- 1Garden & Luo Immune Regulation Laboratory, Department of Clinical Sciences and Advanced Medicine, School of Veterinary Medicine, University of Pennsylvania, Philadelphia, PA, United States
- 2Department of Comparative Biomedical Sciences, Royal Veterinary College, University of London, London, United Kingdom
- 3Dean's Office, School of Veterinary Medicine, Louisiana State University, Baton Rouge, LA, United States
Background: While immune checkpoint inhibitors (ICIs) have been revolutionary in the treatment of cancer, their administration has been associated with a variety of immune-related adverse events (irAEs), including myasthenia gravis (MG), and Lambert-Eaton myasthenic syndrome (LEMS).
Objective: To provide a comprehensive synthesis of the evidence supporting an etiological role for ICIs in MG and LEMS in patients with no prior history of autoimmune disease.
Hypothesis: ICIs may trigger MG and LEMS in patients with no prior susceptibility to autoimmune disease.
Methods: Relevant primary research on Medline was interrogated using a series of search algorithms. Search terms were constructed based on the PICOS tool endorsed by the Cochrane Collaboration, which describes population, intervention, comparison, outcomes, and study design. Papers were screened according to inclusion and exclusion criteria. Additional papers were retrieved from the reference lists of screened papers. Each paper included in the qualitative synthesis was assigned an integrated metric of evidence (IME) value, ranging from 0 to 7, based on study design, quality of data, likelihood of a causal link between the immune checkpoint inhibitor(s) and MG/LEMS, confidence of MG/LEMS diagnosis, and the number of patients treated with an ICI prior to MG/LEMS diagnosis.
Results: Ninety-four papers describing at least one patient treated with ICI(s) prior to the onset of MG and/or LEMS were documented. Overall evidence for a causal link between ICI administration and MG/LEMS was low, with a median IME value of 2.88 (range 2.05–6.61).
Conclusions: There is a paucity of evidence in support of an etiological relationship between ICIs and MG/LEMS, due largely to the lack of mechanistic studies and/or prospective clinical trials with relevant study endpoints. The current literature is dominated by case reports and retrospective cohort studies, which inherently yield only low-level evidence, supporting the need for further work in this area. A role of ICIs in the etiology of MG/LEMS remains plausible, arguing for continued vigilance for irAEs in patients treated with these drugs. We argue that there is a need for future mechanistic, high quality, large-scale studies specifically investigating the possible etiological role of ICIs in MG/LEMS.
Introduction
Immune checkpoint blockade has in recent years become one of the most promising treatments for cancer (1–3). Immune checkpoint inhibitors (ICIs) are monoclonal antibodies that target negative regulators of T cell activation such as programmed death protein-1 (PD-1), programmed death ligand-1 (PD-L1), and cytotoxic T-lymphocyte antigen-4 (CTLA-4) (4). These drugs target the overactive inhibitory T cell pathways in cancer and thus enhance anti-tumor T cell activity (5, 6). While ICIs have yielded promising therapeutic results, their administration has also been associated with numerous immune-related adverse events (irAEs). These irAEs range from minor to severe, with some being life-threatening. One such rare and potentially fatal autoimmune disease is myasthenia gravis (MG) (4, 7–9).
MG is a B-cell mediated autoimmune disease of the neuromuscular junction caused by autoantibodies against acetylcholine receptors (AChR) or, less commonly, muscle-specific kinase (MuSK) or a low-density lipoprotein receptor-related protein (LRP4). This results in dysfunction at the muscle endplate and muscle fatigue and weakness (10). Lambert-Eaton myasthenic syndrome (LEMS) is a junctionopathy clinically resembling MG, in which antibodies directed against presynaptic voltage-gated calcium channels (VGCC) decrease the release of ACh (11, 12). Although T cells are not directly involved in the impairment of neuromuscular transmission in these diseases, CD4+ T helper cells (Th cells) have an important pathogenic role by permitting and facilitating the synthesis of high-affinity autoantibodies (13).
Several systematic reviews and meta-analyses investigating the role of ICIs in irAEs have been published (9, 14–21). Past case reports and narrative reviews have also summarized the literature describing an association of ICIs with MG (22–41). However, to the best of our knowledge, a rigorous analysis of the relationship between ICIs and both MG and LEMS has not been undertaken. We have therefore addressed this unmet need by conducting a species-agnostic systematic review of the current literature, in which we interrogate the evidence for a causal relationship between ICIs and MG/LEMS.
Methods
Literature review
The literature search was conducted in June 2022. Medline and Web of Science were interrogated for relevant primary research, the former by means of PubMed. Search strings were constructed by means of the “population,” “intervention,” “comparison,” “outcomes,” and “study design” (PICOS) tool endorsed by the Cochrane Collaboration (42). In the case of this systematic review, the “population” comprises patients without a history of autoimmune disease who have received ICIs; the “intervention” is ICI administration; the “comparison” is not applicable as the reviewed papers do not offer contemporaneous data on control patients; the “outcomes” are represented by the presence or absence of MG/LEMS; and the “study design” is incorporated into the IME metric. Papers captured by two primary search algorithms {((myasthenia gravis[MeSH Terms]) OR (myasthenia gravis)) AND (immune checkpoint) NOT (review)} and {((lambert-eaton myasthenic syndrome) OR (lambert eaton myasthenic syndrome)) AND (immune checkpoint) NOT (review)}, denoted as A1 and A2, respectively, were screened according to defined inclusion and exclusion criteria, described in Supplementary material. The search algorithm {(immune checkpoint inhibitors) AND ((adverse events) OR (side effects)) NOT (review)} was used to define ICIs commonly associated with adverse events, after which search algorithms for MG or LEMS and individual ICIs were written. Species-specific search algorithms were written for MG or LEMS and ICIs, including humans, mice, rats, dogs, cats, pigs, horses, cattle, sheep, guinea pigs, baboons, macaques, chimpanzees, chickens, and fish, to ensure that all relevant literature was captured in a manner agonistic of species. Only one paper discussing a non-human species was captured (43). Reference lists of relevant papers and reviews were also screened in order to identify any papers that were not captured by the search algorithms.
Curation of records
Records were reviewed following the Preferred Reporting Items for Systematic reviews and Meta-Analyses (PRISMA) guidelines (44). Strict observation of the well-defined inclusion and exclusion criteria avoided disputes over which papers to include in the study. All decisions on data accrual were made in a collaborative manner involving CS and OAG; remaining coauthors had access to the entire dataset and were involved in open discussions as data were collected. Multiple opportunities were presented to coauthors during data analysis for discussion of disparate viewpoints, of which none arose.
A total of 786 papers were captured by the search strings A1 and A2, and ICI and species-specific searches (Figure 1). After the removal of duplicates, 276 papers remained. A screening of titles and abstracts removed 44 papers, including irrelevant records and those with pre-existing MG or autoantibodies and lack of primary data. Of the remaining papers, 138 were excluded due to a lack of a definitive diagnosis of MG or LEMS (n = 61), no MG/LEMS or ICI administration reported (n = 15), a positive AChR titer or diagnosis of MG prior to ICI administration (n = 16), a lack of primary data reported (n = 43), and/or the administration of therapeutic agents other than ICIs (n = 3). The reference lists of records caught by the search strings and reviews were interrogated to identify any papers not captured by the algorithms. Four papers were retrieved, yielding a total of 94 papers for qualitative analysis, including 93 human and one murine study.
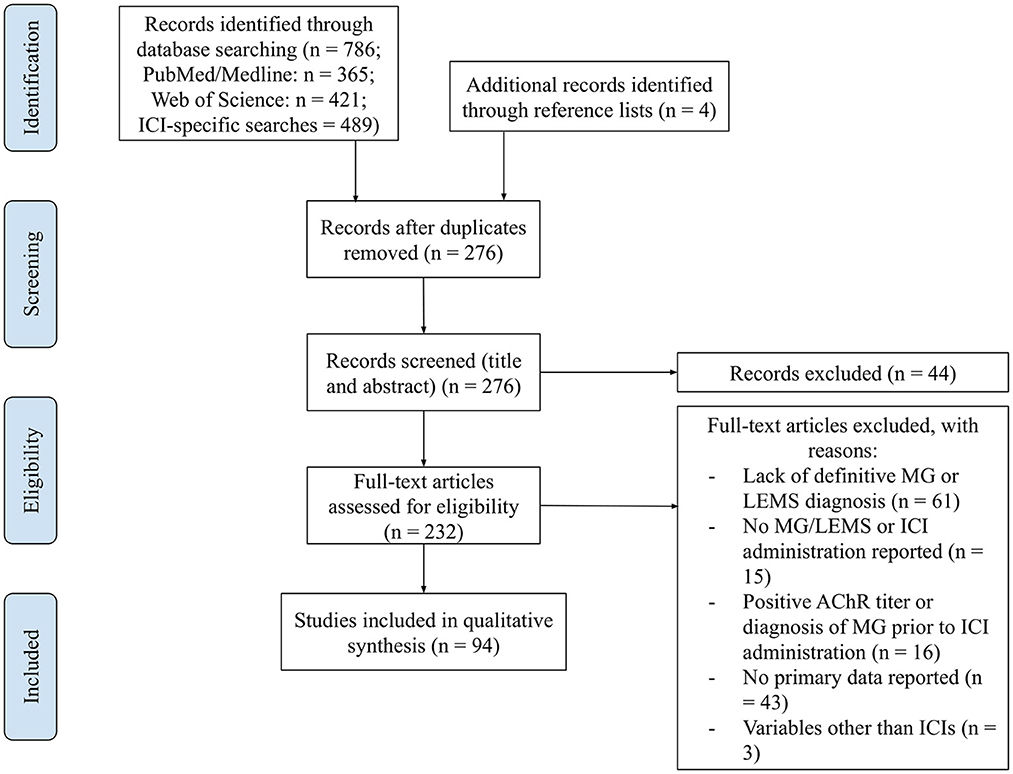
Figure 1. PRISMA flow diagram: curation of records. Papers captured by search algorithms in PubMed (n = 786) and additional records identified through reference lists (n = 4) were manually curated to remove duplicates (n = 276). The titles and abstracts of these papers were screened to assess whether they met the inclusion and exclusion criteria, yielding a total of 232 papers. These full-text articles were then assessed for eligibility, excluding 138 for various reasons. Ninety-four papers were included in the qualitative synthesis.
