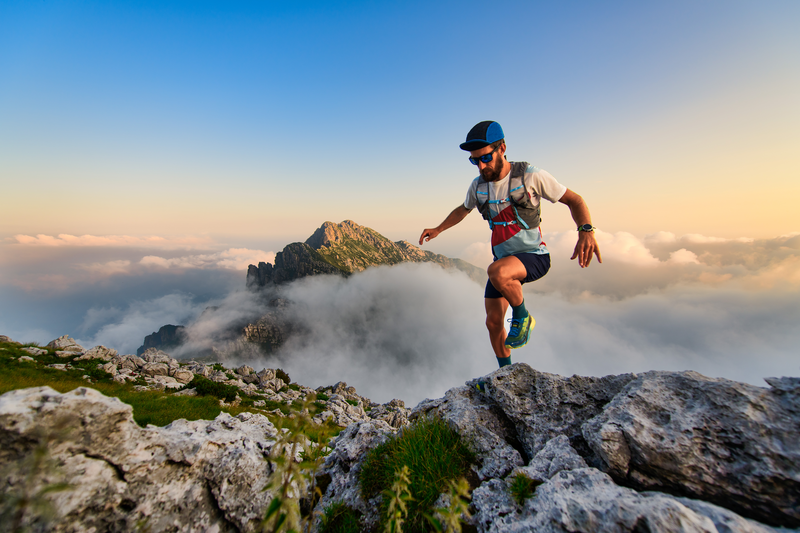
95% of researchers rate our articles as excellent or good
Learn more about the work of our research integrity team to safeguard the quality of each article we publish.
Find out more
ORIGINAL RESEARCH article
Front. Behav. Neurosci. , 17 October 2024
Sec. Learning and Memory
Volume 18 - 2024 | https://doi.org/10.3389/fnbeh.2024.1478656
This article is part of the Research Topic Recent Advances in the Stabilization and Integration of Associative Memories View all articles
Changes in memory performance are one of the main symptoms of normal aging. The storage of similar experiences as different memories (ie. behavioral pattern separation), becomes less efficient as aging progresses. Studies have focused on hippocampus dependent spatial memories and their role in the aging related deficits in behavioral pattern separation (BPS) by targeting high similarity interference conditions. However, parahippocampal cortices such as the perirhinal cortex are also particularly vulnerable to aging. Middle age is thought to be the stage where mild mnemonic deficits begin to emerge. Therefore, a better understanding of the timing of the spatial and object domain memory impairment could shed light over how plasticity changes in the parahipocampal-hippocampal system affects mnemonic function in early aging. In the present work, we compared the performance of young and middle-aged rats in both spatial (spontaneous location recognition) and non-spatial (spontaneous object recognition) behavioral pattern separation tasks to understand the comparative progression of these deficits from early stages of aging. Moreover, we explored the impact of environmental enrichment (EE) as an intervention with important translational value. Although a bulk of studies have examined the contribution of EE for preventing age related memory decline in diverse cognitive domains, there is limited knowledge of how this intervention could specifically impact on BPS function in middle-aged animals. Here we evaluate the effects of EE as modulator of BPS, and its ability to revert the deficits caused by normal aging at early stages. We reveal a domain-dependent impairment in behavioral pattern separation in middle-aged rats, with spatial memories affected independently of the similarity of the experiences and object memories only affected when the stimuli are similar, an effect that could be linked to the higher interference seen in this group. Moreover, we found that EE significantly enhanced behavioral performance in middle-aged rats in the spatial and object domain, and this improvement is specific of the high similarity load condition. In conclusion, these results suggest that memory is differentially affected by aging in the object and spatial domains, but that BPS function is responsive to an EE intervention in a multidomain manner.
Memory impairment is part of the aging process both in animals (Barnes et al., 1980; Barnes, 1988; Rapp and Amaral, 1992; Gage et al., 1988; Rapp and Heindel, 1994; Gallagher and Colombo, 1995; Lester et al., 2017) and humans (Rapp and Heindel, 1994; Lester et al., 2017; Craik, 1990; Gabrieli, 1996; Alexander et al., 2012). Aging has been associated with a disproportionate decrease in performance in particular cognitive domains, such as episodic and spatial memory (Lester et al., 2017; Barnes, 1979; Bohbot et al., 2012; Techentin et al., 2014; Isingrini and Taconnat, 2008; Spencer and Raz, 1995; Moffat, 2009; Bach et al., 1999; Ces et al., 2018; Creer et al., 2010; Rapp et al., 1997; Lacreuse et al., 2014; Moffat et al., 2006; Oler and Markus, 1998; Gallagher and Pelleymounter, 1988; Dunnett et al., 1988; Markowska et al., 1989; Cansino, 2009; O'Callaghan et al., 2009). The deficits found go from a reduced ability to maintain contextual details of an experience (Cansino, 2009; McDonough et al., 2014) to an increase in the susceptibility to interference (Talamini and Gorree, 2012) and a tendency to treat novel stimuli as familiar (ie. false recognition memory) (Yeung et al., 2014; Pidgeon and Morcom, 2014; Burke et al., 2010). All these deficits combine during aging, leading to retention impairments that start to emerge in middle-aged animals (Bizon et al., 2009; Driscoll et al., 2006; Foster et al., 2003; Kumar et al., 2012).
Recently, aging has begun to be considered a nonlinear process, with middle age signaled as the key point of acceleration in this progression (Dohm-Hansen et al., 2024). Human research suggests that one of the earliest behavioral expressions of this decline could be the reduced ability to discriminate similar stimuli in order to avoid interference, also referred as behavioral pattern separation (BPS) (Leal and Yassa, 2015). In fact, performance of memory discrimination tasks in humans was shown to be more sensitive to cognitive decline than standardized neuropsychological screening tools like the delayed recall RAVLT test (Stark et al., 2013). In rodents, the dentate gyrus (DG) region of the HP, is crucial for discrimination of overlapping spatial and non-spatial memory, having a key function in avoiding memory interference (Gilbert et al., 2001; Bekinschtein et al., 2013; Miranda et al., 2018; Miranda et al., 2017; Sahay et al., 2011).
Clear signs of memory decline are highly documented on rodents at a late aging stage (>20 months) (Barnes et al., 1980; Creer et al., 2010; Gallagher and Pelleymounter, 1988; Burke et al., 2012; Gage et al., 1984; Gallagher and Burwell, 1989; Holmes et al., 2010; Rapp et al., 1987; Johnson et al., 2017). However, it is currently believed that is actually during middle age that cognitive decline, and in particular spatial memory deficits, begin to emerge (Bizon et al., 2009; Begega et al., 2012; Frick et al., 1995). In contrast with this, there is a higher level of discrepancies in the impairments reported for non-spatial tasks, with reports of impairment in middle age (Bouet et al., 2011) that contrast with others showing no impairment in object recognition tasks until very late stages (>30 months) (Siette et al., 2013; Teglas et al., 2019). This could be the result of a mild level of mnemonic compromise in middle-aged animals (10 to 16 months old) (Calhoun et al., 1998; Das and Magnusson, 2008; Magnusson, 2001; Magnusson, 1998; Means and Kennard, 1991; Gros and Wang, 2018), that could only be evidenced in particular conditions, such as the high interference conditions present in BPS tasks.
A bulk of studies have suggested the use of a simple behavioral treatment, environmental enrichment (EE) (a multicomponent approach that provides sensory, motor, social and cognitive stimulation), could be effective in alleviating age-related memory dysfunction (Winocur, 1998; Harati et al., 2011; Sampedro-Piquero et al., 2013; Harburger et al., 2007; Harburger et al., 2007; Frick and Fernandez, 2003; Pham et al., 1999; Kempermann et al., 1998; Bennett et al., 2006; Lores-Arnaiz et al., 2006; Mirochnic et al., 2009; Mora et al., 2007; Soffie et al., 1999). In particular, EE has been shown to improve spatial cognitive decline (Kumar et al., 2012; Sampedro-Piquero et al., 2013; Harburger et al., 2007; Frick and Fernandez, 2003; Kempermann et al., 1998; Bennett et al., 2006; Speisman et al., 2013). On the other hand, EE has also proven to improve non-spatial object recognition learning abilities in aged animals (Gresack et al., 2007; Leal-Galicia et al., 2008; Cortese et al., 2018). Moreover, the emergence of studies that indicate that this beneficial effect of enrichment can be initiated at any point in the lifespan increases the therapeutic and translational utility of this approach (Sampedro-Piquero et al., 2013; Harburger et al., 2007; Bennett et al., 2006; Frick and Benoit, 2010; Rosenzweig and Bennett, 1996), with clinical trials showing benefits of enriching activities starting at old age (Ballesteros et al., 2015; Train the Brain Consortium et al., 2017; Cintoli et al., 2021; Ngandu et al., 2015). However, studies have shown that the magnitude of the effect may depend on the time of exposure being higher for middle-aged than aged animals (Kobayashi et al., 2002). In this sense, EE in middle-aged animals emerges as model of cognitive enrichment strategies in humans that, with the right timing, could help mitigate age-related cognitive impairments. Considering that BPS is one of the first functions to be affected by aging, understanding the differential contribution of EE to this function is crucial for the translational value of EE. However, little is known of how EE could differentially impact BPS in different memory domains.
In this work, we used middle-aged population to evaluate their cross-domain memory discrimination abilities under low and high interference memory conditions. For this purpose, we used variants of an incidental memory tasks (ie. object recognition/location tasks) where we quantitatively varied the load of similarity between the to-be-remembered objects in order to reveal subtle differences in memory discrimination performance both in the spatial and non-spatial domain. Here we describe a domain-specific impairment in BPS in middle-aged rats, with spatial memories affected independently of the similarity of the experiences and object memories affected as a function of the similarity load of the experience. Moreover, we studied the ability of EE as a therapeutic strategy to improve memory discrimination abilities in middle-aged rats and discovered that EE has beneficial effects on BPS performance both in the spatial and the object domain.
The subjects were 81 Long-Evans male and female rats from our breeding colony. Young animals were 2–3 months old while middle-aged animals were 11–13 months old.
The rats were housed on a reversed 12-h light/12-h dark cycle (lights on 1900–0700), in groups of two to four. All behavioral testing was conducted during the dark phase of the cycle. Rats were food deprived to no less than 90% of their free feeding weight to increase spontaneous exploration. Water remained available ad libitum throughout the study. All experimentation was conducted in accordance with the National Animal Care and Use Committee (CICUAL).
Animals were housed in subsequently different environments, as follows: (i) the control condition (SE), correspond to the time when animals were housed in standard laboratory cages (33.5*45*21.5 cm) with two animals per cage; (ii) the environmental enrichment condition (EE), corresponds to the condition where 4–6 animals were housed at a time in a big cage with several stages (33.5*45*64.5 cm) and many bottles of water and food hoppers, tubes, and ramps that were repositioned twice a week and changed weekly, as previously described (Dorfman et al., 2013). Rats were caged in these conditions for 4 weeks (Frick and Fernandez, 2003; van Praag et al., 2000; Nithianantharajah and Hannan, 2006). As part of the EE experiment, animals were tested immediately before entering the cage (SE condition) and after 4 weeks in the cage (EE condition). All animals were tested for the s- and d-SOR, as well as the s- and d-SLR on each of these two opportunities, using different and novel objects on each of these sessions. The EE experiment began when animals were 11–12 months old. For the duration of the behavioral testing, animals remained in their housing conditions (ie. EE or SE), as depicted in Supplementary Figure S1A.
The “cognitive training” exposure protocol (CT) consisted of 5 trials of repeated exposure to similar objects (ie, objects that shared one feature AB and BC). Before the CT protocol, there was a 3-day 5 min habituation to the triangular environment for the SOR. After the habituation, animals were tested for the s-SOR task. The CT sessions started following the test of the s-SOR. The CT sessions were separated by 24 h, and lasted 5 min. Animals were placed in the triangular context and were exposed to a couple of objects that shared one feature. The objects were designed by joining two small junk object elements. On each exposure session, the objects were formed by different features that were never repeated during CT protocol of during training or test sessions of the SOR. Immediately after the CT exposure, animals were tested for the s-SOR.
