- 1Department of Neurosurgery, Tangdu Hospital, Fourth Military Medical University, Xi’an, China
- 2Department of Urology, Tangdu Hospital, Fourth Military Medical University, Xi’an, China
Mitochondrial dysfunction plays significant roles in the pathogenesis of Parkinson’s Disease (PD). The inactivation of c-Myc, a down-stream gene of Wnt/β-catenin signaling, may contribute to the mitochondria dysfunction. Inhibition of glycogen synthase kinase 3β (GSK-3β) with Alsterpaullone (Als) can activate the down-stream events of Wnt signaling. Here, we investigated the protective roles of Als against MPP+-induced cell apoptosis in SH-SY5Y cells. The data showed that Als effectively rescued c-Myc from the MPP+-induced decline via Wnt signaling. Furthermore, Als protected SH-SY5Y cells from the MPP+-induced mitochondrial fission and cell apoptosis. However, the protective roles of Als were lost under β-catenin-deficient conditions. These findings indicate that Als, a GSK-3β inhibitor, attenuated the MPP+-induced mitochondria-dependent apoptotic via up-regulation of the Wnt signaling.
Introduction
Parkinson’s Disease (PD) is a common movement disorder characterized by the selective loss of dopaminergic neurons in substania nigra pars compacta (Rostamian Delavar et al., 2018; Sheehan and Yue, 2018). Accumulating evidences have suggested that mitochondrial dysfunction plays important roles in the pathogenesis of PD (Wang et al., 2016; Perez Carrion et al., 2018). However, the underlying molecular mechanisms responsible for mitochondrial dysfunction are elusive and remain to be fully explored. Emerging evidences show that inactivation of c-Myc, a Wnt/β-catenin signaling down-stream gene, may contribute to the fusion of mitochondria (Graves et al., 2012). Besides, the down-regulation of β-catenin and c-Myc has been reported in 6-OHDA induced animal PD models (Wang et al., 2017). The down-regulation of p-Ser9-glycogen synthase kinase 3β (GSK3β) also has been reported in vitro parkinsonism models (Dun et al., 2012).
Wnt signaling modulates various processes in central nervous system development, such as neurogenesis, synapse plasticity and neuronal survival (Lambert et al., 2016; Oliva et al., 2018). β-catenin is one of the main effectors in canonical Wnt signaling (Wang et al., 2017). The enzyme GSK-3β is an inhibitor of canonical Wnt signaling as it leads to the degradation of β-catenin (Rangrez et al., 2016). Inhibition of the GSK-3β activity by molecular compounds and various enzymes is an important step upon activation of the canonical Wnt signaling cascade and the downstream genes expression, including c-Myc and Cyclin D1 (Kouvidi et al., 2016). Alsterpaullone (Als) is demonstrated to act by competing with the ATP for binding to GSK-3β and induce the phosphorylation of GSK-3β on serine-9 (Leost et al., 2000; Teo et al., 2006). Activating downstream events of Wnt signaling by inhibiting GSK-3β with Als induces the recruitment of nerve cells from interstitial stem cells (Teo et al., 2006). Furthermore, the increase of phosphorylated GSK-3β has a crucial role in the prevention of cortical neurons apoptosis (Takadera et al., 2012).
Mitochondria act as an important mediator of the survival and apoptosis in nerve cells. Increasing evidence indicates that the dysfunction of mitochondria plays a crucial role in the pathophysiology of PD (Hu et al., 2014; Monti et al., 2015). Mitochondrial fission often produces small and dysfunctional organelles which is eliminated by autophagosomal machinery, However, the fusion can maintain the integrity of mitochondria and thereby prolonging the mitochondria life spans (Shimauchi et al., 2017). Graves et al. (2012) have reported that c-Myc is responsible for the maintaining of mitochondrial membrane potential and the increasing of membrane fusion.
Wnt/β-catenin signaling plays a vital and direct role in the loss of dopaminergic neurons in PD (Wang et al., 2017). c-Myc, a down-stream gene of Wnt/β-catenin signaling, may modulate the fusion of mitochondria (Graves et al., 2012). Inhibition of GSK-3β with Als can activate the downstream events of Wnt signaling (Takadera et al., 2012). Furthermore, the dysfunction of mitochondria appears to participate in the pathophysiology of PD (Wang et al., 2016). In our study, we investigated the protective effects of Als against the MPP+-induced mitochondrial fission and cell apoptosis in SH-SY5Y cells and the role of c-Myc in these protections.
Materials and Methods
Reagents
Fetal bovine serum (FBS) and Dulbecco’s modified Eagle’s medium (DMEM) was provided by Gibco (Gai-thersburg, MD, USA). MTT, MPP+ and Als were obtained from Sigma-Aldrich (St. Louis, MO, USA). MitoTracker was purchased from Life Technologies (Carlsbad, CA, USA, Cat#1837173). The following antibodies were used: β-catenin (Abcam, Cambridge, UK, Cat#ab19449, RRID:AB_444927), PARP (Abcam, Cambridge, UK, Cat#ab32138, RRID:AB_777101), c-Myc (Abcam, Cambridge, UK, Cat# ab17356, RRID:AB_2148459), GAPDH (Cell Signaling, Beverly, MA, USA, Cat#3683, RRID:AB_1642205), GSK-3β (Cell Signaling, Beverly, MA, USA, Cat#12456, RRID:AB_2636978), p-GSK-3β (ser9; Cell Signaling, Beverly, MA, USA, Cat#9322P, RRID:AB_2115199), cleaved caspase-3 (Cell Signaling, Beverly, MA, USA, Cat#9669, RRID:AB_2069869), cleaved caspase-8 (Cell Signaling, Beverly, MA, USA, Cat#9496L, RRID:AB_2259431), β-actin (Biosynthesis biotechnology, Beijing, china), FITC or TRITC-conjugated secondary antibody (Biosynthesis biotechnology, Beijing, china, Cat#BSTEK021, Cat#BST12B15B31), Anti-mouse-HRP IgG or anti-rabbit -HRP IgG (Biosynthesis biotechnology, Beijing, china, Cat#RS0002, Cat#ZB2305).
Cell Cultures
SH-SY5Y cells, a human dopaminergic neuroblastoma cell line, were purchased from ATCC (Manassas, VA, USA, Cat#CRL-2266, RRID:CVCL_0019). Then the cells were cultured in DMEM supplemented with 10% FBS in a humidified atmosphere of 5% CO2 at 33°C. Cells were incubated with a range of MPP+ concentrations (0–2,000 μM) for 24 h. Various concentrations of Als (0–2.0 μM) were added for 24 h before MPP+ treatment.
Gene Transfection
Human β-catenin in the pcDNA3.0 vector was purchased from Addgene (#16828, Cambridge, MA, USA). The β-catenin small interfering RNA and c-Myc small interfering RNA were obtained from GenePharma (Shanghai, China). SH-SY5Y cells were seeded in the 6-well plates and cultured in DMEM without FBS. Then the cells were transfected with 60 nM siRNA or the indicated plasmids using Lipofectamin 2,000 according to the manufacturer’s instructions. Cells were exposed to the plasmid or siRNA for 6 h, after which the medium was replaced by complete DMEM. The siRNAs sequences are shown in the Supplementary Table S1.
MTT Assay
Cell viability was measured by MTT assay according to the protocols. Briefly, cells grown in 96-well microplates were incubated with MTT labeling reagents for 2 h at 37°C. Then the supernate was removed and 100 μl DMSO was added to each well (Zeng et al., 2017). The absorbance at 490 nm was determined by microplates reader (Bio-Rad, Hercules, CA, USA).
Western Blot Analysis
Total proteins were prepared from cell lines. The equal amounts of proteins were separated by SDS-PAGE and transferred to the polyvinylidene fluoride membranes. The membranes were blocked in 5% BSA at room temperature for 2 h and incubated with primary antibodies at 4°C overnight. After washing three times, membranes were incubated with the HRP-conjugated secondary antibody at room temperature for 2 h. Chemiluminescence detection was used to visualize the interest protein bands.
