- 1Department of Neurology, Shandong Provincial Hospital, Shandong University, Jinan, Shandong, China
- 2Department of Neurology, Shandong Provincial Hospital Affiliated to Shandong First Medical University, Jinan, Shandong, China
- 3Shandong Provincial Clinical Research Center for Neurological Diseases, Jinan, Shandong, China
- 4Shandong Provincial Key Laboratory of Network Based Intelligent Computing, School of Information Science and Engineering, University of Jinan, Jinan, Shandong, China
- 5Aging Research Center and Center for Alzheimer Research, Department of Neurobiology, Care Sciences and Society, Karolinska Institutet-Stockholm University, Stockholm, Sweden
Objective: To explore the associations of macular microvascular parameters with cerebral small vessel disease (CSVD) in rural-dwelling older adults in China.
Methods: This population-based cross-sectional study included 195 participants (age ≥ 60 years; 57.4% women) in the optical coherence tomographic angiography (OCTA) sub-study within the Multimodal Interventions to delay Dementia and disability in rural China (MIND-China). Macular microvascular parameters were measured using the OCTA. We automatically estimated volumes of gray matter, white matter, and white matter hyperintensity (WMH), and manually assessed numbers of enlarged perivascular spaces (EPVS) and lacunes on brain magnetic resonance imaging. Data were analyzed with the general linear models.
Results: Adjusting for multiple confounders, lower vessel skeleton density (VSD) and higher vessel diameter index (VDI) were significantly associated with larger WMH volume (P < 0.05). Lower VSD and foveal density-300 (FD-300) of left eye were significantly associated with lower brain parenchymal volume (P < 0.05). In addition, lower areas of foveal avascular zone (FAZ) and FD-300 of left eye were significantly associated with more EPVS (P < 0.05). The associations of abnormal macular microvascular parameters with WMH volume were evident mainly among females. Macular microvascular parameters were not associated with lacunes.
Conclusion: Macular microvascular signs are associated with WMH, brain parenchymal volume, and EPVS in older adults. The OCTA-assessed macular microvascular parameters can be valuable markers for microvascular lesions in the brain.
Introduction
Cerebral small vessel disease (CSVD) refers to a group of pathological processes with various etiologies affecting the small arteries, venules, and capillaries of the brain (1). Previous studies have shown that CSVD is associated with clinical stroke and cognitive impairment (2). Therefore, it is important to identify simple, non-invasive, and inexpensive markers for CSVD.
Microvasculatures in the brain and retina are similar in embryologic origin, anatomic features, metabolic activities, patterns of vascularization, and extracellular deposits (3). Thus, the retinal microvasculature could be a potential vehicle for studying changes in the cerebral vasculature. Some population-based studies have examined the associations of alterations in retinal microvasculature and imaging markers of CSVD, in which retinal microvascular signs (e.g., retinal focal arteriolar narrowing and arteriovenous nicking) were assessed using fundus photography (4, 5). However, due to limited resolution and sensitivity, traditional fundus imaging techniques can only qualitatively measure signs of microangiopathy (e.g., micro-hemorrhages and micro-aneurysms) or quantitatively measure arteriolar and venular parameters, but cannot directly quantify parameters of the capillary system (e.g., vessel density and vessel diameter index) (6). As a non-invasive and label-free technique, optical coherence tomography angiography (OCTA) can quantitatively detect the movement of red blood cells at capillary-level resolution and offer imaging markers for retinal microvascular signs, such as retinal vessel density (VD), foveal avascular zone (FAZ), and vessel diameter index (VDI) (6). Previous studies showed that VD was related to the CSVD burden or global white matter hyperintensity (WMH) (7, 8). However, most of these studies have been conducted in the clinical settings of patients with mild cognitive impairment or Alzheimer's disease (AD), and data from the general population settings are sparse. In addition, cerebral WMH is typically located in paraventricular and deep white matter regions, and etiopathological mechanisms of WMH differ depending on their location (9). However, the potential differential associations of macular microvascular parameters with paraventricular and deep WMH have rarely been explored. Furthermore, the sex differences in the associations of retinal microvascular signs with CSVD in older adults remain to be clarified. This is important because sex differences in CSVD and retinal capillary plexus have been frequently reported (10, 11) and there are substantial sex differences in the prevalence of lifestyle risk factors (e.g., smoking and alcohol consumption), especially among rural older adults in China (12, 13).
Thus, in this population-based study, we aimed to investigate the associations of macular microvascular signs with markers of CSVD among dementia-free older adults who were living in the rural communities in China. We hypothesize that macular microvascular signs are associated with CSVD markers and that the associations may vary by sex.
Methods
Study design and participants
This population-based cross-sectional study used data from the baseline survey of the Multimodal Interventions to delay Dementia and disability in rural China (MIND-China), which is part of the World-Wide FINGERS Network, as previously reported (14). In brief, baseline assessments of MIND-China targeted people who were aged ≥ 60 years by the end of 2017 and living in the 52 villages of Yanlou Town, Yanggu County, western Shandong Province, China. In March–September 2018, 5,765 participants (57.2% female) were examined during the baseline survey of MIND-China (15).
From June 2019 to November 2020, 284 participants accomplished the brain MRI and OCTA examination in Southwest Lu Hospital. The cluster (village)-randomized sampling approach was used in the selection of participants for the MRI and OCTA substudies in MIND-China. Of these, 47 were excluded due to missing data on OCTA images of left eye (n = 30) or right eye (n = 17). We further excluded 42 participants due to prevalent dementia (n = 2), and suboptimal quality of OCTA images (n = 31) and brain MRI images (n = 9). Therefore, the final analytical sample included 195 dementia-free participants. Compared to the MIND-China participants who were not included in this study (n = 5,570), those in the analytical sample (n = 195) were slightly younger (mean age 67.95 vs. 70.99 years, P < 0.001) and more educated (mean years of schooling education 3.66 vs. 3.16 years, P = 0.041), but the two groups did not differ significantly in the distribution of sex (female 57.44 vs. 57.18%, P = 0.944).