For the spontaneous object recognition task (SOR), the triangular open field made of white foam board was used. Each wall was 60 cm long by 60 cm high. The circular open field (90 cm diameter, 45 cm high) used for the spontaneous location recognition task (SLR) was made of black plastic. Both open fields were situated in the middle of a dimly lit room. The walls of the triangular open field were higher to minimize the visual access to the distal cues in the room. The circular open field was surrounded by six spatial cues. The open field floor was always covered with wood shavings. A video camera was positioned over the arena, and sample and choice phases were recorded for later analysis. The objects for the SOR task were made of two different smaller objects, except for the extra-similar condition, in which they were made by three smaller objects [for examples of the objects used, (see Miranda et al., 2018; Miranda et al., 2017)]. Composite objects were made by attaching together two or three of the smaller items in the conditions described in the Results section. We always used different junk objects for our within-subject design (Miranda et al., 2018; Miranda et al., 2017). Junk object features offer different textures and curvy shapes that are not present in LEGO-based objects. For the SLR, the objects used were either soda cans or beer bottles from which the label had been removed. All objects were fixed to the floor of the open field with Blu-tack and cleaned with a 50% ethanol solution between sample and choice trials. For the SOR task, all three composite objects were aligned close to one of the walls of the arena, and positions within this line were pseudorandomly assigned.
Before every experiment, all rats were handled for 2 days and habituated to each empty context during either 5 min over 3 days (SOR) or 10 min over 5 days (SLR). The SOR and SLR task were performed as previously reported (Miranda et al., 2018; Miranda et al., 2017; Miranda et al., 2021). Briefly, for the SOR task, after habituation, the rats were exposed during a 5-min duration sample phase to three objects made of either two or three features depending on the condition. For the similar condition, two of the objects shared one feature (AB and BC) and the third object was made of two other different features (EF). For the dissimilar condition, all three objects were made of different features (AB, CD, and EF). The choice phase lasted 3 min and was conducted 24 h after the finalization of the sample phase. In this case, the animals were exposed to two objects, one novel and one familiar, that varied in composition according to the condition evaluated. For the similar condition, the novel object was made of the two nonshared features of the objects presented in the sample phase (AC), and the familiar object was a copy of the third object (EF). For the dissimilar condition, the novel object was made of two novel features (GH), and the familiar object was a copy of one of the objects presented during the sample phase (AB, CD, or EF) (see schemas in Figure 1A). We always used different objects and features for the different trials. This means that letters do not indicate the use of shared object or features across trials but only within trials (ie. they are a representation of the features that compose each object during each trial). Different features (A, B, C, etc) were used to reproduce the same task conditions in the consecutive trials of the within subject design. The rationale behind the task was that if the rats were able to separate the two similar objects, their representations should be distinct and resistant to confusion; therefore, the rats should show preference for the novel object during the retrieval phase. However, if the representations of the two similar objects were not sufficiently separated, presentation of the new object would activate a familiar representation in memory and would thus not be distinguishable. The result would be that rats should behave as if the new object was familiar.
Figure 1. Early age-related impairment in object and spatial memory discrimination in high and low interference conditions. (A) Schematic illustration of the dissimilar (Down) and similar (Up) configurations of the spontaneous object recognition (SOR) task. Bold characters represent novel objects during choice session. (B) Schematic illustration of the two configurations of the SLR task, the dissimilar version (Down) and the similar version (Up), with black circles representing the novel position during the choice session. (C) Percentage of time animals spent exploring each object in the high similarity (s-SOR, orange) and low similarity (d-SOR, violet) conditions of the SOR task both in young (left) and middle-aged (right) animals. (D) Percentage of time animals spent exploring each object in the high similarity (s-SLR, orange) and low similarity (d-SLR, violet) conditions of the SLR task both in young (left) and middle-aged (right) animals. n = 19–24. (E) Performance of young and middle-aged rats in the s-SOR and d-SOR task. (F) Performance of young and middle-aged rats in the s-SLR and d-SLR task. n = 12–14. Data expressed as the mean ± SEM; *p < 0.05, ** p < 0.01, # represents p < 0.05 against 0. Light colors represent the values for young animals and darks colors those of aged animals.
For the SLR task, after habituation, rats were exposed to three identical objects, A1, A2, and A3, during a sample phase that lasted for 10 min. For the similar SLR (s-SLR), objects A2 and A3 were placed 50° apart (20.5 cm between them) and object A3 at an equal distance from the other two. For the dissimilar SLR (d-SLR), objects A1, A2, and A3 were equidistant, 120° (49 cm between them) apart from each other. Twenty-four hours after the sample phase, rats were exposed to two new identical copies of the objects, A4 and A5, for 5 min. New identical copies were used to prevent the use of olfactory cues. During this choice phase, object A4 was placed in a familiar location (same position as in the sample phase) and object A5 was placed in a novel location. For the s-SLR task, the novel location was defined as a position exactly in between the ones in which objects A2 and A3 were located during the sample phase (see schemes in Figure 1B). For the d-SLR task, object A4 was placed in a familiar location and object A5 in a position equidistant to the previous locations of A2 and A3 (see schemas in Figure 1B).
For the experiments shown in Figure 1, either young or middle age animals were tested two times. In the case of the SLR task (Figures 1D–F), independent groups of young and middle-aged animals were tested for both the similar and dissimilar version of the SLR task. For the SOR task (Figures 1C–E), independent groups of young and middle-aged animals were tested for both the similar and dissimilar version of the SOR task.
For the experiment of Figure 2, an independent set of animals was used. Animals were first trained in the similar version of the task. There was a reminder session 24 h after, where animals were presented with a familiar object (EF) and a similar novel object (AC), as during a normal choice test session. However, after this reminder, animals were retested twice, once for probing AB memory against a novel object and another for AC memory against a novel object. These two tests were given in a counterbalanced manner.
Figure 2. Early impairment in object memory discrimination is linked to memory interference in the aged group. (A) (Up) Timeline of the experiment. After animals went through a “reminder” choice session, memory for the AB and AC objects was tested against novel objects. (Down, left) Discrimination ratio of young and middle-aged animals during the “reminder” choice session. One sample t test against zero, Young t = 4.400 p = 0.005 Aged t = 0.693 p = 0.510. (Down, right) Discrimination ratio during the second test session 48 h after the original sample session. One sample t test AB young t = 1.401 p = 0.211, AC young t = 0.224 p = 0.830, AB aged t = 3.895, p = 0.006, AC aged t = 3.057 p = 0.018. Data expressed as the mean ± SEM. Light colors represent the values for young animals and darks colors those of aged animals. n = 7–8, ** p < 0.01, # represents p < 0.05 against 0.
For the experiments shown in Figure 3, animals were tested 4 times (d-SOR, s-SOR, d-SLR, s-SLR) both before (SE condition) or after (EE condition) exposure to EE. In all the cases, the order of the tasks was counterbalanced.
Figure 3. Effect of environmental enrichment over memory discrimination performance in low and high similarity conditions of the object and spatial domain. (A) Schematic representation of the task depicting the timeline of the experiment. One year old animals living in a standard environment were tested for the similar and dissimilar versions of both the SLR and SOR (SE condition, light) and after this they went through a 4-week continuous EE intervention. At the end of this month, they were re-tested under the same similar and dissimilar versions of the task (EE condition, dark). (B) Percentage of object exploration time in animals that went through the SOR task (Left) or SLR task (right) before (SE condition) or after (EE condition). (C) Performance of animals in the SE condition or EE condition for both the high similarity and low similarity versions of the SOR task. One sample t test against zero, s-SOR SE t = 0.726 p = 0.488, s-SOR EE t = 3.214 p = 0.012, d-SOR SE t = 0.194 p = 0.088, d-SOR EE t = 0.855 p = 0.418. (D) Performance of animals in the SE condition or EE condition for both the high similarity and low similarity versions of the SLR task. One sample t test against zero, s-SLR SE t = 0.046 p = 0.964, s-SLR EE t = 3.812 p = 0.005, d-SLR SE t = 0.312 p = 0.763, d-SLR EE t = 1.983 p = 0.082. (E) Linear regression between the discrimination ratios in the high similarity condition of the object task (s-SOR) and the high similarity condition of the spatial (s-SLR) task. Data expressed as the mean ± SEM. Light colors represent the values for SE condition and darks colors those of EE condition. n = 9, *p < 0.05, # p < 0.05 against 0, (*) p < 0.07.
Exploration was recorded and scored manually for both the sample and choice phases. For all experiments, exploration of a particular object was defined as the rat having its nose directed at the object at a distance of 2 cm or less, or touching the object with its nose. Rearing with the head oriented upward did not count as exploration. Climbing over or sitting on the objects was not included. Two people scored the videos; one was blind to the novel and familiar objects.
For all the experiments, the results were expressed as a discrimination ratio that was calculated as the time exploring the novel object (SOR) or the object in the novel location (SLR) minus the time exploring the familiar object (SOR) or the object in the familiar location (SLR) divided by total exploration time [(tnovel-tfamiliar)/ttotal]. One sample t tests were used to compare discrimination ratio from the similar and dissimilar conditions against zero. For the sample phase, the percentage of time exploring each object was compared between groups using a repeated measures two-way ANOVA, with age and object as the repeated measures. For the choice phase, discrimination ratios were compared within subject using a paired t test (s-SLR vs. d-SLR, s-SOR vs. d-SOR). In case of non-normal data, non-parametric analysis was used.
To evaluate behavioral pattern separation in the object domain, a modified version of the spontaneous object recognition task was used (SOR), as in previous reports. The task was modified to evaluate the ability to discriminate objects with different degrees of similarity and is highly dependent on the PRC (Miranda et al., 2017; Miranda et al., 2021). In the object domain, this was achieved by controlling the load of object similarity using shared object elements, that were present in the similar version of the task (s-SOR, high load) but absent in the dissimilar version (d-SOR, low load) (see Methods) (Figure 1A).
During the choice session, we found that young animals performed correctly the object version of the task at both high and low load of similarity, as they showed positive discrimination indexes in both (One sample t test against 0; Young d-SOR t(19) = 8.016, p < 0.0001; Young s-SOR t(19) = 4.216, p = 0.0005) (Figure 1E). These results are in line with our previous work showing an ability in young rats to differentiate between similar object memories (Miranda et al., 2018; Miranda et al., 2017; Miranda et al., 2021). On the other side, middle-aged animals exhibited an impaired performance on the similar version of the SOR without an effect over the dissimilar version, as evidenced by its positive discrimination ratio [One sample t test against 0; s-SOR t(24) = 0.248, p = 0.806; d-SOR t(24) = 5.116 p < 0.0001] (Figure 1E). The performance on the s-SOR was significantly reduced when compared with the one of the d-SOR (Figure 1E). Further analysis revealed an interaction between age and condition, with significant differences between aged and young in the similar version of the task but not in the dissimilar [Two Way RM ANOVA, Ftask (1,41) = 4.177 p = 0.047, Fage (1,41) = 5.707, p = 0.022, Finteraction (1,41) = 9.001, p = 0.005] (Figure 1E). Moreover, we found no differences in the total time aged animals spent exploring the objects on each version of the task during the sample sessions [Paired t test, t(23) = 1.303 p = 0.202] or choice sessions [Paired t test, t(22) = 0.733 p = 0.471] (Supplementary Table S1, S1.1). All in all, these results indicate a specific deficit in the discrimination of similar object memories in middle-aged rats.
