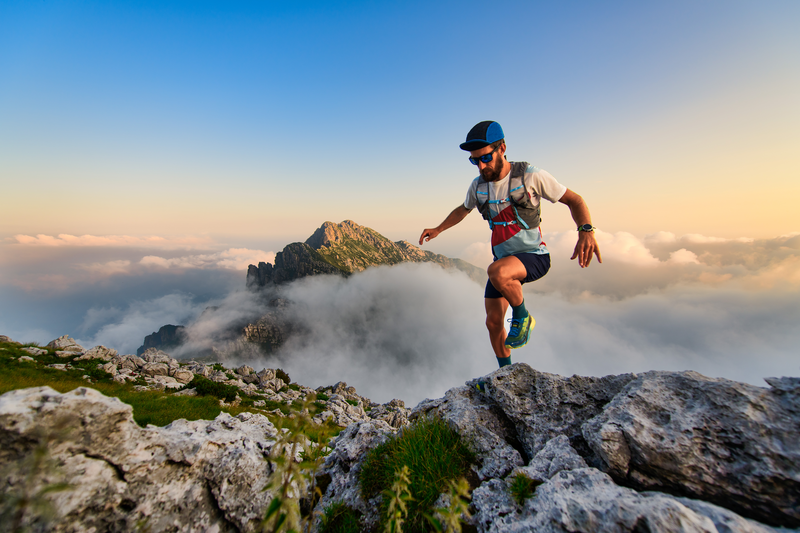
94% of researchers rate our articles as excellent or good
Learn more about the work of our research integrity team to safeguard the quality of each article we publish.
Find out more
ORIGINAL RESEARCH article
Front. Neurol. , 26 August 2022
Sec. Applied Neuroimaging
Volume 13 - 2022 | https://doi.org/10.3389/fneur.2022.946324
Objectives: To investigate the clinical characteristics of cerebral cavernous malformations (CCMs) with MAP3K3 somatic mutation.
Methods: We performed a retrospective review of our CCMs database between May 2017 and December 2019. The patients with simplex CCMs identified to harbor a MAP3K3 or CCM gene somatic mutation were included. Clinical characteristics were recorded. Univariate and multivariate logistic analyses were used to assess the risk factors associated with hemorrhage events of CCMs. To explore the underlying mechanism, we transfected MEKK3-I441M-overexpressing and CCM2-knockdown lentiviruses into human umbilical vein endothelial cells (HUVECs) and investigated thrombomodulin (TM) and tight junctions (TJs) protein expression by western blotting and immunofluorescence. Finally, immunohistochemistry was used to validate TM and TJs protein expression in surgical samples.
Results: Fifty simplex CCMs patients were included, comprising 38 MAP3K3 mutations and 12 CCM gene mutations. Nine (23.7%) patients with MAP3K3 mutations and 11(91.7%) patients with CCM gene mutations exhibited overt hemorrhage, respectively. Multivariate logistic analyses revealed that MAP3K3 mutation was associated with a lower risk of hemorrhage events. In the vitro experiments, ZO-1 expression was not reduced in MEKK3-I441M-overexpressing HUVECs compared with wild type, whereas it was significantly decreased in CCM2-knockdown HUVECs compared with control. In the MEKK3-I441M-overexpressing HUVECs, TM expression was increased, and the NF-κB pathway was significantly activated. After treatment with an NF-κB signaling inhibitor, TM expression was further upregulated. Meanwhile, TM expression was increased, but the NF-κB pathway was not activated in CCM2-knockdown HUVECs. Accordingly, immunohistochemistry showed that ZO-1 expression in the MAP3K3-mutant samples was significantly higher than that in the CCM-mutant samples. TM expression in the MAP3K3-mutant lesions was significantly lower than that in the CCM-mutant samples.
Conclusion: Simplex CCMs with MAP3K3 mutation occasionally present with overt hemorrhage, which is associated with the biological function of MAP3K3 mutation.
Cerebral cavernous malformations (CCMs) of the central nervous system are vascular anomalies affecting 0.16 to 0.5% of the general population (1, 2). These lesions show cerebral venous capillary dysplasia with endothelial clusters filled with blood and susceptible to hemorrhage (3, 4). Several studies have reported that the hemorrhage rates of CCMs vary from 1.6 to 4.5% per patient-year (3, 5, 6). Because of lesion bleeding, CCM lesions frequently lead to epileptic seizures, headaches, focal neurological deficits, or life-threatening strokes (7, 8).
Approximately 20% of CCMs are inherited in an autosomal dominant manner (familial CCMs), while 80% of CCMs occur without a family history (simplex CCMs) (9–11). Somatic mutations in CCM genes (CCM1/KRIT1, CCM2/MGC4607, and CCM3/PDCD10) were reported early in simplex CCM lesions (12), and recent studies reported that over one-third of simplex CCMs contain the MAP3K3 (c.1323C>G [p. Ile441Met]) somatic mutation (13, 14); the MAP3K3 and CCM gene mutations were mutually exclusive (13). CCMs arise from the endothelial gain of MEKK3-KLF2/4 signaling, while MAP3K3 and CCM gene mutations both activate the MEKK3-KLF2/4 signaling pathway (13, 15, 16). Whether MAP3K3 mutation has distinct features compared with CCM gene mutations must be investigated.
