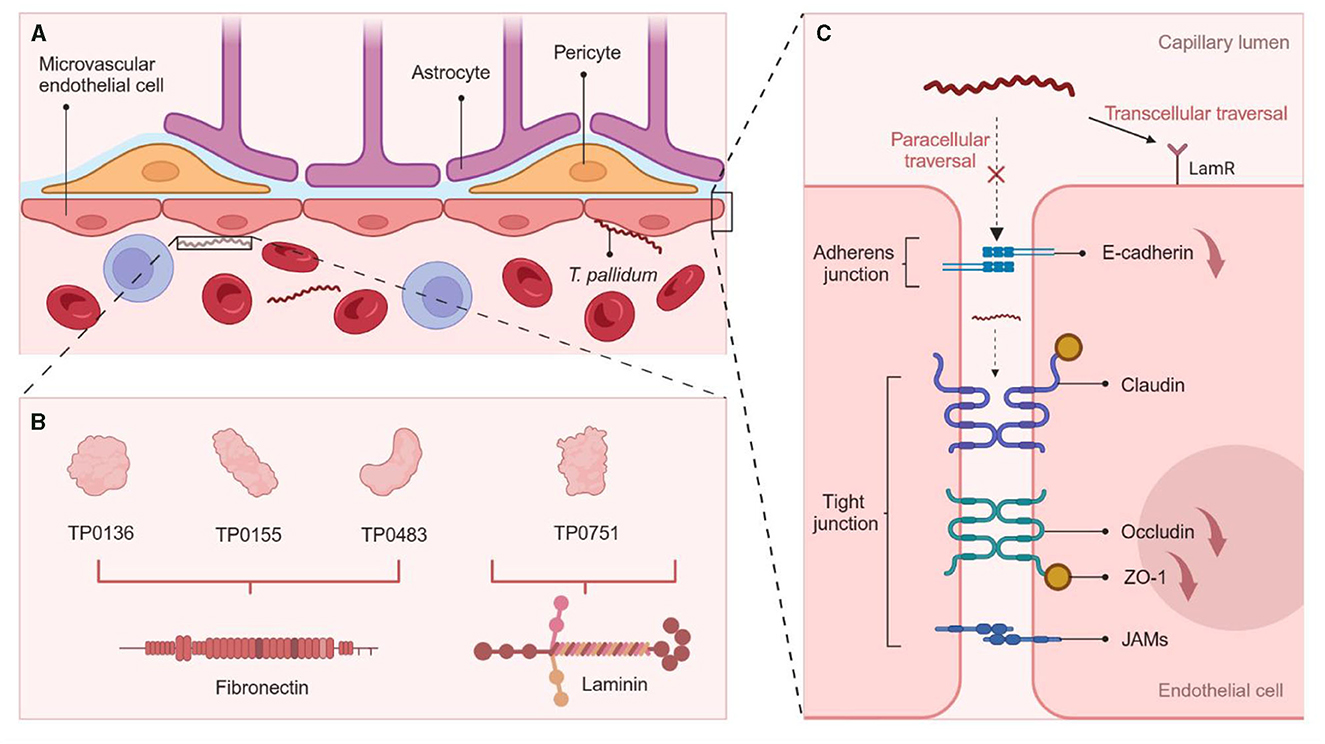
Figure 2. Mechanisms of T. pallidum for Crossing the BBB. (A) The BBB is composed of brain microvascular endothelial cells, astrocytes, and pericytes. Attaching to the host cells is the first step in T. pallidum neuroinvasion (33). (B) Several T. pallidum proteins are identified to be adhesins, including laminin- (TP0751) and fibronectin- (TP0136, TP0155, TP0483) binding proteins (34). (C) T. pallidum adheres to endothelial cells at the site of intercellular junction, resulting in a notable reduction in the expression of tight junction proteins ZO-1 and occludin through TP0751, and disrupting VE-cadherin, thereby entering the BBB through a paracellular pathway; TP0751 coordinates intercellular transport by engaging with host receptors (LamR) in lipid rafts and inducing endothelial uptake and transport in a cholesterol-dependent manner (35). The figure was created in BioRender.
Association of T. pallidum genome, genotypes and neurosyphilis
The T. pallidum genome comprises a circular chromosome with a total of 1041 predicted open reading frames (ORFs) (16). Two major T. pallidum lineages (Nichols and SS14) cocirculated across multiple continents, with a worldwide predominance of SS14 lineages, which may be explained by its resistance to macrolides (44). Whether this lineage is related to neurosyphilis has not been studied, but the genotype has and is discussed below. Notably, the genome exhibits a distinct set of repeat gene family sequences, particularly the tprK gene, which is involved in immune evasion and has potential as a latent vaccine (41). Furthermore, the genome displays a considerable number of motility-associated genes (e.g., FlaB1, FlaB2, FlaB3, FlaA, FliG), enabling efficient movement of T. pallidum in highly viscous environments such as connective tissues by traveling planar waves (16, 17). The periplasmic flagella and flexible peptidoglycan layer are the structural basis for achieving this movement (17).
Findings from the rabbit intravenous infection model have shown that certain strains of T. pallidum, such as Sea 81–4, exhibit a particular affinity for the CNS (45). This finding provides valuable clues regarding the association of strain typing and the neurosyphilis phenotype, and the potential microbiological mechanisms involved. The typing schemes frequently used in T. pallidum are CDC typing (CDCT), enhanced CDC typing (ECDCT), and multilocus sequence typing (MLST), based on analysis of restriction fragment length polymorphism (RFLP) or direct sequencing (46, 47). The loci detected in CDCT schemes include the acidic repeat protein (arp) gene and the T. pallidum repeat family genes [tprE (tp0313), tprG (tp0317), tprJ (tp0621)] (48). Compared to CDCT, ECDCT adds the sequence analysis of the tp0548 gene and has stronger discrimination against strains, while MLST focuses on the analysis of four genes (tp0136, tp0548, tp0705 and 23S rDNA genes) and further improves the typing resolution of SS14-like strains (48, 49). For ECDCT, consistent observations indicate a higher likelihood of neurosyphilis with strain type 14d/f (Table 1) (47, 48, 50, 51). Correspondingly, MLST type 1.1.2 appears to exhibit greater neuroinvasion (47). Except for South Africa, the majority of the CSF specimens (54%) inspected involved the 14a strain type, though subtype 14d was commonly found in genital ulcer specimens from syphilis patients during the same period (46). However, the nonuniform inclusion criteria of neurosyphilis patients and the lack of enough subjects limit the universality of these studies. Considering that strain type 14d/f is predominant among syphilis patients, the base effect linked to this subtype predisposing toward the development of neurosyphilis should be taken into account (52). A systematic review and meta-analysis concluded that CSF from patients with late neurosyphilis had low typing efficiency (46.4%) (53). The typing efficiency of lesion exudate was higher. However, it was difficult to collect from neurosyphilis patients (47). A more sensitive CSF typing method for T. pallidum deserves further investigation. Chen et al. developed a suite of PCR-LwCas13a syphilis assays with excellent sensitivity and specificity, which may be a promising alternative to genotyping (54).
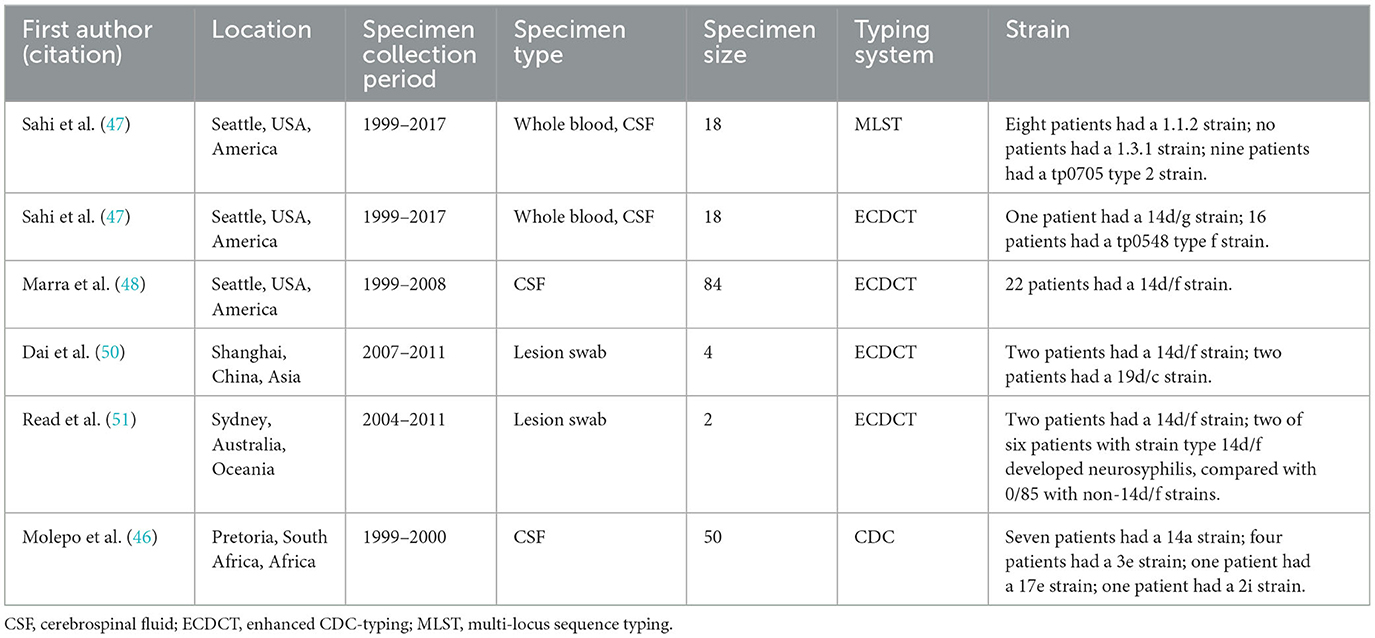
Table 1. Overview of six studies on molecular typing of T. pallidum strains from neurosyphilis patients.
