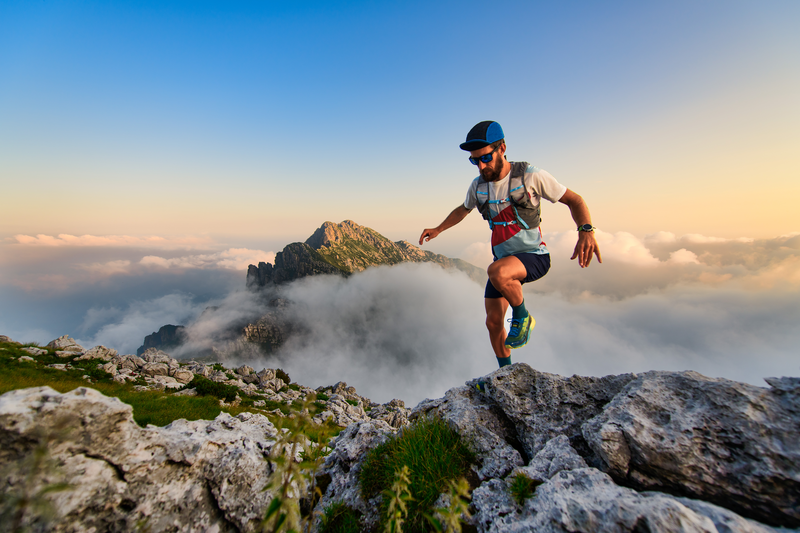
95% of researchers rate our articles as excellent or good
Learn more about the work of our research integrity team to safeguard the quality of each article we publish.
Find out more
REVIEW article
Front. Behav. Neurosci. , 30 June 2022
Sec. Pathological Conditions
Volume 16 - 2022 | https://doi.org/10.3389/fnbeh.2022.887312
This article is part of the Research Topic Endophenotypes Related to Attention, Inhibition, and Executive Control in Different Mental Disorders. The Search of Specific Patterns View all 4 articles
Sensory and sensorimotor gating provide the early processing of information under conditions of rapid presentation of multiple stimuli. Gating deficiency is observed in various psychopathologies, in particular, in schizophrenia. However, there is also a significant proportion of people in the general population with low filtration rates who do not show any noticeable cognitive decline. The review article presents a comparative analysis of existing data on the peculiarities of cholinergic and dopaminergic mechanisms associated with lowering gating in healthy individuals and in patients with schizophrenia. The differences in gating mechanisms in cohorts of healthy individuals and those with schizophrenia are discussed.
The mechanisms of sensory and sensorimotor gating provide adaptive patterns of responses to multiple stimuli presented with rapid succession (Swerdlow et al., 2000) working at the stages of pre-attention and early attention. A lot of studies reported the impairment of these gating mechanisms in patients with schizophrenia (Adler et al., 1982, 2004; Baker et al., 1987; Light and Braff, 1999; Braff et al., 2001; Perry et al., 2001; Cadenhead and Braff, 2002; Swerdlow et al., 2008; San-Martin et al., 2020).
In translational studies, the model of the suppression of P50 auditory event-related potential (AEP) in a condition–test paradigm is considered the putative measure of sensory gating. A deficit of sensory gating may result in sensory overflood and inappropriate assessment of stimuli salience (Evans et al., 2007).
To quantify sensorimotor gating, a model of prepulse inhibition (PPI) of the startle response is applied (Graham, 1975; Braff et al., 2001; Swerdlow et al., 2008). In this experimental paradigm, a quantitative measure of sensorimotor gating is a reduction in the startle reaction magnitude due to weak sensory pre-stimulation delivered with a short (<500 ms) stimulus onset asynchrony (SOA). PPI is regarded as a complex measure reflecting the early mechanisms of stimuli processing and action selection (Blumenthal, 2014).
The individual characteristics of sensory and sensorimotor gating are assumed to contribute to higher cognitive functions (working memory, voluntary attention and executive functions), which are deficient under psychopathological conditions (Bitsios and Giakoumaki, 2005; Holstein et al., 2011; Toyomaki et al., 2015). P50 suppression and PPI are impaired in patients with schizophrenia (Braff et al., 2001; Swerdlow et al., 2008; Braff, 2010; San-Martin et al., 2020, 2022). The measures display a significant degree of heritability (Clementz et al., 1998; Cadenhead et al., 2005; Hasenkamp et al., 2010; Earls et al., 2016; Togay et al., 2020; Li et al., 2021) and are considered to be sendo phenotypes of schizophrenia (Turetsky et al., 2007; DiLalla et al., 2017).
At the same time, numerical studies found the impairment of sensory and/or sensorimotor gating in some other mental and neurological disorders, such as Huntington's disease (Swerdlow et al., 1995), Tourette's syndrome (Swerdlow, 2013; Buse et al., 2016), obsessive–compulsive disorder (Ahmari et al., 2012), bipolar disorder (Baker et al., 1990; Sánchez-Morla et al., 2016; Mao et al., 2019; Atagun et al., 2020), and autism (Crasta et al., 2021). The deficit of PPI and P50 suppression was found in the prodromal stages of Alzheimer's disease and can be used to differentiate the early stages of this pathology from mild cognitive impairment (Jafari et al., 2020).
These data raise questions about the existence of mechanisms of gating impairment that are common or specific for different psychopathologies. Moreover, a lot of clinically unaffected individuals without substantial cognitive deficit display a low level of sensory or sensorimotor gating (Hasenkamp et al., 2010; Knott et al., 2013). Neuroimaging studies showed that patterns of associations between gating rates and the activity of different brain structures are distinct in healthy individuals and in patients with schizophrenia (Kumari et al., 1996; Williams et al., 2011; Naysmith et al., 2021).
These data suggest specificity in the contribution of different neurotransmitter system activities to deficiency of information gating in healthy subjects and in various psychopathologies. Among others, the study of this problem is important for the development of potential therapeutic approaches (Swerdlow et al., 2000). One of the first steps in this direction should be a comparative analysis of the neurotransmitter mechanisms of gating processes in healthy subjects and patients with schizophrenia.
Two main hypotheses about neurotransmitter disorders in schizophrenia consider the abnormal functioning of dopaminergic and glutamatergic signaling. The interaction of glutamatergic and dopaminergic systems in the prefrontal cortex plays a significant role in the mechanisms of attention and executive control (Aquila and Citrome, 2015; Storozheva et al., 2015). Of note, the results of the animal studies showed relatively independent pathways for the participation of glutamatergic and dopaminergic transmission in the mechanisms of PPI (Keith et al., 1991; Callahan et al., 2014; Oliveras et al., 2017). At the same time, the close interaction of dopaminergic and cholinergic activities on sensory and sensorimotor gating has been documented in several studies (De la Salle et al., 2013). It should be noted that only five associations of genetic variants with sensory or sensorimotor gating were confirmed in repeated studies of independent samples. Three of these five polymorphisms are located in genes that regulate dopaminergic (COMT) and cholinergic (CHRNA3 and CHRNA7 acetylcholine receptors) neurotransmission (Rovn et al., 2020).
This work aimed to provide a comparative analysis of the existing data on the involvement of dopaminergic and cholinergic signaling systems in sensory (P50 suppression) and sensorimotor (PPI) gating in populations of mentally healthy people and patients with schizophrenia. The most attention was paid to the results of genetic and pharmacological studies.
The startle reaction is a generalized defensive reaction evoked by a sudden intensive stimulus. In humans, startle is mainly estimated by the blink component. The most often used model is acoustic startle reaction (ASR) evoked by sudden sounds with intensity >95 dB. Prepulse inhibition (PPI) is the phenomenon of suppression of the amplitude of response to the intensive (main) stimulus by weaker (<90 dB) sounds (prepulse) presented with a short interval before the main signal. In different studies, the intervals between prepulse and the main stimulus (stimulus onset asynchrony—SOA) varied within the range from 10 to 300 ms (Braff et al., 2001; Swerdlow et al., 2008, 2018).
PPI is observed across species, including invertebrates (Engel and Hoy, 1999; Frost et al., 2003), fishes (Burgess and Granato, 2007), and mammals (Swerdlow et al., 2008). In mammals, the primary neural circuit that mediates the acoustic startle response originates from cochlear root neurons, which send projections to the caudal pontine reticular nucleus (PnC). Axons of PnC provide the excitation of motor neurons to produce the startle response (Lingenhöhl and Friauf, 1994). The PnC also receives modulatory projections from pedunculopontine and laterodorsal tegmental nuclei, which also receive acoustic information from the inferior and superior colliculi (Fendt et al., 2001). A lot of structures participate in the modulation of ASR, such as the striatum and pallidum, ventral tegmental area, hippocampus, amygdala, raphe nuclei, thalamus, and prefrontal cortex. It can be suggested that some of multiple circuits involved in startle modification differentially participate in PPI at various SOAs (Gómez-Nieto et al., 2020).
The association between PPI at SOA 120 ms and activity in the striatum, thalamus, insula, hippocampal, temporal, inferior frontal and inferior parietal regions was found in fMRI studies in healthy controls and in patients with schizophrenia (Kumari et al., 2007).
The activity of dopaminergic and cholinergic synapses largely determines the functions of both the main PPI circuits and the structures that participate in the modulation of PPI (Davis, 1980; Chandler et al., 2013; Prager and Plotkin, 2019).
Although changes in the activity of the dopaminergic system are considered as one of the main mechanisms of the aetiopathogenesis of schizophrenia, only a small number of polymorphisms in genes that regulate dopaminergic neurotransmission have been found to exert significant effects on sensorimotor gating (Montag et al., 2007; Roussos et al., 2008a,b; Quednow et al., 2018; Rovn et al., 2020). Only one of those, namely polymorphism rs4680 in the catechol-O-methyl transferase (COMT) gene, has been studied both in healthy controls and in patients with schizophrenia.