In this study, we retrospectively analyzed the clinical data of 50 patients with simplex CCMs identified to harbor a CCM gene or MAP3K3 somatic mutation. Meanwhile, we investigated the expression of thrombomodulin (TM) and tight junctions (TJs) proteins in HUVECs in the vitro experiments and surgical samples between MAP3K3 and CCM gene mutations. Our study indicates that, compared with CCM gene mutations, MAP3K3 mutant simplex CCMs have different clinical characteristics.
We performed a retrospective review of our CCMs database between May 2017 and December 2019. This study was performed according to an institutional review board-approved protocol in compliance with local and institutional regulations for the study of human subjects. Written informed consent was obtained from all participating patients (or guardians of patients). The patients with simplex CCMs identified to harbor a MAP3K3 or CCM gene mutation were included consecutively. Patients with available presurgical MRI with poor quality or a gamma knife radiosurgery history were excluded.
The demographic and clinical information of patients with simplex CCMs including age, sex, main complaint and lesion location, size, Zabramski type, concurrent with developmental venous anomaly, and overt hemorrhage was recorded and analyzed. Based on the 1994 Zabramski classification, all CCMs lesions were defined as Type I-IV (17). According to previous studies, a hemorrhage event was defined as a symptomatic event with radiographic evidence of overt intracerebral hemorrhage (5, 11).
Commercially available HUVECs (#8000; ScienCell) were cultured according to the manufacturer's guidelines and were not used beyond P8. HUVECs were cultured in an endothelial cell medium (ECM; #1001; ScienCell) and were maintained in a humidified atmosphere at 37°C in 5% CO2. Pyrrolidinedithiocarbamate ammonium, an inhibitor of nuclear factor NF-κB signaling (S3633; 100 μM; Selleck), and doramapimod, an inhibitor of p38 signaling (S1574; 500 nM; Selleck), were directly added to the endothelial cell medium.
Our study reported that MAP3K3 mutation and CCM gene mutation in the CCMs lesions existed mutually exclusive (13), and therefore, the effect of CCM2 knockdown and MAP3K3 mutation was explored in HUVECs, respectively. MEKK3-I441M-overexpressing or CCM2-knockdown lentiviruses were obtained from SyngenTech (Beijing, China). HUVECs were plated in 6-well dishes at 50% confluence and were infected with MEKK3-I441M-overexpressing lentivirus (termed oeI441M), wild-type MEKK3 lentivirus (termed WT), CCM2-knockdown lentivirus (termed shCCM2), or a negative control (termed shNC). Pools of stable transductions were generated by selection using puromycin (2 μg/ml) for 2 weeks.
Proteins from cultured cells were extracted using radioimmunoprecipitation assay lysis buffer and were quantified using a bicinchoninic acid protein assay kit. Next, equal amounts of protein were separated by sodium dodecyl sulfate–polyacrylamide gel electrophoresis and transferred to a polyvinylidene fluoride transfer membrane. After blocking, the membranes were incubated with the following primary antibodies: CCM2 (1:1000; 123930; Abcam), MEKK3 (1:1000; #5727; CST), TM (1:1000; 109189; Abcam), phospho-NF-κB (1:1000; #3033; CST), ZO-1 (1:750; #13663; CST), Occludin (1:1000; #91131; CST), Claudin-5 (1:1000; 131259; Abcam), VE-cadherin (1:1000; #2500; CST), phospho-p38 (1:1000; #4511; CST), phospho-ERK5 (1:1000; #3371; CST), KLF2 (1:500; 17008; Abcam), KLF4 (1:750; #12173; CST), and GAPDH (1:1000; #5174; CST). The membranes were then incubated with the appropriate secondary antibodies. The proteins were visualized using electrochemiluminescence (ECL) reagents. The blots were quantified by densitometry using ImageJ (NIH Image, Bethesda, MD).
For immunofluorescence staining, cell lines were grown on glass coverslips for 48 h and fixed with 4% paraformaldehyde. After permeabilization in 0.3% Triton X-100 and blocking, the cells were exposed to primary antibodies against ZO-1 (1:100; #13663; CST) and TM (1:200; #109189; Abcam) at 4°C overnight and goat anti-rabbit IgG-H&L (1:1,000; M21002; Abmart) at room temperature for 1 h. 4′,6-Diamidino-2-phenylindole was used to label cell nuclei. The slides were imaged using an inverted microscope (IX71; Olympus, Japan). ImageJ (NIH Image, Bethesda, MD) was used to quantitate the expression of proteins.
Histological sections were obtained from our sample bank as described in our previous study (13, 18) and included 3 MAP3K3 mutant surgical samples, 3 CCM gene mutant surgical samples, and 3 superficial temporal arteries as normal controls. The slides were incubated with primary antibodies against TM (1:200; #109189; Abcam), ZO-1 (1:500; #221547; Abcam), Claudin-5 (1:200; #131259; Abcam), VE-cadherin (1:200; #2500; CST), Endoglin (1:500; #252345; Abcam), VEGF (1:100; #0265; ZSGB-BIO), PCNA (1:100; #0213; ZSGB-BIO), HIF-alpha1 (1:300; #463; Abcam), and Flk1 (1:200; #10012-T24; Sino-Biological) overnight at 4°C and then were incubated with a biotinylated secondary antibody at room temperature for 1 h followed by horseradish peroxidase-labeled streptavidin for 30 min. After the sections were washed with Tris buffer, they were stained with 3,3′-diaminobenzidine, and the nuclei were counterstained with hematoxylin. Images were acquired using a Zeiss Axio Scope A1 microscope. Two authors (R. H. and J. W.) blinded to the mutation status analyzed the immunohistochemical staining for ZO-1, TM, Claudin-5, and VE-cadherin in the recruited patients. Three randomly selected fields in non-adjacent tissue sections per tissue specimen were analyzed as described previously (18, 19). A positive reaction was indicated by a brown color using 3,3′-diaminobenzidine. ImageJ (NIH Image, Bethesda, MD) was used to calculate the integrated optical density.
