- 1International Medical Center, Ward of General Practice and National Clinical Research Center for Geriatrics, West China Hospital, Sichuan University, Chengdu, China
- 2Department of Periodical Press and National Clinical Research Center for Geriatrics, West China Hospital, Sichuan University, Chengdu, China
- 3Nursing Key Laboratory of Sichuan Province, Chengdu, China
- 4Chinese Evidence-Based Medicine Center, West China Hospital, Sichuan University, Chengdu, China
Stroke is the leading cause of global mortality and disability. Cerebral edema and intracranial hypertension are common complications of cerebral infarction and the major causes of mortality. The formation of cerebral edema includes three stages (cytotoxic edema, ionic edema, and vasogenic edema), which involve multiple proteins and ion channels. A range of therapeutic agents that successfully target cerebral edema have been developed in animal studies, some of which have been assessed in clinical trials. Herein, we review the mechanisms of cerebral edema and the research progress of anti-edema therapies for use after ischemic stroke.
Introduction
Stroke is the leading cause of death worldwide (Feigin et al., 2015). Ischemic stroke accounts for 69.6–70.8% of all strokes (Wang W. et al., 2017). The mortality rate of patients with acute ischemic stroke is as high as 2.3–3.2% within 1 month after onset (Huang et al., 2010; Wang D. et al., 2017; Li et al., 2019; He et al., 2020). The formation of cerebral edema is a major cause of death in stroke patients (Simard et al., 2007; Walcott et al., 2014; Rungta et al., 2015).
Cerebral edema is extremely dangerous. Severe cerebral edema after stroke can increase the mortality rate to 80% and is an important predictor of poor prognosis (Kochanek et al., 2011; Battey et al., 2014; Walcott et al., 2014; Nawabi et al., 2019). Thus, an effective treatment of cerebral edema during the early phase of stroke is particularly important. The human brain is enclosed in a hard skull and the interaction between swollen brain tissue and the skull after an ischemic stroke can further increase intracranial pressure. This increase in intracranial pressure can also increase the degree of cerebral hypoxia and ischemia. Unfortunately, the current osmotic therapies focus on treating edema rather than preventing it, and evidence for efficacy remains insufficient (Torbey et al., 2015). Early craniotomy decompression reduces mortality and improves prognosis (Shah et al., 2019), but it deals with downstream events rather than targeting the underlying molecular mechanisms of cerebral edema (Stokum et al., 2015).
Experimentally, a number of agents have been developed that successfully target cerebral edema, one of which has entered clinical trials. This article reviews the mechanisms of cerebral edema and the research progress of anti-edema drugs.
Mechanisms of Cerebral Edema
Cerebral edema after an ischemic stroke includes cytotoxic edema, ionic edema, and vasogenic edema (Liebeskind et al., 2019). These three processes are closely linked and, eventually, ~8.5–30% of patients develop cerebral hemorrhage (Lindley et al., 2004; Paciaroni et al., 2008). However, the mechanisms of cerebral edema formation are not fully understood.
The formation of cerebral edema is still based on the Starling principle. Cytotoxic edema refers to the brain swelling caused by ions (Na+, Cl−) and water entering the cells of neurons or astrocytes (Rungta et al., 2015). Cytotoxic edema occurs quickly after brain tissue ischemia, causing intracellular swelling without increasing the brain tissue volume (Liebeskind et al., 2019). Therefore, cytotoxic edema is intracellular edema. Astrocytes in the brain are most affected by cytotoxic edema (Stokum et al., 2016). Cytotoxic edema leads to changes in ion concentrations on both sides of the blood–brain barrier (BBB), with the intravascular Na+ concentration increasing above that in the interstitium (Mori et al., 2002). The new ionic gradients provide the driving force for ionized edema and vasogenic edema. The sulfonylurea receptor 1-immediate receptor potential melatonin 4 (Sur1-Trpm4), the Na+-K+-2Cl− cotransporter protein-1 (NKCC1), aquaporin-4 (AQP4), the Na+-H+ exchanger, and the Na+-Ca2+ exchanger drive this process (Douglas et al., 2001; Amiry-Moghaddam et al., 2004; Hamann et al., 2010; Xue and Haddad, 2010; Ferrazzano et al., 2011; Jayakumar et al., 2014; Stokum et al., 2016) (Figure 1).
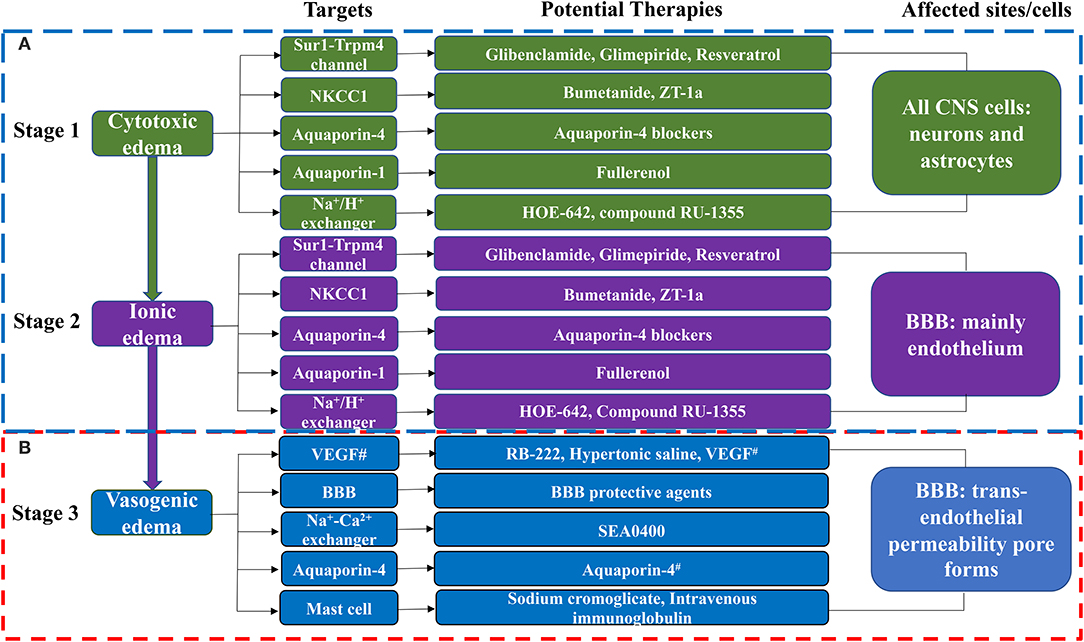
Figure 1. The molecular targets and anti-edema therapies in ischemic stroke. (A) The blood-brain barrier remains intact in this phase. (B) The blood-brain barrier is destroyed in this phase. CNS, Central Nervous System. BBB, blood-brain barrier; NKCC1, Na+-K+-2Cl− cotransporter-1; ZT-1a, a highly effective and selective SPAK inhibitor; HOE-642, a Na+-H+ exchanger inhibitor; Compound RU-1355, a Na+-H+ exchanger inhibitor; RB-222, anti-VEGF neutralizing antibody; SEA0400, a specific inhibitor of the Na+-Ca2+ exchanger; T3, thyroid hormone; VEGF, vascular endothelial growth factor; Anti-miR-1, anti-microRNA-1; Antagomir, inhibitor of miRNA-1. #Note that VEGF can both promote brain edema and have neuroprotective effects. Aquaporin-4 has a protective effect against vasogenic edema. Aquaporin-4 blockers include T3, Inhaled H2S, Acetazolamide, Lactosides B, Mitochondrial aldehyde dehydrogenase 2, Paeoniflorin, Astragaloside IV, and Tongxinluo. BBB protective agents include Alpha-tocopherol, 8-methoxypsoralen, 3-aminobenzamide, Anti-miR-1 or MicroRNA-1 antagomir, MicroRNA-132, MicroRNA-1906, chemokine-like factors, magnesium sulfate, ulinastatin, ginkgo diterpene lactone meglumine injection, olaparib, recombinant human erythropoietin, and 1-trifuoromethoxyphenyl-3-(1-propionylpiperidin-4-yl) urea.