The MIND-China study was approved by the Ethics Committee at Shandong Provincial Hospital. All participants were informed of the study protocol in detail and signed the informed consent form. Research within MIND-China was conducted in accordance with the ethical principles expressed in the Declaration of Helsinki. MIND-China was registered in the Chinese Clinical Trial Registry (Registration No. ChiCTR1800017758).
Data collection and assessments
In March-September 2018, the trained research staff collected data through face-to-face interviews, clinical examinations, neuropsychological tests, and laboratory tests, as part of the baseline examinations of MIND-China (15). The data included demographic features (e.g., age, sex, and education), lifestyles (e.g., smoking and alcohol consumption), health history (e.g., hypertension, diabetes, and dyslipidemia). Smoking and alcohol intake were classified as never and ever smoking or consuming alcohol. The data collection and assessments were previously described in detail (15).
MRI acquisition and assessment protocols
Participants were scanned on the Philips Ingenia 3.0T MR System in the Southwestern Lu Hospital. The MRI protocols (acquisition, sequences, processing, and quantification) were previously described in detail (15, 16). The protocol included a sagittal three-dimensional T1-weighted fast field echo sequence, an axial T2-weighted fast field echo sequence, and a sagittal three-dimensional fluid attenuated inversion recovery (FLAIR) sequence.
We used AccuBrain® (BrainNow Medical Technology Ltd., Shenzhen, Guangdong, China) to assess WMH volume, brain parenchymal volume, and total intracranial volume (ICV), as previously reported (17). AccuBrain® segmented the T1-weighted images and quantified the volumes of gray matter, white matter, and cerebrospinal fluid based on the principle of similarity measures. The ICV was estimated as the sum of gray matter, white matter, and cerebrospinal fluid volume. The brain parenchymal volume was estimated as the sum of gray matter and white matter volume.
To acquire WMH volume, AccuBrain® used T2- FLAIR images to calculate the signal contrast between normal brain tissue and WMH, and set the signal threshold to recognize WMH. Based on predefined threshold, WMH was recognized and extracted using T2-FLAIR images. Finally, AccuBrain® refined and localized WMH using the transformed T1-weighted brain structure mask extracted from our study sample.
Perivascular spaces (PVS) were microscopic fluid-filled structures that surround the small penetrating blood vessels in the brain. The number of enlarged perivascular spaces (EPVS) was counted on T2-weighted images according to a validated protocol (18). The EPVS appear linear when imaged parallel to the course of the vessel, and round or ovoid (diameters <3 mm) when imaged perpendicular to the course of the vessel. EPVS of the basal ganglia (BG) and centrum semiovale (CSO) were visually counted by the trained rater (M.Z.) who was unaware of the clinical information, under the supervision of an experienced clinical neurologist (L.S). The rater first reviewed all MRI slices showing BG and CSO regions and then counted the number of EPVS on the slices with the highest number of EPVS (18). Three months after the initial assessment, EPVS were reassessed on MRI images from 30 randomly selected subjects, and the intra-evaluator correlation coefficients for BG-EPVS and CSO-EPVS were 0.88 and 0.83, respectively. The total number of global EPVS (i.e., BG-EPVS plus CSO-EPVS) was used in our analysis.
Lacune was defined as a region of abnormal signal intensity in a vascular distribution, 3–15 mm in size with a cerebrospinal fluid density on the subtraction image (19). Lacunes were counted on FLAIR images by the trained rater (J.W.) who was blind to clinical information, under the supervision of an experienced clinical neurologist (L.S). Three months after the initial assessment, lacunes were reassessed on MRI images of 200 randomly selected subjects from all participants in MIND-China who undertook brain MRI scans, and the intra-rater correlation coefficients for lacunes was 0.838.
Optical coherence tomography angiography acquisition and assessment
The OCTA examination was conducted by ophthalmologists in Southwestern Lu Hospital using Spectralis HRA+OCT (Software Version 1.10.2.0; Heidelberg Engineering, Inc., Heidelberg, Germany). Macular OCTA images of 6 × 6 mm areas (10° × 10° scan angle that included 512 A-scans × 512 B-scans, no pre-determined automatic real time) centered at the fovea were acquired in a dark room without pupil dilation. We scanned the right eye first and then the left eye. We excluded images of suboptimal quality due to eye movement, decentration, defocus, shadow, Z offset, and segmentation error (20). OCTA images of both eyes were included and analyzed in this study. Because OCTA signals in the deeper layers carry the shadows of blood vessels from the superficial plexus, our analysis only focused on the superficial vascular plexus (extending from the inner limiting membrane to 17 mm above the inner plexiform layer) (21).
All macular OCTA images were processed into a binary image in MATLAB (R2019a, MathWorks, Inc.) using a global threshold, hessian filter, and adaptive threshold. Macular microvascular parameters, including vascular density (e.g., VD, VSD, and FD-300) and vascular morphology (e.g., FAZ and VDI), were calculated. FAZ area was defined as the area of the avascular region in the center of the blood flow image (22). VD was calculated as the ratio of the total image area occupied by the blood flow signal to the total image area in the binary vessel maps (subtract FAZ area) (22). VSD was calculated as the ratio of the length occupied by the blood flow signal to the total area in the skeletonized vessel map (subtract FAZ area) (22). VDI, defined as the average diameter of blood vessels, was calculated as the ratio of the area covered by blood flow signal to the length of vessel skeletonization (23). FD-300 was defined as the foveal vessel density in a 300-μm wide zone around the FAZ (24).
Statistical analysis
We reported frequencies (%) for categorical variables and mean (SD) for continuous variables. Characteristics of study participants by sex were compared using non-parametric test for continuous variables, and chi-square test for categorical variables. General linear models were used to examine the associations of macular microvascular parameters with WMH volume, number of lacunes, brain parenchymal volume, and EPVS count. WMH volume and numbers of lacunes and EPVS were log-transformed due to the skewed distribution. By simultaneously entering the independent variables and their cross-product term into the same model, we tested the statistical interactions of retinal microvascular parameters with sex on CSVD and when statistically significant interaction was detected, we further performed stratified analyses by sex. We reported the main results from two models: model 1 was adjusted for age, sex, and education, if applicable, for ICV; and model 2 was additionally adjusted for alcohol consumption, smoking status, hypertension, diabetes, and dyslipidemia. IBM SPSS Statistics for Windows, Version 25.0 (IBM Corp., Armonk, NY, USA) was used for all the analyses. A two-tailed P < 0.05 was considered to be statistically significant.