Ethics statement
This systematic review used historical data derived from anonymized patients included in published studies subject to ethical review, precluding the need for de novo ethical review or institutional review board approval in this project. No primary samples or prospective data were collected from current human patients.
Quality assessment
A published assessment instrument called the integrated metric of evidence (IME) designed by Garden et al. was applied to each record (45). This metric assesses study design (D), the quality of the paper (Q), the likelihood of a causal link between the ICI and MG or LEMS (L), the confidence of MG or LEMS diagnosis (I), and the number of patients treated with the ICI(s) (N). The IME value was calculated as a sum of normalized scores for each quantity, weighing each value according to its perceived relevance to evidence rating, using the formula:
Each value had a maximum normalized score of 1; therefore, the total maximum IME value was 7. When one value of an individual paper could have multiple scorings, the most parsimonious scores were assigned. Threshold IME values were calculated to categorize associations between ICIs and the onset of MG as being of negligible, low, intermediate, and high evidence. The threshold between negligible and low evidence was taken to be a hypothetical retrospective case report, with a D score of 2, Q score of 14, L and I scores of 2, and N score of 1 (IME = 2.76). The threshold between low and intermediate evidence was taken to be a hypothetical cross-sectional study with a D score of 3, Q score of 24, L score of 3, I score of 2, and N score of 2 (IME = 3.96). Lastly, the threshold between intermediate and high evidence was taken to be a hypothetical prospective cohort/case-control study with a D score of 5, Q score of 28, L and I scores of 3, and N score of 2 (IME = 4.96).
Ten references were selected at random from the pool of papers collected by the search strings, after the removal of duplicates and prior to inclusion/exclusion screening. These papers were independently scored by OAG and CS. The majority of these papers all yielded low level evidence. In order to accommodate papers across the spectrum of evidence, five papers hypothesized to be of higher evidence were specifically selected and independently reviewed by OAG and CS in similar fashion. Agreement between reviewers was evaluated using intraclass correlation.
Diagnosis of MG and LEMS
The diagnosis of MG or LEMS was made based upon clinical signs and physical examination. Many patients experiencing MG symptoms first complain of muscle weakness and fatigue, after which a serological test is often performed. The detection of anti-AChR or anti-MuSK by cell-based assay (CBA), radioimmunoassay (RIA), or enzyme-linked immunoassay (ELISA) was considered diagnostic for MG, while the presence of anti-VGCC autoantibodies was diagnostic for LEMS (46, 47). Since some MG patients are classified as “triple seronegative,” meaning that neither anti-AChR nor anti-MuSK antibodies are detected, repetitive nerve stimulation (RNS) and single-fiber electromyography (SFEMG) compatible with a postsynaptic neuromuscular junction disorder or the presence of anti-striational antibodies was considered supportive of MG (48–50) (Figure 2).
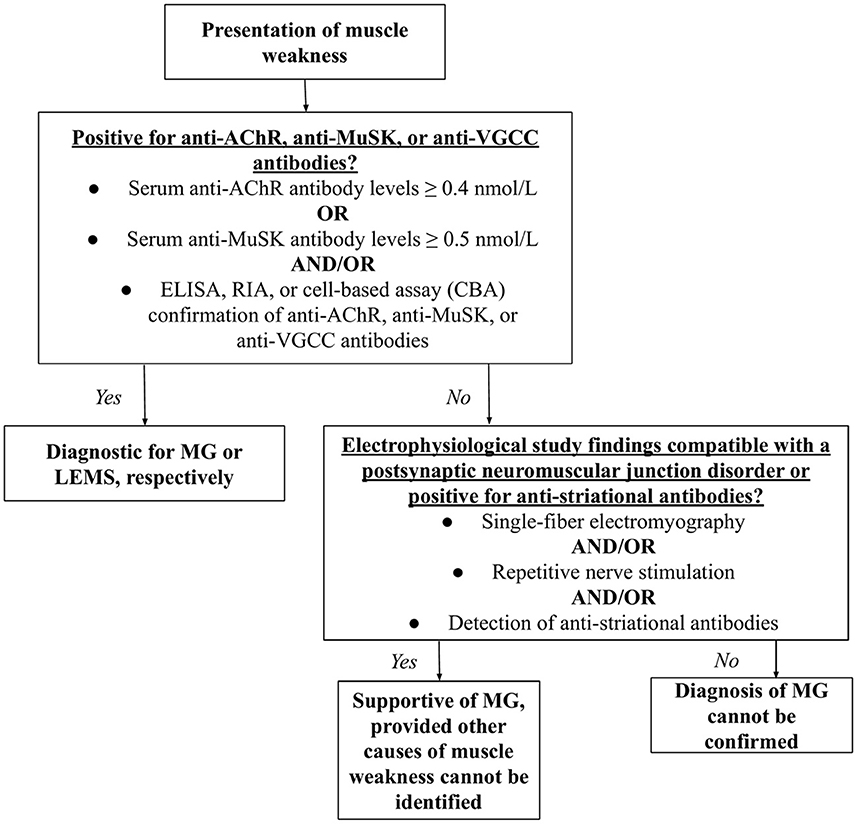
Figure 2. Diagnostic algorithm for MG and LEMS. Having presented with muscle weakness, the patient would be tested for AChR, anti-MuSK, or anti-VGCC antibodies. Detectable levels of such antibodies are diagnostic for MG and LEMS, respectively. If levels of these antibodies are not detectable, single-fiber electromyography, repetitive nerve stimulation, and/or the detection of anti-striational antibodies would support a diagnosis MG, but not LEMS.
Analysis and figures
All analyses were carried out in R (51). Graphs were created using the packages <lattice> and <latticeExtra> (52, 53). Inter-observer correlation coefficient was calculated using the ICC package (54).
Statistical analysis
A Kruskal-Wallis test was performed to investigate the statistical significance between ICI classes and between individual ICI treatment.
Results
Patient data
Out of 220 patients described by papers in this study, information on histological type treated, prior therapy, and ICI administered for 92 was recorded and thus analyzed (Supplementary Table 1). Of these 92 patients, 29 were treated for melanoma, 16 for non-small cell lung cancer (NSCLC), three for small cell lung cancer (SCLC), 16 for renal/urothelial/hepatocellular carcinoma, six for squamous cell carcinoma (SCC), four for thymoma, and 18 for other/unspecified malignancies. Thirty-two patients underwent surgery, 37 chemotherapy, and 18 radiation, either in combination or sole treatment. Six patients received ICIs as first line therapy, and 25 did not report prior therapy.
Paraneoplastic MG/LEMS is a known phenomenon in thymic cancer and SCLC, and was thus considered a confounding variable in these cancer types (11, 55). However, only eight of 92 patients included in our analysis suffered thymic cancer or SCLC in association with MG or LEMS, yielding a negligible impact on overall study conclusions (Supplementary Table 1). In the patients with malignancies other than thymic cancer and SCLC, the probability of paraneoplastic MG/LEMS would have been very low based upon the prevailing literature, arguing against a significant impact on study conclusions. Furthermore, there was no discernible correlation between histological type and MG/LEMS onset in our study cohort.
Reliability of scoring system
To ensure that the qualitative assessment of papers was independently repeatable between reviewers, 10 papers were selected from the initial pool using a random number generator. Most of these papers yielded negligible to low evidence. Five papers hypothesized to be of higher evidence were therefore targeted to ensure assessment of repeatability across the spectrum of evidence. All 15 papers were scored independently by OAG and CS. The intraclass correlation coefficient for the dataset was 0.99. Furthermore, the IME values of articles assigned by both reviewers fell within 0.2 of each other, confirming excellent reliability of the scoring system (Figure 3).
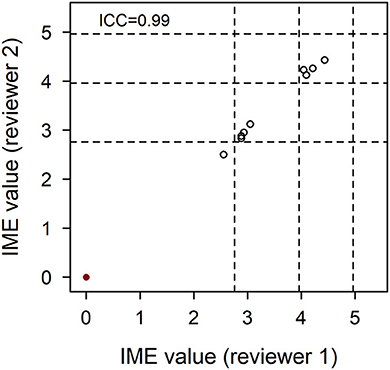
Figure 3. Agreement of integrated metric of evidence (IME) values for 10 randomized and five higher evidence publications. Ten papers from the pool of included records were selected at random and five papers hypothesized to be of higher evidence were selected in targeted fashion. These 15 papers were individually assessed by two reviewers, blinded to each other's scores. The correlation between IME values was 0.99, demonstrating excellent reliability of the scoring system. Horizontal dotted lines indicate the threshold IME values between negligible and low (2.76), low and intermediate (3.96), and intermediate and high (4.96) levels of evidence.
Immune checkpoint inhibitors as an etiological trigger
In total, 94 manuscripts were reviewed (43, 56–147). IME values were calculated for each paper by year of publication (Figure 4). In addition, IME values were computed for three classes of ICI (anti-PD-1, anti-PD-L1, and anti-CTLA-4) and nine individual ICIs (nivolumab, pembrolizumab, cemiplimab, toripalimab, durvalumab, atezolizumab, avelumab, ipilimumab, and tremelimumab) as well as combination therapies (Figures 5, 6). Because most papers directly correlating ICIs with MG or LEMS were retrospective case reports or case series, the median IME values for all categories fell within the low to intermediate range (median = 2.88). Many papers described a link between ICIs and general irAEs instead of focusing on MG or LEMS specifically. In addition, most papers described a correlation of multiple monotherapies and combination therapies with irAEs. Statements are provided for each class of ICI as well as the individual ICIs and combination therapies.