Continuous variables were presented as medians and interquartile range (IQR) or as means ± SD, and categorical variables were expressed as percentages. The Wilcoxon rank-sum test, t-test, chi-squared (χ2) test, and Fisher's exact test were used accordingly. Univariate and multivariate logistic regression analyses were used to assess the risk factors for hemorrhage events of CCMs. Variables with p < 0.20 in the univariate analysis were then used in the multivariate analysis. Analyses were performed using the statistical software SPSS 24.0 (IBM Corp, Armonk, NY, USA) and PRISM (GraphPad, version 8.0). A 2-tailed p < 0.05 was considered statistically significant.
Fifty patients with simplex CCMs were included, comprising 38 (76.0%) recurrent MAP3K3 somatic mutations and 12 (24.0%) CCM gene somatic mutations. Among the 50 patients, 25 (50%) patients had information about PIK3CA mutation, and 14 of the 25 patients harbored PIK3CA mutation. CCM gene mutation, MAP3K3 mutation, and PIK3CA mutation with their mutant allele frequencies are shown in Supplementary Table 1. The clinical information of 50 patients was presented in Table 1. Nine (23.7%) of the 38 patients with MAP3K3 somatic mutations presented with symptomatic events and corresponding overt intracerebral hemorrhage on the magnetic resonance imaging (MRI), whereas 11(91.7%) of the 12 patients with CCM gene somatic mutations exhibited overt intracerebral hemorrhage (13). Multivariate logistic regression analyses showed that compared with the CCM gene mutation, MAP3K3 somatic mutation was associated with a lower risk of hemorrhage events [OR = 0.028 (95% CI: 0.003–0.255); p = 0.001; Table 2]. Among the 25 patients with information about PIK3CA mutation, PIK3CA mutation was not associated with hemorrhage events [OR = 0.581 (95% CI: 0.114–2.872); p = 0.497; Table 2]. Of the 50 patients, 15 of the 38 patients who harbored MAP3K3 mutation and 5 of the 12 patients who harbored CCM gene mutation had repeated follow-up MRI scans before surgical resection (mean follow-up duration: 15.7 ± 24.4 months vs. 13.2 ± 15.8 months; p = 0.834). These data showed that MAP3K3 mutation CCM lesions remained stable with Zabramski classification type II, while CCM gene mutation lesions presented with repeated overt intracerebral hemorrhage. The representative follow-up MRI scans are shown in Figure 1A (MAP3K3 mutation lesion) and Figure 1B (CCM gene mutation lesion), respectively. These findings implied that MAP3K3 mutations were associated with a lower risk of hemorrhage events, which was different from CCM gene mutation.
Table 2. Univariate and multivariate analyses of risk factors associated with hemorrhage events of CCMs.
Figure 1. Representative follow-up MRI scans of MAP3K3 mutation and CCM gene mutation lesions before surgical resection. (A) A patient who harbored a MAP3K3 mutation lesion remained stable based on follow-up MRI scans at 13 and 40 months. (B) A patient who harbored a CCM1 mutation lesion exhibited recurrent overt hemorrhage based on follow-up MRI scans at 3 and 31 months.
Additionally, we explored the differences observed in MAP3K3 and CCM gene mutation related to clinicopathological features (hemorrhagic episodes, lesion size, and symptoms) between the brainstem and supratentorial CCM lesions. In our study, there were 9 lesions with CCM gene mutation located in the brainstem and supratentorial and 37 lesions with MAP3K3 mutation located in the brainstem and supratentorial. In the CCM gene mutation group, no differences were observed in hemorrhagic episodes and symptoms between the brainstem and supratentorial CCM lesions; the difference in lesion size between the brainstem and supratentorial CCM was not available because of limited data. In the MAP3K3 mutant group, patients with lesions located in the brainstem were more likely to undergo focal neurological deficit than supratentorial CCM lesions, and there was no difference observed in hemorrhagic episodes and lesion size between the brainstem and supratentorial CCM lesions (Supplementary Table 2).
Recurrent hemorrhage was the major presentation feature of CCMs, and the mechanisms underlying the hemorrhage of CCMs include loss of cell-cell junctions and local increase in the antithrombotic molecule. CCM endothelium was associated with unstable endothelial cell-cell contacts and locally elevated expression of anticoagulant endothelial receptors TM (20–24). We hypothesized that MAP3K3 mutations were associated with a lower risk of hemorrhage events that might result from the biofunction features of the MAP3K3 mutation concerning the expression of TM and TJ proteins.