Aging, as well as affecting memory functions, could also impair visual attention and perception (Jones et al., 1995; Muir et al., 1999; McGaughy and Sarter, 1995; Sarter and Turchi, 2002). The sample session phase of the SOR task has been designed as an additional high-demand perceptual/attentional task (ie. a variant of the perceptual oddity task), in which a reduction in the time exploring the similar objects (ie. AB and BC in s-SOR) can reveal a perceptual/attentional deficit that could prevent them to be identified as different objects (Bartko et al., 2007a; Bartko et al., 2007b). However, we found no significant differences between the amount of time the animals spent exploring each of the objects during sample phase in the s-SOR [Two way RM ANOVA, Fage(1,41) = 0.748 p = 0.392 Fobj(1.82, 74.41) = 0.168 p = 0.825, Finteraction(2,82) = 0.832 p = 0.439] nor the d-SOR [Two way RM ANOVA, Fage(1.72, 70.32) = 1.930 p = 0.158 Fobj(1,41) = 2.249 p = 0.141, Finteraction(2,82) = 1.728 p = 0.184] for aged compared to young animals during sample session (Figure 1C). This result suggests that middle-aged animals have no significant attentional/perceptual deficits in the object domain that could explain their impaired s-SOR performance.
In order to explore the effect of aging over memory discrimination in the spatial domain, we used an equivalent spatial version of the object task (the SLR task), in which the similarity relies on the distance between the positions of identical objects, leading to two conditions of high (small separation, s-SLR) and low (large separation d-SLR) spatial similarity (Bekinschtein et al., 2013; Miranda et al., 2018) (see Methods) (Figure 1B). During the choice session, we found that young animals could solve both the small and large versions of the task, as they show positive discrimination indexes in both [d-SLR t(13) = 6.941, p < 0.0001; s-SLR t(13) = 5.199, p = 0.0002] that did not differ significantly between d-SOR and s-SOR (Figure 1F). This confirms that young animals can distinguish between similar object positions, as previously reported (Bekinschtein et al., 2013; Miranda et al., 2018). In contrast, middle-aged animals showed a discrimination ratio not different from zero on both the similar and dissimilar versions of the SLR [d-SLR t(11) = 0.310, p = 0.763; s-SLR t(11) = 0.399, p = 0.697] (Figure 1F). Aged animals performed significantly worse than young animals independently of the similarity condition of the task [Two Way RM ANOVA, Ftask(1,24) = 0.250 p = 0.622, Fage(1,24) = 12.16, p = 0.0019, Finteraction (1,24) = 0.515, p = 0.480] (Figure 1F). There were also no significant differences in the time spent exploring during sample session [Paired t test t(11) = 1.722, p = 0.113] or choice session [Paired t test t(11) = 0.559, p = 0.588] (Supplementary Table S1, S1.1). Moreover, there were also no differences in the amount of time spent exploring each object between the young and aged group on each task [RM Two Way ANOVA, s-SLR Fage(1,25) = 1.602 p = 0.217, Fobj(2,60) = 0.495 p = 0.613, Finteraction(2,50) = 0.100 p = 0.905, d-SLR Fage(1,25) = 2.122 p = 0.158, Fobj(2,50) = p = 0.011, Finteraction(2,50) = 0.214 p = 0.808] (Figure 1D). This result shows a highly impaired spatial memory discrimination performance (ie. both high and low similarity load), evident already at early stages of aging.
On whole, these results show that middle-aged rats have an affected spatial memory (independent of the load of similarity), while they exhibit a preserved object memory in which the deficits only manifest under a high load of object similarity.
Previous work has shown that, in conditions of high interference, memory impairment after medial temporal lobe damage can result not from the loss or inaccessibility of information but from novel information appearing as familiar (McTighe et al., 2010; Yeung et al., 2013). Taking this into account, we decided to explore whether the effect of aging on the object domain, which is dependent on the similarity, could be due to middle-aged animals falsely identifying the AC object as familiar. To explore this possibility, a new set of young and aged animals went through the s-SOR sample session and, 24 h after, they were subjected to a “reminder” choice session. The “reminder” choice session consisted of a short 3 min presentation to a novel yet similar AC object and a familiar EF object (as during the test in Figure 1E). Finally, 24 h after the “reminder” session, the memory of AC and AB was assessed against a novel object (Figure 2). The rationale of this experiment is that, if middle-aged animals identify the AC object as familiar, the test session could act as a practice of the AB memory and reinforce it when compared with young animals. In a similar manner, this false identification could lead to an apparently better memory of the AC object than young animals (for whom a 3 min choice session is normally not enough to create a long term memory (Stefanko et al., 2009)). When we compared the memory of AB and AC 48 h after the sample, we found that aged animals showed significantly higher memory of both AB and AC than young animals at 48 h [Two Way RM ANOVA Fage(1,13) = 8.028 p = 0.014, FAB-AC(1,13) = 0.643, p = 0.437, Finteraction(1,13) = 0.055, p = 0.818] (Figure 2A). This suggests that the failure of middle-aged animals to perform the similar version of the task is not due to forgetting but to an inability to differentiate AC from the prior AB mnemonic representation.
Next, we decided to investigate the capacity of an environmental enrichment (EE) protocol to improve both object and spatial domain mnemonic functions. For this purpose, a group of middle-aged rats in a standard environment (SE) was tested for both the spatial and object versions of the task. After this, animals were placed in EE for 4 weeks, and following this time animals were re-evaluated with new object sets for the same two tasks (Figure 2A). This longitudinal approach was chosen because of its enhanced sensitivity and power when compared with cross-sectional studies (Caprioli et al., 1991; Dellu et al., 1997) that better accounts for the high individual variability present in aging animals (Frick et al., 1995; Fischer et al., 1992).
In the object SOR task, during the sample session, the EE group showed a reduced total object exploration time independently of the task condition [Ftask(1,8) = 0.014 p = 0.910, FEE(1,8) = 31.65 p = 0.0005, Finteraction(1,8) = 1.099 p = 0.253] (Supplementary Table S1, S3.1). However, animals did not differ in the percentage of time they spent exploring each object before and after the EE intervention [s-SOR Fobj(1,8) = 1.773 p = 0.202, FEE(2,16) = 1.992 p = 0.196, Finteraction(2,16) = 1.082 p = 0.363; d-SOR FEE(1,8) = 3.960 p = 0.082, Fobj(2,16) = 3.101 p = 0.072, Finteraction(2,16) = 0.051 p = 0.950] (Figure 3B). There was no change in total exploration time during = the choice sessions when compared before and after the EE [Two Way RM ANOVA Ftask(1,8) = 0.063 p = 0.808, FEE(1,8) = 3.340 p = 0.105, Finteraction(1,8) = 0.260 p = 0.624] (Supplementary Table S1, S3.2). During the choice session, we found that EE increased performance for the s-SOR version of the task, but not for the d-SOR version (Figure 3C) [Paired t test Similar t(8) = 4.226 p = 0.003, Dissimilar t(8) = 0.312 p = 0.763]. On the contrary, a cognitive exposure training, where animals were exposed during 5 sessions to objects that share one feature, did not lead to an improvement in memory performance on the object recognition task (Fig. S1B).
In the spatial SLR task, during the sample session, total object exploration was reduced after EE compared to before EE while no differences in total object exploration were evidenced between task conditions [Two Way RM ANOVA Ftask(1,8) = 0.174 p = 0.687, FEE(1,8) = 7.462 p = 0.026, Finteraction(1,8) = 0.097 p = 0.764] (Supplementary Table S1, S3.1). Despite this difference, animals did not differ in the percentage of time spent exploring each object before and after the EE [s-SLR FEE(1,8) = 1.441 p = 0.264, Fobj(1.47,11.83) = 0.583 p = 0.524, Finteraction(1.5,12.8) = 0.462 p = 0.588; d-SLR FEE(1,8) = 0.301 p = 0.598, Fobj(1.48,11.87) = 1.906 p = 0.194, Finteraction(1.41,11.28) = 2.694 p = 0.121] (Figure 3D). There was also a reduction in the exploration time after the EE intervention during the choice session [Two Way RM ANOVA Ftask(1,8) = 4.84 p = 0.059, FEE(1,8) = 12.57 p = 0.007, Finteraction(1,8) = 1.686 p = 0.230] (Supplementary Table S1, S3.2). During the choice phase, EE significantly increased discrimination ratios in the similar version of the task while only trended to a significant increase in the dissimilar version (Figure 3D, Paired t test Similar t(8) = 3.018 p = 0.017, Dissimilar t(8) = 2.152 p = 0.064). When we compared the performance of animals before and after the EE intervention, we found no correlation between s-SOR and s-SLR scores before or after the intervention (Before R2 = 0.013 p = 0.766, After R2 = 0.106 p = 0.392) (Figure 3E). All in all, we found EE intervention has the potential to increase memory function in a similarity specific manner, with differential effects over the spatial and the object memory domains.
In this work, we compared the middle age progression of low and high similarity load memory deficits in the object and spatial domains. Contrary to the similarity-dependent susceptibility in the object domain, we describe a more generalized impairment in spatial domain at this early stage. Additionally, we performed experiments that suggest that this similarity dependent impairment in object recognition could be due to middle-aged animals incorrectly identifying a novel object as familiar. Moreover, we used EE as a model of experience-dependent plasticity to show that 1 month EE exposure can rescue the deficit of animals on tasks of high interference both in the object and in the spatial domain.
A bulk of studies have shown that spatial memory is particularly susceptible to the effects of aging (Barnes, 1979; Moffat et al., 2006; Harburger et al., 2007; Bennett et al., 2006; Gallagher and Nicolle, 1993), and some authors attribute this impairment to a failure in binding contextual elements of an experience (Maguire et al., 1996; Rosenbaum et al., 2001). However, there are also reports of object recognition failure in aged rats (Bartolini et al., 1996; Wiig and Burwell, 1998; de Lima et al., 2005; Pieta Dias et al., 2007; Platano et al., 2008). In particular, aging was related to an inability to detect more subtle changes in object position or features, an ability often referred to as behavioral pattern separation (BPS) (Marr, 1971; Rolls and Kesner, 2006). In humans, studies have reported that older subjects have less efficient pattern separation on both spatial (Stark et al., 2010; Holden and Gilbert, 2012) and object domain tasks (Toner et al., 2009; Yassa et al., 2011; Trelle et al., 2017). Correspondingly, failure in discrimination of similar object positions (Ces et al., 2018; Creer et al., 2010; Gracian et al., 2013; Marrone et al., 2011) and objects with overlapping features (Johnson et al., 2017; Burke et al., 2011) has also been described in aged rodents. Here, we explored in further detail the temporality of these changes. We found a deficit in spatial memory in middle-aged animals that was independent of the similarity load of the task, suggesting that spatial memory function per se was already altered at this early stage and that this deficit is not specific to the BPS function. Certainly, it remains possible that earlier time points or lower interference loads in the task would reveal a BPS specific impairment.
The spatial pattern separation function is linked to the presence of adult neurogenesis in the dentate gyrus (DG) of the hippocampus (HP) and adult born neuronal integration as part of its circuitry (Sahay et al., 2011; Clelland et al., 2009; Nakashiba et al., 2012). Although both the DG (particularly neurogenesis in the DG) (Driscoll et al., 2006; Small et al., 2004; Patrylo and Williamson, 2007; Encinas et al., 2011; Ben Abdallah et al., 2010; Drapeau et al., 2003) and perforant path (Yassa et al., 2011; Barnes and McNaughton, 1980; Geinisman et al., 1992; Smith et al., 2000) are crucially affected by the aging process, we did not see this translate into a differential similarity-dependent deficit of aging since at the time point chosen the spatial memory function itself showed alterations that prevented to reveal this deficit. The presence of defective plasticity mechanisms in the middle-aged rat HP could explain the effect seen over spatial memory. In fact, rigidity in spatial representations could lead to an inability to differentiate between spatial locations (Barnes, 1994), and this inability could be more accentuated toward later time points.