Subsequently, cerebral edema enters the stage of ionic edema. Ionic edema is caused by water and ions crossing the BBB from the vasculature into the brain (Simard et al., 2007). Ionic edema is a subtype of extracellular edema lacking albumin and has been defined in recent years independently of cytotoxic edema. The BBB remains intact in this stage, and ionic edema is completely driven by various ion channels and transporters in the BBB, including the Sur1-Trpm4 channel, NKCC1, Na+-K+-cotransporter (KCC), the Na+-H+ exchanger, and AQP4 (Nilius and Droogmans, 2001; Dolman et al., 2005; Yang et al., 2008; Lam et al., 2009; Previch et al., 2016) (Figure 1).
Following the destruction of BBB, physical connections are made between blood vessels and the interstitium of the brain. Plasma proteins and other macromolecules pass through the BBB into the extracellular space of the brain (Stokum et al., 2016). Vasogenic edema may be considered to be an ultrafiltrate of blood. Vascular endothelial growth factor (VEGF) and free radical metalloprotease (MMP) are involved in vasogenic edema (Zhang et al., 2000; Yang et al., 2007). Mast cells are also involved in this process (Parrella et al., 2019). By contrast, AQP4 helps to clear vasogenic edema (Papadopoulos et al., 2004) (Figure 1).
Sur1-Trpm4 Channel Inhibitors
The Sur1-Trpm4 channel is widely involved in both cytotoxic and ionogenic edema and is the most promising therapeutic target for edema treatment. The Sur1-Trpm4 channel inhibitors are the only drugs to enter clinical trials for the treatment of cerebral edema after ischemic stroke (Table 1).
Glibenclamide
In a phase I trial of intravenous glibenclamide in normal volunteers, two of the 34 subjects in the high dose group had persistent hypoglycemia, while none reported serious adverse events. In a phase II trial of intravenous glibenclamide in 10 patients, there was a reduction in cerebral edema (Sheth et al., 2014a,b), and glibenclamide was well tolerated with no severe hypoglycemia. The phase II trial of intravenous glibenclamide (Sheth et al., 2016) also reported reduced cerebral edema with a lower midline shift but showed no reduction in mortality. Post-exploratory analyses of that trial suggested that glibenclamide could reduce water accumulation, the mass effect, improve survival, decrease midline deviation, and reduce MMP-9 expression (Kimberly et al., 2018; Sheth et al., 2018; Vorasayan et al., 2019). A phase III trial examining whether intravenous glibenclamide can improve functional outcomes at 90 days is currently underway (Table 1).
Glibenclamide requires intravenous administration, which limits its application in the out-of-hospital setting. However, oral preparations can be administered in the early stages of edema. For example, in a study exploring the relationship between oral glibenclamide and cerebral edema in 213 patients with ischemic stroke and 40 patients in a matched cohort (Huang et al., 2019), oral glibenclamide did not increase early death or hypoglycemia but prevented cerebral edema. However, that study did not examine the optimal drug dose or compare the efficacy of oral vs. intravenous glibenclamide administration.
Glibenclamide Combined With Other Therapies
At present, there are no anti-edema drugs approved for clinical practice. The BBB can limit drug entry into the brain, resulting in low central drug concentrations (Patel et al., 2012). Furthermore, cerebral edema is caused by multiple factors and mechanisms. As such, a single target drug may not effectively prevent the formation of cerebral edema (Deng et al., 2019). Deng et al. (2019) reported that nanoparticles of betulinic acid from the Chinese herb Eucommia ulmoides could be loaded with glibenclamide and could effectively penetrate the BBB to reduce infarct size and cerebral edema in mice. Furthermore, this system allowed a lower effective dose of glibenclamide, targeted to Sur1-Trpm4 and oxidation, and improved the anti-cerebral edema efficacy of glibenclamide. In another study, the combined treatment with glibenclamide and therapeutic hypothermia showed a synergistic neuroprotective effect by reducing edema and improving neurobehavioral function in a middle cerebral artery occlusion (MCAO) model in rats and in an oxygen and glucose deprivation-reoxygenation model in endothelial cells (Zhu et al., 2018).
Glimepiride
Preclinical and clinical studies have shown that glibenclamide can reduce cerebral edema but can cause severe hypoglycemia. However, glimepiride, the latest second-generation sulfonylurea, has reduced hypoglycemic actions (Holstein et al., 2001). Glimepiride treatment can reduce stroke in mice (Darsalia et al., 2013) and be as effective as glibenclamide in reducing cerebral edema in wild-type mice (Wang X. et al., 2020). However, in that study, glimepiride was administered at 40 min before reperfusion, which is not feasible in clinical practice. Thus, further studies are required to assess its clinical potential.
Sur1-Trpm4 Inhibitors
A number of drugs that block the Sur1-Trpm4 channel have been developed. For example, resveratrol is a natural product found in a range of plants. Resveratrol has antioxidant actions and can protect against cerebral ischemia, reduce cerebral edema, and prevent BBB damage (Ataie et al., 2016; Pineda-Ramírez et al., 2018, 2020). Furthermore, resveratrol was reported to reduce the expression of Sur1 and AQP4 and the formation of edema (Alquisiras-Burgos et al., 2020).
Vascular Endothelial Growth Factor-Related Drugs
Vascular endothelial growth factor is a mitogen that promotes the formation of new blood vessels, protects nerves, and increases capillary permeability. VEGF when used before the model was reported to reduce the infarct volume and cerebral edema in a rat MCAO model (Harrigan et al., 2003), despite enhancing capillary permeability. The combination therapy of VEGF and nerve growth factor could also reduce infarct volume and cerebral edema, with better efficacy with earlier administration in rabbits (Yang et al., 2018). By contrast, VEGF treatment after cerebral ischemia was found to increase BBB permeability and leakage and aggravate cerebral edema (Chi et al., 2005). Furthermore, Kim et al. (2018) reported that VEGF could promote the formation of cerebral edema in patients with stroke. These contrasting findings may be related to differences in the timing of VEGF administration between the studies.
Interestingly, intraventricular injection of an anti-VEGF neutralizing antibody (RB-222) reduced the number of immature blood vessels following cerebral edema in a rat MCAO model (Zhang et al., 2017). Furthermore, hypertonic saline could reduce osmotic pressure and reduce the formation of cerebral edema via downregulating NKCC expression and inhibiting the VEGF (Huang et al., 2014). Finally, a treatment with 10% hypertonic saline was associated with the downregulation of zonula occludens 1 and occludin expression via the inhibition of the VEGF receptor 2/phospholipase cγ1/endothelial nitric oxide synthase pathway (Wang et al., 2019).
Aquaporin Blockers
Aquaporins are known to contribute to cytotoxic edema. In particular, AQP4 is widely involved in water balance in patients with stroke (Vella et al., 2015; Verkman et al., 2017), with increased expression at 6 h after cerebral infarction and a peak at 3 days (Wei et al., 2015). AQP4 has bidirectional effects of water transport, which is involved in both the formation and the removal of cerebral edema (Stokum et al., 2015). AQP4 promotes the formation of cerebral edema in the early stage (cytotoxic and ionic edema; Tang and Yang, 2016). The brain edema of AQP4-deficient mice was decreased by 35% compared to the wild-type mice (Manley et al., 2000). However, another study had found that AQP4 exacerbates the formation of cerebral edema (Zeng et al., 2012). When cerebral edema enters the stage of vasogenic edema, water enters through the incomplete space between vascular endothelial cells. Cerebral edema is no longer closely related to AQP4, which is more involved in the clearance of cerebral edema (Tang and Yang, 2016). Unfortunately, the boundary between cytotoxic edema, ionic edema, and vasogenic edema is not obvious. However, how to determine the starting and ending time of APQ4 inhibitor is a problem to be solved in the later research. Treatment with thyroid hormone also reduced cerebral edema by inhibiting AQP4 (Mdzinarishvili et al., 2013; Sadana et al., 2015) and may be neuroprotective in patients with stroke. Experimentally, Wei et al. (2015) reported that inhaled hydrogen sulfide (H2S) could reduce cerebral edema in rats by inhibiting AQP4, as well as protect the BBB. In a permanent-MCAO model, the combined treatment with aquaporin inhibitors and cerebrolysin also reduced the formation of cerebral edema (Catalin et al., 2018). Furthermore, acetazolamide (Duan et al., 2019), lactosides B, mitochondrial aldehyde dehydrogenase 2 (Li et al., 2018), paeoniflorin, astragaloside IV (Chu et al., 2017), and the Chinese herbs show the neuroprotective effect (Ni et al., 2020), while Tongxinluo (Cai et al., 2016) showed anti-edema actions via the inhibition of AQP4.
