- 1Thunderbird School of Global Management, Arizona State University Tempe Campus, Phoenix, AZ, United States
- 2Department of Bacteriology, Pasteur Institute of Iran, Tehran, Iran
- 3Department of Laboratory Medicine, School of Medical Sciences, King Khalid University, Abha, Saudi Arabia
- 4Pharmacy Department, Tishk International University, Kurdistan Region, Erbil, Iraq
- 5Medical Laboratory Technique College, The Islamic University, Najaf, Iraq
- 6Medical Laboratory Technique College, The Islamic University of Al Diwaniyah, Al Diwaniyah, Iraq
- 7Medical Laboratory Technique College, The Islamic University of Babylon, Babylon, Iraq
- 8Department of Medical Laboratory, College of Applied Medical Sciences, Prince Sattam bin Abdulaziz University, Al-Kharj, Saudi Arabia
- 9Department of Surgery, National Institute of Medical Sciences, NIMS University Rajasthan, Jaipur, India
Objectives: Streptococcus agalactiae, or group B Streptococcus (GBS), is a significant pathogen associated with severe infections in neonates, particularly sepsis and meningitis. The increasing prevalence of antibiotic resistance among GBS strains is a growing public health concern, necessitating a comprehensive meta-analysis to evaluate the prevalence of this resistance globally.
Methods: We conducted a comprehensive systematic search across four major scientific databases: Scopus, PubMed, Web of Science, and EMBASE, targeting articles published until December 13, 2023. This meta-analysis focused on studies that examined antibiotic resistance in GBS strains. The Joanna Briggs Institute tool was employed to assess the quality of the included studies. This meta-analysis applied a random-effects model to synthesize data on antibiotic resistance in GBS, incorporating subgroup analyses and regression techniques to explore heterogeneity and trends in resistance rates over time. Outliers and influential studies were identified using statistical methods such as Cook’s distance, and funnel plot asymmetry was assessed to evaluate potential publication bias. All analyses were conducted using R software (version 4.2.1) and the metafor package (version 3.8.1).
Results: This study included 266 studies from 57 countries, revealing significant variability in GBS antibiotic resistance rates. The highest resistance rates were observed for tetracycline (80.1, 95% CI: 77.1–82.8%), while tedizolid (0.1, 95% CI: 0.0–0.8%) showed the lowest resistance rates. Significant heterogeneity in resistance rates was observed, particularly for antibiotics such as azithromycin and gentamicin (I2 = 97.29%), variability across studies. On the other hand, tigecycline and ceftaroline exhibited no heterogeneity (I2 = 0%), suggesting consistent resistance patterns. Subgroup analyses revealed disparities in resistance rates based on country, continent, and methodological categories. Significant increase in resistance rates for several antibiotics over time, including clindamycin, erythromycin, ceftriaxone, cefuroxime, ciprofloxacin, levofloxacin, moxifloxacin, chloramphenicol, and ofloxacin. Ofloxacin and cefuroxime showed particularly steep trends. Conversely, a declining resistance trend was observed for oxacillin.
Conclusion: This study emphasizes the growing issue of antibiotic resistance in GBS strains. Notable resistance to older and newer antibiotics, increasing resistance over time, regional disparities, and methodological variations are noted. Rising resistance trends for multiple antibiotics underscore the urgent need for global surveillance and improved antibiotic stewardship.
Systematic Review Registration: https://www.crd.york.ac.uk/PROSPERO/view/CRD42024566269, CRD42024566269.
1 Introduction
The increasing prevalence of antibiotic resistance has emerged as a primary global public health concern, significantly complicating the management of infectious diseases across diverse populations (Murray et al., 2022; Heath and Jardine, 2014; Imperi et al., 2024; Aijaz et al., 2023). Among the myriad pathogens contributing to this crisis, group B Streptococcus (GBS) stands out due to its substantial impact on vulnerable groups, including newborns, pregnant women, and the elderly. GBS is a leading cause of severe infections in neonates, often resulting in life-threatening conditions such as sepsis, pneumonia, and meningitis (Alshamlan and Anumakonda, 2024). The timely and effective administration of antibiotics is crucial for mitigating these risks; however, the emergence of resistance to commonly prescribed antibiotics poses a formidable challenge to healthcare systems worldwide (Muteeb et al., 2023).
The treatment of GBS infections has evolved significantly with the introduction of antibiotics, shaping both clinical outcomes and bacterial resistance patterns (Sabroske et al., 2023). The first antibiotic used against GBS was penicillin, introduced in the 1940s, which revolutionized bacterial infection treatment due to its broad-spectrum activity and sustained efficacy (Aminov, 2010). Despite its continued effectiveness, alternative antibiotics such as erythromycin and clindamycin were introduced in the 1960s and 1970s to provide treatment options for penicillin-allergic patients (Solensky et al., 2000). Over time, additional drugs, including cephalosporins, vancomycin, and linezolid, were developed to combat GBS infections, particularly in cases of emerging multidrug-resistant (MDR) (Li et al., 2020). However, these antibiotics’ widespread and prolonged use has led to the gradual emergence of resistance. While GBS has largely remained susceptible to penicillin, resistance has been increasingly reported against macrolides, lincosamides, and fluoroquinolones (Oliveira et al., 2022). Notably, the growing resistance to erythromycin and clindamycin, first reported in the late 20th century, coincided with their expanded clinical use (Sabroske et al., 2023). The resistance profile of GBS has continued to evolve, highlighting a clear correlation between antibiotic pressure and bacterial adaptation (Sabroske et al., 2023). This trend highlights the need for ongoing resistance monitoring and historical analysis of antibiotic use in GBS to address treatment challenges (Laitin et al., 2024; Muteeb et al., 2023; Hooshiar et al., 2024).
Previous studies have provided valuable insights into GBS antibiotic resistance (Sabroske et al., 2023; Verma et al., 2023); however, gaps still need to be found in understanding its global epidemiology and trends. Studies on antibiotic resistance in GBS have often been limited to specific regions or antibiotics, resulting in fragmented data that fails to capture the global scope of the issue. However, recent research has uncovered a concerning pattern: GBS is increasingly resistant to widely used antibiotics like erythromycin and clindamycin. This trend has been observed across diverse regions, including Europe, North America, and South America, highlighting the growing challenge of managing GBS infections effectively (Al-Subol et al., 2022; Jones et al., 2022). Moreover, earlier meta-analyses often require more granularity to explore potential variations in resistance rates across different geographic regions and periods, which is crucial for understanding the dynamic nature of antibiotic resistance. The mechanisms underlying antibiotic resistance in GBS involve genetic mutations, horizontal gene transfer, and the acquisition of resistance genes from other bacterial species (Liu and Liu, 2022; Arnold et al., 2022). This has led to a rise in strains resistant to traditional therapies, complicating treatment protocols and threatening patient outcomes (Terreni et al., 2021). Reliance on empirical antibiotic therapy, often based on historical susceptibility patterns, may need to be revised in the face of evolving resistance profiles (Merker et al., 2020). Consequently, healthcare providers must effectively adapt their strategies to manage GBS infections (Verma et al., 2023) and use these gaps by conducting a systematic review and meta-analysis to synthesize data from diverse global sources. This study aimed to document the current resistance landscape by analyzing relevant published literature to address the lack of statistical evaluations on antibiotic resistance in GBS. Additionally, we conducted subgroup analyses based on continents, countries, antimicrobial susceptibility testing (AST) categories, bacterial diagnostic methods, and year groups to identify factors influencing resistance variations.
2 Methods
This investigation, implemented following PRISMA guidelines, integrated a meta-analysis to strengthen the outcomes. It was registered in the PROSPERO registry with the assigned code CRD42024566269.
2.1 Eligibility criteria
The eligibility criteria for incorporating articles into the meta-analysis were studies that investigated GBS, reported the proportion of resistance, determined the sample size, and published full-text articles in English. The exclusion criteria were languages other than English, case reports, single-arm studies, cohort studies, and pharmacokinetic studies.
2.2 Information sources
We extensively searched several major online databases, including Scopus, PubMed, Web of Science, and EMBASE, focusing on studies published up to December 2023. These databases were chosen for their extensive and comprehensive coverage of the biomedical literature, ensuring a broad scope for our systematic review.
2.3 Search strategy
The search syntax used for PubMed and other databases was as follows: ((“Streptococcus agalactiae OR group B strep* OR GBS OR S. agalactiae)).
The search syntax was adjusted according to each database’s guidelines (see the Supplementary material for detailed search syntax used for each database). This meticulous methodological approach aimed to cover all the necessary research topics.
2.4 Selection process
The systematic online database search results were imported into EndNote (version 20), and duplicates were removed. Two authors (SK and MB) independently searched and analyzed relevant publications to prevent bias. A third author (MH) investigated these disparities.
2.5 Data collection process
The extracted data included the first author(s), publication year, country, diagnostic method, sample source, number of positive tests, and the total number of individuals (sample size). To avoid errors in data extraction, the two authors independently extracted the necessary data and agreed on discrepant data.
2.6 Quality assessment and subgroup analysis
The Joanna Briggs Institute (JBI) tool was used to evaluate the quality of the included articles. Two authors (MB and SK) independently assessed their quality, and a third author (MH) investigated these disparities.
To assess the quality of the included studies, we performed a comprehensive risk of bias assessment based on key methodological criteria. These criteria included the clarity of sample inclusion, detailed description of study subjects and settings, standard and objective criteria for measuring the condition, identification, and management of confounding factors, validity and reliability of outcome measurements, and the appropriateness of statistical analyses.
In addition, to validate the robustness of our findings, we conducted subgroup analyses based on study quality. The studies were categorized into three quality groups: low risk (L), some concerns (S), and high risk (H).
This approach allowed us to assess the potential influence of study quality on the overall results and ensure that our conclusions were not unduly affected by studies with a higher risk of bias.
2.7 Effect measures
This meta-analysis investigated the prevalence of antibiotic resistance by analyzing the proportion of resistant isolates across various research studies. Subgroup analyses and meta-regression were employed to understand the factors contributing to the differences in resistance rates, considering variables such as country of origin. In addition, this study explored changing trends in antibiotic resistance over time.
2.8 Synthesis methods
The analysis was performed using proportions as outcome measures. This study’s primary objective was to determine the prevalence of antibiotic resistance in bacterial strains. Its secondary goal was to identify the sources of heterogeneity between the groups through subgroup analysis and regression based on country. Additionally, we investigated the trends in antibiotic resistance rates across the years.
2.9 Statistics
A random effects model was used to fit the data. The amount of heterogeneity (i.e., τ2) was estimated using the DerSimonian–Laird estimator. In addition to the estimate of τ2, the Q-test for heterogeneity and I2 statistic were reported. Any heterogeneity was detected (i.e., τ2 > 0, regardless of the results of the Q-test). Meta-regression analysis was conducted using moderator analysis to investigate the trends in antibiotic resistance rates over time. Studentized residuals and Cook’s distance were used to examine whether the studies were outliers or influential in the model context. Studies with a studentized residual larger than the 100 × (1–0.05/(2 × k))th percentile of a standard normal distribution were considered potential outliers (i.e., using a Bonferroni correction with two-sided α = 0.05, for k studies included in the meta-analysis). Studies with Cook’s distance more extensive than the median plus six times the interquartile range of Cook’s distances were considered influential. Rank correlation and regression tests using the standard error of the observed outcomes as predictors were used to check for funnel plot asymmetry. The analysis used R (version 4.2.1) and the metafor package (version 3.8.1) (DerSimonian and Laird, 1986; Cochran, 1954; Higgins and Thompson, 2002; Viechtbauer and Cheung, 2010; Begg and Mazumdar, 1994; Sterne and Egger, 2005; Viechtbauer, 2010; Kuhn et al., 2020).
2.10 Reporting bias assessment and certainty assessment
We used rank correlation and Egger’s regression tests to evaluate funnel plot asymmetry and to detect potential reporting bias. To enhance the reliability of our findings, we also applied Fail-Safe N and Trim-and-Fill methods, ensuring that our conclusions remain robust and credible despite publication biases.