Normal cognitive decline has been related to reduced synapse formation, expression of neuroprotectors like neurotrophins, altered neurogenesis and plasticity (Burke et al., 2010; Gallagher and Nicolle, 1993; Encinas et al., 2011; Ben Abdallah et al., 2010; Lu et al., 2005; Mattson and Arumugam, 2018). Although several interventions were studied to prevent this decline, EE has shown a particular interest for its translational value as a model of cognitive stimulation in humans. Even though EE has reported benefits over spatial memory performance in aged rodents (Harati et al., 2011; Bennett et al., 2006), how these benefits are dependent on the similarity load of the task is not clear. Moreover, prior work highlights the possibility that earlier interventions (ie. middle age) could be necessary in order to achieve a reproducible effect. Recent work has shown that the effect of EE over memory discrimination abilities in young adult rodents is associated with an EE-dependent increase in adult hippocampal neurogenesis (Frick and Fernandez, 2003; Pham et al., 1999; Paylor et al., 1992; Kempermann et al., 1997; van Praag et al., 1999; Auvergne et al., 2002; Cao et al., 2004; Bruel-Jungerman et al., 2005; Trinchero et al., 2017). The work also shows that these changes favor the sparsity of the network and a higher level of remapping in the presence of small environmental changes (Ventura et al., 2024). To add up to these findings, previous research indicated that the ability to encode small environmental changes is also influenced by the presence of the brain derived neurotrophic factor (BDNF) (Bekinschtein et al., 2014), a plasticity related molecule that has proven to be increased in the HP of EE housed animals and associated with newborn neuronal survival (Choi et al., 2009; Meng et al., 2015). We could speculate that EE, through an increase in neurogenesis and/or BDNF, could also reduce the rigidity of the spatial representations in middle-aged rodents. In line with this idea, EE can prevent age-related decline in precursor cell activity in the DG (Kempermann et al., 2002), and accelerate the neuronal development and integration of adult born granule cells in the DG in a BDNF-dependent manner (Trinchero et al., 2017). In this regard, previous work has shown that EE reverses the age-associated decrease in the expression of hippocampal tPA, a protease that controls the conversion from the precursor proBDNF to its mature form (Nagappan et al., 2009; Pang et al., 2004), that would ultimately change the balance between apoptotic and neurotrophic effects (ie. promoting neurogenesis) (Kempermann et al., 1997) and LTD/LTP (Lu et al., 2005; Nagappan et al., 2009; Pang et al., 2004; Figurov et al., 1996; Ghosh et al., 1994; Teng et al., 2005; Woo et al., 2005). Moreover, aged rats with preserved spatial reference memory showed higher number of new neurons in comparison to rats with spatial memory impairments (Driscoll et al., 2006; Drapeau et al., 2003). Considering the extensiveness of the molecular mechanisms of action of EE, from synaptic plasticity and cellular atrophy (Duffy et al., 2001; Eckert and Abraham, 2013; Park and Bischof, 2013) to dendritic growth (Leggio et al., 2005) and neurotransmitter systems (Segovia et al., 2006; Nichols et al., 2007; Segovia et al., 2008; Segovia et al., 2008), it is not surprising that its cognitive effects are widespread across domains. However, the fact that we do not see an impact over the dissimilar condition speaks of a mechanism that specifically impact the ability of the brain to avoid interference.
On the other side, in the object recognition domain, previous work has shown a specific deficit in a high similarity object condition in advanced aged (24–25 months) (Johnson et al., 2017; Burke et al., 2011). Here we extended these data by showing that this deficit could be present at earlier stages of aging (ie, middle-aged). Although the literature suggests there could be a decline in the ability to discriminate novel stimuli during aging (Burke et al., 2010; Bartolini et al., 1996; de Lima et al., 2005; Bastin and Van der Linden, 2003; Davidson and Glisky, 2002; Prull et al., 2006; Toth and Parks, 2006), likely due to a “false recognition” (Burke et al., 2010; Toner et al., 2009; Norman and Schacter, 1997; Jacoby et al., 2005), part of the memory field considers that recognition memory judgments are preserved and only recollection is affected by aging (Spencer and Raz, 1995; Driscoll et al., 2006; Bastin and Van der Linden, 2003; Davidson and Glisky, 2002; Daselaar et al., 2006; Pitarque et al., 2016; Koen and Yonelinas, 2016; Koen and Yonelinas, 2014). According to the “false recognition” theory, aging object recognition impairment is related to a difficulty in differentiating a novel object when it shares features with familiar ones leading to interpret it as a familiar object (ie. reduced exploration of the novel object). This false recognition has been associated with a higher susceptibility to interference occurring during the delay period (Burke et al., 2010; Trelle et al., 2017; Burke et al., 2011). In accordance with this theory, we described a pattern of memory practice effects that paradoxically increase object recognition memory only in middle-aged animals. This result evidences that the lack of discrimination of similar objects in middle-aged animals is not due to a retrieval deficit, as memory the next day is even higher for the middle-aged group compared to controls. More likely, this deficit is due to an incorrect identification of the AC object as familiar, what leads to a “practice-driven” increase in recognition of AB a day later.
This work has chosen a longitudinal approach for evaluating the role of EE. This choice could lead to a potential confounding effect of practice over the results presented. However, we do not believe this effect could explain our results since we have shown that the repeated presentation (or “practice”) of objects of similar identities over extended periods of time does not lead to an increase in the performance in aged animals. This result reinforces the view that the effect of EE as a multidimensional cognitive enhancement strategy entails more than just the repeated exposure to novel sensory experience. Additionally, although there is evidence of visual attentional deficits in aged rodents (Harati et al., 2011; Jones et al., 1995; Muir et al., 1999; McGaughy and Sarter, 1995; Sarter and Turchi, 2002), our results during sample session suggest that the differences observed in the test cannot be attributed to perceptual or attentional deficits in the middle-aged animals but rather to an early object memory discrimination deficit in this group. This is consistent with work showing that implicit visual-perceptual memory appears to be unaffected by aging (Gabrieli, 1996).
Although both SOR and SLR task are known to rely on the medial temporal lobe, the SLR is proposed to be more heavily dependent on the hippocampus and the SOR on the perirhinal cortex (Miranda et al., 2017; Miranda et al., 2021; Aggleton et al., 2004). The lack of correlation between the improved SLR and SOR scores posterior to the intervention further suggest that these two tasks could rely on different neural substrates in middle-aged animals. The lack of neurogenesis in cortical regions key for object recognition memory points to a different EE dependent mechanism for s-SOR discrimination recovery than the one postulated for the HP-dependent spatial memory task. One possible mechanism for the object recognition memory recovery seen after EE exposure is the increase in cortical thickness, an effect of EE vastly reported in the literature (Diamond et al., 1972; Fares et al., 2013), and more recently shown in particular for the perirhinal/entorhinal cortex (Scholz et al., 2015). Additionally, previous reports indicate that cortical BDNF protein levels are correlated with exercise-induced improvements in non-spatial memory (Hopkins and Bucci, 2010). EE-induced long-term increase in task-induced BDNF levels could lead to the improvements shown in the similar version of the task.
We found changes in object exploration levels in the EE condition either on the sample or choice session of the tasks. Previous publications have described decreased spontaneous activity and reductions in anxiety-related behaviors due to EE (Chapillon et al., 1999; Fox et al., 2006; Wolfer et al., 2004) and attributed this to probable reductions in novelty seeking as a result of the abundance of sensory stimulations present in the EE (Brenes et al., 2009; Schrijver et al., 2002). However, the longitudinal nature of our EE experiment does not allow us to link this decrease to the continuous presence of objects inside the environmental enrichment context, since we cannot exclude the possibility of the decrease in exploration time being due to the prior exposure to objects as part of the protocol.
The importance of comparing the two streams of information capable of high cognitive stimulus discrimination (spatial and object domain) becomes critical given their translational value. Interestingly, recent studies in humans comparing both discrimination domains indicate that discrimination of similar object features is more sensitive to aging compared to similar spatial locations (Reagh et al., 2016) and that only the performance of memory for objects and not scenes, is predictive of early cognitive decline (Fidalgo et al., 2016). Studies found that aging particularly compromises the binding of different elements of an episode (Chalfonte and Johnson, 1996; Naveh-Benjamin, 2000; Old and Naveh-Benjamin, 2008). The present study provides key evidence to better understand the progression of BPS memory deficits in both spatial and object domain, as a part of early stages of the normal cognitive decline process. We evidence here that environmental factors contribute to shape BPS function in the object and spatial domain. Understanding how experience dependent plasticity (addressed here through the EE intervention) can contribute to a differential modulation of each of these cognitive domains could foster new theoretical developments and help in the design of non-invasive therapeutic strategies.
The raw data supporting the conclusions of this article will be made available by the authors, without undue reservation.
The animal study was approved by the National Animal Care and Use Committee (CICUAL). The study was conducted in accordance with the local legislation and institutional requirements.
MM: Conceptualization, Formal analysis, Investigation, Methodology, Supervision, Visualization, Writing – original draft, Writing – review & editing. MN: Investigation, Writing – original draft, Writing – review & editing. MZ: Formal analysis, Investigation, Writing – original draft, Writing – review & editing. DP: Investigation, Writing – original draft, Writing – review & editing. NW: Writing – original draft, Writing – review & editing. PB: Conceptualization, Supervision, Writing – original draft, Writing – review & editing.
The author(s) declare that financial support was received for the research, authorship, and/or publication of this article. This work was supported by the CONICET (PUE 0052) to PB, FONCYT (PICT 2018–1062) to PB.
We thank David Jaime for his help with animal care.
The authors declare that the research was conducted in the absence of any commercial or financial relationships that could be construed as a potential conflict of interest.
All claims expressed in this article are solely those of the authors and do not necessarily represent those of their affiliated organizations, or those of the publisher, the editors and the reviewers. Any product that may be evaluated in this article, or claim that may be made by its manufacturer, is not guaranteed or endorsed by the publisher.