COMT is the main enzyme that degrades dopamine in the prefrontal cortex (Chen et al., 2004). Polymorphism rs4680 (Val158Met substitution) is associated with the enzymatic activity of COMT, meaning that the presence of the Val allele leads to an increased dopamine degradation rate and a decrease in its level in the prefrontal cortex. The effect of the COMT rs4680 polymorphism on PPI in healthy volunteers has been shown in a number of studies. The association of rs4680 with PPI in the general population was confirmed by a previous meta-analysis (Quednow et al., 2018). In the sample of Greek/Caucasian men, the highest level of PPI was observed in carriers of the methionine homozygote and the lowest was in carriers of the Val/Val genotype, while carriers of the Val/Met variant displayed intermediate values. Polynomial contrasts showed a linear relationship between PPI levels and Val allele load (Roussos et al., 2008b). Similar results were obtained in a Russian population of healthy men (Kirenskaya et al., 2015). In the study of Quednow et al. (2009), the effect of rs4680 on PPI was only significant in the male cohort, but not in the total gender-mixed Caucasian sample of healthy volunteers. In all of these studies of rs4680, the effects of rs4680 on PPI were found at SOAs 60 and 120 ms.
The study in young-adult Chinese women revealed the positive association of PPI (at SOA 120 ms) with the Met allele and also showed the stabilizing effect of the Met allele under conditions of menstrual-cycle-dependent PPI variation (Wu et al., 2019).
Montag et al. (2007) did not show the effect of rs4680 on PPI at SOA 120 ms in the cohort of mentally healthy Caucasian females.
In summary, the existing literature data allow an association to be proposed between the valine allele of the rs4680 polymorphism with a low level of PPI in a population of mentally healthy males. The question of the existence of such an association in mentally healthy females can be considered open.
Data on the effects of the COMT polymorphism rs4680 on PPI obtained in cohorts of patients with schizophrenia are contradictory. In a gender-mixed Chinese sample of patients with first-episode schizophrenia, no effect of rs4680 on PPI at SOA 120 ms was found (Liu et al., 2013). The study in Russian male patients with schizophrenia also failed to reveal any effects of rs4680 on PPI tested at SOAs 60 and 120 ms (Quednow et al., 2010; Kirenskaya et al., 2015). Quednow et al. (2009) found the effect of rs4680 on PPI at SOA 120 ms in a gender-mixed Caucasian cohort of patients with schizophrenia. In this study, the analyses of polynomial contrasts across the three genotype groups revealed a significant quadratic trend, unlike healthy controls who displayed a linear trend (see above). Accordingly, carriers of the Met/Met genotype displayed higher PPI levels compared to carriers of the Val/Met genotype, while the difference between Met/Met and Val/Val and between Val/Met and Val/Val was not significant. In summary, analysis of the available data suggests that the effects of the rs4680 polymorphism on the level of PPI are significantly less pronounced in patients with schizophrenia than in healthy subjects.
Most of the initial data on the effects of dopamine agonists and antagonists on PPI have been obtained in animal experiments. As the data were accumulated, comparative analysis showed that the effects of dopamine agonists and antagonists on PPI can differ in animals and humans. Accordingly, studies in patients and healthy controls are important for verification of the translational models of schizophrenia spectrum and other mental disorders.
In healthy individuals, the mixed D1/D2 agonist apomorphine decreased PPI in high-intensity (85 dB) prepulse trials and enhanced sensorimotor gating in persons with low baseline PPI under conditions of low (75 dB) prepulse intensity (Schellekens et al., 2010). In this study, the effects of apomorphine on the stability and selectivity of attention were estimated in the continuous performance test (AXCPT version). In brief, subjects were instructed to detect targets and non-targets within a stream of presented letters. Targets were the letter X when they followed the letter A and X following non-A cues were non-targets. The response to the non-target letter A is usually regarded as a commission error. In turn, the number of commission errors is a negative measure of the selectivity of attention. The authors found the impairment of AXCPT performance after apomorphine administration and an association between the high selectivity of attention (low commission errors) with reduced baseline sensorimotor gating at SOA 100 ms and low prepulse intensity. No studies have been conducted on the effects of apomorphine on PPI in patients with schizophrenia.
Data on the effects of D2 agonists on PPI in healthy persons are contradictory. Hutchison and Swift (1999) found a reduction in PPI at an SOA of 120 ms after the acute administration of 20 mg dexamphetamine. In another study with the same dose of amphetamine (Swerdlow et al., 2003), a significant decrease in PPI was observed at an SOA of 10 and 20 ms, but not at 30 or 60 ms; the decrease in PPI at an SOA of 120 ms was at the level of trend.
Kumari et al. (1998) found that the acute administration of d-amphetamine decreased PPI across 30-ms−60-ms−120-ms intervals in smoking individuals but not in the total sample of healthy males. However, the sample in this study was not large enough to estimate drug x SOA interaction.
A number of studies in healthy individuals did not find any substantial effect of amphetamines on PPI (Swerdlow et al., 2002a; Talledo et al., 2009; Chitty et al., 2014).
Swerdlow et al. (2002b) found that another D2 agonist bromocriptine decreased PPI at SOA 120 ms (but not at SOA 60 ms) and increased PPI at SOA 20 ms. The disruption of PPI at SOA 120 ms after bromocriptine administration was found also by Abduljawad et al. (1998, 1999) and Martin-Iverson (1999).
In the study of Swerdlow et al. (2002b), the D2 agonist amantadine decreased PPI at an SOA of 60 ms and 120 ms, but increased PPI at an SOA of 10 ms. In another study by these authors, the decrease in PPI at SOA 100 ms was found 15 min after amantadine administration; however, in the course of successive test sessions (from first to fifth with 15-min intervals), PPI increased to a level above that of the control group. Whether this trend is the result of a change in the effect of the drug over time or is related to the effect of amantadine on the effectiveness of retraining needs to be investigated.
When interpreting the effect of amantadine, it should be taken into account that it is also an NMDA glutamate receptor antagonist (Raupp-Barcaro et al., 2021).
In patients with schizophrenia, acute amphetamine enhanced PPI at an SOA of 60 ms (but not at an SOA of 120 ms) and increased it to a level comparable to that of control values. The increase in PPI after amphetamine administration was more pronounced in carriers of the methionine allele of rs4680 and displayed a correlation with positive symptoms (Swerdlow et al., 2018).
In healthy individuals, some authors (Abduljawad et al., 1998; Martin-Iverson, 1999; Oranje et al., 2004a) found the disruption of PPI estimated at an SOA of 120 ms after the administration of the D2 antagonist haloperidol. At the same time, haloperidol attenuated the disruptive effect of bromocriptine on PPI (Abduljawad et al., 1999; Martin-Iverson, 1999). Csomor et al. (2008) found that haloperidol had no effect on PPI in subjects with low baseline levels of PPI but attenuated PPI in subjects with high baseline gating levels. In this study, a positive association of high baseline PPI with the successful performance of the spatial working memory task was observed. Kumari et al. (1998) reported that haloperidol disrupted PPI in normal smoking subjects but had no effect on non-smoking individuals; however, the modest sample size of smoking participants in this work made it impossible to estimate the significance of the treatment x SOA interaction on PPI. Some studies reported no effect of haloperidol on PPI in healthy volunteers (Abduljawad et al., 1999; Graham et al., 2004).
None of the studies revealed the effect of haloperidol on PPI at different SOAs in patients with schizophrenia (Duncan et al., 2003; Kumari et al., 2007; Wynn et al., 2007). Also, long-term (2–6 weeks) treatment with amisulpride showed no effects on PPI, either in the cohort of initially antipsychotic-naive, first-episode schizophrenia patients or in mentally healthy individuals (Düring et al., 2014).
Thus, the data of both genetic and pharmacological studies suggest differences in dopaminergic mechanisms that determine the effectiveness of sensorimotor gating in healthy subjects and in patients with schizophrenia.
Studies of the association between polymorphic variants of the genes involved in cholinergic neurotransmission and the individual levels of sensorimotor gating revealed significant effects for the polymorphisms rs1051730 and rs1317286 in the gene encoding the alpha 3 subunit of nicotinic receptors (CHRNA3) (Petrovsky et al., 2010). In the human brain, high densities of CHRNA3 are observed in the frontal, temporal, and parietal cortex, thalamus, striatum, and hippocampus (Gotti and Clementi, 2004), that is in the structures that are considered to be involved in the mechanisms of PPI.
In healthy subjects, the effects of the rs1051730 and rs1317286 polymorphisms of the CHRNA3 gene on PPI were described by a linear trend (CC>CT>TT and AA>GA>GG, accordingly), while a significant quadratic trend was observed in patients with schizophrenia with regard to the maximum values of PPI in CT/GA heterozygotes (Petrovsky et al., 2010).
In healthy individuals, the effects of rs1051730 and rs1317286 on PPI were assessed at SOAs of 30 ms, 60 ms, and 120 ms; however, the authors did not report any significant interaction of interval x genotype effects. In patients with schizophrenia, the effects of the rs1051730/rs1317286 haplotype were estimated at SOA 120ms (Petrovsky et al., 2010).
In another study by the same authors, the effect of acute nicotine administration on PPI was evaluated in mentally healthy carriers of different variants to rs1051730. Nicotine has been shown to increase PPI in carriers of the TT variant (associated with low baseline PPI) and decrease it in carriers of the C allele. These effects of nicotine were observed at SOAs of 120 and 240 ms but not at an SOA of 30 or 60 ms (Petrovsky et al., 2010).