To investigate the expression of cell-cell junction proteins in MAP3K3 c.1323C>G mutation and CCM2 knockdown endothelial cells, we infected HUVECs with lentivirus overexpressing MEKK3-I441M (MAP3K3 encodes MEKK3), wild-type MEKK3 (WT), knockdown CCM2 (shCCM2), and negative control (shNC), respectively. Compared with the WT group, the expression of ZO-1, an essential cell-cell junction protein that plays a vital role in TJs formation, was not reduced after MEKK3-I441M overexpression, as shown by Western blotting and immunofluorescence staining (Figures 2A,C and Supplementary Figures 1A,C), and after MEKK3-I441M overexpression, Occludin expression was reduced, whereas Claudin-5 and VE-cadherin expression levels were not reduced (Figure 2A and Supplementary Figure 1A). Previous studies have indicated that high KLF2 and KLF4 expression levels may result in decreased ZO-1 expression and that p38 activation can increase ZO-1 expression (20, 25, 26). Our previous findings suggested that MEKK3-I441M enhances ERK5-KLF2/4 and p38 signaling, while CCM2 knockdown only activated ERK5-KLF2/4 signaling (13). Therefore, we hypothesized that MEKK3-I441M could downregulate ZO-1 expression by increasing KLF2/4 expression while upregulating ZO-1 expression by activating p38 signaling. Western blotting showed that the KLF2, KLF4, and phospho-p38 levels were significantly increased after MEKK3-I441M overexpression compared with those in the wild-type group (Figure 2A and Supplementary Figure 1A). After treatment with doramapimod, an inhibitor of p38 signaling, ZO-1 expression was significantly downregulated in the MEKK3-I441M-overexpressing HUVECs (Figures 2B,C and Supplementary Figures 1B,C).
Figure 2. MAP3K3 mutation has different effects on ZO-1 expression compared with CCM2 knockdown in HUVECs. (A–C) HUVECs were infected by lentivirus with wild-type MEKK3 (WT) or MEKK3-I441M overexpression (oeI441M). Panel A shows that the expression of phospho-p38 (p-p38), phospho-ERK5 (p-ERK5), KLF2, and KLF4 was increased, but the expression of ZO-1, Claudin-5, and VE-cadherin was not decreased in MEKK3-I441M-overexpressing HUVECs, as shown by Western blotting. Panels B and C show that after treatment with doramapimod (Dora), an inhibitor of p38 signaling, the expression of ZO-1 was significantly downregulated. (D) Western blotting showed that compared with shNC, the expression of ZO-1, Claudin-5, Occludin, and VE-cadherin was significantly decreased in CCM2-knockdown HUVECs. HUVECs were infected with CCM2-knockdown lentivirus (shCCM2) or a negative control (termed shNC). One representative experiment of 3 is shown. Scale bar, 200 μm.
Consistent with previous studies (20, 27), after CCM2 was inactivated in HUVECs by lentivirus, Western blotting showed that ZO-1 expression was significantly decreased compared with that in the controls (Figure 2D and Supplementary Figure 1D), and immunofluorescence staining also showed that ZO-1 expression was obviously decreased after CCM2 knockdown (Supplementary Figure 3A). In addition to ZO-1, Claudin-5, Occludin, and VE-cadherin also showed decreased expression levels after CCM2 knockdown (Figure 2D and Supplementary Figure 1D). These findings suggest that MAP3K3 mutation has different effects on ZO-1 expression compared with CCM2 knockdown.
Thrombomodulin (TM) is a 557-amino acid protein with a broad cell and tissue distribution consistent with its wide-ranging physiological roles. TM is expressed on the lumenal surface of vascular endothelial cells in both large vessels and capillaries, and its primary function is to mediate endothelial thromboresistance (28). Previous studies have shown that the TM levels are increased in human CCM lesions, as well as in the plasma of patients with CCMs. In mice, endothelial-specific genetic inactivation of KRIT1 or PDCD10, which causes CCM formation, results in increased levels of vascular TM. Increased TM expression occurs because of the upregulation of transcription factors KLF2 and KLF4 consequent with the loss of KRIT1 or PDCD10. Increased TM expression contributes to CCM hemorrhage (24).
To investigate the expression of TM in MAP3K3 c.1323C>G mutation and CCM2 knockdown endothelial cells, we infected HUVECs with lentivirus overexpressing MEKK3-I441M, WT, shCCM2, and shNC, respectively. Previous studies have shown that KLF2 and KLF4 can increase TM expression and that activation of the NF-κB pathway can decrease TM expression (24, 29). In our study, compared with that in wild-type cells, after overexpression of MEKK3-I441M, the expression of KLF2, KLF4, and phospho-NF-κB was significantly increased, while Western blotting and immunofluorescence staining showed that TM expression was elevated in MEKK3-I441M-overexpressing HUVECs (Figures 3A,C and Supplementary Figures 2A,C). Interestingly, after treatment with pyrrolidinedithiocarbamate ammonium, an NF-κB signaling inhibitor, TM expression was further upregulated in MEKK3-I441M-overexpressing HUVECs (Figures 3B,C and Supplementary Figures 2B,C).