The Supplementary material for this article can be found online at: https://www.frontiersin.org/articles/10.3389/fnbeh.2024.1478656/full#supplementary-material
Aggleton, J. P., Kyd, R. J., and Bilkey, D. K. (2004). When is the perirhinal cortex necessary for the performance of spatial memory tasks? Neurosci. Biobehav. Rev. 28, 611–624. doi: 10.1016/j.neubiorev.2004.08.007
Alexander, G. E., Ryan, L., Bowers, D., Foster, T. C., Bizon, J. L., Geldmacher, D. S., et al. (2012). Characterizing cognitive aging in humans with links to animal models. Front. Aging Neurosci. 4:21. doi: 10.3389/fnagi.2012.00021
Auvergne, R., Lere, C., El Bahh, B., Arthaud, S., Lespinet, V., Rougier, A., et al. (2002). Delayed kindling epileptogenesis and increased neurogenesis in adult rats housed in an enriched environment. Brain Res. 954, 277–285. doi: 10.1016/S0006-8993(02)03355-3
Bach, M. E., Barad, M., Son, H., Zhuo, M., Lu, Y. F., Shih, R., et al. (1999). Age-related defects in spatial memory are correlated with defects in the late phase of hippocampal long-term potentiation in vitro and are attenuated by drugs that enhance the cAMP signaling pathway. Proc. Natl. Acad. Sci. USA 96, 5280–5285. doi: 10.1073/pnas.96.9.5280
Ballesteros, S., Mayas, J., Prieto, A., Toril, P., Pita, C., Laura Pde, L., et al. (2015). A randomized controlled trial of brain training with non-action video games in older adults: results of the 3-month follow-up. Front. Aging Neurosci. 7:45. doi: 10.3389/fnagi.2015.00045
Barnes, C. A. (1979). Memory deficits associated with senescence: a neurophysiological and behavioral study in the rat. J. Comp. Physiol. Psychol. 93, 74–104. doi: 10.1037/h0077579
Barnes, C. A. (1988). Aging and the physiology of spatial memory. Neurobiol. Aging 9, 563–568. doi: 10.1016/S0197-4580(88)80114-3
Barnes, C. A. (1994). Normal aging: regionally specific changes in hippocampal synaptic transmission. Trends Neurosci. 17, 13–18. doi: 10.1016/0166-2236(94)90029-9
Barnes, C. A., and McNaughton, B. L. (1980). Physiological compensation for loss of afferent synapses in rat hippocampal granule cells during senescence. J. Physiol. 309, 473–485. doi: 10.1113/jphysiol.1980.sp013521
Barnes, C. A., Nadel, L., and Honig, W. K. (1980). Spatial memory deficit in senescent rats. Can. J. Psychol. 34, 29–39. doi: 10.1037/h0081022
Bartko, S. J., Winters, B. D., Cowell, R. A., Saksida, L. M., and Bussey, T. J. (2007a). Perceptual functions of perirhinal cortex in rats: zero-delay object recognition and simultaneous oddity discriminations. J. Neurosci. 27, 2548–2559. doi: 10.1523/JNEUROSCI.5171-06.2007
Bartko, S. J., Winters, B. D., Cowell, R. A., Saksida, L. M., and Bussey, T. J. (2007b). Perirhinal cortex resolves feature ambiguity in configural object recognition and perceptual oddity tasks. Learn. Mem. 14, 821–832. doi: 10.1101/lm.749207
Bartolini, L., Casamenti, F., and Pepeu, G. (1996). Aniracetam restores object recognition impaired by age, scopolamine, and nucleus basalis lesions. Pharmacol. Biochem. Behav. 53, 277–283. doi: 10.1016/0091-3057(95)02021-7
Bastin, C., and Van der Linden, M. (2003). The contribution of recollection and familiarity to recognition memory: a study of the effects of test format and aging. Neuropsychology 17, 14–24. doi: 10.1037/0894-4105.17.1.14
Begega, A., Cuesta, M., Rubio, S., Mendez, M., Santin, L. J., and Arias, J. L. (2012). Functional networks involved in spatial learning strategies in middle-aged rats. Neurobiol. Learn. Mem. 97, 346–353. doi: 10.1016/j.nlm.2012.02.007
Bekinschtein, P., Kent, B. A., Oomen, C. A., Clemenson, G. D., Gage, F. H., Saksida, L. M., et al. (2013). BDNF in the dentate gyrus is required for consolidation of "pattern-separated" memories. Cell Rep. 5, 759–768. doi: 10.1016/j.celrep.2013.09.027
Bekinschtein, P., Kent, B. A., Oomen, C. A., Clemenson, G. D., Gage, F. H., Saksida, L. M., et al. (2014). Brain-derived neurotrophic factor interacts with adult-born immature cells in the dentate gyrus during consolidation of overlapping memories. Hippocampus 24, 905–911. doi: 10.1002/hipo.22304
Ben Abdallah, N. M., Slomianka, L., Vyssotski, A. L., and Lipp, H. P. (2010). Early age-related changes in adult hippocampal neurogenesis in C57 mice. Neurobiol. Aging 31, 151–161. doi: 10.1016/j.neurobiolaging.2008.03.002
Bennett, J. C., McRae, P. A., Levy, L. J., and Frick, K. M. (2006). Long-term continuous, but not daily, environmental enrichment reduces spatial memory decline in aged male mice. Neurobiol. Learn. Mem. 85, 139–152. doi: 10.1016/j.nlm.2005.09.003
Bizon, J. L., LaSarge, C. L., Montgomery, K. S., McDermott, A. N., Setlow, B., and Griffith, W. H. (2009). Spatial reference and working memory across the lifespan of male Fischer 344 rats. Neurobiol. Aging 30, 646–655. doi: 10.1016/j.neurobiolaging.2007.08.004
Bohbot, V. D., McKenzie, S., Konishi, K., Fouquet, C., Kurdi, V., Schachar, R., et al. (2012). Virtual navigation strategies from childhood to senescence: evidence for changes across the life span. Front. Aging Neurosci. 4:28. doi: 10.3389/fnagi.2012.00028
Bouet, V., Freret, T., Dutar, P., Billard, J. M., and Boulouard, M. (2011). Continuous enriched environment improves learning and memory in adult NMRI mice through theta burst-related-LTP independent mechanisms but is not efficient in advanced aged animals. Mech. Ageing Dev. 132, 240–248. doi: 10.1016/j.mad.2011.04.006
Brenes, J. C., Padilla, M., and Fornaguera, J. (2009). A detailed analysis of open-field habituation and behavioral and neurochemical antidepressant-like effects in postweaning enriched rats. Behav. Brain Res. 197, 125–137. doi: 10.1016/j.bbr.2008.08.014
Bruel-Jungerman, E., Laroche, S., and Rampon, C. (2005). New neurons in the dentate gyrus are involved in the expression of enhanced long-term memory following environmental enrichment. Eur. J. Neurosci. 21, 513–521. doi: 10.1111/j.1460-9568.2005.03875.x
Burke, S. N., Ryan, L., and Barnes, C. A. (2012). Characterizing cognitive aging of recognition memory and related processes in animal models and in humans. Front. Aging Neurosci. 4:15. doi: 10.3389/fnagi.2012.00015
Burke, S. N., Wallace, J. L., Hartzell, A. L., Nematollahi, S., Plange, K., and Barnes, C. A. (2011). Age-associated deficits in pattern separation functions of the perirhinal cortex: a cross-species consensus. Behav. Neurosci. 125, 836–847. doi: 10.1037/a0026238
Burke, S. N., Wallace, J. L., Nematollahi, S., Uprety, A. R., and Barnes, C. A. (2010). Pattern separation deficits may contribute to age-associated recognition impairments. Behav. Neurosci. 124, 559–573. doi: 10.1037/a0020893
Calhoun, M. E., Kurth, D., Phinney, A. L., Long, J. M., Hengemihle, J., Mouton, P. R., et al. (1998). Hippocampal neuron and synaptophysin-positive Bouton number in aging C57BL/6 mice. Neurobiol. Aging 19, 599–606. doi: 10.1016/S0197-4580(98)00098-0
Cansino, S. (2009). Episodic memory decay along the adult lifespan: a review of behavioral and neurophysiological evidence. Int. J. Psychophysiol. 71, 64–69. doi: 10.1016/j.ijpsycho.2008.07.005
Cao, L., Jiao, X., Zuzga, D. S., Liu, Y., Fong, D. M., Young, D., et al. (2004). VEGF links hippocampal activity with neurogenesis, learning and memory. Nat. Genet. 36, 827–835. doi: 10.1038/ng1395
Caprioli, A., Ghirardi, O., Giuliani, A., Ramacci, M. T., and Angelucci, L. (1991). Spatial learning and memory in the radial maze: a longitudinal study in rats from 4 to 25 months of age. Neurobiol. Aging 12, 605–607. doi: 10.1016/0197-4580(91)90093-Y
Ces, A., Burg, T., Herbeaux, K., Heraud, C., Bott, J. B., Mensah-Nyagan, A. G., et al. (2018). Age-related vulnerability of pattern separation in C57BL/6J mice. Neurobiol. Aging 62, 120–129. doi: 10.1016/j.neurobiolaging.2017.10.013
Chalfonte, B. L., and Johnson, M. K. (1996). Feature memory and binding in young and older adults. Mem. Cogn. 24, 403–416. doi: 10.3758/BF03200930
Chapillon, P., Manneche, C., Belzung, C., and Caston, J. (1999). Rearing environmental enrichment in two inbred strains of mice: 1. Effects on emotional reactivity. Behav Genet 29, 41–46
Choi, S. H., Li, Y., Parada, L. F., and Sisodia, S. S. (2009). Regulation of hippocampal progenitor cell survival, proliferation and dendritic development by BDNF. Mol. Neurodegener. 4:52. doi: 10.1186/1750-1326-4-52
Cintoli, S., Radicchi, C., Noale, M., Maggi, S., Meucci, G., Tognoni, G., et al. (2021). Effects of combined training on neuropsychiatric symptoms and quality of life in patients with cognitive decline. Aging Clin. Exp. Res. 33, 1249–1257. doi: 10.1007/s40520-019-01280-w
Clelland, C. D., Choi, M., Romberg, C., Clemenson, G. D. Jr., Fragniere, A., Tyers, P., et al. (2009). A functional role for adult hippocampal neurogenesis in spatial pattern separation. Science 325, 210–213. doi: 10.1126/science.1173215
Cortese, G. P., Olin, A., O'Riordan, K., Hullinger, R., and Burger, C. (2018). Environmental enrichment improves hippocampal function in aged rats by enhancing learning and memory, LTP, and mGluR5-Homer1c activity. Neurobiol. Aging 63, 1–11. doi: 10.1016/j.neurobiolaging.2017.11.004
Craik, F. I. (1990). Changes in memory with normal aging: a functional view. Adv. Neurol. 51, 201–205
Creer, D. J., Romberg, C., Saksida, L. M., van Praag, H., and Bussey, T. J. (2010). Running enhances spatial pattern separation in mice. Proc. Natl. Acad. Sci. USA 107, 2367–2372. doi: 10.1073/pnas.0911725107
Das, S. R., and Magnusson, K. R. (2008). Relationship between mRNA expression of splice forms of the zeta1 subunit of the N-methyl-D-aspartate receptor and spatial memory in aged mice. Brain Res. 1207, 142–154. doi: 10.1016/j.brainres.2008.02.046
Daselaar, S. M., Fleck, M. S., and Cabeza, R. (2006). Triple dissociation in the medial temporal lobes: recollection, familiarity, and novelty. J. Neurophysiol. 96, 1902–1911. doi: 10.1152/jn.01029.2005
Davidson, P. S., and Glisky, E. L. (2002). Neuropsychological correlates of recollection and familiarity in normal aging. Cogn. Affect. Behav. Neurosci. 2, 174–186. doi: 10.3758/CABN.2.2.174
de Lima, M. N., Laranja, D. C., Caldana, F., Bromberg, E., Roesler, R., and Schroder, N. (2005). Reversal of age-related deficits in object recognition memory in rats with l-deprenyl. Exp. Gerontol. 40, 506–511. doi: 10.1016/j.exger.2005.03.004
Dellu, F., Mayo, W., Vallee, M., Le Moal, M., and Simon, H. (1997). Facilitation of cognitive performance in aged rats by past experience depends on the type of information processing involved: a combined cross-sectional and longitudinal study. Neurobiol. Learn. Mem. 67, 121–128. doi: 10.1006/nlme.1996.3750
Diamond, M. C., Rosenzweig, M. R., Bennett, E. L., Lindner, B., and Lyon, L. (1972). Effects of environmental enrichment and impoverishment on rat cerebral cortex. J. Neurobiol. 3, 47–64. doi: 10.1002/neu.480030105
Dohm-Hansen, S., English, J. A., Lavelle, A., Fitzsimons, C. P., Lucassen, P. J., and Nolan, Y. M. (2024). The 'middle-aging' brain. Trends Neurosci. 47, 259–272. doi: 10.1016/j.tins.2024.02.001
Dorfman, D., Fernandez, D. C., Chianelli, M., Miranda, M., Aranda, M. L., and Rosenstein, R. E. (2013). Post-ischemic environmental enrichment protects the retina from ischemic damage in adult rats. Exp. Neurol. 240, 146–156. doi: 10.1016/j.expneurol.2012.11.017
Drapeau, E., Mayo, W., Aurousseau, C., Le Moal, M., Piazza, P. V., and Abrous, D. N. (2003). Spatial memory performances of aged rats in the water maze predict levels of hippocampal neurogenesis. Proc. Natl. Acad. Sci. USA 100, 14385–14390. doi: 10.1073/pnas.2334169100
Driscoll, I., Howard, S. R., Stone, J. C., Monfils, M. H., Tomanek, B., Brooks, W. M., et al. (2006). The aging hippocampus: a multi-level analysis in the rat. Neuroscience 139, 1173–1185. doi: 10.1016/j.neuroscience.2006.01.040
