Erratum on: Presynaptic mechanisms of neuronal plasticity and their role in epilepsy
- 1RNA Editing and Hyperexcitability Disorders, Max Delbrück Centre for Molecular Medicine, Neurosciences, Berlin, Germany
- 2Oscar Langendorff Institute of Physiology, University of Rostock, Rostock, Germany
Synaptic communication requires constant adjustments of pre- and postsynaptic efficacies. In addition to synaptic long term plasticity, the presynaptic machinery underlies homeostatic regulations which prevent out of range transmitter release. In this minireview we will discuss the relevance of selected presynaptic mechanisms to epilepsy including voltage- and ligand-gated ion channels as well as cannabinoid and adenosine receptor signaling.
Introduction
Many studies highlighted the importance of homeostasis in neuronal signaling either within neurons, then called “intrinsic plasticity” or between neurons, referred to as “synaptic scaling” (Davis and Bezprozvanny, 2001; Eichler and Meier, 2008; Turrigiano, 2011). Classic synaptic plasticity in its non-homeostatic “Hebbian” form and pathological disturbances need counterbalancing homeostatic scaling mechanisms (Abbott and Nelson, 2000). The latter was mainly regarded from the postsynaptic perspective (Thiagarajan et al., 2005; Groth et al., 2011). However, in addition to presynaptic expression of synaptic long term plasticity (Nicoll and Schmitz, 2005), slow homeostatic regulations occur in the presynapse, e.g., in form of chronic receptor or ion channel modulations.
Presynaptic Ion Channel and Glycine Receptor Plasticity
Because transmitter release is controlled by action potential-(AP)-triggered calcium influx in the synaptic terminal, regulation of ion channels which shape the axonal AP and terminal depolarization is an effective mechanism of presynaptic plasticity. With this definition, the AP initiation zone (AIZ) could be viewed as part of the presynaptic equipment. A striking form of homeostatic plasticity has been documented for the AIZ: this entire subcellular structure including voltage-gated Na+ (Nav) and K+ (Kv) channels can be shifted along the axon (Figure 1), thereby counteracting hyperexcitation by increasing thresholds for AP generation (Grubb and Burrone, 2010). Such axonal remodeling may be facilitated via ion channel trafficking regulated by alternative splicing, as shown for “shaw-related” Kv3 channels (Gu et al., 2012). Other important constituents of presynaptic control are “shaker-related” KV1 channels (Wang et al., 1994). Their role is well demonstrated for the large glutamatergic mossy fiber boutons of dentate granule cells, which impinge on hippocampal CA3 pyramidal cells (Geiger and Jonas, 2000; Bischofberger et al., 2006). Here, Kv channels could gain further importance during temporal lobe epilepsy (TLE), when seizures invade the hippocampus and feedforward inhibition of CA3 pyramidal cells via interneurons is compromised (Lawrence and McBain, 2003). Indeed, seizures trigger a transcriptional upregulation of Kv1.1 channels in granule cells, thereby delaying their AP responses considerably, as recently shown in a TLE mouse model (Kirchheim et al., 2013). Consistent with the view that KV1.1 is a promising antiepileptic target, KV1.1 knockout mice develop epilepsy (Wenzel et al., 2007) and lentiviral overexpression of KV1.1 ameliorates seizures in an animal model of neocortical epilepsy (Wykes et al., 2012). The interaction of activity-dependent downscaling and potentiation of presynaptic excitability may involve the adenylyl cyclase pathway (Nicoll and Schmitz, 2005) but it is still unclear how exactly these seemingly opposed mechanisms interact in the same presynaptic compartment.