Nuclear Extraction
Cells were transferred to the 1.5 ml microcentrifuge tube and centrifuge at 3,000 rpm for 5 min at 4°C. A pipette was used to carefully discard the supernatant. 0.6 ml hypotonic buffer was added to the microcentrifuge tube. Cell pellet in the microcentrifuge tube was suspended vigorously and incubated on ice for 10 min. Then the tube was centrifuged at 3,000 rpm for 5 min at 4°C and the supernatant was removed. The precipitate was suspended vigorously after 0.2 ml lysis buffer was added. The tube was incubated on ice for 20 min and centrifuged at 15,000 rpm for 5 min at 4°C. The supernatant was nuclear extraction of SH-SY5Y cells.
Quantitative Real-Time PCR
The total RNA was isolated from SH-SY5Y cells using Trizol Reagent (Invitrogen), and then cDNA was synthesized using the AMV reverse transcriptase (Promega, Madison, WI, USA). The quantitative real-time PCR was conducted using ABI Prism 7,500 real-time PCR instrument (Applied Biosystems, Carlsbad, CA, USA). The primer sequences are shown in Supplementary Table S2.
Immunofluorescence
Cells on the slides were washed with PBS and fixed with 4% formaldehyde solution for 8 min. After permeabilizing for 30 min in the 0.1% Triton-100, the cells were blocked with 1% BSA for 2 h and incubated with primary antibodies at 4°C overnight. The next day, cells were washed with PBS for three times and labeled with secondary antibodies. Then the nuclei were labeled with DAPI for 15 min at room temperature. Nikon fluorescence microscope (C2 Si; Nikon, Japan) was used to capture the fluorescence images.
Mitochondrial Staining
Mitochondrial staining was performed according to the manufacturer’s instructions. Briefly, SH-SY5Y cells were grown on a confocal Petri dish. After designed treatment, they were stained with 10 nM MitoTracker Red CMXRos (Life Technologies) for 20 min at 33°C. Then the cells were fixed with 4% formaldehyde solution for 8 min and labeled with DAPI for 15 min at room temperature. Nikon fluorescence microscope (C2 Si; Nikon, Japan) was used to observe the mitochondria. The ratio between the major and minor axes of an ellipse equivalent to the shape of the mitochondrial is regard as the aspect ratio of mitochondria. Twenty fields of view in a coverslip are randomly recorded to analyze the aspect ratio of mitochondria in an experiment (Koopman et al., 2008; Zeng et al., 2017).
TUNEL Assay
TUNEL assay was conducted using the one step TUNEL Apoptosis Kit (Beyotime, JS, China). After treatment, cells plated in confocal Petri dishes were fixed and incubated with TUNEL reaction buffer at 37°C for 1 h according to the manufacture’s protocol. Then the cells were washed with PBS for three times and stained with DAPI. The TUNEL positive cells were imaged under a Nikon fluorescence microscope (C2 Si; Nikon, Japan).
Statistical Analysis
Statistical difference was measured using one-way analysis of variance (ANOVA) followed by Bonferroni test for multiple groups. All values were reported as the mean ± SEM. P values < 0.05 were considered to be statistically significant.
Results
Effects of Als on MPP+-Induced Neurotoxicity and Inactivation of Wnt Signaling in SH-SY5Y Cells
Cells were treated with a series of concentrations (0, 125, 250, 500, 1,000, 2,000 μM) of MPP+ for 24 h. The MTT assay indicated that MPP+ induced a dose-dependent decrease of the cell viability, and the cell viability decreased almost 45% at an exposure to 500 μM MPP+ (Figure 1A). However, the cell viability of SH-SY5Y cells exhibited an increase when cells were pretreated with various concentrations of Als (0.5, 1.0, 2.0 μM) for 24 h before MPP+= treatment (Figure 1B). Moreover, Als (0.25 μM or 0.5 μM) was applied to SH-SY5Y cells treated with MPP+ and the cell viability was assessed 0 h, 12 h, 24 h or 48 h later. The results indicated that the decrease of cell viability induced by MPP+ could be abrogated by 0.5 μM and 24 h Als treatment (Supplementary Figure S1). Although 0.5 μM Als treatment alone showed no difference on the cell viability compared to that of the control group, we still found an activation of Wnt signaling in 0.5 μM Als treatment alone group compared with control group (Figures 1B,C). Besides, the down-regulation of Wnt signaling induced by MPP+ (500 μM) could be abrogated by pretreatment of Als (0.5, 1.0, 2.0 μM; Figures 1C–F). Then the cells were pretreated with 0.5 μM Als for 24 h before adding MPP+ in the following experiments. Furthermore, these results indicated that Als could protect SH-SY5Y cells from the MPP+-induced down-regulation of cell viability and Wnt signaling in a dose-dependent manner.
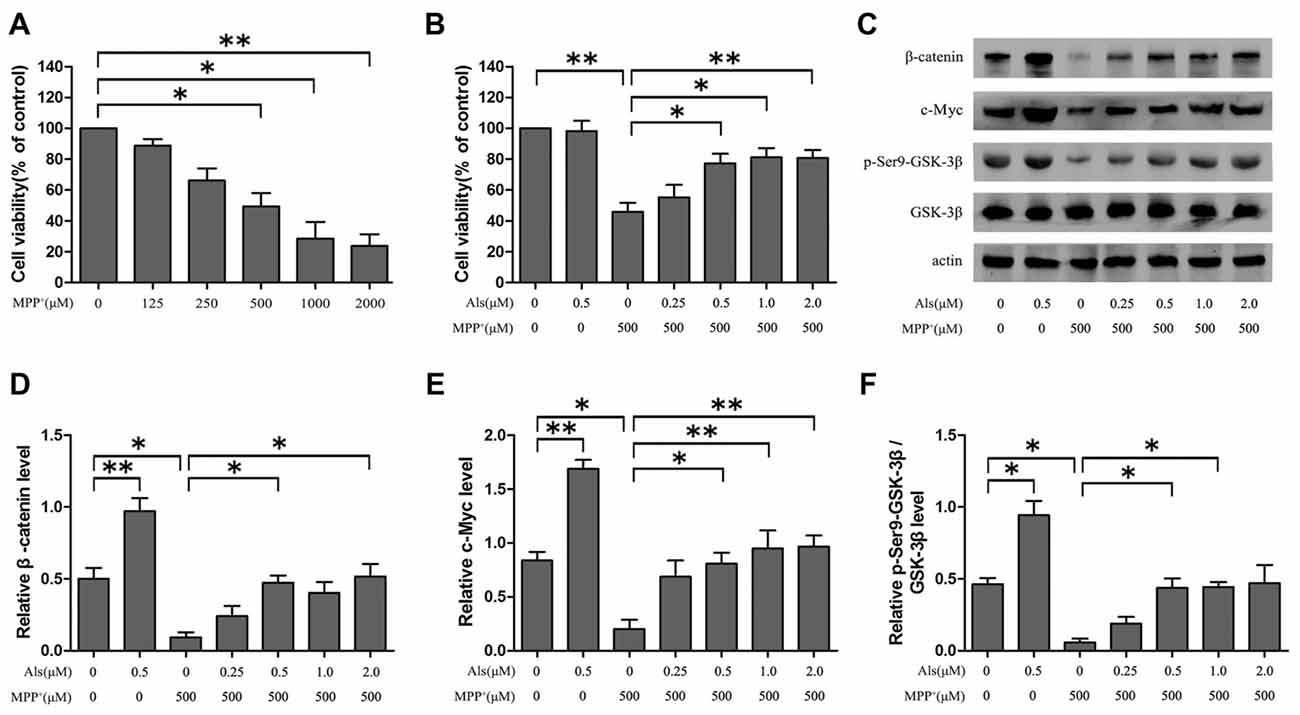
Figure 1. The effect of Alsterpaullone (Als) against MPP+-induced cytotoxicity and inactivation of Wnt signaling. (A) Cells were treated with a range of MPP+ concentrations (0–2,000 μM) for 24 h, and MTT assay was used to detect the cell viability. (B) Various concentrations of Als (0–2.0 μM) were added for 24 h before MPP+ treatment (500 μM). Cell viability was evaluated using the MTT assay (the means ± SEM; n = 3; *p < 0.05, **p < 0.01). (C) Cells were treated as described in (B) and Western blot was conducted in the different groups. (D–F) Relative amounts of β-catenin/actin, c-Myc/actin and p-glycogen synthase kinase 3β (GSK-3β; ser9)/GSK-3β were analyzed (the means ± SEM; n = 3; *p < 0.05, **p < 0.01).