Duffy, S. N., Craddock, K. J., Abel, T., and Nguyen, P. V. (2001). Environmental enrichment modifies the PKA-dependence of hippocampal LTP and improves hippocampus-dependent memory. Learn. Mem. 8, 26–34. doi: 10.1101/lm.36301
Dunnett, S. B., Evenden, J. L., and Iversen, S. D. (1988). Delay-dependent short-term memory deficits in aged rats. Psychopharmacology 96, 174–180
Eckert, M. J., and Abraham, W. C. (2013). Effects of environmental enrichment exposure on synaptic transmission and plasticity in the hippocampus. Curr. Top. Behav. Neurosci. 15, 165–187. doi: 10.1007/7854_2012_215
Encinas, J. M., Michurina, T. V., Peunova, N., Park, J. H., Tordo, J., Peterson, D. A., et al. (2011). Division-coupled astrocytic differentiation and age-related depletion of neural stem cells in the adult hippocampus. Cell Stem Cell 8, 566–579. doi: 10.1016/j.stem.2011.03.010
Fares, R. P., Belmeguenai, A., Sanchez, P. E., Kouchi, H. Y., Bodennec, J., Morales, A., et al. (2013). Standardized environmental enrichment supports enhanced brain plasticity in healthy rats and prevents cognitive impairment in epileptic rats. PLoS One 8:e53888. doi: 10.1371/journal.pone.0053888
Fidalgo, C. O., Changoor, A. T., Page-Gould, E., Lee, A. C., and Barense, M. D. (2016). Early cognitive decline in older adults better predicts object than scene recognition performance. Hippocampus 26, 1579–1592. doi: 10.1002/hipo.22658
Figurov, A., Pozzo-Miller, L. D., Olafsson, P., Wang, T., and Lu, B. (1996). Regulation of synaptic responses to high-frequency stimulation and LTP by neurotrophins in the hippocampus. Nature 381, 706–709. doi: 10.1038/381706a0
Fischer, W., Chen, K. S., Gage, F. H., and Bjorklund, A. (1992). Progressive decline in spatial learning and integrity of forebrain cholinergic neurons in rats during aging. Neurobiol. Aging 13, 9–23. doi: 10.1016/0197-4580(92)90003-G
Foster, T. C., Sharrow, K. M., Kumar, A., and Masse, J. (2003). Interaction of age and chronic estradiol replacement on memory and markers of brain aging. Neurobiol. Aging 24, 839–852. doi: 10.1016/S0197-4580(03)00014-9
Fox, C., Merali, Z., and Harrison, C. (2006). Therapeutic and protective effect of environmental enrichment against psychogenic and neurogenic stress. Behav. Brain Res. 175, 1–8. doi: 10.1016/j.bbr.2006.08.016
Frick, K. M., Baxter, M. G., Markowska, A. L., Olton, D. S., and Price, D. L. (1995). Age-related spatial reference and working memory deficits assessed in the water maze. Neurobiol. Aging 16, 149–160. doi: 10.1016/0197-4580(94)00155-3
Frick, K. M., and Benoit, J. D. (2010). Use it or lose it: environmental enrichment as a means to promote successful cognitive aging. ScientificWorldJournal 10, 1129–1141. doi: 10.1100/tsw.2010.111
Frick, K. M., and Fernandez, S. M. (2003). Enrichment enhances spatial memory and increases synaptophysin levels in aged female mice. Neurobiol. Aging 24, 615–626. doi: 10.1016/S0197-4580(02)00138-0
Gabrieli, J. D. (1996). Memory systems analyses of mnemonic disorders in aging and age-related diseases. Proc. Natl. Acad. Sci. USA 93, 13534–13540. doi: 10.1073/pnas.93.24.13534
Gage, F. H., Bjorklund, A., Stenevi, U., Dunnett, S. B., and Kelly, P. A. (1984). Intrahippocampal septal grafts ameliorate learning impairments in aged rats. Science 225, 533–536. doi: 10.1126/science.6539949
Gage, F. H., Chen, K. S., Buzsaki, G., and Armstrong, D. (1988). Experimental approaches to age-related cognitive impairments. Neurobiol. Aging 9, 645–655. doi: 10.1016/S0197-4580(88)80129-5
Gallagher, M., and Burwell, R. D. (1989). Relationship of age-related decline across several behavioral domains. Neurobiol. Aging 10, 691–708. doi: 10.1016/0197-4580(89)90006-7
Gallagher, M., and Colombo, P. J. (1995). Ageing: the cholinergic hypothesis of cognitive decline. Curr. Opin. Neurobiol. 5, 161–168. doi: 10.1016/0959-4388(95)80022-0
Gallagher, M., and Nicolle, M. M. (1993). Animal models of normal aging: relationship between cognitive decline and markers in hippocampal circuitry. Behav. Brain Res. 57, 155–162. doi: 10.1016/0166-4328(93)90131-9
Gallagher, M., and Pelleymounter, M. A. (1988). Spatial learning deficits in old rats: a model for memory decline in the aged. Neurobiol. Aging 9, 549–556. doi: 10.1016/S0197-4580(88)80112-X
Geinisman, Y., deToledo-Morrell, L., Morrell, F., Persina, I. S., and Rossi, M. (1992). Age-related loss of axospinous synapses formed by two afferent systems in the rat dentate gyrus as revealed by the unbiased stereological dissector technique. Hippocampus 2, 437–444. doi: 10.1002/hipo.450020411
Ghosh, A., Carnahan, J., and Greenberg, M. E. (1994). Requirement for BDNF in activity-dependent survival of cortical neurons. Science 263, 1618–1623. doi: 10.1126/science.7907431
Gilbert, P. E., Kesner, R. P., and Lee, I. (2001). Dissociating hippocampal subregions: double dissociation between dentate gyrus and CA1. Hippocampus 11, 626–636. doi: 10.1002/hipo.1077
Gracian, E. I., Shelley, L. E., Morris, A. M., and Gilbert, P. E. (2013). Age-related changes in place learning for adjacent and separate locations. Neurobiol. Aging 34, 2304–2309. doi: 10.1016/j.neurobiolaging.2013.03.033
Gresack, J. E., Kerr, K. M., and Frick, K. M. (2007). Life-long environmental enrichment differentially affects the mnemonic response to estrogen in young, middle-aged, and aged female mice. Neurobiol. Learn. Mem. 88, 393–408. doi: 10.1016/j.nlm.2007.07.015
Gros, A., and Wang, S. H. (2018). Behavioral tagging and capture: long-term memory decline in middle-aged rats. Neurobiol. Aging 67, 31–41. doi: 10.1016/j.neurobiolaging.2018.02.023
Harati, H., Majchrzak, M., Cosquer, B., Galani, R., Kelche, C., Cassel, J. C., et al. (2011). Attention and memory in aged rats: impact of lifelong environmental enrichment. Neurobiol. Aging 32, 718–736. doi: 10.1016/j.neurobiolaging.2009.03.012
Harburger, L. L., Lambert, T. J., and Frick, K. M. (2007). Age-dependent effects of environmental enrichment on spatial reference memory in male mice. Behav. Brain Res. 185, 43–48. doi: 10.1016/j.bbr.2007.07.009
Harburger, L. L., Nzerem, C. K., and Frick, K. M. (2007). Single enrichment variables differentially reduce age-related memory decline in female mice. Behav. Neurosci. 121, 679–688. doi: 10.1037/0735-7044.121.4.679
Holden, H. M., and Gilbert, P. E. (2012). Less efficient pattern separation may contribute to age-related spatial memory deficits. Front. Aging Neurosci. 4:9. doi: 10.3389/fnagi.2012.00009
Holmes, M. C., Carter, R. N., Noble, J., Chitnis, S., Dutia, A., Paterson, J. M., et al. (2010). 11beta-hydroxysteroid dehydrogenase type 1 expression is increased in the aged mouse hippocampus and parietal cortex and causes memory impairments. J. Neurosci. 30, 6916–6920. doi: 10.1523/JNEUROSCI.0731-10.2010
Hopkins, M. E., and Bucci, D. J. (2010). BDNF expression in perirhinal cortex is associated with exercise-induced improvement in object recognition memory. Neurobiol. Learn. Mem. 94, 278–284. doi: 10.1016/j.nlm.2010.06.006
Isingrini, M., and Taconnat, L. (2008). Episodic memory, frontal functioning, and aging. Rev. Neurol. (Paris) 164, S91–S95. doi: 10.1016/S0035-3787(08)73297-1
Jacoby, L. L., Bishara, A. J., Hessels, S., and Toth, J. P. (2005). Aging, subjective experience, and cognitive control: dramatic false remembering by older adults. J. Exp. Psychol. Gen. 134, 131–148. doi: 10.1037/0096-3445.134.2.131
Johnson, S. A., Turner, S. M., Santacroce, L. A., Carty, K. N., Shafiq, L., Bizon, J. L., et al. (2017). Rodent age-related impairments in discriminating perceptually similar objects parallel those observed in humans. Hippocampus 27, 759–776. doi: 10.1002/hipo.22729
Jones, D. N., Barnes, J. C., Kirkby, D. L., and Higgins, G. A. (1995). Age-associated impairments in a test of attention: evidence for involvement of cholinergic systems. J. Neurosci. 15, 7282–7292. doi: 10.1523/JNEUROSCI.15-11-07282.1995
Kempermann, G., Brandon, E. P., and Gage, F. H. (1998). Environmental stimulation of 129/SvJ mice causes increased cell proliferation and neurogenesis in the adult dentate gyrus. Curr. Biol. 8, 939–944. doi: 10.1016/S0960-9822(07)00377-6
Kempermann, G., Gast, D., and Gage, F. H. (2002). Neuroplasticity in old age: sustained fivefold induction of hippocampal neurogenesis by long-term environmental enrichment. Ann. Neurol. 52, 135–143. doi: 10.1002/ana.10262
Kempermann, G., Kuhn, H. G., and Gage, F. H. (1997). More hippocampal neurons in adult mice living in an enriched environment. Nature 386, 493–495. doi: 10.1038/386493a0
Kobayashi, S., Ohashi, Y., and Ando, S. (2002). Effects of enriched environments with different durations and starting times on learning capacity during aging in rats assessed by a refined procedure of the Hebb-Williams maze task. J. Neurosci. Res. 70, 340–346. doi: 10.1002/jnr.10442
Koen, J. D., and Yonelinas, A. P. (2014). The effects of healthy aging, amnestic mild cognitive impairment, and Alzheimer's disease on recollection and familiarity: a meta-analytic review. Neuropsychol. Rev. 24, 332–354. doi: 10.1007/s11065-014-9266-5
Koen, J. D., and Yonelinas, A. P. (2016). Recollection, not familiarity, decreases in healthy ageing: converging evidence from four estimation methods. Memory 24, 75–88. doi: 10.1080/09658211.2014.985590
Kumar, A., Rani, A., Tchigranova, O., Lee, W. H., and Foster, T. C. (2012). Influence of late-life exposure to environmental enrichment or exercise on hippocampal function and CA1 senescent physiology. Neurobiol. Aging 33, e1–e17. doi: 10.1016/j.neurobiolaging.2011.06.023
Lacreuse, A., Russell, J. L., Hopkins, W. D., and Herndon, J. G. (2014). Cognitive and motor aging in female chimpanzees. Neurobiol. Aging 35, 623–632. doi: 10.1016/j.neurobiolaging.2013.08.036
Leal, S. L., and Yassa, M. A. (2015). Neurocognitive aging and the Hippocampus across species. Trends Neurosci. 38, 800–812. doi: 10.1016/j.tins.2015.10.003
Leal-Galicia, P., Castaneda-Bueno, M., Quiroz-Baez, R., and Arias, C. (2008). Long-term exposure to environmental enrichment since youth prevents recognition memory decline and increases synaptic plasticity markers in aging. Neurobiol. Learn. Mem. 90, 511–518. doi: 10.1016/j.nlm.2008.07.005
Leggio, M. G., Mandolesi, L., Federico, F., Spirito, F., Ricci, B., Gelfo, F., et al. (2005). Environmental enrichment promotes improved spatial abilities and enhanced dendritic growth in the rat. Behav. Brain Res. 163, 78–90. doi: 10.1016/j.bbr.2005.04.009
Lester, A. W., Moffat, S. D., Wiener, J. M., Barnes, C. A., and Wolbers, T. (2017). The aging navigational system. Neuron 95, 1019–1035. doi: 10.1016/j.neuron.2017.06.037
Lores-Arnaiz, S., Bustamante, J., Arismendi, M., Vilas, S., Paglia, N., Basso, N., et al. (2006). Extensive enriched environments protect old rats from the aging dependent impairment of spatial cognition, synaptic plasticity and nitric oxide production. Behav. Brain Res. 169, 294–302. doi: 10.1016/j.bbr.2006.01.016
Lu, B., Pang, P. T., and Woo, N. H. (2005). The yin and yang of neurotrophin action. Nat. Rev. Neurosci. 6, 603–614. doi: 10.1038/nrn1726
Magnusson, K. R. (1998). Aging of glutamate receptors: correlations between binding and spatial memory performance in mice. Mech. Ageing Dev. 104, 227–248. doi: 10.1016/S0047-6374(98)00076-1
Magnusson, K. R. (2001). Influence of diet restriction on NMDA receptor subunits and learning during aging. Neurobiol. Aging 22, 613–627. doi: 10.1016/S0197-4580(00)00258-X
Maguire, E. A., Frackowiak, R. S., and Frith, C. D. (1996). Learning to find your way: a role for the human hippocampal formation. Proc. Biol. Sci. 263, 1745–1750.