Risk factors for neurosyphilis in HIV-negative patients
Sex and age
An increased prevalence of syphilis has been observed among men in China, the United States, and European countries, with a significant proportion of men having sex with men (MSM) (5, 55–57). For instance, in the United States, from 2008 to 2018, the estimated number of male patients with syphilis rose from 40,300 to 121,000 (55, 58). However, the prevalence of syphilis is not the highest in upper-middle-income and high-income countries (59). On the one hand, wealth can increase the opportunities for MSM to achieve syphilis by increasing regional mobility (59). On the other hand, the convenience of syphilis detection and treatment is convenient, which can reduce the spread of syphilis (59). The prevalence of syphilis depends on the balance of various situations. However, studies conducted in sub-Saharan Africa have shown that women have a higher prevalence of syphilis, which is related to the cultural, economic, and social marginalization of women in the region (60). Male sex has been identified as a correlating risk factor for neurosyphilis, in addition to its epidemiological significance (3, 61, 62). As we strive to better understand the pathophysiology of neurosyphilis, studies investigating the impact of sex hormones on disease development and progression may yield valuable insights (61).
Older age (≥45/60 years) is an independent risk factor for HIV-negative neurosyphilis patients (3, 62). The link involved may be attributable to the longer courses of disease in elderly individuals. Accordingly, aging is accompanied by progressive immunosenescence, which raises the possibility of infections (63). Interestingly, neurosyphilis has been found to correlated with certain neurodegenerative disorders, such as Alzheimer's disease (AD) (64). Pathological evidence has demonstrated that curly fibers discovered in AD correspond to individual spirochetes, and their aggregation in colonies produces similar senile plaques (65).
Serum non-treponemal test titer
Previous studies have indicated that non-treponemal tests, such as the rapid plasma reagin (RPR) test, and toluidine red unheated serum test (TRUST), have stronger specificity than treponemal tests in the diagnosis of neurosyphilis (66). This may be explained by the fact that blood-derived anti-treponemal IgG antibodies can cross through the BBB and enter the CSF, thus leading to false-positive results (67). A multivariate analysis revealed that the risk of developing neurosyphilis, but not secondary syphilis, increases with an elevation in serum RPR titer (3). Jiang et al. retrospectively found that HIV-negative syphilis patients with serum TRUST titers ≥1:16 were eight times more susceptible to developing neurosyphilis (68). Of note, patients with a fourfold decrease in serum RPR titers after treatment are more likely to develop asymptomatic neurosyphilis (ANS), indicating a correlation between ANS and treatment failure (69).
Specific genes carried by the host
Single-nucleotide polymorphisms (SNPs) of immune regulatory genes may influence susceptibility to neurosyphilis. The −1082 GG and −592 CC genotypes of the IL-10 promoter and the TLR1_1805GG, TLR2_2258GA, and TLR6_745CT/TT genotypes are associated with an increased risk of neurosyphilis (15, 70). At present, the majority of risk factors found in studies are immutable, posing challenges in intervening in risk factors to reduce disease risk. It is imperative to further explore factors such as psychosocial and physical activity factors to broaden our understanding of potential prevention.
Risk factors for neurosyphilis in HIV-positive patients
According to the European guideline on the management of syphilis, there is no increased risk of neurological involvement in HIV-infected patients with early syphilis (57). However, a study that did not distinguish syphilis stages has shown that syphilis patients with HIV infection are more likely to develop neurosyphilis (71). One possible explanation for this is that HIV-positive patients may be more inclined to undergo comprehensive and timely examinations. From a pathophysiological perspective, the CNS is a more immunologically privileged site due to the presence of the BBB and limited movement of immune cells, combined with the decrease in CD4 T cells and meningeal lesions in HIV-positive patients, which weakens the CNS's ability to defend against T. pallidum (72, 73).
In addition to male sex, advanced age, and high serological titers, HIV-positive individuals have several other risk factors regarding immune suppression. Numerous studies have reported an increased risk of neurosyphilis in patients with higher viral load and lower CD4(+) T cells count (< 350 cells/μl) compared to the general population (74, 75). T. pallidum itself can induce programmed cell death of CD4(+) and CD8(+) T cells with an increase in HIV-ribonucleic acid (RNA) viral load (76, 77). Actually, the synergism of HIV and T. pallidum is complicated and lacks sufficient research. It is well acknowledged that the characteristics of neurosyphilis can be confused with HIV infection, as primarily characterized by an increased karyocyte count and protein concentration (78). Antiretroviral therapy (ART) may ameliorate this status, but it is still unclear whether ART-naive patients with syphilis should undergo lumbar puncture (73). However, what is certain is that the absence of ART or syphilis treatment represents a significant risk factor for neurosyphilis (70, 74, 75).
Predictive indicators for neurosyphilis risks and treatment responses
Predictors can be roughly divided into two types: temporally advanced indicators and heterotopic predictive indicators. Current research on predicting the risk of neurosyphilis primarily focuses on the latter, particularly certain peripheral blood indicators. Li et al. constructed a prediction model incorporating age, serum TRUST titer, and various blood routine indicators, which offers a valuable reference for the empirical treatment of ANS (79). Serum neurofilament light chain (NfL) and the neutrophil-to-lymphocyte ratio (NLR), which serve as markers of neuroaxonal injury and inflammation, respectively, show promise as novel predictors for neurosyphilis (80, 81). Although diagnosis of neurosyphilis necessitates lumbar puncture, peripheral blood predictors can to some extent indicate changes in the CNS, thereby reducing the need for unnecessary invasive procedures (82). Additionally, applications of metabolomics, transcriptomics, mRNA modification, SNPs, and neuroimaging analysis in neurosyphilis have facilitated screening of additional biomarkers (15, 70, 83–86).
To accurately predict the effectiveness of treatment, temporally advanced indicators and heterotopic predictive indicators should both be explored. The serological response, namely, normalization or decrease of the serum RPR titer, can to some degree predict CSF normalization, and the rate of follow-up lumbar puncture may be reduced if neurological symptoms resolve (87, 88). As a fourfold decline in CSF RPR titer is a reliable predictor for treatment efficacy in CSF RPR-positive general paresis patients within 12 months after completing therapy, it is unnecessary to repeat CSF tests for HIV-negative people (14, 89). A recently developed minimal proteomic array not only allows for disease staging, but also monitors response to appropriate treatment, helping to confirm pharmacological cure (90). Furthermore, it is necessary to discuss factors like socioeconomic status, access to healthcare, and cultural influences which may contribute to the outcomes of neurosyphilis in the future. We should mention two conditions related to syphilis treatment: Jarisch-Herxheimer reaction (JHR) and serofast status. JHR is a transient inflammatory phenomenon observed in syphilis patients receiving antibiotic treatment (91). JHR cannot be predicted reliably, but it was observed that its frequency increases proportionally with white cell count and total protein level in CSF (92). The serofast state refers to a situation in which non-treponemal antibodies decline after treatment but fail to completely revert to a nonreactive state, which may be related to CNS infection (93, 94). Studies have shown that certain genetic factors, such as the strain genotype of 14i/a, host interleukin-10 promoter polymorphisms, differentially expressed cytokines and microRNAs, can predict increased risk of serofast status (94–97). However, regardless of whether it is a risk factor or predictive indicator, there remains uncertainty in the risk or prediction of disease occurrence, as it only represents a possibility with varying degrees of likelihood (98).
Diagnosis of neurosyphilis
The identification of neurosyphilis typically necessitates the integration of epidemiological information, neurologic or neuropsychiatric manifestations, serologic analysis of blood and CSF, and, in certain instances, imaging assessment. For syphilis patients with neurological symptoms, almost all major guidelines recommend lumbar puncture (99–101). Nonetheless, the neurological symptoms of neurosyphilis are not specific. Early neurosyphilis can affect the meninges and central blood vessels, including syphilitic meningitis, meningovascular neurosyphilis and syphilitic gummas, often manifested as headache, nausea, vomiting, blurred consciousness, and neck stiffness; late neurosyphilis affects the spinal cord and brain parenchyma, including general paresis and tabes dorsalis, manifested as ataxia, impaired memory, disorientation, depression, hallucinations, and mania (102, 103). Furthermore, as a great imitator, neurosyphilis can mimic a wide range of neurological and psychiatric diseases (104), including but not limited to autoimmune encephalitis (105), acute ischemic stroke (106), status epilepticus (107), posterior uveitis (108), asymptomatic optic perineuritis (109). These phenotypes suggest that T. pallidum can invade and affect one or more components of the CNS.