Als Rescued c-Myc From the MPP+-Induced Decline via Wnt Signaling
The expression levels of c-Myc and Wnt signaling were analyzed by western blotting. The results showed that MPP+ treatment led to the down-regulation of c-Myc and Wnt signaling. Then the β-catenin was overexpressed using pcDNA3.0-β-catenin in SH-SY5Y cells (Supplementary Figure S2). Overexpression of β-catenin could reverse the inactivation of c-Myc induced by MPP+ treatment (Figures 2A–D). Besides, Als attenuated the MPP+-induced down-regulation of c-Myc and Wnt signaling. Overexpression of β-catenin exacerbated the increase of c-Myc in SH-SY5Y cells pretreated with Als before adding MPP+ (Figures 2E,F). Moreover, Als could reverse the down-regulation of c-Myc induced by the adding of MPP+ and silencing β-catenin largely abrogated this positive effect of Als in SH-SY5Y cells (Figures 2G,H, Supplementary Figure S3).
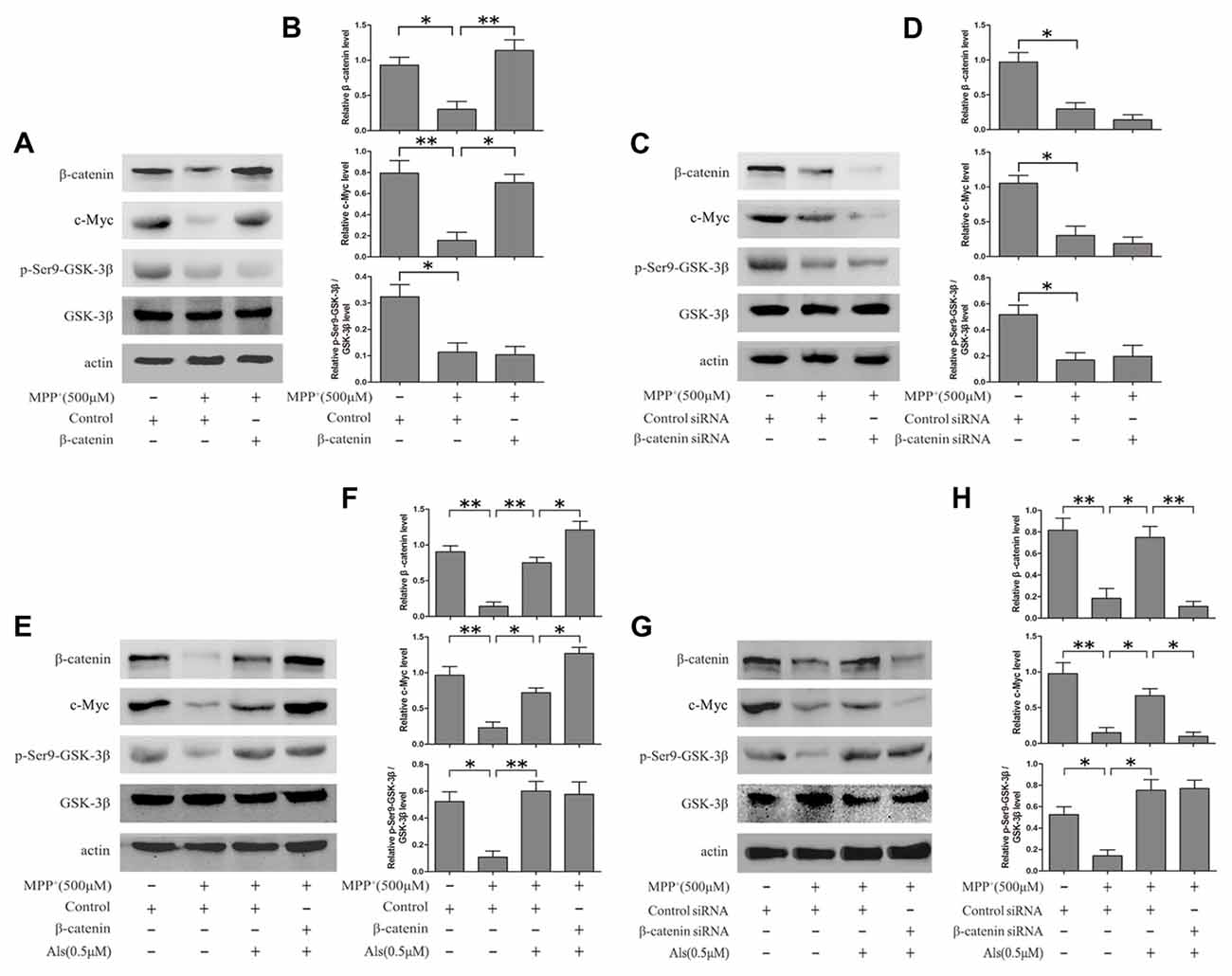
Figure 2. Als effectively rescued c-Myc from the MPP+-induced decline via Wnt signaling. (A) Cells transfected with pcDNA3.0-β-catenin were treated with MPP+ (500 μM) for 24 h. Western blot was conducted in the Control, MPP+ and MPP++β-catenin groups. (B) Relative amounts of β-catenin/actin, c-Myc/actin and p- GSK-3β (ser9)/GSK-3β level were quantified (the means ± SEM; n = 3; *p < 0.05, **p < 0.01). (C) Cells transfected with β-catenin siRNA were treated with MPP+ (500 μM) for 24 h. Western blot was conducted in the Control siRNA, MPP+ and β-catenin siRNA groups. (D) The ratios of β-catenin/actin, c-Myc/actin and p-GSK-3β(ser9)/GSK-3β were analyzed (the means ± SEM; n = 3; *p < 0.05, **p < 0.01). (E) Cells transfected with pcDNA3.0 or pcDNA3.0-β-catenin were pretreated with Als for 24 h before MPP+ treatment. Western blot was conducted in different groups. (F) Quantification graphs of the ratios of β-catenin/actin, c-Myc/actin and p-GSK-3β (ser9)/GSK-3β (the means ± SEM; n = 3; *p < 0.05, **p < 0.01). (G) Cells transfected with control siRNA or β-catenin siRNA were pretreated with Als for 24 h before MPP+ treatment and Western blot was conducted in different groups. (H) Quantification graphs indicating the ratios of β-catenin/actin, c-Myc/actin and p-GSK-3β(ser9)/GSK-3β (the means ± SEM; n = 3; *p < 0.05, **p < 0.01).
To analyze the level of c-Myc and β-catenin in nuclear fraction, we performed the immunofluorescence. The results indicated that Als attenuated the MPP+-induced down-regulation of c-Myc and β-catenin and the increase of c-Myc could be exacerbated by the overexpression of β-catenin in the nuclear fraction of SH-SY5Y cells (Figure 3A). Western blotting results also revealed that Als attenuated MPP+-induced down-regulation of c-Myc and β-catenin in the nuclear fraction of SH-SY5Y cells (Figures 3B,C). The mRNA level of c-Myc and β-catenin decreased after MPP+ treatment and Als could reverse the decrease of c-Myc mRNA induced by MPP+ (Figure 3D). Then the immunofluorescence showed that Als reversed the down-regulation of c-Myc induced by MPP+ and silencing β-catenin largely abrogated this positive effect of Als in the nuclear fraction of SH-SY5Ycells (Figure 3E). Same conclusion was obtained in the western blotting of the nuclear fraction and quantitative real-time PCR test (Figures 3F–H).