3 Results
3.1 Study selection
The present investigation involved compiling 50,007 studies from four prominent online databases: Scopus, PubMed, EMBASE, and Web of Science. Subsequently, 5,551 duplicate studies were excluded from the dataset. Additionally, 7,560 studies of non-relevant study types were systematically removed to ensure precision and relevance in the analytical framework. Furthermore, an exhaustive review excluded studies explicitly related to animal subjects. Ultimately, the assessment focused on the removed titles and abstracts of 9,790 animal studies. The 27,106 remaining studies were conducted to refine the dataset and ensure its appropriateness for subsequent analysis. Subsequently, 26,840 studies that did not include the number or percentage of antibiotic-resistant isolates were excluded from the meta-analysis. The present systematic review and meta-analysis included 266 eligible studies (Abdallah et al., 2018; Abotorabi et al., 2023; Ábrók et al., 2019; Ahmad, 2015; Akpaka et al., 2022; Al Abbas et al., 2022; Al Benwan and Al Banwan, 2024; Al Romaihi et al., 2018; Al-Matary et al., 2019; Al-Subol et al., 2022; Al-Tulaibawi, 2019; Alani and AlMeani, 2022; Alemán et al., 2022; Alhhazmi et al., 2016; Ali et al., 2022; Ali et al., 2020; Alp et al., 2016; Alzayer et al., 2023; AlZuheiri et al., 2021; Asghar et al., 2020; Bae et al., 2022; Baldan et al., 2021; Balkhi et al., 2018; Belard et al., 2015; Bergal et al., 2015; Bhola et al., 2020; Biedenbach et al., 2015; Biobaku Oluwafunmilola et al., 2017; Bitew et al., 2021; Björnsdóttir et al., 2019; Bob-Manuel et al., 2021; Bolukaoto et al., 2015; Brigtsen et al., 2015; Burcham et al., 2019; Campisi et al., 2016; Carvalhaes et al., 2022; Chang et al., 2014; Cheng et al., 2020; Choi et al., 2021; Cooper et al., 2016; del Pilar Crespo-Ortiz et al., 2014; Creti et al., 2017; Dashtizade et al., 2020; de Figueiredo et al., 2021; Dehbashi et al., 2015; Dehdashtian et al., 2021; Dilrukshi et al., 2021; Dilrukshi et al., 2023; Dobrut et al., 2022; Dong et al., 2017; Doumith et al., 2017; Dube et al., 2022; Duncan et al., 2017; Dutra et al., 2014; Ebrahem et al., 2023; El Shahaway et al., 2019; El-Gendy et al., 2021; Emaneini et al., 2016; Emaneini et al., 2014; Eskandarian et al., 2015; Evangelia et al., 2015; Fahim et al., 2022; Felemban et al., 2019; Feuerschuette et al., 2022; Flamm et al., 2014; Florindo et al., 2014; Foster-Nyarko et al., 2016; Frej-Mądrzak et al., 2020; Fröhlicher et al., 2014; Fujiya et al., 2019; Gajic et al., 2019; Gao et al., 2019; Ge et al., 2021; Ghamari et al., 2022; Gharabeigi et al., 2023; Gherardi et al., 2014; Girma et al., 2020; Gizachew et al., 2018; Gizachew et al., 2020; Gogoi et al., 2021; Gomi et al., 2019; Goudarzi et al., 2015; Goudarzi and Navidinia, 2019; Graux et al., 2021; Guan et al., 2018; Guo D. et al., 2018; Guo et al., 2019; Guo et al., 2016; Guo Y. et al., 2018; Hadavand et al., 2015; Haimbodi et al., 2021; Hamad et al., 2023; Hayes et al., 2017; Hays et al., 2016; Hernandez et al., 2022; Hirai et al., 2020; Hiriote et al., 2017; Hon et al., 2020; Horn et al., 2021; Houri et al., 2017; Hsu et al., 2023a, 2023b; Hsueh, 2015; Husen et al., 2023; Ikebe et al., 2015; Ikebe et al., 2023; Iweriebor et al., 2023; Jalalifar et al., 2019; Jamrozy et al., 2020; Ji et al., 2017; Jiang et al., 2016; Jiang et al., 2017; Jiao et al., 2022; Jisuvei et al., 2020; Jones et al., 2022; Kaminska et al., 2020; Kang et al., 2017; Kao et al., 2019; Kardos et al., 2019; Karim et al., 2019; Karlowsky et al., 2014; Karlowsky et al., 2016; Karlowsky et al., 2015; Karlowsky et al., 2017; Kawaguchiya et al., 2022; Kekic et al., 2021; Kernéis et al., 2017; Khan et al., 2015; Khan et al., 2023; Khodaei et al., 2018; Kitamura et al., 2019; Ko et al., 2021; Kumalo et al., 2023; Laczeski et al., 2014; Lagunas-Rangel, 2018; Lee et al., 2022; Lee and Lai, 2015; Leykun et al., 2021; Li et al., 2018; Li G. et al., 2022; Li J. et al., 2019; Li X. et al., 2019; Li X. et al., 2022; Li et al., 2023; Li Y. et al., 2019; Liu et al., 2021; Liu et al., 2022; Liu et al., 2023; Lopes et al., 2017; Lu et al., 2016; Lu et al., 2018; Ma et al., 2021; Madrid et al., 2018; Majigo et al., 2023; Majigo et al., 2022; Malek-Jafarian et al., 2015; Malița et al., 2023; Martins et al., 2017; Matani et al., 2016; Mathur et al., 2014; de Melo et al., 2016; Mendes et al., 2015a, 2015b; Metcalf et al., 2017; Miloshevski and Miloshevska, 2015; Minotti et al., 2023; Mišić et al., 2018; Mohamed, 2023; Mohamed et al., 2020; Mohamed et al., 2024; Moltó-García et al., 2016; Morfin-Otero et al., 2015; Moroi et al., 2019; Morozumi et al., 2014; Motallebirad et al., 2021a, 2021b; Mousavi et al., 2016; Mubanga et al., 2015; Mudzana et al., 2021; Mudzikati and Dramowski, 2015; Mukesi et al., 2019; Mulu et al., 2015; Mwei et al., 2018; Nabavinia et al., 2020; Nagano et al., 2019; Newland et al., 2020; Ngom et al., 2023; Ngonzi et al., 2018; Njoku et al., 2017; Nkembe et al., 2018; Novosak et al., 2020; Numanović et al., 2017; de Oliveira Luiz et al., 2019; Ojo et al., 2019; Palacios-Saucedo et al., 2022; Panahi et al., 2023; Perim et al., 2015; Perme et al., 2020; Pfaller et al., 2016; Piccinelli et al., 2015; Piérard and Stone, 2021; Pimentel et al., 2016; Proudmore et al., 2023; Qadi et al., 2021; Qiu et al., 2021; Rasamiravaka et al., 2016; Renteria et al., 2014; Rostami et al., 2021; Saad et al., 2018; Safari et al., 2021; Saffar et al., 2016; Sahraee et al., 2019; Said et al., 2019; Santana et al., 2020; Sapugahawatte et al., 2022; Schuab et al., 2015; Shabayek and Abdalla, 2014; Shadbad et al., 2020; Shen et al., 2019; Shipitsyna et al., 2020; Shrestha et al., 2020; Sigaúque et al., 2018; Simoni et al., 2018; Slotved et al., 2021; Soares et al., 2013; Stewart et al., 2020; Størdal et al., 2022; Suhaimi et al., 2017; Sulung et al., 2023; Swann et al., 2014; Tan et al., 2022; Tang et al., 2020; Teatero et al., 2015a; Teatero et al., 2017; Teatero et al., 2015b; Tesfaye et al., 2022; Tsai et al., 2019; Tulyaprawat et al., 2021; Van Du et al., 2021; Venkatnarayan et al., 2014; Vuillemin et al., 2021; Wang et al., 2015a, 2015b; Wang S. et al., 2018; Wang X. et al., 2018; Wang Y-H. et al., 2015; Wang et al., 2014; Warrier et al., 2022; Wataradee et al., 2023; Wilkie et al., 2019; Williams et al., 2023; Woldu et al., 2014; Wu et al., 2019; Yan et al., 2016; Yayan et al., 2015; Yong et al., 2015; Yoon et al., 2015; Yu et al., 2021; Zakerifar et al., 2023; Zeng et al., 2016; Zhang et al., 2015; Zhang et al., 2021; Zhang et al., 2022; Zhang et al., 2023; Zhou et al., 2022; Zhou et al., 2023). Supplementary Table 1 presents the detailed characteristics of these studies and extracted data. The PRISMA flowchart, presented in Figure 1, summarizes the screening and selection process for the included presagers.
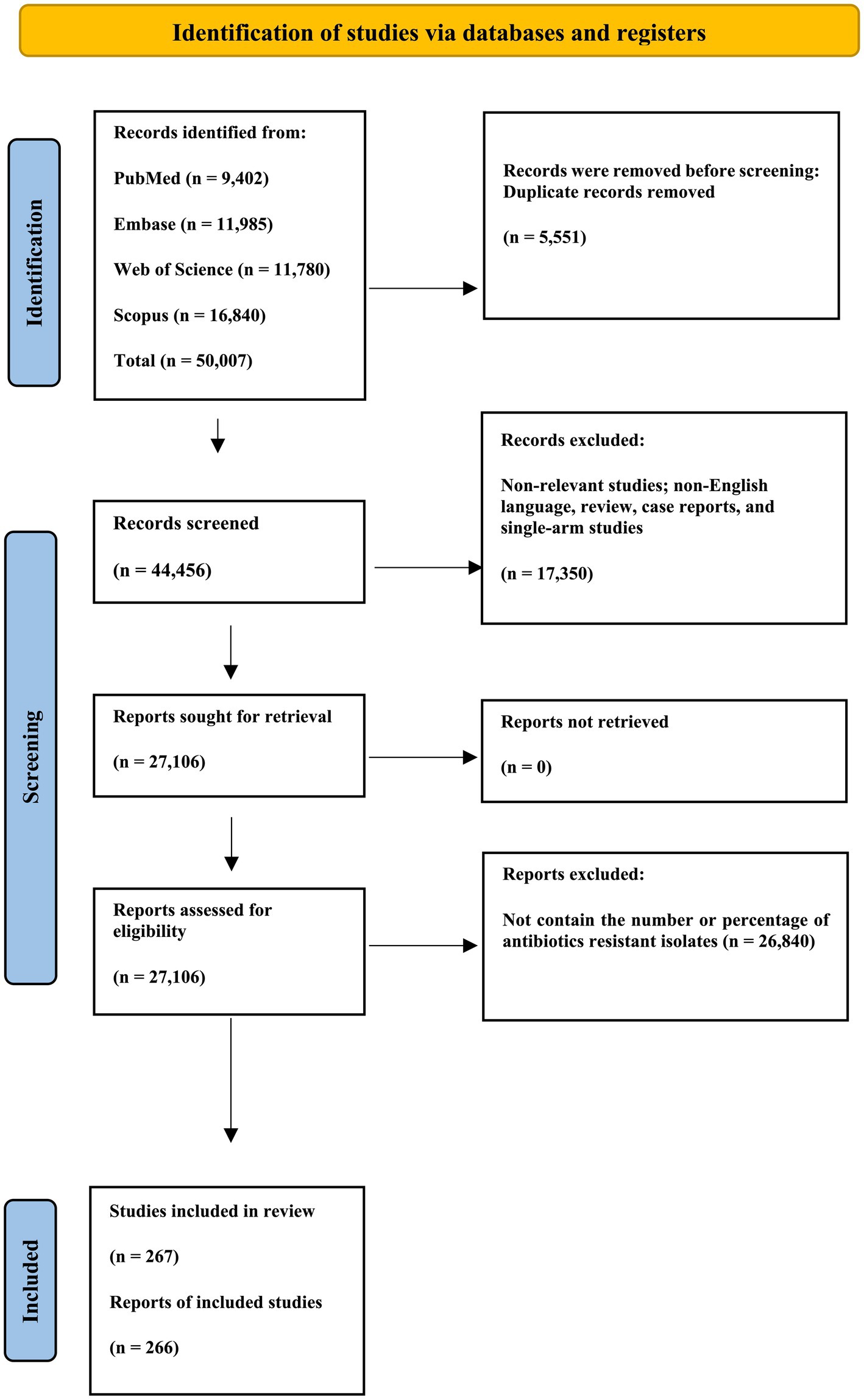
Figure 1. PRISMA flow chart of the article selection procedure. This PRISMA flow diagram illustrates identifying and selecting relevant studies for inclusion in the review. Studies were identified through a comprehensive search of PubMed, EMBASE, Web of Science, and Scopus databases. After removing duplicates and excluding irrelevant studies, 267 were included in the final review.
3.2 Study characteristics
The reports came from 57 countries (Australia, Nigeria, Iran, Japan, Serbia, Brazil, Cameroon, Portugal, Argentina, Poland, Malaysia, Taiwan, Switzerland, Norway, Indonesia, Kenya, Tanzania, Ethiopia, Bahrain, Palestinian Territories, Namibia, Bosnia & Herzegovina, Canada, Hong Kong SAR China, Unknown, Germany, Botswana, Nepal, Saudi Arabia, Italy, Ireland, Kuwait, Qatar, Thailand, Mozambique, Egypt, Syria, United States, Hungary, India, Sri Lanka, Iceland, Slovenia, Mexico, Colombia, China, France, Pakistan, Trinidad & Tobago, United Arab Emirates, Malawi, Gambia, Lesotho, South Africa, Iraq, Vietnam, Zimbabwe). Six continents [Oceania, Africa, Asia, Europe, North America, South America, and NA (Not Applicable)]. NA indicates studies that include data from multiple continents rather than being confined to a single geographic region. These studies were included in this meta-analysis. Reports cover the years from 2013 to 2023.