Nicotinic receptors that contain alpha 4 (CHRNA4) subunit display high affinity for nicotine and are expressed in the cortex, hippocampus, basal ganglia, and cerebellum (Gotti and Clementi, 2004). In the striatum, CHRNA4 is found in the dopaminergic neurons and is involved in the interaction of dopamine and cholinergic mechanisms of attention and working memory (Cools et al., 2007; Markett et al., 2010; Giessing et al., 2011). Also, it was found that the pattern of thalamo-cortical connectivity is associated with polymorphism of the CHRNA4 gene (Winterer et al., 2007; Giessing et al., 2011).
In the study of Shi et al. (2016), the effects of three polymorphisms in the CHRNA4 gene: rs3746372, rs1044396 and rs3787140 on PPI at an SOA of 30ms, 60ms, and 120ms, were estimated in Chinese samples of healthy volunteers and patients with schizophrenia.
In healthy individuals, no significant effects of the studied polymorphisms on PPI were found. In patients with schizophrenia, the level of PPI at an SOA of 120 ms was significantly lower in patients with the GG genotype of rs3746372 and the TT genotype of rs1044396 compared to other variants (Shi et al., 2016). Thus, the specific participation of CHRNA4 in mechanisms of PPI in patients with schizophrenia could be proposed.
In contrast to CHRNA3 and CHRNA4, no effects of the polymorphism of CHRNA7 on PPI were found in healthy individuals or in patients with schizophrenia (Liu et al., 2013; Bertelsen et al., 2015).
The data obtained in a number of studies indicate that the acute administration of nicotine, as well as its chronic consumption (smoking), increases the PPI in both healthy individuals and in patients with schizophrenia (Kumari et al., 1996, 1997; Postma et al., 2006; Baschnagel and Hawk, 2008; Rabin et al., 2009; Song et al., 2014), although some studies reported a decrease in the PPI after smoking high nicotine cigarettes compared to PPI after smoking low nicotine ones (Hutchison et al., 2000). George et al. (2006) found that the acute withdrawal from smoking impairs PPI in patients with schizophrenia but not in healthy controls. In addition, smoking reinstatement reverses the deficit of PPI caused by abstinence in patients without any effect on sensorimotor gating in control smokers. Mecamylamine, a preferential antagonist of subtype α(4)β(2) and α(3)-containing cholinergic receptors, prevented the effect of smoking on PPI in patients with schizophrenia without any effect on the control group. In the study by Duncan et al. (2001), a stimulating effect of nicotine on PPI after nocturnal withdrawal was found in healthy smoking subjects. However, taking into account the design of the experiment, it is also likely that the authors observed a direct effect of acute nicotine on PPI, regardless of abstinence.
Thus, it can be assumed that there are differences in the effects of the withdrawal and recovery of nicotine consumption on PPIs in healthy individuals and patients with schizophrenia; this difference is dependent on the activity of nicotine receptors.
The results obtained in the study of Postma et al. (2006) indicate multiple interactions of diagnosis, nicotine, SOA and time elapsed after nicotine administration on PPI.
Rabin et al. (2009) found that PPI is positively associated with cognitive performance (Wisconsin Card Sorting Test) in smoking patients with schizophrenia but not in non-smoking patients or healthy individuals.
In summary, although the existing data are not numerous, they indicate the difference in the genetic mechanisms that determine the effectiveness of sensorimotor gating in healthy subjects and in patients with schizophrenia. In particular, the difference in the rules of allele interactions between patients with schizophrenia and controls was revealed when the effects exerted on PPI by polymorphism rs4680 in the COMT gene, as well as polymorphisms rs1051730 and rs1317286 in the CHRNA3 gene, were estimated. Also, the effects of polymorphisms rs3746372 and rs1044396 in the CHRNA4 gene were only observed in schizophrenia, but not in controls. The results of pharmacological studies show that acute withdrawal from smoking and the reinstatement of nicotine consumption exert an effect on PPI only in patients with schizophrenia, but not in healthy people. Moreover, these effects that are specific to patients are mediated by α(4)β(2) and α(3)-containing cholinergic receptors, which is in agreement with the results of previous genetic studies.
The available results of pharmacological studies show that the dopaminergic mechanisms of sensorimotor gating in humans are almost as mysterious as the dopaminergic mechanisms of schizophrenia.
The effects of dopamine receptor agonists, such as apomorphine, bromocriptine, and amantadine, have not been studied in patients with schizophrenia, which makes comparative analysis impossible. However, data obtained in a population of mentally healthy individuals deserve analysis. Of great interest is the pronounced dependence of the effects of these drugs on SOA length. Surprisingly, both agonists (amphetamine, bromocriptine and amantadine) and antagonists (haloperidol) of D2 receptors reduce PPI in healthy subjects. The most frequent decrease in PPI after D2 agonist administration was observed at an SOA of 120 ms.
The effects of D2 agonists on PPI at SOAs <30 ms displayed an association with their effects on dopamine synthesis (detected in vitro). Thus, amphetamine, which elevates dopamine synthesis in presynaptic endings, significantly decreased PPI at an SOAs of 10 ms and 20 ms, while bromocriptine, which suppresses the synthesis of dopamine in synaptosomes, increased PPI at an SOA of 20 ms (Tissari, 1988; Tissari and Lillgäls, 1993; Swerdlow et al., 2003). These data suggest the mechanisms of “early” PPI may depend on the activity of dopaminergic projections to pontine and medullary structures (Kitahama et al., 2000; Sharma et al., 2018).
Importantly, there were no effects of amphetamine on “early” PPI in patients with schizophrenia (Swerdlow et al., 2018).
It should be noted that PPI at an SOA of 120 ms displayed the highest level of heritability (Roussos et al., 2016). At the same time, data from studies evaluating the differences in PPI between patients and healthy individuals with various SOAs are inconsistent.
Thus, most authors reported that PPI deficits in schizophrenia were observed at an SOA of 60-ms intervals but not at 30 or 120 ms (Wang et al., 2013; Swerdlow et al., 2015, 2018). Some other studies revealed the lowering of PPI at SOA 120 ms in patients with schizophrenia (Hong et al., 2007; Wynn et al., 2007). Ludewig and Vollenweider (2002) found that patients with schizophrenia with deficit syndrome showed an impairment of PPI at an SOA of 60 ms, while patients with a non-deficit syndrome showed a PPI that was lowered at longer SOAs (up to 240 ms). In this regard, of particular interest are the data obtained by Swerdlow et al. (2017), who found that amphetamine caused an increase in PPI at an SOA of 60 ms in patients with schizophrenia and the level of enhanced PPI positively correlated with positive symptoms. Positive correlations between PPI level and hallucinations were also found in schizophrenic patients (Storozheva et al., 2015). At that, Cadenhead (2011) assessed PPI in medication naive early psychosis are at risk psychosis subjects. She found that subjects who later developed psychosis had a greater PPI at an SOA of 60 ms compared to the healthy controls.
Thus, dopaminergic mechanisms can be involved in compensatory processes in conditions of psychosis development. The association of the effects of amphetamine on PPI with the polymorphism of COMT, discovered by Swerdlow et al. (2018), suggests the dependence of such compensatory processes on activity of the prefrontal cortex. It is also worth noting that the modulating effect of amphetamine on PPI is only observed in animals with a mature prefrontal cortex (Mena et al., 2021). Such cortical-dependent compensatory processes may be of particular interest in understanding the pathogenesis of schizophrenia.
It is believed that sensorimotor gating protects contextual information from the interfering effects of extreme signals, providing working memory processes. However, excessive attention to contextual information (extremely high PPI) can also disturb mental functions and behavioral processes. Indeed, while Bitsios et al. (2006) found positive associations of PPI with successful strategy formation and the speed of spatial memory task performance, Schellekens et al. (2010) revealed an association between high selectivity of attention in continuous performance tasks and reduced levels of gating.
These data raise questions surrounding the existence of optimal level of gating, and comprehensive comparison of healthy individuals and patients with schizophrenia with high and low PPI levels should be provided. Such a systematic study may include the analysis of relevant genetic polymorphisms, as well as psychophysiological and cognitive tests.
The assumption of the participation of the dopaminergic system in maintenance of the optimal level of sensorimotor gating is also supported by the observed effects of haloperidol, which increase the PPI in healthy individuals with initially low levels and reduce in healthy individuals with initially high PPI levels.
Auditory sensory gating is usually assessed in a paired-click paradigm that involves the presentation of two identical clicks (S1 and S2). Estimation of the auditory event-related potential at around 50 ms post-click (mid-latency P50 component) reveals a reduced amplitude in response to S2 (testing stimulus) relative to the amplitude in response to S1 (conditioning stimulus). The optimal interval between S1 and S2 is 500 ms.
Neuronal correlates of sensory gating were found in the hippocampus, temporo-parietal, and prefrontal cortex (Grunwald et al., 2003; Freedman et al., 2020). The mechanisms of gating per se are mainly associated with hippocampal and prefrontal activity (Yvert et al., 2001; Korzyukov et al., 2007; Freedman et al., 2020). Accordingly, polymorphism rs4680 in the COMT gene was proposed to be associated with the efficacy of frontal gating mechanisms.
Some studies revealed the effect of rs4680 genotype on sensory gating in schizophrenia patients with significantly lower P50 suppression rate in Val/Val carriers (Lu et al., 2007; Liu et al., 2013). The study of Lu et al. (2007) included the samples of patients and healthy individuals; the latter did not display any significant effect of rs4680 genotype on sensory gating.
The association of the Val/Val genotype with decreased P50 gating was found in healthy individuals by De la Salle et al. (2013).