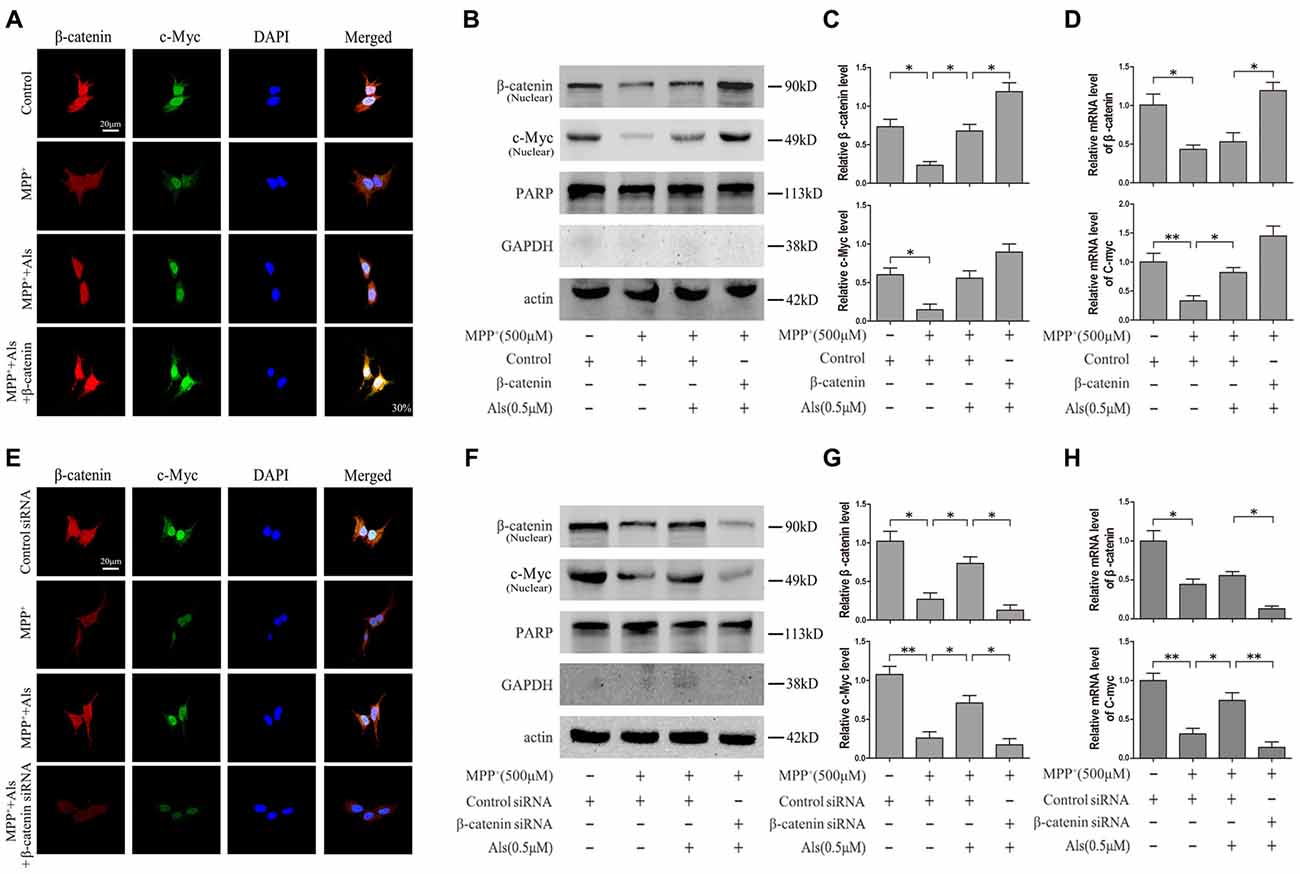
Figure 3. Expression of c-Myc and β-catenin in the nuclear fractions and mRNA levels of SH-SY5Y cells. (A) Immunofluorescence analysis of c-Myc and β-catenin in SH-SY5Y cells. Cells were treated as described in Figure 2E (scale bar, 20 μm; Transfection efficiency, 30%). (B) Western blot analysis of the nuclear fractions of SH-SY5Y cells which were treated as described in Figure 2E. GAPDH and PARP was the markers for the cytoplasmic and nuclear fractions, respectively. (C) Quantification graphs of the β-catenin/actin and c-Myc/actin in the nuclear fractions (the means ± SEM; n = 3; *p < 0.05). (D) The mRNA level of β-catenin and c-Myc in different groups of SH-SY5Y cells, and actin served as the internal control to ensure equal loading (the means ± SEM; n = 3; *p < 0.05, **p < 0.01). (E) Immunofluorescence analysis of c-Myc and β-catenin in SH-SY5Y cells which were treated as described in Figure 2G (scale bar, 20 μM). (F) Western blot analysis of the nuclear fractions of SH-SY5Y cells which were treated as described in Figure 2G. (G) The ratios of β-catenin/actin and c-Myc/actin were analyzed (the means ± SEM; n = 3; *p < 0.05, **p < 0.01). (H) The mRNA level of β-catenin and c-Myc in different groups of SH-SY5Y cells, and actin served as the internal control to ensure equal loading (the means ± SEM; n = 3; *p < 0.05, **p < 0.01).
Als Protected SH-SY5Y Cells From the MPP+-Induced Mitochondrial Fission via c-Myc
The β-catenin-overexpressing or β-catenin-knockdown SH-SY5Y cells were pretreated with 0.5 μM Als for 24 h before adding MPP+. The level of mitochondrial fission and fusion was determined using MitoTracker (Figures 4A,B). The results showed that MPP+ induced significant mitochondrial fission. After the pretreatment of Als for 24 before adding MPP+, the mitochondrial fission caused by MPP+ was partially alleviated (Figure 4C). Conversely, knockdown of β-catenin largely abrogated the protective effect of Als against the mitochondrial fission phenotype induced by MPP+ treatment (Figure 4D).
LiCl, an inhibitor of GSK-3β, was often used to investigate the beneficial role of Wnt signaling. In the present study, both Als and LiCl could reverse the decrease of β-catenin protein induced by MPP+. However, Als was more effective than LiCl in the protection of mitochondrial fission caused by MPP+. The protective effect of Als against the mitochondrial fission phenotype induced by MPP+ could also be abrogated by knockdown of c-Myc (Supplementary Figures S3, S4).