Results
Characteristics of the study participants
The mean age of the 195 participants in the analytical sample was 67.95 (SD = 4.01) years, and 57.4% were females. Compared with females, male participants were more educated, and more likely to smoke and drink alcohol, but less likely to have dyslipidemia (P < 0.05). In addition, male participants had a smaller FAZ area on both eyes and a smaller brain parenchymal volume but a larger ICV volume (P < 0.05; Table 1). The two groups did not differ significantly in mean age, FD-300, VD, VSD, VDI (on both eyes), global WMH volume, periventricular WMH (PWMH) volume, deep WMH (DWMH) volume, numbers of EPVS and lacunes, and in the prevalence of diabetes and hypertension (P > 0.05).
Associations of macular microvascular parameters with global cerebral small vessel disease
Adjusting for age, sex, education, and if applicable, for ICV, lower VSD but higher VDI were significantly associated with higher global WMH volume (P < 0.05); Lower FD-300 and VSD of the left eye were significantly associated with lower brain parenchymal volume (P < 0.05); Lower FD-300 and FAZ areas of the left eye were significantly associated with more EPVS (P < 0.05); these associations remained statistically significant even after further controlling for dyslipidemia, diabetes, hypertension, smoking, and alcohol consumption (P < 0.05; Table 2). The number of lacunes was not significantly associated with any of the examined macular microvascular parameters (P > 0.05; Table 2).
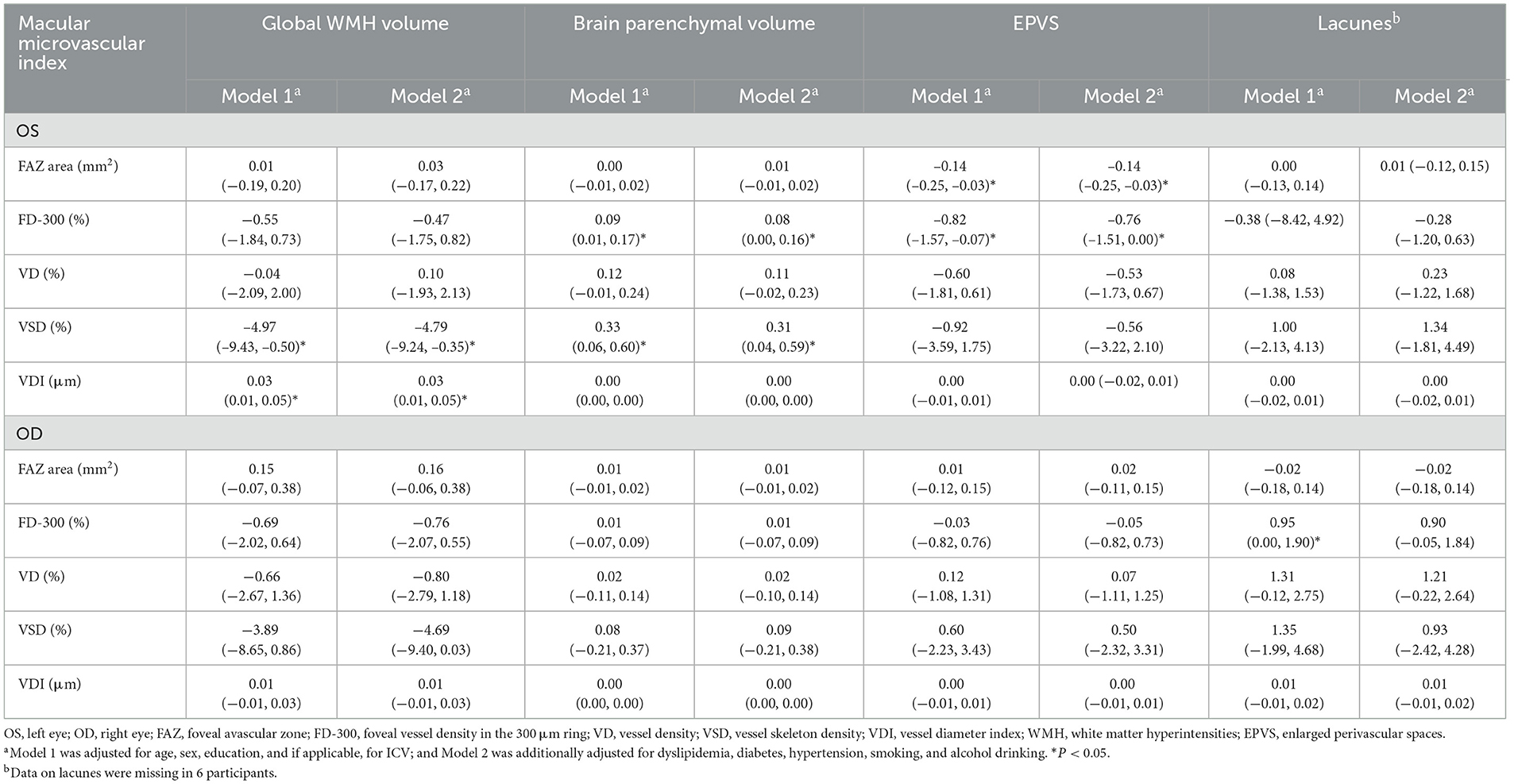
Table 2. β coefficient (95% confidence interval) of cerebral small vessel disease associated with macular microvascular parameters (n = 195).
Associations of macular microvascular parameters with regional WMH volume
We further examined the associations of macular microvascular parameters with volumes of periventricular and deep WMH. Controlling for age, sex, education, and ICV, lower VSD and higher VDI of the left eye were significantly associated with larger PWMH volume (P < 0.05); the associations remained statistically significant even after further controlling for dyslipidemia, diabetes, hypertension, smoking, and alcohol consumption (P < 0.05; Table 3). In addition, lower VSD of the right eye was significantly associated with larger PWMH volume, even in the fully-adjusted model (P < 0.05; Table 3). There was no significant association between macular microvascular parameters and DWMH volume (P > 0.05; Table 3).