Markowska, A. L., Stone, W. S., Ingram, D. K., Reynolds, J., Gold, P. E., Conti, L. H., et al. (1989). Individual differences in aging: behavioral and neurobiological correlates. Neurobiol. Aging 10, 31–43. doi: 10.1016/S0197-4580(89)80008-9
Marr, D. (1971). Simple memory: a theory for archicortex. Philos. Trans. R. Soc. Lond. Ser. B Biol. Sci. 262, 23–81
Marrone, D. F., Adams, A. A., and Satvat, E. (2011). Increased pattern separation in the aged fascia dentata. Neurobiol. Aging 32, e23–e32. doi: 10.1016/j.neurobiolaging.2010.03.021
Mattson, M. P., and Arumugam, T. V. (2018). Hallmarks of brain aging: adaptive and pathological modification by metabolic states. Cell Metab. 27, 1176–1199. doi: 10.1016/j.cmet.2018.05.011
McDonough, I. M., Cervantes, S. N., Gray, S. J., and Gallo, D. A. (2014). Memory's aging echo: age-related decline in neural reactivation of perceptual details during recollection. NeuroImage 98, 346–358. doi: 10.1016/j.neuroimage.2014.05.012
McGaughy, J., and Sarter, M. (1995). Behavioral vigilance in rats: task validation and effects of age, amphetamine, and benzodiazepine receptor ligands. Psychopharmacology 117, 340–357. doi: 10.1007/BF02246109
McTighe, S. M., Cowell, R. A., Winters, B. D., Bussey, T. J., and Saksida, L. M. (2010). Paradoxical false memory for objects after brain damage. Science 330, 1408–1410. doi: 10.1126/science.1194780
Means, L. W., and Kennard, K. J. (1991). Working memory and the aged rat: deficient two-choice win-stay water-escape acquisition and retention. Physiol. Behav. 49, 301–307. doi: 10.1016/0031-9384(91)90047-R
Meng, F. T., Zhao, J., Ni, R. J., Fang, H., Zhang, L. F., Zhang, Z., et al. (2015). Beneficial effects of enriched environment on behaviors were correlated with decreased estrogen and increased BDNF in the hippocampus of male mice. Neuro Endocrinol. Lett. 36, 490–497
Miranda, M., Kent, B. A., Morici, J. F., Gallo, F., Saksida, L. M., Bussey, T. J., et al. (2018). NMDA receptors and BDNF are necessary for discrimination of overlapping spatial and non-spatial memories in perirhinal cortex and hippocampus. Neurobiol. Learn. Mem. 155, 337–343. doi: 10.1016/j.nlm.2018.08.019
Miranda, M., Kent, B. A., Morici, J. F., Gallo, F., Weisstaub, N. V., Saksida, L. M., et al. (2017). Molecular mechanisms in Perirhinal cortex selectively necessary for discrimination of overlapping memories, but independent of memory persistence. eNeuro 4, ENEURO.0293–ENEU17.2017. doi: 10.1523/ENEURO.0293-17.2017
Miranda, M., Morici, J. F., Gallo, F., Piromalli Girado, D., Weisstaub, N. V., and Bekinschtein, P. (2021). Molecular mechanisms within the dentate gyrus and the perirhinal cortex interact during discrimination of similar nonspatial memories. Hippocampus 31, 140–155. doi: 10.1002/hipo.23269
Mirochnic, S., Wolf, S., Staufenbiel, M., and Kempermann, G. (2009). Age effects on the regulation of adult hippocampal neurogenesis by physical activity and environmental enrichment in the APP23 mouse model of Alzheimer disease. Hippocampus 19, 1008–1018. doi: 10.1002/hipo.20560
Moffat, S. D. (2009). Aging and spatial navigation: what do we know and where do we go? Neuropsychol. Rev. 19, 478–489. doi: 10.1007/s11065-009-9120-3
Moffat, S. D., Elkins, W., and Resnick, S. M. (2006). Age differences in the neural systems supporting human allocentric spatial navigation. Neurobiol. Aging 27, 965–972. doi: 10.1016/j.neurobiolaging.2005.05.011
Mora, F., Segovia, G., and del Arco, A. (2007). Aging, plasticity and environmental enrichment: structural changes and neurotransmitter dynamics in several areas of the brain. Brain Res. Rev. 55, 78–88. doi: 10.1016/j.brainresrev.2007.03.011
Muir, J. L., Fischer, W., and Bjorklund, A. (1999). Decline in visual attention and spatial memory in aged rats. Neurobiol. Aging 20, 605–615. doi: 10.1016/S0197-4580(99)00098-6
Nagappan, G., Zaitsev, E., Senatorov, V. V. Jr., Yang, J., Hempstead, B. L., and Lu, B. (2009). Control of extracellular cleavage of ProBDNF by high frequency neuronal activity. Proc. Natl. Acad. Sci. USA 106, 1267–1272. doi: 10.1073/pnas.0807322106
Nakashiba, T., Cushman, J. D., Pelkey, K. A., Renaudineau, S., Buhl, D. L., McHugh, T. J., et al. (2012). Young dentate granule cells mediate pattern separation, whereas old granule cells facilitate pattern completion. Cell 149, 188–201. doi: 10.1016/j.cell.2012.01.046
Naveh-Benjamin, M. (2000). Adult age differences in memory performance: tests of an associative deficit hypothesis. J. Exp. Psychol. Learn. Mem. Cogn. 26, 1170–1187. doi: 10.1037/0278-7393.26.5.1170
Ngandu, T., Lehtisalo, J., Solomon, A., Levalahti, E., Ahtiluoto, S., Antikainen, R., et al. (2015). A 2 year multidomain intervention of diet, exercise, cognitive training, and vascular risk monitoring versus control to prevent cognitive decline in at-risk elderly people (FINGER): a randomised controlled trial. Lancet 385, 2255–2263. doi: 10.1016/S0140-6736(15)60461-5
Nichols, J. A., Jakkamsetti, V. P., Salgado, H., Dinh, L., Kilgard, M. P., and Atzori, M. (2007). Environmental enrichment selectively increases glutamatergic responses in layer II/III of the auditory cortex of the rat. Neuroscience 145, 832–840. doi: 10.1016/j.neuroscience.2006.12.061
Nithianantharajah, J., and Hannan, A. J. (2006). Enriched environments, experience-dependent plasticity and disorders of the nervous system. Nat. Rev. Neurosci. 7, 697–709. doi: 10.1038/nrn1970
Norman, K. A., and Schacter, D. L. (1997). False recognition in younger and older adults: exploring the characteristics of illusory memories. Mem. Cogn. 25, 838–848. doi: 10.3758/BF03211328
O'Callaghan, R. M., Griffin, E. W., and Kelly, A. M. (2009). Long-term treadmill exposure protects against age-related neurodegenerative change in the rat hippocampus. Hippocampus 19, 1019–1029. doi: 10.1002/hipo.20591
Old, S. R., and Naveh-Benjamin, M. (2008). Differential effects of age on item and associative measures of memory: a meta-analysis. Psychol. Aging 23, 104–118. doi: 10.1037/0882-7974.23.1.104
Oler, J. A., and Markus, E. J. (1998). Age-related deficits on the radial maze and in fear conditioning: hippocampal processing and consolidation. Hippocampus 8, 402–415. doi: 10.1002/(SICI)1098-1063(1998)8:4<402::AID-HIPO8>3.0.CO;2-I
Pang, P. T., Teng, H. K., Zaitsev, E., Woo, N. T., Sakata, K., Zhen, S., et al. (2004). Cleavage of proBDNF by tPA/plasmin is essential for long-term hippocampal plasticity. Science 306, 487–491. doi: 10.1126/science.1100135
Park, D. C., and Bischof, G. N. (2013). The aging mind: neuroplasticity in response to cognitive training. Dialogues Clin. Neurosci. 15, 109–119. doi: 10.31887/DCNS.2013.15.1/dpark
Patrylo, P. R., and Williamson, A. (2007). The effects of aging on dentate circuitry and function. Prog. Brain Res. 163, 679–696. doi: 10.1016/S0079-6123(07)63037-4
Paylor, R., Morrison, S. K., Rudy, J. W., Waltrip, L. T., and Wehner, J. M. (1992). Brief exposure to an enriched environment improves performance on the Morris water task and increases hippocampal cytosolic protein kinase C activity in young rats. Behav. Brain Res. 52, 49–59. doi: 10.1016/S0166-4328(05)80324-9
Pham, T. M., Ickes, B., Albeck, D., Soderstrom, S., Granholm, A. C., and Mohammed, A. H. (1999). Changes in brain nerve growth factor levels and nerve growth factor receptors in rats exposed to environmental enrichment for one year. Neuroscience 94, 279–286. doi: 10.1016/S0306-4522(99)00316-4
Pidgeon, L. M., and Morcom, A. M. (2014). Age-related increases in false recognition: the role of perceptual and conceptual similarity. Front. Aging Neurosci. 6:283. doi: 10.3389/fnagi.2014.00283
Pieta Dias, C., Martins de Lima, M. N., Presti-Torres, J., Dornelles, A., Garcia, V. A., Siciliani Scalco, F., et al. (2007). Memantine reduces oxidative damage and enhances long-term recognition memory in aged rats. Neuroscience 146, 1719–1725. doi: 10.1016/j.neuroscience.2007.03.018
Pitarque, A., Melendez, J., Sales, A., Mayordomo, T., Escudero, J., and Algarabel, S. (2016). Differences in false recollection according to the cognitive reserve of healthy older people. Neuropsychol. Dev. Cogn. B Aging Neuropsychol. Cogn. 23, 625–637. doi: 10.1080/13825585.2016.1146221
Platano, D., Fattoretti, P., Balietti, M., Bertoni-Freddari, C., and Aicardi, G. (2008). Long-term visual object recognition memory in aged rats. Rejuvenation Res. 11, 333–339. doi: 10.1089/rej.2008.0687
Prull, M. W., Dawes, L. L., Martin, A. M. 3rd, Rosenberg, H. F., and Light, L. L. (2006). Recollection and familiarity in recognition memory: adult age differences and neuropsychological test correlates. Psychol. Aging 21, 107–118. doi: 10.1037/0882-7974.21.1.107
Rapp, P. R., and Amaral, D. G. (1992). Individual differences in the cognitive and neurobiological consequences of normal aging. Trends Neurosci. 15, 340–345. doi: 10.1016/0166-2236(92)90051-9
Rapp, P. R., and Heindel, W. C. (1994). Memory systems in normal and pathological aging. Curr. Opin. Neurol. 7, 294–298. doi: 10.1097/00019052-199408000-00003
Rapp, P. R., Kansky, M. T., and Roberts, J. A. (1997). Impaired spatial information processing in aged monkeys with preserved recognition memory. Neuroreport 8, 1923–1928. doi: 10.1097/00001756-199705260-00026
Rapp, P. R., Rosenberg, R. A., and Gallagher, M. (1987). An evaluation of spatial information processing in aged rats. Behav. Neurosci. 101, 3–12. doi: 10.1037/0735-7044.101.1.3
Reagh, Z. M., Ho, H. D., Leal, S. L., Noche, J. A., Chun, A., Murray, E. A., et al. (2016). Greater loss of object than spatial mnemonic discrimination in aged adults. Hippocampus 26, 417–422. doi: 10.1002/hipo.22562
Rolls, E. T., and Kesner, R. P. (2006). A computational theory of hippocampal function, and empirical tests of the theory. Prog. Neurobiol. 79, 1–48. doi: 10.1016/j.pneurobio.2006.04.005
Rosenbaum, R. S., Winocur, G., and Moscovitch, M. (2001). New views on old memories: re-evaluating the role of the hippocampal complex. Behav. Brain Res. 127, 183–197. doi: 10.1016/S0166-4328(01)00363-1