Among the diagnostic criteria, CSF examination is necessary. Research on susceptibility to neurosyphilis provides key insights into the pathogenesis and clinical strategies, though many questions still remain, particularly regarding when patients should undergo CSF testing to screen for neurosyphilis. Based on a general survey of relevant guidelines, scholars pay great emphasis to neurological symptoms, syphilis stages, and HIV infection in lumbar puncture (Table 2). As mentioned above, there is a consensus that syphilis patients with neurological symptoms require CSF examination. Additionally, there is limited support for routine lumbar puncture among HIV-positive and HIV-negative persons without neurologic symptoms owing to a lack of evidence that routine lumbar puncture improves clinical outcomes (79). It is necessary to supplement the probability of ANS developing into symptomatic neurosyphilis and the risks and cost-effectiveness of lumbar puncture must be weighed.
A positive CSF venereal disease research laboratory (VDRL) test is considered highly specific for neurosyphilis, whereas a nonreactive CSF fluorescent treponemal antibody absorption (FTA-ABS) test is likely to exclude neurosyphilis (112). The occurrence of false positive results can be attributed to the ability of anti-T. pallidum IgG antibodies to traverse the BBB and access the CSF. Consequently, in comparison to non-treponemal tests, the specificity of treponemal tests for CSF examination are lower (57).
Currently, there is no gold standard for diagnosing neurosyphilis, making it difficult to evaluate the diagnostic efficiency of new methods (113). The key to indirect detection lies in distinguishing the specific antibodies synthesized intrathecally, and the antibody index of specific anti-Treponema IgG is a promising new tool (114). PCR lacks sensitivity as a direct detection method (115). At present, there have been no successful development of T. pallidum antigen detection kits. The prevailing situations may indicate that T. pallidum has the ability to conceal or attach itself to the human body, and the widespread use of antibiotics in other diseases, thus posing a challenge for conventional techniques to detect nucleic acids or antigens in the blood. Overall, application of new technologies with higher sensitivity, such as nested PCR (nPCR) and loop-mediated isothermal amplification (LAMP) assay, may pave the way for the detection of T. pallidum in CSF. T. pallidum DNA detection rate by LAMP assay was 87.5% in secondary syphilis, which is higher than that in a nPCR study, which achieved T. pallidum DNA detection rate respectively in 47.5%, 60.7% of urine sediment and plasma samples from patients with secondary syphilis (116, 117). Actually, researchers have also made many efforts, such as improving sample preprocessing methods and seeking inspiration from case reports to find new sample types (such as saliva) (118, 119).
Treatment and antibiotic resistance of neurosyphilis
In treatment of neurosyphilis, it is crucial to consider the BBB penetration and effective drug concentrations in CSF (120). The recommended treatment for neurosyphilis is intravenous aqueous crystalline penicillin G (18–24 million units per day, continuous infusion for 10–14 days) (14). Doxycycline and ceftriaxone are considered viable alternatives for penicillin-allergic patients with great BBB penetration ability (121, 122). Doxycycline can be administered orally and can also treat other sexually transmitted infections simultaneously (123). Similar outcomes have been observed in patients with neurosyphilis treated with procaine G penicillin vs. doxycycline (124). Ceftriaxone, by contrast, requires parenteral administration, and its therapeutic effect appears to be controversial (123, 125). A follow-up study suggested that ceftriaxone was associated with a 23% failure rate of treatment for HIV-infected patients with ANS (125). However, another prospective pilot study of HIV-infected patients with ANS has shown no difference in the serologic response to treatment with ceftriaxone vs. procaine penicillin plus probenecid (126). Probenecid can reduce renal tubular secretion and inhibit the active transport of intracranial penicillin by inhibiting Oat3, thereby increasing the bioavailability of penicillin G (127). An enhanced regimen consisting of benzathine penicillin G, ceftriaxone, and doxycycline has demonstrated greater efficacy than the recommended regimen (128). Additionally, a preclinical study has demonstrated linezolid as a promising clinical treatment for syphilis (129). Linezolid is low-cost, safe, and generally well tolerated during short course oral administration, with sufficient concentration in CSF (130). The recent breakthrough in in vitro cultivation of T. pallidum has facilitated identification of potential candidates for syphilis treatment, as determined by the minimum inhibitory concentration (MIC) (131). Future studies involving neurosyphilis-related strains will provide more convincing evidence for the selection of drugs for the treatment of neurosyphilis.
To date, no clinical manifestations of penicillin resistance have been found in T. pallidum, despite reports of increased gene mutations associated with penicillin resistance and that treatment may fail over time (132). Molecular epidemiology study has revealed a growing prevalence of macrolide resistance in T. pallidum, which coincides with the increased usage of macrolides due to guideline recommendations (133). In fact, the 2020 European guideline excluded azithromycin as an alternative treatment for syphilis at any stage (57). To tackle the potential crisis of drug resistance, subtractive genomics approaches have been employed to identify salvicine as a potential therapeutic molecule against T. pallidum (134).
Prevention of neurosyphilis
Although penicillin treatment is effective in patients diagnosed with early-stage neurosyphilis, early diagnosis of neurosyphilis is rather difficult, and prevention is particularly important considering the poor prognosis of late-stage patients (135). In addition, given the fatal consequences of congenital syphilis, development of syphilis vaccines is of utmost importance for enhancing public health (136).
The specific significance of the syphilis vaccine for neurosyphilis is to prevent transmission of T. pallidum from the infected site and progression to neurosyphilis (137). However, development of a syphilis vaccine is hindered by challenges, including the difficulty of culturing T. pallidum in vitro, antigenic mutation in TprK, and the low content and vulnerability of the outer membrane proteins (OMPs); thus, no syphilis vaccine has yet progressed to clinical trials (17, 138). Despite these hurdles, researchers have shown positive responses to this pursuit. To evaluate the efficacy of a syphilis vaccine, a heterologous antigen presentation system (noninfectious Borrelia burgdorferi) was designed to express T. pallidum antigens (139). Recent advancements in long-term culture systems (by cocultivation with rabbit epithelial cells in a microaerophilic atmosphere) and in vitro drug sensitivity testing provide potential solutions for the culture of T. pallidum (138). In addition, enrichment techniques that do not rely on culture, or high sensitivity methods, have also been introduced for assays or genetic analyses (140). Vulnerability to outer membrane extraction can be minimized through the use of gel microdroplet techniques, while bioinformatics methods aid in predicting OMPs without the performance of fragile outer membrane (141). Additionally, antigenic variation can be addressed by designing strains that impair the ability to alter TprK (41). The latest field of molecular biology and bioinformatics provides unprecedented opportunities for the identification of new vaccine targets. Several adhesins, such as TP0136 (142), TP0751 (143), and TP0954 (144), have been identified as major vaccine candidates, highlighting their role in invasion and dissemination of T. pallidum. Current limitations of these candidates include the requirement of extremely high doses, lack of cross-protection and potential side effects of adjuvants (142, 144). Although some scientists have attempted multicomponent vaccines, complete protection against infection has not yet been achieved (137). Undeniably, inducing partial protection vaccines contributes to the attenuation of transmission and, hence, blockade of progression to neurosyphilis (145). The general topic of vaccines for T. pallidum has been thoroughly addressed elsewhere and here will not go into more detail (136, 146).
T. pallidum is primarily transmitted through skin-to-skin or mucosal contact during sexual encounters, as well as through vertical transmission (147). Over the past three decades, public attention to sexually transmitted infections has focused primarily on HIV infection, while other infections such as syphilis have gradually been marginalized (148). Preexposure prophylaxis (PrEP) has been, extensively studied as a crucial intervention to prevent HIV transmission but has not been applied to syphilis (149). Interestingly, the incidence of syphilis is higher among MSM taking HIV PrEP than among those who do not in Australia (150). This causal relationship may be attributed to a phenomenon called “risk compensation,” whereby a reduction in HIV risk may cause lax thinking and increased risky behavior (151). However, a German study believes that HIV PrEP was associated with no impact on the prevalence of syphilis among MSM and concerns about risk compensation should not be the barrier to PrEP use in men with behavioral risk for HIV acquisition (152). It is necessary to carry out more studies on the impact of HIV PrEP on the prevalence of syphilis in other countries or regions. These findings underscore the significance of employing combination prevention strategies or developing a syphilis-specific PrEP.
Treatment as prevention (TasP) is an additional method that is effective in controlling the source of infection (153). For HIV-positive patients, it refers to taking HIV medications to prevent sexual transmission of HIV. The proportion of infants born to syphilis mothers suffering from neurosyphilis is higher than expected. For syphilis patients, antenatal treatment has demonstrated high efficacy in reducing the risk of congenital syphilis with the dose of at least 2.4 MU penicillin given at least 28 days before delivery (154). However, a case-control study revealed that treatment of neurosyphilis remains difficult, even if most pregnant women with syphilis are treated with penicillin, and that this is related to inadequate treatment of sexual partners (155). Effective management and control of neurosyphilis continues to face significant obstacles.
Discussion
Taken together, neurosyphilis denotes an infection of the CNS with a poor prognosis among individuals with syphilis. Although neurosyphilis has been described for centuries, numerous aspects remain unknown (as outlined in Table 3). To address the dearth of epidemiological investigations on neurosyphilis, it is imperative to conduct additional observational studies and consider the implementation of comprehensive surveillance systems on a national or regional scale. As discussed, recent literature increasingly indicates the interrelation between neurosyphilis, the immune response, and genetic factors. However, time is needed for the specific implementation of these strategies in clinical practice. In addition to focusing on the T. pallidum itself, pathogen-host interactions should also be taken into account, especially the pathogens with high neuroinvasion properties and host with high susceptibility to infection.