Aquaporin-1(AQP1) is also involved in the formation of cerebral edema (Qiu et al., 2014), while AQP1 inhibitors, such as fullerenols, can reduce cerebral edema (Darabi and Mohammadi, 2017). However, AQP1 is expressed in different species (Arciénega et al., 2010; Badaut et al., 2011) and different organs (Buffoli, 2010), which limits the use of AQP1 inhibitors. Importantly, while aquaporins are involved in cytotoxic edema, the inhibition of AQPs is only effective in the early stage of the disease.
ION Channel Blockers
Cation-Cl− Cotransporter Inhibitors
Na+-K+-2Cl− cotransporter protein-1 and KCC2 play important roles in the formation of both cytotoxic and ionic edema. NKCC1 and KCC2 are cationic Cl− cotransporters that have opposing actions on intracranial water and electrolyte balance (Russell, 2000; Blaesse et al., 2009). Bumetanide, which inhibits NKCC1, can reduce cytotoxic edema (Yan et al., 2003). Furthermore, Wang et al. (2014) reported that bumetanide reduced cerebral edema in an ischemia-reperfusion model without changing the KCC2 protein expression. Bumetanide plays a role in both the acute and chronic phases of cerebral ischemia (Xu et al., 2016, 2017). Zhang et al. (2020a) also found that ZT-1a, a novel selective SPS1-related proline/alanine-rich kinase (SPAK) inhibitor that inhibits NKCC1 while activating KCC2, reduced cerebral edema and improved stroke prognosis.
Na+-H+ Exchanger Inhibitors
As for NKCC1, the Na+-H+ exchanger plays an important role in the early stage of cerebral edema after ischemia. Hypoxia can stimulate BBB Na+-H+ exchanger activity, thereby promoting the formation of cerebral edema. Intravenous injection of a Na+-H+ exchanger inhibitor (HOE-642; compound RU-1355) significantly reduced the Na+ concentration and reduced cerebral edema (O'Donnell et al., 2013; Spasov et al., 2016). Interestingly, a combined treatment with HOE-642 and bumetanide had similar efficacy to HOE-642 alone, suggesting no additive effect of two types of drugs.
Na+-Ca2+ Exchanger Inhibitors
Maintaining intracellular Ca2+ homeostasis is important for protecting the BBB. Koyama et al. (2004) found that the Na+-Ca2+ exchanger was involved in vasogenic edema and BBB destruction. Furthermore, Na+-Ca2+ exchanger inhibitors (SEA0400) could reduce the formation of cerebral edema and cerebral infarction volume after cerebral ischemia (Matsuda et al., 2001).
Conivaptan
Arginine vasopressin and its receptors, V1a and V2, play important roles in cerebral edema after ischemic stroke (Vakili et al., 2005). Conivaptan is a V1a and V2 receptor blocker that can reduce cerebral edema (Ansari et al., 2018). Intraperitoneal administration of conivaptan was reported to be superior to continuous intravenous administration (Zeynalov et al., 2016). Zeynalov et al. (2017) also found that conivaptan treatment at 3 h after ischemia could reduce edema, suggesting a role in early cytotoxic edema.
MicroRNAs
MicroRNAs (miRNAs) regulate the gene expression at the transcriptional level and are promising targets for disease therapy (Carleton et al., 2007; Li G. et al., 2018). The expression of miRNA-1 is related to ischemic damage and cellular apoptosis (Chen et al., 2006). Treatment with anti-miR-1 reduced the infarct volume (Selvamani et al., 2012), while treatment with the miRNA-1 antagomir significantly reduced cerebral edema and BBB damage (Talebi et al., 2019). Of note, other miRNAs, including miRNA-132 (Zuo et al., 2019) and miRNA-1906 (Yu and Li, 2020), are potential targets for the treatment of edema.
BBB Protective Agents
Vasogenic edema is accompanied by BBB destruction. Thus, maintaining BBB integrity is an important outcome measure for anti-edema treatments. Alpha-tocopherol can protect the BBB via its antioxidant actions (Chaudhary et al., 2003; Hsiao et al., 2007) and reduce edema formation after ischemic stroke (Azar et al., 2017). Treatment with 8-methoxypsoralen can also improve BBB ultrastructure by increasing the NFE2-related factor 2 and hemeoxygenase 1 protein expression, thus reducing edema (Liu et al., 2018). Furthermore, 3-aminobenzamide and calcitriol can both maintain BBB integrity and have anti-edema effects (Sadeghian et al., 2019; Wang J. et al., 2020). Mast cells are also involved in BBB destruction (Ocak et al., 2019), while the modulation of mast cells with agents such as sodium cromoglycate (McKittrick et al., 2015) and intravenous immunoglobulin (Widiapradja et al., 2012) can reduce edema. Furthermore, chemokine-like factors can maintain the BBB integrity and inhibit the formation of cerebral edema (Kong et al., 2016). Potential drugs targeting BBB include magnesium sulfate (Shadman et al., 2019), ulinastatin (Li X.-F. et al., 2018), ginkgo diterpene lactone meglumine injection (Li et al., 2017), olaparib (Teng et al., 2016), recombinant human erythropoietin (Wang et al., 2015), and 1-trifuoromethoxyphenyl-3-(1-propionylpiperidin-4-yl) urea (Zhang et al., 2020b).
Perspectives
Cerebral edema following ischemic stroke is associated with a poor prognosis. Unfortunately, there are currently limited specific anti-cerebral edema treatment options available for clinical use. Traditional osmotic therapies are not specific to the molecular mechanism of cerebral edema. Their main role is to reduce intracranial hypertension and relieve mass effects (Stokum et al., 2020), which may cause serious complications such as water and electrolyte disorders and kidney damage (Zhang et al., 2019). These drugs are usually used only when high levels of intracranial hypertension are reached and cerebral perfusion is threatened. Potential new drugs target the pathophysiological mechanism of cerebral edema formation and prevent the formation of cerebral edema while avoiding the above complications.
A range of therapeutic agents (Table 2) have been developed that successfully target cerebral edema and reduce brain injury in animal models. These include targeted ion channels, transporters, and specific targets. Also include anti-apoptotic, anti-inflammatory, and antioxidant drugs (Tan et al., 2019; Moghadam and Fereidoni, 2020; Yang et al., 2020). The Sur1-Trpm4 inhibitors are of particular interest and have been studied in three clinical trials. However, as of yet, no drugs have been approved, which may relate to the design of the preclinical animal experiments. To improve the clinical translation, further preclinical animal studies are required in a range of models, which should examine the optimal therapeutic windows for the various agents. It is also important to consider that cerebral edema is caused by multiple mechanisms and that combination therapies may be the most effective treatment strategy.
Author Contributions
YZ and XL designed and conceptualized the review. YY drafted and revised the manuscript. YL revised the manuscript. RY and JL retrieved and screened the review. All authors contributed to the manuscript and approved the final version.
Funding
This work was supported by the National Key Research and Development Program of China (no. 2017YFC0907303), the 1-3-5 project for disciplines of excellence—Clinical Research Incubation Project, West China Hospital, Sichuan University (no. 2018HXFH005), and National Clinical Research Center for Geriatrics, West China Hospital, Sichuan University (no. Z20191009).