3.3 Results of syntheses
3.3.1 Comprehensive antibiotic-specific meta-analysis results
The proportion of penicillin resistance in 108 reports, with 979 resistant isolates among 68,461 investigated isolates, was 0.017 (0.013, 0.024), and heterogeneity between reports was not significant (I2 = 0.00%, p > 0.999). The proportion of ampicillin resistance in 63 reports, with 622 resistant isolates among 15,558 investigated isolates, was 0.031 (0.02, 0.046), and heterogeneity between reports was insignificant (I2 = 0.00%, p = 0.993). The proportion of SAM resistance through six reports, with four resistant isolates among 229 investigated isolates, was 0.043 (0.012, 0.14), and heterogeneity between reports was insignificant (I2 = 49.25%, p = 0.080). The proportion of cefazolin-resistant isolates in 11 reports, with 39 resistant isolates among the 1744 isolates, was 0.013 (0.002, 0.079), and the heterogeneity between reports was insignificant (I2 = 0.00%, p = 0.694). The proportion of clindamycin-resistant isolates among 108 reports, with 14,263 resistant isolates among 51,066 investigated isolates, was 0.293 (0.269, 0.319), and heterogeneity between reports was significant (I2 = 83.33%, p = 0.001). The proportion of erythromycin resistance in 217 reports, with 15,548 resistant isolates among 47,934 investigated isolates, was 0.35 (0.324, 0.378), and the heterogeneity between reports was significant (I2 = 95.76%, p = 0.001). The proportion of vancomycin resistance in 93 reports, with 604 resistant isolates among 45,009 investigated isolates, was 0.014 (0.01, 0.02), and the heterogeneity between reports was significant (I2 = 87.35%, p = 0.001). The proportion of ceftriaxone resistance in 77 reports, with 724 resistant isolates among 30,196 investigated isolates, was 0.062 (0.039, 0.097), and the heterogeneity between reports was significant (I2 = 91.54%, p = 0.001). The proportion of amoxicillin-resistant isolates in 10 reports, with 43 resistant isolates among the 9,837 investigated isolates, was 0.035 (0.006, 0.178), and the heterogeneity between reports was significant (I2 = 91.79%, p = 0.001). The proportion of cefuroxime resistance through 17 reports, with 53.3 resistant isolates among 4,806 investigated isolates, was 0.03 (0.012, 0.07), and heterogeneity between reports was significant (I2 = 82.72%, p = 0.001). The proportion of cefotaxime resistance through 33 reports, with 367 resistant isolates among 6,595 investigated isolates, was 0.032 (0.017, 0.06), and heterogeneity between reports was not significant (I2 = 0.00%, p > 0.999). The proportion of meropenem resistance in 13 reports, with 24 resistant isolates among 26,329 investigated isolates, was 0.007 (0.003, 0.017), and heterogeneity between reports was not significant (I2 = 0.00%, p > 0.999). The proportion of imipenem resistance through 11 reports, with 26 resistant isolates among 384 investigated isolates, was 0.065 (0.023, 0.166), and heterogeneity between reports was significant (I2 = 58.66%, p = 0.007). The proportion of azithromycin resistance in 21 reports, with 3,580 resistant isolates among 21,334 investigated isolates, was 0.41 (0.28, 0.554), and the heterogeneity between reports was significant (I2 = 97.29%, p = 0.001). The proportion of clarithromycin-resistant isolates in 11 reports, with 480 resistant isolates among the 1,468 investigated isolates, was 0.434 (0.303, 0.575), and the heterogeneity between reports was significant (I2 = 93.05%, p = 0.001). The proportion of erythromycin resistance through seven reports, with 300 resistant isolates among 554 investigated isolates, was 0.597 (0.31, 0.829), and heterogeneity between reports was significant (I2 = 96.27%, p = 0.001). The proportion of tetracycline resistance in 62 reports, with 21,931 resistant isolates among 28,322 investigated isolates, was 0.801 (0.771, 0.828), and heterogeneity between reports was significant (I2 = 80.79%, p = 0.001). The proportion of doxycycline resistance through five reports, with 188 resistant isolates among 372 investigated isolates, was 0.649 (0.371, 0.853), and heterogeneity between reports was significant (I2 = 93.66%, p = 0.001). The proportion of TMP-SMX resistant isolates in 29 reports, with 371 resistant isolates among the 5,705 investigated isolates, was 0.213 (0.107, 0.378), and the heterogeneity between reports was significant (I2 = 93.33%, p = 0.001). The proportion of ciprofloxacin resistance through 42 reports, with 502.9 resistant isolates among 3,558 investigated isolates, was 0.179 (0.127, 0.246), and heterogeneity between reports was significant (I2 = 87.16%, p = 0.001). The proportion of levofloxacin resistance in 62 reports, with 3,756 resistant isolates among 46,465 investigated isolates, was 0.086 (0.068, 0.108), and heterogeneity between reports was significant (I2 = 51.53%, p = 0.001). The proportion of gentamicin resistance through 32 reports, with 649 resistant isolates among 12,155 investigated isolates, was 0.19 (0.08, 0.389), and heterogeneity between reports was significant (I2 = 97.29%, p = 0.001). The proportion of linezolid resistance in 45 reports, with 17 resistant isolates among 18,117 investigated isolates, was 0.008 (0.006, 0.011), and heterogeneity between reports was not significant (I2 = 0.00%, p > 0.999). The proportion of daptomycin resistance in 22 reports, with four resistant isolates among 10,690 investigated isolates, was 0.003 (0.002, 0.007), and the heterogeneity between reports was insignificant (I2 = 5.23%, p = 0.390). The proportion of tigecycline-resistant isolates among the 30 reports, with zero resistant isolates among the 3,066 investigated isolates, was 0.007 (0.004, 0.012), and heterogeneity between reports was insignificant (I2 = 0.00%, p = 0.996). The proportion of nitrofurantoin-resistant isolates in the 12 reports, with 73 resistant isolates among the 627 investigated isolates, was 0.124 (0.055, 0.258), and the heterogeneity between reports was significant (I2 = 83.52%, p = 0.001). The proportion of ceftaroline resistance through four reports, with zero resistant isolates among 176 investigated isolates, was 0.012 (0.003, 0.048), and heterogeneity between reports was insignificant (I2 = 0.00%, p = 0.933). The proportion of tedizolid resistance through four reports, with zero resistant isolates among 5,213 investigated isolates, was 0.001 (0, 0.008), and heterogeneity between reports was insignificant (I2 = 49.62%, p = 0.114). The proportion of cefepime resistance in 25 reports, with 179 resistant isolates among the 5,231 investigated isolates, was 0.063 (0.027, 0.142), and heterogeneity between reports was significant (I2 = 89.76%, p = 0.001). The proportion of moxifloxacin resistance in 20 reports, with 110 resistant isolates among the 1,431 investigated isolates, was 0.063 (0.033, 0.12), and heterogeneity between reports was significant (I2 = 86.10%, p = 0.001). The proportion of oxacillin resistance in nine reports, with 41 resistant isolates among the 1,069 investigated isolates, was 0.062 (0.012, 0.261), and heterogeneity between reports was significant (I2 = 85.61%, p = 0.001). The proportion of teicoplanin resistance in eight reports, with three resistant isolates among the 1,274 investigated isolates, was 0.007 (0.003, 0.021), and heterogeneity between reports was insignificant (I2 = 0.00%, p = 0.882). The proportion of Q/D resistance in 15 reports, with 67 resistant isolates among the 1,260 investigated isolates, was 0.014 (0.003, 0.065), and heterogeneity between reports was insignificant (I2 = 0.00%, p = 0.472). The proportion of chloramphenicol resistance in 57 reports, with 500 resistant isolates among 10,245 investigated isolates, was 0.072 (0.048, 0.107), and the heterogeneity between reports was significant (I2 = 93.25%, p = 0.001). The proportion of cefditoren resistance in four reports, with 44 resistant isolates among 20,636 investigated isolates, was 0.003 (0, 0.238), and heterogeneity between reports was significant (I2 = 95.23%, p = 0.001). The proportion of norfloxacin resistance in nine reports, with 168 resistant isolates among the 865 investigated isolates, was 0.157 (0.084, 0.274), and the heterogeneity between reports was significant (I2 = 87.99%, p = 0.001). The proportion of AMC resistance in six reports, with 31 resistant isolates among the 2033 investigated isolates, was 0.196 (0.023, 0.713), and heterogeneity between reports was significant (I2 = 75.79%, p = 0.001). The proportion of cefoxitin resistance through four reports, with 14 resistant isolates among 184 investigated isolates, was 0.186 (0.031, 0.622), and heterogeneity between reports was significant (I2 = 81.58%, p = 0.001). The proportion of norfloxacin resistance in four reports, with 18 resistant isolates among the 499 investigated isolates, was 0.096 (0.006, 0.648), and the heterogeneity between reports was significant (I2 = 91.42%, p = 0.001). The proportion of ofloxacin resistance through six reports, with 98 resistant isolates among 292 investigated isolates, was 0.273 (0.049, 0.731), and heterogeneity between reports was significant (I2 = 94.61%, p = 0.001). The proportion of amikacin resistance in nine reports, with 652 resistant isolates among the 9,033 investigated isolates, was 0.196 (0.076, 0.422), and heterogeneity between reports was significant (I2 = 73.85%, p = 0.001). The proportion of nalidixic acid resistance through three reports, with 102 resistant isolates among 135 investigated isolates, was 0.749 (0.42, 0.925), and heterogeneity between reports was insignificant (I2 = 3.10%, p = 0.356).
Figure 2 shows a forest plot of the observed outcomes and the estimate based on the random-effects model. Table 1 details the antibiotic resistance patterns among GBS spp.
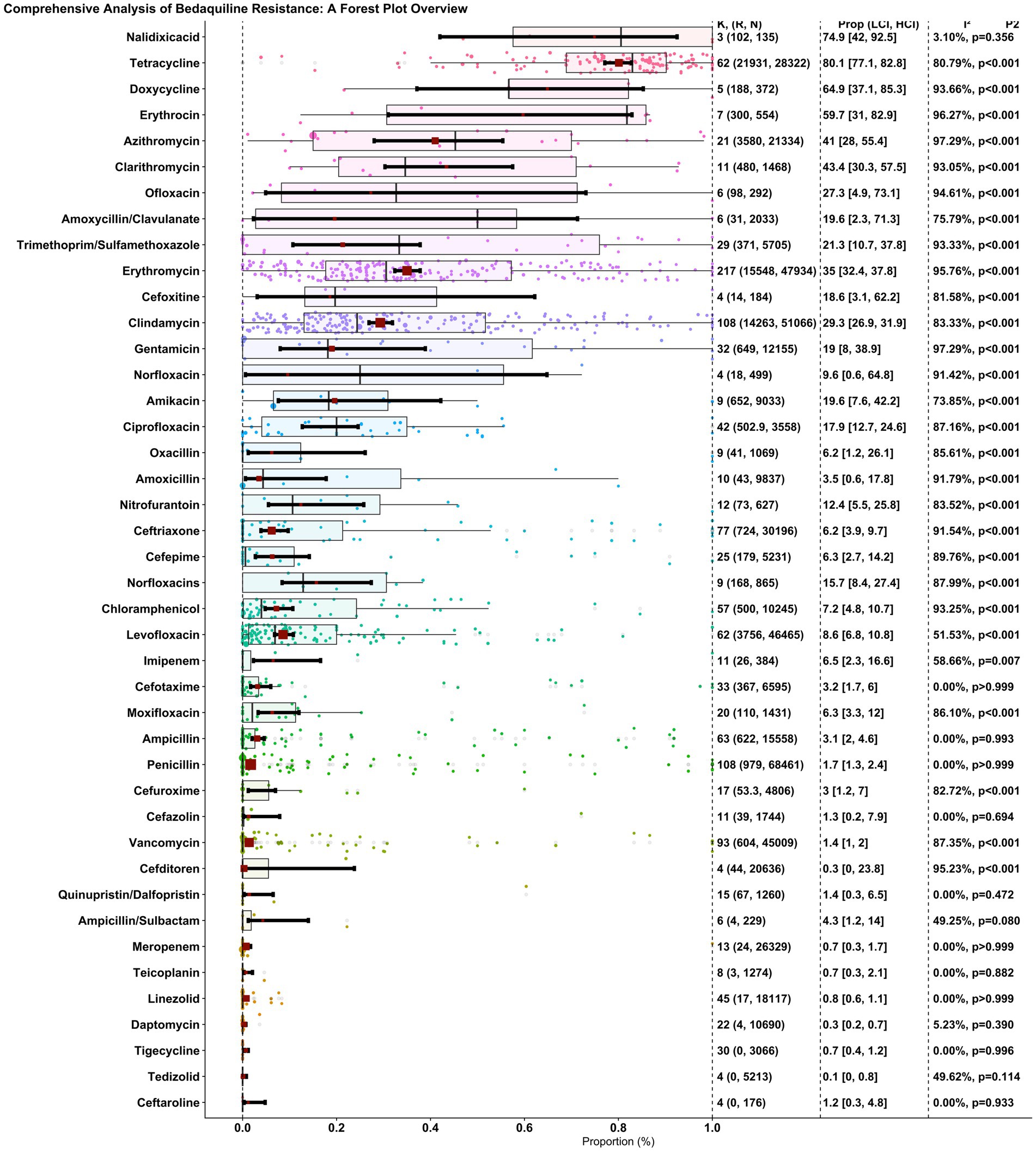
Figure 2. Overall, forest plot of the proportion of antibiotic resistance of GBS in individual studies. Each dot represents the resistance rate in a single study, with the size of the dot reflecting the sample size. The red square and error bars indicate the pooled estimate and 95% confidence interval.
3.4 Subgroup analysis
This passage offers a comprehensive overview of the subgroup analyses of antibiotic resistance. Supplementary Table 2 and Figures 3, 4 present detailed data on the subgroups, offering a complete view of resistance patterns and trends. The analyses investigated differences in resistance rates based on geography, AST methods, trends over time, and study quality.
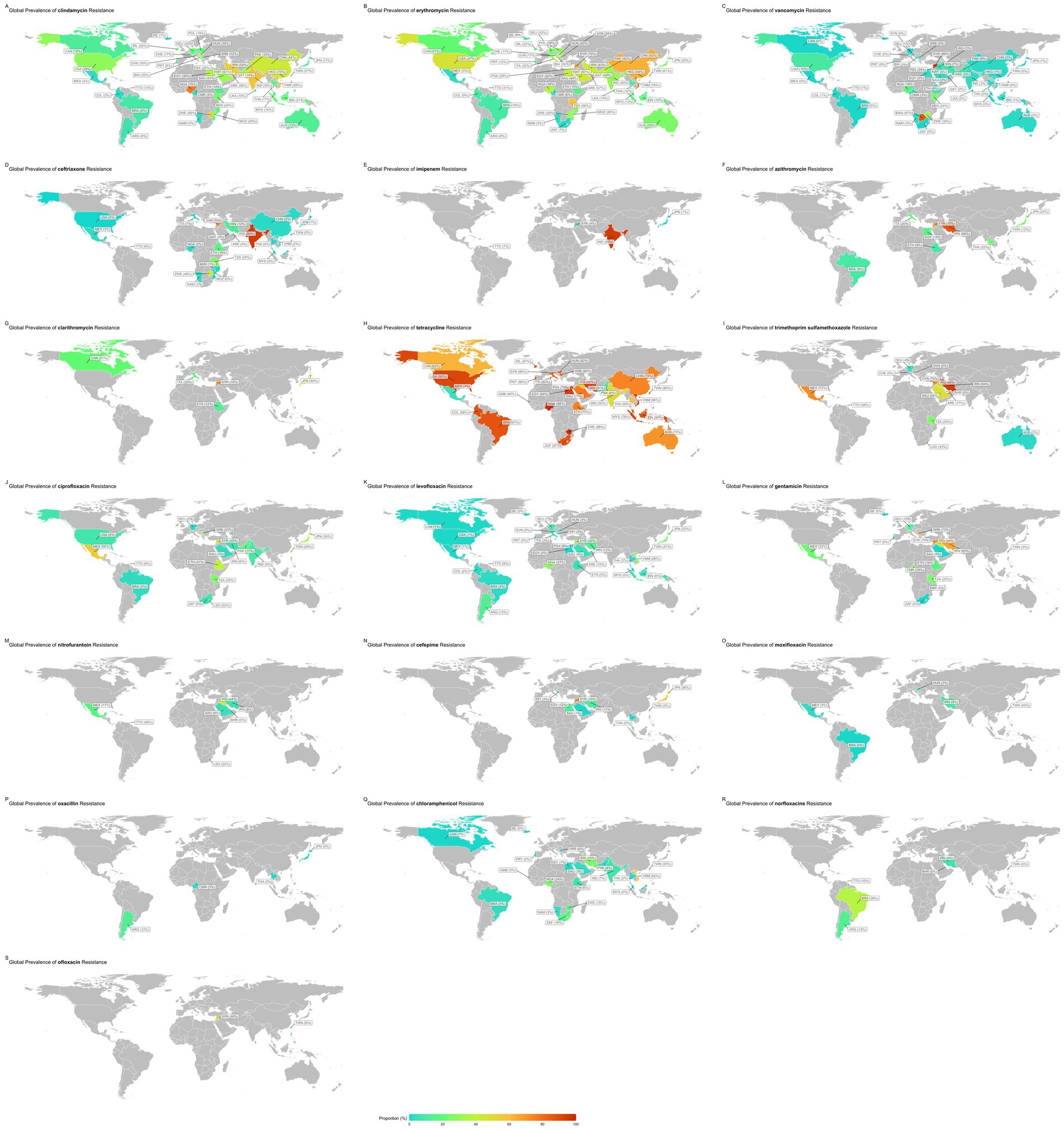
Figure 4. Subgroup analysis results of GBS isolates. (A) Compression of the prevalence of antibiotic-resistant in GBS isolates between continents. (B) Compression of the prevalence of antibiotic-resistant in GBS isolates between AST methods. (C) Compression of the prevalence of antibiotic-resistant in GBS isolates based on different bacterial diagnostic methods. (D) Compression of the prevalence of antibiotic-resistant in GBS based on years.
