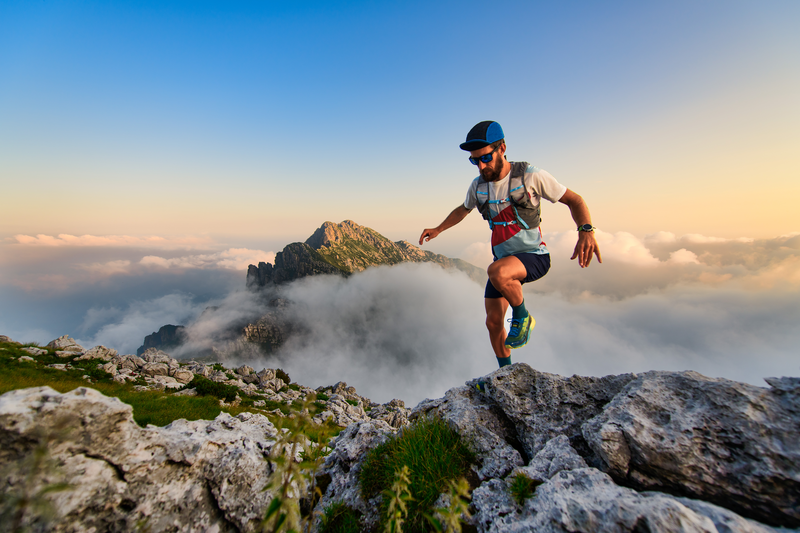
94% of researchers rate our articles as excellent or good
Learn more about the work of our research integrity team to safeguard the quality of each article we publish.
Find out more
MINI REVIEW article
Front. Behav. Neurosci. , 10 November 2022
Sec. Learning and Memory
Volume 16 - 2022 | https://doi.org/10.3389/fnbeh.2022.1041929
This article is part of the Research Topic Dopaminergic Control of Experience Encoding, Memory and Cognition View all 9 articles
Associative aversive learning enables animals to predict and avoid threats and thus is critical for survival and adaptive behavior. Anxiety disorders are characterized with deficits in normal aversive learning mechanisms and hence understanding the neural circuits underlying aversive learning and memory has high clinical relevance. Recent studies have revealed the dopamine system as one of the key modulators of aversive learning. In this review, we highlight recent advances that provide insights into how distinct dopaminergic circuits contribute to aversive learning and memory.
The ability to learn that certain stimuli and situations are associated with aversive outcomes helps animals to predict and avoid danger and hence is crucial for survival. In the laboratory, associative aversive learning is most commonly studied in rodents as well as humans using Pavlovian fear conditioning (reviewed in Duvarci and Pare, 2014). In this form of learning, an initially neutral stimulus (conditioned stimulus, CS) typically a tone is paired in time with an aversive unconditioned stimulus (US), such as a mild electrical foot shock. As the CS-US association is formed, the CS acquires the ability to elicit fear responses that are associated with the US (such as behavioral freezing) so that it can elicit conditioned fear responses when later presented alone. Much evidence indicates that anxiety disorders, such as post-traumatic stress disorder (PTSD), panic disorder and phobias, result from dysregulation of normal fear learning mechanisms (Lüthi and Lüscher, 2014; Duits et al., 2015; Craske et al., 2017), and thus understanding neural mechanisms underlying aversive learning has high clinical relevance. A considerable body of research has revealed that aversive learning and memory are mediated by a distributed network of brain structures including mainly the amygdala, medial prefrontal cortex (mPFC) and hippocampus (Duvarci and Pare, 2014; Herry and Johansen, 2014; Tovote et al., 2015; Ressler and Maren, 2019).
Recent lines of research further revealed the dopamine (DA) system as a critical regulator of aversive learning and memory. DA is a neuromodulator vitally involved in a wide range of functions including motor behavior, motivation, reward learning, cognition and aversion. DA neurons are mainly located in the ventral tegmental area (VTA) and substantia nigra (SN), but are also found in the hypothalamus, periaqueductal gray/dorsal raphe (PAG/DR) as well as the retrorubral field. DAergic transmission is mediated by metabotropic DA receptors that can be classified into two main types with the DA D1-type receptors (Gs-coupled) comprised of D1 and D5 and the DA D2-type receptors (Gi-coupled) comprised of D2, D3, and D4 subtypes (Missale et al., 1998). Although the crucial role DA neurons play in reward learning is well-established (Schultz, 2016), the role DA plays in aversive learning has more recently begun to be elucidated. Importantly, DA deficient mice exhibit impaired fear conditioning indicating the critical role DA plays in aversive learning, and enhancing DAergic transmission in these mice by systemic application of the DA precursor L-DOPA or viral-mediated restoration of DA synthesis restores fear learning (Fadok et al., 2009). Pharmacological antagonism and receptor knockout (KO) studies further demonstrate that both D1- and D2-type receptors are necessary for aversive learning (Nader and LeDoux, 1999; Greba and Kokkinidis, 2000; Inoue et al., 2000; Fadok et al., 2009). Moreover, disruption of phasic firing in DA neurons by inactivating NMDA receptors selectively in DA neurons resulted in impaired aversive learning (Zweifel et al., 2009, 2011). In line with these results, some midbrain DA neurons exhibit phasic activation in response to aversive stimuli as well as to cues predicting such stimuli (Guarraci and Kapp, 1999; Brischoux et al., 2009; Matsumoto and Hikosaka, 2009; Mileykovskiy and Morales, 2011; Zweifel et al., 2011; Jo et al., 2018; Salinas-Hernández et al., 2018). Together, these findings highlight the role of DA as a crucial neuromodulator for aversive learning.
It is important to note here that the midbrain DA system is composed of functionally distinct and mostly non-overlapping subpopulations of DA neurons each of which projects mainly to a single brain region (Lammel et al., 2008; Roeper, 2013; Beier et al., 2015; Lerner et al., 2015; Menegas et al., 2015). In particular, DA neurons projecting to brain structures that constitute the brain’s fear circuitry, such as the amygdala and the mPFC, have been implicated in aversion and aversive learning. In this short review, we highlight recent findings that have revealed the DAergic circuits underlying associative aversive learning. We mainly focus our discussion on cued fear conditioning in rodents where considerable progress has been made. Recent studies have also revealed DA as a critical regulator of fear extinction. However, in this review, we will not discuss fear extinction but rather refer the reader to prior reviews (Abraham et al., 2014; Salinas-Hernández and Duvarci, 2021). We begin by discussing how DA exerts its effects on the different components of the amygdala circuitry to regulate aversive learning and memory. We next focus on the role of DA input to the mPFC in mediating aversion and aversive learning. Finally, we discuss recent studies demonstrating how DA projections to different subregions of the striatum control aversive processes as well as the potential role DA plays in striatal circuits in mediating aversive learning.