Conflict of Interest
The authors declare that the research was conducted in the absence of any commercial or financial relationships that could be construed as a potential conflict of interest.
Acknowledgments
We thank Liwen Bianji, Edanz Editing China (www.liwenbianji.cn/ac), for editing the English text of a draft of this manuscript.
Abbreviations
BBB, The blood–brain barrier; Sur1-Trpm4, The sulfonylurea receptor 1-immediate receptor potential melatonin 4; NKCC1, the Na+-K+-2Cl– cotransporter protein-1; AQP4, Aquaporin-4; AQP1, Aquaporin-1; KCC, Na+-K+-cotransporter; KCC2, Na+-K+-cotransporter 2; VEGF, Vascular endothelial growth factor; MMP, Free radical metalloprotease; MMP-9, Free radical metalloprotease-9; MCAO, A middle cerebral artery occlusion model; H2S, Hydrogen sulfide; SPAK, SPS1-related proline/alanine-rich kinase; ZT-1a, 5-chloro-N-[5-chloro-4-((4-chlorophenyl)(cyano) methyl)-2-methylphenyl]-2-hydroxybenzamide; HOE-642, The specific Na+-H+exchange inhibitor; Compound RU-1355, The new inhibitor of Na+-H+ exchanger; miRNAs, MicroRNAs; SEA0400, 2-[4-[(2,5-Difluorophenyl)methoxy]phenoxy]-5-ethoxyaniline.
References
Alquisiras-Burgos, I., Ortiz-Plata, A., Franco-Perez, J., Millan, A., and Aguilera, P. (2020). Resveratrol reduces cerebral edema through inhibition of de novo SUR1 expression induced after focal ischemia. Exp. Neurol. 330:113353. doi: 10.1016/j.expneurol.2020.113353
Amiry-Moghaddam, M., Xue, R., Haug, F. M., Neely, J. D., Bhardwaj, A., Agre, P., et al. (2004). Alpha-syntrophin deletion removes the perivascular but not endothelial pool of aquaporin-4 at the blood-brain barrier and delays the development of brain edema in an experimental model of acute hyponatremia. FASEB J. 18, 542–544. doi: 10.1096/fj.03-0869fje
Ansari, S., Krishnan, R., Shahripour, R. B., Moein, P., Kerro, A., Pandhi, A., et al (2018). Combined antagonism of vasopressin receptor subtypes with conivaptan attenuates cerebral edema following ischemic stroke. Neurology 90. Available online at: https://n.neurology.org/content/90/15_Supplement/P5.202
Arciénega, I. I., Brunet, J. F., Bloch, J., and Badaut, J. (2010). Cell locations for AQP1, AQP4 and 9 in the non-human primate brain. Neuroscience 167, 1103–1114. doi: 10.1016/j.neuroscience.2010.02.059
Ataie, A., Shadifar, M., and Ataee, R. (2016). Polyphenolic antioxidants and neuronal regeneration. Basic Clin. Neurosci. 7, 81–90. doi: 10.15412/J.BCN.03070201
Azar, A. H., Oryan, S., Bohlooli, S., and Panahpour, H. (2017). Alpha-tocopherol reduces brain edema and protects blood-brain barrier integrity following focal cerebral ischemia in rats. Med. Principles Pract. 26, 17–22. doi: 10.1159/000450648
Badaut, J., Ashwal, S., and Obenaus, A. (2011). Aquaporins in cerebrovascular disease: a target for treatment of brain edema? Cerebrovasc. Dis. 31, 521–531. doi: 10.1159/000324328
Battey, T. W., Karki, M., Singhal, A. B., Wu, O., Sadaghiani, S., Campbell, B. C., et al. (2014). Brain edema predicts outcome after nonlacunar ischemic stroke. Stroke 45, 3643–3648. doi: 10.1161/STROKEAHA.114.006884
Blaesse, P., Airaksinen, M. S., Rivera, C., and Kaila, K. (2009). Cation-chloride cotransporters and neuronal function. Neuron 61, 820–838. doi: 10.1016/j.neuron.2009.03.003
Buffoli, B. (2010). Aquaporin biology and nervous system. Curr. Neuropharmacol. 8, 97–104. doi: 10.2174/157015910791233204
Cai, M., Yu, Z., Wang, L., Song, X., Zhang, J., Zhang, Z., et al. (2016). Tongxinluo reduces brain edema and inhibits post-ischemic inflammation after middle cerebral artery occlusion in rats. J. Ethnopharmacol. 181, 136–145. doi: 10.1016/j.jep.2016.01.026
Carleton, M., Cleary, M. A., and Linsley, P. S. (2007). MicroRNAs and cell cycle regulation. Cell Cycle 6, 2127–2132. doi: 10.4161/cc.6.17.4641
Catalin, B., Rogoveanu, O.-C., Pirici, I., Balseanu, T. A., Stan, A., Tudorica, V., et al. (2018). Cerebrolysin and aquaporin 4 inhibition improve pathological and motor recovery after ischemic stroke. CNS Neurol. Disord. Drug Targets 17, 299–308. doi: 10.2174/1871527317666180425124340
Chaudhary, G., Sinha, K., and Gupta, Y. K. (2003). Protective effect of exogenous administration of alpha-tocopherol in middle cerebral artery occlusion model of cerebral ischemia in rats. Fundam. Clin. Pharmacol. 17, 703–707. doi: 10.1046/j.0767-3981.2003.00209.x
Chen, J. F., Mandel, E. M., Thomson, J. M., Wu, Q., Callis, T. E., Hammond, S. M., et al. (2006). The role of microRNA-1 and microRNA-133 in skeletal muscle proliferation and differentiation. Nat. Genet. 38, 228–233. doi: 10.1038/ng1725
Chi, O. Z., Hunter, C., Liu, X., and Weiss, H. R. (2005). Effects of VEGF and nitric oxide synthase inhibition on blood-brain barrier disruption in the ischemic and non-ischemic cerebral cortex. Neurol. Res 27, 864–868. doi: 10.1179/016164105X49418
Chu, H., Huang, C., Gao, Z., Dong, J., Tang, Y., and Dong, Q. (2017). Reduction of ischemic brain edema by combined use of paeoniflorin and astragaloside IV via down-regulating connexin 43. Phytother. Res. 31, 1410–1418. doi: 10.1002/ptr.5868
Darabi, S., and Mohammadi, M. T. (2017). Fullerenol nanoparticles decrease ischaemia-induced brain injury and oedema through inhibition of oxidative damage and aquaporin-1 expression in ischaemic stroke. Brain Inj. 31, 1142–1150. doi: 10.1080/02699052.2017.1300835
Darsalia, V., Ortsäter, H., Olverling, A., Darlöf, E., Wolbert, P., Nyström, T., et al. (2013). The DPP-4 inhibitor linagliptin counteracts stroke in the normal and diabetic mouse brain. Diabetes 62:1289. doi: 10.2337/db12-0988
Deng, G., Ma, C., Zhao, H., Zhang, S., Liu, J., Liu, F., et al. (2019). Anti-edema and antioxidant combination therapy for ischemic stroke via glyburide-loaded betulinic acid nanoparticles. Theranostics 9, 6991–7002. doi: 10.7150/thno.35791
Dolman, D., Drndarski, S., Abbott, N. J., and Rattray, M. (2005). Induction of aquaporin 1 but not aquaporin 4 messenger RNA in rat primary brain microvessel endothelial cells in culture. J. Neurochem. 93, 825–833. doi: 10.1111/j.1471-4159.2005.03111.x
Douglas, R. M., Schmitt, B. M., Xia, Y., Bevensee, M. O., Biemesderfer, D., Boron, W. F., et al. (2001). Sodium-hydrogen exchangers and sodium-bicarbonate co-transporters: ontogeny of protein expression in the rat brain. Neuroscience 102, 217–228. doi: 10.1016/S0306-4522(00)00473-5
Duan, H., Hao, C., Duan, S., Wang, S., Gao, W., and Zhang, C. (2019). Effect of acetazolamide on brain swelling and aquaporin-4 expression in rats brain after ischemic stroke. FASEB J. 33, 1–7. doi: 10.1016/j.jocn.2020.01.079
Feigin, V. L., Krishnamurthi, R. V., Parmar, P., Norrving, B., Mensah, G. A., Bennett, D. A., et al. (2015). Update on the global burden of ischemic and hemorrhagic stroke in 1990-2013: the GBD 2013 study. Neuroepidemiology 45, 161–176. doi: 10.1159/000441085
Ferrazzano, P., Shi, Y., Manhas, N., Wang, Y., Hutchinson, B., Chen, X., et al. (2011). Inhibiting the Na+/H+ exchanger reduces reperfusion injury: a small animal MRI study. Front. Biosci. 3, 81–88. doi: 10.2741/e222
Hamann, S., Herrera-Perez, J. J., Zeuthen, T., and Alvarez-Leefmans, F. J. (2010). Cotransport of water by the Na+-K+-2Cl(-) cotransporter NKCC1 in mammalian epithelial cells. J. Physiol. 588(Pt 21), 4089–4101. doi: 10.1113/jphysiol.2010.194738
Harrigan, M. R., Ennis, S. R., Sullivan, S. E., and Keep, R. F. (2003). Effects of intraventricular infusion of vascular endothelial growth factor on cerebral blood flow, edema, and infarct volume. Acta Neurochir. 145, 49–53. doi: 10.1007/s00701-002-1035-1
He, Y. B., Su, Y. Y., Rajah, G.B., Zhang, Y. B., Fan, L. L., Liu, G., et al. (2020). Trans-cranial Doppler predicts early neurologic deterioration in anterior circulation ischemic stroke after successful endovascular treatment. Chin Med J (Engl). 133, 1655–1661.