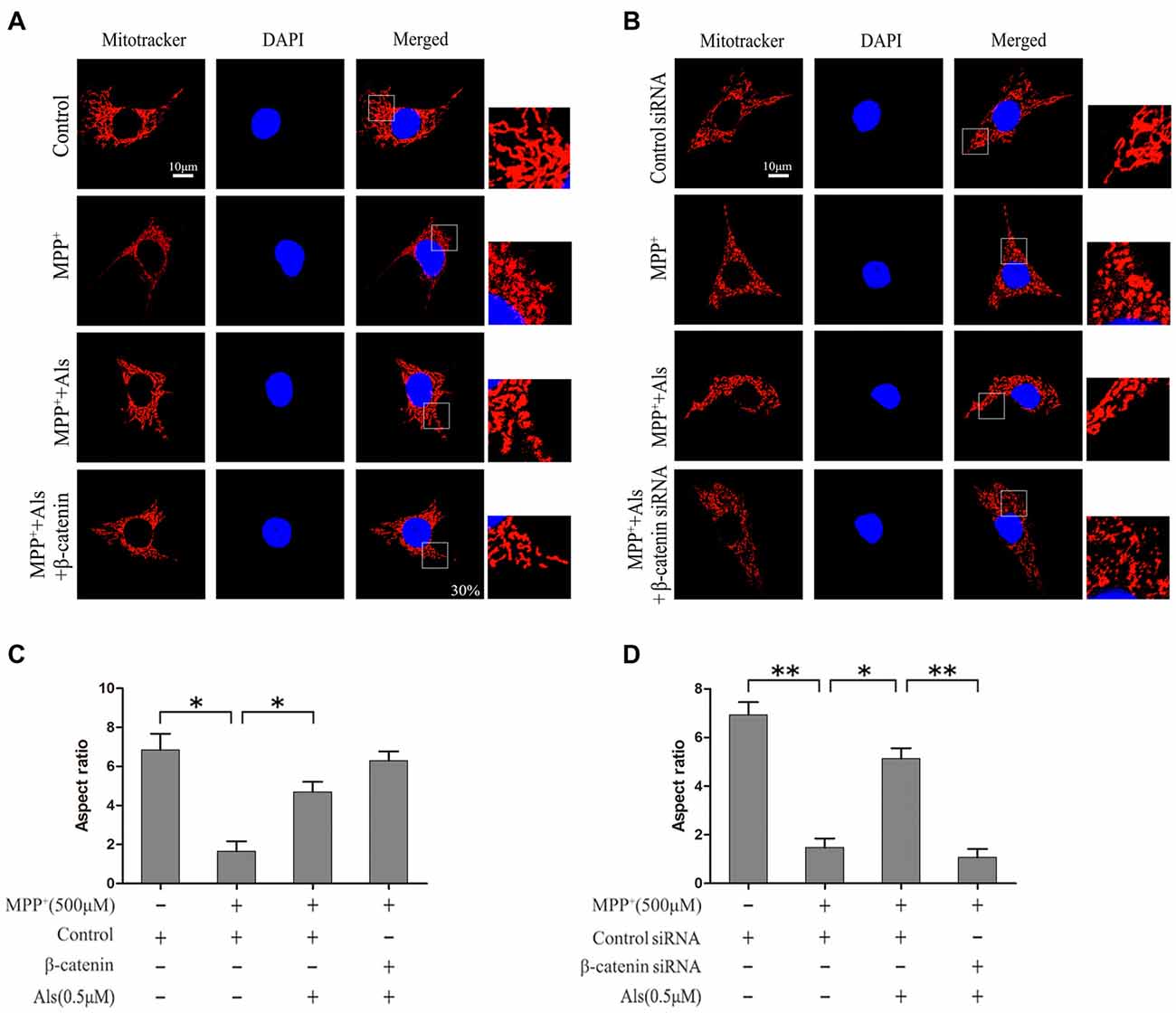
Figure 4. Protection of Als against the MPP+-induced mitochondrial fission in SH-SY5Y cells. (A) Cells were treated as described in Figure 2E. The morphology of mitochondrial was imaged using a confocal microscope (scale bar, 10 μm; Transfection efficiency, 30%). (B) Cells were treated as described in Figure 2G. Mitochondrial morphology was captured using a confocal microscope. (C,D) Quantification of the morphology of mitochondria. The ratio between the major and minor axes of an ellipse equivalent to the shape of the mitochondrial is regard as the aspect ratio of mitochondria. Twenty fields of view in a coverslip are randomly recorded to analyze the aspect ratio of mitochondria. The experiments were independently taken three times (the means ± SEM; n = 3; *p < 0.05, **p < 0.01).
MPP+ Induced SH-SY5Y Cell Apoptosis Could Be Prevented by Als via Wnt Signaling
The effect of Als on the MPP+ induced cell apoptosis was evaluated by TUNEL staining, cell viability, cleaved caspase-3 level and cleaved caspase-8 level. The results indicated that MPP+ treatment induced the increase of TUNEL-positive cells. The pretreatment of Als before adding MPP+ caused a significantly decrease in the number of TUNEL-positive SH-SY5Y cells compared with MPP+ treatment group (Figures 5A,B). These findings were also supported by the results of cell viability (Figure 5C). However, knockdown of β-catenin abolished the protective effects of Als against MPP+-induced cell apoptosis in SH-SY5Y cells, as indicated by the change of TUNEL-positive cells and cell viability (Figures 5D–F).
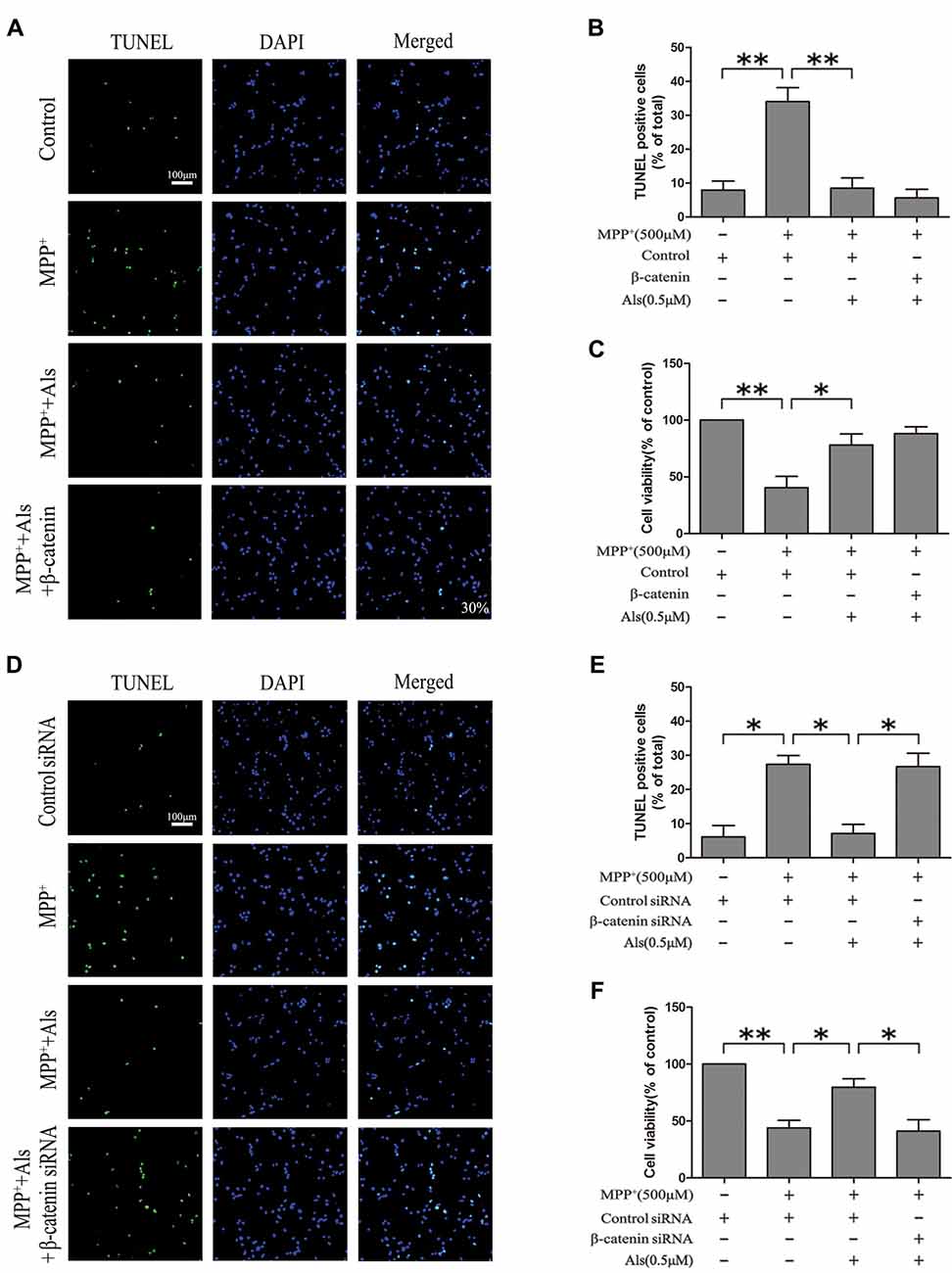
Figure 5. Modulation of the SH-SY5Y cell apoptosis by Als via the Wnt signaling. (A) SH-SY5Y cells treated as in Figure 2E were stained for TUNEL (scale bar, 100 μm; Transfection efficiency, 30%). (B) The ratios of TUNEL-positive cells/total cells were analyzed (the means ± SEM; n = 3; **p < 0.01). (C) Cells were treated as described in (A) and the cell viability was detected by the MTT assay (the means ± SEM; n = 3; *p < 0.05, **p < 0.01). (D) Cells treated as described in Figure 2G were assessed for cell apoptosis by TUNEL staining. (E) Quantification graphs of the ratios of TUNEL-positive cells/total cells (the means ± SEM; n = 3; *p < 0.05). (F) The MTT assay was conducted in cells treated as described in (D; the means ± SEM; n = 3; *p < 0.05, **p < 0.01).