Rosenzweig, M. R., and Bennett, E. L. (1996). Psychobiology of plasticity: effects of training and experience on brain and behavior. Behav. Brain Res. 78, 57–65. doi: 10.1016/0166-4328(95)00216-2
Sahay, A., Scobie, K. N., Hill, A. S., O'Carroll, C. M., Kheirbek, M. A., Burghardt, N. S., et al. (2011). Increasing adult hippocampal neurogenesis is sufficient to improve pattern separation. Nature 472, 466–470. doi: 10.1038/nature09817
Sampedro-Piquero, P., Begega, A., Zancada-Menendez, C., Cuesta, M., and Arias, J. L. (2013). Age-dependent effects of environmental enrichment on brain networks and spatial memory in Wistar rats. Neuroscience 248, 43–53. doi: 10.1016/j.neuroscience.2013.06.003
Sarter, M., and Turchi, J. (2002). Age- and dementia-associated impairments in divided attention: psychological constructs, animal models, and underlying neuronal mechanisms. Dement. Geriatr. Cogn. Disord. 13, 46–58. doi: 10.1159/000048633
Scholz, J., Allemang-Grand, R., Dazai, J., and Lerch, J. P. (2015). Environmental enrichment is associated with rapid volumetric brain changes in adult mice. NeuroImage 109, 190–198. doi: 10.1016/j.neuroimage.2015.01.027
Schrijver, N. C., Bahr, N. I., Weiss, I. C., and Wurbel, H. (2002). Dissociable effects of isolation rearing and environmental enrichment on exploration, spatial learning and HPA activity in adult rats. Pharmacol. Biochem. Behav. 73, 209–224. doi: 10.1016/S0091-3057(02)00790-6
Segovia, G., Del Arco, A., de Blas, M., Garrido, P., and Mora, F. (2008). Effects of an enriched environment on the release of dopamine in the prefrontal cortex produced by stress and on working memory during aging in the awake rat. Behav. Brain Res. 187, 304–311. doi: 10.1016/j.bbr.2007.09.024
Segovia, G., Del Arco, A., Garrido, P., de Blas, M., and Mora, F. (2008). Environmental enrichment reduces the response to stress of the cholinergic system in the prefrontal cortex during aging. Neurochem. Int. 52, 1198–1203. doi: 10.1016/j.neuint.2007.12.007
Segovia, G., Yague, A. G., Garcia-Verdugo, J. M., and Mora, F. (2006). Environmental enrichment promotes neurogenesis and changes the extracellular concentrations of glutamate and GABA in the hippocampus of aged rats. Brain Res. Bull. 70, 8–14. doi: 10.1016/j.brainresbull.2005.11.005
Siette, J., Westbrook, R. F., Cotman, C., Sidhu, K., Zhu, W., Sachdev, P., et al. (2013). Age-specific effects of voluntary exercise on memory and the older brain. Biol. Psychiatry 73, 435–442. doi: 10.1016/j.biopsych.2012.05.034
Small, S. A., Chawla, M. K., Buonocore, M., Rapp, P. R., and Barnes, C. A. (2004). Imaging correlates of brain function in monkeys and rats isolates a hippocampal subregion differentially vulnerable to aging. Proc. Natl. Acad. Sci. USA 101, 7181–7186. doi: 10.1073/pnas.0400285101
Smith, T. D., Adams, M. M., Gallagher, M., Morrison, J. H., and Rapp, P. R. (2000). Circuit-specific alterations in hippocampal synaptophysin immunoreactivity predict spatial learning impairment in aged rats. J. Neurosci. 20, 6587–6593. doi: 10.1523/JNEUROSCI.20-17-06587.2000
Soffie, M., Hahn, K., Terao, E., and Eclancher, F. (1999). Behavioural and glial changes in old rats following environmental enrichment. Behav. Brain Res. 101, 37–49. doi: 10.1016/S0166-4328(98)00139-9
Speisman, R. B., Kumar, A., Rani, A., Pastoriza, J. M., Severance, J. E., Foster, T. C., et al. (2013). Environmental enrichment restores neurogenesis and rapid acquisition in aged rats. Neurobiol. Aging 34, 263–274. doi: 10.1016/j.neurobiolaging.2012.05.023
Spencer, W. D., and Raz, N. (1995). Differential effects of aging on memory for content and context: a meta-analysis. Psychol. Aging 10, 527–539. doi: 10.1037/0882-7974.10.4.527
Stark, S. M., Yassa, M. A., Lacy, J. W., and Stark, C. E. (2013). A task to assess behavioral pattern separation (BPS) in humans: data from healthy aging and mild cognitive impairment. Neuropsychologia 51, 2442–2449. doi: 10.1016/j.neuropsychologia.2012.12.014
Stark, S. M., Yassa, M. A., and Stark, C. E. (2010). Individual differences in spatial pattern separation performance associated with healthy aging in humans. Learn. Mem. 17, 284–288. doi: 10.1101/lm.1768110
Stefanko, D. P., Barrett, R. M., Ly, A. R., Reolon, G. K., and Wood, M. A. (2009). Modulation of long-term memory for object recognition via HDAC inhibition. Proc. Natl. Acad. Sci. USA 106, 9447–9452. doi: 10.1073/pnas.0903964106
Talamini, L. M., and Gorree, E. (2012). Aging memories: differential decay of episodic memory components. Learn. Mem. 19, 239–246. doi: 10.1101/lm.024281.111
Techentin, C., Voyer, D., and Voyer, S. D. (2014). Spatial abilities and aging: a meta-analysis. Exp. Aging Res. 40, 395–425. doi: 10.1080/0361073X.2014.926773
Teglas, T., Nemeth, Z., Koller, A., Van der Zee, E. A., Luiten, P. G. M., and Nyakas, C. (2019). Effects of Long-term moderate intensity exercise on cognitive behaviors and cholinergic forebrain in the aging rat. Neuroscience 411, 65–75. doi: 10.1016/j.neuroscience.2019.05.037
Teng, H. K., Teng, K. K., Lee, R., Wright, S., Tevar, S., Almeida, R. D., et al. (2005). ProBDNF induces neuronal apoptosis via activation of a receptor complex of p75NTR and sortilin. J. Neurosci. 25, 5455–5463. doi: 10.1523/JNEUROSCI.5123-04.2005
Toner, C. K., Pirogovsky, E., Kirwan, C. B., and Gilbert, P. E. (2009). Visual object pattern separation deficits in nondemented older adults. Learn. Mem. 16, 338–342. doi: 10.1101/lm.1315109
Toth, J. P., and Parks, C. M. (2006). Effects of age on estimated familiarity in the process dissociation procedure: the role of noncriterial recollection. Mem. Cogn. 34, 527–537. doi: 10.3758/BF03193576
Train the Brain ConsortiumMaffei, L., Picano, E., Andreassi, M. G., Angelucci, A., Baldacci, F., et al. (2017). Randomized trial on the effects of a combined physical/cognitive training in aged MCI subjects: the train the brain study. Sci. Rep. 7:39471. doi: 10.1038/srep39471
Trelle, A. N., Henson, R. N., Green, D. A. E., and Simons, J. S. (2017). Declines in representational quality and strategic retrieval processes contribute to age-related increases in false recognition. J. Exp. Psychol. Learn. Mem. Cogn. 43, 1883–1897. doi: 10.1037/xlm0000412
Trinchero, M. F., Buttner, K. A., Sulkes Cuevas, J. N., Temprana, S. G., Fontanet, P. A., Monzon-Salinas, M. C., et al. (2017). High plasticity of new granule cells in the aging Hippocampus. Cell Rep. 21, 1129–1139. doi: 10.1016/j.celrep.2017.09.064
van Praag, H., Kempermann, G., and Gage, F. H. (1999). Running increases cell proliferation and neurogenesis in the adult mouse dentate gyrus. Nat. Neurosci. 2, 266–270. doi: 10.1038/6368
van Praag, H., Kempermann, G., and Gage, F. H. (2000). Neural consequences of environmental enrichment. Nat. Rev. Neurosci. 1, 191–198. doi: 10.1038/35044558
Ventura, S., Duncan, S., and Ainge, J. A. (2024). Increased flexibility of CA3 memory representations following environmental enrichment. Curr. Biol. 34, 2011–2019.e7. doi: 10.1016/j.cub.2024.03.054
Wiig, K. A., and Burwell, R. D. (1998). Memory impairment on a delayed non-matching-to-position task after lesions of the perirhinal cortex in the rat. Behav. Neurosci. 112, 827–838. doi: 10.1037/0735-7044.112.4.827
Winocur, G. (1998). Environmental influences on cognitive decline in aged rats. Neurobiol. Aging 19, 589–597. doi: 10.1016/S0197-4580(98)00107-9
Wolfer, D. P., Litvin, O., Morf, S., Nitsch, R. M., Lipp, H. P., and Wurbel, H. (2004). Laboratory animal welfare: cage enrichment and mouse behaviour. Nature 432, 821–822. doi: 10.1038/432821a
Woo, N. H., Teng, H. K., Siao, C. J., Chiaruttini, C., Pang, P. T., Milner, T. A., et al. (2005). Activation of p75NTR by proBDNF facilitates hippocampal long-term depression. Nat. Neurosci. 8, 1069–1077. doi: 10.1038/nn1510
Yassa, M. A., Mattfeld, A. T., Stark, S. M., and Stark, C. E. (2011). Age-related memory deficits linked to circuit-specific disruptions in the hippocampus. Proc. Natl. Acad. Sci. USA 108, 8873–8878. doi: 10.1073/pnas.1101567108
Yeung, S. T., Myczek, K., Kang, A. P., Chabrier, M. A., Baglietto-Vargas, D., and Laferla, F. M. (2014). Impact of hippocampal neuronal ablation on neurogenesis and cognition in the aged brain. Neuroscience 259, 214–222. doi: 10.1016/j.neuroscience.2013.11.054
Keywords: spatial memory, middle-aged, environmental enrichment, object memory, recognition, interference
Citation: Miranda M, Navas MC, Zanoni Saad MB, Piromalli Girado D, Weisstaub N and Bekinschtein P (2024) Environmental enrichment in middle age rats improves spatial and object memory discrimination deficits. Front. Behav. Neurosci. 18:1478656. doi: 10.3389/fnbeh.2024.1478656
Received: 10 August 2024; Accepted: 30 September 2024;
Published: 17 October 2024.
Edited by:
Timothy J. Jarome, Virginia Tech, United StatesReviewed by:
Sydney Trask, Purdue University, United StatesCopyright © 2024 Miranda, Navas, Zanoni Saad, Piromalli Girado, Weisstaub and Bekinschtein. This is an open-access article distributed under the terms of the Creative Commons Attribution License (CC BY). The use, distribution or reproduction in other forums is permitted, provided the original author(s) and the copyright owner(s) are credited and that the original publication in this journal is cited, in accordance with accepted academic practice. No use, distribution or reproduction is permitted which does not comply with these terms.
*Correspondence: Magdalena Miranda, bWlyYW5kYS5tYWdkYWxlbmEubGJAZ21haWwuY29t
†These authors have contributed equally to this work and share last authorship
‡Present address: Magdalena Miranda, Institute of Functional Genomics, CRNS, INSERM, University of Montpellier, Montpellier, France
Disclaimer: All claims expressed in this article are solely those of the authors and do not necessarily represent those of their affiliated organizations, or those of the publisher, the editors and the reviewers. Any product that may be evaluated in this article or claim that may be made by its manufacturer is not guaranteed or endorsed by the publisher.
Research integrity at Frontiers
Learn more about the work of our research integrity team to safeguard the quality of each article we publish.