Holstein, A., Plaschke, A., and Egberts, E. H. (2001). Lower incidence of severe hypoglycaemia in patients with type 2 diabetes treated with glimepiride versus glibenclamide. Diabetes Metab. Res. Rev. 17, 467–473. doi: 10.1002/dmrr.235
Hsiao, G., Lee, J. J., Chen, Y. C., Lin, J. H., Shen, M. Y., Lin, K. H., et al. (2007). Neuroprotective effects of PMC, a potent alpha-tocopherol derivative, in brain ischemia-reperfusion: reduced neutrophil activation and anti-oxidant actions. Biochem. Pharmacol. 73, 682–693. doi: 10.1016/j.bcp.2006.11.009
Huang, K., Hu, Y., Wu, Y., Ji, Z., Wang, S., Lin, Z., et al. (2019). Exploratory analysis of oral glibenclamide in acute ischemic stroke. Acta Neurol. Scand. 140, 212–218. doi: 10.1111/ane.13134
Huang, L. Q., Zhu, G. F., Deng, Y. Y., Jiang, W. Q., Fang, M., Chen, C. B., et al. (2014). Hypertonic saline alleviates cerebral edema by inhibiting microglia-derived TNF-α and IL-1β-induced Na-K-Cl cotransporter up-regulation. J. Neuroinflamm. 11:102. doi: 10.1186/1742-2094-11-102
Huang, Y., Wang, J. G., Wei, J. W., Headley, A. P., Wong, L. K., Heeley, E. L., et al. (2010). Age and gender variations in the management of ischaemic stroke in China. Int. J. Stroke 5, 351–359. doi: 10.1111/j.1747-4949.2010.00460.x
Jayakumar, A. R., Valdes, V., Tong, X. Y., Shamaladevi, N., Gonzalez, W., and Norenberg, M. D. (2014). Sulfonylurea receptor 1 contributes to the astrocyte swelling and brain edema in acute liver failure. Transl. Stroke Res 5, 28–37. doi: 10.1007/s12975-014-0328-z
Kim, E., Yang, J., Park, K. W., and Cho, S. (2018). Inhibition of VEGF signaling reduces diabetes-exacerbated brain swelling, but not infarct size, in large cerebral infarction in mice. Transl. Stroke Res. 9, 540–548. doi: 10.1007/s12975-017-0601-z
Kimberly, W. T., Bevers, M. B., von Kummer, R., Demchuk, A. M., Romero, J. M., Elm, J. J., et al. (2018). Effect of IV glyburide on adjudicated edema endpoints in the GAMES-RP trial. Neurology 91, e2163–e2169. doi: 10.1212/WNL.0000000000006618
Kochanek, K. D., Xu, J., Murphy, S. L., Miniño, A. M., and Kung, H. C. (2011). Deaths: final data for 2009. Natl. Vital Stat. Rep 60, 1–116.
Kong, L.-L., Wang, Z.-Y., Hu, J.-F., Yuan, Y.-H., Li, H., and Chen, N.-H. (2016). Inhibition of chemokine-like factor 1 improves blood-brain barrier dysfunction in rats following focal cerebral ischemia. Neurosci. Lett. 627, 192–198. doi: 10.1016/j.neulet.2016.06.003
Koyama, Y., Matsui, S., Itoh, S., Osakada, M., Baba, A., and Matsuda, T. (2004). The selective Na+-Ca2+ exchange inhibitor attenuates brain edema after radiofrequency lesion in rats. Eur. J. Pharmacol. 489, 193–196. doi: 10.1016/j.ejphar.2004.03.018
Lam, T. I., Wise, P. M., and O'Donnell, M. E. (2009). Cerebral microvascular endothelial cell Na/H exchange: evidence for the presence of NHE1 and NHE2 isoforms and regulation by arginine vasopressin. Am. J. Physiol. Cell Physiol. 297, C278–289. doi: 10.1152/ajpcell.00093.2009
Li, G., Morris-Blanco, K. C., Lopez, M. S., Yang, T., Zhao, H., Vemuganti, R., et al. (2018). Impact of microRNAs on ischemic stroke: From pre- to post-disease. Prog. Neurobiol. 163–164, 59–78. doi: 10.1016/j.pneurobio.2017.08.002
Li, S. Y., Yang, X. M., Zhao, X. Q., Liu, L. P., Wang, Y.L., Jiang, Y., et al. (2019). Newly detected atrial fibrillation is associated with cortex-involved ischemic stroke. Chin Med J (Engl). 132, 2053–2058.
Li, X.-F., Zhang, X.-J., Zhang, C., Wang, L.-N., Li, Y.-R., Zhang, Y., et al. (2018). Ulinastatin protects brain against cerebral ischemia/reperfusion injury through inhibiting MMP-9 and alleviating loss of ZO-1 and occludin proteins in mice. Exp. Neurol. 302, 68–74. doi: 10.1016/j.expneurol.2017.12.016
Li, Y., Liu, S.-l., and Qi, S.-h. (2018). ALDH2 protects against ischemic stroke in rats by facilitating 4-HNE clearance and AQP4 down-regulation. Neurochem. Res. 43, 1339–1347. doi: 10.1007/s11064-018-2549-0
Li, Z.-Q., Cao, Z.-Y., Cao, L., Ke, Z.-P., Wang, Z.-Z., and Xiao, W. (2017). Cerebral vascular protective effect of ginkgo diterpene lactone meglumine injection. Zhongguo Zhong Yao Za Zhi 42, 4738–4743. doi: 10.19540/j.cnki.cjcmm.2017.0210
Liebeskind, D. S., Jüttler, E., Shapovalov, Y., Yegin, A., Landen, J., and Jauch, E. C. (2019). Cerebral edema associated with large hemispheric infarction. Stroke 50, 2619–2625. doi: 10.1161/STROKEAHA.118.024766
Lindley, R. I., Wardlaw, J. M., Sandercock, P. A., Rimdusid, P., Lewis, S. C., Signorini, D. F., et al. (2004). Frequency and risk factors for spontaneous hemorrhagic transformation of cerebral infarction. J. Stroke Cerebrovasc. Dis. 13, 235–246. doi: 10.1016/j.jstrokecerebrovasdis.2004.03.003
Liu, C., Zhong, L., Tian, X. L., and Han, Y. C. (2018). Protective effects of 8-MOP on blood-brain barrier via the Nrf-2/HO-1 pathway in mice model of cerebral infarction2. Eur. Rev. Med. Pharmacol. Sci. 22, 4278–4287. doi: 10.3892/etm.2019.7859
Manley, G. T., Fujimura, M., Ma, T., Noshita, N., Filiz, F., Bollen, A. W., et al. (2000). Aquaporin-4 deletion in mice reduces brain edema after acute water intoxication and ischemic stroke. Nat. Med. 6, 159–163. doi: 10.1038/72256
Matsuda, T., Arakawa, N., Takuma, K., Kishida, Y., Kawasaki, Y., Sakaue, M., et al. (2001). SEA0400, a novel and selective inhibitor of the Na+-Ca2+ exchanger, attenuates reperfusion injury in the in vitro and in vivo cerebral ischemic models. J. Pharmacol. Exp. Ther. 298, 249–256.