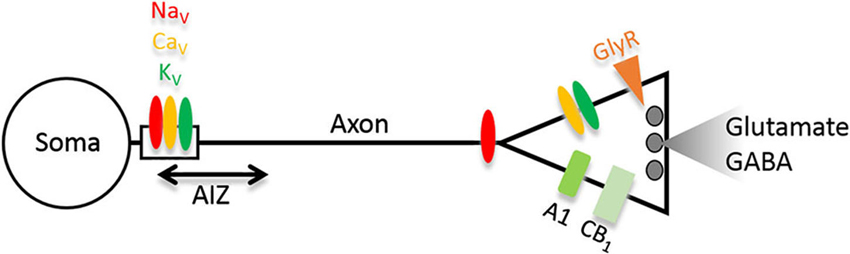
Figure 1. Scheme depicting strategic molecules relevant for homeostasis of presynaptic function and epilepsy discussed in this minireview. In glutamatergic neurons, gain-of-function of molecules colored red/orange will increase network excitability, whereas those colored green will decrease it. The opposite holds true if the changes occur in GABAergic neurons. Abbreviations: NaV, CaV, KV, voltage-gated sodium, calcium and potassium channels; AIZ, action potential (AP) initiation zone; GlyR, glycine receptors; A1, adenosine receptor; CB1, cannabinoid receptor 1.
Epilepsy often comes with cognitive dysfunction and neuropsychiatric comorbidities (García-Morales et al., 2008). We discovered a molecule which in this regard may have an important impact: an RNA variant of the neurotransmitter receptor for glycine (GlyR). The GlyRs are subject to increased RNA editing in resected hippocampi of TLE patients (Eichler et al., 2008) which profoundly influences biophysical receptor properties. The reason is an amino acid substitution in the ligand binding domain leading to gain-of-function receptors with increased neurotransmitter affinity (Meier et al., 2005; Eichler et al., 2008; Legendre et al., 2009) and spontaneous channel activity (Kletke et al., 2013; Winkelmann et al., 2014). In addition, RNA splicing governs presynaptic GlyR expression (Winkelmann et al., 2014), and in hippocampal neurons, the lack of the GlyR β subunit (Weltzien et al., 2012) which governs postsynaptic receptor clustering (Meyer et al., 1995; Meier et al., 2000, 2001; Eichler et al., 2009; Förstera et al., 2010; Kowalczyk et al., 2013), certainly facilitates GlyR expression and function at presynapses (Figure 1). Presynaptic GlyRs are tightly packed (~200 receptor channels in a cluster with ~100 nm radius; Notelaers et al., 2012, 2014a,b), which implies that a single presynaptic cluster from the spontaneously active GlyR RNA variant will have a considerable functional impact on synaptic neurotransmitter release, even if the contribution of the glycinergic system to this brain region appears limited (Zeilhofer et al., 2005). Consistent with the excitatory nature of presynaptic chloride channels and the well documented presynaptic GlyR expression in the hippocampus (Kubota et al., 2010; Ruiz et al., 2010; Waseem and Fedorovich, 2010; Winkelmann et al., 2014), we found that the spontaneously active GlyR RNA variant actually increased presynaptic excitability and the functional impact of glutamatergic neurons or parvalbumin-positive interneurons in vivo and, depending on the type of neuron, triggered cognitive dysfunction or anxiety in our mouse model of epilepsy (Winkelmann et al., 2014). In agreement with the proposed critical role of presynaptic GlyRs in the regulation of neural network excitability, application of a low, non-receptor-saturating, glycine concentration (10 µM) to corticohippocampal slice preparations was sufficient to enhance epileptiform activity induced by block of KV1 channels (Chen et al., 2014).