Currently, clinical suspicion of neurosyphilis primarily arises in patients with syphilis who exhibit neurological and/or psychiatric symptoms. In such cases, it is appropriate to consider serum non-treponemal test titer and serofast status. Accelerating the development of potential serological predictors, standardizing their use, and promptly introducing them into clinical practice would greatly assist clinicians in determining the optimal timing for lumbar puncture. Furthermore, further clinical studies with higher levels of evidence are necessary to explore the efficacy and long-term prognosis of antibiotic therapy and prior to implementation, both the BBB penetration ability and the potential neurotoxicity must be meticulously evaluated.
Given the sustained sensitivity of T. pallidum to penicillin, the prevalence of neurosyphilis signifies a disregard for prevention and management, thus necessitating development of vaccines. However, this endeavor faces significant challenges and lags behind the development of vaccines for other bacteria. The latest field of molecular biology and bioinformatics provides unprecedented opportunities for the identification of new vaccine targets. In order to effectively control neurosyphilis, it is also crucial to address the stigma associated with the disease. This involves not only providing proper care for the mental health of patients but also engaging in scientific education. Moving forward, emphasis needs to be placed on the most cost-effective strategies of prevention to mitigate the global burden of neurosyphilis.
Author contributions
SW: Conceptualization, Visualization, Writing – original draft. FY: Writing – review & editing. YW: Writing – review & editing. DL: Supervision, Writing – review & editing.
Funding
The author(s) declare financial support was received for the research, authorship, and/or publication of this article. This research received grants from the Key Research and Development Projects of Sichuan Provincial Department of Science and Technology (2022YFS0309).
Conflict of interest
The authors declare that the research was conducted in the absence of any commercial or financial relationships that could be construed as a potential conflict of interest.
Publisher's note
All claims expressed in this article are solely those of the authors and do not necessarily represent those of their affiliated organizations, or those of the publisher, the editors and the reviewers. Any product that may be evaluated in this article, or claim that may be made by its manufacturer, is not guaranteed or endorsed by the publisher.
References
2. WHO. Global Progress Report on HIV, Viral Hepatitis and Sexually Transmitted Infections, 2021. Geneva: World Health Organization (2021).
3. Shi M, Peng RR, Gao Z, Zhang S, Lu H, Guan Z, et al. Risk profiles of neurosyphilis in hiv-negative patients with primary, secondary and latent syphilis: implications for clinical intervention. J Eur Acad Dermatol Venereol. (2016) 30:659–66. doi: 10.1111/jdv.13514
4. de Voux A, Kidd S, Torrone EA. Reported cases of neurosyphilis among early syphilis cases-United States, 2009 to 2015. Sex Transm Dis. (2018) 45:39–41. doi: 10.1097/OLQ.0000000000000687
5. Daey Ouwens IM, Koedijk FD, Fiolet AT, van Veen MG, van den Wijngaard KC, Verhoeven WM, et al. Neurosyphilis in the mixed urban-rural community of the Netherlands. Acta Neuropsychiatr. (2014) 26:186–92. doi: 10.1017/neu.2013.53
6. Ramachandran PS, Baird RW, Markey P, Singleton S, Lowe M, Currie BJ, et al. Neurosyphilis: still prevalent and overlooked in an at risk population. PLoS ONE. (2020) 15:e0238617. doi: 10.1371/journal.pone.0238617
7. Quintero-Moreno JF, Valencia-Vasquez A, Aguirre-Castaneda C. Clinical and socio-demographic profile of neurosyphilis: a retrospective study in a reference centre in Colombia. Rev Neurol. (2019) 69:53–8. doi: 10.33588/rn.6902.2018381
8. Tang W, Huang S, Chen L, Yang L, Tucker JD, Zheng H, et al. Late neurosyphilis and tertiary syphilis in Guangdong province, China: results from a cross-sectional study. Sci Rep. (2017) 7:45339. doi: 10.1038/srep45339
9. Ren M, Dashwood T, Walmsley S. The intersection of HIV and syphilis: update on the key considerations in testing and management. Curr HIV/AIDS Rep. (2021) 18:280–8. doi: 10.1007/s11904-021-00564-z
10. Marks M, Jarvis JN, Howlett W, Mabey DCW. Neurosyphilis in Africa: a systematic review. PLoS Negl Trop Dis. (2017) 11:e0005880. doi: 10.1371/journal.pntd.0005880
11. Rowley J, Vander Hoorn S, Korenromp E, Low N, Unemo M, Abu-Raddad LJ, et al. Chlamydia, gonorrhoea, trichomoniasis and syphilis: global prevalence and incidence estimates, 2016. Bull World Health Organ. (2019) 97:548–62p. doi: 10.2471/BLT.18.228486
12. Du FZ, Zhang X, Zhang RL, Wang QQ. Care-Ns, a research strategy for neurosyphilis. Front Med. (2022) 9:1040133. doi: 10.3389/fmed.2022.1040133
13. Cejková D, Zobaníková M, Chen L, Pospíšilová P, Strouhal M, Qin X, et al. Whole genome sequences of three Treponema pallidum ssp. pertenue strains: yaws and syphilis treponemes differ in less than 02% of the genome sequence. PLoS Negl Trop Dis. (2012) 6:e1471. doi: 10.1371/journal.pntd.0001471
14. Workowski KA, Bachmann LH, Chan PA, Johnston CM, Muzny CA, Park I, et al. Sexually transmitted infections treatment guidelines, 2021. MMWR Recomm Rep. (2021) 70:1–187. doi: 10.15585/mmwr.rr7004a1
15. Pastuszczak M, Jakiela B, Jaworek AK, Wypasek E, Zeman J, Wojas-Pelc A. Association of interleukin-10 promoter polymorphisms with neurosyphilis. Hum Immunol. (2015) 76:469–72. doi: 10.1016/j.humimm.2015.06.010
16. Fraser CM, Norris SJ, Weinstock GM, White O, Sutton GG, Dodson R, et al. Complete genome sequence of Treponema pallidum, the syphilis spirochete. Science. (1998) 281:375–88. doi: 10.1126/science.281.5375.375
17. Liu J, Howell JK, Bradley SD, Zheng Y, Zhou ZH, Norris SJ. Cellular architecture of Treponema pallidum: novel flagellum, periplasmic cone, and cell envelope as revealed by cryo electron tomography. J Mol Biol. (2010) 403:546–61. doi: 10.1016/j.jmb.2010.09.020
18. Nakamura S. Spirochete flagella and motility. Biomolecules. (2020) 10:550. doi: 10.3390/biom10040550
19. Radolf JD, Kumar S. The Treponema pallidum outer membrane. Curr Top Microbiol Immunol. (2018) 415:1–38. doi: 10.1007/82_2017_44
20. Wolgemuth CW. Flagellar motility of the pathogenic spirochetes. Semin Cell Dev Biol. (2015) 46:104–12. doi: 10.1016/j.semcdb.2015.10.015
21. Lu S, Zheng K, Wang J, Xu M, Xie Y, Yuan S, et al. Characterization of Treponema pallidum dissemination in C57bl/6 mice. Front Immunol. (2020) 11:577129. doi: 10.3389/fimmu.2020.577129
22. Pham K, Gottesdiener L, Simon MS, Trzebucki A, Maldarelli GA, Cisse B, et al. Meningovascular syphilis presenting as a brain mass in an immunocompetent male. Open Forum Infect Dis. (2021) 8:ofab455. doi: 10.1093/ofid/ofab455
23. Zhou X, Peng S, Song T, Tie D, Tao X, Jiang L, et al. Neurosyphilis with ocular involvement and normal magnetic resonance imaging results affirmed by metagenomic next-generation sequencing. Front Cell Infect Microbiol. (2022) 12:985373. doi: 10.3389/fcimb.2022.985373
24. Yu H, Chang Q, Sun T, He X, Wen L, An J, et al. Metabolic reprogramming and polarization of microglia in Parkinson's disease: role of inflammasome and iron. Ageing Res Rev. (2023) 90:102032. doi: 10.1016/j.arr.2023.102032
25. Sakai K, Fukuda T, Iwadate K, Maruyama-Maebashi K, Asakura K, Ozawa M, et al. A fatal fall associated with undiagnosed parenchymatous neurosyphilis. Am J Forensic Med Pathol. (2014) 35:4–7. doi: 10.1097/PAF.0000000000000068
26. Li W, Chang H, Wu W, Xu D, Jiang M, Gao J, et al. Increased CSF soluble trem2 concentration in patients with neurosyphilis. Front Neurol. (2020) 11:62. doi: 10.3389/fneur.2020.00062
27. Hu YT, Wu KX, Wang XT, Zhao YY, Jiang XY, Liu D, et al. Treponema pallidum promoted microglia apoptosis and prevented itself from clearing by human microglia via blocking autophagic flux. PLoS Pathog. (2023) 19:e1011594. doi: 10.1371/journal.ppat.1011594
28. Xie L, Li W, Zheng XQ, Liu LL, Lin LR, Niu JJ, et al. Treponema pallidum membrane protein Tp47 induced autophagy and inhibited cell migration in Hmc3 cells Via the Pi3k/Akt/Foxo1 pathway. J Cell Mol Med. (2023) 29:3065–74. doi: 10.1111/jcmm.17872