The amygdala is a key structure underlying aversive learning and memory formation. Particularly, within the amygdala circuitry, the basolateral amygdala (BLA), consisting of the lateral and basal nuclei, the central nucleus of the amygdala (CEA) and the intercalated cells of the amygdala (ITCs) are the critical nuclei required for acquisition, consolidation and expression of aversive memories (Figure 1; Johansen et al., 2011; Duvarci and Pare, 2014; Herry and Johansen, 2014; Tovote et al., 2015; Ressler and Maren, 2019). Both D1- and D2-type DA receptors are expressed abundantly in the amygdala (Meador-Woodruff et al., 1991) and early pharmacological studies have established that both DA receptor types in the amygdala are critically involved in aversive learning (Pezze and Feldon, 2004).
Figure 1. Dopaminergic circuits underlying aversive learning and memory. Schematic of the seven major DAergic projections involved in aversive learning are shown. BLA, basolateral amygdala; CEA, central nucleus of the amygdala; D1R, DA D1 receptors; D2R, DA D2 receptors; ITCs, intercalated cells; LC, locus coeruleus; mPFC, medial prefrontal cortex; PAG/DR, periaqueductal gray/dorsal raphe; SNL, substantia nigra lateralis; TS, tail of the striatum; v-NAc, ventral nucleus accumbens; VTA, ventral tegmental area. VTA DA projection to BLA encodes salience of stimuli during associative learning. Asterisk (*) indicate a possible DAergic projection to lateral ITCs in mediating disinhibition of BLA and dorsomedial ITCs during aversive learning. The role of this projection in aversive learning remains to be tested. CEA receives DA input from both VTA and PAG/DR. Whereas, PAG/DR DA projections encode an aversive prediction error signal to drive aversive learning, VTA DA projections mediate fear discrimination. Asterisk (*) indicate a possible scenario in which PAG/DR and VTA DA neurons selectively innervate D1R- and D2R- expressing CEA neurons, respectively. Whether different subtypes of CEA neurons indeed receive separate inputs from these two different DA projections is an open question that remains to be investigated. DA input to mPFC originates from both VTA and LC. VTA DA projections are critical for expression of conditioned fear responses. In addition, this DA input to mPFC biases behavior toward aversion. On the other hand, the role of DA input from LC neurons in aversive learning needs to be investigated (?). VTA DA projections to v-NAc are implicated in aversion and likely encode motivational salience. Whether this DA projection is crucial for associative aversive learning remains to be tested. SNL DA neurons projecting to TS mediate threat avoidance. It will be important to investigate whether this DA projection is critically involved in associative aversive learning.
Studies administering D1 and D2 receptor agonists and antagonists in the BLA indicated the essential role these receptors play in BLA for aversive learning and memory (Lamont and Kokkinidis, 1998; Guarraci et al., 1999; Nader and LeDoux, 1999; Greba and Kokkinidis, 2000; Greba et al., 2001). Plasticity in the synapses carrying sensory information about the CS and the US underlies acquisition and consolidation of conditioned fear memories in BLA (Sigurdsson et al., 2007; Ressler and Maren, 2019). As a result, BLA neurons exhibit increased CS-evoked responses following fear conditioning (Quirk et al., 1995; Rogan et al., 1997; Collins and Pare, 2000; Repa et al., 2001; Goosens et al., 2003; Herry et al., 2008; Amano et al., 2011). Importantly, activation of DA receptors is critical for development of CS-evoked responses in BLA neurons (Rosenkranz and Grace, 2002). Consistent with this, DA has been shown to increase excitability of principal BLA neurons (Kröner et al., 2005; Yamamoto et al., 2007; Li et al., 2011). Other potential targets of DA in the BLA microcircuitry are GABAergic interneurons. Inhibitory circuits have been shown to play an essential role in gating aversive learning in BLA (Krabbe et al., 2018). In line with this, DA suppresses feedforward inhibition onto principal BLA neurons through D2-receptor mediated inhibition of fast-spiking interneurons, and as a result facilitates synaptic plasticity in the BLA (Bissière et al., 2003). Yet, there are several distinct subtypes of interneurons in the BLA that are critically involved in aversive learning (Krabbe et al., 2018). How DA regulates the activity of each of these interneuron subtypes is largely unknown and will be an important question for future research.
Recent findings indicate that the source of DA mediating aversive learning in BLA originates from DA neurons located in the VTA (Figure 1; Lutas et al., 2019; Tang et al., 2020). These studies demonstrated that VTA DA neurons projecting to BLA are activated by aversive USs as well as CSs associated with them (Lutas et al., 2019; Tang et al., 2020); furthermore, optogenetic inhibition of VTA DA projections to BLA impairs aversive learning (Tang et al., 2020). This raises the question of whether these BLA projecting VTA DA neurons are activated exclusively by aversive events and thus mediate only aversive learning. The BLA is also important for reward learning which is mediated by a subpopulation of neurons that is distinct from those mediating aversive learning, based on gene expression pattern and projection targets (Namburi et al., 2015; Kim J. et al., 2016). Intriguingly, individual VTA DA axons in the BLA are activated by both reward and aversive events as well as CSs predicting them (Lutas et al., 2019), suggesting that activation of the same DA neuron mediates both aversive and reward learning. These results suggest that BLA-projecting VTA DA neurons respond to the salience of stimuli, rather than their positive or negative value; and mediate associative learning in general for both negative and positive outcomes.
As mentioned earlier, the second crucial component of the amygdala circuitry underlying aversive learning and memory is the CEA. Much evidence indicates that plastic changes in the activity of CEA neurons mediate acquisition, consolidation, and expression of conditioned fear responses (Wilensky et al., 2006; Zimmerman et al., 2007; Ciocchi et al., 2010; Haubensak et al., 2010; Duvarci et al., 2011; Li et al., 2011; Fadok et al., 2018). In this review, we will not discuss the CEA microcircuitry and the different subtypes of CEA neurons but rather refer the reader to prior reviews (Duvarci and Pare, 2014; Fadok et al., 2018; Kong and Zweifel, 2021). Like BLA, CEA receives DA inputs originating from the VTA but also from the PAG/DR (Figure 1; Hasue and Shammah-Lagnado, 2002). Interestingly, recent studies investigating the function of these DA projections to CEA have revealed that these two distinct DA inputs play different roles in aversive learning and memory. Notably, PAG/DR DA neurons projecting to CEA encode a positive prediction error (PE) signal for aversive stimuli that drives associative aversive learning in CEA (Groessl et al., 2018). On the other hand, optogenetic inhibition of VTA DA projections to CEA does not have an effect on acquisition of conditioned fear suggesting that this DA projection is not necessary for associative aversive learning (Jo et al., 2018; Tang et al., 2020). VTA DA projections to CEA are instead critical for fear discrimination—that is discriminating cues associated with danger from cues associated with safety (Jo et al., 2018). Together, these findings indicate that these two distinct DA projections to CEA have different roles in aversive learning.