Retrograde Autocrine and Paracrine Signaling
Although the idea of cannabis as a potential antiepileptic drug is ancient, it remained elusive how it could work reliably (Adams and Martin, 1996; Miller, 2013). Recent discoveries on endogenous cannabinoid receptors (CB), of which particularly CB1 is widely expressed in presynaptic terminals of excitatory and inhibitory neurons (Figure 1), could lead to a better understanding of CB mechanisms in epilepsy (Alger, 2004; Katona and Freund, 2008; Hill et al., 2012). In GABAergic neurons, activation of CB1, e.g., via neuronal activity-dependent retrograde post-to-presynapse release of CBs anandamide or 2-AG has been shown to decrease synaptic GABA release, a mechanism termed depolarization-induced suppression of inhibition (DSI; Ohno-Shosaku et al., 2001; Wilson et al., 2001). Consistently, elevated CB1 presence observed in epilepsy models and TLE patients (Goffin et al., 2011; Karlócai et al., 2011; Bojnik et al., 2012) has been interpreted as proconvulsive (Chen et al., 2003, 2007). On the other hand, CB1 is also expressed on glutamatergic terminals, where its activation reduces glutamate release (Domenici et al., 2006; Kawamura et al., 2006). Furthermore, CB1 activation increases inward rectifier K+ (Kir) currents (Mackie et al., 1995; Chemin et al., 2001) mediated via postsynaptic channels which are also upregulated in TLE (Young et al., 2009; Stegen et al., 2012). In summary, while elevation of CB1 at GABAergic synapses and reduction at glutamatergic synapses likely constitute endogenous adaptations to epilepsy, exogenous CB1 overexpression and activation in principal neurons, possibly via receptors physiologically rarely activated, could effectively protect against seizures (Blair et al., 2006; Guggenhuber et al., 2010; Hofmann and Frazier, 2013).
Adenosine triphosphate (ATP) is released from astrocytes and can enhance neuronal excitability through its direct action onto purinergic receptors. However, ATP can also exert indirect effects upon its enzymatic conversion to adenosine and signaling through adenosine A1 receptors, which reduces synaptic glutamate release (Nicoll and Schmitz, 2005; Boison, 2013; Dias et al., 2013). Therefore, adenosine signaling is another mechanism of presynaptic homeostasis with recognized relevance to epilepsy; while too much adenosine clearance via gliosis-enhanced adenosine kinase activity is a proconvulsive factor, adenosine augmentation in the epileptic focus represents a powerful anticonvulsive principle (Boison, 2012).
Perspective
In agreement with the proposed critical role of presynaptic compartments in the regulation of neural network homeostasis, diverse pharmacological agents with a presynaptic mode of action were reported to be effective in the treatment of epilepsy. In particular drugs which provide rapid adaptation against excessive excitation, e.g., via use-dependent inhibition of Nav or Cav channels (phenytoin, carbamazepine, lamotrigine, topiramate, and levetiracetam), likely act primarily in axons (Stefani et al., 1996; Wu et al., 1998; Catterall, 1999; Vogl et al., 2012). Interestingly, these drugs also have effects on psychiatric symptoms (Barbosa et al., 2003; Lexi-Comp, 2009; Andrus and Gilbert, 2010) indicating common underlying mechanisms of cognitive dysfunction and psychiatric symptoms of epilepsy. With more research on neuron type-specific roles in behavior (Lovett-Barron et al., 2014; Winkelmann et al., 2014), new antiepileptic strategies could ground on these insights and specifically target presynaptic molecules in the affected cell types.
Conflict of Interest Statement
The authors declare that the research was conducted in the absence of any commercial or financial relationships that could be construed as a potential conflict of interest.