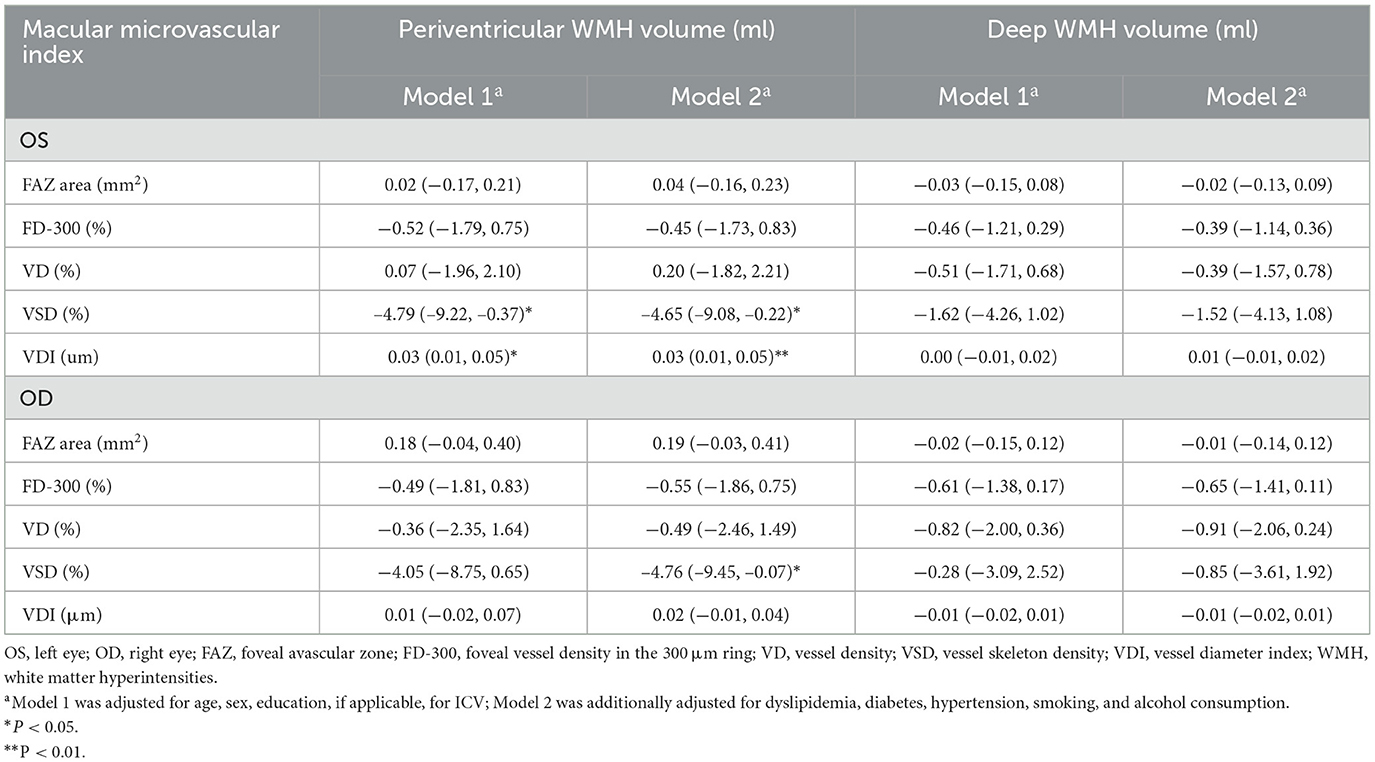
Table 3. β coefficient (95% confidence interval) of deep and periventricular white matter hyperintensity volume associated with macular microvascular parameters (n = 195).
Interactions of macular microvascular parameters with sex on cerebral small vessel disease
We found statistical interactions of macular microvascular parameters (e.g., VSD and FD-300) with sex on the global WMH and PWMH volumes (P-interactions < 0.05). Further analyses stratified by sex showed that macular microvascular parameters (e.g., VSD and FD-300) were significantly associated with higher global WMH and PWMH volumes only among females not in males (P < 0.05; Figure 1).
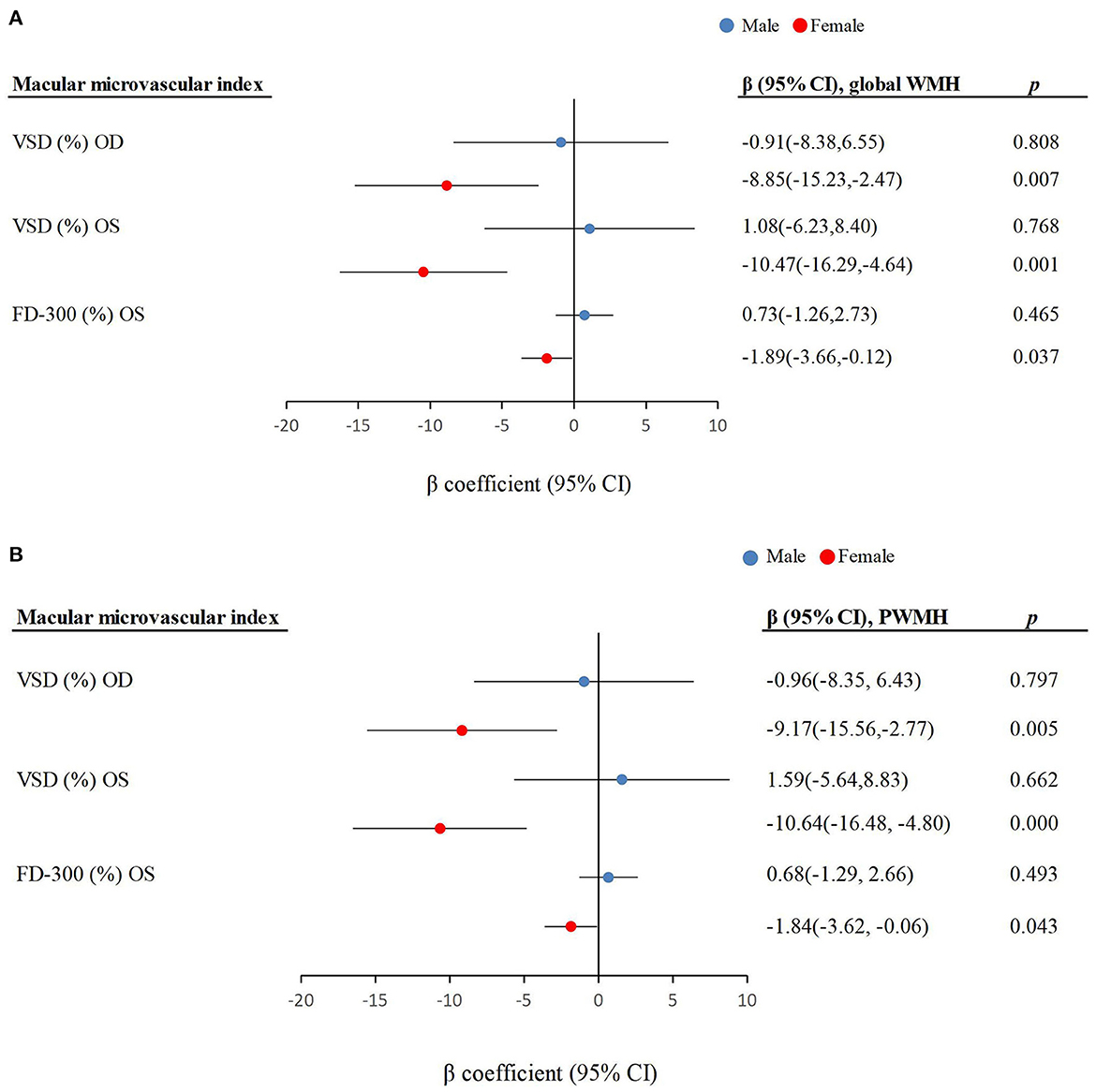
Figure 1. Associations of macular microvascular parameters with (A) global white matter hyperintensity volume and (B) periventricular white matter hyperintensity volume by sex (n = 195). CI, confidence interval; OD, right eye; OS, left eye; VSD, vessel skeleton density; FD-300, foveal vessel density in the 300 μm ring; PWMH, periventricular white matter hyperintensity.
Discussion
In this population-based cross-sectional study of rural-dwelling Chinese older adults, we found that macular microvascular parameters (e.g., FAZ, FD-300, VSD, and VDI), especially in the left eye, were associated with signs of CSVD (e.g., WMH volume, EPVS count, and brain parenchymal volume). Furthermore, macular microvascular parameters (e.g., VSD and VDI) were associated with larger PWMH volume but not DWMH volume. Our study also revealed that the associations of macular vascular parameters (e.g., VSD and VDI) with volumes of global WMH and PWMH were only evident among females.
The associations between macular microvascular parameters and signs of CSVD have been reported in several case-control studies, but the findings were inconsistent (7, 25). The lower vascular density is indicative of reduced retinal blood flow. Our study indicated that lower vascular density (e.g., VSD and FD-300) was associated with higher global WMH volume and more EPVS, which were in line with the previous clinical-based studies (26, 27). Furthermore, we revealed that lower VSD and FD-300 were strongly associated with lower brain parenchymal volume, which has rarely been reported in previous studies. The FAZ area is most sensitive to ischemia and enlargement of FAZ area indicates macular ischemia (28). Our findings suggested that lower FAZ area was associated with more EPVS. However, a clinical-based case-control study in Shanghai found no association between FAZ area and EPVS count, which may be partially due to the fact that the case-control study included young adults (age ≥ 18 years) (26). VDI, which reflects vascular dilation, represents the average vessel caliber regardless of the vessel length (22). Our data suggested that higher VDI was associated with higher global WMH volume. This may hypothetically be due to the loss of smaller capillaries or compensatory vasodilation of the perfused capillaries secondary to a more hypoxic environment and increased local inflammatory molecules (29, 30). Previously, a population-based cohort study has linked the larger venular diameters with the progression of WMH (5). By contrast, the small-scale case-control study (n = 64) in Wuhan, China found no association between VDI and WMH (e.g., PWMH and DWMH), partly due to limited statistical power (31). Taken together, the findings from our population-based study and previous case-control studies suggest that macular microvascular parameters can be valuable markers for microvascular lesions in the brain.