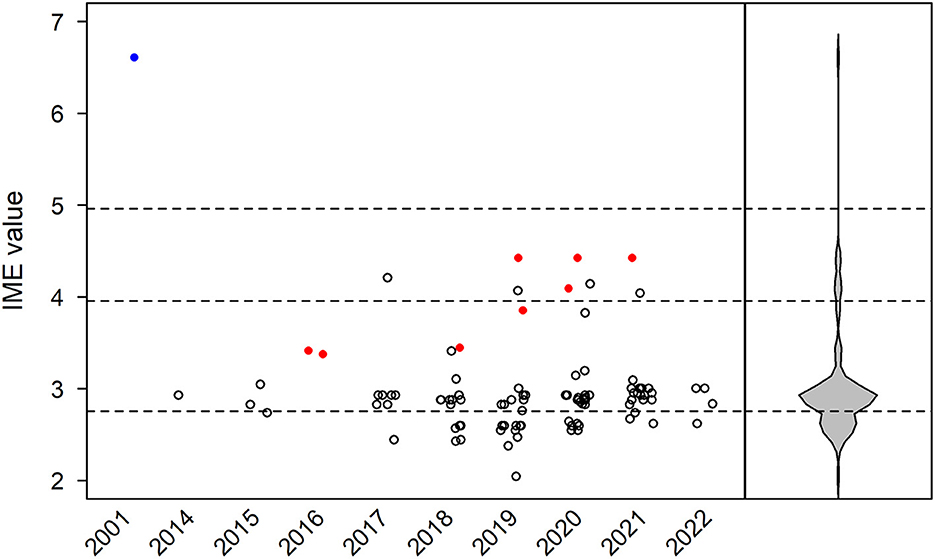
Figure 4. Integrated metric of evidence (IME) values for immune checkpoint inhibitors. Horizontal dotted lines indicate the threshold IME values between negligible and low (2.76), low and intermediate (3.96), and intermediate and high (4.96) levels of evidence. The median IME value was 2.88 (range 2.05–6.61). The sole prospective, blinded, randomized control trial is represented by a blue circle. Papers excluded from formal analysis but considered in our qualitative review are represented by a red circle.
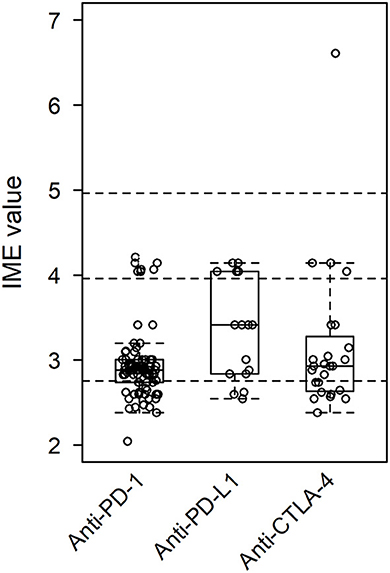
Figure 5. Integrated metric of evidence (IME) values for class of ICI. The median IME values were 2.88, 3.41, and 2.93 for anti-PD-1, anti-PD-L1, and anti-CTLA-4, respectively.
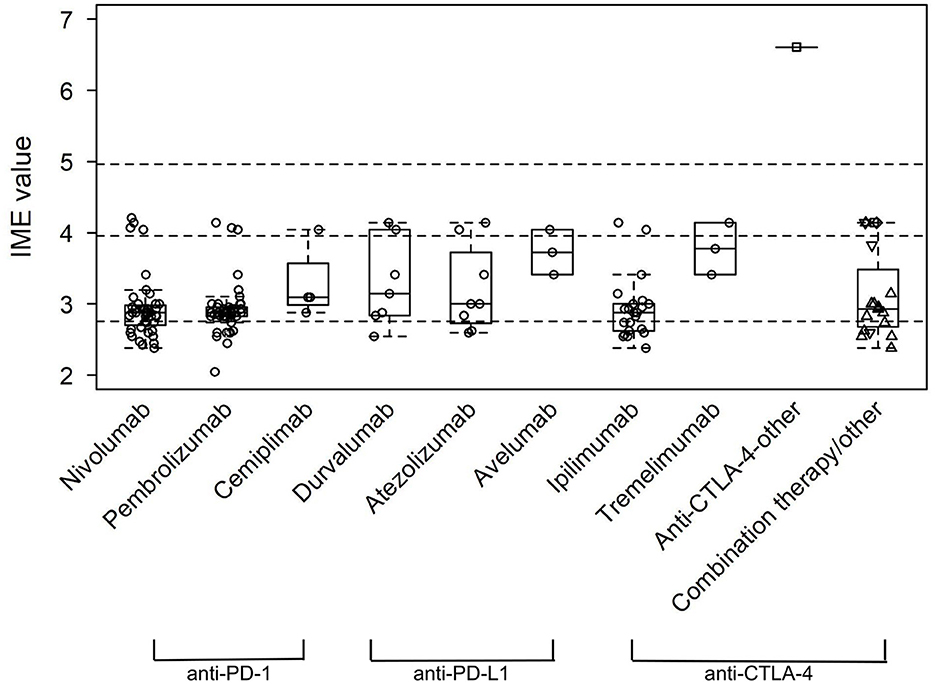
Figure 6. Integrated metric of evidence (IME) values for specific ICIs. The median IME values were 2.88, 2.88, 3.10, 3.00, 3.14, 3.00, 3.73, 2.88, and 3.78 for nivolumab, pembrolizumab, cemiplimab, toripalimab, durvalumab, atezolizumab, avelumab, ipilimumab, and tremelimumab, respectively. For combination therapy/other, other single-agent is represented by a square, nivolumab + ipilimumab by an up-pointing triangle, durvalumab + tremelimumab by a diamond, and other/non-specified combination by a down-pointing triangle.
Anti-PD-1
Of the papers describing a link between the administration of anti-PD-1 drugs and the onset of MG/LEMS, 43 manuscripts mentioned nivolumab, 45 mentioned pembrolizumab, three mentioned cemiplimab, one mentioned toripalimab, and 14 mentioned combination therapies involving an anti-PD-1 drug. The median IME values for nivolumab, pembrolizumab, cemiplimab, and toripalimab association were 2.88, 2.88, 3.10, and 3.00, respectively, while the median IME for papers mentioning combination therapy using at least one anti-PD-1 drug was 2.86. Overall, the IME values ranged from 2.05 to 4.21, with a median of 2.88 (Figures 5, 6). These data suggest low evidence for a causal link between anti-PD-1 administration and MG/LEMS.
Anti-PD-L1
Six papers mentioned the administration of durvalumab, seven mentioned atezolizumab, and two mentioned avelumab in association with the onset of MG/LEMS. The median IME values for durvalumab, atezolizumab, and avelumab administration were 3.14, 3.00, and 3.73, respectively. One paper mentioned the administration of at least one anti-PD-L1 drug in combination therapy, of which the IME value was 4.14. The IME values ranged from 2.55 to 4.14, with a median of 3.41 (Figures 5, 6). These data suggest low evidence for a causal link between anti-PD-L1 administration and MG/LEMS.
Anti-CTLA-4
Twenty-one manuscripts mentioned the administration of ipilimumab, while two papers mentioned tremelimumab. The median IME values for papers describing ipilimumab and tremelimumab administration were 2.88 and 3.78, respectively. In addition, 15 papers mentioned an association between combination therapy involving at least one anti-CTLA-4 drug and the onset of MG/LEMS, of which the median IME value was 2.88. Overall, the median IME values for anti-CTLA-4 administration ranged from 2.38 to 6.61, with a median of 2.93. Interestingly, the only manuscript that described induction of experimental autoimmune MG (EAMG) explored possible mechanisms in mice and was thus able to demonstrate a high level of evidence for anti-CTLA-4 antibodies (Figures 5, 6) (43). Overall, this dataset demonstrated low evidence for a causal link between anti-CTLA-4 administration and MG/LEMS.
Combination therapy
Overall, 20 of the 94 manuscripts mentioned at least one type of combination therapy with or without ICI monotherapy. The most common combination was nivolumab and ipilimumab, mentioned in 13 papers. Fourteen papers mentioned combination therapy with at least one anti-PD-1 drug (median IME value = 2.86), one with at least one anti-PD-L1 drug (IME value = 4.14), and 15 with at least one anti-CTLA-4 drug (median IME value = 2.88; Figure 6). These data suggest low and intermediate evidence for a causal link between anti-PD-1, anti-CTLA-4, and anti-PD-L1 combination therapies and MG/LEMS onset.
Statistical significance
There was no significant difference between the IME values of ICI classes, of individual ICIs, or of combination therapies (Figures 5, 6). These data suggest no difference in the associations between the administration of distinct ICIs and the onset of MG/LEMS in the evaluated dataset.
Discussion
ICIs are commonly used to treat cancer. Tumors activate immune checkpoints such as CTLA-4 and PD-1 to inhibit anti-tumor T cell responses (148, 149). ICIs block these checkpoints, thus reversing the attenuation of T cell anti-tumor defense mechanisms (150, 151). While these drugs help in blocking the growth and metastasis of cancer, their inhibition of the immune system can also cause uncontrolled irAEs such as MG and LEMS, prototypical antibody-mediated autoimmune diseases (19). Because ICIs are widely used, understanding their risks to patients, especially those predisposed to autoimmune disease, is important. Owing to the phenomenon of paraneoplastic autoimmune disease, some cancer patients, such as those with thymic cancer or SCLC, could potentially be at increased risk of MG/LEMS with ICI administration (152, 153). For these patients, particular caution may be warranted since ICI administration may trigger the clinical manifestation of autoimmune diseases such as MG and LEMS.
Numerous reviews and meta-analyses have been conducted to elucidate the safety of ICIs. Hottinger outlines the many possible, albeit rare, neurological irAEs known to be associated with ICI administration, highlighting the lack of guidelines available to identify those at risk of developing irAEs (20). In a 2022 meta-analysis, Farooq et al. describe the increased risk of neurological adverse events with the use of ICIs compared to controls, but the ICIs presented less of a risk than chemotherapies (21). As such, while the role of ICIs in irAEs has been investigated, a thorough analysis of their potential role in MG and LEMS has not been undertaken to the best of our knowledge. In order to address this unmet need, we have conducted a systematic review of the literature to investigate the potential role of ICIs in MG/LEMS.