The opposite result was obtained by Storozheva et al. (2019) who found the maximum amplitude for the first stimulus in the pair (A1) and the highest level of P50 suppression in healthy carriers of Val/Val genotype. No effects of COMT on P50 were found in patients with schizophrenia.
Some authors failed to find any substantial effects of the rs4680 polymorphism in the COMT gene on P50 suppression rate in patients with schizophrenia or in healthy persons (Shaikh et al., 2011; Demily et al., 2016; Mao et al., 2016).
Therefore, it is not possible to draw an unambiguous conclusion about the similarity or difference between the effects of rs4680 on sensory gating in patients and healthy people.
It is worth noting that a significant effect of the COMT genotype was observed in healthy persons for another measure of sensory gating—N100 suppression, with the poorer gating in Met/Met individuals compared to carriers of the Val allele (Majic et al., 2011).
Millar et al. (2011) and Knott et al. (2010) found the effects of polymorphisms in the dopamine transporter (VNTR) and D2 receptor (TaqIA) genes on P50 gating in healthy individuals, but similar studies have not been conducted in patients with schizophrenia.
Data on the effects of compounds that directly affect dopaminergic neurotransmission on sensory gating are controversial. Light et al. (1999) found that amphetamine disrupted P50 suppression in healthy persons. In a study by Csomor et al. (2008), it was found that haloperidol increased P50 suppression in healthy subjects exhibiting low P50 gating and disrupted P50 suppression in individuals with initially high P50 gating rates. In another study, the disruptive effect of haloperidol in healthy persons was observed (Witten et al., 2016). A lot of studies have found no effects on PPI of substances that affect dopaminergic transmission (Arango et al., 2003; Oranje et al., 2004b; Düring et al., 2014). Overall, the dopaminergic mechanisms of sensory gating remain poorly understood.
In a family study (Freedman et al., 2006), the P50 sensory gating level was found to be genetically linked to 15q13–14.3, which contains the alpha 7 nicotinic receptor subunit (CHRNA7) gene.
In hominids, CHRNA7 gene was partially duplicated, forming a new gene, CHRFAM7A, the product of which acts as a negative regulator of 7alpha function. A 2-bp deletion in exon 6 of CHRFAM7A (rs67158670) enhances its inhibitory activity (Araud et al., 2011). It was shown that the presence of at least one 2-bp deletion is associated with a deficit of P50 gating in healthy individuals (Flomen et al., 2013). In patients with schizophrenia, the contribution of this polymorphism to the mechanisms of sensory gating was found to be significantly less pronounced than in the control sample (Raux et al., 2002).
Leonard et al. (2002) screened the promoter zone of CHRNA7 and found multiple polymorphic patterns that displayed significant associations with sensory gating in the control group but not in patients with schizophrenia. However, Houy et al. (2004) failed to replicate these results. Moreover, in contrast to previous results, one of the polymorphisms in the CHRNA7 promoter region (-194C) showed a positive association with P50 suppression, which was observed in the total sample of control subjects and patients.
In some studies, there was no association between different single-nucleotide polymorphisms in the CHRNA7 gene and P50 parameters or suppression rates (Cabranes et al., 2013; Bertelsen et al., 2015), so the problem needs further investigation.
Nicotine intake (smoking) was found to enhance sensory gating in healthy individuals with baseline low rates of P50 suppression (mainly, in carriers of Val/Val variant of COMT rs4680 polymorphism) and in patients with schizophrenia, but not in bipolar disorder (Whitton et al., 2021). In healthy persons, the effect of nicotine on P50 gating was relatively modest and was significantly enhanced after combined nicotine and MAO-A inhibitor compared to placebo and to the nicotine-alone condition (Smith et al., 2015).
In an attempt to determine the types of receptors mediating the effect of nicotine on sensory filtration, Hong et al. (2007) studied the effects of moderate doses (titration) of varenicline and found an increase in the level of P50 suppression in patients with schizophrenia. Since varenicline in low doses activates the alpha-4-beta-2 subunit containing cholinergic receptors, it can be assumed that the mechanisms of sensory filtration in schizophrenia are associated with the activity of this type of high-affinity receptors. At the same time, genetic studies show that the activity of low-affinity α7 receptors is substantial for P50 suppression mechanisms in healthy subjects.
The existence of different mechanisms of the deficit of P50 suppression that are specific in healthy and schizophrenic individuals is also supported by the fact that only patients with schizophrenia who do acute cigarette smoking increase the correlation between prefrontal executive cognitive functioning and P50 suppression (Rabin et al., 2009).
Although the results of genetic and pharmacological studies are not numerous in the literature, they indicate the relative specificity in the patterns of dopaminergic and cholinergic activities associated with the level of information gating in healthy and schizophrenic individuals. Some data also point to the expediency of developing the concept of the optimal level of sensory and sensorimotor activity.
This can help to identify specific patterns of attention disorders and executive functions in numerous psychopathologies.
An important condition for the success of such studies is the use of unified protocols in cohorts of healthy individuals and those with mental disorders.
AP: formulation of the idea of the review, search and analysis of literature, writing, and design of articles.
The author declares that the research was conducted in the absence of any commercial or financial relationships that could be construed as a potential conflict of interest.
The handling editor ZS declared a past co-authorship with the author.
All claims expressed in this article are solely those of the authors and do not necessarily represent those of their affiliated organizations, or those of the publisher, the editors and the reviewers. Any product that may be evaluated in this article, or claim that may be made by its manufacturer, is not guaranteed or endorsed by the publisher.
Abduljawad, K. A., Langley, R. W., Bradshaw, C. M., and Szabadi, E. (1998). Effects of bromocriptine and haloperidol on prepulse inhibition of the acoustic startle response in man. J. Psychopharmacol. 3, 239–245. doi: 10.1177/026988119801200302
Abduljawad, K. A., Langley, R. W., Bradshaw, C. M., and Szabadi, E. (1999). Effects of bromocriptine and haloperidol on prepulse inhibition: comparison of the acoustic startle eyeblink response and the N1/P2 auditory-evoked response in man. J. Psychopharmacol. 1, 3–9. doi: 10.1177/026988119901300101
Adler, L. E., Olincy, A., Cawthra, E. M., McRae, K. A., Harris, J. G., Nagamoto, H. T., et al. (2004). Varied effects of atypical neuroleptics on P50 auditory gating in schizophrenia patients. Am. J. Psychiatry. 10, 1822–1828. doi: 10.1176/ajp.161.10.1822
Adler, L. E., Pachtman, E., Franks, R. D., Pecevich, M., Waldo, M. C., and Freedman, R. (1982). Neurophysiological evidence for a defect in neuronal mechanisms involved in sensory gating in schizophrenia. Biol. Psychiatry. 6, 639–654.
Ahmari, S. E., Risbrough, V. B., Geyer, M. A., and Simpson, H. B. (2012). Impaired sensorimotor gating in unmedicated adults with obsessive-compulsive disorder. Neuropsychopharmacology 5, 1216–1223. doi: 10.1038/npp.2011.308
Aquila, R., and Citrome, L. (2015). Cognitive impairment in schizophrenia: the great unmet need. CNS Spectr. (2015) 1, 35–39. doi: 10.1017/S109285291500070X
Arango, C., Summerfelt, A., and Buchanan, R. W. (2003), Olanzapine effects on auditory sensory gating in schizophrenia. Am. J. Psychiatry 11, 2066–2068. doi: 10.1176/appi.ajp.160.11.2066
Araud, T., Graw, S., Berger, R., Lee, M., Neveu, E., Bertrand, D., et al. (2011). The chimeric gene CHRFAM7A, a partial duplication of the CHRNA7 gene, is a dominant negative regulator of α7*nAChR function. Biochem. Pharmacol. 8, 904–914. doi: 10.1016/j.bcp.2011.06.018
Atagun, M. I., Drukker, M., Hall, M. H., Altun, I. K., Tatli, S. Z., Guloksuz, S., et al. (2020). Meta-analysis of auditory P50 sensory gating in schizophrenia and bipolar disorder. Psychiatry Res. Neuroimaging 300:111078. doi: 10.1016/j.pscychresns.2020.111078
Baker, N., Adler, L. E., Franks, R. D., Waldo, M., Berry, S., Nagamoto, H. et al. (1987). Neurophysiological assessment of sensory gating in psychiatric inpatients: comparison between schizophrenia and other diagnoses. Biol. Psychiatry. 22, 603–617. doi: 10.1016/0006-3223(87)90188-0
Baker, N. J., Staunton, M., Adler, L. E., Gerhardt, G. A., Drebing, C., Waldo, M., et al. (1990). Sensory gating deficits in psychiatric inpatients: relation to catecholamine metabolites in different diagnostic groups. Biol. Psychiatry 5, 519–528. doi: 10.1016/0006-3223(90)90443-6
Baschnagel, J. S., and Hawk, L. W., (2008). The effects of nicotine on the attentional modification of the acoustic startle response in nonsmokers. Psychopharmacology 1, 93–101. doi: 10.1007/s00213-008-1094-y