PWMH and DWMH have distinct etiopathogenic mechanisms. PWMH is more likely to be determined by chronic hemodynamic insufficiency, whereas DWMH may be more attributed to small vessel disease (32). In line with this view, two hospital-based case-control studies suggested that lower vessel density was associated with severity of DWMH but not PWMH (26, 31). However, our study indicated that lower VSD and higher VDI were associated with higher PWMH volume, but not with DWMH volume. The discrepancy across studies may be partly attributed to differences in the study settings (e.g., clinical vs. the general population setting) and characteristics of study populations (e.g., young adults vs. older adults). Thus, further longitudinal studies with larger sample sizes are needed to clarify the potential causal relationship between macular microvascular parameters and WMH by anatomic locations.
In this study, we further examined the interactions of macular microvascular parameters with sex on CSVD markers and found that the associations of macular vascular parameters (e.g., VSD and VDI) with volumes of global WMH and PWMH were only evident among females. This was consistent with the previous community-based study, which showed that associations between WMH severity and arteriovenous nicking were stronger in women than in men (33). It has been hypothesized that estrogens can increase tissue perfusion of the retina and brain probably by reducing vascular resistance, protecting against oxidative stress, and stimulating synaptogenesis in premenopausal women (34, 35). However, estrogen levels decline markedly after the menopause, the vascular protection of estrogen disappears and women become more susceptible to vascular changes and diseases in the brain (34, 36).
Another interesting observation from our study is that the associations between macular microvascular parameters and CSVD were evident mainly in the left eye. This is in agreement with a hospital-based study of adults (age ≥ 18 years) in Beijing, which showed that lower VD of the left eye, but not right eye, was associated with higher burdens of WMH and EPVS (37). This may partially be attributed to structural and functional brain asymmetry occurred during normal human brain development (32, 38). Furthermore, previous studies also showed that macular microvascular signs of left eye reflect function and connectivity of the right hemisphere (39) and the small vascular lesions of the right hemisphere occur earlier and are much more severer than those of the left hemisphere (40). Finally, there was evidence showing that the left eye appeared to be more sensitive to damage due to hypoxia compared with the right eye (41).