Figure 3. MAP3K3 mutation has different effects on TM expression compared with CCM2 knockdown in HUVECs. (A–C) HUVECs were infected by lentivirus with wild-type MEKK3 (WT) or MEKK3-I441M overexpression (oeI441M). Panel A shows that the expression of phospho-NF-κB (p-NF-κB) and TM was highly increased compared with that of WT. Panels B and C show that after treatment with pyrrolidinedithiocarbamate ammonium (PDTC), an NF-κB signaling inhibitor, TM expression was further upregulated. (D) Western blotting showed that compared with shNC, TM expression was highly increased in CCM2-knockdown HUVECs. HUVECs were infected with CCM2-knockdown lentivirus (shCCM2) or a negative control (termed shNC). One representative experiment of 3 is shown. Scale bar, 200 μm.
Consistent with a previous study (24), Western blotting in our study showed that compared with the control, TM expression was significantly increased and phospho-NF-κB was not activated in CCM2-knockdown HUVECs (Figure 3D and Supplementary Figure 2D). Immunofluorescence staining confirmed that TM expression was increased after CCM2 knockdown (Supplementary Figure 3B). These findings suggest that MAP3K3 mutation has distinct effects on TM expression compared with CCM2 knockdown.
To validate the different effects of MAP3K3 or CCM gene mutations on ZO-1 and TM expression, we performed immunohistochemical staining in surgical samples with MAP3K3 mutation, CCM gene mutations, and normal arteries, including 3 MAP3K3 mutant samples, 3 CCM-mutant samples, and 3 superficial temporal arteries as controls. Immunohistochemical staining showed that ZO-1 expression in the samples harboring CCM gene mutations was significantly lower than that in the control samples (p < 0.0001, t-test), whereas ZO-1 expression in the MAP3K3-mutant samples was not different from that in the control samples (p = 0.3810; t-test) (Figure 4A). The expression of Claudin-5 and VE-cadherin in the lesions with CCM gene mutations was significantly lower than that in the control samples (p < 0.05; t-test), while no difference was found in VE-cadherin expression in the MAP3K3-mutant samples compared with that in the control samples (p =0.2069; t-test) (Figures 4B,C). These findings suggest that the ZO-1 expression level is different between CCM-mutant lesions and MAP3K3-mutant lesions.
Figure 4. Comparison of ZO-1 and TM in surgical samples between MAP3K3 and CCM gene mutations. (A) Immunohistochemical staining for ZO-1 in the CCM gene mutant, MAP3K3 mutant and control samples. The histogram shows semiquantitative grading of the ZO-1 expression levels in the CCM gene mutant, MAP3K3 mutant, and control samples. (B) Immunohistochemical staining for Claudin-5 in the CCM gene mutant, MAP3K3 mutant, and control samples. The histogram shows semiquantitative grading of the Claudin-5 expression levels in the CCM mutant, MAP3K3 mutant, and control samples. (C) Immunohistochemical staining for VE-cadherin expression in CCM gene mutant, MAP3K3 mutant, and control samples. The histogram shows semiquantitative grading of the VE-cadherin expression levels in the CCM gene mutant, MAP3K3 mutant, and control samples. (D) Immunohistochemical staining for TM in the CCM gene mutant, MAP3K3-mutant, and control samples. The histogram shows semiquantitative grading of the TM expression levels in the CCM gene mutant, MAP3K3-mutant, and control samples. Control indicates the superficial temporal artery (n = 3); MAP3K3 mutant indicates CCM lesions with MAP3K3 mutation (n = 3); CCM mutant indicates CCM lesions with CCM gene mutation (n = 3), including 1 CCM1 and 2 CCM2 mutant lesions. Scale bar, 200 μm. *p < 0.05; ***p < 0.001; ****p < 0.0001.
The TM expression level in both CCM gene and MAP3K3 mutant samples was significantly higher than that in the control samples (p < 0.0001; t-test); however, the level of TM in the CCM gene mutant lesions was significantly higher than that in the MAP3K3 mutant lesions (p < 0.0001; t-test) (Figure 4D). These findings suggest that the expression level of TM is different between CCM gene mutant lesions and MAP3K3 mutant lesions.
Additionally, we performed immunohistochemical staining to detect the expression of angiogenic markers as Endoglin, VEGF, PCNA, HIF-alpha1, and Flk1 in CCM mutant lesions and normal superficial temporal arteries as control. Compared with control, the expression of Endoglin, VEGF, and PCNA was increased in CCM mutant lesions, and there was no significant difference in HIF-alpha1 and Flk1 expression between CCM mutant lesions and control (Supplementary Figure 4). Our results were consistent with previous studies (30–35).
In this study, we demonstrated that MAP3K3 mutation presents distinct clinical characteristics compared with CCM gene mutations: MAP3K3 mutation leads to less destruction of the brain–blood barrier, and less local anticoagulant molecule accumulation in the endothelium may explain its lower risk of hemorrhage events. Our results may imply that simplex CCMs have two distinct clinical subtypes.
Currently, molecular classifications are widely used in intracranial tumors, particularly in gliomas. A study reported that the molecular classification of glioblastoma involving IDH1, PDGFRA, EGFR, and NF1 substantially benefits the prediction of prognosis and response to therapy in glioblastoma patients (36). Vascular anomalies can be caused by inherited or somatic genetic mutations (13, 37, 38). The identification of inherited and somatic mutations in vascular anomalies has led to the evaluation of tailored strategies with preexisting cancer drugs that interfere with these signaling pathways (39). However, the molecular classification of simplex vascular diseases has not yet been well established. In this study, we indicated that simplex CCMs might comprise two clinical subtypes relevant to CCM genes and MAP3K3 somatic mutations. The two subclasses of simplex CCMs had different risks of hemorrhage events: CCM gene mutant lesions were susceptible to frequent overt hemorrhage, whereas MAP3K3 mutant lesions rarely led to overt hemorrhage and remained stable. Furthermore, somatic mutations of the two genotypes demonstrated different effects on the anti-coagulation and TJs in the endothelium, at least partially explaining the underlying mechanism of the specific clinical manifestations. Our findings may contribute to predicting the prognosis and treatment choices in patients with simplex CCMs.