3.4.1 Subgroup analysis based on countries
The subgroup analysis revealed a statistically significant disparity in the prevalence of antibiotic resistance, including resistance to chloramphenicol, ciprofloxacin, clindamycin, erythromycin, gentamicin, imipenem, levofloxacin, moxifloxacin, norfloxacin, ofloxacin, tetracycline, and vancomycin. Serbia had the lowest chloramphenicol resistance rate, with a prevalence of 0.1%. Conversely, Vietnam had the highest resistance rate, with a prevalence of 52.4%.
Germany had the lowest prevalence rate of ciprofloxacin resistance, 1.5%. Conversely, Mexico had the highest resistance rate, 55.6%.
Iceland had the lowest rate of resistance to clindamycin, with a prevalence rate of 1%. Conversely, Nigeria showed the highest resistance rate (76.2%).
South Africa had the lowest erythromycin resistance rate, at 1.4%. Conversely, the country with the highest resistance rate, at 88.9%, was unknown.
Portugal has the lowest prevalence of gentamicin (0.3%). In contrast, Serbia showed the highest resistance rate (72.7%).
Japan had the lowest imipenem resistance rate, at 1.1%. Conversely, India had the highest resistance rate, at 95%.
Portugal had a 0.1% prevalence of resistance to the antibiotic levofloxacin. Conversely, Syria had the highest resistance rate, at 30%.
Brazil has the lowest resistance rate to moxifloxacin, with a prevalence rate of 0.4%. Conversely, Taiwan had the highest resistance rate, with a prevalence of 44.6%.
Bahrain had the lowest resistance rate for the antibiotic norfloxacin, with a prevalence rate of 0.4%. Conversely, Brazil showed the highest rate of resistance (37.5%).
Taiwan had the lowest prevalence rate of resistance to the antibiotic ofloxacin, at 5.8%. Conversely, Syria had the highest resistance, at 50%. Mexico had the lowest rate of resistance to tetracycline prevalence, at 4.3%. Conversely, Nigeria had the highest resistance rate, with a prevalence of 98.5%. Switzerland had the lowest vancomycin resistance rate at 0%. Conversely, Syria had the highest resistance rate, with a prevalence of 95.5% (Figure 3).
Supplementary Table 4 provides a subgroup analysis highlighting the countries with the highest and lowest resistance patterns for each antibiotic.
3.4.2 Subgroup analysis based on continents
The subgroup analysis revealed a statistically significant disparity in the prevalence of antibiotic resistance, including resistance to azithromycin, cefuroxime, chloramphenicol, clindamycin, erythromycin, levofloxacin, moxifloxacin, norfloxacin, ofloxacin, TMP-SMX, and vancomycin. For the antibiotic azithromycin, the continent with the lowest resistance rate was the Americas, with a prevalence of 9.1%. Conversely, the continent with the highest resistance rate was NA, with a prevalence of 72.2%.
Europe had the lowest resistance rate to cefuroxime, with a prevalence of 0.2%. Conversely, the continent with the highest resistance rate was the Americas, which had a prevalence of 11.7%.
Europe had the lowest prevalence of chloramphenicol use (0.4%), while Asia had the highest prevalence (14.1%).
The Americas had the lowest resistance rate to clindamycin, at 11.5%. Conversely, Asia had the highest resistance rate, at 37.7%.
For the antibiotic erythromycin, the continent with the lowest resistance rate was the Americas, which had a prevalence of 14.9%. Conversely, Asia had the highest resistance rate, with a prevalence of 43.4%. Europe had the lowest prevalence of levofloxacin (1.7%), while Asia had the highest prevalence (12.7%). The continent with the lowest resistance rate to moxifloxacin was the Americas, with a prevalence of 0.8%. Conversely, the continents with the highest resistance rates were observed in Asia, with a prevalence of 22.6%. Asia had the lowest prevalence of norfloxacin (1.1%).
Conversely, NA had the highest prevalence (29%). Asia had the lowest resistance rate to the antibiotic ofloxacin, with a prevalence rate of 11.2%. Conversely, the continent with the highest resistance rate was NA, with a prevalence of 78.4%. Europe had the lowest resistance rate to TMP-SMX, with a prevalence rate of 0.8%. Conversely, the continent with the highest resistance rate was the Americas, with a prevalence rate of 61.5%.
Europe had the lowest vancomycin prevalence (0.4%), and Africa had the highest (8.5%) (Figure 4A).
3.4.3 Subgroup analysis based on AST category
The subgroup analysis revealed a statistically significant disparity in the prevalence of antibiotic resistance to amikacin and amoxicillin. Among the various AST categories are clavulanate, ceftriaxone, ciprofloxacin, clindamycin, doxycycline, erythromycin, imipenem, TMP-SMX, and vancomycin. The disc method was the AST category with the lowest resistance to amikacin, with a prevalence rate of 11.1%. Conversely, the AST category with the highest resistance rate (99.3%) was observed in the MIC database. For the AMC, the AST category with the lowest resistance rate was the disc method, which exhibited a prevalence rate of 27.6%. Conversely, the AST category with the highest resistance rate was observed in other categories, with a prevalence rate of 97.6%.
The combination method had the lowest resistance rate for ceftriaxone, with a prevalence of 0.6%. Conversely, the disc method had the highest resistance rate, with a prevalence of 14.1%.
For ciprofloxacin, the AST category with the lowest resistance rate was MIC base, with a prevalence rate of 4.7%. Conversely, the AST category with the highest resistance rate was the disc method, with a prevalence of 24.2%.
For clindamycin, the AST category with the lowest resistance rate was MIC base, with a prevalence rate of 27.2%. Conversely, the AST category with the highest resistance rate was observed in other categories, with a prevalence rate of 46.8%.
For doxycycline, the AST category with the lowest resistance rate was the MIC, with a prevalence of 52.9%. Conversely, the AST category with the highest resistance rate (99.2%) was observed for the disc method.
For erythromycin, the AST category with the lowest resistance rate was the combination method, with a prevalence of 25.5%. Conversely, the AST category with the highest resistance rate was observed in other categories, with a prevalence rate of 52.8%.
For imipenem, the AST category with the lowest resistance rate was MIC base, with a prevalence rate of 1.1%. Conversely, the AST category with the highest resistance rate was the disc method, with a prevalence of 10.5%.
For TMP-SMX, the AST category with the lowest resistance rate was MIC, with a prevalence rate of 0.9%. Conversely, the AST category with the highest resistance rate was observed in other categories, with a prevalence rate of 74.8%.
For vancomycin, the AST category with the lowest resistance rate was MIC base, with a prevalence rate of 0.7%. Conversely, the AST category with the highest resistance rate was observed for the disc method, with a prevalence of 2.6% (Figure 4B).
3.4.4 Subgroup analysis based on different bacterial diagnostic methods
The data highlights variability in resistance rates depending on the diagnostic technique used. When culture methods were employed, resistance was notably higher for antibiotics such as ampicillin (43.7%) and amoxicillin (33.8%). Trimethoprim (30.2%) and amoxicillin-clavulanate (21%) showed significant resistance in culture-based approaches.
PCR-based diagnostics, however, revealed differing resistance rates, with certain antibiotics demonstrating lower or less reported resistance levels. When culture and serotyping or PCR were combined, resistance patterns diversified further, reflecting the sensitivity and specificity of these diagnostic techniques. The distribution underscores the influence of diagnostic methodologies on reported antibiotic resistance rates, emphasizing the importance of standardized approaches for reliable assessments (Figure 4C).
3.4.5 Subgroup analysis based on year-group
Subgroup analysis revealed a statistically significant disparity in the prevalence of antibiotic resistance, including resistance to cefditoren, ceftriaxone, clindamycin, erythromycin, levofloxacin, and moxifloxacin. For cefditoren, the year with the lowest resistance rate was 2020–2023, with a prevalence rate of 0%. Conversely, the year with the highest resistance rate was 2013–2019, with a prevalence rate of 6%.
For the antibiotic ceftriaxone, the year with the lowest resistance rate was 2013–2019, with a prevalence rate of 3.1%. Conversely, the year with the highest resistance rate was 2020–2023, with a prevalence rate of 9.7%.
For the antibiotic clindamycin, the year group with the lowest resistance rate was 2013–2019, with a prevalence rate of 24.6%. Conversely, the year with the highest resistance rate was 2020–2023, with a prevalence rate of 32.3%.
For the antibiotic erythromycin, the year group with the lowest resistance rate was 2013–2019, with a prevalence rate of 29.9%. Conversely, the year group with the highest resistance rate was observed in 2020–2023, with a prevalence rate of 38.8%.
For the antibiotic levofloxacin, the year group with the lowest resistance rate was 2013–2019, with a prevalence rate of 4.1%. Conversely, the year group with the highest resistance rate was observed in 2020–2023, with a prevalence rate of 13.1%.
For the antibiotic moxifloxacin, the year group with the lowest resistance rate was 2013–2019, with a prevalence rate of 1.8%. Conversely, the year group with the highest resistance rate was observed in 2020–2023, with a prevalence rate of 10.9% (Figure 4D).
3.4.6 Subgroup analysis based on quality group
Subgroup analysis revealed a statistically significant disparity in the prevalence of antibiotic resistance, including amikacin, cefditoren, cefepime, chloramphenicol, clindamycin, norfloxacin, and tetracycline. For amikacin, the quality group with the lowest resistance rate was low, with a prevalence rate of 9.7%. Conversely, the quality group with the highest resistance rate was observed to be at some risk, with a prevalence rate of 77.1%.
The group with the lowest resistance rate to cefditoren was at risk, with a prevalence rate of 0%. Conversely, the high-risk group had the highest resistance rate observed in high risk, with a prevalence rate of 22%. For cefepime, the quality group with the lowest resistance rate was in the low-risk category, with a prevalence rate of 4.4%. Conversely, the quality group with the highest resistance rate was observed in the Some-Risk category, with a prevalence rate of 59%.
For the antibiotic chloramphenicol, the quality group with the lowest resistance rate was low-risk, with a prevalence rate of 6%. Conversely, the quality group with the highest resistance rate was observed to be at some risk, with a prevalence of 32.9%.
For the antibiotic clindamycin, the quality group with the lowest resistance rate was at high risk, with a prevalence rate of 9.9%. Conversely, the quality group with the highest resistance rate was observed to be at some risk, with a prevalence of 34.6%.
For norfloxacin, the quality group with the lowest resistance rate was at risk, with a prevalence rate of 0.5%. Conversely, the low-risk group had the highest resistance rate observed in low risk, with a prevalence rate of 18.5%.
For the antibiotic tetracycline, the quality group with the lowest resistance rate was at high risk, with a prevalence of 7.8%. Conversely, the low-risk group had the highest resistance rate observed in low risk, with a prevalence rate of 81.8%.
3.5 Risk of bias assessment
Overall, 266 studies were assessed for methodological quality using the JBI checklist. Of the included studies, 209 (78.6%) were classified as low risk, demonstrating strong methodological rigor with minimal risk of bias. Forty-two studies (15.8%) were identified as having some concerns, indicating minor methodological limitations that may affect the reliability of findings. Additionally, 15 studies (5.6%) were classified as high risk, highlighting significant methodological weaknesses that could impact study validity and interpretation. Overall, the majority of studies exhibited a low risk of bias, ensuring a high level of confidence in the synthesized evidence. However, the presence of studies with some concerns or a high risk of bias suggests the need for careful interpretation of findings, particularly in areas where methodological limitations may have influenced results. The results of this assessment are summarized in Supplementary Table 3, which provides a detailed risk of bias evaluation for each included study.
3.6 Meta-regression
The meta-regression analysis revealed a statistically significant positive correlation between resistance rates over the years for several antibiotics, indicating an increasing trend in resistance. Notable findings include clindamycin [r = 0.07, p = 0.001, 95% CI (0.028, 0.112)], erythromycin [r = 0.08, p < 0.001, 95% CI (0.038, 0.123)], ceftriaxone [r = 0.24, p = 0.008, 95% CI (0.063, 0.417)], cefuroxime [r = 0.416, p = 0.007, 95% CI (0.116, 0.716)], ciprofloxacin [r = 0.204, p = 0.003, 95% CI (0.068, 0.339)], levofloxacin [r = 0.23, p < 0.001, 95% CI (0.145, 0.316)], moxifloxacin [r = 0.315, p = 0.034, 95% CI (0.024, 0.605)], chloramphenicol [r = 0.148, p = 0.047, 95% CI (0.002, 0.293)], and ofloxacin [r = 0.698, p < 0.001, 95% CI (0.296, 1.101)]. Among these, ofloxacin, cefuroxime, and moxifloxacin exhibited particularly steep increases in resistance over time. In contrast, oxacillin showed a statistically significant negative correlation with resistance rates over the years [r = −0.78, p = 0.040, 95% CI (−1.381, −0.034)], indicating a declining trend in resistance. These findings underscore the growing threat of antibiotic resistance, particularly for commonly used antibiotics while highlighting a rare positive trend in the decreasing resistance to oxacillin (Figure 5).