References
Abbott, L. F., and Nelson, S. B. (2000). Synaptic plasticity: taming the beast. Nat. Neurosci. 3(Suppl), 1178–1183. doi: 10.1038/81453
Adams, I. B., and Martin, B. R. (1996). Cannabis: pharmacology and toxicology in animals and humans. Addiction 91, 1585–1614. doi: 10.1046/j.1360-0443.1996.911115852.x
Alger, B. E. (2004). Endocannabinoids and their implications for epilepsy. Epilepsy Curr. 4, 169–173. doi: 10.1111/j.1535-7597.2004.04501.x
Andrus, M. R., and Gilbert, E. (2010). Treatment of civilian and combat-related posttraumatic stress disorder with topiramate. Ann. Pharmacother. 44, 1810–1816. doi: 10.1345/aph.1P163
Barbosa, L., Berk, M., and Vorster, M. (2003). A double-blind, randomized, placebo-controlled trial of augmentation with lamotrigine or placebo in patients concomitantly treated with fluoxetine for resistant major depressive episodes. J. Clin. Psychiatry 64, 403–407. doi: 10.4088/jcp.v64n0407
Bischofberger, J., Engel, D., Frotscher, M., and Jonas, P. (2006). Timing and efficacy of transmitter release at mossy fiber synapses in the hippocampal network. Pflugers Arch. 453, 361–372. doi: 10.1007/s00424-006-0093-2
Blair, R. E., Deshpande, L. S., Sombati, S., Falenski, K. W., Martin, B. R., and DeLorenzo, R. J. (2006). Activation of the cannabinoid type-1 receptor mediates the anticonvulsant properties of cannabinoids in the hippocampal neuronal culture models of acquired epilepsy and status epilepticus. J. Pharmacol. Exp. Ther. 317, 1072–1078. doi: 10.1124/jpet.105.100354
Boison, D. (2013). Adenosine kinase: exploitation for therapeutic gain. Pharmacol. Rev. 65, 906–943. doi: 10.1124/pr.112.006361
Bojnik, E., Turunc, E., Armagan, G., Kanit, L., Benyhe, S., Yalcin, A., et al. (2012). Changes in the cannabinoid (CB1) receptor expression level and G-protein activation in kainic acid induced seizures. Epilepsy Res. 99, 64–68. doi: 10.1016/j.eplepsyres.2011.10.020
Catterall, W. A. (1999). Molecular properties of brain sodium channels: an important target for anticonvulsant drugs. Adv. Neurol. 79, 441–456.
Chemin, J., Monteil, A., Perez-Reyes, E., Nargeot, J., and Lory, P. (2001). Direct inhibition of T-type calcium channels by the endogenous cannabinoid anandamide. EMBO J. 20, 7033–7040. doi: 10.1093/emboj/20.24.7033
Chen, K., Neu, A., Howard, A. L., Foldy, C., Echegoyen, J., Hilgenberg, L., et al. (2007). Prevention of plasticity of endocannabinoid signaling inhibits persistent limbic hyperexcitability caused by developmental seizures. J. Neurosci. 27, 46–58. doi: 10.1523/jneurosci.3966-06.2007
Chen, R., Okabe, A., Sun, H., Sharopov, S., Hanganu-Opatz, I. L., Kolbaev, S. N., et al. (2014). Activation of glycine receptors modulates spontaneous epileptiform activity in the immature rat hippocampus. J. Physiol. 592(Pt. 10), 2153–2168. doi: 10.1113/jphysiol.2014.271700
Chen, K., Ratzliff, A., Hilgenberg, L., Gulyas, A., Freund, T. F., Smith, M., et al. (2003). Long-term plasticity of endocannabinoid signaling induced by developmental febrile seizures. Neuron 39, 599–611. doi: 10.1016/s0896-6273(03)00499-9
Davis, G. W., and Bezprozvanny, I. (2001). Maintaining the stability of neural function: a homeostatic hypothesis. Annu. Rev. Physiol. 63, 847–869. doi: 10.1146/annurev.physiol.63.1.847