Our study had some limitations. The genotypes of simplex CCMs are difficult to obtain in patients under long-term observation: we have difficulties to have surgical samples from long-term follow-up patients. Therefore, we are not able to investigate the lesion evolution with confirmed genotypes in a long-term follow-up in a large cohort, and larger cohort studies are needed to further strengthen the results of this study. The expression levels of TJs proteins and TM consequent to CCM gene mutation and MAP3K3 mutation were limited to in vitro experiments, and the findings required future studies in animal models.
Compared with CCM gene mutations, simplex CCMs with MAP3K3 mutation occasionally present with overt hemorrhage, which is associated with the biological function of MAP3K3 mutation in the endothelium. Future studies in animal models and larger cohort CCMs are needed to further strengthen the results of this study.
The original contributions presented in the study are included in the article/Supplementary material, further inquiries can be directed to the corresponding author/s.
The studies involving human participants were reviewed and approved by Institutional Review Board of Tiantan Hospital. Written informed consent to participate in this study was provided by the participants' legal guardian/next of kin. The animal study was reviewed and approved by Institutional Review Board of Tiantan Hospital. Written informed consent was obtained from the individual(s) for the publication of any potentially identifiable images or data included in this article.
RH and JW designed the study, conducted experiments, analyzed and interpreted the data, and drafted the manuscript for intellectual content. Y-FS, J-CW, HL, and Y-MJ collected and interpreted the data and revised the manuscript for intellectual content. H-YX, J-ZZhan, S-ZZ, and Q-HH collected the data and revised the manuscript for intellectual content. SW and J-ZZhao designed the study and revised the manuscript for intellectual content. YC provided overall oversight of the research. All authors contributed to the article and approved the submitted version.
This study was supported by the National Natural Science Foundation of China (Grant No. 82171267) and the National Key Research and Development Program of China (Grant No. 2021YFC2501102).
We are grateful to Yi Zhai (China National Clinical Research Center for Neurological Diseases) for her advice in statistical assistance.
The authors declare that the research was conducted in the absence of any commercial or financial relationships that could be construed as a potential conflict of interest.
All claims expressed in this article are solely those of the authors and do not necessarily represent those of their affiliate organizations, or those of the publisher, the editors and the reviewers. Any product that may be evaluated in this article, or claim that may be made by its manufacturer, is not guaranteed or endorsed by the publisher.
The Supplementary Material for this article can be found online at: https://www.frontiersin.org/articles/10.3389/fneur.2022.946324/full#supplementary-material
CCMs, cerebral cavernous malformations; HUVECs, human umbilical vein endothelial cells; MRI, magnetic resonance imaging; TM, thrombomodulin; TJs, tight junctions.
1. Morris Z, Whiteley WN, Longstreth WT, Weber F, Lee YC, Tsushima Y, et al. Incidental findings on brain magnetic resonance imaging: systematic review and meta-analysis. BMJ Clin Res Ed. (2009) 339:b3016. doi: 10.1136/bmj.b3016
2. Al-Holou WN, O'Lynnger TM, Pandey AS, Gemmete JJ, Thompson BG, Muraszko KM, et al. Natural history and imaging prevalence of cavernous malformations in children and young adults. J Neurosurg Pediatr. (2012) 9:198–205. doi: 10.3171/2011.11.PEDS11390
3. Porter PJ, Willinsky RA, Harper W, Wallace MC. Cerebral cavernous malformations: natural history and prognosis after clinical deterioration with or without hemorrhage. J Neurosurg. (1997) 87:190–7. doi: 10.3171/jns.1997.87.2.0190
4. Mikati AG, Khanna O, Zhang L, Girard R, Shenkar R, Guo X, et al. Vascular permeability in cerebral cavernous malformations. J Cereb Blood Flow Metabol Official J Int Soc Cerebral Blood Flow Metab. (2015) 35:1632–9. doi: 10.1038/jcbfm.2015.98
5. Jeon JS, Kim JE, Chung YS, Oh S, Ahn JH, Cho WS, et al. A risk factor analysis of prospective symptomatic haemorrhage in adult patients with cerebral cavernous malformation. J Neurol Neurosurg Psychiatry. (2014) 85:1366–70. doi: 10.1136/jnnp-2013-306844
6. Moriarity JL, Wetzel M, Clatterbuck RE, Javedan S, Sheppard JM, Hoenig-Rigamonti K, et al. The natural history of cavernous malformations: a prospective study of 68 patients. Neurosurgery. (1999) 44:1166–71. doi: 10.1227/00006123-199906000-00003