These observations raise the question of whether different subtypes of CEA neurons receive separate inputs from these different DA projections. Anatomical studies demonstrate that although D2 receptors are more abundant, both D1 and D2 receptors are expressed in CEA (Weiner et al., 1991; Perez de la Mora et al., 2012; McCullough et al., 2018). Moreover, expression of D1 and D2 receptors for the most part does not overlap in CEA neurons (McCullough et al., 2018). Interestingly, a recent study demonstrated that activation of D2 receptors in the CEA mediates fear discrimination (De Bundel et al., 2016). It would therefore be interesting to investigate whether VTA DA neurons innervate preferentially the D2 receptor-expressing neurons in CEA. On the other hand, PAG/DR DA neurons exhibit phasic activation to aversive USs as well as CSs associated with an aversive US (Groessl et al., 2018) suggesting that this DA input would activate preferentially the D1 receptor expressing CEA neurons during aversive learning. Tracing of monosynaptic inputs to genetically defined CEA neurons will be necessary to determine whether they receive differential inputs from VTA and PAG/DR DA neurons.
Another important target of DA in the amygdala circuitry are the ITCs which are a network of interconnected GABAergic cell groups located in the external and the intermediate capsules surrounding the BLA (Figure 1). The source of DA input to ITCs has largely remained elusive due to lack of molecular tools that can selectively target these neurons. However, recent studies suggest that DA input to ITCs originates from VTA/SN, similar to BLA (Ferrazzo et al., 2019; Aksoy-Aksel et al., 2021). D1, but not D2, receptors are abundantly expressed in the ITCs (Jacobsen et al., 2006). D1 receptors are typically Gs-coupled receptors and when activated they are expected to function in an excitatory fashion (Missale et al., 1998). Interestingly, D1 receptor signaling in ITCs is unusual in that activation of D1 receptors hyperpolarizes ITCs and inhibits these neurons (Marowsky et al., 2005; Mańko et al., 2011). ITCs are ideally positioned to gate sensory inputs to BLA as they exert feedforward and also feedback inhibition onto principal BLA neurons (Figure 1; Marowsky et al., 2005; Asede et al., 2015, 2021). DA inhibits these neurons and hence can facilitate synaptic plasticity in BLA (Marowsky et al., 2005). Furthermore, medial ITCs, located in the intermediate capsule, also gate the information flow from BLA to CEA. They receive excitatory input from BLA and send inhibitory projections to CEA, and thus mediate feedforward inhibition of CEA (Royer et al., 1999; Paré et al., 2003; Mańko et al., 2011; Gregoriou et al., 2019). In particular, plasticity in the dorsally located medial ITCs (dm-ITCs) has been implicated in aversive learning (Busti et al., 2011; Asede et al., 2015; Kwon et al., 2015). dm-ITCs are activated during acquisition and retrieval of conditioned fear (Busti et al., 2011; Hagihara et al., 2021). Both aversive USs and CSs predicting an aversive US excite dm-ITCs (Hagihara et al., 2021). Bidirectional chemogenetic manipulations during fear retrieval further demonstrated that activation of dm-ITCs is critical for expression of conditioned fear (Hagihara et al., 2021). Since DA inhibits ITCs, it is unlikely that dm-ITCs receive direct DA input during fear acquisition and expression. However, different ITC clusters are connected and exert feedforward inhibition onto each other (Royer et al., 1999, 2000; Paré et al., 2003; Asede et al., 2015, 2021). Notably, a recent study demonstrated that lateral ITCs (l-ITCs) located in the external capsule send feedforward inhibition onto dm-ITCs and DA application reduces this inhibition onto dm-ITCs (Aksoy-Aksel et al., 2021). These findings suggest that DA likely inhibits l-ITCs, dampening the feedforward inhibition these neurons exert on BLA and dm-ITCs, and thus indirectly activates BLA and dm-ITC neurons during aversive learning (Figure 1). Based on this scenario, DA neurons would be expected to differentially innervate and modulate distinct clusters of ITCs. How DA input regulates activity of each ITC cluster to mediate aversive learning is therefore an important question for future studies.
Considerable research has indicated that mPFC is a brain region critically involved in aversive learning. In particular, the prelimbic subregion of mPFC mediates expression of conditioned fear responses (Sotres-Bayon and Quirk, 2010; Giustino and Maren, 2015; Tovote et al., 2015; Rozeske and Herry, 2018). Early studies investigating the effect of aversive events and stressors in mPFC revealed that such events enhance prefrontal DA levels (Abercrombie et al., 1989; Sorg and Kalivas, 1993; Sullivan and Gratton, 1998). Importantly, an increase in DA release has also been observed in mPFC during fear conditioning (Wilkinson et al., 1998; Feenstra, 2000; Feenstra et al., 2001). Consistent with this, pharmacological studies demonstrated that activation of DA receptors in mPFC is required for expression of conditioned fear (Pezze et al., 2003; Jing Li et al., 2018). Together, these findings demonstrate the crucial role DA plays in aversive learning and memory in mPFC.
Recent studies reveal that DA input to mPFC originates from both VTA DA neurons and noradrenaline (NA) neurons located in the locus coeruleus (LC; Figure 1; Lammel et al., 2008; Beier et al., 2015; Devoto et al., 2020). Notably, mPFC-projecting VTA DA neurons have been implicated in aversive processing (Lammel et al., 2011, 2012; Gunaydin et al., 2014). Fiber photometry recordings of VTA DA terminals in mPFC demonstrate that these DA cells are selectively activated by aversive events, but not rewards (Kim C. K. et al., 2016). Consistent with this, a recent study revealed that DA increases the signal-to-noise ratio of responses in particular to aversive stimuli in mPFC. This study further showed that optogenetic activation of VTA DA terminals in mPFC biased behavioral responses to aversive stimuli in an associative stimulus competition task where aversive and reward CSs were presented simultaneously (Vander Weele et al., 2018). Together, these findings suggest that DA released from VTA DA neurons is a critical modulator of aversive processing and acts as a pro-aversive signal in the mPFC. On the other hand, the role that DA input from LC NA neurons plays during aversive learning has remained elusive (Figure 1). Whereas VTA DA neurons target the deep layers, LC NA neurons innervate the superficial layers of mPFC (Vander Weele et al., 2018). This differential innervation pattern suggests that DA released from LC NA neurons might mediate functions different from DA released from VTA. It will be important for future studies to examine the different roles these two distinct DA inputs play in mPFC during aversive learning.
Recent studies using the latest cell type- and projection-specific techniques have highlighted two regions of the striatum, the nucleus accumbens (NAc) and the posterior tail of the striatum (TS), as the striatal targets of aversion encoding DA neurons. As mentioned earlier, it is well-established that DA neurons encode reward PE signals to drive reward learning and in particular DA projections to NAc constitute the brain’s canonical reward circuitry (Wise, 2002; Schultz, 2016). More recent studies, however, have revealed that DA projections to NAc are not homogeneous, in that different subpopulations of DA neurons indeed project to distinct subregions of NAc and mediate different functions (Roeper, 2013). Earlier studies examining DA release suggested an involvement of DA in NAc during aversive processing (Young, 2004; Badrinarayan et al., 2012; Budygin et al., 2012; Oleson et al., 2012; Carelli and West, 2014). Two recent studies performing fiber photometry recordings of calcium transients in DA neuron terminals across different subregions of the NAc have revealed that in particular the ventral NAc (vNAc) projecting DA neurons mediate aversive processes (Figure 1; de Jong et al., 2019; Yuan et al., 2019). These two studies demonstrated that DA projections to vNAc are activated by aversive stimuli and CSs that predict them. However, similar to BLA projecting DA neurons, these DA terminals exhibit increased responses also to rewards (de Jong et al., 2019; Yuan et al., 2019) suggesting that they likely encode motivational salience rather than aversiveness per se. However, since recordings of bulk calcium transients from DA terminals in vNAc were performed in these studies, it is unclear whether the same DA neurons are activated by both rewards and aversive events. Studies recording the activity of vNAc-projecting DA neurons at single cell resolution will be necessary to address this question. Importantly, although vNAc-projecting DA neurons exhibit increased CS-evoked responses following pairing of the CS with an aversive US (de Jong et al., 2019), whether this activity is indeed required for acquisition and expression of associative aversive memories is not known. Causal manipulations investigating the necessity of DA projections to vNAc during aversive learning will be crucial to address this question.