Bertelsen, B., Oranje, B., Melchior, L., Fagerlund, B., Werge, T. M., Mikkelsen, J. D., et al. (2015). Association study of CHRNA7 promoter variants with sensory and sensorimotor gating in schizophrenia patients and healthy controls: a Danish case-control study. Neuromol. Med. 4, 423–430. doi: 10.1007/s12017-015-8371-9
Bitsios, P., and Giakoumaki, S. G. (2005). Relationship of prepulse inhibition of the startle reflex to attentional and executive mechanisms in man. Int. J. Psychophysiol. 2, 229–241. doi: 10.1016/j.ijpsycho.2004.08.002
Bitsios, P., Giakoumaki, S. G., Theou, K., and Frangou, S. (2006). Increased prepulse inhibition of the acoustic startle response is associated with better strategy formation and execution times in healthy males. Neuropsychologia 44, 2494–2499. doi: 10.1016/j.neuropsychologia.2006.04.001
Blumenthal, T. D. (2014). Presidential address 2014: The more-or-less interrupting effects of the startle response. Psychophysiology 11, 1417–1431. doi: 10.1111/psyp.12506
Braff, D. L. (2010). Prepulse inhibition of the startle reflex: a window on the brain in schizophrenia. Curr. Top. Behav. Neurosci. 4, 349–371. doi: 10.1007/7854_2010_61
Braff, D. L., Geyer, M. A., and Swerdlow, N. R. (2001). Human studies of prepulse inhibition of startle: normal subjects, patient groups, and pharmacological studies. Psychopharmacology 2–3, 234–258. doi: 10.1007/s002130100810
Burgess, H. A., and Granato, M. (2007). Sensorimotor gating in larval zebrafish. J. Neurosci. 27, 4984–4894. doi: 10.1523/JNEUROSCI.0615-07.2007
Buse, J., Beste, C., Herrmann, E., and Roessner, V. (2016). Neural correlates of altered sensorimotor gating in boys with Tourette Syndrome: A combined EMG/fMRI study. World J. Biol. Psychiatry 3, 187–197. doi: 10.3109/15622975.2015.1112033
Cabranes, J. A., Ancín, I., Santos, J. L., Sánchez-Morla, E., García-Jiménez, M. Á., López-Ibor, J. J., et al. (2013). No effect of polymorphisms in the non-duplicated region of the CHRNA7 gene on sensory gating P50 ratios in patients with schizophrenia and bipolar disorder. Psychiatry Res. 3, 276–278. doi: 10.1016/j.psychres.2012.08.015
Cadenhead, K. S. (2011). Startle reactivity and prepulse inhibition in prodromal and early psychosis: effects of age, antipsychotics, tobacco and cannabis in a vulnerable population. Psychiatry Res. 2, 208–216. doi: 10.1016/j.psychres.2011.04.011
Cadenhead, K. S., and Braff, D. L. (2002). Endophenotypingschizotypy: a prelude to genetic studies within the schizophrenia spectrum. Schizophr Res. 1–2, 47–57. doi: 10.1016/s0920-9964(01)00351-6
Cadenhead, K. S., Light, G. A., Shafer, K. M., and Braff, D. L. (2005). P50 suppression in individuals at risk for schizophrenia: the convergence of clinical, familial, and vulnerability marker risk assessment. Biol. Psychiatry 12, 1504–1509. doi: 10.1016/j.biopsych.2005.03.003
Callahan, P. M., Terry, A. V., and . Tehim, A. (2014). Effects of the nicotinic α7 receptor partial agonist GTS-21 on NMDA-glutamatergic receptor related deficits in sensorimotor gating and recognition memory in rats. Psychopharmacology (Berl) 18, 3695–3706. doi: 10.1007/s00213-014-3509-2
Chandler, D. J., Lamperski, C. S., and Waterhouse, B. D. (2013). Identification and distribution of projections from monoaminergic and cholinergic nuclei to functionally differentiated subregions of prefrontal cortex. Brain Res. 1522, 38–58. doi: 10.1016/j.brainres.2013.04.057
Chen, J., Lipska, B. K., Halim, N., Ma, Q. D., Matsumoto, M., Melhem, S., et al. (2004). Functional analysis of genetic variation in catechol-O-methyltransferase (COMT): effects on mRNA, protein, and enzyme activity in postmortem human brain. Am. J. Hum. Genet. 75, 807–821. doi: 10.1086/425589
Chitty, K., Albrecht, M. A., Graham, K., Kerr, C., Lee, J. W., Iyyalol, R., et al. (2014). Dexamphetamine effects on prepulse inhibition (PPI) and startle in healthy volunteers. Psychopharmacology (Berl) 11, 2327–2337. doi: 10.1007/s00213-013-3395-z
Clementz, B. A., Geyer, M. A., and Braff, D. L. (1998). Poor P50 suppression among schizophrenia patients and their first-degree biological relatives. Am. J. Psychiatry. 12, 1691–1694. doi: 10.1176/ajp.155.12.1691
Cools, R., Sheridan, M., Jacobs, E., and D'Esposito, M. (2007). Impulsive personality predicts dopamine-dependent changes in frontostriatal activity during component processes of working memory. J. Neurosci. 20, 5506–5514. doi: 10.1523/jneurosci.0601-07.2007
Crasta, J. E., Gavin, W. J., and Davies, P. L. (2021). Expanding our understanding of sensory gating in children with autism spectrum disorders. Clin. Neurophysiol. 1, 180–190. doi: 10.1016/j.clinph.2020.09.020
Csomor, P. A., Stadler, R. R., Feldon, J., Yee, B. K., Geyer, M. A., and Vollenweider, F. X. (2008). Haloperidol differentially modulates prepulse inhibition and p50 suppression in healthy humans stratified for low and high gating levels. Neuropsychopharmacology 33, 497–512. doi: 10.1038/sj.npp.1301421
Davis, M. (1980). Neurochemical modulation of sensory-motor reactivity: acoustic and tactile startle reflexes. Neurosci. Biobehav. Rev. 2, 241–263. doi: 10.1016/0149-7634(80)90016-0
De la Salle, S., Smith, D., Choueiry, J., Impey, D., Philippe, T., Dort, H., et al. (2013). Effects of COMT genotype on sensory gating and its modulation by nicotine: Differences in low and high P50 suppressors. Neuroscience 241, 147–156. doi: 10.1016/j.neuroscience.2013.03.029
Demily, C., Louchart-de-la-Chapelle, S., Nkam, I., Ramoz, N., Denise, P., Nicolas, A., et al. (2016). Does COMT val158met polymorphism influence P50 sensory gating, eye tracking or saccadic inhibition dysfunctions in schizophrenia? Psychiatry Res. 246, 738–744. doi: 10.1016/j.psychres.2016.07.066
DiLalla, L. F., McCrary, M., and Diaz, E. (2017). A review of endophenotypes in schizophrenia and autism: The next phase for understanding genetic etiologies. Am. J. Med. Genet. C Semin. Med. Genet. 3, 354–361. doi: 10.1002/ajmg.c.31566
Duncan, E., Madonick, S., Chakravorty, S., Parwani, A., Szilagyi, S., Efferen, T., et al. (2001). Effects of smoking on acoustic startle and prepulse inhibition in humans. Psychopharmacology (Berl) 2–3, 266–272. doi: 10.1007/s002130100719
Duncan, E., Szilagyi, S., Schwartz, M., Kunzova, A., Negi, S., Efferen, T., et al. (2003). Prepulse inhibition of acoustic startle in subjects with schizophrenia treated with olanzapine or haloperidol. Psychiatry Res. 1, 1–12. doi: 10.1016/s0165-1781(03)00161-6
Düring, S., Glenthøj, B. Y., Andersen, G. S., and Oranje, B. (2014). Effects of dopamine D2/D3 blockade on human sensory and sensorimotor gating in initially antipsychotic-naive, first-episode schizophrenia patients. Neuropsychopharmacology 13, 3000–3008. doi: 10.1038/npp.2014.152
Earls, H. A., Curran, T., and Mittal, V. (2016). A meta-analytic review of auditory event-related potential components as endophenotypes for schizophrenia: perspectives from first-degree relatives. Schizophr. Bull. 6, 1504–1516. doi: 10.1093/schbul/sbw047
Engel, J. E., and Hoy, R. R. (1999). Experience-dependent modification of ultrasound auditory processing in a cricket escape response. J. Exp. Biol. 20, 2797–2806
Evans, L. H., Gray, N. S., and Snowden, R. J. (2007). Reduced P50 suppression is associated with the cognitive disorganisation dimension of schizotypy. Schizophr Res. 1–3, 152–162. doi: 10.1016/j.schres.2007.07.019
Fendt, M., Li, L., and Yeomans, J. S. (2001). Brain stem circuits mediating prepulse inhibition of the startle reflex. Psychopharmacology (Berl) 2–3, 216–224. doi: 10.1007/s002130100794
Flomen, R. H., Shaikh, M., Walshe, M., Schulze, K., Hall, M., H., et al. (2013). Association between the 2-bp deletion polymorphism in the duplicated version of the alpha7 nicotinic receptor gene and P50 sensory gating. Eur. J. Hum. Genet. 1, 76–81. doi: 10.1038/ejhg.2012.81
Freedman, R., Coon, H., Myles-Worsley, M., Orr-Urtreger, A., Olincy, A., Davis, A., et al. (2006). Linkage of a neurophysiological deficit in schizophrenia to a chromosome 15 locus. Proc. Natl. Acad. Sci. U.S.A. 94, 587–592. doi: 10.1073/pnas.94.2.587
Freedman, R., Olsen-Dufour, A. M., and Olincy, A. (2020). Consortium on the genetics of schizophrenia. P50 inhibitory sensory gating in schizophrenia: analysis of recent studies. Schizophr Res. 218, 93–98. doi: 10.1016/j.schres.2020.02.003
Frost, W. N., Tian, L. M., Hoppe, T. A., Mongeluzi, D. L., and Wang, J. (2003). A cellular mechanism for prepulse inhibition. Neuron 5, 991–1001. doi: 10.1016/s0896-6273(03)00731-1
George, T. P., Termine, A., Sacco, K. A., Allen, T. M., Reutenauer, E., Vessicchio, J. C., et al. (2006). A preliminary study of the effects of cigarette smoking on prepulse inhibition in schizophrenia: involvement of nicotinic receptor mechanisms. Schizophr. Res. 1–3, 307–315. doi: 10.1016/j.schres.2006.05.022
Giessing, C., Neber, T., and Thiel, C. M. (2011). Genetic variation in nicotinic receptors affects brain networks involved in reorienting attention. Neuroimage 1, 831–839. doi: 10.1016/j.neuroimage.2011.07.061
Gómez-Nieto, R., Hormigo, S., and López, D. E. (2020). Prepulse inhibition of the auditory startle reflex assessment as a hallmark of brainstem sensorimotor gating mechanisms. Brain Sci. 9:639. doi: 10.3390/brainsci10090639
Gotti, C., and Clementi, F. (2004). Neuronal nicotinic receptors: from structure to pathology. Prog. Neurobiol. 6, 363–396. doi: 10.1016/j.pneurobio.2004.09.006
Graham, F. K. (1975). The more or less startling effects of weak prestimulation. Psychophysiology 12, 238–248.