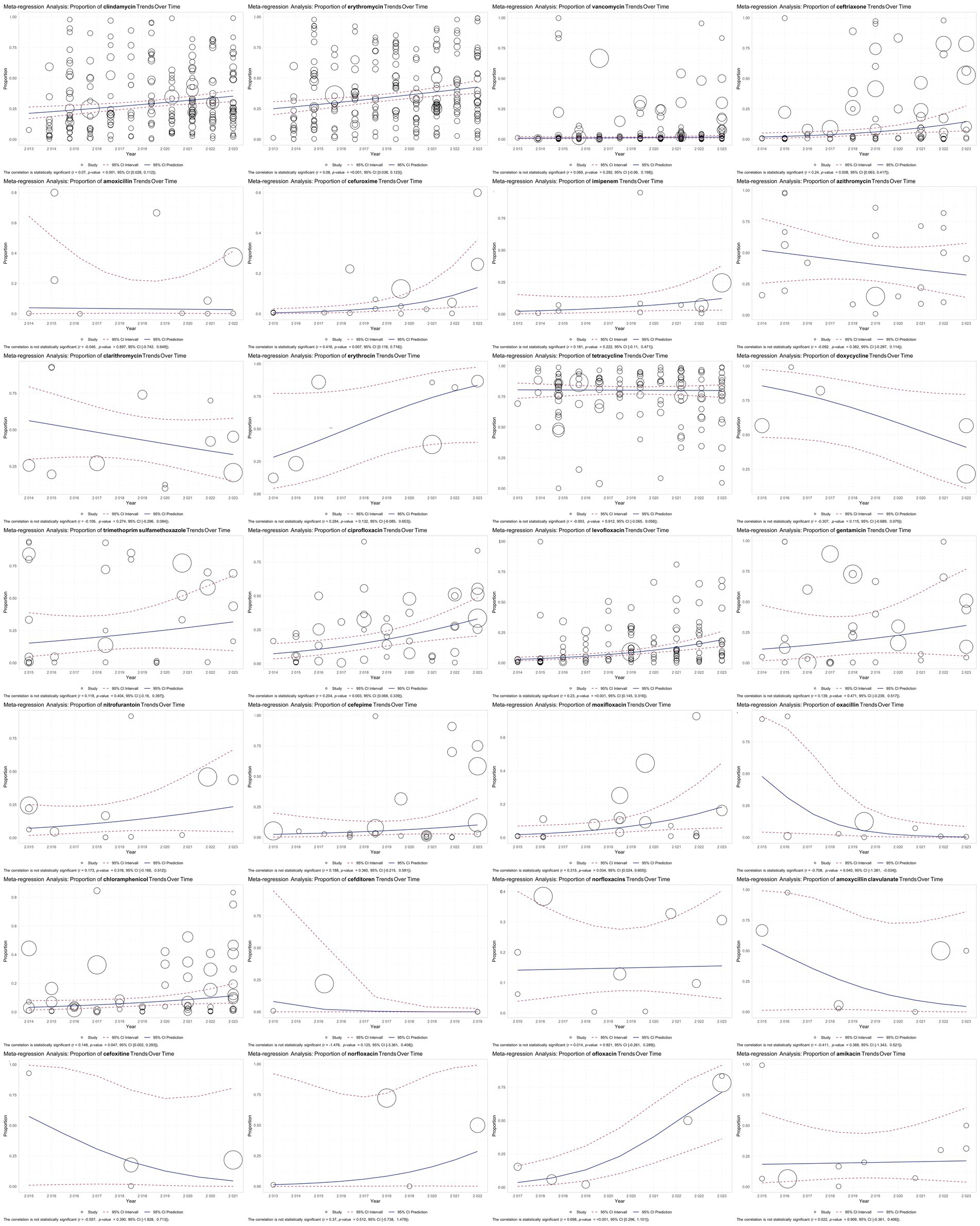
Figure 5. Meta-regression analysis of antibiotic resistance in GBS isolates over time. The results are visualized in scatter plots, illustrating the trend in the proportion of antibiotic-resistant GBS isolates over the years. The analysis revealed significant increases in resistance for several antibiotics, including clindamycin, erythromycin, ceftriaxone, cefuroxime, ciprofloxacin, levofloxacin, moxifloxacin, chloramphenicol, and ofloxacin, with notably steep increases in cefuroxime, moxifloxacin, and ofloxacin. Conversely, oxacillin showed a significant decrease in resistance over time.
3.7 Publication bias assessment
The Egger and Begg tests were employed to assess funnel plot asymmetry and rank correlation, which indicate publication bias.
For antibiotics such as tetracycline (p = 0.055) and doxycycline (p < 0.001), the Egger test indicated varying levels of potential publication bias, with doxycycline suggesting more substantial evidence of bias due to its p-value below 0.05. The Begg test, however, yielded p-values above 0.05 for both antibiotics, suggesting less consistent evidence of bias. Similarly, ciprofloxacin (p = 0.040) and levofloxacin (p < 0.001) showed low p-values in the Egger test, supporting the likelihood of bias, while the Begg test was more mixed in its findings. The “Fail-Safe N” values were substantial for tetracycline (141,092) and levofloxacin (185,807), highlighting robust findings, whereas ciprofloxacin (5,542) and doxycycline (0) demonstrated lower thresholds.
For macrolides like azithromycin (p = 0.047) and clarithromycin (p = 0.084), the Egger test suggested borderline evidence of publication bias, while the Begg test returned higher p-values, indicating weaker support for bias. The “Fail-Safe N” values were moderate for azithromycin (Aijaz et al., 2023; Mohamed et al., 2024) and clarithromycin (Piccinelli et al., 2015), suggesting fewer studies would be needed to overturn the observed results. The “Trim and Fill” analysis adjusted the effect sizes to 0.410 for azithromycin and 0.434 for clarithromycin, reflecting the estimated true effect sizes after correcting for potential bias.
Other antibiotics, such as tigecycline (p = 0.003) and daptomycin (p = 0.025), also showed significant Egger test results, suggesting potential bias, with moderate “Fail-Safe N” values of 4,002 and 3,588, respectively. Their “Trim and Fill” adjusted effect sizes were 0.007 and 0.011, indicating minimal impact after correcting for bias.
Lastly, for agents like nitrofurantoin (p = 0.006) and cefepime (p = 0.007), the Egger test revealed significant results, while the Begg test returned mixed findings. The “Fail-Safe N” values were relatively low for nitrofurantoin (Mwambia, 2021) and moderate for cefepime (2,095), reflecting varying levels of robustness. Their adjusted effect sizes under Trim and Fill were 0.150 and 0.118, providing insights into the corrected estimates after addressing bias. Table 2 and Supplementary Figure 1 summarize antibiotic resistance trends in GBS isolates, combining detailed statistical analysis with visual representation to highlight resistance patterns and assess the robustness of the findings.
4 Discussion
GBS, known as Streptococcus agalactiae, is a significant pathogen in neonates, pregnant women, and immunocompromised individuals (Shrestha et al., 2020). It can cause severe infections such as sepsis, pneumonia, meningitis in newborns and various invasive diseases in adults (Lin et al., 2021). Antibiotic resistance in GBS infections is an increasing concern in clinical settings (Hayes et al., 2020). Penicillin and ampicillin are the primary antibiotics used to treat GBS infections (Alotaibi et al., 2023). Although these drugs are highly effective, resistance to them remains rare. However, alternative antibiotics, such as erythromycin and clindamycin, are used for individuals allergic to penicillin (Nadeau and Edwards, 2019).
The resistance profile of GBS to various antibiotics is a critical consideration in treatment strategies (Di Renzo et al., 2015). Studies have shown that while GBS is resistant to certain antibiotics, such as gentamicin, erythromycin, and clindamycin, it remains susceptible to essential drugs, such as penicillin, cefuroxime, cefotaxime, and vancomycin (Mwambia, 2021; Kasem et al., 2024). This information is crucial for selecting appropriate antibiotics for managing GBS infections, ensuring effective treatment, and reducing the risk of complications (Alotaibi et al., 2023). The emergence of antibiotic-resistant strains, especially pathogens such as GBS, underscores the need for continuous monitoring and surveillance to track resistance patterns and inform clinical practice (Yang et al., 2024). This systematic review and meta-analysis provide a comprehensive overview of the antibiotic resistance rates of GBS isolates to various antibiotics, highlighting significant findings and trends.
First, this systematic review and meta-analysis successfully identified 334 eligible studies from 57 countries across six continents, representing a substantial and diverse dataset. Comprehensive geographical coverage underscores the global nature of GBS. The variations in antibiotic resistance rates across different regions and countries highlight the importance of localized strategies for combating antibiotic resistance, considering regional factors such as healthcare practices, antibiotic use, and socioeconomic conditions (Coque et al., 2023). The use of standards for antimicrobial susceptibility testing is crucial for this discussion.
Key findings indicated that resistance rates for penicillin and ampicillin were meager at 1.7 and 3.1%, respectively, with no significant heterogeneity observed. This finding reinforces the continued effectiveness of antibiotics for GBS. Similarly, vancomycin and linezolid showed low resistance rates (1.4 and 0.8%, respectively), suggesting their reliability for treating GBS infections. Meropenem and daptomycin also demonstrated resistance rates of 0.7 and 0.3%, respectively, indicating their strong efficacy against GBS.
Moderate resistance rates of 6.2 and 6.3% were observed for ceftriaxone and cefepime, respectively, along with significant heterogeneity, indicating varied efficacy across different regions or settings. Clindamycin and erythromycin, however, showed notably high resistance rates of 29.3 and 35%, respectively, with significant heterogeneity, raising concerns regarding their reliability in treating GBS infections. Tetracycline and doxycycline exhibited extremely high resistance rates of 80.1 and 64.9%, respectively, with significant heterogeneity, suggesting the limited utility of these antibiotics in treating GBS infections. Similarly, azithromycin and clarithromycin showed high resistance rates (41 and 43.4%, respectively), indicating widespread resistance.
Subgroup analyses have provided valuable insights into the evolving landscape of antibiotic resistance in GBS. The variations observed based on year groups, continental locations, countries, detection methods, antimicrobial susceptibility test guidelines, and risk of bias assessment demonstrated the dynamic nature of the resistance problem.
This comprehensive review and subgroup analysis emphasizes the complex and multifaceted nature of antibiotic resistance in GBS. These findings highlight the importance of localized data, standardized testing methods, and high-quality research in understanding and addressing antibiotic resistance. Effective management of GBS infections requires ongoing surveillance, tailored antibiotic stewardship programs, and continuous adaptation of treatment guidelines based on the latest evidence. By addressing these challenges, healthcare providers can better manage GBS infections and mitigate the impact of antibiotic resistance on public health.
Subgroup analysis revealed significant geographical variations in antibiotic resistance rates for GBS, underscoring the importance of local epidemiological data in informing treatment guidelines and antibiotic stewardship programs. The resistance rates for clindamycin, erythromycin, and tetracycline showed marked differences across regions.
The significant geographical variations in antibiotic resistance rates suggest that local factors, such as antibiotic prescription practices, public health policies, and access to healthcare resources, play crucial roles in shaping resistance patterns (Felemban et al., 2019; Iweriebor et al., 2023). Studies have shown that differences in antibiotic prescribing practices across regions contribute to varying resistance rates; for instance, countries with stringent antibiotic regulations and robust healthcare infrastructure often report lower resistance levels, whereas those with lax policies and limited healthcare resources face higher resistance challenges (Felemban et al., 2019; Iweriebor et al., 2023).
Significant heterogeneity was observed for several antibiotics, including clindamycin, erythromycin, and tetracycline. This variation suggests that regional antibiotic use policies and differences in bacterial strains may significantly influence resistance patterns. For example, research has demonstrated that GBS strains exhibit varying resistance levels depending on local prescribing habits and public health policies, which can contribute to increased resistance rates (Wang S. et al., 2018; Hsu et al., 2021). Furthermore, antibiotic resistance tends to be higher in regions with poor healthcare access and inadequate regulation of antibiotic sales, leading to self-medication and misuse (Chen et al., 2023; Campisi et al., 2016). Therefore, addressing local antibiotic use policies and strengthening healthcare infrastructure is essential for controlling GBS resistance and ensuring effective treatment options.
Furthermore, the high heterogeneity (I2 values) observed across multiple antibiotics indicates substantial variability among the included studies, reflecting differences in study methodologies, geographic distribution, and temporal trends in GBS resistance. One key source of heterogeneity stems from variations in AST methods, including differences in breakpoints, testing protocols, and regional laboratory standards. Furthermore, microbiological diagnostic approaches may vary, with some studies relying solely on culture-based methods while others incorporate molecular techniques such as PCR for pathogen identification and resistance gene detection. Studies that combine culture with serotyping or culture with PCR-based detection may introduce further variability in resistance estimates. Additionally, geographic differences in antibiotic prescribing practices and selective pressure, along with temporal shifts in resistance trends, likely contribute to the observed heterogeneity. Addressing these methodological discrepancies through standardized protocols and region-specific analyses is crucial for improving the comparability and interpretability of resistance data in GBS research.
Subgroup analysis revealed geographical variations in resistance rates, with clindamycin resistance being the lowest in Iceland (1%) and highest in Nigeria (76.2%), which could be attributed to higher usage rates, over-the-counter availability, and lack of stringent antibiotic policies, emphasizing the importance of local surveillance and tailored antibiotic stewardship programs. Erythromycin resistance also varied, being the lowest in South Africa (1.4%), possibly reflecting stringent control measures and targeted antibiotic use. An unspecified region reported a resistance rate as high as 88.9%, indicating severe misuse or overuse of erythromycin and the necessity for immediate public health interventions and highest in the unspecified regions (88.9%).
Continental differences were noted, with Asia showing the highest resistance rates to several antibiotics, including erythromycin (43.4%) and clindamycin (37.7%), compared to lower rates in the Americas and Europe. This suggests that broader regional factors, such as healthcare infrastructure and antibiotic use policies, might affect resistance patterns and the need for enhanced antibiotic stewardship and surveillance programs to curb the rise of resistance.