Western blotting revealed that MPP+ treatment markedly increased cleaved caspase-3 and cleaved caspase-8 expression in the SH-SY5Y cells. Als pretreatment ameliorated the activation of cleaved caspase-3 and cleaved caspase-8 induced by MPP+ treatment. Furthermore, the expression of cleaved caspase-8 was decreased in β-catenin overexpression SH-SY5Y cells pretreated with Als before adding MPP+ compared with cells pretreated with Als before adding MPP+ (Figures 6A,B). However, silencing β-catenin largely abrogated this positive effect of Als against MPP+-induced up-regulation of cleaved caspase-3 and cleaved caspase-8 (Figures 6C,D, Supplementary Figure S5).
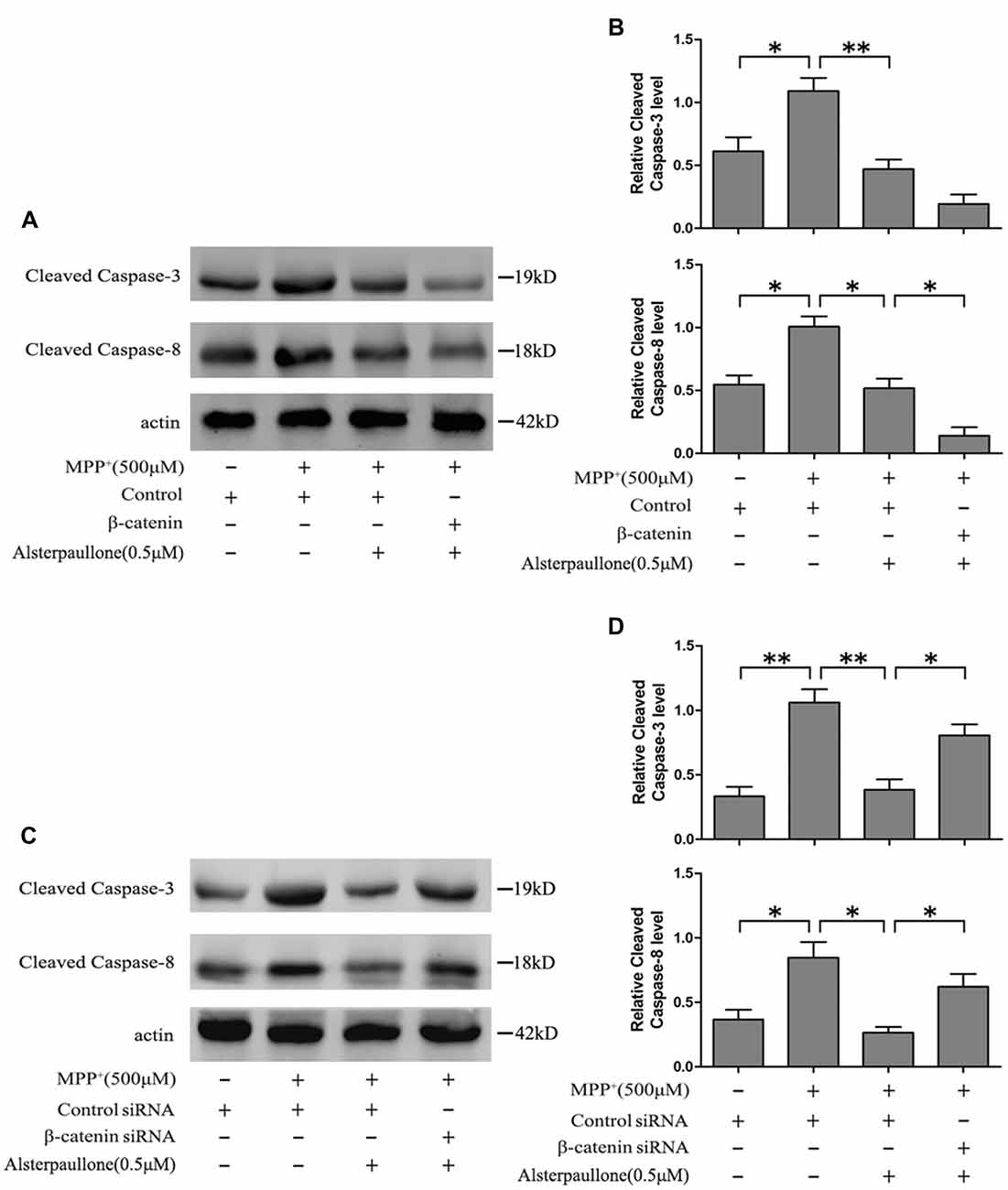
Figure 6. Als attenuated MPP+-induced mitochondrial-mediated cell apoptosis via Wnt signaling. (A) Western blot of cleaved-caspase-3, 8 expression in cells treated as described in Figure 2E. (B) The relative optical density of cleaved-caspase-3, 8 normalized to actin was analyzed (the means ± SEM; n = 3; *p < 0.05, **p < 0.01). (C) Cells were treated as described in Figure 2G and Western blot of cleaved-caspase-3, 8 expression was conducted. (D) The expression of cleaved-caspase-3, 8 was analyzed (the means ± SEM; n = 3; *p < 0.05, **p < 0.01).
Discussion
The loss of dopaminergic neurons is an important pathological feature in the progression of PD (Kaasinen and Vahlberg, 2017; Fox et al., 2018). At present the main objective of PD research is to identify a disease-modifying agent to alleviate the degeneration of dopaminergic neurons (Kim et al., 2016). It has been described that Wnt signaling is associated with the pathogenesis of PD in vivo and in vitro (Filatova et al., 2011; Dun et al., 2012; Zhang et al., 2016). Previous studies have shown that knockdown of Dkk1, a protein that can inhibit Wnt signaling, exhibited a neuroprotective effect in PC12 cells treated with MPP+ (Dun et al., 2013). The neuroprotective role of Wnt signaling in the rat PD model also has been studied (Wang Y.-L. et al., 2017).
GSK-3β is sequestered in cytoplasm fraction and targets β-catenin for ubiquitination and phosphorylation (Oh et al., 2017). Inhibition of GSK-3β function in the ventral midbrain of rats may contribute to the increase of β-catenin level (Dun et al., 2012). In the current study, western blot showed that Als, a GSK-3β inhibitor, attenuated the MPP+-induced down-regulation of c-Myc and Wnt signaling. Besides, Als alleviated the SH-SY5Y cell apoptosis induced by MPP+ treatment, as indicated by the change of cell viability and TUNEL-positive SH-SY5Y cells. Als also inhibits Cyclin-dependent kinase 5 (CDK5) which has significant role in the pathogenesis of PD (Wen et al., 2014). However, knockdown of β-catenin or c-Myc abolished the protective effects of Als against MPP+-induced cell apoptosis in SH-SY5Y cells. These results indicated that the neuroprotective effect of Als may mainly require the activation of Wnt signaling.