McKittrick, C. M., Lawrence, C. E., and Carswell, H. V. (2015). Mast cells promote blood brain barrier breakdown and neutrophil infiltration in a mouse model of focal cerebral ischemia. J. Cereb. Blood Flow Metab. 35, 638–647. doi: 10.1038/jcbfm.2014.239
Mdzinarishvili, A., Sutariya, V., Talasila, P. K., Geldenhuys, W. J., and Sadana, P. (2013). Engineering triiodothyronine (T3) nanoparticle for use in ischemic brain stroke. Drug Deliv. Transl. Res. 3, 309–317. doi: 10.1007/s13346-012-0117-8
Moghadam, B. F., and Fereidoni, M. (2020). Neuroprotective effect of menaquinone-4 (MK-4) on transient global cerebral ischemia/reperfusion injury in rat. PLoS ONE 15:e0229769. doi: 10.1371/journal.pone.0229769
Mori, K., Miyazaki, M., Iwase, H., and Maeda, M. (2002). Temporal profile of changes in brain tissue extracellular space and extracellular ion [Na(+), K(+)] concentrations after cerebral ischemia and the effects of mild cerebral hypothermia. J. Neurotrauma 19, 1261–1270. doi: 10.1089/08977150260338047
Nawabi, J., Flottmann, F., Hanning, U., Bechstein, M., Schön, G., Kemmling, A., et al. (2019). Futile recanalization with poor clinical outcome is associated with increased edema volume after ischemic stroke. Invest. Radiol. 54, 282–287. doi: 10.1097/RLI.0000000000000539
Ni, X., Lin, H., Li, H., Liao, W., Luo, X., Wu, D., et al. (2020). Evidence-based practice guideline on integrative medicine for stroke 2019. J. Evid. Based Med. 13, 137–152. doi: 10.1111/jebm.12386
Nilius, B., and Droogmans, G. (2001). Ion channels and their functional role in vascular endothelium. Physiol. Rev. 81, 1415–1459. doi: 10.1152/physrev.2001.81.4.1415
Ocak, U., Ocak, P. E., Wang, A., Zhang, J. H., Boling, W., Wu, P., et al. (2019). Targeting mast cell as a neuroprotective strategy. Brain Inj. 33, 723–733. doi: 10.1080/02699052.2018.1556807
O'Donnell, M. E., Chen, Y. J., Lam, T. I., Taylor, K. C., Walton, J. H., and Anderson, S. E. (2013). Intravenous HOE-642 reduces brain edema and Na uptake in the rat permanent middle cerebral artery occlusion model of stroke: evidence for participation of the blood-brain barrier Na/H exchanger. J. Cereb. Blood Flow Metab. 33, 225–234. doi: 10.1038/jcbfm.2012.160
Paciaroni, M., Agnelli, G., Corea, F., Ageno, W., Alberti, A., Lanari, A., et al. (2008). Early hemorrhagic transformation of brain infarction: rate, predictive factors, and influence on clinical outcome: results of a prospective multicenter study. Stroke 39, 2249–2256. doi: 10.1161/STROKEAHA.107.510321
Papadopoulos, M. C., Manley, G. T., Krishna, S., and Verkman, A. S. (2004). Aquaporin-4 facilitates reabsorption of excess fluid in vasogenic brain edema. FASEB J. 18, 1291–1293. doi: 10.1096/fj.04-1723fje
Parrella, E., Porrini, V., Benarese, M., and Pizzi, M. (2019). The role of mast cells in stroke. Cells 8:437. doi: 10.3390/cells8050437
Patel, T., Zhou, J., Piepmeier, J. M., and Saltzman, W. M. (2012). Polymeric nanoparticles for drug delivery to the central nervous system. Adv. Drug Deliv. Rev. 64, 701–705. doi: 10.1016/j.addr.2011.12.006
Pineda-Ramírez, N., Alquisiras-Burgos, I., Ortiz-Plata, A., Ruiz-Tachiquín, M. E., Espinoza-Rojo, M., and Aguilera, P. (2020). Resveratrol activates neuronal autophagy through AMPK in the ischemic brain. Mol. Neurobiol. 57, 1055–1069. doi: 10.1007/s12035-019-01803-6
Pineda-Ramírez, N., Gutiérrez Aguilar, G. F., Espinoza-Rojo, M., and Aguilera, P. (2018). Current evidence for AMPK activation involvement on resveratrol-induced neuroprotection in cerebral ischemia. Nutr. Neurosci. 21, 229–247. doi: 10.1080/1028415X.2017.1284361
Previch, L. E., Ma, L., Wright, J. C., Singh, S., Geng, X., and Ding, Y. (2016). Progress in AQP research and new developments in therapeutic approaches to ischemic and hemorrhagic stroke. Int. J. Mol. Sci. 17:1146. doi: 10.3390/ijms17071146
Qiu, B., Li, X., Sun, X., Wang, Y., Jing, Z., Zhang, X., et al. (2014). Overexpression of aquaporin-1 aggravates hippocampal damage in mouse traumatic brain injury models. Mol. Med. Rep. 9, 916–922. doi: 10.3892/mmr.2014.1899
Rungta, R. L., Choi, H. B., Tyson, J. R., Malik, A., Dissing-Olesen, L., Lin, P. J. C., et al. (2015). The cellular mechanisms of neuronal swelling underlying cytotoxic edema. Cell 161, 610–621. doi: 10.1016/j.cell.2015.03.029
Russell, J. M. (2000). Sodium-potassium-chloride cotransport. Physiol. Rev. 80, 211–276. doi: 10.1152/physrev.2000.80.1.211
Sadana, P., Coughlin, L., Burke, J., Woods, R., and Mdzinarishvili, A. (2015). Anti-edema action of thyroid hormone in MCAO model of ischemic brain stroke: Possible association with AQP4 modulation. J. Neurol. Sci. 354, 37–45. doi: 10.1016/j.jns.2015.04.042
Sadeghian, N., Shadman, J., Moradi, A., Golmohammadi, M. G., and Panahpour, H. (2019). Calcitriol protects the Blood-Brain Barrier integrity against ischemic stroke and reduces vasogenic brain edema via antioxidant and antiapoptotic actions in rats. Brain Res. Bull. 150, 281–289. doi: 10.1016/j.brainresbull.2019.06.010
Selvamani, A., Sathyan, P., Miranda, R. C., and Sohrabji, F. (2012). An antagomir to microRNA Let7f promotes neuroprotection in an ischemic stroke model. PLoS ONE 7:e32662. doi: 10.1371/journal.pone.0032662
Shadman, J., Sadeghian, N., Moradi, A., Bohlooli, S., and Panahpour, H. (2019). Magnesium sulfate protects blood-brain barrier integrity and reduces brain edema after acute ischemic stroke in rats. Metab. Brain Dis. 34, 1221–1229. doi: 10.1007/s11011-019-00419-y
Shah, A., Almenawer, S., and Hawryluk, G. (2019). Timing of Decompressive Craniectomy for Ischemic Stroke and Traumatic Brain Injury: A Review. Front. Neurol 10, 11. doi: 10.3389/fneur.2019.00011
Sheth, K. N., Elm, J. J., Molyneaux, B. J., Hinson, H., Beslow, L. A., Sze, G. K., et al. (2016). Safety and efficacy of intravenous glyburide on brain swelling after large hemispheric infarction (GAMES-RP): a randomised, double-blind, placebo-controlled phase 2 trial. Lancet Neurol 15, 1160–1169. doi: 10.1016/S1474-4422(16)30196-X
Sheth, K. N., Kimberly, W. T., Elm, J. J., Kent, T. A., Mandava, P., Yoo, A. J., et al. (2014a). Pilot study of intravenous glyburide in patients with a large ischemic stroke. Stroke 45, 281–283. doi: 10.1161/STROKEAHA.113.003352
Sheth, K. N., Kimberly, W. T., Elm, J. J., Kent, T. A., Yoo, A. J., Thomalla, G., et al. (2014b). Exploratory analysis of glyburide as a novel therapy for preventing brain swelling. Neurocrit. Care 21, 43–51. doi: 10.1007/s12028-014-9970-2
Sheth, K. N., Petersen, N. H., Cheung, K., Elm, J. J., Hinson, H. E., Molyneaux, B. J., et al. (2018). Long-term outcomes in patients aged 70 years with intravenous glyburide from the phase II GAMES-RP study of large hemispheric infarction: an exploratory analysis. Stroke 49, 1457–1463. doi: 10.1161/STROKEAHA.117.020365
Simard, J. M., Kent, T. A., Chen, M., Tarasov, K. V., and Gerzanich, V. (2007). Brain oedema in focal ischaemia: molecular pathophysiology and theoretical implications. Lancet Neurol. 6, 258–268. doi: 10.1016/S1474-4422(07)70055-8
Spasov, A. A., Murav'eva, V. U., Gurova, N. A., Cheplyaeva, N. I., Reznikov, E. V., and Anisimova, V. A. (2016). Neuroprotective properties of a new inhibitor of NA+/H+ exchanger (compound RU-1355) on the model of focal ischemia in rats. Eksperimental'naia i klinicheskaia farmakologiia 79, 3–7.