The second striatal region that has lately been implicated in aversive processing is the posterior tail of the dorsal striatum (TS). Earlier studies investigating the role of dorsal striatum (DS) and DA projections to DS have particularly implicated dorsolateral striatum (DLS) in aversive learning. Notably, DLS-projecting DA neurons have been shown to be activated by aversive stimuli (Lerner et al., 2015). However, the causal contribution of DS-projecting DA neurons in aversive learning has remained elusive. For more detailed information on the role of DS in aversion, the reader is referred to prior reviews (Stanley et al., 2021). In this review, we will concentrate on DA projections to TS (Figure 1) where recent progress has been made. Indeed, recent studies have identified a unique subpopulation of DA neurons that project to the TS, located in the most lateral part of SN (SNL) and which exhibit a distinct input-output organization (Menegas et al., 2015). These DA neurons respond particularly strongly to novel and aversive stimuli, but only weakly to rewards. Consistent with this, they encode an aversive PE rather than a reward PE signal and exhibit CS-evoked responses following pairing of the CS with an aversive US (Menegas et al., 2017, 2018). Selective ablation or activation of these DA neurons further demonstrates that they are involved in threat avoidance (Menegas et al., 2018). However, whether this DA projection is crucial for aversive learning is currently unknown. Considering these DA neurons have been demonstrated to encode an aversive PE signal (Menegas et al., 2017, 2018), it will be important to investigate whether they are critically involved in associative aversive learning.
Recent advances in cell type- and projection-specific strategies in recording and manipulating neuronal activity have led to considerable progress in understanding the role of defined DAergic circuits in aversive learning. The studies reviewed above provide insights into how DA neurons mediate different aspects of aversive processing through their projections to the amygdala, mPFC and the striatum. The main conclusions of these studies and outstanding questions are summarized in Figure 1. In the amygdala, DA projections to BLA encode salience of stimuli to mediate associative learning. However, how DA regulates the activity of BLA neurons, in particular the activity of different subtypes of inhibitory interneurons in mediating aversive learning is incompletely understood. Likewise, how DA input controls the activity of different ITC clusters during aversive learning has also remained elusive. An important outstanding question for future research is whether DA neurons differentially innervate distinct clusters of ITCs to mediate aversive learning. CEA receives two distinct DA inputs from VTA and PAG/DR which play different roles in aversive learning. Whereas projections from PAG/DR encode an aversive PE signal to drive associative aversive learning, projections from VTA mediate fear discrimination. Whether different subtypes of CEA neurons, based on their DA receptor expression, receive separate inputs from these two distinct DA projections is an open question remains to be investigated. In the mPFC, DA input from VTA is critical for expression of conditioned fear responses and furthermore biases behavior toward aversion. mPFC receives DA input also from LC but the role of this input during aversive learning is largely unknown. In the striatum, two striatal subregions have recently emerged as the targets of DA neurons mediating aversive processing. Whereas DA projections to vNAc are involved in aversion and likely encode motivational salience, DA projections to TS mediate threat avoidance. Yet, whether these DA projections to the striatum are crucial for associative aversive learning remains to be an important outstanding question for future studies. Overall, the findings summarized in this review highlight DA as a critical modulator of aversive learning. Understanding the key role DA plays in aversive learning has high clinical significance since dysregulation of aversive learning mechanisms is a hallmark of human anxiety disorders. In particular, elucidating how DA mediates different aspects of aversive processing through its actions in distinct brain circuits can help develop novel therapeutic strategies in the treatment of anxiety disorders.
DZ and SD wrote the manuscript. Both authors contributed to the article and approved the submitted version.
This work was supported by the Deutsche Forschungsgemeinschaft (DFG Grant DU 1433/5-1 to SD).
We thank Torfi Sigurdsson for helpful discussions and comments on the manuscript.
The authors declare that the research was conducted in the absence of any commercial or financial relationships that could be construed as a potential conflict of interest.
All claims expressed in this article are solely those of the authors and do not necessarily represent those of their affiliated organizations, or those of the publisher, the editors and the reviewers. Any product that may be evaluated in this article, or claim that may be made by its manufacturer, is not guaranteed or endorsed by the publisher.
Abercrombie, E. D., Keefe, K. A., DiFrischia, D. S., and Zigmond, M. J. (1989). Differential effect of stress on in vivo dopamine release in striatum, nucleus accumbens, and medial frontal cortex. J. Neurochem. 52, 1655–1658. doi: 10.1111/j.1471-4159.1989.tb09224.x
Abraham, A. D., Neve, K. A., and Lattal, K. M. (2014). Dopamine and extinction: A convergence of theory with fear and reward circuitry. Neurobiol. Learn. Mem. 108, 65–77. doi: 10.1016/j.nlm.2013.11.007
Aksoy-Aksel, A., Gall, A., Seewald, A., Ferraguti, F., and Ehrlich, I. (2021). Midbrain dopaminergic inputs gate amygdala intercalated cell clusters by distinct and cooperative mechanisms in male mice. Elife 10:e63708. doi: 10.7554/eLife.63708.sa2
Amano, T., Duvarci, S., Popa, D., and Paré, D. (2011). The fear circuit revisited: Contributions of the basal amygdala nuclei to conditioned fear. J. Neurosci. 31, 15481–15489. doi: 10.1523/JNEUROSCI.3410-11.2011
Asede, D., Bosch, D., Lüthi, A., Ferraguti, F., and Ehrlich, I. (2015). Sensory inputs to intercalated cells provide fear-learning modulated inhibition to the basolateral amygdala. Neuron 86, 541–554. doi: 10.1016/j.neuron.2015.03.008