29. Luthra A, Anand A, Hawley KL, LeDoyt M, La Vake CJ, Caimano MJ, et al. A homology model reveals novel structural features and an immunodominant surface loop/opsonic target in the Treponema pallidum bama ortholog Tp_0326. J Bacteriol. (2015) 197:1906–20. doi: 10.1128/JB.00086-15
30. Houston S, Hof R, Honeyman L, Hassler J, Cameron CE. Activation and proteolytic activity of the Treponema pallidum metalloprotease, pallilysin. PLoS Pathog. (2012) 8:e1002822. doi: 10.1371/journal.ppat.1002822
31. Zheng XQ Li Z, Meng QQ Li W, Li QL, Xie L, et al. Treponema pallidum recombinant protein Tp47 activates nod-like receptor family protein 3 inflammasomes in macrophages via glycolysis. Int Immunopharmacol. (2023) 126:111204. doi: 10.1016/j.intimp.2023.111204
32. Xie B, Zhao T, Zhao S, Zhou J, Zhao F. Possible effects of Treponema pallidum infection on human vascular endothelial cells. J Clin Lab Anal. (2022) 36:e24318. doi: 10.1002/jcla.24318
33. Lafond RE, Lukehart SA. Biological basis for syphilis. Clin Microbiol Rev. (2006) 19:29–49. doi: 10.1128/CMR.19.1.29-49.2006
34. Djokic V, Giacani L, Parveen N. Analysis of host cell binding specificity mediated by the Tp0136 adhesin of the syphilis agent Treponema pallidum subsp. Pallidum PLoS Negl Trop Dis. (2019) 13:e0007401. doi: 10.1371/journal.pntd.0007401
35. Lithgow KV, Tsao E, Schovanek E, Gomez A, Swayne LA, Cameron CE. Treponema pallidum disrupts ve-cadherin intercellular junctions and traverses endothelial barriers using a cholesterol-dependent mechanism. Front Microbiol. (2021) 12:691731. doi: 10.3389/fmicb.2021.691731
36. Wu F, Zhang JP, Wang QQ. Scanning electron microscopy of the adhesion of Treponema pallidum subspecies pallidum (nichol strain) to human brain microvascular endothelial cells in vitro. J Eur Acad Dermatol Venereol. (2017) 31:e221–e3. doi: 10.1111/jdv.13984
37. Lithgow KV, Church B, Gomez A, Tsao E, Houston S, Swayne LA, et al. Identification of the neuroinvasive pathogen host target, lamr, as an endothelial receptor for the Treponema pallidum adhesin Tp0751. mSphere. (2020) 5:2. doi: 10.1128/mSphere.00195-20
38. Lu S, Wang J, He Z, He S, Zheng K, Xu M, et al. Treponema pallidum Tp0751 alters the expression of tight junction proteins by promoting bend3 cell apoptosis and il-6 secretion. Int J Med Microbiol. (2022) 312:151553. doi: 10.1016/j.ijmm.2022.151553
39. Zhang RL, Zhang JP, Wang QQ. Recombinant Treponema pallidum protein tp0965 activates endothelial cells and increases the permeability of endothelial cell monolayer. PLoS ONE. (2014) 9:e115134. doi: 10.1371/journal.pone.0115134
40. Reiber H, Peter JB. Cerebrospinal fluid analysis: disease-related data patterns and evaluation programs. J Neurol Sci. (2001) 184:101–22. doi: 10.1016/S0022-510X(00)00501-3
41. Romeis E, Lieberman NAP, Molini B, Tantalo LC, Chung B, Phung Q, et al. Treponema pallidum subsp. pallidum with an artificially impaired TPRK antigenic variation system is attenuated in the rabbit model of syphilis. PLoS Pathog. (2023) 19:e1011259. doi: 10.1371/journal.ppat.1011259
42. Cai CX Li SL, Lin HL, Wei ZH, Xie L, Lin LR, et al. Treponema pallidum protein Tp0136 promoting Mmps/Timps imbalance via Pi3k, Mapk and Nf-?b signalling pathways in Hdvsmcs. Heliyon. (2022) 8:e12065. doi: 10.1016/j.heliyon.2022.e12065
43. Deka RK, Liu WZ, Norgard MV, Brautigam CA. Biophysical and biochemical characterization of Tp0037, a D-lactate dehydrogenase, supports an acetogenic energy conservation pathway in Treponema pallidum. mBio. (2020) 11:20. doi: 10.1128/mBio.02249-20
44. Pětrošová H, Pospíšilová P, Strouhal M, Cejková D, Zobaníková M, Mikalová L, et al. Resequencing of Treponema pallidum ssp. pallidum strains nichols and Ss14: correction of sequencing errors resulted in increased separation of syphilis treponeme subclusters. PLoS ONE. (2013) 8:e74319. doi: 10.1371/journal.pone.0074319
45. Tantalo LC, Lukehart SA, Marra CM. Treponema pallidum strain-specific differences in neuroinvasion and clinical phenotype in a rabbit model. J Infect Dis. (2005) 191:75–80. doi: 10.1086/426510
46. Molepo J, Pillay A, Weber B, Morse SA, Hoosen AA. Molecular typing of Treponema pallidum strains from patients with neurosyphilis in pretoria, South Africa. Sex Transm Infect. (2007) 83:189–92. doi: 10.1136/sti.2006.023895
47. Sahi SK, Zahlan JM, Tantalo LC, Marra CM, A. Comparison of Treponema pallidum subspecies pallidum molecular typing systems: multilocus sequence typing vs. enhanced centers for disease control and prevention typing sex. Transm Dis. (2021) 48:670–4. doi: 10.1097/OLQ.0000000000001378
48. Marra C, Sahi S, Tantalo L, Godornes C, Reid T, Behets F, et al. Enhanced molecular typing of Treponema pallidum: geographical distribution of strain types and association with neurosyphilis. J Infect Dis. (2010) 202:1380–8. doi: 10.1086/656533
49. Grillová L, Bawa T, Mikalová L, Gayet-Ageron A, Nieselt K, Strouhal M, et al. Molecular characterization of Treponema pallidum subsp. pallidum in Switzerland and france with a new multilocus sequence typing scheme. PLoS One. (2018) 13:e0200773. doi: 10.1371/journal.pone.0200773
50. Dai T, Li K, Lu H, Gu X, Wang Q, Zhou P. Molecular typing of Treponema pallidum: a 5-year surveillance in Shanghai, China. J Clin Microbiol. (2012) 50:3674–7. doi: 10.1128/JCM.01195-12
51. Read P, Tagg KA, Jeoffreys N, Guy RJ, Gilbert GL, Donovan B. Treponema pallidum strain types and association with macrolide resistance in Sydney, Australia: new tp0548 gene types identified. J Clin Microbiol. (2016) 54:2172–4. doi: 10.1128/JCM.00959-16
52. Liu D, He SM, Zhu XZ, Liu LL, Lin LR, Niu JJ, et al. Molecular Characterization based on Mlst and Ecdc typing schemes and antibiotic resistance analyses of Treponema pallidum subsp. Pallidum in Xiamen, China. Front Cell Infect Microbiol. (2020) 10:618747. doi: 10.3389/fcimb.2020.618747
53. Peng RR, Wang AL Li J, Tucker JD, Yin YP, Chen XS. Molecular typing of Treponema pallidum: a systematic review and meta-analysis. PLoS Negl Trop Dis. (2011) 5:e1273. doi: 10.1371/journal.pntd.0001273
54. Chen W, Luo H, Zeng L, Pan Y, Parr JB, Jiang Y, et al. A suite of Pcr-Lwcas13a assays for detection and genotyping of Treponema pallidum in clinical samples. Nat Commun. (2022) 13:4671. doi: 10.1038/s41467-022-32250-y
55. Kreisel KM, Spicknall IH, Gargano JW, Lewis FMT, Lewis RM, Markowitz LE, et al. Sexually transmitted infections among US women and men: prevalence and incidence estimates, 2018. Sex Transm Dis. (2021) 48:208–14. doi: 10.1097/OLQ.0000000000001355
56. Huang J, Wu H, Lin S, Lu L, Zheng J, Liu B, et al. Spatial-temporal analysis of HIV/AIDS and syphilis in mainland China from 2007 to 2017. J Med Virol. (2022) 94:3328–37. doi: 10.1002/jmv.27725
57. Janier M, Unemo M, Dupin N, Tiplica GS, Potočnik M, Patel R. 2020 European guideline on the management of syphilis. J Eur Acad Dermatol Venereol. (2021) 35:574–88. doi: 10.1111/jdv.16946
58. Satterwhite CL, Torrone E, Meites E, Dunne EF, Mahajan R, Ocfemia MC, et al. Sexually transmitted infections among US women and men: prevalence and incidence estimates, 2008. Sex Transm Dis. (2013) 40:187–93. doi: 10.1097/OLQ.0b013e318286bb53
59. Tsuboi M, Evans J, Davies EP, Rowley J, Korenromp EL, Clayton T, et al. Prevalence of syphilis among men who have sex with men: a global systematic review and meta-analysis from 2000-20. The Lancet Global health. (2021) 9:e1110–e8. doi: 10.1016/S2214-109X(21)00221-7
60. Oware K, Adiema L, Rono B, Violette LR, McClelland RS, Donnell D, et al. Characteristics of Kenyan women using Hiv prep enrolled in a randomized trial on doxycycline postexposure prophylaxis for sexually transmitted infection prevention. BMC Womens Health. (2023) 23:296. doi: 10.1186/s12905-023-02413-0
61. Cui W, Yan J, Weng W, Gao Y, Zhu W. Factors associated with neurosyphilis in patients with syphilis treatment failure: a retrospective study of 165 HIV-negative patients. Front Med. (2022) 9:757354. doi: 10.3389/fmed.2022.757354
62. Fu Y, Yang L, Du J, Khan R, Liu D. Establishment of HIV-negative neurosyphilis risk score model based on logistic regression. Eur J Med Res. (2023) 28:200. doi: 10.1186/s40001-023-01177-5