7. Al-Shahi Salman R, Berg MJ, Morrison L, Awad IA. Hemorrhage from cavernous malformations of the brain: definition and reporting standards. Angioma Alliance Sci Advisory Board Stroke. (2008) 39:3222–30. doi: 10.1161/STROKEAHA.108.515544
8. Stapleton CJ, Barker FG II. Cranial cavernous malformations: natural history and treatment. Stroke. (2018) 49:1029–35. doi: 10.1161/STROKEAHA.117.017074
9. Riant F, Bergametti F, Ayrignac X, Boulday G, Tournier-Lasserve E. Recent insights into cerebral cavernous malformations: the molecular genetics of CCM. FEBS J. (2010) 277:1070–5. doi: 10.1111/j.1742-4658.2009.07535.x
10. Chohan MO, Marchiò S, Morrison LA, Sidman RL, Cavenee WK, Dejana E, et al. Emerging pharmacologic targets in cerebral cavernous malformation and potential strategies to alter the natural history of a difficult disease: a review. JAMA Neurol. (2019) 76:492–500. doi: 10.1001/jamaneurol.2018.3634
11. Akers A, Al-Shahi Salman R, Awad IA, Dahlem K, Flemming K, Hart B, et al. Synopsis of guidelines for the clinical management of cerebral cavernous malformations: consensus recommendations based on systematic literature review by the angioma alliance scientific advisory board clinical experts panel. Neurosurgery. (2017) 80:665–80. doi: 10.1093/neuros/nyx091
12. McDonald DA, Shi C, Shenkar R, Gallione CJ, Akers AL Li S, et al. Lesions from patients with sporadic cerebral cavernous malformations harbor somatic mutations in the CCM genes: evidence for a common biochemical pathway for CCM pathogenesis. Hum Mol Genet. (2014) 23:4357–70. doi: 10.1093/hmg/ddu153
13. Weng J, Yang Y, Song D, Huo R, Li H, Chen Y, et al. Somatic MAP3K3 mutation defines a subclass of cerebral cavernous malformation. Am J Hum Genet. (2021) 108:942–50. doi: 10.1016/j.ajhg.2021.04.005
14. Hong T, Xiao X, Ren J, Cui B, Zong Y, Zou J, et al. Somatic MAP3K3 and PIK3CA mutations in sporadic cerebral and spinal cord cavernous malformations. Brain. (2021) 144:2648–58. doi: 10.1093/brain/awab117
15. Zhou Z, Tang AT, Wong WY, Bamezai S, Goddard LM, Shenkar R, et al. Cerebral cavernous malformations arise from endothelial gain of MEKK3-KLF2/4 signalling. Nature. (2016) 532:122–6. doi: 10.1038/nature17178
16. Snellings DA, Hong CC, Ren AA, Lopez-Ramirez MA, Girard R, Srinath A, et al. Cerebral cavernous malformation: from mechanism to therapy. Circ Res. (2021) 129:195–215. doi: 10.1161/CIRCRESAHA.121.318174
17. Zabramski JM, Wascher TM, Spetzler RF, Johnson B, Golfinos J, Drayer BP, et al. The natural history of familial cavernous malformations: results of an ongoing study. J Neurosurg. (1994) 80:422–32. doi: 10.3171/jns.1994.80.3.0422
18. Fu W, Huo R, Yan Z, Xu H, Li H, Jiao Y, et al. Mesenchymal behavior of the endothelium promoted by SMAD6 downregulation is associated with brain arteriovenous malformation microhemorrhage. Stroke. (2020) 51:2197–207. doi: 10.1161/STROKEAHA.120.030046
19. Huo R, Fu W, Li H, Jiao Y, Yan Z, Wang L, et al. RNA sequencing reveals the activation of wnt signaling in low flow rate brain arteriovenous malformations. J Am Heart Assoc. (2019) 8:e012746. doi: 10.1161/JAHA.119.012746
20. Lopez-Ramirez MA, Fonseca G, Zeineddine HA, Girard R, Moore T, Pham A, et al. Thrombospondin1 (TSP1) replacement prevents cerebral cavernous malformations. J Exp Med. (2017) 214:3331–46. doi: 10.1084/jem.20171178
21. Jakimovski D, Schneider H, Frei K, Kennes LN, Bertalanffy H. Bleeding propensity of cavernous malformations: impact of tight junction alterations on the occurrence of overt hematoma. J Neurosurg. (2014) 121:613–20. doi: 10.3171/2014.6.JNS132775
22. Whitehead KJ, Chan AC, Navankasattusas S, Koh W, London NR, Ling J, et al. The cerebral cavernous malformation signaling pathway promotes vascular integrity via Rho GTPases. Nat Med. (2009) 15:177–84. doi: 10.1038/nm.1911
23. Awad IA, Polster SP. Cavernous angiomas: deconstructing a neurosurgical disease. J Neurosurg. (2019) 131:1–13. doi: 10.3171/2019.3.JNS181724
24. Lopez-Ramirez MA, Pham A, Girard R, Wyseure T, Hale P, Yamashita A, et al. Cerebral cavernous malformations form an anticoagulant vascular domain in humans and mice. Blood. (2019) 133:193–204. doi: 10.1182/blood-2018-06-856062
25. Sangwung P, Zhou G, Nayak L, Chan ER, Kumar S, Kang DW, et al. KLF2 and KLF4 control endothelial identity and vascular integrity. JCI Insight. (2017) 2:e91700. doi: 10.1172/jci.insight.91700
26. Dai C, Zhao DH, Jiang M. VSL#3 probiotics regulate the intestinal epithelial barrier in vivo and in vitro via the p38 and ERK signaling pathways. Int J Mol Med. (2012) 29:202–8.