Graham, S. J., Scaife, J. C., Balboa Verduzco, A. M., Langley, R. W., Bradshaw, C. M., et al. (2004). Effects of quetiapine and haloperidol on prepulse inhibition of the acoustic startle (eyeblink) response and the N1/P2 auditory evoked response in man. J. Psychopharmacol. 2, 173–180. doi: 10.1177/0269881104042615
Grunwald, T., Boutros, N. N., Pezer, N., von Oertzen, J., Fernández, G., Schaller, C., et al. (2003). Neuronal substrates of sensory gating within the human brain. Biol. Psychiatry. 6, 511–519. doi: 10.1016/s0006-3223(02)01673-6
Hasenkamp, W., Epstein, M. P., Green, A., Wilcox, L., Boshoven, W., Lewison, B., et al. (2010). Heritability of acoustic startle magnitude, prepulse inhibition, and startle latency in schizophrenia and control families. Psychiatry Res. 2, 236–243. doi: 10.1016/j.psychres.2009.11.012
Holstein, D. H., Csomor, P. A., Geyer, M. A., Huber, T., Brugger, N., Studerus, E., et al. (2011). The effects of sertindole on sensory gating, sensorimotor gating, and cognition in healthy volunteers. J. Psychopharmacol. 12, 1600–1613. doi: 10.1177/0269881111415734
Hong, L. E., Wonodi, I., Lewis, J., and Thaker, G. K. (2007). Nicotine effect on prepulse inhibition and prepulse facilitation in schizophrenia patients. Neuropsychopharmacology 9, 2167–2174. doi: 10.1038/sj.npp.1301601
Houy, E., Raux, G., Thibaut, F., Belmont, A., Demily, C., Allio, G., et al. (2004). The promoter−194 C polymorphism of the nicotinic alpha 7 receptor gene has a protective effect against the P50 sensory gating deficit. Mol. Psychiatry 3, 320–322. doi: 10.1038/sj.mp.4001443
Hutchison, K. E., Niaura, R., and Swift, R. (2000). The effects of smoking high nicotine cigarettes on prepulse inhibition, startle latency, and subjective responses. Psychopharmacology (Berl) 3, 244–252. doi: 10.1007/s002130000399
Hutchison, K. E., and Swift, R. (1999). Effect of d-amphetamine on prepulse inhibition of the startle reflex in humans. Psychopharmacology (Berl) 4, 394–400. doi: 10.1007/s002130050964
Jafari, Z., Kolb, B. E., and Mohajerani, M. H. (2020). Prepulse inhibition of the acoustic startle reflex and P50 gating in aging and Alzheimer's disease. Ageing Res. Rev. 59:101028. doi: 10.1016/j.arr.2020.101028/
Keith, V. A., Mansbach, R. S., and Geyer, M. A. (1991). Failure of haloperidol to block the effects of phencyclidine and dizocilpine on prepulse inhibition of startle. Biol. Psychiatry 6, 557–566. doi: 10.1016/0006-3223(91)90025-h
Kirenskaya, A. V., Storozheva, Z. I., Sherstnev, V. V., and Kolobov, V. V. (2015). The acoustic startle response and polymorphism of the gene for catechol-O-methyltransferase in the norm and in schizophrenia. Neurochem. J. 1, 76–83. doi: 10.1134/S1819712415010031
Kitahama, K., Nagatsu, I., Geffard, M., and Maeda, T. (2000). Distribution of dopamine-immunoreactive fibers in the rat brainstem. J. Chem. Neuroanat. 18, 1–9. doi: 10.1016/s0891-0618(99)00047-2
Knott, V., de la Salle, S., Smith, D., Phillipe, T., Dort, H., Choueiry, J., et al. (2013), Baseline dependency of nicotine's sensory gating actions: similarities and differences in low, medium and high P50 suppressors. J. Psychopharmacol. 9, 790–800. doi: 10.1177/0269881113490449.
Knott, V., Millar, A., Fisher, D., and Albert, P. (2010). Effects of nicotine on the amplitude and gating of the auditory P50 and its influence by dopamine D2 receptor gene polymorphism. Neuroscience 1, 145–156. doi: 10.1016/j.neuroscience.2009.11.053
Korzyukov, O., Pflieger, M. E., Wagner, M., Bowyer, S. M., Rosburg, T., Sundaresan, K., et al. (2007). Generators of the intracranial P50 response in auditory sensory gating. Neuroimage 35, 814–826. doi: 10.1016/j.neuroimage.2006.12.011
Kumari, V., Antonova, E., Geyer, M. A., Ffytche, D., Williams, S. C., et al. (2007). A fMRI investigation of startle gating deficits in schizophrenia patients treated with typical or atypical antipsychotics. Int. J. Neuropsychopharmacol. 4, 463–477. doi: 10.1017/S1461145706007139
Kumari, V., Checkley, S. A., and Gray, J. A. (1996). Effect of cigarette smoking on prepulse inhibition of the acoustic startle reflex in healthy male smokers. Psychopharmacology (Berl) 1, 54–60. doi: 10.1007/s002130050109
Kumari, V., Cotter, P. A., Checkley, S. A., and Gray, J. A. (1997). Effect of acute subcutaneous nicotine on prepulse inhibition of the acoustic startle reflex in healthy male non-smokers. Psychopharmacology (Berl) 4, 389–395. doi: 10.1007/s002130050360
Kumari, V., Mulligan, O. F., Cotter, P. A., Poon, L., Toone, B. K., Checkley, S. A., et al. (1998). Effects of single oral administrations of haloperidol and d-amphetamine on prepulse inhibition of the acoustic startle reflex in healthy male volunteers. Behav. Pharmacol. 7, 567–576. doi: 10.1097/00008877-199811000-00012
Leonard, S., Gault, J., Hopkins, J., Logel, J., Vianzon, R., Short, M., et al. (2002). Association of promoter variants in the alpha7 nicotinic acetylcholine receptor subunit gene with an inhibitory deficit found in schizophrenia. Arch. Gen. Psychiatry. 12, 1085–1096. doi: 10.1001/archpsyc.59.12.1085
Li, W., Mao, Z., Bo, Q., Sun, Y., Wang, Z., and Wang, C. (2021). Prepulse inhibition in first-degree relatives of schizophrenia patients: A systematic review. Early Interv. Psychiatry 2021, 3652–3661. doi: 10.1111/eip.13003
Light, G. A., and Braff, D. L. (1999). Human and animal studies of schizophrenia-related gating deficits. Curr. Psychiatry Rep. 1, 31–40. doi: 10.1007/s11920-999-0008-y
Light, G. A., Malaspina, D., Geyer, M. A., Luber, B. M., Coleman, E. A., and Sackeim, H. A. (1999). Amphetamine disrupts P50 suppression in normal subjects. Biol. Psychiatry 7, 990–996. doi: 10.1016/s0006-3223(99)00034-7
Lingenhöhl, K., and Friauf, E. (1994). Giant neurons in the rat reticular formation: a sensorimotor interface in the elementary acoustic startle circuit? J. Neurosci. 14, 1176–1194. doi: 10.1523/JNEUROSCI.14-03-01176.1994
Liu, X., Hong, X., Chan, R. C., Kong, F., Peng, Z., Wan, X., et al. (2013). Association study of polymorphisms in the alpha 7 nicotinic acetylcholine receptor subunit and catechol-o-methyl transferase genes with sensory gating in first-episode schizophrenia. Psychiatry Res. 3, 431–348. doi: 10.1016/j.psychres.2013.03.027
Lu, B. Y., Martin, K. E., Edgar, J. C., Smith, A. K., Lewis, S. F., Escamilla, M. A., et al. (2007). Effect of catechol O-methyltransferase val(158)met polymorphism on the p50 gating endophenotype in schizophrenia. Biol. Psychiatry 7, 822–825. doi: 10.1016/j.biopsych.2006.11.030
Ludewig, K., and Vollenweider, F. X. (2002). Impaired sensorimotor gating in schizophrenia with deficit and with nondeficit syndrome. Swiss Med Wkly. 132, 159–165.