Dias, R. B., Rombo, D. M., Ribeiro, J. A., Henley, J. M., and Sebastiao, A. M. (2013). Adenosine: setting the stage for plasticity. Trends Neurosci. 36, 248–257. doi: 10.1016/j.tins.2012.12.003
Domenici, M. R., Azad, S. C., Marsicano, G., Schierloh, A., Wotjak, C. T., Dodt, H. U., et al. (2006). Cannabinoid receptor type 1 located on presynaptic terminals of principal neurons in the forebrain controls glutamatergic synaptic transmission. J. Neurosci. 26, 5794–5799. doi: 10.1523/jneurosci.0372-06.2006
Eichler, S. A., Förstera, B., Smolinsky, B., Juttner, R., Lehmann, T. N., Fahling, M., et al. (2009). Splice-specific roles of glycine receptor α3 in the hippocampus. Eur. J. Neurosci. 30, 1077–1091. doi: 10.1111/j.1460-9568.2009.06903.x
Eichler, S. A., Kirischuk, S., Jüttner, R., Schäfermeier, P. K., Legendre, P., Lehmann, T. N., et al. (2008). Glycinergic tonic inhibition of hippocampal neurons with depolarising GABAergic transmission elicits histopathological signs of temporal lobe epilepsy. J. Cell. Mol. Med. 12, 2848–2866. doi: 10.1111/j.1582-4934.2008.00357.x
Eichler, S. A., and Meier, J. C. (2008). E-I balance and human diseases - from molecules to networking. Front. Mol. Neurosci. 1:2. doi: 10.3389/neuro.02.002.2008
Förstera, B., Belaidi, A. A., Jüttner, R., Bernert, C., Tsokos, M., Lehmann, T. N., et al. (2010). Irregular RNA splicing curtails postsynaptic gephyrin in the cornu ammonis of patients with epilepsy. Brain 133, 3778–3794. doi: 10.1093/brain/awq298
García-Morales, I., de la Pena, M. P., and Kanner, A. M. (2008). Psychiatric comorbidities in epilepsy: identification and treatment. Neurologist 14, S15–S25. doi: 10.1097/01.nrl.0000340788.07672.51
Geiger, J. R., and Jonas, P. (2000). Dynamic control of presynaptic Ca(2+) inflow by fast-inactivating K(+) channels in hippocampal mossy fiber boutons. Neuron 28, 927–939. doi: 10.1016/s0896-6273(00)00164-1
Goffin, K., Van, P. W., and Van, L. K. (2011). In vivo activation of endocannabinoid system in temporal lobe epilepsy with hippocampal sclerosis. Brain 134, 1033–1040. doi: 10.1093/brain/awq385
Groth, R. D., Lindskog, M., Thiagarajan, T. C., Li, L., and Tsien, R. W. (2011). Beta Ca2+/CaM-dependent kinase type II triggers upregulation of GluA1 to coordinate adaptation to synaptic inactivity in hippocampal neurons. Proc. Natl. Acad. Sci. U S A 108, 828–833. doi: 10.1073/pnas.1018022108
Grubb, M. S., and Burrone, J. (2010). Activity-dependent relocation of the axon initial segment fine-tunes neuronal excitability. Nature 465, 1070–1074. doi: 10.1038/nature09160
Gu, Y., Barry, J., McDougel, R., Terman, D., and Gu, C. (2012). Alternative splicing regulates kv3.1 polarized targeting to adjust maximal spiking frequency. J. Biol. Chem. 287, 1755–1769. doi: 10.1074/jbc.m111.299305
Guggenhuber, S., Monory, K., Lutz, B., and Klugmann, M. (2010). AAV vector-mediated overexpression of CB1 cannabinoid receptor in pyramidal neurons of the hippocampus protects against seizure-induced excitoxicity. PLoS One 5:e15707. doi: 10.1371/journal.pone.0015707
Hill, A. J., Williams, C. M., Whalley, B. J., and Stephens, G. J. (2012). Phytocannabinoids as novel therapeutic agents in CNS disorders. Pharmacol. Ther. 133, 79–97. doi: 10.1016/j.pharmthera.2011.09.002
Hofmann, M. E., and Frazier, C. J. (2013). Marijuana, endocannabinoids and epilepsy: potential and challenges for improved therapeutic intervention. Exp. Neurol. 244, 43–50. doi: 10.1016/j.expneurol.2011.11.047