Asede, D., Doddapaneni, D., Chavez, A., Okoh, J., Ali, S., Von-Walter, C., et al. (2021). Apical intercalated cell cluster: A distinct sensory regulator in the amygdala. Cell Rep. 35:109151. doi: 10.1016/j.celrep.2021.109151
Badrinarayan, A., Wescott, S. A., Vander Weele, C. M., Saunders, B. T., Couturier, B. E., Maren, S., et al. (2012). Aversive stimuli differentially modulate real-time dopamine transmission dynamics within the nucleus accumbens core and shell. J. Neurosci. 32, 15779–15790. doi: 10.1523/JNEUROSCI.3557-12.2012
Beier, K. T., Steinberg, E. E., DeLoach, K. E., Xie, S., Miyamichi, K., Schwarz, L., et al. (2015). Circuit architecture of VTA dopamine neurons revealed by systemic input-output mapping. Cell 162, 622–634. doi: 10.1016/j.cell.2015.07.015
Bissière, S., Humeau, Y., and Luthi, A. (2003). Dopamine gates LTP induction in lateral amygdala by suppressing feedforward inhibition. Nat. Neurosci. 6, 587–592. doi: 10.1038/nn1058
Brischoux, F., Chakraborty, S., Brierley, D. I., and Ungless, M. A. (2009). Phasic excitation of dopamine neurons in ventral VTA by noxious stimuli. Proc. Natl. Acad. Sci. U.S.A. 106, 4894–4899. doi: 10.1073/pnas.0811507106
Budygin, E. A., Park, J., Bass, C. E., Grinevich, V. P., Bonin, K. D., and Wightman, R. M. (2012). Aversive stimulus differentially triggers subsecond dopamine release in reward regions. Neuroscience 201, 331–337. doi: 10.1016/j.neuroscience.2011.10.056
Busti, D., Geracitano, R., Whittle, N., Dalezios, Y., Manko, M., Kaufmann, W., et al. (2011). Different fear states engage distinct networks within the intercalated cell clusters of the amygdala. J. Neurosci. 31, 5131–5144. doi: 10.1523/JNEUROSCI.6100-10.2011
Carelli, R. M., and West, E. A. (2014). When a good taste turns bad: Neural mechanisms underlying the emergence of negative affect and associated natural reward devaluation by cocaine. Neuropharmacology 76, 360–369. doi: 10.1016/j.neuropharm.2013.04.025
Ciocchi, S., Herry, C., Grenier, F., Wolff, S. B., Letzkus, J. J., Vlachos, I., et al. (2010). Encoding of conditioned fear in central amygdala inhibitory circuits. Nature 468, 277–282. doi: 10.1038/nature09559
Collins, D. R., and Pare, D. (2000). Differential fear conditioning induces reciprocal changes in the sensory responses of lateral amygdala neurons to the CS(+) and CS(-). Learn. Mem. 7, 97–103. doi: 10.1101/lm.7.2.97
Craske, M. G., Stein, M. B., Eley, T. C., Milad, M. R., Holmes, A., Rapee, R. M., et al. (2017). Anxiety disorders. Nat. Rev. Dis. Primers 3:17024. doi: 10.1038/nrdp.2017.24
De Bundel, D., Zussy, C., Espallergues, J., Gerfen, C. R., Girault, J. A., and Valjent, E. (2016). Dopamine D2 receptors gate generalization of conditioned threat responses through mTORC1 signaling in the extended amygdala. Mol. Psychiatry 21, 1545–1553. doi: 10.1038/mp.2015.210
de Jong, J. W., Afjei, S. A., Dorocic, I. P., Peck, J. R., Liu, C., Kim, C. K., et al. (2019). A neural circuit mechanism for encoding aversive stimuli in the mesolimbic dopamine system. Neuron 101, 133–151. doi: 10.1016/j.neuron.2018.11.005
Devoto, P., Sagheddu, C., Santoni, M., Flore, G., Saba, P., Pistis, M., et al. (2020). Noradrenergic source of dopamine assessed by microdialysis in the medial prefrontal cortex. Front. Pharmacol. 11:588160. doi: 10.3389/fphar.2020.588160
Duits, P., Cath, D. C., Lissek, S., Hox, J. J., Hamm, A. O., Engelhard, I. M., et al. (2015). Updated meta-analysis of classical fear conditioning in the anxiety disorders. Depress. Anxiety 32, 239–253. doi: 10.1002/da.22353
Duvarci, S., and Pare, D. (2014). Amygdala microcircuits controlling learned fear. Neuron 82, 966–980. doi: 10.1016/j.neuron.2014.04.042
Duvarci, S., Popa, D., and Paré, D. (2011). Central amygdala activity during fear conditioning. J. Neurosci. 31, 289–294.
Fadok, J. P., Dickerson, T. M., and Palmiter, R. D. (2009). Dopamine is necessary for cue-dependent fear conditioning. J. Neurosci. 29, 11089–11097. doi: 10.1523/JNEUROSCI.1616-09.2009
Fadok, J. P., Markovic, M., Tovote, P., and Lüthi, A. (2018). New perspectives on central amygdala function. Curr. Opin. Neurobiol. 49, 141–147.
Feenstra, M. G. (2000). Dopamine and noradrenaline release in the prefrontal cortex in relation to unconditioned and conditioned stress and reward. Prog. Brain Res. 126, 133–163. doi: 10.1016/S0079-6123(00)26012-3
Feenstra, M. G., Vogel, M., Botterblom, M. H., Joosten, R. N., and de Bruin, J. P. (2001). Dopamine and noradrenaline efflux in the rat prefrontal cortex after classical aversive conditioning to an auditory cue. Eur. J. Neurosci. 13, 1051–1054. doi: 10.1046/j.0953-816x.2001.01471.x
Ferrazzo, S., Gunduz-Cinar, O., Stefanova, N., Pollack, G. A., Holmes, A., Schmuckermair, C., et al. (2019). Increased anxiety-like behavior following circuit-specific catecholamine denervation in mice. Neurobiol. Dis. 125, 55–66. doi: 10.1016/j.nbd.2019.01.009
Giustino, T. F., and Maren, S. (2015). The role of the medial prefrontal cortex in the conditioning and extinction of fear. Front. Behav. Neurosci. 9:298. doi: 10.3389/fnbeh.2015.00298
Goosens, K. A., Hobin, J. A., and Maren, S. (2003). Auditory-evoked spike firing in the lateral amygdala and pavlovian fear conditioning: Mnemonic code or fear bias? Neuron 40, 1013–1022. doi: 10.1016/S0896-6273(03)00728-1
Greba, Q., and Kokkinidis, L. (2000). Peripheral and intraamygdalar administration of the dopamine D1 receptor antagonist SCH 23390 blocks fear-potentiated startle but not shock reactivity or the shock sensitization of acoustic startle. Behav. Neurosci. 114, 262–272. doi: 10.1037/0735-7044.114.2.262
Greba, Q., Gifkins, A., and Kokkinidis, L. (2001). Inhibition of amygdaloid dopamine D2 receptors impairs emotional learning measured with fear-potentiated startle. Brain Res. 899, 218–226. doi: 10.1016/s0006-8993(01)02243-0
Gregoriou, G. C., Kissiwaa, S. A., Patel, S. D., and Bagley, E. E. (2019). Dopamine and opioids inhibit synaptic outputs of the main island of the intercalated neurons of the amygdala. Eur. J. Neurosci. 50, 2065–2074. doi: 10.1111/ejn.14107
Groessl, F., Munsch, T., Meis, S., Griessner, J., Kaczanowska, J., Pliota, P., et al. (2018). Dorsal tegmental dopamine neurons gate associative learning of fear. Nat. Neurosci. 21, 952–962. doi: 10.1038/s41593-018-0174-5
Guarraci, F. A., Frohardt, R. J., and Kapp, B. S. (1999). Amygdaloid D1 dopamine receptor involvement in Pavlovian fear conditioning. Brain Res. 827, 28–40.