Most the papers included in our qualitative analysis yield a low to intermediate level of evidence in support of a causal link. However, we suggest that this lack of cogent evidence stems from the design of the papers themselves. Many of the studies reviewed were case reports or case series, intrinsically unable to provide convincing evidence. There was a noticeable lack of mechanistic studies that could present higher levels of evidence by virtue of study design. The sole prospective, blinded, randomized control trial that met our inclusion criteria, a paper in which EAMG was induced in mice, yielded an IME value of 6.61, demonstrating high evidence for a causal link (Figure 4). This observation suggests that if more mechanistic studies had been conducted and thus included in our analysis, the median IME value of the dataset would demonstrate higher levels of evidence. While the phenomenon of paraneoplastic autoimmune disease renders it difficult to distinguish between MG/LEMS as paraneoplastic in origin or a consequence of ICI administration in specific individuals, it poses limited relevance in this study since evidence supporting a causal link was lacking; “false positives” were not observed. However, future studies must account for the phenomenon of paraneoplastic MG/LEMS as a potential confounding variable, especially for patients with thymic cancer or SCLC.
Some of the larger studies sourced by our literature search failed to satisfy our inclusion criteria and were therefore excluded from formal analysis but were considered in our qualitative review in order to maximize information derived from the dataset (Figure 4). These studies did not specify the methods of MG/LEMS diagnosis, yet demonstrated some of the attributes necessary for a study to yield strong evidence. Two such papers describe multicenter, open-label phase 1/2 and 1b trials, yielding high scores for quality of study design scores (154, 155). While these studies did not specifically investigate the role of ICIs in MG/LEMS, they demonstrate the rigor of study design necessary for future work specifically focusing on these autoimmune diseases. Six of the papers describe large-scale database studies (156–161), offering the power of large patient populations in drawing conclusions. While the IME values of two of these papers would have fallen in the low to intermediate evidence range when given the most parsimonious I value of 1 (IME = 3.44. 3.85), the other four would have fallen in the intermediate to high range (IME = 4.09, 4.43, 4.43, 4.43). These eight papers thus highlight the potential value of large-scale patient populations, especially when aligned with uniform assessment and diagnostic criteria for MG/LEMS.
In recent years, the administration of ICIs has become a mainstream treatment for cancer (162, 163). Physicians must therefore understand the risks associated with their administration to vulnerable patients. Although irAEs associated with ICIs are rare, the possibility of developing potentially fatal autoimmune diseases such as MG and LEMS warrants future study. While our data suggest no significant differences between ICI classes or individual ICIs in the development of MG/LEMS, future studies would also yield more conclusive inferences on whether some drugs have a greater propensity to induce autoimmune diseases than others. We propose that there is a need for mechanistic and large-scale prospective studies investigating the etiological role of ICIs in MG/LEMS, an important prerequisite in better understanding the risk of these diseases with increasing use of ICIs in clinical medicine.
Data availability statement
The original contributions presented in the study are included in the article/Supplementary material, further inquiries can be directed to the corresponding author.
Author contributions
Under the supervision of OG, CS developed the search algorithms, assessed the relevance of all abstracts and full texts, analyzed and interpreted the results, and wrote the first draft of the manuscript. OG analyzed and assessed a subset of articles to ensure concordance of scores. OG and CS developed the scoring system on the basis of a published instrument created by OG and Y-MC. Y-MC helped with statistical analysis and created some of the figures. OG and JL conceptualized the idea for the manuscript and edited the manuscript. All authors contributed to the article and approved the submitted version.
Acknowledgments
The authors thank Kotaro Sasaki for translating papers written in Japanese and Sandy LaMonaca and Joanne Sands for scheduling multiple international meetings between study authors.
Conflict of interest
The authors declare that the research was conducted in the absence of any commercial or financial relationships that could be construed as a potential conflict of interest.
Publisher's note
All claims expressed in this article are solely those of the authors and do not necessarily represent those of their affiliated organizations, or those of the publisher, the editors and the reviewers. Any product that may be evaluated in this article, or claim that may be made by its manufacturer, is not guaranteed or endorsed by the publisher.
Supplementary material
The Supplementary Material for this article can be found online at: https://www.frontiersin.org/articles/10.3389/fneur.2022.1004810/full#supplementary-material
References
1. Bagchi S, Yuan R, Engleman EG. Immune checkpoint inhibitors for the treatment of cancer: Clinical impact and mechanisms of response and resistance. Annu Rev Pathol. (2021) 16:223–49. doi: 10.1146/annurev-pathol-042020-042741
2. Darvin P, Toor SM, Sasidharan Nair V, Elkord E. Immune checkpoint inhibitors: Recent progress and potential biomarkers. Exp Mol Med. (2018) 50:1–11. doi: 10.1038/s12276-018-0191-1
3. Robert C. A decade of immune-checkpoint inhibitors in cancer therapy. Nat Commun. (2020) 11:3801. doi: 10.1038/s41467-020-17670-y
4. Dalvin LA, Shields CL, Orloff M, Sato T, Shields JA. Checkpoint inhibitor immune therapy: Systemic indications and ophthalmic side effects. Retina. (2018) 38:1063–78. doi: 10.1097/IAE.0000000000002181
5. Ribas A, Wolchok JD. Cancer immunotherapy using checkpoint blockade. Science. (2018) 359:1350–5. doi: 10.1126/science.aar4060
6. Akinleye A, Rasool Z. Immune checkpoint inhibitors of PD-L1 as cancer therapeutics. J Hematol Oncol. (2019) 12:92. doi: 10.1186/s13045-019-0779-5
8. Gilhus NE, Verschuuren JJ. Myasthenia gravis: Subgroup classification and therapeutic strategies. Lancet Neurol. (2015) 14:1023–36. doi: 10.1016/S1474-4422(15)00145-3
9. Michot JM, Bigenwald C, Champiat S, Collins M, Carbonnel F, Postel-Vinay S, et al. Immune-related adverse events with immune checkpoint blockade: A comprehensive review. Eur J Cancer. (2016) 54:139–48. doi: 10.1016/j.ejca.2015.11.016
10. Hargadon KM, Johnson CE, Williams CJ. Immune checkpoint blockade therapy for cancer: An overview of FDA-approved immune checkpoint inhibitors. Int Immunopharmacol. (2018) 62:29–39. doi: 10.1016/j.intimp.2018.06.001
11. Kesner VG, Oh SJ, Dimachkie MM, Barohn RJ. Lambert-Eaton Myasthenic syndrome. Neurol Clin. (2018) 36:379–94. doi: 10.1016/j.ncl.2018.01.008
12. Jayarangaiah A, Theetha Kariyanna P. Lambert Eaton Myasthenic Syndrome. Treasure Island: StatPearls Publishing.
13. Zhang XM, Liu CY, Shao ZH. Advances in the role of helper T cells in autoimmune diseases. Chin Med J. (2020) 133:968–74. doi: 10.1097/CM9.0000000000000748
14. Darnell EP, Mooradian MJ, Baruch EN, Yilmaz M, Reynolds KL. Immune-Related Adverse Events (irAEs): Diagnosis, management, and clinical pearls. Curr Oncol Rep. (2020) 22:39. doi: 10.1007/s11912-020-0897-9
15. Khan S, Gerber DE. Autoimmunity, checkpoint inhibitor therapy and immune-related adverse events: A review. Semin Cancer Biol. (2020) 64:93–101. doi: 10.1016/j.semcancer.2019.06.012