63. Michaud M, Balardy L, Moulis G, Gaudin C, Peyrot C, Vellas B, et al. Proinflammatory cytokines, aging, and age-related diseases. J Am Med Dir Assoc. (2013) 14:877–82. doi: 10.1016/j.jamda.2013.05.009
64. Danics K, Forrest SL, Kapas I, Erber I, Schmid S, Töro K, et al. Neurodegenerative proteinopathies associated with neuroinfections. J Neural Transm. (2021) 128:1551–66. doi: 10.1007/s00702-021-02371-7
65. Miklossy J. Historic evidence to support a causal relationship between spirochetal infections and Alzheimer's disease. Front Aging Neurosci. (2015) 7:46. doi: 10.3389/fnagi.2015.00046
66. Xie JW, Wang M, Zheng YW, Lin Y, He Y, Lin LR. Performance of the nontreponemal tests and treponemal tests on cerebrospinal fluid for the diagnosis of neurosyphilis: a meta-analysis. Front Public Health. (2023) 11:1105847. doi: 10.3389/fpubh.2023.1105847
67. Gao ZX, Gou Y, Liu XQ, Peng LW. Advances in laboratory diagnostic methods for cerebrospinal fluid testing for neurosyphilis. Front Public Health. (2022) 10:1030480. doi: 10.3389/fpubh.2022.1030480
68. Jiang Y, Chen X, Ma X, Yang Y, Peng F, Hu X. The usefulness of toluidine red unheated serum test in the diagnosis of hiv-negative neurosyphilis. Sex Transm Dis. (2011) 38:244–5. doi: 10.1097/OLQ.0b013e3181f42093
69. Weng W, Hou J, Song B, Zhang M, Zhang T, Gao Y. Identification of the factors associated with post-treatment asymptomatic neurosyphilis in HIV-negative patients with serological non-response syphilis: a retrospective study. Int J STD AIDS. (2021) 32:331–5. doi: 10.1177/0956462420965850
70. Marra CM, Sahi SK, Tantalo LC, Ho EL, Dunaway SB, Jones T, et al. Toll-like receptor polymorphisms are associated with increased neurosyphilis risk. Sex Transm Dis. (2014) 41:440–6. doi: 10.1097/OLQ.0000000000000149
71. Haule A, Msemwa B, Mgaya E, Masikini P, Kalluvya S. Prevalence of syphilis, neurosyphilis and associated factors in a cross-sectional analysis of HIV infected patients attending bugando medical centre, Mwanza, Tanzania. BMC Public Health. (2020) 20:1862. doi: 10.1186/s12889-020-09984-9
72. Shrestha R, Millington O, Brewer J, Bushell T. Is central nervous system an immune-privileged site? Kathmandu Univ Med J (KUMJ). (2013) 11:102–7. doi: 10.3126/kumj.v11i1.11055
73. Hobbs E, Vera JH, Marks M, Barritt AW, Ridha BH, Lawrence D. Neurosyphilis in patients with HIV. Pract Neurol. (2018) 18:211–8. doi: 10.1136/practneurol-2017-001754
74. Yu J, Shi J, Wan H, Li J, Shao Y, Ye J, et al. clinical characteristics, diagnosis, and predictors of neurosyphilis patients with human immunodeficiency virus co-infection: a retrospective study at infectious diseases hospitals in two cities of China. Medicine (Baltimore). (2021) 100:e27430. doi: 10.1097/MD.0000000000027430
75. Rotman L, Luo X, Thompson A, Mackesy-Amiti ME, Young LR, Young JD. Risk of neurosyphilis in HIV-infected persons with syphilis lacking signs or symptoms of central nervous system infection. HIV Med. (2019) 20:27–32. doi: 10.1111/hiv.12677
76. Xia W, Zhao J, Su B, Jiao Y, Weng W, Zhang M, et al. Syphilitic infection impairs immunity by inducing both apoptosis and pyroptosis of Cd4(+) and Cd8(+) T lymphocytes. Innate Immun. (2021) 27:99–106. doi: 10.1177/1753425920952840
77. Kofoed K, Gerstoft J, Mathiesen LR, Benfield T. Syphilis and human immunodeficiency virus (HIV)-1 coinfection: influence on Cd4 T-cell count, Hiv-1 viral load, and treatment response. Sex Transm Dis. (2006) 33:143–8. doi: 10.1097/01.olq.0000187262.56820.c0
78. Farhi D, Dupin N. Management of syphilis in the HIV-infected patient: facts and controversies. Clin Dermatol. (2010) 28:539–45. doi: 10.1016/j.clindermatol.2010.03.012
79. Li W, Han J, Zhao P, Wang D, Sun T, Guo J, et al. Predicting asymptomatic neurosyphilis using peripheral blood indicators. BMC Infect Dis. (2021) 21:1191. doi: 10.1186/s12879-021-06846-6
80. Marra CM, Sahi SK, Tantalo LC, Zetterberg H. Serum neurofilament light in neurosyphilis: a pilot study. Sex Transm Dis. (2023) 50:42–4. doi: 10.1097/OLQ.0000000000001717
81. He C, Shang X, Liu W, Hang S, Chen J, Ci C. Combination of the neutrophil to lymphocyte ratio and serum toluidine red unheated serum test titer as a predictor of neurosyphilis in HIV-negative patients. Exp Ther Med. (2021) 21:185. doi: 10.3892/etm.2021.9616
82. Sun JJ, Wang ZY, Shen JY, Shen YZ, Liu L, Wang JR, et al. Serum trsut titer ≥1: 16 IS a predictor for neurosyphilis among hiv-infected patients with concurrent syphilis and no neurological symptoms. Medicine. (2015) 94:e2023. doi: 10.1097/MD.0000000000002023
83. Liu LL, Lin Y, Chen W, Tong ML, Luo X, Lin LR, et al. Metabolite profiles of the cerebrospinal fluid in neurosyphilis patients determined by untargeted metabolomics analysis. Front Neurosci. (2019) 13:150. doi: 10.3389/fnins.2019.00150
84. Chen H, Zhou Y, Wang ZY, Yan BX, Zhou WF, Wang TT, et al. Exosomal microrna profiles from serum and cerebrospinal fluid in neurosyphilis. Sex Transm Infect. (2019) 95:246–50. doi: 10.1136/sextrans-2018-053813
85. Chen B, Shi H, Hou L, Zhong X, Wang Y, Wu Z, et al. Medial temporal lobe atrophy as a predictor of poor cognitive outcomes in general paresis. Early Interv Psychiatry. (2019) 13:30–8. doi: 10.1111/eip.12441
86. Li Z, Teng M, Jiang Y, Zhang L, Luo X, Liao Y, et al. Ythdf1 negatively regulates Treponema pallidum-induced inflammation in Thp-1 macrophages by promoting socs3 translation in an M6a-dependent manner. Front Immunol. (2022) 13:857727. doi: 10.3389/fimmu.2022.857727
87. Xiao Y, Tong ML, Lin LR, Liu LL, Gao K, Chen MJ, et al. Serological response predicts normalization of cerebrospinal fluid abnormalities at six months after treatment in HIV-negative neurosyphilis patients. Sci Rep. (2017) 7:9911. doi: 10.1038/s41598-017-10387-x
88. Marra CM, Maxwell CL, Tantalo LC, Sahi SK, Lukehart SA. Normalization of serum rapid plasma reagin titer predicts normalization of cerebrospinal fluid and clinical abnormalities after treatment of neurosyphilis. Clin Infect Dis. (2008) 47:893–9. doi: 10.1086/591534
89. Jiang Y, Weng R, Zhang Y, Fan R, Liu Y, Chen Z, et al. The performance of rapid plasma reagin (RPR) titer in HIV-negative general paresis after neurosyphilis therapy. BMC Infect Dis. (2018) 18:144. doi: 10.1186/s12879-018-3062-4
90. Haynes AM, Konda KA, Romeis E, Siebert J, Vargas SK, Reyes Diaz M, et al. Evaluation of a minimal array of Treponema pallidum antigens as biomarkers for syphilis diagnosis, infection staging, and response to treatment. Microbiol Spectr. (2023):e0346623. doi: 10.1128/spectrum.03466-23
91. Mori H, Shibata E, Kondo E, Sasaki N, Sawada Y, Yoshino K. The incidence of jarisch-herxheimer reactions and associated risk factors in pregnant women and nonpregnant women: a retrospective chart review at a university hospital in Japan. J Obstet Gynaecol Res. (2023) 49:1435–42. doi: 10.1111/jog.15583
92. Zifko U, Lindner K, Wimberger D, Volc B, Grisold W. Jarisch-herxheimer reaction in a patient with neurosyphilis. J Neurol Neurosurg Psychiatry. (1994) 57:865–7. doi: 10.1136/jnnp.57.7.865
93. Clement ME, Okeke NL, Hicks CB. Treatment of syphilis: a systematic review. JAMA. (2014) 312:1905–17. doi: 10.1001/jama.2014.13259
94. Zhang RL, Wang QQ, Zhang JP, Yang LJ. Molecular subtyping of Treponema pallidum and associated factors of serofast status in early syphilis patients: identified novel genotype and cytokine marker. PLoS ONE. (2017) 12:e0175477. doi: 10.1371/journal.pone.0175477
95. Qiang D, Wang J, Ci C, Tang B, Ke G, Chang X, et al. Evaluation of serum cytokines to predict serofast in syphilis patients. J Infect Chemother. (2020) 26:970–6. doi: 10.1016/j.jiac.2020.04.020
96. Jia X, Wang Z, Liu X, Zheng H, Li J. Peripheral blood mononuclear cell microrna profiles in syphilitic patients with serofast status. Mol Biol Rep. (2020) 47:3407–21. doi: 10.1007/s11033-020-05421-7
97. Pastuszczak M, Jakiela B, Wojas-Pelc A. Association of interleukin-10 promoter polymorphisms with serofast state after syphilis treatment. Sex Transm Infect. (2019) 95:163–8. doi: 10.1136/sextrans-2018-053753
98. Chiolero A. Risk factor (predictive) medicine as a driver of fear and overdiagnosis. BMJ. (2014) 349:g7078. doi: 10.1136/bmj.g7078
99. Mehta N, Bhari N, Gupta S. Asian guidelines for syphilis. J Infect Chemother. (2022) 28:1084–91. doi: 10.1016/j.jiac.2022.04.023