The observed differences in resistance rates based on AST methods highlight the importance of methodological standardization. Using reliable and validated testing methods consistently ensures accurate resistance data, critical for effective clinical decision-making and public health strategies.
MIC-based methods reported an extremely high resistance rate to amikacin (99.3%) compared to disc methods (11.1%), highlighting the importance of standardized testing methods to ensure consistent and reliable data. These results show that MIC methods might be more sensitive for detecting resistant strains.
Similar variations were noted for other antibiotics, underscoring the need for standardized testing protocols to ensure consistent and reliable resistance data across different laboratories and studies.
Recent studies (2020–2023) have generally reported higher resistance rates for several antibiotics, including ceftriaxone (9.7%) and clindamycin (32.3%). This indicates a potentially increasing trend in the resistance.
Temporal trends indicate an increase in resistance rates for several antibiotics over recent years (2020–2023), including ceftriaxone (9.7%) and clindamycin (32.3%). This reflects a worrying upward trend in GBS resistance and underscores the need for ongoing surveillance and updated treatment guidelines.
The quality of the studies also affected the reported resistance rates. Studies categorized as “high risk” often reported higher resistance rates. For instance, tetracycline resistance was highest in low-risk studies (81.8%), suggesting that study quality can impact the reported resistance rates.
Variations in study quality underscore the need for high methodological standards in the research. High-quality studies provide reliable data for accurately assessing the burden of antibiotic resistance and informing effective interventions.
Given the observed antibiotic resistance trends in GBS, it is crucial to consider the clinical implications for treatment guidelines and healthcare policies. The emergence of MDR strains underscores the need for updated treatment protocols, particularly for high-risk populations such as neonates, pregnant women, and the elderly. Clinicians should be guided by more dynamic, region-specific susceptibility data rather than relying solely on historical resistance patterns. Additionally, antibiotic stewardship programs should be strengthened to reduce unnecessary antibiotic use and slow the progression of resistance. Policies should focus on regular surveillance and implementing rapid diagnostic tools, including PCR-based methods, to ensure timely and appropriate treatment. Developing new antibiotics and alternatives to current therapies should also be prioritized to address the growing challenge of multidrug resistance in GBS infections.
Despite the rigorous methodology employed in this study, several limitations should be acknowledged. One key limitation is the potential influence of publication bias, even though we assessed it using funnel plots and statistical tests. Studies with negative or inconclusive results are less likely to be published, which may lead to overestimating antibiotic resistance rates in GBS. This selective reporting bias could distort the true resistance landscape, as studies showing lower resistance rates or no significant trends might be underrepresented.
Additionally, missing or unpublished data may result in geographic disparities, particularly in regions with limited surveillance studies or where only certain resistance profiles are reported. The exclusion of smaller studies or those published in non-indexed journals further contributes to potential data gaps. Variability in study methodologies, including differences in AST protocols and diagnostic approaches (culture-based vs. PCR-based methods), may also introduce heterogeneity in the findings.
Furthermore, while we performed subgroup analyses based on study quality, the risk of bias in included studies may still affect the overall conclusions. Some studies lacked detailed reporting on key methodological aspects, such as sample selection criteria and control for confounding variables, which could influence resistance estimates. Future research should prioritize standardized surveillance methodologies and explore strategies such as gray literature searches, data sharing initiatives, and the inclusion of preprint studies to mitigate publication bias. Expanding resistance monitoring programs across diverse geographic regions and clinical settings will be essential to ensure a more comprehensive and globally representative understanding of GBS antibiotic resistance trends.
The variability of GBS resistance patterns across different continents and even within various regions of the same country is an essential limitation of this study. Several factors, including differences in local antimicrobial use, regional healthcare practices, and pathogen characteristics, may influence this variability. Our data represent a broad overview of global resistance patterns, but these patterns may not be universally applicable. We recommend that future studies explore regional variations in more detail, as this could provide valuable insights into the local drivers of resistance and support the development of region-specific treatment strategies.
5 Conclusion
In conclusion, this study highlighted the varying resistance patterns of GBS across different antibiotics, regions, and testing methods. The low resistance rates to penicillin, ampicillin, vancomycin, and linezolid suggest that these remain effective treatments for GBS infection. However, the high resistance rates to clindamycin, erythromycin, and tetracycline necessitate cautious use and consideration of local resistance patterns when choosing treatment options. This systematic review and meta-analysis, encompassing data from 334 studies across 57 countries, revealed significant geographical variations and trends, underscoring the need for localized antibiotic stewardship programs and continuous global surveillance to combat antibiotic resistance effectively. Increasing antibiotic resistance in GBS infections poses a significant challenge, particularly for neonates, pregnant women, and immunocompromised individuals. In recent years, the increasing trend in resistance rates has highlighted the urgent need for continuous surveillance, high-quality research, and adaptive treatment guidelines. Future research should focus on standardizing AST methods and improving study quality to ensure more reliable and comparable data across regions and periods, ultimately enhancing our ability to manage GBS infections and mitigate the impact of antibiotic resistance on public health.
Data availability statement
The original contributions presented in the study are included in the article/Supplementary material. Further inquiries can be directed to the corresponding authors.
Author contributions
C-YH: Investigation, Supervision, Writing – original draft. SM: Investigation, Visualization, Writing – original draft. MS: Investigation, Methodology, Writing – original draft. SU: Formal analysis, Writing – original draft, Writing – review & editing. AZ: Data curation, Writing – original draft. AH: Visualization, Writing – original draft. RV: Methodology, Writing – original draft, Writing – review & editing. MB: Conceptualization, Data curation, Formal analysis, Writing – original draft, Writing – review & editing.
Funding
The author(s) declare that no financial support was received for the research and/or publication of this article.
Acknowledgments
The authors express their gratitude to the Deanship of Scientific Research at King Khalid University.
Conflict of interest
The authors declare that the research was conducted in the absence of any commercial or financial relationships that could be construed as a potential conflict of interest.
Generative AI statement
The authors declare that no Gen AI was used in the creation of this manuscript.
Publisher’s note
All claims expressed in this article are solely those of the authors and do not necessarily represent those of their affiliated organizations, or those of the publisher, the editors and the reviewers. Any product that may be evaluated in this article, or claim that may be made by its manufacturer, is not guaranteed or endorsed by the publisher.
Supplementary material
The Supplementary material for this article can be found online at: https://www.frontiersin.org/articles/10.3389/fmicb.2025.1541524/full#supplementary-material
References
Abdallah, M. S., Philemon, R., Kadri, A., Al-Hinai, A., Saajan, A. M., Gidabayda, J. G., et al. (2018). Prevalence, aetiological agents, and antimicrobial sensitivity pattern of bacterial meningitis among children receiving care at KCMC referral hospital in Tanzania. East Afr. Health Res. J. 2, 1–9. doi: 10.24248/eahrj.v2i1.560
Abotorabi, S., Rasooli, Z., Pakniat, H., and Baloo, F. (2023). Virulence determinants, serotypes, and antimicrobial resistance of rectovaginal isolates of Streptococcus agalactiae. Iran. J. Pathol. 18, 82–89. doi: 10.30699/ijp.2023.559137.2943
Ábrók, M., Tigyi, P., Kostrzewa, M., Burián, K., and Deák, J. (2019). Evaluation of the results of group B Streptococcus screening by MALDI-TOF MS among pregnant women in a Hungarian hospital. Pathogens 9:1. doi: 10.3390/pathogens9010001
Ahmad, S. (2015). Asymptomatic group B streptococcal bacteriuria among pregnant women in Saudi Arabia. Br. J. Biomed. Sci. 72, 135–139. doi: 10.1080/09674845.2015.11666810
Aijaz, M., Ahmad, M., Ansari, M. A., and Ahmad, S. (2023). Antimicrobial resistance in a globalized world: current challenges and future perspectives. Int. J. Pharm. Drug Des. 1, 7–22.
Akpaka, P. E., Henry, K., Thompson, R., and Unakal, C. (2022). Colonization of Streptococcus agalactiae among pregnant patients in Trinidad and Tobago. IJID Reg. 3, 96–100. doi: 10.1016/j.ijregi.2022.03.010
Al Abbas, L. A., Khaddam, W., and Shreibati, F. (2022). Investigating bacteria isolated from diabetic foot ulcers and studying their sensitivity to antibiotics—Syria. Bull. Pharm. Sci. 45, 451–458. doi: 10.21608/bfsa.2022.239586
Al Benwan, K., and Al Banwan, D. (2024). Microbiological profiles and inflammatory biomarkers of bacteremia in children in a teaching Hospital in Kuwait: an 8-year retrospective Study. Med. Princ. Pract. 33, 21–30. doi: 10.1159/000534716
Al Romaihi, E., Alkhawaja, S., Saeed, N. K., Barakat, A. M., El Moez Azam, N. F. A., and Haji, S. (2018). Prevalence of antimicrobial resistance in uropathogens among patients visiting primary health centers: implications for empiric therapy. Bahrain Med. Bull. 40, 207–211.
Alani, S. N., and AlMeani, S. A. L. (2022). Inhibition of Streptococcus Agalactiae biofilm formation in response to purified phytochemical antimicrobial materials. J. Pharm. Negat. Results 13, 601–607. doi: 10.47750/pnr.2022.13.04.079
Alemán, T., Vielot, N. A., Herrera, R., Velasquez, R., Berrios, T., Toval-Ruíz, C., et al. (2022). Rectovaginal colonization with serotypes of group B streptococci with reduced penicillin susceptibility among pregnant women in León, Nicaragua. Pathogens 11:415. doi: 10.3390/pathogens11040415
Alhhazmi, A., Hurteau, D., and Tyrrell, G. J. (2016). Epidemiology of invasive group B streptococcal disease in Alberta, Canada, from 2003 to 2013. J. Clin. Microbiol. 54, 1774–1781. doi: 10.1128/JCM.00355-16
Ali, M., MAA, A., Ali, G., Alzubaidi, K., Ali, B., Ismail, A., et al. (2022). Microbiological and clinical characteristics of invasive group B streptococcal blood stream infections in children and adults from Qatar. BMC Infect. Dis. 22:881. doi: 10.1186/s12879-022-07801-9
Ali, M. M., Woldeamanuel, Y., Asrat, D., Fenta, D. A., Beall, B., Schrag, S., et al. (2020). Features of Streptococcus agalactiae strains recovered from pregnant women and newborns attending different hospitals in Ethiopia. BMC Infect. Dis. 20:848. doi: 10.1186/s12879-020-05581-8
Al-Matary, A., Heena, H., AlSarheed, A. S., Ouda, W., AlShahrani, D. A., Wani, T. A., et al. (2019). Characteristics of neonatal sepsis at a tertiary care hospital in Saudi Arabia. J. Infect. Public Health 12, 666–672. doi: 10.1016/j.jiph.2019.03.007
Alotaibi, N. M., Alroqi, S., Alharbi, A., Almutiri, B., Alshehry, M., Almutairi, R., et al. (2023). Clinical characteristics and treatment strategies for group B Streptococcus (GBS) infection in pediatrics: a systematic review. Medicina 59:1279. doi: 10.3390/medicina59071279
Alp, F., Findik, D., Dagi, H. T., Arslan, U., Pekin, A. T., and Yilmaz, S. A. (2016). Screening and genotyping of group B Streptococcus in pregnant and non-pregnant women in Turkey. J. Infect. Dev. Countries 10, 222–226. doi: 10.3855/jidc.6190
Alshamlan, A., and Anumakonda, V. (2024). A review of group B Streptococcus (GBS) in young adults: emerging concerns beyond the neonatal population. J. Med. Clin. Case Rep. 1. doi: 10.61615/JMCCR/2024/AUG027140817
Al-Subol, I., Abdul-Aziz, M., Almikhlafy, A. A., and Alqahtani, T. (2022). An initial survey on the prevalence of group B Streptococcus (GBS) among Yemeni pregnant women in Sana’a city. Infect. Dis. Obstet. Gynecol. 2022:6279343. doi: 10.1155/2022/6279343
Al-Tulaibawi, N. A. J. (2019). Prevalence and sensitivity of bacterial urinary tract infection among adult diabetic patients in Misan Province, Iraq. J. Pure Appl. Microbiol. 13, 847–853. doi: 10.22207/JPAM.13.2.20
Alzayer, M., Alkhulaifi, M. M., Alyami, A., Aldosary, M., Alageel, A., Garaween, G., et al. (2023). Molecular typing and antimicrobial resistance of group B Streptococcus clinical isolates in Saudi Arabia. J. Glob. Antimicrob. Resist. 35, 244–251. doi: 10.1016/j.jgar.2023.10.007
AlZuheiri, S. T. S., Dube, R., Menezes, G., and Qasem, S. (2021). Clinical profile and outcome of group B streptococcal colonization in mothers and neonates in Ras Al Khaimah, United Arab Emirates: a prospective observational study. Saudi J.Med. Med. Sci. 9, 235–240. doi: 10.4103/sjmms.sjmms_213_21