16. Kubo T, Hirohashi Y, Tsukahara T, Kanaseki T, Murata K, Morita R, et al. Immunopathological basis of immune-related adverse events induced by immune checkpoint blockade therapy. Immunol Med. (2022) 45:108–18. doi: 10.1080/25785826.2021.1976942
17. Myers G. Immune-related adverse events of immune checkpoint inhibitors: A brief review. Curr Oncol. (2018) 25:342–7. doi: 10.3747/co.25.4235
18. Postow MA, Sidlow R, Hellmann MD. Immune-related adverse events associated with immune checkpoint blockade. N Engl J Med. (2018) 378:158–68. doi: 10.1056/NEJMra1703481
19. Ramos-Casals M, Brahmer JR, Callahan MK, Flores-Chávez A, Keegan N, Khamashta MA, et al. Immune-related adverse events of checkpoint inhibitors. Nat Rev Dis Primers. (2020) 6:38. doi: 10.1038/s41572-020-0160-6
20. Hottinger AF. Neurologic complications of immune checkpoint inhibitors. Curr Opin Neurol. (2016) 29:806–12. doi: 10.1097/WCO.0000000000000391
21. Farooq MZ, Aqeel SB, Lingamaneni P, Pichardo RC, Jawed A, Khalid S, et al. Association of immune checkpoint inhibitors with neurologic adverse events: A systematic review and meta-analysis. J Am Med Assoc Netw Open. (2022) 5:e227722. doi: 10.1001/jamanetworkopen.2022.7722
22. Bai J, Li D, Yang PD, Xu KY, Wang YN, Li Q, et al. Camrelizumab-related myocarditis and myositis with myasthenia gravis: A case report and literature review. Front Oncol. (2022) 11:778185. doi: 10.3389/fonc.2021.778185
23. Becquart O, Lacotte J, Malissart P, Nadal J, Lesage C, Guillot B, et al. Myasthenia gravis induced by immune checkpoint inhibitors. J Immunother. (2019) 42:309–12. doi: 10.1097/CJI.0000000000000278
24. Chen JH, Lee KY, Hu CJ, Chung CC. Coexisting myasthenia gravis, myositis, and polyneuropathy induced by ipilimumab and nivolumab in a patient with non-small-cell lung cancer: A case report and literature review. Medicine. (2017) 96:e9262. doi: 10.1097/MD.0000000000009262
25. Cheng W, Sun T, Liu C, Zhou Z, Duan J, Zhao Y, et al. A systematic review of myasthenia gravis complicated with myocarditis. Brain Behav. (2021) 11:e2242. doi: 10.1002/brb3.2242
26. Dugena O, Zheng C, Taylor J, Wong A. Pembrolizumab-induced myasthenia gravis: Literature review of ocular manifestations and a refractory case. J Immunother. (2022) 45:267–73. doi: 10.1097/CJI.0000000000000422
27. Gonzalez NL, Puwanant A, Lu A, Marks SM, Živković SA. Myasthenia triggered by immune checkpoint inhibitors: New case and literature review. Neuromuscul Disord. (2017) 27:266–8. doi: 10.1016/j.nmd.2017.01.002
28. Guidon AC. Lambert-Eaton Myasthenic syndrome, botulism, and immune checkpoint inhibitor-related myasthenia gravis. Continuum. (2019) 25:1785–806. doi: 10.1212/CON.0000000000000807
29. Hamada N, Maeda A, Takase-Minegishi K, Kirino Y, Sugiyama Y, Namkoong H, et al. Incidence and distinct features of immune checkpoint inhibitor-related myositis from idiopathic inflammatory myositis: A single-center experience with systematic literature review and meta-analysis. Front Immunol. (2021) 12:803410. doi: 10.3389/fimmu.2021.803410
30. Huang YT, Chen YP, Lin WC, Su WC, Sun YT. Immune checkpoint inhibitor-induced myasthenia gravis. Front Neurol. (2020) 11:634. doi: 10.3389/fneur.2020.00634
31. Liang S, Yang J, Lin Y, Li T, Zhao W, Zhao J, et al. Immune myocarditis overlapping with myasthenia gravis due to anti-PD-1 treatment for a chordoma patient: A case report and literature review. Front Immunol. (2021) 12:682262. doi: 10.3389/fimmu.2021.682262
32. Makarious D, Horwood K, Coward JIG. Myasthenia gravis: An emerging toxicity of immune checkpoint inhibitors. Eur J Cancer. (2017) 82:128–36. doi: 10.1016/j.ejca.2017.05.041
33. Nicolau S, Kao JC, Liewluck T. Trouble at the junction: When myopathy and myasthenia overlap. Muscle Nerve. (2019) 60:648–57. doi: 10.1002/mus.26676
34. Pathak R, Katel A, Massarelli E, Villaflor VM, Sun V, Salgia R. Immune checkpoint inhibitor-induced myocarditis with myositis/myasthenia gravis overlap syndrome: A systematic review of cases. Oncologist. (2021) 26:1052–61. doi: 10.1002/onco.13931
35. Reyes-Bueno JA, Rodriguez-Santos L, Serrano-Castro PJ. Myasthenia gravis induced by inmuno checkpoints inhibitors: first case report secondary to avelumab therapy and review of published cases. Rev Neurol. (2019) 68:333–8. doi: 10.33588/rn.6808.2018497
36. Safa H, Johnson DH, Trinh VA, Rodgers TE, Lin H, Suarez-Almazor ME, et al. Immune checkpoint inhibitor related myasthenia gravis: single center experience and systematic review of the literature. J Immunother Cancer. (2019) 7:319. doi: 10.1186/s40425-019-0774-y
37. Sheikh S, Alvi U, Soliven B, Rezania K. Drugs that induce or cause deterioration of myasthenia gravis: An update. J Clin Med. (2021) 10:71537. doi: 10.3390/jcm10071537
38. Shi JY, Tan Y, Huang YY, Li K, Yan JW, Guan YZ, et al. Association between clinical factors and result of immune checkpoint inhibitor related myasthenia gravis: A single center experience and systematic review. Front Neurol. (2022) 13:858628. doi: 10.3389/fneur.2022.858628
39. Siu DHW, O'Neill RS, Harris CA, Wang J, Ardolino L, Downton T, et al. Immune checkpoint inhibitor-induced myocarditis, myositis, myasthenia gravis and transaminitis: A case series and review. Immunotherapy. (2022) 14:511–20. doi: 10.2217/imt-2021-0225
40. Veccia A, Kinspergher S, Grego E, Peterlana D, Berti A, Tranquillini E, et al. Myositis and myasthenia during nivolumab administration for advanced lung cancer: A case report and review of the literature. Anticancer Drugs. (2020) 31:540–4. doi: 10.1097/CAD.0000000000000903
41. Yao S, Li X, Nong J, Zhang Y. Immune checkpoint inhibitors-induced myasthenia gravis: From diagnosis to treatment. Zhongguo Fei Ai Za Zhi. (2020) 23:685–8. doi: 10.3779/j.issn.1009-3419.2020.102.25
42. Higgins JPT, Green S. Cochrane Handbook for Systematic Reviews of Interventions, Version 5.1.10. The Cochrane Collaboration. (2013). Available online at: www.cochrane-handbook.org (accessed July 27, 2022).
43. Wang HB, Shi FD Li H, Chambers BJ, Link H, Ljunggren HG. Anti-CTLA-4 antibody treatment triggers determinant spreading and enhances murine myasthenia gravis. J Immunol. (2001) 166:6430–6. doi: 10.4049/jimmunol.166.10.6430
44. Moher D, Liberati A, Tetzlaff J, Altman DG. Preferred reporting items for systematic reviews and meta-analyses: The PRISMA statement. PLoS Med. (2009) 6:e1000097. doi: 10.1371/journal.pmed.1000097
45. Garden OA, Kidd L, Mexas AM, Chang YM, Jeffery U, Blois SL, et al. ACVIM consensus statement on the diagnosis of immune-mediated hemolytic anemia in dogs and cats. J Vet Intern Med. (2019) 33:313–34. doi: 10.1111/jvim.15441
46. Rousseff RT. Diagnosis of myasthenia gravis. J Clin Med. (2021) 10:1736. doi: 10.3390/jcm10081736
47. Gilhus NE. Lambert-Eaton Myasthenic syndrome; pathogenesis, diagnosis, and therapy. Autoimmune Dis. (2011) 2011:973808. doi: 10.4061/2011/973808
48. Kim SW, Sunwoo MK, Kim SM, Shin HY, Sunwoo IN. Repetitive nerve stimulation in MuSK-antibody-positive myasthenia gravis. J Clin Neurol. (2017) 13:287–92. doi: 10.3988/jcn.2017.13.3.287
49. Romi F, Skeie GO, Gilhus NE, Aarli JA. Striational antibodies in myasthenia gravis: Reactivity and possible clinical significance. Arch Neurol. (2005) 62:442–6. doi: 10.1001/archneur.62.3.442
50. Selvan VA. Single-fiber EMG: A review. Ann Ind Acad Neurol. (2011) 14:64–7. doi: 10.4103/0972-2327.78058
51. R Core Core Team. R: A Language Environment for Statistical Computing. Vienna: R Foundation for Statistical Computing (2020). Available online at: https://urldefense.com/v3/__https://www.R-project.org/__;!!IBzWLUs!ETzLDRiTpDf4wkm8URwzi61g4DNC3nBuePAMAvL Wz588KVKj4ov7HJklBDDQeqULAr4$ (accessed July 27, 2022).
52. Sarkar D. Lattice: Multivariate Data Visualization with R. New York, NY: Springer (2008). ISBN 978-0-387-75968-5.
53. Sarkar D, Andrews F. latticeExtra: Extra Graphical Utilities Based on Lattice. R package version 0.629. (2019). Available online at: https://urldefense.com/v3/__https://CRAN.R-project.org/package=latticeExtra__;!!IBzWLUs!ETzLDRiTpDf4wkm8URwzi61g4DNC3nBuePAMAvL Wz588KVKj4ov7HJklBDDQRTLE0nk$ (accessed July 27, 2022).