100. Workowski KA, Bolan GA. Sexually transmitted diseases treatment guidelines, 2015. MMWR Recomm Rep. (2015) 64:1–137.
101. Birrell JM, Lowe M, Gunathilake M, Krause VL. Neurosyphilis in the northern territory of Australia: a clinical guideline. Intern Med J. (2023) 53:738–44. doi: 10.1111/imj.15691
102. Klein M, Angstwurm K, Esser S, Hahn K, Maschke M, Scheithauer S, et al. German guidelines on the diagnosis and treatment of neurosyphilis. Neurol Res Pract. (2020) 2:33. doi: 10.1186/s42466-020-00081-1
103. Corrêa DG, de Souza SR, Freddi TAL, Fonseca APA, Dos Santos RQ, Hygino da Cruz LC. Imaging features of neurosyphilis. J Neuroradiol. (2023) 50:241–52. doi: 10.1016/j.neurad.2023.01.003
104. Jum'ah A, Aboul Nour H, Alkhoujah M, Zoghoul S, Eltous L, Miller D. Neurosyphilis in disguise. Neuroradiology. (2022) 64:433–41. doi: 10.1007/s00234-021-02827-3
105. Teixeira Urzêdo Queiroz D, de Cássia Marques Leocádio J, Poggianella Esteves Santana LH, Sales Carlos C, Lage Guerra JV, Silva Ribeiro P, et al. Neurosyphilis masquerading as autoimmune encephalitis. Pract Neurol. (2023). doi: 10.1136/pn-2023-003943. [Epub ahead of print].
106. Krishnan D, Zaini SS, Latif KA, Joseph JP. Neurosyphilis presenting as acute ischemic stroke. Clin Med. (2020) 20:95–7. doi: 10.7861/clinmed.2019-0368
107. Qi Y, Jiang Z, Xie D, Lei N, Cai Z. Status epilepticus with focal neurologic deficits: a rare presentation of neurosyphilis. Int J Infect Dis. (2022) 117:345–8. doi: 10.1016/j.ijid.2022.02.029
108. Xu Y, Li J, Xu Y, Xia W, Mo X, Feng M, et al. Case report: visual acuity loss as a warning sign of ocular syphilis: a retrospective analysis of 17 cases. Front Med. (2022) 9:1037712. doi: 10.3389/fmed.2022.1037712
109. Parker SE, Pula JH. neurosyphilis presenting as asymptomatic optic perineuritis. Case Rep Ophthalmol Med. (2012) 2012:621872. doi: 10.1155/2012/621872
110. National Center for STD Control CCfDCaPVG Chinese Chinese Society of Dermatology Subcommittee on Venereology China Dermatologist Association. Guidelines for diagnosis and treatment of syphilis, gonorrhea and genital chlamydia trachomatis infection. Chin J Dermatol. (2020) 53:168–79. doi: 10.35541/cjd.20190808
111. Kingston M, French P, Higgins S, McQuillan O, Sukthankar A, Stott C, et al. UK national guidelines on the management of syphilis 2015. Int J STD AIDS. (2016) 27:421–46. doi: 10.1177/0956462415624059
112. Marra CM, Maxwell CL, Dunaway SB, Sahi SK, Tantalo LC. Cerebrospinal fluid Treponema pallidum particle agglutination assay for neurosyphilis diagnosis. J Clin Microbiol. (2017) 55:1865–70. doi: 10.1128/JCM.00310-17
113. Alberto C, Lambeng N, Deffert C, Breville G, Gayet-Ageron A, Lalive P, et al. Multicentric evaluation of a specific intrathecal anti-Treponema pallidum igg index as a diagnostic biomarker of neurosyphilis: results from a retro-prospective case-control study. Sex Transm Infect. (2023). doi: 10.1136/sextrans-2023-055913
114. Alberto C, Deffert C, Lambeng N, Breville G, Gayet-Ageron A, Lalive P, et al. Intrathecal synthesis index of specific anti-treponema igg: a new tool for the diagnosis of neurosyphilis. Microbiol Spectr. (2022) 10:e0147721. doi: 10.1128/spectrum.01477-21
115. Vanhaecke C, Grange P, Benhaddou N, Blanche P, Salmon D, Parize P, et al. Clinical and biological characteristics of 40 patients with neurosyphilis and evaluation of Treponema pallidum nested polymerase chain reaction in cerebrospinal fluid samples. Clin Infect Dis. (2016) 63:1180–6. doi: 10.1093/cid/ciw499
116. Xiao Y, Xie Y, Xu M, Liu S, Jiang C, Zhao F, et al. Development and evaluation of a loop-mediated isothermal amplification assay for the detection of Treponema pallidum DNA in the peripheral blood of secondary syphilis patients. Am J Trop Med Hyg. (2017) 97:1673–8. doi: 10.4269/ajtmh.17-0051
117. Wang C, Zheng X, Guan Z, Zou D, Gu X, Lu H, et al. quantified detection of Treponema pallidum DNA by PCR assays in urine and plasma of syphilis patients. Microbiol Spectr. (2022) 10:e0177221. doi: 10.1128/spectrum.01772-21
118. Wei ZH, Xie L, Wang YJ, Zhuang JX, Niu JJ, Liu LL. Red blood cell lysis pretreatment can significantly improve the yield of Treponema pallidum DNA FROM blood. Microbiol Spectr. (2023) 11:e0519822. doi: 10.1128/spectrum.05198-22
119. Wang C, Hu Z, Zheng X, Ye M, Liao C, Shang M, et al. A new specimen for syphilis diagnosis: evidence by high loads of Treponema pallidum DNA in saliva. Clin Infect Dis. (2021) 73:e3250–e8. doi: 10.1093/cid/ciaa1613
120. Du FZ, Wu MZ, Zhang X, Zhang RL, Wang QQ. Ceftriaxone compared with penicillin G for the treatment of neurosyphilis: study protocol for a multicenter randomized controlled trial. Trials. (2022) 23:835. doi: 10.1186/s13063-022-06769-w
121. Bettuzzi T, Jourdes A, Robineau O, Alcaraz I, Manda V, Molina JM, et al. Ceftriaxone compared with benzylpenicillin in the treatment of neurosyphilis in France: a retrospective multicentre study. Lancet Infect Dis. (2021) 21:1441–7. doi: 10.1016/S1473-3099(20)30857-4
122. Yim CW, Flynn NM, Fitzgerald FT. Penetration of oral doxycycline into the cerebrospinal fluid of patients with latent or neurosyphilis. Antimicrob Agents Chemother. (1985) 28:347–8. doi: 10.1128/AAC.28.2.347
123. Psomas KC, Brun M, Causse A, Atoui N, Reynes J, Le Moing V. Efficacy of ceftriaxone and doxycycline in the treatment of early syphilis. Med Mal Infect. (2012) 42:15–9. doi: 10.1016/j.medmal.2011.10.003
124. Girometti N, Junejo MH, Nugent D, McOwan A, Whitlock G. Clinical and serological outcomes in patients treated with oral doxycycline for early neurosyphilis. J Antimicrob Chemother. (2021) 76:1916–9. doi: 10.1093/jac/dkab100
125. Dowell ME, Ross PG, Musher DM, Cate TR, Baughn RE. Response of latent syphilis or neurosyphilis to ceftriaxone therapy in persons infected with human immunodeficiency virus. Am J Med. (1992) 93:481–8. doi: 10.1016/0002-9343(92)90574-U
126. Smith NH, Musher DM, Huang DB, Rodriguez PS, Dowell ME, Ace W, et al. Response of HIV-infected patients with asymptomatic syphilis to intensive intramuscular therapy with ceftriaxone or procaine penicillin. Int J STD AIDS. (2004) 15:328–32. doi: 10.1177/095646240401500511
127. Drago F, Ciccarese G, Rebora A. Treatment of late-stage syphilis. JAMA. (2015) 313:969. doi: 10.1001/jama.2015.0672
128. Drago F, Ciccarese G, Broccolo F, Sartoris G, Stura P, Esposito S, et al. A new enhanced antibiotic treatment for early and late syphilis. J Glob Antimicrob Resist. (2016) 5:64–6. doi: 10.1016/j.jgar.2015.12.006
129. Haynes AM, Giacani L, Mayans MV, Ubals M, Nieto C, Pérez-Mañá C, et al. Efficacy of linezolid on Treponema pallidum, the syphilis agent: a preclinical study. EBioMedicine. (2021) 65:103281. doi: 10.1016/j.ebiom.2021.103281