The pathophysiologic mechanisms underlying the associations of macular microvascular parameters with CSVD are not fully understood but can be speculated. First, anatomically, the retina is regarded as an extension of the diencephalon and has a similar pattern of angiogenesis. Physiologically, similar to the brain, the retina has a highly isolated and protected vascular system (3, 42). Second, retinal and brain microvascular lesions share common cardiovascular risk factors (43). Third, dysfunction of the blood-retina barrier from retina hypoxia owing to long-term exposures to cardiovascular risk factors, which is analogous to and associated with the blood-brain barrier dysfunction, might play a role in the pathogenesis of both retinal microvascular changes and CSVD (42, 44). Finally, inflammation and endothelial dysfunction may also be involved in the process of retinal and cerebral microangiopathy (29). Given the homology of microvasculature in the brain and the retina, the link of macular microvascular parameters with signs of CSVD may reflect underlying pathological processes common in both the brain and the retina (25, 27).
The major strengths of our study include the population-based design and comprehensive assessments of macular microvascular and CSVD indicators. Our study also has limitations. First, the cross-sectional study cannot determine a causal relationship. Second, the study sample was relatively small and the statistical power might not be big enough to detect weak-to-moderately strong associations between retinal parameters and markers of CSVD. Third, data on cerebral microbleeds and microinfarcts were not available, which might underestimate the associations of macular microvascular parameters with CSVD. Finally, the study participants were derived from just one rural area and they were also slightly younger and more educated than the MIND-China total sample, which might not be representative of rural population in China. These should be borne in mind when extrapolating the findings to other rural populations.
Conclusions
In summary, our population-based study of rural-dwelling older adults showed that macular microvascular parameters were independently associated with CSVD markers, and that the observed associations with WMH volume were evident mainly among females. This suggests that quantitative macular microvascular parameters could be useful markers for CSVD. These findings may have potential implications for clinical management of macular microvascular signs in older adults. For instance, ophthalmologists should be aware of the potential link of macular microvascular signs with brain lesions. Structural brain MRI examination may be considered when abnormal macular microvascular signs were detected, especially among older women. Future large-scale prospective cohort studies are warranted to clarify the potential causal relationships of macular microvascular signs with CSVD markers as well as the mechanisms underlying their associations. In addition, the functional consequences (e.g., cognitive and physical dysfunction) of macular microvascular signs among older adults deserve further investigation.
Data availability statement
The raw data supporting the conclusions of this article will be made available by the authors, without undue reservation.
Ethics statement
The studies involving human participants were reviewed and approved by Ethics Committee at Shandong Provincial Hospital. The patients/participants provided their written informed consent to participate in this study.
Author contributions
ZX, QZ, YDu, and CQ: study concept and design. ZX, YW, LS, SN, SW, MZ, JW, YDo, TH, LC, ST, and XH: data collection and assessments. ZX: data analysis and writing the first draft of the manuscript. YDu and CQ: study supervision. All authors have critically revised the manuscript for important intellectual content and approved the final manuscript.
Funding
MIND-China was supported in part by grants from the National Natural Science Foundation of China (Grants Nos. 82171175, 81861138008, and 81772448), the Alzheimer's Association Grant (Grant No. AACSFD-22-922844), the National Key R&D Program of China Ministry of Sciences and Technology (Grant No. 2017YFC1310100), the Natural Science Foundation of Shandong Province (Grant No. ZR2021MH005), the Academic Promotion Program of Shandong First Medical University (Grant No. 2019QL020), the Integrated Traditional Chinese and Western Medicine Program in Shandong Province (Grant No. YXH2019ZXY008), and the Brain Science and Brain-like Intelligence Technology Research Projects of China (Grant Nos. 2021ZD0201801 and 2021ZD0201808). LC received grants from the Shandong Provincial Key Research and Development Program (Grant No. 2021LCZX03). CQ received grants from the Swedish Research Council (Grant Nos. 2017-05819 and 2020-01574), the Swedish Foundation for International Cooperation in Research and Higher Education (Grant No. CH2019-8320), and Karolinska Institutet (Grant Nos. 2018-01854 and 2020-01456), Stockholm, Sweden. The funding agency had no role in the study design, data collection and analysis, the writing of this manuscript, and in the decision to submit the work for publication.
Acknowledgments
We would like to thank all the participants of the MIND-China Project as well as staff at the Yanlou Town Hospital and Shandong Provincial Hospital who were involved in the data collection and management.
Conflict of interest
The authors declare that the research was conducted in the absence of any commercial or financial relationships that could be construed as a potential conflict of interest.
Publisher's note
All claims expressed in this article are solely those of the authors and do not necessarily represent those of their affiliated organizations, or those of the publisher, the editors and the reviewers. Any product that may be evaluated in this article, or claim that may be made by its manufacturer, is not guaranteed or endorsed by the publisher.