Stokum, J. A., Gerzanich, V., Sheth, K. N., Kimberly, W. T., and Simard, J. M. (2020). Emerging pharmacological treatments for cerebral edema: evidence from clinical studies. Annu. Rev. Pharmacol. Toxicol. 60, 291–309. doi: 10.1146/annurev-pharmtox-010919-023429
Stokum, J. A., Gerzanich, V., and Simard, J. M. (2016). Molecular pathophysiology of cerebral edema. J. Cereb. Blood Flow Metab. 36, 513–538. doi: 10.1177/0271678X15617172
Stokum, J. A., Kurland, D. B., Gerzanich, V., and Simard, J. M. (2015). Mechanisms of astrocyte-mediated cerebral edema. Neurochem. Res. 40, 317–328. doi: 10.1007/s11064-014-1374-3
Talebi, A., Rahnema, M., and Bigdeli, M. R. (2019). Effect of intravenous injection of antagomiR-1 on brain ischemia. Mol. Biol. Rep. 46, 1149–1155. doi: 10.1007/s11033-018-04580-y
Tan, L., Li, J., Wang, Y., and Tan, R. (2019). Anti-neuroinflammatory effect of alantolactone through the suppression of the NF-kappa B and MAPK signaling pathways. Cells 8:739. doi: 10.3390/cells8070739
Tang, G., and Yang, G. Y. (2016). Aquaporin-4: a potential therapeutic target for cerebral edema. Int. J. Mol. Sci. 17:1413. doi: 10.3390/ijms17101413
Teng, F., Zhu, L., Su, J., Zhang, X., Li, N., Nie, Z., et al. (2016). Neuroprotective effects of poly(ADP-ribose)polymerase inhibitor olaparib in transient cerebral ischemia. Neurochem. Res. 41, 1516–1526. doi: 10.1007/s11064-016-1864-6
Torbey, M. T., Bösel, J., Rhoney, D. H., Rincon, F., Staykov, D., Amar, A. P., et al. (2015). Evidence-based guidelines for the management of large hemispheric infarction: a statement for health care professionals from the Neurocritical Care Society and the German Society for Neuro-intensive Care and Emergency Medicine. Neurocrit. Care 22, 146–164. doi: 10.1007/s12028-014-0085-6
Vakili, A., Kataoka, H., and Plesnila, N. (2005). Role of arginine vasopressin V1 and V2 receptors for brain damage after transient focal cerebral ischemia. J. Cereb. Blood Flow Metab. 25, 1012–1019. doi: 10.1038/sj.jcbfm.9600097
Vella, J., Zammit, C., Di Giovanni, G., Muscat, R., and Valentino, M. (2015). The central role of aquaporins in the pathophysiology of ischemic stroke. Front. Cell. Neurosci. 9:108. doi: 10.3389/fncel.2015.00108
Verkman, A. S., Smith, A. J., Phuan, P. W., Tradtrantip, L., and Anderson, M. O. (2017). The aquaporin-4 water channel as a potential drug target in neurological disorders. Expert Opin. Ther. Targets 21, 1161–1170. doi: 10.1080/14728222.2017.1398236
Vorasayan, P., Bevers, M. B., Beslow, L. A., Sze, G., Molyneaux, B. J., Hinson, H. E., et al. (2019). Intravenous glibenclamide reduces lesional water uptake in large hemispheric infarction. Stroke 50, 3021–3027. doi: 10.1161/STROKEAHA.119.026036
Walcott, B. P., Miller, J. C., Kwon, C. S., Sheth, S. A., Hiller, M., Cronin, C. A., et al. (2014). Outcomes in severe middle cerebral artery ischemic stroke. Neurocrit. Care 21, 20–26. doi: 10.1007/s12028-013-9838-x
Wang, D., Liu, J., Liu, M., Lu, C., Brainin, M., and Zhang, J. (2017). Patterns of stroke between university hospitals and nonuniversity hospitals in mainland China: prospective multicenter hospital-based registry study. World Neurosurg. 98, 258–265. doi: 10.1016/j.wneu.2016.11.006
Wang, G., Huang, H., He, Y., Ruan, L., and Huang, J. (2014). Bumetanide protects focal cerebral ischemia-reperfusion injury in rat. Int. J. Clin. Exp. Pathol. 7, 1487–1494.