54. Wolak ME, Fairbairn DJ, Paulsen YR. Guidelines for estimating repeatability. Methods Ecol Evol. (2012) 3:129–37. doi: 10.1111/j.2041-210X.2011.00125.x
55. Melzer N, Ruck T, Fuhr P, Gold R, Hohlfeld R, Marx A, et al. Clinical features, pathogenesis, and treatment of myasthenia gravis: A supplement to the Guidelines of the German Neurological Society. J Neurol. (2016) 263:1473–94. doi: 10.1007/s00415-016-8045-z
56. Agrawal K, Agrawal N. Lambert-Eaton Myasthenic syndrome secondary to nivolumab and ipilimumab in a patient with small-cell lung cancer. Case Rep Neurol Med. (2019) 2019:5353202. doi: 10.1155/2019/5353202
57. Aldrich J, Pundole X, Tummala S, Palaskas N, Andersen CR, Shoukier M, et al. Inflammatory myositis in cancer patients receiving immune checkpoint inhibitors. Arthritis Rheumatol. (2021) 73:866–74. doi: 10.1002/art.41604
58. Algaeed M, Mukharesh L, Heinzelmann M, Kaminski HJ. Pearls and Oy-sters: Pembrolizumab-induced myasthenia gravis. Neurology. (2018) 91:e1365–e7. doi: 10.1212/WNL.0000000000006278
59. Algaeed M, Mukharesh L, Heinzelmann M, Kaminski HJ. Pembrolizumab-induced myasthenia gravis. Neurology. (2019) 92:7846. doi: 10.1212/WNL.0000000000007846
60. Alnahhas I, Wong J. A case of new-onset antibody-positive myasthenia gravis in a patient treated with pembrolizumab for melanoma. Muscle Nerve. (2017) 55:E25–e6. doi: 10.1002/mus.25496
61. Anquetil C, Salem JE, Lebrun-Vignes B, Johnson DB, Mammen AL, Stenzel W, et al. Immune checkpoint inhibitor-associated myositis: Expanding the spectrum of cardiac complications of the immunotherapy revolution. Circulation. (2018) 138:743–5. doi: 10.1161/CIRCULATIONAHA.118.035898
62. Bagley BA, Sigua NL, Snook RJ. An eye-opening complication: A rare case of myasthenia gravis secondary to immune checkpoint inhibitors. Am J Respirat Crit Care Med. (2020) 201:3141. doi: 10.1164/ajrccm-conference.2020.201.1_MeetingAbstracts.A3141
63. Canino F, Pugliese G, Baldessari C, Greco S, Depenni R, Dominici M. Cemiplimab- and nivolumab-induced myasthenia gravis: Two clinical cases. Tumori. (2021) 2021:3008916211040559. doi: 10.1177/03008916211040559
64. Cautela J, Zeriouh S, Gaubert M, Bonello L, Laine M, Peyrol M, et al. Intensified immunosuppressive therapy in patients with immune checkpoint inhibitor-induced myocarditis. J Immunother Cancer. (2020) 8:1887. doi: 10.1136/jitc-2020-001887
65. Chang E, Sabichi AL, Sada YH. Myasthenia gravis after nivolumab therapy for squamous cell carcinoma of the bladder. J Immunother. (2017) 40:114–6. doi: 10.1097/CJI.0000000000000161
66. Choi YJ, Utset M, Wright R, Soni M. A case of muscle biopsy confirmed inflammatory myositis with coexisting seronegative myasthenia gravis associated with ipilimumab and pembrolizumab use in a patient with advanced melanoma. Muscle Nerve. (2018) 58:S45–S. Available online at: https://doi-org.proxy.library.upenn.edu/10.1002/mus.26353
67. Cook C, Moore A, Sharpe M. Immune checkpoint inhibitor-induced myasthenia gravis: A rare neurologic complication of immunotherapy for urothelial cell cancer. Chest. (2020) 158:A731. doi: 10.1016/j.chest.2020.08.685
68. Coustal C, Du Thanh A, Roubille F, Assenat E, Maria ATJ. Rare cutaneous toxicity of immune checkpoint inhibitors: A case of durvalumab-induced dermatomyositis. Eur J Cancer. (2021) 155:25–7. doi: 10.1016/j.ejca.2021.06.031
69. Crusz SM, Radunovic A, Shepherd S, Shah S, Newey V, Phillips M, et al. Rituximab in the treatment of pembrolizumab-induced myasthenia gravis. Eur J Cancer. (2018) 102:49–51. doi: 10.1016/j.ejca.2018.07.125
70. de Chabot G, Justeau G, Pinquié F, Nadaj-Pakleza A, Hoppé E, Hureaux J, et al. Unexpected adverse events of immunotherapies in non-small cell lung cancer: About 2 cases. Rev Pneumol Clin. (2017) 73:326–30. doi: 10.1016/j.pneumo.2017.08.012
71. Dhenin A, Samartzi V, Lejeune S, Seront E. Cascade of immunologic adverse events related to pembrolizumab treatment. BMJ Case Rep. (2019) 12:229149. doi: 10.1136/bcr-2018-229149
72. Diamantopoulos PT, Tsatsou K, Benopoulou O, Bonou M, Anastasopoulou A, Mastrogianni E, et al. Concomitant development of neurologic and cardiac immune-related adverse effects in patients treated with immune checkpoint inhibitors for melanoma. Melanoma Res. (2020) 30:484–91. doi: 10.1097/CMR.0000000000000681
73. Dumortier J, Simon M, Bouhour F. Fatal myositis and myasthenia induced by atezolizumab for the treatment of hepatocellular carcinoma. Clin Res Hepatol Gastroenterol. (2022) 46:101854. doi: 10.1016/j.clinre.2021.101854
74. Fazel M, Jedlowski PM. Severe myositis, myocarditis, and myasthenia gravis with elevated anti-striated muscle antibody following single dose of ipilimumab-nivolumab therapy in a patient with metastatic melanoma. Case Rep Immunol. (2019) 2019:2539493. doi: 10.1155/2019/2539493
75. Fellner A, Makranz C, Lotem M, Bokstein F, Taliansky A, Rosenberg S, et al. Neurologic complications of immune checkpoint inhibitors. J Neurooncol. (2018) 137:601–9. doi: 10.1007/s11060-018-2752-5
76. Figueroa-Perez N, Kashyap R, Bal D, Khan SA, Pattan V. Autoimmune myasthenia, primary adrenal insufficiency, and progressive hypothyroidism due to pembrolizumab and axitinib combination regimen. Cureus. (2021) 13:16933. doi: 10.7759/cureus.16933
77. Fuentes-Antrás J, Peinado P, Guevara-Hoyer K, Del Arco CD, Sánchez-Ramón S, Aguado C. Fatal autoimmune storm after a single cycle of anti-PD-1 therapy: A case of lethal toxicity but pathological complete response in metastatic lung adenocarcinoma. Hematol Oncol Stem Cell Ther. (2020) 4:6. doi: 10.1016/j.hemonc.2020.04.006
78. Fukasawa Y, Sasaki K, Natsume M, Nakashima M, Ota S, Watanabe K, et al. Nivolumab-induced myocarditis concomitant with myasthenia gravis. Case Rep Oncol. (2017) 10:809–12. doi: 10.1159/000479958
79. Fukazawa R, Takezawa H, Tsuji Y, Noto Y, Banba M, Fujii A, et al. Case of myasthenia gravis developed during pembrolizumab administration, suggesting an excitation-contraction connection disorder. Rinsho Shinkeigaku. (2020) 60:37–40. doi: 10.5692/clinicalneurol.cn-001323
80. Garcia-Garcia J, Diaz-Maroto I, Gonzalez-Villar E, Martinez-Martin A. Myasthenia gravis induced by atezolizumab. Rev Neurol. (2022) 74:343–4. doi: 10.33588/rn.7410.2021264
81. Giglio D, Berntsson H, Fred Å, Ny L. Immune checkpoint inhibitor-induced polymyositis and myasthenia gravis with fatal outcome. Case Rep Oncol. (2020) 13:1252–7. doi: 10.1159/000510740
82. Gill AJ, Gandhy S, Lancaster E. Nivolumab-associated Lambert-Eaton Myasthenic syndrome and cerebellar dysfunction in a patient with a neuroendocrine tumor. Muscle Nerve. (2021) 63:E18–e21. doi: 10.1002/mus.27141
83. Guidon AC, David W. Myasthenia gravis following nivolumab and pembrolizumab/ipilimumab therapy. Muscle Nerve. (2015) 52:S143–S. Available online at: https://doi-org.proxy.library.upenn.edu/10.1002/mus.24891
84. Hayakawa N, Kikuchi E, Suzuki S, Oya M. Myasthenia gravis with myositis induced by pembrolizumab therapy in a patient with metastatic urothelial carcinoma. Int Cancer Conf J. (2020) 9:123–6. doi: 10.1007/s13691-020-00408-4
85. Heleno CT, Mustafa A, Gotera NA, Tesar A. Myasthenia gravis as an immune-mediated side effect of checkpoint inhibitors. Cureus. (2021) 13:16316. doi: 10.7759/cureus.16316
86. Hernandez AP, Clemente MB, Garcia DE, Calvo RV, Garcia BN, Dominguez JFO, et al. Checkpoint inhibitor-induced fulminant myocarditis, complete atrioventricular block and myasthenia gravis-a case report. Cardiovasc Diagn Ther. (2021) 11:1013–9. doi: 10.21037/cdt-21-147
87. Ho AK, Cooksley T. Immune checkpoint inhibitor-mediated myasthenia gravis. J Emerg Med. (2020) 59:561–2. doi: 10.1016/j.jemermed.2020.05.004
88. Huh SY, Shin SH, Kim MK, Lee SY, Son KH, Shin HY. Emergence of myasthenia gravis with myositis in a patient treated with pembrolizumab for thymic cancer. J Clin Neurol. (2018) 14:115–7. doi: 10.3988/jcn.2018.14.1.115
89. Isami A, Uchiyama A, Shimaoka Y, Suzuki S, Kawachi I, Fujita N. A case of anti-titin antibody positive nivolumab-related necrotizing myopathy with myasthenia gravis. Rinsho Shinkeigaku. (2019) 59:431–5. doi: 10.5692/clinicalneurol.cn-001270
90. Jang J, Stream S. A case of pembrolizumab-induced complete heart block and myasthenia gravis. J Gen Intern Med. (2018) 33:S408–S9. Available online at: https://doi-org.proxy.library.upenn.edu/10.1007/s11606-022-07653-8
91. Jespersen MS, Fano S, Stenor C, Moller AK. A case report of immune checkpoint inhibitor-related steroid-refractory myocarditis and myasthenia gravis-like myositis treated with abatacept and mycophenolate mofetil. Eur Heart J Case Rep. (2021) 5:ytab342. doi: 10.1093/ehjcr/ytab342
92. Jeyakumar N, Etchegaray M, Henry J, Lelenwa L, Zhao B, Segura A, et al. The terrible triad of checkpoint inhibition: A case report of myasthenia gravis, myocarditis, and myositis induced by cemiplimab in a patient with metastatic cutaneous squamous cell carcinoma. Case Rep Immunol. (2020) 2020:5126717. doi: 10.1155/2020/5126717
93. Johnson DB, Saranga-Perry V, Lavin PJ, Burnette WB, Clark SW, Uskavitch DR, et al. Myasthenia gravis induced by ipilimumab in patients with metastatic melanoma. J Clin Oncol. (2015) 33:e122–4. doi: 10.1200/jco.2015.33.15_suppl.9019
94. Kamada S, Hanazono A, Sanpei Y, Fukunaga H, Inoue T, Suzuki S, et al. Anti-titin antibody, one of the antistriational autoantibodies was found in a case of seronegative myasthenia gravis associated with anti-PD-1 therapy. J Neurol Sci. (2017) 381:1145. doi: 10.1016/j.jns.2017.08.3224
95. Kankanala VL, Kotecha N. Myasthenia gravis: A rare immune related adverse event of pembrolizumab. Am J Respirat Crit Care Med. (2019) 199:6542. doi: 10.1164/ajrccm-conference.2019.199.1_MeetingAbstracts.A6542
96. Kee W, Ng KYY, Lee JJX, Tan DSW. Myasthenia gravis and myocarditis after administration of pembrolizumab in a patient with metastatic non-small cell lung cancer and resected thymoma. Clin Lung Cancer. (2022) 23:e293–e5. doi: 10.1016/j.cllc.2021.12.001
97. Kim JS, Nam TS, Kim J, Kho BG, Park CK, Oh IJ, et al. Myasthenia gravis and myopathy after nivolumab treatment for non-small cell lung carcinoma: A case report. Thorac Cancer. (2019) 10:2045–9. doi: 10.1111/1759-7714.13177
98. Komatsu M, Hirai M, Kobayashi K, Hashidate H, Fukumoto J, Sato A, et al. A rare case of nivolumab-related myasthenia gravis and myocarditis in a patient with metastatic gastric cancer. BMC Gastroenterol. (2021) 21:4. doi: 10.1186/s12876-021-01904-4
99. Kovalev D, Muranova A, Patel C. First dose pembrolizumab-induced toxicity in young patient treated for invasive thymoma: An overlap syndrome of myasthenia gravis and myositis. Ann Neurol. (2020) 88:S240–S.