References
1. Pantoni L. Cerebral small vessel disease: from pathogenesis and clinical characteristics to therapeutic challenges. Lancet Neurol. (2010) 9:689–701. doi: 10.1016/S1474-4422(10)70104-6
2. Debette S, Beiser A, DeCarli C, Au R, Himali JJ, Kelly-Hayes M, et al. Association of mri markers of vascular brain injury with incident stroke, mild cognitive impairment, dementia, and mortality: the framingham offspring study. Stroke. (2010) 41:600–6. doi: 10.1161/STROKEAHA.109.570044
3. London A, Benhar I, Schwartz M. The retina as a window to the brain-from eye research to Cns disorders. Nat Rev Neurol. (2013) 9:44–53. doi: 10.1038/nrneurol.2012.227
4. Qiu C, Cotch MF, Sigurdsson S, Klein R, Jonasson F, Klein BEK, et al. Microvascular lesions in the brain and retina: the age, gene/environment susceptibility-reykjavik study. Ann Neurol. (2009) 65:569–76. doi: 10.1002/ana.21614
5. Ikram MK, De Jong FJ, Van Dijk EJ, Prins ND, Hofman A, Breteler MMB, et al. Retinal vessel diameters and cerebral small vessel disease: the Rotterdam scan study. Brain. (2006) 129:182–8. doi: 10.1093/brain/awh688
6. Spaide RF, Fujimoto JG, Waheed NK, Sadda SR, Staurenghi G. Optical coherence tomography angiography. Prog Retin Eye Res. (2018) 64:3. doi: 10.1016/j.preteyeres.2017.11.003
7. Lee J-Y, Kim JP, Jang H, Kim J, Kang SH, Kim JS, et al. Optical coherence tomography angiography as a potential screening tool for cerebral small vessel diseases. Alzheimers Res Ther. (2020) 12:73. doi: 10.1186/s13195-020-00638-x
8. Peng C, Kwapong WR, Xu S, Muse FM, Yan J, Qu M, et al. Structural and microvascular changes in the macular are associated with severity of white matter lesions. Front Neurol. (2020) 11:521. doi: 10.3389/fneur.2020.00521
9. Wardlaw JM, Smith EE, Biessels GJ, Cordonnier C, Fazekas F, Frayne R, et al. Neuroimaging standards for research into small vessel disease and its contribution to ageing and neurodegeneration. Lancet Neurol. (2013) 12:822–38. doi: 10.1016/S1474-4422(13)70124-8
10. Jiménez-Sánchez L, Hamilton OKL, Clancy U, Backhouse EV, Stewart CR, Stringer MS, et al. Sex differences in cerebral small vessel disease: a systematic review and meta-analysis. Front Neurol. (2021) 12:756887. doi: 10.3389/fneur.2021.756887
11. Su B, Zhu X, Yang K, Xiao Y, Li C, Shi K, et al. Age- and sex-related differences in the retinal capillary plexus in healthy Chinese adults. Eye Vis. (2022) 9:38. doi: 10.1186/s40662-022-00307-0
12. Song A, Liang Y, Yan Z, Sun B, Cai C, Jiang H, et al. Highly prevalent and poorly controlled cardiovascular risk factors among chinese elderly people living in the rural community. Eur J Prev Cardiol. (2014) 21:1267–74. doi: 10.1177/2047487313487621
13. Han X, Jiang Z, Li Y, Wang Y, Liang Y, Dong Y, et al. Sex disparities in cardiovascular health metrics among rural-dwelling older adults in China: a population-based study. BMC Geriatr. (2021) 21:158. doi: 10.1186/s12877-021-02116-x
14. Kivipelto M, Mangialasche F, Snyder HM, Allegri R, Andrieu S, Arai H, et al. World-wide fingers network: a global approach to risk reduction and prevention of dementia. Alzheimers Dement. (2020) 16:1078–94. doi: 10.1002/alz.12123
15. Wang Y, Han X, Zhang X, Zhang Z, Cong L, Tang S, et al. Health status and risk profiles for brain aging of rural-dwelling older adults: data from the interdisciplinary baseline assessments in mind-China. Alzheimers Dement (N Y). (2022) 8:e12254. doi: 10.1002/trc2.12254
16. Wang M, Li Y, Cong L, Hou T, Luo Y, Shi L, et al. High-density lipoprotein cholesterol and brain aging amongst rural-dwelling older adults: a population-based magnetic resonance imaging study. Eur J Neurol. (2021) 28:2882–92. doi: 10.1111/ene.14939
17. Abrigo J, Shi L, Luo Y, Chen Q, Chu WCW, Mok VCT. Standardization of hippocampus volumetry using automated brain structure volumetry tool for an initial Alzheimer's disease imaging biomarker. Acta Radiol. (2019) 60:769–76. doi: 10.1177/0284185118795327
18. Potter GM, Chappell FM, Morris Z, Wardlaw JM. Cerebral perivascular spaces visible on magnetic resonance imaging: development of a qualitative rating scale and its observer reliability. Cerebrovasc Dis. (2015) 39:224–31. doi: 10.1159/000375153
19. Zhang J, Han F, Liang X, Li M, Zhang D, Zhai F, et al. Lacune and large perivascular space: two kinds of cavities are of different risk factors and stroke risk. Cerebrovasc Dis. (2020) 49:522–30. doi: 10.1159/000508732
20. Kamalipour A, Moghimi S, Hou H, Penteado RC, Oh WH, Proudfoot JA, et al. Oct angiography artifacts in glaucoma. Ophthalmology. (2021) 128:1426–37. doi: 10.1016/j.ophtha.2021.03.036
21. Sampson DM, Dubis AM, Chen FK, Zawadzki RJ, Sampson DD. Towards standardizing retinal optical coherence tomography angiography: a review. Light Sci Appl. (2022) 11:63. doi: 10.1038/s41377-022-00740-9
22. Kalra G, Zarranz-Ventura J, Chahal R, Bernal-Morales C, Lupidi M, Chhablani J. Optical coherence tomography (Oct) angiolytics: a review of oct angiography quantitative biomarkers. Surv Ophthalmol. (2022) 67:1118–34. doi: 10.1016/j.survophthal.2021.11.002
23. Xu Q, Sun H, Huang X, Qu Y. Retinal microvascular metrics in untreated essential hypertensives using optical coherence tomography angiography. Graefes Arch Clin Exp Ophthalmol. (2021) 259:395–403. doi: 10.1007/s00417-020-04714-8
24. Inanc M, Tekin K, Kiziltoprak H, Ozalkak S, Doguizi S, Aycan Z. Changes in retinal microcirculation precede the clinical onset of diabetic retinopathy in children with type 1 diabetes mellitus. Am J Ophthalmol. (2019) 207:37–44. doi: 10.1016/j.ajo.2019.04.011