Mitochondria are important intercellular organelles in the nerve cells, producing energy through the mitochondrial respiratory chain and acting as a mediator of the cell survival and death (Keilhoff et al., 2016; Mendivil-Perez et al., 2016). Accumulating studies of PD animals and PD patients suggested that mitochondrial dysfunction may play crucial roles in the PD pathogenesis (Martin, 2006; Desmet et al., 2017). The fission of mitochondria produces dysfunctional organelles which can be eliminated by autophagosomal machinery. Fusion, however, is partially effective at preserving the integrity of mitochondria (Kawano et al., 1995; Graves et al., 2012). The mitochondrial abundance may be regulated by the activation of Wnt signaling (Bernkopf et al., 2018). c-Myc is an important downstream genes of Wnt signaling (Hamdoun et al., 2017). The depletion of c-Myc is associated with a rapid decrease in the mitochondrial structural integrity and function. In contrast, Overexpression of c-Myc restores the mitochondrial volume and induces an increased fusion activity (Graves et al., 2012). Then we propose that c-Myc serves to link the Wnt signaling with mitochondrial function and biogenesis via the regulation of fusion and fission processes. Als ameliorated the MPP+-induced depletion of c-Myc and this effect could be abrogated by the knockdown of β-catenin. Furthermore, Als alleviated MPP+-induced mitochondrial fission in SH-SY5Y cells. Knockdown of β-catenin or c-Myc largely abrogated the protection effect of Als against the mitochondrial dysfunction induced by the adding of MPP+. In consideration of the roles of c-Myc on regulation mitochondrial fusion and fission processes, we hypothesize that Als protects the SH-SY5Y cells from the MPP+-induced mitochondrial fission in a β-catenin- c-Myc-dependent manner.
Cleaved caspase-3 and cleaved caspase-8 expression have been shown to relate with the mitochondria-dependent apoptotic pathway (Zhang et al., 2013; Shih et al., 2017). In the present study, the activation of caspase-3 and caspase-8 induced by MPP+ could be prevented by the pretreatment of Als. Besides, Als lost its protective effect against MPP+-induced activation of caspase-3 and caspase-8 when β-catenin was knocked down in SH-SY5Y cells, indicating that Als alleviated the MPP+-induced mitochondria-dependent apoptosis via Wnt signaling.
Together with the results presented in our study, the findings indicate that Wnt signaling is a point of the pathogenesis of PD. The Wnt signaling downstream gene c-Myc directly regulates the mitochondria morphology and function. Als, a GSK-3β inhibitor, attenuates the MPP+-induced mitochondria-dependent apoptotic via activation of the Wnt signaling. Our study provides potential therapeutic implications of PD as well as the underlying molecular mechanisms of the neuroprotective effect of Als. Considering the complexity of the pathogenesis of PD, further studies should be conducted to elucidate the accurate mechanism.
Author Contributions
JW and LL designed the study. LG performed the mitochondrial staining and TUNEL assay. YL carried out the immunofluorescence and western blots. The cell culture was conducted by FY. GG and LL wrote the manuscript.
Funding
This work was supported by grants from the National Natural science Foundation of China (81401044, 81230043).
Conflict of Interest Statement
The authors declare that the research was conducted in the absence of any commercial or financial relationships that could be construed as a potential conflict of interest.
Acknowledgments
We thank Chen Li for comments on this manuscript.
Abbreviations
Als, Alsterpaullone; CDK5, Cyclin-dependent kinase 5; DMEM, Dulbecco’s modified Eagle’s medium; FBS, Fetal bovine serum; GSK-3β, glycogen synthase kinase 3β; PD, Parkinson’s Disease.
Supplementary Material
The Supplementary Material for this article can be found online at: https://www.frontiersin.org/articles/10.3389/fncel.2018.00283/full#supplementary-material
References
Bernkopf, D. B., Jalal, K., Brückner, M., Knaup, K. X., Gentzel, M., Schambony, A., et al. (2018). Pgam5 released from damaged mitochondria induces mitochondrial biogenesis via Wnt signaling. J. Cell Biol. 217, 1383–1394. doi: 10.1083/jcb.201708191
Desmet, A. S., Cirillo, C., Tack, J., Vandenberghe, W., and Vanden, B. P. (2017). Live calcium and mitochondrial imaging in the enteric nervous system of Parkinson patients and controls. Elife 6:e26850. doi: 10.7554/eLife.26850
Dun, Y., Li, G., Yang, Y., Xiong, Z., Feng, M., Wang, M., et al. (2012). Inhibition of the canonical Wnt pathway by Dickkopf-1 contributes to the neurodegeneration in 6-OHDA-lesioned rats. Neurosci. Lett. 525, 83–88. doi: 10.1016/j.neulet.2012.07.030
Dun, Y., Yang, Y., Xiong, Z., Feng, M., Zhang, Y., Wang, M., et al. (2013). Induction of Dickkopf-1 contributes to the neurotoxicity of MPP+ in PC12 cells via inhibition of the canonical Wnt signaling pathway. Neuropharmacology 67, 168–175. doi: 10.1016/j.neuropharm.2012.10.031
Filatova, E. V., Shadrina, M. I., Fedotova, E., Slominskii, P. A., Illarioshkin, S. N., Ivanova-Smolenskaia, I. A., et al. (2011). Analysis of single nucleotide polymorphism rs415430 in the WNT3 gene in the Russian population with the Parkinson disease. Mol. Gen. Mikrobiol. Virusol. 2, 3–4.
Fox, S. H., Katzenschlager, R., Lim, S. Y., Barton, B., de Bie, R., Seppi, K., et al. (2018). International Parkinson and movement disorder society evidence-based medicine review: update on treatments for the motor symptoms of Parkinson’s disease. Mov. Disord. doi: 10.1002/mds.27372 [Epub ahead of print].
Graves, J. A., Wang, Y., Sims-Lucas, S., Cherok, E., Rothermund, K., Branca, M. F., et al. (2012). Mitochondrial structure, function and dynamics are temporally controlled by c-Myc. PLoS One 7:e37699. doi: 10.1371/journal.pone.0037699
Hamdoun, S., Fleischer, E., Klinger, A., and Efferth, T. (2017). Lawsone derivatives target the Wnt/beta-catenin signaling pathway in multidrug-resistant acute lymphoblastic leukemia cells. Biochem. Pharmacol. 146, 63–73. doi: 10.1016/j.bcp.2017.10.008
Hu, W., Guan, L. S., Dang, X. B., Ren, P. Y., and Zhang, Y. L. (2014). Small-molecule inhibitors at the PSD-95/nNOS interface attenuate MPP+-induced neuronal injury through Sirt3 mediated inhibition of mitochondrial dysfunction. Neurochem. Int. 79, 57–64. doi: 10.1016/j.neuint.2014.10.005
Kaasinen, V., and Vahlberg, T. (2017). Striatal dopamine in Parkinson disease: a meta-analysis of imaging studies. Ann. Neurol. 82, 873–882. doi: 10.1002/ana.25103
Kawano, S., Takano, H., and Kuroiwa, T. (1995). Sexuality of mitochondria: fusion, recombination and plasmids. Int. Rev. Cytol. 161, 49–110. doi: 10.1016/s0074-7696(08)62496-1
Keilhoff, G., Becker, A., Kropf, S., and Schild, L. (2016). Sciatic nerve ligation causes impairment of mitochondria associated with changes in distribution, respiration and cardiolipin composition in related spinal cord neurons in rats. Mol. Cell. Biochem. 421, 41–54. doi: 10.1007/s11010-016-2782-2