Karlócai, M. R., Toth, K., Watanabe, M., Ledent, C., Juhasz, G., Freund, T. F., et al. (2011). Redistribution of CB1 cannabinoid receptors in the acute and chronic phases of pilocarpine-induced epilepsy. PLoS One 6:e27196. doi: 10.1371/journal.pone.0027196
Katona, I., and Freund, T. F. (2008). Endocannabinoid signaling as a synaptic circuit breaker in neurological disease. Nat. Med. 14, 923–930. doi: 10.1038/nm.f.1869
Kawamura, Y., Fukaya, M., Maejima, T., Yoshida, T., Miura, E., Watanabe, M., et al. (2006). The CB1 cannabinoid receptor is the major cannabinoid receptor at excitatory presynaptic sites in the hippocampus and cerebellum. J. Neurosci. 26, 2991–3001. doi: 10.1523/jneurosci.4872-05.2006
Kirchheim, F., Tinnes, S., Haas, C. A., Stegen, M., and Wolfart, J. (2013). Regulation of action potential delays via voltage-gated potassium Kv1.1 channels in dentate granule cells during hippocampal epilepsy. Front. Cell. Neurosci. 7:248. doi: 10.3389/fncel.2013.00248
Kletke, O., Sergeeva, O. A., Lorenz, P., Oberland, S., Meier, J. C., Hatt, H., et al. (2013). New insights in endogenous modulation of ligand-gated ion channels: Histamine is an inverse agonist at strychnine sensitive glycine receptors. Eur. J. Pharmacol. 710, 59–66. doi: 10.1016/j.ejphar.2013.04.002
Kowalczyk, S., Winkelmann, A., Smolinsky, B., Förstera, B., Neundorf, I., Schwarz, G., et al. (2013). Direct binding of GABA(A) receptor β2 and β3 subunits to gephyrin. Eur. J. Neurosci. 37, 544–554. doi: 10.1111/ejn.12078
Kubota, H., Alle, H., Betz, H., and Geiger, J. R. (2010). Presynaptic glycine receptors on hippocampal mossy fibers. Biochem. Biophys. Res. Commun. 393, 587–591. doi: 10.1016/j.bbrc.2010.02.019
Lawrence, J. J., and McBain, C. J. (2003). Interneuron diversity series: containing the detonation—feedforward inhibition in the CA3 hippocampus. Trends Neurosci. 26, 631–640. doi: 10.1016/j.tins.2003.09.007
Legendre, P., Förstera, B., Jüttner, R., and Meier, J. C. (2009). Glycine receptors caught between genome and proteome—functional implications of RNA editing and splicing. Front. Mol. Neurosci. 2:23. doi: 10.3389/neuro.02.023.2009
Lexi-Comp. (2009). “Carbamazepine,” in The Merck Manual, ed R. S. Porter (Whitehouse Station, N.J., U.S.A: Merck Sharp and Dohme Corp., a subsidiary of Merck and Co., Inc.).
Lovett-Barron, M., Kaifosh, P., Kheirbek, M. A., Danielson, N., Zaremba, J. D., Reardon, T. R., et al. (2014). Dendritic inhibition in the hippocampus supports fear learning. Science 343, 857–863. doi: 10.1126/science.1247485
Mackie, K., Lai, Y., Westenbroek, R., and Mitchell, R. (1995). Cannabinoids activate an inwardly rectifying potassium conductance and inhibit Q-type calcium currents in AtT20 cells transfected with rat brain cannabinoid receptor. J. Neurosci. 15, 6552–6561.
Meier, J. C., Henneberger, C., Melnick, I., Racca, C., Harvey, R. J., Heinemann, U., et al. (2005). RNA editing produces glycine receptor α3P185L resulting in high agonist potency. Nat. Neurosci. 8, 736–744. doi: 10.1038/nn1467
Meier, J., Meunier-Durmort, C., Forest, C., Triller, A., and Vannier, C. (2000). Formation of glycine receptor clusters and their accumulation at synapses. J. Cell Sci. 113, 2783–2795.