100. Lara MS, Afify A, Ellis MP, Phan CT, Richman DP, Riess JW. Immune checkpoint inhibitor-induced myasthenia gravis in a patient with advanced NSCLC and remote history of thymoma. Clin Lung Cancer. (2019) 20:e489–e91. doi: 10.1016/j.cllc.2019.04.007
101. Liao B, Shroff S, Kamiya-Matsuoka C, Tummala S. Atypical neurological complications of ipilimumab therapy in patients with metastatic melanoma. Neuro Oncol. (2014) 16:589–93. doi: 10.1093/neuonc/nou001
102. Liewluck T, Kao JC, Mauermann ML. PD-1 inhibitor-associated myopathies: Emerging immune-mediated myopathies. J Immunother. (2018) 41:208–11. doi: 10.1097/CJI.0000000000000196
103. Liu Q, Ayyappan S, Broad A, Narita A. Pembrolizumab-associated ocular myasthenia gravis. Clin Exp Ophthalmol. (2019) 47:796–8. doi: 10.1111/ceo.13499
104. Liu SY, Chan J, Brinc D, Gandhi S, Izenberg A, Delgado D, et al. Immune checkpoint inhibitor-associated myocarditis with persistent troponin elevation despite abatacept and prolonged immunosuppression. JACC Cardiooncol. (2020) 2:800–4. doi: 10.1016/j.jaccao.2020.10.013
105. Loochtan AI, Nickolich MS, Hobson-Webb LD. Myasthenia gravis associated with ipilimumab and nivolumab in the treatment of small cell lung cancer. Muscle Nerve. (2015) 52:307–8. doi: 10.1002/mus.24648
106. Lorenzo CJ, Fitzpatrick H, Campdesuner V, George J, Lattanzio N. Pembrolizumab-induced ocular myasthenic crisis. Cureus. (2020) 12:e9192. doi: 10.7759/cureus.9192
107. Luo YB, Tang WT, Zeng QM, Duan WW, Li SY, Yang XS, et al. Case report: The neuromusclar triad of immune checkpoint inhibitors: A case report of myositis, myocarditis, and myasthenia gravis overlap following toripalimab treatment. Front Cardiovasc Med. (2021) 8:714460. doi: 10.3389/fcvm.2021.714460
108. Mancano MA, Bulow JEV, Ro M. ISMP adverse drug reactions: Lithium-induced cardiomyopathy fixed drug eruption due to cetirizine, levocetirizine, and hydroxyzine nivolumab-induced myasthenia gravis nivolumab-induced cholangitic liver disease torsade de pointes caused by psychiatric polypharmacy trichotillomania associated with aripiprazole. Hosp Pharm. (2018) 53:371–5. doi: 10.1177/0018578718795225
109. March KL, Samarin MJ, Sodhi A, Owens RE. Pembrolizumab-induced myasthenia gravis: A fatal case report. J Oncol Pharm Pract. (2018) 24:146–9. doi: 10.1177/1078155216687389
110. Matas-García A, Milisenda JC, Selva-O'Callaghan A, Prieto-González S, Padrosa J, Cabrera C, et al. Emerging PD-1 and PD-1L inhibitors-associated myopathy with a characteristic histopathological pattern. Autoimmun Rev. (2020) 19:102455. doi: 10.1016/j.autrev.2019.102455
111. Mathews EP, Romito JW. Management of immune checkpoint inhibitor-related acute hypoxic neuromuscular respiratory failure using high-flow nasal cannula. Proc Bayl Univ Med Cent. (2020) 33:407–8. doi: 10.1080/08998280.2020.1744793
112. Mehta JJ, Maloney E, Srinivasan S, Seitz P, Cannon M. Myasthenia gravis induced by nivolumab: A case report. Cureus. (2017) 9:e1702. doi: 10.7759/cureus.1702
113. Miñón-Fernández B, Losada-Domingo JM, Sánchez-Horvath MT, Bárcena-Llona J. Myasthenia gravis associated with nivolumab. Rev Neurol. (2020) 70:72–3. doi: 10.33588/rn.7002.2019153
114. Montes V, Sousa S, Pita F, Guerreiro R, Carmona C. Myasthenia gravis induced by ipilimumab in a patient with metastatic melanoma. Front Neurol. (2018) 9:150. doi: 10.3389/fneur.2018.00150
115. Nakanishi S, Nishida S, Miyazato M, Goya M, Saito S. A case report of nivolumab-induced myasthenia gravis and myositis in a metastatic renal cell carcinoma patient. Urol Case Rep. (2020) 29:101105. doi: 10.1016/j.eucr.2019.101105
116. Nakatani Y, Tanaka N, Enami T, Minami S, Okazaki T, Komuta K. Lambert-Eaton Myasthenic syndrome caused by nivolumab in a patient with squamous cell lung cancer. Case Rep Neurol. (2018) 10:346–52. doi: 10.1159/000494078
117. Ng AH, Molinares DM, Ngo-Huang AT, Bruera E. Immunotherapy-related skeletal muscle weakness in cancer patients: A case series. Ann Palliat Med. (2021) 10:2359–65. doi: 10.21037/apm-20-454
118. Noda T, Kageyama H, Miura M, Tamura T, Ito H. A case of myasthenia gravis and myositis induced by pembrolizumab. Rinsho Shinkeigaku. (2019) 59:502–8. doi: 10.5692/clinicalneurol.cn-001251
119. Onda A, Miyagawa S, Takahashi N, Gochi M, Takagi M, Nishino I, et al. Pembrolizumab-induced ocular myasthenia gravis with anti-titin antibody and necrotizing myopathy. Intern Med. (2019) 58:1635–8. doi: 10.2169/internalmedicine.1956-18
120. Phua CS, Murad A, Fraser C, Bray V, Cappelen-Smith C. Myasthenia gravis and concurrent myositis following PD-L1 checkpoint inhibitor for non-small cell lung cancer. Br Med J Neurol Open. (2020) 2:e000028. doi: 10.1136/bmjno-2019-000028
121. Rhee JY, Torun N, Neilan TG, Guidon AC. Consider myocarditis when patients treated with immune checkpoint inhibitors present with ocular symptoms. Oncologist. (2022) 27:E402–E5. doi: 10.1093/oncolo/oyac033
122. Rota E, Varese P, Agosti S, Celli L, Ghiglione E, Pappalardo I, et al. Concomitant myasthenia gravis, myositis, myocarditis and polyneuropathy, induced by immune-checkpoint inhibitors: A life-threatening continuum of neuromuscular and cardiac toxicity. eNeurologicalSci. (2019) 14:4–5. doi: 10.1016/j.ensci.2018.11.023
123. Rugiero M, Bettini M, Silveira F, Albacete FS, Christiansen S. Pembrolizumab induced myasthenia gravis and necrotizing myopathy with severe respiratory failure. Neuromuscular Disord. (2018) 28:S49-S. doi: 10.1016/j.nmd.2018.06.089
124. Sanchez-Sancho P, Selva-O'Callaghan A, Trallero-Araguas E, Ros J, Montoro B. Myositis and myasteniform syndrome related to pembrolizumab. Br Med J Case Rep. (2021) 14:241766. doi: 10.1136/bcr-2021-241766
125. Sawai T, Hosokawa T, Shigekiyo T, Ogawa S, Sano E, Arawaka S. An autopsy case of nivolumab-induced myasthenia gravis and myositis. Rinsho Shinkeigaku. (2019) 59:360–4. doi: 10.5692/clinicalneurol.cn-001282
126. Seki M, Uruha A, Ohnuki Y, Kamada S, Noda T, Onda A, et al. Inflammatory myopathy associated with PD-1 inhibitors. J Autoimmun. (2019) 100:105–13. doi: 10.1016/j.jaut.2019.03.005
127. Sekiguchi K, Hashimoto R, Noda Y, Tachibana H, Otsuka Y, Chihara N, et al. Diaphragm involvement in immune checkpoint inhibitor-related myositis. Muscle Nerve. (2019) 60:E23–e5. doi: 10.1002/mus.26640
128. Serapio C, Saltman A. A triad of myositis, myasthenia gravis, and myocarditis in patients receiving immune checkpoint inhibitor therapy for advanced cancer: A case series. J Rheumatol. (2021) 48:1158. Available online at: https://doi-org.proxy.library.upenn.edu/10.3899/jrheum.210406
129. Shelly S, Triplett JD, Pinto MV, Milone M, Diehn FE, Zekeridou A, et al. Immune checkpoint inhibitor-associated myopathy: A clinicoseropathologically distinct myopathy. Brain Commun. (2020) 2:fcaa181. doi: 10.1093/braincomms/fcaa181
130. Sugiyama Y, Esa Y, Watanabe A, Kobayashi J, Suzuki S, Takahashi D. Immune checkpoint inhibitor-induced anti-striational antibodies in myasthenia gravis and myositis: A case report. Rinsho Shinkeigaku. (2021) 61:630–4. doi: 10.5692/clinicalneurol.cn-001604
131. Sun RQ, Shah V, Tummala S, Chen M. Nivolumab-induced myasthenia gravis with myositis in patients with genitourinary cancer. Neurology. (2019) 92.