Aminov, R. I. (2010). A brief history of the antibiotic era: lessons learned and challenges for the future. Front. Microbiol. 1:134. doi: 10.3389/fmicb.2010.00134
Arnold, B. J., Huang, I.-T., and Hanage, W. P. (2022). Horizontal gene transfer and adaptive evolution in bacteria. Nat. Rev. Microbiol. 20, 206–218. doi: 10.1038/s41579-021-00650-4
Asghar, S., Khan, J. A., Mahmood, M. S., and Arshad, M. I. (2020). A cross-sectional study of group B Streptococcus–associated sepsis, coinfections, and antibiotic susceptibility profile in neonates in Pakistan. Adv. Neonatal Care 20, E59–E69. doi: 10.1097/ANC.0000000000000701
Bae, H. G., Hong, J., Kim, Y.-J., Lee, K.-R., Lee, K., Choi, S. J., et al. (2022). A retrospective national study on colonization rate and antimicrobial susceptibility of Streptococcus agalactiae in pregnant Korean women, 2018–2020. Yonsei Med. J. 63, 717–723. doi: 10.3349/ymj.2022.63.8.717
Baldan, R., Droz, S., Casanova, C., Knabben, L., Huang, D. J., Brülisauer, C., et al. (2021). Group B streptococcal colonization in elderly women. BMC Infect. Dis. 21:408. doi: 10.1186/s12879-021-06102-x
Balkhi, B., Mansy, W., Alghadeer, S., Alnuaim, A., Alshehri, A., and Somily, A. (2018). Antimicrobial susceptibility of microorganisms causing urinary tract infections in Saudi Arabia. J. Infect. Dev. Countries 12, 220–227. doi: 10.3855/jidc.9517
Begg, C. B., and Mazumdar, M. (1994). Operating characteristics of a rank correlation test for publication bias. Biometrics 50, 1088–1101. doi: 10.2307/2533446
Belard, S., Toepfner, N., Capan-Melser, M., Mombo-Ngoma, G., Zoleko-Manego, R., Groger, M., et al. (2015). Streptococcus agalactiae serotype distribution and antimicrobial susceptibility in pregnant women in Gabon, Central Africa. Sci. Rep. 5:17281. doi: 10.1038/srep17281
Bergal, A., Loucif, L., Benouareth, D., Bentorki, A., Abat, C., and Rolain, J.-M. (2015). Molecular epidemiology and distribution of serotypes, genotypes, and antibiotic resistance genes of Streptococcus agalactiae clinical isolates from Guelma, Algeria and Marseille, France. Eur. J. Clin. Microbiol. Infect. Dis. 34, 2339–2348. doi: 10.1007/s10096-015-2487-6
Bhola, P., Mvelase, N., Balakrishna, Y., Mlisana, K., and Swe-Han, K. S. (2020). Antimicrobial susceptibility patterns of uropathogens isolated from pregnant women in KwaZulu-Natal Province: 2011–2016. S. Afr. Med. J. 110, 872–876. doi: 10.7196/SAMJ.2020.v110i9.14468
Biedenbach, D. J., Arhin, F. F., Moeck, G., Lynch, T. F., and Sahm, D. F. (2015). In vitro activity of oritavancin and comparator agents against staphylococci, streptococci and enterococci from clinical infections in Europe and North America, 2011–2014. Int. J. Antimicrob. Agents 46, 674–681. doi: 10.1016/j.ijantimicag.2015.08.014
Biobaku Oluwafunmilola, R., Olaleye Atinuke, O., Adefusi Olorunwa, F., Adeyemi Babalola, A., Onipede Anthony, O., Loto Olabisi, M., et al. (2017). Group B Streptococcus colonization and HIV in pregnancy: a cohort study in Nigeria. J. Neonatal-Perinatal Med. 10, 91–97. doi: 10.3233/NPM-1685
Bitew, A., Mengist, A., Belew, H., Aschale, Y., and Reta, A. (2021). The prevalence, antibiotic resistance pattern, and associated factors of bacterial vaginosis among women of the reproductive age group from Felege Hiwot Referral Hospital, Ethiopia. Infect. Drug Resist. 14, 2685–2696. doi: 10.2147/IDR.S305329
Björnsdóttir, E. S., Martins, E. R., Erlendsdottir, H., Haraldsson, G., Melo-Cristino, J., Ramirez, M., et al. (2019). Group B streptococcal neonatal and early infancy infections in Iceland, 1976–2015. Pediatr. Infect. Dis. J. 38, 620–624. doi: 10.1097/INF.0000000000002214
Bob-Manuel, M., McGee, L., Igunma, J. A., Alex-Wele, M. A., Obunge, O. K., and Wariso, K. T. (2021). Whole genome sequence based capsular typing and antimicrobial resistance prediction of group B streptococcal isolates from colonized pregnant women in Nigeria. BMC Genomics 22:627. doi: 10.1186/s12864-021-07929-z
Bolukaoto, J. Y., Monyama, C. M., Chukwu, M. O., Lekala, S. M., Nchabeleng, M., Maloba, M. R., et al. (2015). Antibiotic resistance of Streptococcus agalactiae isolated from pregnant women in Garankuwa, South Africa. BMC. Res. Notes 8:364. doi: 10.1186/s13104-015-1328-0
Brigtsen, A. K., Dedi, L., Melby, K. K., Holberg-Petersen, M., Radtke, A., Lyng, R. V., et al. (2015). Comparison of PCR and serotyping of group B Streptococcus in pregnant women: the Oslo GBS-study. J. Microbiol. Methods 108, 31–35. doi: 10.1016/j.mimet.2014.11.001
Burcham, L. R., Spencer, B. L., Keeler, L. R., Runft, D. L., Patras, K. A., Neely, M. N., et al. (2019). Determinants of group B streptococcal virulence potential amongst vaginal clinical isolates from pregnant women. PLoS One 14:e0226699. doi: 10.1371/journal.pone.0226699
Campisi, E., Rosini, R., Ji, W., Guidotti, S., Rojas-Lopez, M., Geng, G., et al. (2016). Genomic analysis reveals multi-drug resistance clusters in group B Streptococcus CC17 hypervirulent isolates causing neonatal invasive disease in southern mainland China. Front. Microbiol. 7:1265. doi: 10.3389/fmicb.2016.01265
Carvalhaes, C. G., Sader, H. S., Streit, J. M., and Mendes, R. E. (2022). Five-year analysis of the in vitro activity of tedizolid against a worldwide collection of indicated species causing clinical infections: results from the Surveillance of Tedizolid Activity and Resistance (STAR) programme. JAC Antimicrob. Resist. 4:dlac088. doi: 10.1093/jacamr/dlac088
Chang, B., Wada, A., Hosoya, M., Oishi, T., Ishiwada, N., Oda, M., et al. (2014). Characteristics of group B Streptococcus isolated from infants with invasive infections: a population-based study in Japan. Jpn. J. Infect. Dis. 67, 356–360. doi: 10.7883/yoken.67.356
Chen, X., Wu, J., Liu, Y., Munang’andu, H. M., and Peng, B. (2023). Fructose promotes ampicillin killing of antibiotic-resistant Streptococcus agalactiae. Virulence 14:2180938. doi: 10.1080/21505594.2023.2180938
Cheng, Z., Qu, P., Ke, P., Yang, X., Zhou, Q., Lan, K., et al. (2020). Antibiotic resistance and molecular epidemiological characteristics of Streptococcus agalactiae isolated from pregnant women in Guangzhou, South China. Can. J. Infect. Dis. Med. Microbiol. 2020:1368942. doi: 10.1155/2020/1368942
Choi, S. J., Kang, J., and Uh, Y. (2021). Recent epidemiological changes in group B Streptococcus among pregnant Korean women. Ann. Lab. Med. 41, 380–385. doi: 10.3343/alm.2021.41.4.380
Cochran, W. G. (1954). The combination of estimates from different experiments. Biometrics 10, 101–129. doi: 10.2307/3001666
Cooper, K., Abbott, F., and Gould, I. (2016). Reduced penicillin susceptibility of group B Streptococcus: an assessment of emergence in Grampian, Scotland. Br. J. Biomed. Sci. 73, 25–27. doi: 10.1080/09674845.2016.1144550
Coque, T. M., Cantón, R., Pérez-Cobas, A. E., Fernández-de-Bobadilla, M. D., and Baquero, F. (2023). Antimicrobial resistance in the global health network: known unknowns and challenges for efficient responses in the 21st century. Microorganisms 11:1050. doi: 10.3390/microorganisms11041050
Creti, R., Imperi, M., Berardi, A., Pataracchia, M., Recchia, S., Alfarone, G., et al. (2017). Neonatal group B Streptococcus infections: prevention strategies, clinical and microbiologic characteristics in 7 years of surveillance. Pediatr. Infect. Dis. J. 36, 256–262. doi: 10.1097/INF.0000000000001414
Dashtizade, M., Zolfaghari, M. R., Yousefi, M., and Nazari-Alam, A. (2020). Antibiotic susceptibility patterns and prevalence of streptococcus agalactiae rectovaginal colonization among pregnant women in Iran. Rev. Bras. Ginecol. Obstet. 42, 454–459. doi: 10.1055/s-0040-1710299
de Figueiredo, S. G., Lannes-Costa, P. S., Cristoforêto, M. C., Doran, K. S., Mattos-Guaraldi, A. L., and Nagao, P. E. (2021). Streptococcus agalactiae strains isolated from cancer patients in Rio de Janeiro, Brazil. Braz. J. Microbiol. 52, 303–310. doi: 10.1007/s42770-020-00419-6
de Melo, S. C. C. S., de Souza Santos, N. C., de Oliveira, M., de Lima Scodro, R. B., Cardoso, R. F., Pádua, R. A. F., et al. (2016). Antimicrobial susceptibility of Streptococcus agalactiae isolated from pregnant women. Rev. Inst. Med. Trop. Sao Paulo 58:83. doi: 10.1590/S1678-9946201658083
de Oliveira Luiz, F. B., Alves, K. B., and Barros, R. R. (2019). Prevalence and long-term persistence of beta-haemolytic streptococci throat carriage among children and young adults. J. Med. Microbiol. 68, 1526–1533. doi: 10.1099/jmm.0.001054
Dehbashi, S., Pourmand, M., Mahmoudi, M., and Mashhadi, R. (2015). Molecular identification of streptococcus agalactiae using gbsl805 gene and determination of the antibiotic susceptibility pattern of isolates. J. Babol Univ. Med. Sci. 16, 29–35. doi: 10.22088/jbums.17.2.29
Dehdashtian, M., Moosavian, M., Boghrati, M., Arshadi, M., Malakian, A., and Aramesh, M. R. (2021). Neonatal nasopharyngeal bacterial colonization: prevalence, antimicrobial resistance, and concomitant early-onset sepsis. Jundishapur J. Microbiol. 14. doi: 10.5812/jjm.115643
del Pilar Crespo-Ortiz, M., Castañeda-Ramirez, C. R., Recalde-Bolaños, M., and Vélez-Londoño, J. D. (2014). Emerging trends in invasive and noninvasive isolates of Streptococcus agalactiae in a Latin American hospital: a 17-year study. BMC Infect. Dis. 14:428. doi: 10.1186/1471-2334-14-428
DerSimonian, R., and Laird, N. (1986). Meta-analysis in clinical trials. Control. Clin. Trials 7, 177–188. doi: 10.1016/0197-2456(86)90046-2
Di Renzo, G. C., Melin, P., Berardi, A., Blennow, M., Carbonell-Estrany, X., Donzelli, G., et al. (2015). Intrapartum GBS screening and antibiotic prophylaxis: a European consensus conference. J. Matern. Fetal Neonatal Med. 28, 766–782. doi: 10.3109/14767058.2014.934804
Dilrukshi, N., Kottahachchi, J., Dissanayake, T., and Fernando, N. (2023). Antibiotic sensitivity of group B Streptococcus from pregnant mothers and its association with resistance genes. Med. Princ. Pract. 32, 126–132. doi: 10.1159/000530525
Dilrukshi, G., Kottahachchi, J., Dissanayake, D., Pathiraja, R., Karunasingha, J., Sampath, M., et al. (2021). Group B Streptococcus colonisation and their antimicrobial susceptibility among pregnant women attending antenatal clinics in tertiary care hospitals in the Western Province of Sri Lanka. J. Obstet. Gynaecol. 41, 1–6. doi: 10.1080/01443615.2020.1716313
Dobrut, A., Ochońska, D., Brzozowska, E., Górska, S., Kaszuba-Zwoinska, J., Gołda-Cępa, M., et al. (2022). Molecular characteristic, antibiotic resistance, and detection of highly immunoreactive proteins of group B Streptococcus strains isolated from urinary tract infections in polish adults. Front. Microbiol. 13:809724. doi: 10.3389/fmicb.2022.809724
Dong, Y., Jiang, S.-Y., Zhou, Q., and Cao, Y. (2017). Group B Streptococcus causes severe sepsis in term neonates: 8 years experience of a major Chinese neonatal unit. World J. Pediatr. 13, 314–320. doi: 10.1007/s12519-017-0034-5
Doumith, M., Mushtaq, S., Martin, V., Chaudhry, A., Adkin, R., Coelho, J., et al. (2017). Genomic sequences of Streptococcus agalactiae with high-level gentamicin resistance, collected in the BSAC bacteraemia surveillance. J. Antimicrob. Chemother. 72, 2704–2707. doi: 10.1093/jac/dkx207
Dube, R., Al-Zuheiri, S. T. S., Syed, M., Harilal, L., Zuhaira, D. A. L., and Kar, S. S. (2022). Prevalence, clinico-bacteriological profile, and antibiotic resistance of symptomatic urinary tract infections in pregnant women. Antibiotics 12:33. doi: 10.3390/antibiotics12010033
Duncan, L. R., Sader, H. S., Smart, J. I., Flamm, R. K., and Mendes, R. E. (2017). Telavancin activity in vitro tested against a worldwide collection of Gram-positive clinical isolates (2014). J. Glob. Antimicrob. Resist. 10, 271–276. doi: 10.1016/j.jgar.2017.03.018
Dutra, V. G., Alves, V. M., Olendzki, A. N., Dias, C. A., de Bastos, A. F., Santos, G. O., et al. (2014). Streptococcus agalactiae in Brazil: serotype distribution, virulence determinants and antimicrobial susceptibility. BMC Infect. Dis. 14:323. doi: 10.1186/1471-2334-14-323
Ebrahem, A. M., Jameel, S. K., and Abbas, A. H. (2023). Investigation of biofilm formation and antibiotic resistant of bacteria isolated from septic neonates. J. Med. Chem. Sci. 6, 816–826. doi: 10.26655/JMCHEMSCI.2023.4.13
El Shahaway, A. A., El Maghraby, H. M., Mohammed, H. A., Abd Elhady, R. R., and Abdelrhman, A. A. (2019). Diagnostic performance of direct latex agglutination, post-enrichment latex agglutination and culture methods in screening of group B streptococci in late pregnancy: a comparative study. Infect. Drug Resist. 12, 2583–2588. doi: 10.2147/IDR.S203543
El-Gendy, A.-E. A., Hassan, S. E. T., Gertz, B., Bernard, B., Ahmed, M. M., Elzohry, H. A., et al. (2021). Serotyping and antibiotic susceptibility of invasive Streptococcus agalactiae in Egyptian patients with or without diabetes mellitus. Am. J. Trop. Med. Hyg. 105, 1684–1689. doi: 10.4269/ajtmh.21-0300
Emaneini, M., Jabalameli, F., Mirsalehian, A., Ghasemi, A., and Beigverdi, R. (2016). Characterization of virulence factors, antimicrobial resistance pattern and clonal complexes of group B streptococci isolated from neonates. Microb. Pathog. 99, 119–122. doi: 10.1016/j.micpath.2016.08.016
Emaneini, M., Mirsalehian, A., Beigvierdi, R., Fooladi, A. A., Asadi, F., Jabalameli, F., et al. (2014). High incidence of macrolide and tetracycline resistance among Streptococcus Agalactiae strains isolated from clinical samples in Tehran, Iran. Maedica 9, 157–161
Eskandarian, N., Ismail, Z., Neela, V., van Belkum, A., Desa, M., and Amin, N. S. (2015). Antimicrobial susceptibility profiles, serotype distribution and virulence determinants among invasive, non-invasive and colonizing Streptococcus agalactiae (group B Streptococcus) from Malaysian patients. Eur. J. Clin. Microbiol. Infect. Dis. 34, 579–584. doi: 10.1007/s10096-014-2265-x
Evangelia, K. E. S., Iliana, T., Evi, C., Ioanna, T., Vassiliki, G., and Stavroula, B. (2015). Decreased susceptibility to clindamycin, erythromycin and tetracycline of streptococcus agalactiae isolated from vaginal samples. Acta Microbiol. Hellenica 60:180.