Meier, J., Vannier, C., Serge, A., Triller, A., and Choquet, D. (2001). Fast and reversible trapping of surface glycine receptors by gephyrin. Nat. Neurosci. 4, 253–260. doi: 10.1038/85099
Meyer, G., Kirsch, J., Betz, H., and Langosch, D. (1995). Identification of a gephyrin binding motif on the glycine receptor beta subunit. Neuron 15, 563–572. doi: 10.1016/0896-6273(95)90145-0
Miller, J. W. (2013). Slim evidence for cannabinoids for epilepsy. Epilepsy Curr. 13, 81–82. doi: 10.5698/1535-7597-13.2.81
Nicoll, R. A., and Schmitz, D. (2005). Synaptic plasticity at hippocampal mossy fibre synapses. Nat. Rev. Neurosci. 6, 863–876. doi: 10.1038/nrn1786
Notelaers, K., Smisdom, N., Rocha, S., Janssen, D., Meier, J. C., Rigo, J. M., et al. (2012). Ensemble and single particle fluorimetric techniques in concerted action to study the diffusion and aggregation of the glycine receptor alpha3 isoforms in the cell plasma membrane. Biochim. Biophys. Acta 1818, 3131–3140. doi: 10.1016/j.bbamem.2012.08.010
Notelaers, K., Rocha, S., Paesen, R., Smisdom, N., De, C. B., Meier, J. C., et al. (2014a). Analysis of alpha3 GlyR single particle tracking in the cell membrane. Biochim. Biophys. Acta 1843, 544–553. doi: 10.1016/j.bbamcr.2013.11.019
Notelaers, K., Rocha, S., Paesen, R., Swinnen, N., Vangindertael, J., Meier, J. C., et al. (2014b). Membrane distribution of the glycine receptor alpha3 studied by optical super-resolution microscopy. Histochem. Cell Biol. doi: 10.1007/s00418-014-1197-y. [Epub ahead of print].
Ohno-Shosaku, T., Maejima, T., and Kano, M. (2001). Endogenous cannabinoids mediate retrograde signals from depolarized postsynaptic neurons to presynaptic terminals. Neuron 29, 729–738. doi: 10.1016/s0896-6273(01)00247-1
Ruiz, A., Campanac, E., Scott, R. S., Rusakov, D. A., and Kullmann, D. M. (2010). Presynaptic GABAA receptors enhance transmission and LTP induction at hippocampal mossy fiber synapses. Nat. Neurosci. 13, 431–438. doi: 10.1038/nn.2512
Stefani, A., Spadoni, F., Siniscalchi, A., and Bernardi, G. (1996). Lamotrigine inhibits Ca2+ currents in cortical neurons: functional implications. Eur. J. Pharmacol. 307, 113–116. doi: 10.1016/0014-2999(96)00265-8
Stegen, M., Kirchheim, F., Hanuschkin, A., Staszewski, O., Veh, R. W., and Wolfart, J. (2012). Adaptive intrinsic plasticity in human dentate gyrus granule cells during temporal lobe epilepsy. Cereb. Cortex 22, 2087–2101. doi: 10.1093/cercor/bhr294
Thiagarajan, T. C., Lindskog, M., and Tsien, R. W. (2005). Adaptation to synaptic inactivity in hippocampal neurons. Neuron 47, 725–737. doi: 10.1016/j.neuron.2005.06.037
Turrigiano, G. (2011). Too many cooks? Intrinsic and synaptic homeostatic mechanisms in cortical circuit refinement. Annu. Rev. Neurosci. 34, 89–103. doi: 10.1146/annurev-neuro-060909-153238
Vogl, C., Mochida, S., Wolff, C., Whalley, B. J., and Stephens, G. J. (2012). The synaptic vesicle glycoprotein 2A ligand levetiracetam inhibits presynaptic Ca2+ channels through an intracellular pathway. Mol. Pharmacol. 82, 199–208. doi: 10.1124/mol.111.076687
Wang, H., Kunkel, D. D., Schwartzkroin, P. A., and Tempel, B. L. (1994). Localization of Kv1.1 and Kv1.2, two K channel proteins, to synaptic terminals, somata and dendrites in the mouse brain. J. Neurosci. 14, 4588–4599.