130. Stamm LV. Hope for new antibiotics for syphilis treatment. EBioMedicine. (2021) 66:103320. doi: 10.1016/j.ebiom.2021.103320
131. Tantalo LC, Lieberman NAP, Pérez-Mañá C, Suñer C, Vall Mayans M, Ubals M, et al. Antimicrobial susceptibility of Treponema pallidum subspecies pallidum: an in-vitro study. Lancet Microbe. (2023) 4:e994–1004. doi: 10.2139/ssrn.4289479
132. Mi HF, Shen X, Chen XQ, Zhang XL, Ke WJ, Xiao Y. Association between treatment failure in patients with early syphilis and penicillin resistance-related gene mutations of Treponema pallidum: protocol for a multicentre nested case-control study. Front Med. (2023) 10:1131921. doi: 10.3389/fmed.2023.1131921
133. Venter JME, Müller EE, Mahlangu MP, Kularatne RS. Treponema pallidum macrolide resistance and molecular epidemiology in Southern Africa, 2008 to 2018. J Clin Microbiol. (2021) 59:e0238520. doi: 10.1128/JCM.02385-20
134. Dwivedi UN, Tiwari S, Singh P, Singh S, Awasthi M, Pandey VP. Treponema pallidum putative novel drug target identification and validation: rethinking syphilis therapeutics with plant-derived terpenoids. OMICS. (2015) 19:104–14. doi: 10.1089/omi.2014.0154
135. Ghanem KG. Review: neurosyphilis: a historical perspective and review. CNS Neurosci Ther. (2010) 16:e157–68. doi: 10.1111/j.1755-5949.2010.00183.x
136. Lithgow KV, Cameron CE. Vaccine development for syphilis. Expert Rev Vaccines. (2017) 16:37–44. doi: 10.1080/14760584.2016.1203262
137. Lukehart SA, Molini B, Gomez A, Godornes C, Hof R, Fernandez MC, et al. Immunization with a tri-antigen syphilis vaccine significantly attenuates chancre development, reduces bacterial load, and inhibits dissemination of Treponema pallidum. Vaccine. (2022) 40:7676–92. doi: 10.1016/j.vaccine.2022.11.002
138. Edmondson DG, Hu B, Norris SJ. Long-term in vitro culture of the syphilis spirochete Treponema pallidum subsp. pallidum. mBio. (2018) 9:18. doi: 10.1128/mBio.01153-18
139. Parveen N, Fernandez MC, Haynes AM, Zhang RL, Godornes BC, Centurion-Lara A, et al. Non-pathogenic borrelia burgdorferi expressing Treponema pallidum Tprk and Tp0435 antigens as a novel approach to evaluate syphilis vaccine candidates. Vaccine. (2019) 37:1807–18. doi: 10.1016/j.vaccine.2019.02.022
140. Chen W, Šmajs D, Hu Y, Ke W, Pospíšilová P, Hawley KL, et al. Analysis of Treponema pallidum strains from China using improved methods for whole-genome sequencing from primary syphilis chancres. J Infect Dis. (2021) 223:848–53. doi: 10.1093/infdis/jiaa449
141. Cox DL, Luthra A, Dunham-Ems S, Desrosiers DC, Salazar JC, Caimano MJ, et al. Surface immunolabeling and consensus computational framework to identify candidate rare outer membrane proteins of Treponema pallidum. Infect Immun. (2010) 78:5178–94. doi: 10.1128/IAI.00834-10
142. Xu M, Xie Y, Zheng K, Luo H, Tan M, Zhao F, et al. Two potential syphilis vaccine candidates inhibit dissemination of Treponema pallidum. Front Immunol. (2021) 12:759474. doi: 10.3389/fimmu.2021.759474
143. Lithgow KV, Hof R, Wetherell C, Phillips D, Houston S, Cameron CE, et al. Defined syphilis vaccine candidate inhibits dissemination of Treponema pallidum subspecies pallidum. Nat Commun. (2017) 8:14273. doi: 10.1038/ncomms14273
144. He Y, Chen D, Fu Y, Huo X, Zhao F, Yao L, et al. Immunization with Tp0954, an adhesin of Treponema pallidum, provides protective efficacy in the rabbit model of experimental syphilis. Front Immunol. (2023) 14:1130593. doi: 10.3389/fimmu.2023.1130593
145. Kojima N, Konda KA, Klausner JD. Notes on syphilis vaccine development. Front Immunol. (2022) 13:952284. doi: 10.3389/fimmu.2022.952284
146. Chen J, Huang J, Liu Z, Xie Y. Treponema pallidum outer membrane proteins: current status and prospects. Pathog Dis. (2022) 80:23. doi: 10.1093/femspd/ftac023
147. Lorenz Z, Rybolt L, Ghanem KG, Shiroky-Kochavi J. A patient with secondary syphilis following incomplete treatment of primary infection. Lancet Infect Dis. (2023). doi: 10.1016/S1473-3099(23)00211-6
148. De Ambrogi M. Turning the spotlight on sexually transmitted infections. Lancet Infect Dis. (2017) 17:792–3. doi: 10.1016/S1473-3099(17)30363-8
149. Barry MJ, Nicholson WK, Silverstein M, Chelmow D, Coker TR, Davis EM, et al. Preexposure prophylaxis to prevent acquisition of HIV: us preventive services task force recommendation statement. JAMA. (2023) 330:736–45. doi: 10.1001/jama.2023.14461
150. Traeger MW, Cornelisse VJ, Asselin J, Price B, Roth NJ, Willcox J, et al. Association of HIV preexposure prophylaxis with incidence of sexually transmitted infections among individuals at high risk of HIV infection. JAMA. (2019) 321:1380–90. doi: 10.1001/jama.2019.2947
151. Blumenthal J, Haubrich RH. Will risk compensation accompany pre-exposure prophylaxis for HIV? Virtual Mentor. (2014) 16:909–15. doi: 10.1001/virtualmentor.2014.16.11.stas1-1411
152. Streeck H, Jansen K, Crowell TA, Esber A, Jessen HK, Cordes C, et al. HIV pre-exposure prophylaxis was associated with no impact on sexually transmitted infection prevalence in a high-prevalence population of predominantly men who have sex with men, Germany, 2018 to 2019. Euro Surveill. (2022) 27:591. doi: 10.2807/1560-7917.ES.2022.27.14.2100591
153. Padilla M, Gutierrez M, Basu M, Fagan J. Attitudes and beliefs about hiv treatment as prevention among people who are not engaged in Hiv care, 2018-2019. AIDS Behav. (2023) 27:3122–32. doi: 10.1007/s10461-023-04032-0
154. Moseley P, Bamford A, Eisen S, Lyall H, Kingston M, Thorne C, et al. Resurgence of congenital syphilis: new strategies against an old foe. Lancet Infect Dis. (2023). doi: 10.1016/S1473-3099(23)00314-6
Keywords: neurosyphilis, Treponema pallidum, HIV, susceptibility, pathogenesis, antibiotic treatment, prevention
Citation: Wu S, Ye F, Wang Y and Li D (2024) Neurosyphilis: insights into its pathogenesis, susceptibility, diagnosis, treatment, and prevention. Front. Neurol. 14:1340321. doi: 10.3389/fneur.2023.1340321
Received: 17 November 2023; Accepted: 27 December 2023;
Published: 11 January 2024.
Edited by:
U. K. Misra, Sanjay Gandhi Post Graduate Institute of Medical Sciences (SGPGI), IndiaReviewed by:
Mukesh Kumar, T. S. Misra Medical College and Hospital, IndiaPradeep Nair, Jawaharlal Institute of Postgraduate Medical Education and Research (JIPMER), India
Copyright © 2024 Wu, Ye, Wang and Li. This is an open-access article distributed under the terms of the Creative Commons Attribution License (CC BY). The use, distribution or reproduction in other forums is permitted, provided the original author(s) and the copyright owner(s) are credited and that the original publication in this journal is cited, in accordance with accepted academic practice. No use, distribution or reproduction is permitted which does not comply with these terms.
*Correspondence: Dongdong Li, amlhbmd4aWxpMTIxOSYjeDAwMDQwOzE2My5jb20=