Guarraci, F. A., and Kapp, B. S. (1999). An electrophysiological characterization of ventral tegmental area dopaminergic neurons during differential pavlovian fear conditioning in the awake rabbit. Behav. Brain Res. 99, 169–179. doi: 10.1016/S0166-4328(98)00102-8
Gunaydin, L. A., Grosenick, L., Finkelstein, J. C., Kauvar, I. V., Fenno, L. E., Adhikari, A., et al. (2014). Natural neural projection dynamics underlying social behavior. Cell 157, 1535–1551. doi: 10.1016/j.cell.2014.05.017
Hagihara, K. M., Bukalo, O., Zeller, M., Aksoy-Aksel, A., Karalis, N., Limoges, A., et al. (2021). Intercalated amygdala clusters orchestrate a switch in fear state. Nature 594, 403–407. doi: 10.1038/s41586-021-03593-1
Hasue, R. H., and Shammah-Lagnado, S. J. (2002). Origin of the dopaminergic innervation of the central extended amygdala and accumbens shell: A combined retrograde tracing and immunohistochemical study in the rat. J. Comp. Neurol. 454, 15–33. doi: 10.1002/cne.10420
Haubensak, W., Kunwar, P. S., Cai, H., Ciocchi, S., Wall, N. R., Ponnusamy, R., et al. (2010). Genetic dissection of an amygdala microcircuit that gates conditioned fear. Nature 468, 270–276. doi: 10.1038/nature09553
Herry, C., and Johansen, J. P. (2014). Encoding of fear learning and memory in distributed neuronal circuits. Nat. Neurosci. 17, 1644–1654. doi: 10.1038/nn.3869
Herry, C., Ciocchi, S., Senn, V., Demmou, L., Müller, C., and Lüthi, A. (2008). Switching on and off fear by distinct neuronal circuits. Nature 454, 600–606. doi: 10.1038/nature07166
Inoue, T., Izumi, T., Maki, Y., Muraki, I., and Koyama, T. (2000). Effect of the dopamine D(1/5) antagonist SCH 23390 on the acquisition of conditioned fear. Pharmacol. Biochem. Behav. 66, 573–578. doi: 10.1016/S0091-3057(00)00254-9
Jacobsen, K. X., Höistad, M., Staines, W. A., and Fuxe, K. (2006). The distribution of dopamine D1 receptor and mu-opioid receptor 1 receptor immunoreactivities in the amygdala and interstitial nucleus of the posterior limb of the anterior commissure: Relationships to tyrosine hydroxylase and opioid peptide terminal systems. Neuroscience 141, 2007–2018. doi: 10.1016/j.neuroscience.2006.05.054
Jing Li, J., Szkudlarek, H., Renard, J., Hudson, R., Rushlow, W., and Laviolette, S. R. (2018). Fear memory recall potentiates opiate reward sensitivity through dissociable dopamine D1 versus D4 receptor-dependent memory mechanisms in the prefrontal cortex. J. Neurosci. 38, 4543–4555. doi: 10.1523/JNEUROSCI.3113-17.2018
Jo, Y. S., Heymann, G., and Zweifel, L. S. (2018). Dopamine neurons reflect the uncertainty in fear generalization. Neuron 100, 916–925. doi: 10.1016/j.neuron.2018.09.028
Johansen, J. P., Cain, C. K., Ostroff, L. E., and LeDoux, J. E. (2011). Molecular mechanisms of fear learning and memory. Cell 147, 509–524. doi: 10.1016/j.cell.2011.10.009
Kim, C. K., Yang, S. J., Pichamoorthy, N., Young, N. P., Kauvar, I., Jennings, J. H., et al. (2016). Simultaneous fast measurement of circuit dynamics at multiple sites across the mammalian brain. Nat. Methods 13, 325–328. doi: 10.1038/nmeth.3770
Kim, J., Pignatelli, M., Xu, S., Itohara, S., and Tonegawa, S. (2016). Antagonistic positive and negative neurons of the basolateral amygdala. Nat. Neurosci. 19, 1636–1646. doi: 10.1038/nn.4414
Kong, M. S., and Zweifel, L. S. (2021). Central amygdala circuits in valence and salience processing. Behav. Brain Res. 410:113355. doi: 10.1016/j.bbr.2021.113355
Krabbe, S., Gründemann, J., and Lüthi, A. (2018). Amygdala inhibitory circuits regulate associative fear conditioning. Biol. Psychiatry 83, 800–809. doi: 10.1016/j.biopsych.2017.10.006
Kröner, S., Rosenkranz, J. A., Grace, A. A., and Barrionuevo, G. J. (2005). Dopamine modulates excitability of basolateral amygdala neurons in vitro. J. Neurophysiol. 93, 1598–1610. doi: 10.1152/jn.00843.2004
Kwon, O. B., Lee, J. H., Kim, H. J., Lee, S., Lee, S., Jeong, M. J., et al. (2015). Dopamine regulation of amygdala inhibitory circuits for expression of learned fear. Neuron 88, 378–389. doi: 10.1016/j.neuron.2015.09.001
Lammel, S., Hetzel, A., Häckel, O., Jones, I., Liss, B., and Roeper, J. (2008). Unique properties of mesoprefrontal neurons within a dual mesocorticolimbic dopamine system. Neuron 57, 760–773. doi: 10.1016/j.neuron.2008.01.022
Lammel, S., Ion, D. I., Roeper, J., and Malenka, R. C. (2011). Projection-specific modulation of dopamine neuron synapses by aversive and rewarding stimuli. Neuron 70, 855–862. doi: 10.1016/j.neuron.2011.03.025
Lammel, S., Lim, B. K., Ran, C., Huang, K. W., Betley, M. J., Tye, K. M., et al. (2012). Input-specific control of reward and aversion in the ventral tegmental area. Nature 491, 212–217. doi: 10.1038/nature11527
Lamont, E. W., and Kokkinidis, L. (1998). Infusion of the dopamine D1 receptor antagonist SCH 23390 into the amygdala blocks fear expression in a potentiated startle paradigm. Brain Res. 795, 128–136. doi: 10.1016/s0006-8993(98)00281-9
Lerner, T. N., Shilyansky, C., Davidson, T. J., Evans, K. E., Beier, K. T., Zalocusky, K. A., et al. (2015). Intact-brain analyses reveal distinct information carried by SNc dopamine subcircuits. Cell 162, 635–647. doi: 10.1016/j.cell.2015.07.014
Li, C., Dabrowska, J., Hazra, R., and Rainnie, D. G. (2011). Synergistic activation of dopamine D1 and TrkB receptors mediate gain control of synaptic plasticity in the basolateral amygdala. PLoS One 6:e26065. doi: 10.1371/journal.pone.0026065
Lutas, A., Kucukdereli, H., Alturkistani, O., Carty, C., Sugden, A. U., Fernando, K., et al. (2019). State-specific gating of salient cues by midbrain dopaminergic input to basal amygdala. Nat. Neurosci. 22, 1820–1833. doi: 10.1038/s41593-019-0506-0
Lüthi, A., and Lüscher, C. (2014). Pathological circuit function underlying addiction and anxiety disorders. Nat. Neurosci. 17, 1635–1643. doi: 10.1038/nn.3849
Mańko, M., Geracitano, R., and Capogna, M. (2011). Functional connectivity of the main intercalated nucleus of the amygdala. J. Physiol. 15, 1911–1925. doi: 10.1113/jphysiol.2010.201475
Marowsky, A., Yanagawa, Y., Obata, K., and Vogt, K. E. (2005). A specialized subclass of interneurons mediates dopaminergic facilitation of amygdala function. Neuron 48, 1025–1037. doi: 10.1016/j.neuron.2005.10.029
Matsumoto, M., and Hikosaka, O. (2009). Two types of dopamine neurons distinctly convey positive and negative motivational signals. Nature 459, 837–841. doi: 10.1038/nature08028
McCullough, K. M., Daskalakis, N. P., Gafford, G., Morrison, F. G., and Ressler, K. J. (2018). Cell-type-specific interrogation of CeA Drd2 neurons to identify targets for pharmacological modulation of fear extinction. Transl. Psychiatry 8:164. doi: 10.1038/s41398-018-0190-y
Meador-Woodruff, J. H., Mansour, A., Healy, D. J., Kuehn, R., Zhou, Q. Y., Bunzow, J. R., et al. (1991). Comparison of the distributions of D1 and D2 dopamine receptor mRNAs in rat brain. Neuropsychopharmacology 5, 231–242.