132. Sutaria R, Patel P, Danve A. Autoimmune myositis and myasthenia gravis resulting from a combination therapy with nivolumab and ipilimumab for metastatic melanoma. Eur J Rheumatol. (2019) 6:153–4. doi: 10.5152/eurjrheum.2019.18159
133. Suzuki S. Myasthenia gravis and myositis (PD-1 myopathy). Gan To Kagaku Ryoho. (2020) 47:219–23.
134. Suzuki S, Ishikawa N, Konoeda F, Seki N, Fukushima S, Takahashi K, et al. Nivolumab-related myasthenia gravis with myositis and myocarditis in Japan. Neurology. (2017) 89:1127–34. doi: 10.1212/WNL.0000000000004359
135. Szuchan C, Elson L, Alley E, Leung K, Camargo AL, Elimimian E, et al. Checkpoint inhibitor-induced myocarditis and myasthenia gravis in a recurrent/metastatic thymic carcinoma patient: A case report. Eur Heart J Case Rep. (2020) 4:1–8. doi: 10.1093/ehjcr/ytaa051
136. Tahir N, Mahboob A, Piao X, Ying G, Shrestha J, Sherchan R, et al. Nivolumab, a double-edged sword: A case report of nivolumab-induced myasthenia gravis. J Med Cases. (2021) 12:424–8. doi: 10.14740/jmc3783
137. Takai M, Kato D, Iinuma K, Maekawa YM, Nakane K, Tsuchiya T, et al. Simultaneous pembrolizumab-induced myasthenia gravis and myocarditis in a patient with metastatic bladder cancer: A case report. Urol Case Rep. (2020) 31:101145. doi: 10.1016/j.eucr.2020.101145
138. Tan RYC, Toh CK, Takano A. Continued response to one dose of nivolumab complicated by myasthenic crisis and myositis. J Thorac Oncol. (2017) 12:e90–e1. doi: 10.1016/j.jtho.2017.02.024
139. Tedbirt B, De Pontville M, Branger P, Picard C, Baroudjian B, Lebbé C, et al. Rechallenge of immune checkpoint inhibitor after pembrolizumab-induced myasthenia gravis. Eur J Cancer. (2019) 113:72–4. doi: 10.1016/j.ejca.2019.03.006
140. Thakolwiboon S, Karukote A, Wilms H. De novo myasthenia gravis induced by atezolizumab in a patient with urothelial carcinoma. Cureus. (2019) 11:e5002. doi: 10.7759/cureus.5002
141. Tozuka T, Sugano T, Noro R, Takano N, Hisakane K, Takahashi S, et al. Pembrolizumab-induced agranulocytosis in a pulmonary pleomorphic carcinoma patient who developed interstitial lung disease and ocular myasthenia gravis. Oxf Med Case Rep. (2018) 2018:omy094. doi: 10.1093/omcr/omy094
142. Verma N, Jaffer M, Pina Y, Peguero E, Mokhtari S. Rituximab for immune checkpoint inhibitor myasthenia gravis. Cureus J Med Sci. (2021) 13:16337. doi: 10.7759/cureus.16337
143. Vermeulen L, Depuydt CE, Weckx P, Bechter O, Van Damme P, Thal DR, et al. Myositis as a neuromuscular complication of immune checkpoint inhibitors. Acta Neurol Belg. (2020) 120:355–64. doi: 10.1007/s13760-020-01282-w
144. Wakefield C, Shultz C, Patel B, Malla M. Life-threatening immune checkpoint inhibitor-induced myocarditis and myasthenia gravis overlap syndrome treated with abatacept: A case report. Br Med J Case Rep. (2021) 14:244334. doi: 10.1136/bcr-2021-244334
145. Werner JM, Schweinsberg V, Schroeter M, von Reutern B, Malter MP, Schlaak M, et al. Successful treatment of myasthenia gravis following PD-1/CTLA-4 combination checkpoint blockade in a patient with metastatic melanoma. Front Oncol. (2019) 9:84. doi: 10.3389/fonc.2019.00084
146. Wu D, Condit D, Nascimento JC. Pembrolizumab induced myasthenia gravis. Am J Respirat Crit Care Med. (2021) 203.
147. Yanase T, Moritoki Y, Kondo H, Ueyama D, Akita H, Yasui T. Myocarditis and myasthenia gravis by combined nivolumab and ipilimumab immunotherapy for renal cell carcinoma: A case report of successful management. Urol Case Rep. (2021) 34:101508. doi: 10.1016/j.eucr.2020.101508
148. Mellman I, Coukos G, Dranoff G. Cancer immunotherapy comes of age. Nature. (2011) 480:480–9. doi: 10.1038/nature10673
149. Iwai Y, Ishida M, Tanaka Y, Okazaki T, Honjo T, Minato N. Involvement of PD-L1 on tumor cells in the escape from host immune system and tumor immunotherapy by PD-L1 blockade. Proc Natl Acad Sci USA. (2002) 99:12293–7. doi: 10.1073/pnas.192461099
150. Seidel JA, Otsuka A, Kabashima K. Anti-PD-1 and anti-CTLA-4 therapies in cancer: Mechanisms of action, efficacy, and limitations. Front Oncol. (2018) 8:86. doi: 10.3389/fonc.2018.00086
151. Waldman AD, Fritz JM, Lenardo MJ. A guide to cancer immunotherapy: From T cell basic science to clinical practice. Nat Rev Immunol. (2020) 20:651–68. doi: 10.1038/s41577-020-0306-5
152. Sieb JP. Myasthenia gravis: An update for the clinician. Clin Exp Immunol. (2014) 175:408–18. doi: 10.1111/cei.12217
153. Titulaer MJ, Lang B, Verschuuren JJ. Lambert-Eaton Myasthenic syndrome: From clinical characteristics to therapeutic strategies. Lancet Neurol. (2011) 10:1098–107. doi: 10.1016/S1474-4422(11)70245-9
154. Antonia S, Goldberg SB, Balmanoukian A, Chaft JE, Sanborn RE, Gupta A, et al. Safety and antitumour activity of durvalumab plus tremelimumab in non-small cell lung cancer: A multicentre, phase 1b study. Lancet Oncol. (2016) 17:299–308. doi: 10.1016/S1470-2045(15)00544-6
155. Antonia SJ, López-Martin JA, Bendell J, Ott PA, Taylor M, Eder JP, et al. Nivolumab alone and nivolumab plus ipilimumab in recurrent small-cell lung cancer (CheckMate 032): A multicentre, open-label, phase 1/2 trial. Lancet Oncol. (2016) 17:883–95. doi: 10.1016/S1470-2045(16)30098-5
156. Hasegawa S, Ikesue H, Nakao S, Shimada K, Mukai R, Tanaka M, et al. Analysis of immune-related adverse events caused by immune checkpoint inhibitors using the Japanese Adverse Drug Event Report database. Pharmacoepidemiol Drug Saf. (2020) 29:1279–94. doi: 10.1002/pds.5108
157. Johnson DB, Manouchehri A, Haugh AM, Quach HT, Balko JM, Lebrun-Vignes B, et al. Neurologic toxicity associated with immune checkpoint inhibitors: A pharmacovigilance study. J Immunother Cancer. (2019) 7:134. doi: 10.1186/s40425-019-0617-x
158. Mikami T, Liaw B, Asada M, Niimura T, Zamami Y, Green-LaRoche D, et al. Neuroimmunological adverse events associated with immune checkpoint inhibitor: A retrospective, pharmacovigilance study using FAERS database. J Neurooncol. (2021) 152:135–44. doi: 10.1007/s11060-020-03687-2
159. Salem JE, Manouchehri A, Moey M, Lebrun-Vignes B, Bastarache L, Pariente A, et al. Cardiovascular toxicities associated with immune checkpoint inhibitors: An observational, retrospective, pharmacovigilance study. Lancet Oncol. (2018) 19:1579–89. doi: 10.1016/S1470-2045(18)30608-9
160. Sato K, Mano T, Iwata A, Toda T. Neurological and related adverse events in immune checkpoint inhibitors: A pharmacovigilance study from the Japanese Adverse Drug Event Report database. J Neurooncol. (2019) 145:1–9. doi: 10.1007/s11060-019-03273-1
161. Xia T, Brucker AJ, McGeehan B, VanderBeek BL. Risk of non-infectious uveitis or myasthenia gravis in patients on checkpoint inhibitors in a large healthcare claims database. Br J Ophthalmol. (2020) 2020:317060. doi: 10.1136/bjophthalmol-2020-317060
162. Fritz JM, Lenardo MJ. Development of immune checkpoint therapy for cancer. J Exp Med. (2019) 216:1244–54. doi: 10.1084/jem.20182395
Keywords: myasthenia gravis, Lambert-Eaton myasthenic syndrome, autoimmunity, immune checkpoint inhibitors, etiology
Citation: Seligman C, Chang Y-M, Luo J and Garden OA (2023) Exploring the role of immune checkpoint inhibitors in the etiology of myasthenia gravis and Lambert-Eaton myasthenic syndrome: A systematic review. Front. Neurol. 13:1004810. doi: 10.3389/fneur.2022.1004810
Received: 27 July 2022; Accepted: 12 December 2022;
Published: 09 January 2023.
Edited by:
Christian Krarup, University of Copenhagen, DenmarkReviewed by:
Ankit Mangla, Case Western Reserve University, United StatesMarc De Baets, Maastricht University, Netherlands
Copyright © 2023 Seligman, Chang, Luo and Garden. This is an open-access article distributed under the terms of the Creative Commons Attribution License (CC BY). The use, distribution or reproduction in other forums is permitted, provided the original author(s) and the copyright owner(s) are credited and that the original publication in this journal is cited, in accordance with accepted academic practice. No use, distribution or reproduction is permitted which does not comply with these terms.
*Correspondence: Oliver A. Garden, ogarden@lsu.edu
†These authors share senior authorship