Wang, J., Ma, C., Zhu, J., Rao, G., and Li, H. (2020). Effect of 3-aminobenzamide on the ultrastructure of astrocytes and microvessels after focal cerebral ischemia in rats. Dose-Response 18, 1–8. doi: 10.1177/1559325819901242
Wang, Q., Deng, Y., Huang, L., Zeng, W., Chen, S., Lv, B., et al. (2019). Hypertonic saline downregulates endothelial cell-derived VEGF expression and reduces blood-brain barrier permeability induced by cerebral ischaemia via the VEGFR2/eNOS pathway. Int. J. Mol. Med. 44, 1078–1090. doi: 10.3892/ijmm.2019.4262
Wang, R., Wu, X., Liang, J., Qi, Z., Liu, X., Min, L., et al. (2015). Intra-artery infusion of recombinant human erythropoietin reduces blood-brain barrier disruption in rats following cerebral ischemia and reperfusion. Int. J. Neurosci. 125, 693–702. doi: 10.3109/00207454.2014.966354
Wang, W., Jiang, B., Sun, H., Ru, X., Sun, D., Wang, L., et al. (2017). Prevalence, incidence, and mortality of stroke in China: results from a nationwide population-based survey of 480 687 adults. Circulation 135, 759–771. doi: 10.1161/CIRCULATIONAHA.116.025250
Wang, X., Chang, Y., He, Y., Lyu, C., Li, H., Zhu, J., et al. (2020). Glimepiride and glibenclamide have comparable efficacy in treating acute ischemic stroke in mice. Neuropharmacology 162:107845. doi: 10.1016/j.neuropharm.2019.107845
Wei, X., Zhang, B., Cheng, L., Chi, M., Deng, L., Pan, H., et al. (2015). Hydrogen sulfide induces neuroprotection against experimental stroke in rats by down-regulation of AQP4 via activating PKC. Brain Res. 1622, 292–299. doi: 10.1016/j.brainres.2015.07.001
Widiapradja, A., Vegh, V., Lok, K. Z., Manzanero, S., Thundyil, J., Gelderblom, M., et al. (2012). Intravenous immunoglobulin protects neurons against amyloid beta-peptide toxicity and ischemic stroke by attenuating multiple cell death pathways. J. Neurochem. 122, 321–332. doi: 10.1111/j.1471-4159.2012.07754.x
Xu, W., Mu, X., Wang, H., Song, C., Ma, W., Jolkkonen, J., et al. (2017). Chloride co-transporter NKCC1 inhibitor bumetanide enhances neurogenesis and behavioral recovery in rats after experimental stroke. Mol. Neurobiol. 54, 2406–2414. doi: 10.1007/s12035-016-9819-0
Xu, W.-s., Sun, X., Song, C.-g., Mu, X.-p., Ma, W.-p., Zhang, X.-h., et al. (2016). Bumetanide promotes neural precursor cell regeneration and dendritic development in the hippocampal dentate gyrus in the chronic stage of cerebral ischemia. Neural Regener. Res. 11, 745–751. doi: 10.4103/1673-5374.182700
Xue, J., and Haddad, G. G. (2010). “The Na+/H+exchanger: a target for therapeutic intervention in cerebral ischemia,” in New Strategies in Stroke Intervention: Ionic Transporters, Pumps, and New Channels, ed L. Annunziato (Totowa, NJ: Humana Press), 113–128.
Yan, Y., Dempsey, R. J., Flemmer, A., Forbush, B., and Sun, D. (2003). Inhibition of Na(+)-K(+)-Cl(-) cotransporter during focal cerebral ischemia decreases edema and neuronal damage. Brain Res. 961, 22–31. doi: 10.1016/S0006-8993(02)03832-5
Yang, B., Zador, Z., and Verkman, A. S. (2008). Glial cell aquaporin-4 overexpression in transgenic mice accelerates cytotoxic brain swelling. J. Biol. Chem. 283, 15280–15286. doi: 10.1074/jbc.M801425200
Yang, J., Yang, B., Xiu, B., Qi, J., and Liu, H. (2018). Effect of combination therapy with neuroprotective and vasoprotective agents on cerebral ischemia. Can. J. Neurol. Sci. 45, 325–331. doi: 10.1017/cjn.2018.8
Yang, J., Yuan, L., Wen, Y., Zhou, H., Jiang, W., Xu, D., et al. (2020). Protective effects of naringin in cerebral infarction and its molecular mechanism. Med. Sci. Monitor 26:e918772. doi: 10.12659/MSM.918772
Yang, Y., Estrada, E. Y., Thompson, J. F., Liu, W., and Rosenberg, G. A. (2007). Matrix metalloproteinase-mediated disruption of tight junction proteins in cerebral vessels is reversed by synthetic matrix metalloproteinase inhibitor in focal ischemia in rat. J. Cereb. Blood Flow Metab. 27, 697–709. doi: 10.1038/sj.jcbfm.9600375
Yu, X., and Li, X. (2020). microRNA-1906 protects cerebral ischemic injury through activating Janus kinase 2/signal transducer and activator of transcription 3 pathway in rats. Neuroreport 31, 871–878. doi: 10.1097/WNR.0000000000001456
Zeng, X. N., Xie, L. L., Liang, R., Sun, X. L., Fan, Y., and Hu, G. (2012). AQP4 knockout aggravates ischemia/reperfusion injury in mice. CNS Neurosci. Ther. 18, 388–394. doi: 10.1111/j.1755-5949.2012.00308.x
Zeynalov, E., Jones, S. M., and Elliott, J. P. (2016). Continuous IV infusion is the choice treatment route for arginine-vasopressin receptor blocker conivaptan in mice to study stroke-evoked brain edema. J. Vis. Exp. 115:54170. doi: 10.3791/54170
Zeynalov, E., Jones, S. M., and Elliott, J. P. (2017). Therapeutic time window for conivaptan treatment against stroke-evoked brain edema and blood-brain barrier disruption in mice. PLoS ONE 12:e0183985. doi: 10.1371/journal.pone.0183985
Zhang, H.-T., Zhang, P., Gao, Y., Li, C.-L., Wang, H.-J., Chen, L.-C., et al. (2017). Early VEGF inhibition attenuates blood-brain barrier disruption in ischemic rat brains by regulating the expression of MMPs. Mol. Med. Rep. 15, 57–64. doi: 10.3892/mmr.2016.5974
Zhang, J., Bhuiyan, M. I. H., Zhang, T., Karimy, J. K., Wu, Z., Fiesler, V. M., et al. (2020a). Modulation of brain cation-Cl- cotransport via the SPAK kinase inhibitor ZT-1a. Nat. Commun. 11:78. doi: 10.1038/s41467-019-13851-6
Zhang, L., Xu, S., Wu, X., Muse, F. M., Chen, J., Cao, Y., et al. (2020b). Protective effects of the soluble epoxide hydrolase inhibitor 1-trifluoromethoxyphenyl-3-(1-propionylpiperidin-4-yl) urea in a rat model of permanent middle cerebral artery occlusion. Front. Pharmacol. 11:182. doi: 10.3389/fphar.2020.00182
Zhang, W., Neal, J., Lin, L., Dai, F., Hersey, D. P., McDonagh, D. L., et al. (2019). Mannitol in critical care and surgery over 50+ years: a systematic review of randomized controlled trials and complications with meta-analysis. J. Neurosurg. Anesthesiol. 31, 273–284. doi: 10.1097/ANA.0000000000000520
Zhang, Z. G., Zhang, L., Jiang, Q., Zhang, R., Davies, K., Powers, C., et al. (2000). VEGF enhances angiogenesis and promotes blood-brain barrier leakage in the ischemic brain. J. Clin. Invest. 106, 829–838. doi: 10.1172/JCI9369
Zhu, S., Gao, X., Huang, K., Gu, Y., Hu, Y., Wu, Y., et al. (2018). Glibenclamide enhances the therapeutic benefits of early hypothermia after severe stroke in rats. Aging Dis. 9, 685–695. doi: 10.14336/AD.2017.0927
Keywords: ischemia stroke, cerebral edema, molecular mechanism, drug therapy, preclinical drug evaluation, clinical trial
Citation: Yao Y, Zhang Y, Liao X, Yang R, Lei Y and Luo J (2021) Potential Therapies for Cerebral Edema After Ischemic Stroke: A Mini Review. Front. Aging Neurosci. 12:618819. doi: 10.3389/fnagi.2020.618819
Received: 18 October 2020; Accepted: 28 December 2020;
Published: 04 February 2021.
Edited by:
Sheng Chen, Zhejiang University, ChinaReviewed by:
Zhen Zhao, University of Southern California, Los Angeles, United StatesQi Wan, Qingdao University, China
Copyright © 2021 Yao, Zhang, Liao, Yang, Lei and Luo. This is an open-access article distributed under the terms of the Creative Commons Attribution License (CC BY). The use, distribution or reproduction in other forums is permitted, provided the original author(s) and the copyright owner(s) are credited and that the original publication in this journal is cited, in accordance with accepted academic practice. No use, distribution or reproduction is permitted which does not comply with these terms.
*Correspondence: Yonggang Zhang, jebm_zhang@yahoo.com; Xiaoyang Liao, liaoxiaoyang@wchscu.cn; Yi Lei, leiyi111@scu.edu.cn