Menegas, W., Akiti, K., Amo, R., Uchida, N., and Watabe-Uchida, M. (2018). Dopamine neurons projecting to the posterior striatum reinforce avoidance of threatening stimuli. Nat. Neurosci. 21, 1421–1430. doi: 10.1038/s41593-018-0222-1
Menegas, W., Babayan, B. M., Uchida, N., and Watabe-Uchida, M. (2017). Opposite initialization to novel cues in dopamine signaling in ventral and posterior striatum in mice. Elife 6:e21886. doi: 10.7554/eLife.21886
Menegas, W., Bergan, J. F., Ogawa, S. K., Isogai, Y., Venkataraju, K. U., Osten, P., et al. (2015). Dopamine neurons projecting to the posterior striatum form an anatomically distinct subclass. Elife 4:e10032. doi: 10.7554/eLife.10032.021
Mileykovskiy, B., and Morales, M. (2011). Duration of inhibition of ventral tegmental area dopamine neurons encodes a level of conditioned fear. J. Neurosci. 31, 7471–7476. doi: 10.1523/JNEUROSCI.5731-10.2011
Missale, C., Nash, S. R., Robinson, S. W., Jaber, M., and Caron, M. G. (1998). Dopamine receptors: From structure to function. Physiol. Rev. 78, 189–225. doi: 10.1152/physrev.1998.78.1.189
Nader, K., and LeDoux, J. (1999). The dopaminergic modulation of fear: Quinpirole impairs the recall of emotional memories in rats. Behav. Neurosci. 113, 152–165. doi: 10.1037/0735-7044.113.1.152
Namburi, P., Beyeler, A., Yorozu, S., Calhoon, G. G., Halbert, S. A., Wichmann, R., et al. (2015). A circuit mechanism for differentiating positive and negative associations. Nature 520, 675–678. doi: 10.1038/nature14366
Oleson, E. B., Gentry, R. N., Chioma, V. C., and Cheer, J. F. (2012). Subsecond dopamine release in the nucleus accumbens predicts conditioned punishment and its successful avoidance. J. Neurosci. 32, 14804–14808. doi: 10.1523/JNEUROSCI.3087-12.2012
Paré, D., Royer, S., Smith, Y., and Lang, E. J. (2003). Contextual inhibitory gating of impulse traffic in the the intra-amygdaloid network. Ann. N. Y. Acad. Sci. 985, 78–91. doi: 10.1111/j.1749-6632.2003.tb07073.x
Perez de la Mora, M., Gallegos-Cari, A., Crespo-Ramirez, M., Marcellino, D., Hansson, A. C., and Fuxe, K. (2012). Distribution of dopamine D(2)-like receptors in the rat amygdala and their role in the modulation of unconditioned fear and anxiety. Neuroscience 201, 252–266. doi: 10.1016/j.neuroscience.2011.10.045
Pezze, M. A., and Feldon, J. (2004). Mesolimbic dopaminergic pathways in fear conditioning. Prog. Neurobiol. 74, 301–320. doi: 10.1016/j.pneurobio.2004.09.004
Pezze, M. A., Bast, T., and Feldon, J. (2003). Significance of dopamine transmission in the rat medial prefrontal cortex for conditioned fear. Cereb. Cortex 13, 371–380. doi: 10.1093/cercor/13.4.371
Quirk, G. J., Repa, C., and LeDoux, J. E. (1995). Fear conditioning enhances short-latency auditory responses of lateral amygdala neurons: Parallel recordings in the freely behaving rat. Neuron 15, 1029–1039. doi: 10.1016/0896-6273(95)90092-6
Repa, J. C., Muller, J., Apergis, J., Desrochers, T. M., Zhou, Y., and LeDoux, J. E. (2001). Two different lateral amygdala cell populations contribute to the initiation and storage of memory. Nat. Neurosci. 4, 724–731. doi: 10.1038/89512
Ressler, R. L., and Maren, S. (2019). Synaptic encoding of fear memories in the amygdala. Curr. Opin. Neurobiol. 54, 54–59. doi: 10.1016/j.conb.2018.08.012
Roeper, J. (2013). Dissecting the diversity of midbrain dopamine neurons. Trends Neurosci. 36, 336–342. doi: 10.1016/j.tins.2013.03.003
Rogan, M. T., Staubli, U. V., and LeDoux, J. E. (1997). Fear conditioning induces associative long-term potentiation in the amygdala. Nature 390, 604–607. doi: 10.1038/37601
Rosenkranz, J. A., and Grace, A. A. (2002). Dopamine-mediated modulation of odour-evoked amygdala potentials during pavlovian conditioning. Nature 417, 282–287. doi: 10.1038/417282a
Royer, S., Martina, M., and Paré, D. (1999). An inhibitory interface gates impulse traffic between the input and output stations of the amygdala. J. Neurosci. 19, 10575–10583. doi: 10.1523/JNEUROSCI.19-23-10575.1999
Royer, S., Martina, M., and Paré, D. (2000). Polarized synaptic interactions between intercalated neurons of the amygdala. J. Neurophysiol. 83, 3509–3518. doi: 10.1152/jn.2000.83.6.3509
Rozeske, R. R., and Herry, C. (2018). Neuronal coding mechanisms mediating fear behavior. Curr. Opin. Neurobiol. 52, 60–64. doi: 10.1016/j.conb.2018.04.017
Salinas-Hernández, X. I., and Duvarci, S. (2021). Dopamine in fear extinction. Front. Synaptic Neurosci. 13:635879. doi: 10.3389/fnsyn.2021.635879
Salinas-Hernández, X. I., Vogel, P., Betz, S., Kalisch, R., Sigurdsson, T., and Duvarci, S. (2018). Dopamine neurons drive fear extinction learning by signaling the omission of expected aversive outcomes. Elife 7:e38818. doi: 10.7554/eLife.38818.024
Schultz, W. (2016). Dopamine reward prediction-error signaling: A two component response. Nat. Rev. Neurosci. 17, 183–195. doi: 10.1038/nrn.2015.26
Sigurdsson, T., Doyère, V., Cain, C. K., and LeDoux, J. E. (2007). Long-term potentiation in the amygdala: A cellular mechanism of fear learning and memory. Neuropharmacology 52, 215–227. doi: 10.1016/j.neuropharm.2006.06.022
Sorg, B. A., and Kalivas, P. W. (1993). Effects of cocaine and footshock stress on extracellular dopamine levels in the medial prefrontal cortex. Neuroscience 53, 695–703. doi: 10.1016/0306-4522(93)90617-O