Majic, T., Rentzsch, J., Gudlowski, Y., Ehrlich, S., Juckel, G., Sander, T., et al. (2011). COMT Val108/158Met genotype modulates human sensory gating. Neuroimage. 2, 818–824. doi: 10.1016/j.neuroimage.2010.12.031
Mao, Q., Tan, Y. L., Luo, X. G., Tian, L., Wang, Z. R., Tan, S. P., et al. (2016). Association of catechol-O-methyltransferase Val(108/158) Met genetic polymorphism with schizophrenia, P50 sensory gating, and negative symptoms in a Chinese population. Psychiatry Res. 242, 271–276. doi: 10.1016/j.psychres.2016.04.029
Mao, Z., Bo, Q., Li, W., Wang, Z., Ma, X., and Wang, C. (2019). Prepulse inhibition in patients with bipolar disorder: a systematic review and meta-analysis. BMC Psychiatry 1:282. doi: 10.1186/s12888-019-2271-8
Markett, S. A., Montag, C., and Reuter, M. (2010). The association between dopamine DRD2 polymorphisms and working memory capacity is modulated by a functional polymorphism on the nicotinic receptor gene CHRNA4. J. Cogn. Neurosci. 9, 1944–1954. doi: 10.1162/jocn.2009.21354
Martin-Iverson, M. T. (1999). Effects of bromocriptine and haloperidol on prepulse inhibition of the acoustic startle response in man. J. Psychopharmacol. 2, 198–199. doi: 10.1177/026988119901300215
Mena, A., López, S., Ruiz-Salas, J. C., Fernández, A., Pérez-Díaz, F. J., and López, J. C. (2021). Sensitivity to amphetamine in prepulse inhibition response requires a mature medial prefrontal cortex. Behav. Neurosci. 1, 32–38. doi: 10.1037/bne0000458
Millar, A., Smith, D., Choueiry, J., Fisher, D., Albert, P., and Knott, V. (2011). The moderating role of the dopamine transporter 1 gene on P50 sensory gating and its modulation by nicotine. Neuroscience 180, 148–156. doi: 10.1016/j.neuroscience.2011.02.008
Montag, C., Hartmann, P., Merz, M., Burk, C., and Reuter, M. (2007). D2 receptor density and prepulse inhibition in humans: negative findings from a molecular genetic approach. Behav. Brain Res. 2, 428–432. doi: 10.1016/j.bbr.2007.10.006
Naysmith, L. F., Kumari, V., and Williams, S. C. R. (2021). Neural mapping of prepulse-induced startle reflex modulation as indices of sensory information processing in healthy and clinical populations: A systematic review. Hum. Brain Mapp. 16, 5495–5518. doi: 10.1002/hbm.25631
Oliveras, I., Sánchez-González, A., Sampedro-Viana, D., Piludu, M. A., Río-Alamos, C., Giorgi, O., et al. (2017). Differential effects of antipsychotic and propsychotic drugs on prepulse inhibition and locomotor activity in Roman high- (RHA) and low-avoidance (RLA) rats. Psychopharmacology 6, 957–975. doi: 10.1007/s00213-017-4534-84
Oranje, B., Gispen-de Wied, C. C., Westenberg, H. G., Kemner, C., Verbaten, M. N., and Kahn, R. S. (2004b). Increasing dopaminergic activity: effects of L-dopa and bromocriptine on human sensory gating. J. Psychopharmacol. 3, 388–394. doi: 10.1177/026988110401800310
Oranje, B., Kahn, R. S., Kemner, C., and Verbaten, M. N. (2004a). Modulating sensorimotor gating in healthy volunteers: the effects of desipramine and haloperidol. Psychiatry Res. 3, 195–205. doi: 10.1016/j.psychres.2004.04.002
Perry, W., Minassian, A., Feifel, D., and Braff, D. L. (2001). Sensorimotor gating deficits in bipolar disorder patients with acute psychotic mania. Biol. Psychiatry. 50, 418–424. doi: 10.1016/s0006-3223(01)01184-2
Petrovsky, N., Quednow, B. B., Ettinger, U., Schmechtig, A., Mössner, R., Collier, D. A., et al. (2010). Sensorimotor gating is associated with CHRNA3 polymorphisms in schizophrenia and healthy volunteers. Neuropsychopharmacology 7, 1429–1439. doi: 10.1038/npp.2010.12
Postma, P., Gray, J. A., Sharma, T., Geyer, M., Mehrotra, R., Das, M., et al. (2006). A behavioural and functional neuroimaging investigation into the effects of nicotine on sensorimotor gating in healthy subjects and persons with schizophrenia. Psychopharmacology (Berl) 3–4, 589–599. doi: 10.1007/s00213-006-0307-5
Prager, E. M., and Plotkin, J. L. (2019). Compartmental function and modulation of the striatum. J. Neurosci. Res. 12, 1503–1514. doi: 10.1002/jnr.24522
Quednow, B. B., Ejebem, K., Wagner, M., Giakoumaki, S. G., Bitsios, P., Kumari, V., et al. (2018). Meta-analysis on the association between genetic polymorphisms and prepulse inhibition of the acoustic startle response. Schizophr. Res. 198, 52–59. doi: 10.1016/j.schres.2017.12.011
Quednow, B. B., Schmechtig, A., Ettinger, U., Petrovsky, N., Collier, D. A., Vollenweider, F. X., et al. (2009). Sensorimotor gating depends on polymorphisms of the serotonin-2A receptor and catechol-O-methyltransferase, but not on neuregulin-1 Arg38Gln genotype: a replication study. Biol. Psychiatry 6, 614–620. doi: 10.1016/j.biopsych.2009.05.007
Quednow, B. B., Wagner, M., Mössner, R., Maier, W., and Kühn, K. U. (2010). Sensorimotor gating of schizophrenia patients depends on Catechol O-methyltransferase Val158Met polymorphism. Schizophr. Bull. 2, 341–346. doi: 10.1093/schbul/sbn088
Rabin, R. A., Sacco, K. A., and George, T. P. (2009). Correlation of prepulse inhibition and Wisconsin Card Sorting Test in schizophrenia and controls: Effects of smoking status. Schizophrenia Res. 1–3, 91–97. doi: 10.1016/j.schres.2009.07.00
Raupp-Barcaro, I. F. M., da Silva Dias, I. C., Meyer, E., Vieira, J. C. F., da Silva Pereira, G., Petkowicz, A. R., et al. (2021). Involvement of dopamine D2 and glutamate NMDA receptors in the antidepressant-like effect of amantadine in mice. Behav. Brain Res. 413:113443. doi: 10.1016/j.bbr.2021.113443
Raux, G., Bonnet-Brilhault, F., Louchart, S., Houy, E., Gantier, R., Levillain, D., et al. (2002). The−2 bp deletion in exon 6 of the 'alpha 7-like' nicotinic receptor subunit gene is a risk factor for the P50 sensory gating deficit. Mol. Psychiatry 9, 1006–1011. doi: 10.1038/sj.mp.4001140
Roussos, P., Giakoumaki, S. G., and Bitsios, P. (2008a). The dopamine D(3) receptor Ser9Gly polymorphism modulates prepulse inhibition of the acoustic startle reflex. Biol. Psychiatry 3, 235–240. doi: 10.1016/j.biopsych.2008a.01.020
Roussos, P., Giakoumaki, S. G., Rogdaki, M., Pavlakis, S., Frangou, S., and Bitsios, P. (2008b). Prepulse inhibition of the startle reflex depends on the catechol O-methyltransferase Val158Met gene polymorphism. Psychol. Med. 11, 1651–1658. doi: 10.1017/S0033291708002912
Roussos, P., Giakoumaki, S. G., Zouraraki, C., Fullard, J. F., Karagiorga, V. E., Tsapakis, E. M., et al. (2016). The relationship of common risk variants and polygenic risk for schizophrenia to sensorimotor gating. Biol. Psychiatry. 79, 988–996. doi: 10.1016/j.biopsych.2015.06.019
Rovn, R., Besterciov,á, D., and Riečansk,ý, I. (2020). Genetic determinants of gating functions: do we get closer to understanding schizophrenia etiopathogenesis? Front. Psychiatry. 11:550225. doi: 10.3389/fpsyt.2020.550225
Sánchez-Morla, E. M., Mateo, J., Aparicio, A., García-Jiménez, M. Á., Jiménez, E., and Santos, J. L. (2016). Prepulse inhibition in euthymic bipolar disorder patients in comparison with control subjects. Acta Psychiatr. Scand. 134, 350–359. doi: 10.1111/acps.12604
San-Martin, R., Castro, L. A., Menezes, P. R., Fraga, F. J., Simões, P. W., and Salum, C. (2020). Meta-analysis of sensorimotor gating deficits in patients with schizophrenia evaluated by prepulse inhibition test. Schizophr. Bull. 6, 1482–1497. doi: 10.1093/schbul/sbaa059
San-Martin, R., Zimiani, M. I., de Ávila, M. A. V., Shuhama, R., Del-Ben, C. M., Menezes, P. R., et al. (2022). Early schizophrenia and bipolar disorder patients display reduced neural prepulse inhibition. Brain Sci. 12:93. doi: 10.3390/brainsci12010093
Schellekens, A. F., Grootens, K. P., Neef, C., Movig, K. L., Buitelaar, J. K., Ellenbroek, B., et al. (2010). Effect of apomorphine on cognitive performance and sensorimotor gating in humans. Psychopharmacology (Berl) 207, 559–569. doi: 10.1007/s00213-009-1686-1
Shaikh, M., Hall, M. H., Schulze, K., Dutt, A., Walshe, M., Williams, I., et al. (2011). Do COMT, BDNF and NRG1 polymorphisms influence P50 sensory gating in psychosis? Psychol. Med. 41, 263–276. doi: 10.1017/S003329170999239X
Sharma, S., Kim, L. H., Mayr, K. A., Elliott, D. A., and Whelan, P. J. (2018). Parallel descending dopaminergic connectivity of A13 cells to the brainstem locomotor centers. Sci. Rep. 8, 7972. doi: 10.1038/s41598-018-25908-5
Shi, J., Wang, Z., Tan, Y., Fan, H., An, H., Zuo, L., et al. (2016). CHRNA4 was associated with prepulse inhibition of schizophrenia in Chinese: a pilot study. Cogn. Neuropsychiatry. 21, 156–167. doi: 10.1080/13546805.2016.1155437