Kim, I. S., Ganesan, P., and Choi, D. K. (2016). Cx43 mediates resistance against MPP+-induced apoptosis in SH-SY5Y neuroblastoma cells via modulating the mitochondrial apoptosis pathway. Int. J. Mol. Sci. 17:E1819. doi: 10.3390/ijms17111819
Koopman, W. J., Distelmaier, F., Esseling, J. J., Smeitink, J. A., and Willems, P. H. (2008). Computer-assisted live cell analysis of mitochondrial membrane potential, morphology and calcium handling. Methods 46, 304–311. doi: 10.1016/j.ymeth.2008.09.018
Kouvidi, K., Berdiaki, A., Tzardi, M., Karousou, E., Passi, A., Nikitovic, D., et al. (2016). Receptor for hyaluronic acid- mediated motility (RHAMM) regulates HT1080 fibrosarcoma cell proliferation via a β-catenin/c-myc signaling axis. Biochim. Biophys. Acta 1860, 814–824. doi: 10.1016/j.bbagen.2016.01.019
Lambert, C., Cisternas, P., and Inestrosa, N. C. (2016). Role of Wnt signaling in central nervous system injury. Mol. Neurobiol. 53, 2297–2311. doi: 10.1007/s12035-015-9138-x
Leost, M., Schultz, C., Link, A., Wu, Y. Z., Biernat, J., Mandelkow, E. M., et al. (2000). Paullones are potent inhibitors of glycogen synthase kinase-3β and cyclin-dependent kinase 5/p25. Eur. J. Biochem. 267, 5983–5994. doi: 10.1046/j.1432-1327.2000.01673.x
Martin, L. J. (2006). Mitochondriopathy in Parkinson disease and amyotrophic lateral sclerosis. J. Neuropathol. Exp. Neurol. 65, 1103–1110. doi: 10.1097/01.jnen.0000248541.05552.c4
Mendivil-Perez, M., Velez-Pardo, C., and Jimenez-Del-Rio, M. (2016). Neuroprotective effect of the LRRK2 kinase inhibitor PF-06447475 in human nerve-like differentiated cells exposed to oxidative stress stimuli: implications for Parkinson’s disease. Neurochem. Res. 41, 2675–2692. doi: 10.1007/s11064-016-1982-1
Monti, C., Bondi, H., Urbani, A., Fasano, M., and Alberio, T. (2015). Systems biology analysis of the proteomic alterations induced by MPP+, a Parkinson’s disease-related mitochondrial toxin. Front. Cell. Neurosci. 9:14. doi: 10.3389/fncel.2015.00014
Oh, S., Kim, H., Nam, K., and Shin, I. (2017). Silencing of Glut1 induces chemoresistance via modulation of Akt/GSK-3β/β-catenin/survivin signaling pathway in breast cancer cells. Arch. Biochem. Biophys. 636, 110–122. doi: 10.1016/j.abb.2017.08.009
Oliva, C. A., Montecinos-Oliva, C., and Inestrosa, N. C. (2018). Wnt signaling in the central nervous system: new insights in health and disease. Prog. Mol. Biol. Transl. Sci. 153, 81–130. doi: 10.1016/bs.pmbts.2017.11.018
Perez Carrion, M., Pischedda, F., Biosa, A., Russo, I., Straniero, L., Civiero, L., et al. (2018). The LRRK2 variant E193K prevents mitochondrial fission upon MPP+ treatment by altering LRRK2 binding to DRP1. Front. Mol. Neurosci. 11:64. doi: 10.3389/fnmol.2018.00064
Rangrez, A. Y., Eden, M., Poyanmehr, R., Kuhn, C., Stiebeling, K., Dierck, F., et al. (2016). Myozap deficiency promotes adverse cardiac remodeling via differential regulation of mitogen-activated protein kinase/serum-response factor and β-catenin/GSK-3β protein signaling. J. Biol. Chem. 291, 4128–4143. doi: 10.1074/jbc.M115.689620
Rostamian Delavar, M., Baghi, M., Safaeinejad, Z., Kiani-Esfahani, A., Ghaedi, K., and Nasr-Esfahani, M. H. (2018). Differential expression of miR-34a, miR-141 and miR-9 in MPP+-treated differentiated PC12 cells as a model of Parkinson’s disease. Gene 662, 54–65. doi: 10.1016/j.gene.2018.04.010
Sheehan, P., and Yue, Z. (2018). Deregulation of autophagy and vesicle trafficking in Parkinson’s disease. Neurosci. Lett. doi: 10.1016/j.neulet.2018.04.013 [Epub ahead of print].
Shih, Y. L., Hung, F. M., Lee, C. H., Yeh, M. Y., Lee, M. H., Lu, H. F., et al. (2017). Fisetin induces apoptosis of HSC3 human oral cancer cells through endoplasmic reticulum stress and dysfunction of mitochondria-mediated signaling pathways. In Vivo 31, 1103–1114. doi: 10.21873/invivo.11176
Shimauchi, T., Nishimura, A., Ishikawa, T., and Nishida, M. (2017). Eco-pharma of approved drug focused on mitochondria fission. Nihon Yakurigaku Zasshi 149, 269–273. doi: 10.1254/fpj.149.269
Takadera, T., Fujibayashi, M., Koriyama, Y., and Kato, S. (2012). Apoptosis induced by SRC-family tyrosine kinase inhibitors in cultured rat cortical cells. Neurotox. Res. 21, 309–316. doi: 10.1007/s12640-011-9284-5
Teo, R., Möhrlen, F., Plickert, G., Muller, W. A., and Frank, U. (2006). An evolutionary conserved role of Wnt signaling in stem cell fate decision. Dev. Biol. 289, 91–99. doi: 10.1016/j.ydbio.2005.10.009
Wang, B., Cai, Z., Tao, K., Zeng, W., Lu, F., Yang, R., et al. (2016). Essential control of mitochondrial morphology and function by chaperone-mediated autophagy through degradation of PARK7. Autophagy 12, 1215–1228. doi: 10.1080/15548627.2016.1179401
Wang, Y.-L., Ju, B., Zhang, Y. Z., Yin, H. L., Liu, Y. J., Wang, S. S., et al. (2017). Protective effect of curcumin against oxidative stress-induced injury in rats with Parkinson’s disease through the Wnt/β-catenin signaling pathway. Cell. Physiol. Biochem. 43, 2226–2241. doi: 10.1159/000484302
Wang, J., Zhu, G., Huang, L., Nie, T., Tao, K., Li, Y., et al. (2017). Morphine administration induces change in anxiety-related behavior via Wnt/β-catenin signaling. Neurosci. Lett. 639, 199–206. doi: 10.1016/j.neulet.2017.01.005
Wen, Z., Shu, Y., Gao, C., Wang, X., Qi, G., Zhang, P., et al. (2014). CDK5-mediated phosphorylation and autophagy of RKIP regulate neuronal death in Parkinson’s disease. Neurobiol. Aging 35, 2870–2880. doi: 10.1016/j.neurobiolaging.2014.05.034
Zeng, W., Zhang, W., Lu, F., Gao, L., and Gao, G. (2017). Resveratrol attenuates MPP+-induced mitochondrial dysfunction and cell apoptosis via AKT/GSK-3β pathway in SN4741 cells. Neurosci. Lett. 637, 50–56. doi: 10.1016/j.neulet.2016.11.054
Zhang, L., Deng, J., Pan, Q., Zhan, Y., Fan, J. B., Zhang, K., et al. (2016). Targeted methylation sequencing reveals dysregulated Wnt signaling in Parkinson disease. J. Genet. Genomics 43, 587–592. doi: 10.1016/j.jgg.2016.05.002
Keywords: Parkinson’s disease, mitochondria, wnt signaling, c-Myc, alsterpaullone
Citation: Wang J, Li Y, Gao L, Yan F, Gao G and Li L (2018) GSK-3β Inhibitor Alsterpaullone Attenuates MPP+-Induced Cell Damage in a c-Myc-Dependent Manner in SH-SY5Y Cells. Front. Cell. Neurosci. 12:283. doi: 10.3389/fncel.2018.00283
Received: 23 May 2018; Accepted: 09 August 2018;
Published: 30 August 2018.
Edited by:
Ludovic Martin, Duke University, United StatesReviewed by:
Faraj Terro, University of Limoges, FranceAlejandro Lopez Tobon, Istituto Europeo di Oncologia s.r.l., Italy
Copyright © 2018 Wang, Li, Gao, Yan, Gao and Li. This is an open-access article distributed under the terms of the Creative Commons Attribution License (CC BY). The use, distribution or reproduction in other forums is permitted, provided the original author(s) and the copyright owner(s) are credited and that the original publication in this journal is cited, in accordance with accepted academic practice. No use, distribution or reproduction is permitted which does not comply with these terms.
*Correspondence: Lihong Li, bGlob25nbGk3NzdAMTYzLmNvbQ==
Guodong Gao, Z2d1b2RvbmdAb3V0bG9vay5jb20=
† These authors have contributed equally to this work