Sotres-Bayon, F., and Quirk, G. J. (2010). Prefrontal control of fear: More than just extinction. Curr. Opin. Neurobiol. 20, 231–235. doi: 10.1016/j.conb.2010.02.005
Stanley, A. T., Lippiello, P., Sulzer, D., and Miniaci, M. C. (2021). Roles for the dorsal striatum in aversive behavior. Front. Cell. Neurosci. 15:634493. doi: 10.3389/fncel.2021.634493
Sullivan, R. M., and Gratton, A. (1998). Relationships between stress-induced increases in medial prefrontal cortical dopamine and plasma corticosterone levels in rats: Role of cerebral laterality. Neuroscience 83, 81–91. doi: 10.1016/S0306-4522(97)00370-9
Tang, W., Kochubey, O., Kintscher, M., and Schneggenburger, R. (2020). A VTA to basal amygdala dopamine projection contributes to signal salient somatosensory events during fear learning. J. Neurosci. 40, 3969–3680. doi: 10.1523/JNEUROSCI.1796-19.2020
Tovote, P., Fadok, J. P., and Lüthi, A. (2015). Neuronal circuits for fear and anxiety. Nat. Rev. Neurosci. 16, 317–331. doi: 10.1038/nrn3945
Vander Weele, C. M., Siciliano, C. A., Matthews, G. A., Namburi, P., Izadmehr, E. M., Espinel, I. C., et al. (2018). Dopamine enhances signal-to-noise ratio in cortical-brainstem encoding of aversive stimuli. Nature 563, 397–401. doi: 10.1038/s41586-018-0682-1
Weiner, D. M., Levey, A. I., Sunahara, R. K., Niznik, H. B., O’Dowd, B. F., Seeman, P., et al. (1991). D1 and D2 dopamine receptor mRNA in rat brain. Proc. Natl. Acad. Sci. U.S.A. 88, 1859–1863.
Wilensky, A. E., Schafe, G. E., Kristensen, M. P., and LeDoux, J. E. (2006). Rethinking the fear circuit: The central nucleus of the amygdala is required for the acquisition, consolidation, and expression of Pavlovian fear conditioning. J. Neurosci. 26, 12387–12396. doi: 10.1523/JNEUROSCI.4316-06.2006
Wilkinson, L. S., Humby, T., Killcross, A. S., Torres, E. M., Everitt, B. J., and Robbins, T. W. (1998). Dissociations in dopamine release in medial prefrontal cortex and ventral striatum during the acquisition and extinction of classical aversive conditioning in the rat. Eur. J. Neurosci. 10, 1019–1026. doi: 10.1046/j.1460-9568.1998.00119.x
Wise, R. A. (2002). Brain reward circuitry: Insights from unsensed incentives. Neuron 36, 229–240. doi: 10.1016/S0896-6273(02)00965-0
Yamamoto, R., Ueta, Y., and Kato, N. J. (2007). Dopamine induces a slow afterdepolarization in lateral amygdala neurons. J. Neurophysiol. 98, 984–992. doi: 10.1152/jn.00204.2007
Young, A. M. (2004). Increased extracellular dopamine in nucleus accumbens in response to unconditioned and conditionedaversive stimuli: Studies using 1 min microdialysis in rats. J. Neurosci. Methods 138, 57–63. doi: 10.1016/j.jneumeth.2004.03.003
Yuan, L., Dou, Y. N., and Sun, Y. G. (2019). Topography of reward and aversion encoding in the mesolimbic dopaminergic system. J. Neurosci. 39, 6472–6481. doi: 10.1523/JNEUROSCI.0271-19.2019
Zimmerman, J. M., Rabinak, C. A., McLachlan, I. G., and Maren, S. (2007). The central nucleus of the amygdala is essential for acquiring and expressing conditional fear after overtraining. Learn. Mem. 14, 634–644. doi: 10.1101/lm.607207
Zweifel, L. S., Fadok, J. P., Argilli, E., Garelick, M. G., Jones, G. L., Dickerson, T. M., et al. (2011). Activation of dopamine neurons is critical for aversive conditioning and prevention of generalized anxiety. Nat. Neurosci. 14, 620–626. doi: 10.1038/nn.2808
Zweifel, L. S., Parker, J. G., Lobb, C. J., Rainwater, A., Wall, V. Z., Fadok, J. P., et al. (2009). Disruption of NMDAR-dependent burst firing by dopamine neurons provides selective assessment of phasic dopamine-dependent behavior. Proc. Natl. Acad. Sci. U.S.A. 106, 7281–7288. doi: 10.1073/pnas.0813415106
Keywords: aversion, fear conditioning, dopamine, amygdala, medial prefrontal cortex (mPFC), striatum, nucleus accumbens (NAc)
Citation: Zafiri D and Duvarci S (2022) Dopaminergic circuits underlying associative aversive learning. Front. Behav. Neurosci. 16:1041929. doi: 10.3389/fnbeh.2022.1041929
Received: 11 September 2022; Accepted: 25 October 2022;
Published: 10 November 2022.
Edited by:
Denise Manahan-Vaughan, Ruhr University Bochum, GermanyReviewed by:
Robert Sears, Nathan Kline Institute for Psychiatric Research, United StatesCopyright © 2022 Zafiri and Duvarci. This is an open-access article distributed under the terms of the Creative Commons Attribution License (CC BY). The use, distribution or reproduction in other forums is permitted, provided the original author(s) and the copyright owner(s) are credited and that the original publication in this journal is cited, in accordance with accepted academic practice. No use, distribution or reproduction is permitted which does not comply with these terms.
*Correspondence: Sevil Duvarci, ZHV2YXJjaUBtZWQudW5pLWZyYW5rZnVydC5kZQ==
Disclaimer: All claims expressed in this article are solely those of the authors and do not necessarily represent those of their affiliated organizations, or those of the publisher, the editors and the reviewers. Any product that may be evaluated in this article or claim that may be made by its manufacturer is not guaranteed or endorsed by the publisher.
Research integrity at Frontiers
Learn more about the work of our research integrity team to safeguard the quality of each article we publish.