25. Tao W, Kwapong WR, Xie J, Wang Z, Guo X, Liu J, et al. Retinal microvasculature and imaging markers of brain frailty in normal aging adults. Front Aging Neurosci. (2022) 14:945964. doi: 10.3389/fnagi.2022.945964
26. Fu W, Zhou X, Wang M, Li P, Hou J, Gao P, et al. Fundus changes evaluated by octa in patients with cerebral small vessel disease and their correlations: a cross-sectional study. Front Neurol. (2022) 13:843198. doi: 10.3389/fneur.2022.843198
27. Wang X, Wei Q, Wu X, Cao S, Chen C, Zhang J, et al. The vessel density of the superficial retinal capillary plexus as a new biomarker in cerebral small vessel disease: an optical coherence tomography angiography study. Neurol Sci. (2021) 42:3615–24. doi: 10.1007/s10072-021-05038-z
28. Bulut M, Kurtuluş F, Gözkaya O, Erol MK, Cengiz A, Akidan M, et al. Evaluation of optical coherence tomography angiographic findings in Alzheimer's type dementia. Br J Ophthalmol. (2018) 102:233–7. doi: 10.1136/bjophthalmol-2017-310476
29. Lenin R, Thomas SM, Gangaraju R. Endothelial activation and oxidative stress in neurovascular defects of the retina. Curr Pharm Des. (2018) 24:4742–54. doi: 10.2174/1381612825666190115122622
30. Wong TY, Islam FMA, Klein R, Klein BEK, Cotch MF, Castro C, et al. Retinal vascular caliber, cardiovascular risk factors, and inflammation: the multi-ethnic study of atherosclerosis (Mesa). Invest Ophthalmol Vis Sci. (2006) 47:2341–50. doi: 10.1167/iovs.05-1539
31. Zhou X, Li T, Qu W, Pan D, Qiu Q, Wu L, et al. Abnormalities of retinal structure and microvasculature are associated with cerebral white matter hyperintensities. Eur J Neurol. (2022) 29:2289–98. doi: 10.1111/ene.15378
32. Kim KW, MacFall JR, Payne ME. Classification of white matter lesions on magnetic resonance imaging in elderly persons. Biol Psychiatry. (2008) 64:273–80. doi: 10.1016/j.biopsych.2008.03.024
33. Longstreth W, Larsen EKM, Klein R, Wong TY, Sharrett AR, Lefkowitz D, et al. Associations between findings on cranial magnetic resonance imaging and retinal photography in the elderly: the cardiovascular health study. Am J Epidemiol. (2007) 165:78–84. doi: 10.1093/aje/kwj350
34. Nuzzi R, Scalabrin S, Becco A, Panzica G. Gonadal hormones and retinal disorders: a review. Front Endocrinol. (2018) 9:66. doi: 10.3389/fendo.2018.00066
35. Krause DN, Duckles SP, Pelligrino DA. Influence of sex steroid hormones on cerebrovascular function. J Appl Physiol. (2006) 101:1252–61. doi: 10.1152/japplphysiol.01095.2005
36. Lohner V, Pehlivan G, Sanroma G, Miloschewski A, Schirmer MD, Stöcker T, et al. Relation between sex, menopause, and white matter hyperintensities: the rhineland study. Neurology. (2022) 99:e935–e43. doi: 10.1212/WNL.0000000000200782
37. Ma L, Wang M, Chen H, Qu Y, Yang L, Wang Y. Association between retinal vessel density and neuroimaging features and cognitive impairment in cerebral small vessel disease. Clin Neurol Neurosurg. (2022) 221:107407. doi: 10.1016/j.clineuro.2022.107407
38. Saltoun K, Adolphs R, Paul LK, Sharma V, Diedrichsen J, Yeo BTT, et al. Dissociable brain structural asymmetry patterns reveal unique phenome-wide profiles. Nat Hum Behav. (2022) 7:251–68. doi: 10.1038/s41562-022-01461-0
39. Wang C, Burtis DB, Ding M, Mo J, Williamson JB, Heilman KM. The effects of left and right monocular viewing on hemispheric activation. J Clin Exp Neuropsychol. (2018) 40:198–204. doi: 10.1080/13803395.2017.1332169
40. Zhou H, Tang Y, Yuan Z. White matter asymmetries in patients with cerebral small vessel disease. J Integr Neurosci. (2018) 17:159–67. doi: 10.31083/JIN-170037
41. Garcia-Martin E, Ruiz-de Gopegui E, León-Latre M, Otin S, Altemir I, Polo V, et al. Influence of cardiovascular condition on retinal and retinal nerve fiber layer measurements. PLoS ONE. (2017) 12:e0189929. doi: 10.1371/journal.pone.0189929
42. Patton N, Aslam T, Macgillivray T, Pattie A, Deary IJ, Dhillon B. Retinal vascular image analysis as a potential screening tool for cerebrovascular disease: a rationale based on homology between cerebral and retinal microvasculatures. J Anat. (2005) 206:319–48. doi: 10.1111/j.1469-7580.2005.00395.x
43. Cheung CY-l, Ikram MK, Sabanayagam C, Wong TY. Retinal microvasculature as a model to study the manifestations of hypertension. Hypertension. (2012) 60:1094–103. doi: 10.1161/HYPERTENSIONAHA.111.189142
Keywords: macular microvascular signs, cerebral small vessel disease, optical coherence tomography angiography, magnetic resonance imaging, population-based study
Citation: Xu Z, Dong Y, Wang Y, Song L, Niu S, Wang S, Zhao M, Wang J, Cong L, Han X, Hou T, Tang S, Zhang Q, Du Y and Qiu C (2023) Associations of macular microvascular parameters with cerebral small vessel disease in rural older adults: A population-based OCT angiography study. Front. Neurol. 14:1133819. doi: 10.3389/fneur.2023.1133819
Received: 17 January 2023; Accepted: 27 February 2023;
Published: 16 March 2023.
Edited by:
Alon Harris, Icahn School of Medicine at Mount Sinai, United StatesReviewed by:
Anna Fabczak-Kubicka, Icahn School of Medicine at Mount Sinai, United StatesAharon Wegner, Technical University of Munich, Germany
Copyright © 2023 Xu, Dong, Wang, Song, Niu, Wang, Zhao, Wang, Cong, Han, Hou, Tang, Zhang, Du and Qiu. This is an open-access article distributed under the terms of the Creative Commons Attribution License (CC BY). The use, distribution or reproduction in other forums is permitted, provided the original author(s) and the copyright owner(s) are credited and that the original publication in this journal is cited, in accordance with accepted academic practice. No use, distribution or reproduction is permitted which does not comply with these terms.
*Correspondence: Qinghua Zhang, cindy-217@163.com; Yifeng Du, duyifeng2013@163.com