27. Glading A, Han J, Stockton RA, Ginsberg MH. KRIT-1/CCM1 is a Rap1 effector that regulates endothelial cell cell junctions. J Cell Biol. (2007) 179:247–54. doi: 10.1083/jcb.200705175
28. Martin FA, Murphy RP, Cummins PM. Thrombomodulin and the vascular endothelium: insights into functional, regulatory, and therapeutic aspects. Am J Physiol Heart Circulat Physiol. (2013) 304:H1585–H97. doi: 10.1152/ajpheart.00096.2013
29. Sohn RH, Deming CB, Johns DC, Champion HC, Bian C, Gardner K, et al. Regulation of endothelial thrombomodulin expression by inflammatory cytokines is mediated by activation of nuclear factor-kappa B. Blood. (2005) 105:3910–7. doi: 10.1182/blood-2004-03-0928
30. Zhu Y, Peters C, Hallier-Neelsen M, Miller D, Pagenstecher A, Bertalanffy H, et al. Phosphatase and tensin homolog in cerebral cavernous malformation: a potential role in pathological angiogenesis. J Neurosurg. (2009) 110:530–9. doi: 10.3171/2008.7.17626
31. Zhu Y, Wloch A, Wu Q, Peters C, Pagenstecher A, Bertalanffy H, et al. Involvement of PTEN promoter methylation in cerebral cavernous malformations. Stroke. (2009) 40:820–6. doi: 10.1161/STROKEAHA.108.526376
32. Baev NI, Awad IA. Endothelial cell culture from human cerebral cavernous malformations. Stroke. (1998) 29:2426–34. doi: 10.1161/01.STR.29.11.2426
33. Gewiss C, Hagel C, Krajewski K. Cerebral cavernomas in adults and children express relaxin. J Neurosurg Pediatr. (2019). doi: 10.3171/2019.9.PEDS19333 [Epub ahead of print].
34. Abe T, Morishige M, Ooba H, Kamida T, Fujiki M, Kobayashi H, et al. The association between high VEGF levels and multiple probable punctuate cavernous malformations. Acta Neurochir (Wien). (2009) 151:855–9. doi: 10.1007/s00701-009-0410-6
35. Lopez-Ramirez MA, Lai CC, Soliman SI, Hale P, Pham A, Estrada EJ, et al. Astrocytes propel neurovascular dysfunction during cerebral cavernous malformation lesion formation. J Clin Invest. (2021) 131:e139570. doi: 10.1172/JCI139570
36. Verhaak RG, Hoadley KA, Purdom E, Wang V, Qi Y, Wilkerson MD, et al. Integrated genomic analysis identifies clinically relevant subtypes of glioblastoma characterized by abnormalities in PDGFRA, IDH1, EGFR, and NF1. Cancer Cell. (2010) 17:98–110. doi: 10.1016/j.ccr.2009.12.020
37. Ren AA, Snellings DA, Su YS, Hong CC, Castro M, Tang AT, et al. PIK3CA and CCM mutations fuel cavernomas through a cancer-like mechanism. Nature. (2021) 594:271–6. doi: 10.1038/s41586-021-03562-8
38. Li H, Nam Y, Huo R, Fu W, Jiang B, Zhou Q, et al. De novo germline and somatic variants convergently promote endothelial-to-mesenchymal transition in simplex brain arteriovenous malformation. Circ Res. (2021) 129:825–39. doi: 10.1161/CIRCRESAHA.121.319004
Keywords: cerebral cavernous malformations, MAP3K3 mutation, CCM gene mutations, clinical characteristics, thrombomodulin, tight junctions
Citation: Huo R, Wang J, Sun Y-F, Weng J-C, Li H, Jiao Y-M, Xu H-Y, Zhang J-Z, Zhao S-Z, He Q-H, Wang S, Zhao J-Z and Cao Y (2022) Simplex cerebral cavernous malformations with MAP3K3 mutation have distinct clinical characteristics. Front. Neurol. 13:946324. doi: 10.3389/fneur.2022.946324
Received: 27 May 2022; Accepted: 02 August 2022;
Published: 26 August 2022.
Edited by:
Kenji Tanigaki, Shiga Medical Center, JapanReviewed by:
Douglas A. Marchuk, Duke University, United StatesCopyright © 2022 Huo, Wang, Sun, Weng, Li, Jiao, Xu, Zhang, Zhao, He, Wang, Zhao and Cao. This is an open-access article distributed under the terms of the Creative Commons Attribution License (CC BY). The use, distribution or reproduction in other forums is permitted, provided the original author(s) and the copyright owner(s) are credited and that the original publication in this journal is cited, in accordance with accepted academic practice. No use, distribution or reproduction is permitted which does not comply with these terms.
*Correspondence: Yong Cao, Y2FveW9uZ0BianR0aC5vcmc=
†These authors have contributed equally to this work
Disclaimer: All claims expressed in this article are solely those of the authors and do not necessarily represent those of their affiliated organizations, or those of the publisher, the editors and the reviewers. Any product that may be evaluated in this article or claim that may be made by its manufacturer is not guaranteed or endorsed by the publisher.
Research integrity at Frontiers
Learn more about the work of our research integrity team to safeguard the quality of each article we publish.