Waseem, T. V., and Fedorovich, S. V. (2010). Presynaptic glycine receptors influence plasma membrane potential and glutamate release. Neurochem. Res. 35, 1188–1195. doi: 10.1007/s11064-010-0174-7
Weltzien, F., Puller, C., O’Sullivan, G. A., Paarmann, I., and Betz, H. (2012). Distribution of the glycine receptor beta-subunit in the mouse CNS as revealed by a novel monoclonal antibody. J. Comp. Neurol. 520, 3962–3981. doi: 10.1002/cne.23139
Wenzel, H. J., Vacher, H., Clark, E., Trimmer, J. S., Lee, A. L., Sapolsky, R. M., et al. (2007). Structural consequences of Kcna1 gene deletion and transfer in the mouse hippocampus. Epilepsia 48, 2023–2046. doi: 10.1111/j.1528-1167.2007.01189.x
Wilson, R. I., Kunos, G., and Nicoll, R. A. (2001). Presynaptic specificity of endocannabinoid signaling in the hippocampus. Neuron 31, 453–462. doi: 10.1016/s0896-6273(01)00372-5
Winkelmann, A., Maggio, N., Eller, J., Caliskan, G., Semtner, M., Häussler, U., et al. (2014). Changes in neural network homeostasis trigger neuropsychiatric symptoms. J. Clin. Invest. 124, 696–711. doi: 10.1172/jci71472
Wu, S. P., Tsai, J. J., and Gean, P. W. (1998). Frequency-dependent inhibition of neuronal activity by topiramate in rat hippocampal slices. Br. J. Pharmacol. 125, 826–832. doi: 10.1038/sj.bjp.0702096
Wykes, R. C., Heeroma, J. H., Mantoan, L., Zheng, K., MacDonald, D. C., Deisseroth, K., et al. (2012). Optogenetic and potassium channel gene therapy in a rodent model of focal neocortical epilepsy. Sci. Transl. Med. 4:161ra152. doi: 10.1126/scitranslmed.3004190
Young, C. C., Stegen, M., Bernard, R., Muller, M., Bischofberger, J., Veh, R. W., et al. (2009). Upregulation of inward rectifier K+ (Kir2) channels in dentate gyrus granule cells in temporal lobe epilepsy. J. Physiol. 587, 4213–4233. doi: 10.1113/jphysiol.2009.170746
Keywords: epilepsy, axon, RNA editing, potassium channels, glycine receptor, homeostatic regulation, neuropsychiatric disorders, hippocampus
Citation: Meier JC, Semtner M, Winkelmann A and Wolfart J (2014) Presynaptic mechanisms of neuronal plasticity and their role in epilepsy. Front. Cell. Neurosci. 8:164. doi: 10.3389/fncel.2014.00164
Received: 22 April 2014; Accepted: 29 May 2014;
Published online: 17 June 2014.
Edited by:
Jaichandar Subramanian, Massachusetts Institute of Technology, USAReviewed by:
Detlev Boison, Legacy Research Institute, USARory McQuiston, Virginia Commonwealth University, USA
Copyright © 2014 Meier, Semtner, Winkelmann and Wolfart. This is an open-access article distributed under the terms of the Creative Commons Attribution License (CC BY). The use, distribution or reproduction in other forums is permitted, provided the original author(s) or licensor are credited and that the original publication in this journal is cited, in accordance with accepted academic practice. No use, distribution or reproduction is permitted which does not comply with these terms.
*Correspondence: Jochen C. Meier, RNA Editing and Hyperexcitability Disorders, Max Delbrück Centre for Molecular Medicine, Neurosciences, Building C31.2, Robert-Rössle-Straße 10, 13125 Berlin, Germany e-mail: jochen.meier@mdc-berlin.de