Smith, D. M., Fisher, D., Blier, P., Illivitsky, V., and Knott, V. (2015). The separate and combined effects of monoamine oxidase inhibition and nicotine on P50 sensory gating. Psychopharmacology (Berl) 232, 1911–1920. doi: 10.1007/s00213-014-3823-8
Song, L., Chen, X., Chen, M., Tang, Y., Wang, J., Zhang, M., et al. (2014). Differences in P50 and prepulse inhibition of the startle reflex between male smokers and non-smokers with first episode schizophrenia without medical treatment. Chin. Med. J. 127, 1651–1655
Storozheva, Z. I., Kirenskaya, A. V., Bochkarev, V. K., and Ilushina, E. A. (2019). Effects of the Val158Met polymorphism of the catechol-o-methyltransferase gene on measures of sensory gating in health and schizophrenia. Neurosci. Behav. Physiol. 49, 595–602. doi: 10.1007/s11055-019-00775-3
Storozheva, Z. I., Kirenskaya, A. V., and Proshin, A. T. (2015). The neuromediator mechanisms of the cognitive deficit in schizophrenia. Neurochem. J. 9, 186–200. doi: 10.1134/S1819712415030095
Swerdlow, N. R. (2013). Update: studies of prepulse inhibition of startle, with particular relevance to the pathophysiology or treatment of Tourette Syndrome. Neurosci. Biobehav. Rev. 37, 1150–1156. doi: 10.1016/j.neubiorev.2012.09.002
Swerdlow, N. R., Bhakta, S. G., Talledo, J. A., Franz, D. M., Hughes, E. L., Rana, B. K., et al. (2018). Effects of amphetamine on sensorimotor gating and neurocognition in antipsychotic-medicated schizophrenia patients. Neuropsychopharmacology 43, 708–717. doi: 10.1038/npp.2017.285
Swerdlow, N. R., Braff, D. L., and Geyer, M. A. (2000). Animal models of deficient sensorimotor gating: what we know, what we think we know, and what we hope to know soon. Behav. Pharmacol. 11, 185–204. doi: 10.1097/00008877-200006000-00002
Swerdlow, N. R., Eastvold, A., Karban, B., Ploum, Y., Stephany, N., Geyer, M. A., et al. (2002a). Dopamine agonist effects on startle and sensorimotor gating in normal male subjects: time course studies. Psychopharmacology (Berl) 161, 189–201. doi: 10.1007/s00213-002-1040-3
Swerdlow, N. R., Gur, R. E., and Braff, D. L. (2015). Consortium on the genetics of schizophrenia (COGS) assessment of endophenotypes for schizophrenia: an introduction to this special issue of schizophrenia research. Schizophr. Res. 163:9–16. doi: 10.1016/j.schres.2014.09.047
Swerdlow, N. R., Paulsen, J., Braff, D. L., Butters, N., Geyer, M. A., and Swenson, M. R. (1995). Impaired prepulse inhibition of acoustic and tactile startle response in patients with Huntington's disease. J. Neurol. Neurosurg. Psychiatry 58, 192–200. doi: 10.1136/jnnp.58.2.192
Swerdlow, N. R., Stephany, N., Shoemaker, J. M., Ross, L., Wasserman, L. C., Talledo, J., et al. (2002b). Effects of amantadine and bromocriptine on startle and sensorimotor gating: parametric studies and cross-species comparisons. Psychopharmacology (Berl) 164, 82–92. doi: 10.1007/s00213-002-1172-5
Swerdlow, N. R., Stephany, N., Wasserman, L. C., Talledo, J., Shoemaker, J., and Auerbach, P. P. (2003). Amphetamine effects on prepulse inhibition across-species: replication and parametric extension. Neuropsychopharmacology 28, 640–650. doi: 10.1038/sj.npp.1300086
Swerdlow, N. R., Tarasenko, M., Bhakta, S. G., Talledo, J., Alvarez, A. I., Hughes, E. L., et al. (2017). Amphetamine enhances gains in auditory discrimination training in adult schizophrenia patients. Schizophr. Bull. 43, 872–880. doi: 10.1093/schbul/sbw148
Swerdlow, N. R., Weber, M., Qu, Y., Light, G. A., and Braff, D. L. (2008). Realistic expectations of prepulse inhibition in translational models for schizophrenia research. Psychopharmacology (Berl) 199, 331–388. doi: 10.1007/s00213-008-1072-4
Talledo, J. A., Sutherland Owens, A. N., Schortinghuis, T., and Swerdlow, N. R. (2009). Amphetamine effects on startle gating in normal women and female rats. Psychopharmacology (Berl) 204, 165–175. doi: 10.1007/s00213-008-1446-7
Tissari, A. H. (1988). Synaptosomal dopamine autoreceptors: sensitivity changes after in vitro and in vivo treatments. Pharmacol. Res. Commun. 20, 1001–1011. doi: 10.1016/s0031-6989(88)80128-0
Tissari, A. H., and Lillgäls, M. S. (1993). Reduction of dopamine synthesis inhibition by dopamine autoreceptor activation in striatal synaptosomes with in vivo reserpine administration. J. Neurochem. 61, 231–238. doi: 10.1111/j.1471-4159.1993.tb03559.x
Togay, B., Çikrikçil,i, U., Bayraktaroglu, Z., Uslu, A., Noyan, H., and Üçok, A. (2020). Lower prepulse inhibition in clinical high-risk groups but not in familial risk groups for psychosis compared with healthy controls. Early Interv. Psychiatry 14, 196–202. doi: 10.1111/eip.12845
Toyomaki, A., Hashimoto, N., Kako, Y., Tomimatsu, Y., Koyama, T., and Kusumi, I. (2015). Different P50 sensory gating measures reflect different cognitive dysfunctions in schizophrenia. Schizophr. Res. Cogn. 3, 166–169. doi: 10.1016/j.scog.2015.07.002
Turetsky, B. I., Calkins, M. E., Light, G. A., Olincy, A., Radant, A. D., and Swerdlow, N. R. (2007). Neurophysiological endophenotypes of schizophrenia: the viability of selected candidate measures. Schizophr. Bull. 33, 69–94. doi: 10.1093/schbul/sbl060
Wang, Z. R., Tan, Y. L., Yang, F. D., Zhang, W. F., Zou, Y. Z., Tan, S. P., et al. (2013). Impaired prepulse inhibition of acoustic startle in Chinese patients with first-episode, medication-naïve schizophrenia. Chin. Med. J. 126, 526–531
Whitton, A. E., Lewandowski, K. E., and Hall, M. H. (2021). Smoking as a common modulator of sensory gating and reward learning in individuals with psychotic disorders. Brain Sci. 11:1581. doi: 10.3390/brainsci11121581
Williams, T. J., Nuechterlein, K. H., Subotnik, K. L., and Yee, C. M. (2011). Distinct neural generators of sensory gating in schizophrenia. Psychophysiology 48, 470–478. doi: 10.1111/j.1469-8986.2010.01119.x
Winterer, G., Musso, F., Konrad, A., Vucurevic, G., Stoeter, P., Sander, T., et al. (2007). Association of attentional network function with exon 5 variations of the CHRNA4 gene. Hum. Mol. Genet. 16, 2165–2174. doi: 10.1093/hmg/ddm168
Witten, L., Bastlund, J. F., Glenthoj, B. Y., Bundgaard, C., Steiniger-Brach, B., Mork, A., et al. (2016). Comparing pharmacological modulation of sensory gating in healthy humans and rats: the effects of reboxetine and haloperidol. Neuropsychopharmacology 41, 638–645. doi: 10.1038/npp.2015.194
Wu, C., Ding, Y., Chen, B., Gao, Y., Wang, Q., Wu, Z., et al. (2019). Both Val158Met polymorphism of catechol-o-methyltransferase gene and menstrual cycle affect prepulse inhibition but not attentional modulation of prepulse inhibition in younger-adult females. Neuroscience 404, 396–406. doi: 10.1016/j.neuroscience.2019.02.001
Wynn, J. K., Green, M. F., Sprock, J., Light, G. A., Widmark, C., Reist, C., et al. (2007). Effects of olanzapine, risperidone and haloperidol on prepulse inhibition in schizophrenia patients: a double-blind, randomized controlled trial. Schizophr. Res. 95, 134–142. doi: 10.1016/j.schres.2007.05.039
Keywords: dopaminergic and cholinergic mechanisms, prepulse inhibition, sensorimotor gating, sensory gating, schizophrenia
Citation: Proshin AT (2022) Comparative Analysis of Dopaminergic and Cholinergic Mechanisms of Sensory and Sensorimotor Gating in Healthy Individuals and in Patients With Schizophrenia. Front. Behav. Neurosci. 16:887312. doi: 10.3389/fnbeh.2022.887312
Received: 01 March 2022; Accepted: 01 June 2022;
Published: 30 June 2022.
Edited by:
Zinaida Storozheva, Ministry of Health, RussiaReviewed by:
Samanta Rodrigues, Federal University of São Paulo, BrazilCopyright © 2022 Proshin. This is an open-access article distributed under the terms of the Creative Commons Attribution License (CC BY). The use, distribution or reproduction in other forums is permitted, provided the original author(s) and the copyright owner(s) are credited and that the original publication in this journal is cited, in accordance with accepted academic practice. No use, distribution or reproduction is permitted which does not comply with these terms.
*Correspondence: Andrey T. Proshin, cHJvc2hpbl9hdEBtYWlsLnJ1
Disclaimer: All claims expressed in this article are solely those of the authors and do not necessarily represent those of their affiliated organizations, or those of the publisher, the editors and the reviewers. Any product that may be evaluated in this article or claim that may be made by its manufacturer is not guaranteed or endorsed by the publisher.
Research integrity at Frontiers
Learn more about the work of our research integrity team to safeguard the quality of each article we publish.