Fahim, N. A. E., Ragay, M. B., Salah El-Deen, N. N., and ElMasry, S. A. (2022). Diagnostic performance of two chromogenic media for Streptococcus agalactiae screening in pregnant women. Microbes Infect. Dis. 3, 947–955. doi: 10.21608/mid.2022.148315.1338
Felemban, E. M., Al Juaid, D. A., Alsanie, W. F., Hassan, M. M., and Gaber, A. (2019). Correlation between strain distribution and antibiotic resistance genes pattern of Streptococcus agalactiae group B from patients in Taif, Saudi Arabia. J. Pure Appl. Microbiol. 13, 257–263. doi: 10.22207/JPAM.13.1.27
Feuerschuette, O. H. M., Alves, E. V., Scheffer, M. C., Vilela, A. P. P., Barazzetti, F. H., Feuerschuette, H. M., et al. (2022). Genetic diversity and antimicrobial resistance of invasive, noninvasive and colonizing group B Streptococcus isolates in southern Brazil. Access Microbiol. 4:000370. doi: 10.1099/acmi.0.000370
Flamm, R. K., Sader, H. S., and Jones, R. N. (2014). Ceftaroline activity tested against contemporary Latin American bacterial pathogens (2011). Braz. J. Infect. Dis. 18, 187–195. doi: 10.1016/j.bjid.2013.11.005
Florindo, C., Damiao, V., Silvestre, I., Farinha, C., Rodrigues, F., Nogueira, F., et al. (2014). Epidemiological surveillance of colonising group B Streptococcus epidemiology in the Lisbon and Tagus Valley regions, Portugal (2005 to 2012): emergence of a new epidemic type IV/clonal complex 17 clone. Eur. Secur. 19:20825. doi: 10.2807/1560-7917.ES2014.19.23.20825
Foster-Nyarko, E., Kwambana, B., Aderonke, O., Ceesay, F., Jarju, S., Bojang, A., et al. (2016). Associations between nasopharyngeal carriage of group B Streptococcus and other respiratory pathogens during early infancy. BMC Microbiol. 16:97. doi: 10.1186/s12866-016-0714-7
Frej-Mądrzak, M., Jama-Kmiecik, A., Sarowska, J., Teryks-Wołyniec, D., Gryboś, A., Gryboś, M., et al. (2020). Streptococcus agalactiae and Chlamydia trachomatis detection in women without symptoms of infection. Adv. Clin. Exp. Med. 29, 707–713. doi: 10.17219/acem/122397
Fröhlicher, S., Reichen-Fahrni, G., Müller, M., Surbek, D., Droz, S. C., Spellerberg, B., et al. (2014). Serotype distribution and antimicrobial susceptibility of group B streptococci in pregnant women: results from a Swiss tertiary Centre. Swiss Med. Wkly. 144:w13935. doi: 10.4414/smw.2014.13935
Fujiya, Y., Hayakawa, K., Gu, Y., Yamamoto, K., Mawatari, M., Kutsuna, S., et al. (2019). Age-related differences in clinical characteristics of invasive group G streptococcal infection: comparison with group A and group B streptococcal infections. PLoS One 14:e0211786. doi: 10.1371/journal.pone.0211786
Gajic, I., Plainvert, C., Kekic, D., Dmytruk, N., Mijac, V., Tazi, A., et al. (2019). Molecular epidemiology of invasive and non-invasive group B Streptococcus circulating in Serbia. Int. J. Med. Microbiol. 309, 19–25. doi: 10.1016/j.ijmm.2018.10.005
Gao, K., Fu, J., Guan, X., Zhu, S., Zeng, L., Xu, X., et al. (2019). Incidence, bacterial profiles, and antimicrobial resistance of culture-proven neonatal sepsis in South China. Infect. Drug Resist. 12, 3797–3805. doi: 10.2147/IDR.S223597
Ge, Y., Pan, F., Bai, R., Mao, Y., Ji, W., Wang, F., et al. (2021). Prevalence of group B Streptococcus colonization in pregnant women in Jiangsu, East China. BMC Infect. Dis. 21:492. doi: 10.1186/s12879-021-06186-5
Ghamari, M., Jabalameli, F., Emaneini, M., and Beigverdi, R. (2022). Multiple-locus variable-number tandem repeat analysis for genotyping of erythromycin-resistant group B streptococci in Iran. New Microbes New Infect. 45:100957. doi: 10.1016/j.nmni.2022.100957
Gharabeigi, N., Bafroee, A. S. T., and Amini, K. (2023). Molecular serotyping and antibiotic resistance profile of group B Streptococcus strains isolated from Iranian pregnant women with urinary tract infection. Iran. J. Med. Sci. 48, 542–550. doi: 10.30476/ijms.2023.96346.2787
Gherardi, G., Imperi, M., Palmieri, C., Magi, G., Facinelli, B., Baldassarri, L., et al. (2014). Genetic diversity and virulence properties of Streptococcus dysgalactiae subsp. equisimilis from different sources. J. Med. Microbiol. 63, 90–98. doi: 10.1099/jmm.0.062109-0
Girma, W., Yimer, N., Kassa, T., and Yesuf, E. (2020). Group B Streptococcus recto-vaginal colonization in near-term pregnant women, Southwest Ethiopia. Ethiop. J. Health Sci. 30. doi: 10.4314/ejhs.v30i5.7
Gizachew, M., Tiruneh, M., Moges, F., Adefris, M., Tigabu, Z., and Tessema, B. (2018). Newborn colonization and antibiotic susceptibility patterns of Streptococcus agalactiae at the University of Gondar Referral Hospital, Northwest Ethiopia. BMC Pediatr. 18:378. doi: 10.1186/s12887-018-1350-1
Gizachew, M., Tiruneh, M., Moges, F., Adefris, M., Tigabu, Z., and Tessema, B. (2020). Molecular characterization of Streptococcus agalactiae isolated from pregnant women and newborns at the University of Gondar Comprehensive Specialized Hospital, Northwest Ethiopia. BMC Infect. Dis. 20:35. doi: 10.1186/s12879-020-4776-7
Gogoi, M., Das, M. K., Das, J. K., Barman, N., Das, P., and Devi, U. (2021). Group B Streptococcus colonising the genital tract of pregnant women from Dibrugarh, Assam: circulating serotypes, susceptibility pattern and phylogenetic analysis. J. Clin. Diagn. Res. 15, 13–18. doi: 10.7860/JCDR/2021/47750.14824
Gomi, Y., Wang, L., Matsushima, H., Kawabe, A., Kikugawa, A., Takagi, A., et al. (2019). Variations in antibiotic susceptibility of group B Streptococcus in Japanese women: a long-term population-based cohort study. Taiwan. J. Obstet. Gynecol. 58, 805–807. doi: 10.1016/j.tjog.2019.09.014
Goudarzi, G., Ghafarzadeh, M., Shakib, P., and Anbari, K. (2015). Culture and real-time PCR based maternal screening and antibiotic susceptibility for group B Streptococcus: an Iranian experience. Global J. Health Sci. 7:233. doi: 10.5539/gjhs.v7n6p233
Goudarzi, M., and Navidinia, M. (2019). Overview perspective of bacterial strategies of resistance to biocides and antibiotics. Arch. Clin. Infect. Dis. 14:e65744. doi: 10.5812/archcid.65744
Graux, E., Hites, M., Martiny, D., Maillart, E., Delforge, M., Melin, P., et al. (2021). Invasive group B Streptococcus among non-pregnant adults in Brussels-Capital Region, 2005–2019. Eur. J. Clin. Microbiol. Infect. Dis. 40, 515–523. doi: 10.1007/s10096-020-04041-0
Guan, X., Mu, X., Ji, W., Yuan, C., He, P., Zhang, L., et al. (2018). Epidemiology of invasive group B streptococcal disease in infants from urban area of South China, 2011–2014. BMC Infect. Dis. 18:14. doi: 10.1186/s12879-017-2811-0
Guo, D., Cao, X., Li, S., Ou, Q., Lin, D., Yao, Z., et al. (2018). Neonatal colonization of group B Streptococcus in China: prevalence, antimicrobial resistance, serotypes, and molecular characterization. Am. J. Infect. Control 46, e19–e24. doi: 10.1016/j.ajic.2017.10.020
Guo, Y., Deng, X., Liang, Y., Zhang, L., Zhao, G.-P., and Zhou, Y. (2018). The draft genomes and investigation of serotype distribution, antimicrobial resistance of group B Streptococcus strains isolated from urine in Suzhou, China. Ann. Clin. Microbiol. Antimicrob. 17:28. doi: 10.1186/s12941-018-0280-y
Guo, H., Fu, M., Peng, Q., Chen, Z., Liu, J., Qiu, Y., et al. (2019). Antimicrobial resistance and molecular characterization of Streptococcus agalactiae from pregnant women in southern China. J. Infect. Dev. Countries 13, 802–809. doi: 10.3855/jidc.11395
Guo, L. Y., Zhang, Z. X., Wang, X., Zhang, P. P., Shi, W., Yao, K. H., et al. (2016). Clinical and pathogenic analysis of 507 children with bacterial meningitis in Beijing, 2010–2014. Int. J. Infect. Dis. 50, 38–43. doi: 10.1016/j.ijid.2016.07.010
Hadavand, S., Ghafoorimehr, F., Rajabi, L., Davati, A., and Zafarghandi, N. (2015). Frequency of group B streptococcal colonization in pregnant women aged 35–37 weeks in clinical centers of Shahed University, Tehran, Iran. Iran. J. Pathol. 10, 120–126. doi: 10.7508/ijp.2015.02.006
Haimbodi, E. L., Mukesi, M., and Moyo, S. R. (2021). Prevalence and molecular characterization of group B Streptococcus in pregnant women from hospitals in Ohangwena and Oshikoto regions of Namibia. BMC Microbiol. 21:224. doi: 10.1186/s12866-021-02283-2
Hamad, A. A., Farrag, A. A., El-Waseif, A. A., and Almawla, S. O. G. (2023). Novel molecular diagnosis of Cyl E, Spb 1 and bib A virulence genes of Streptococcus agalactiae from pregnant women. Mater. Today Proc. 80, 2347–2352. doi: 10.1016/j.matpr.2021.06.347
Hayes, K., Cotter, L., Barry, L., and O’Halloran, F. (2017). Emergence of the L phenotype in Group B streptococci in the south of Ireland. Epidemiol. Infect. 145, 3535–3542. doi: 10.1017/S0950268817002461
Hayes, K., O’Halloran, F., and Cotter, L. (2020). A review of antibiotic resistance in group B Streptococcus: the story so far. Crit. Rev. Microbiol. 46, 253–269. doi: 10.1080/1040841X.2020.1758626
Hays, C., Louis, M., Plainvert, C., Dmytruk, N., Touak, G., Trieu-Cuot, P., et al. (2016). Changing epidemiology of group B Streptococcus susceptibility to fluoroquinolones and aminoglycosides in France. Antimicrob. Agents Chemother. 60, 7424–7430. doi: 10.1128/AAC.01374-16
Heath, P. T., and Jardine, L. A. (2014). Neonatal infections: group B Streptococcus. BMJ Clin. Evid. 2014:0323
Hernandez, L. B., Cadona, J. S., Traverso, F., Altamiranda, S. M., Bustamante, A. V., and Sanso, A. M. (2022). Virulence profiles and antimicrobial resistance of Streptococcus agalactiae infective and colonizing strains from Argentina. Curr. Microbiol. 79:392. doi: 10.1007/s00284-022-03050-w
Higgins, J. P., and Thompson, S. G. (2002). Quantifying heterogeneity in a meta-analysis. Stat. Med. 21, 1539–1558. doi: 10.1002/sim.1186
Hirai, N., Kasahara, K., Nakano, R., Ogawa, Y., Suzuki, Y., Ogawa, M., et al. (2020). Clinical characteristics and molecular epidemiology of invasive Streptococcus agalactiae infections between 2007 and 2016 in Nara, Japan. PLoS One 15:e0240590. doi: 10.1371/journal.pone.0240590
Hiriote, W., Tor-Udom, S., and Tor-Udom, P. (2017). Maternal to child group B Streptococcus transmission rate at Thammasat University hospital, Thailand. Southeast Asian J. Trop. Med. Public Health 48, 841–849.
Hon, K. L., Chow, T. C., Cheung, T. S., Lam, W. T., Hung, L. T., So, K. W., et al. (2020). Severe group A and group B Streptococcus diseases at a pediatric ICU: are they still sensitive to the penicillins? Curr. Clin. Pharmacol. 15, 125–131. doi: 10.2174/1574884714666190926124714
Hooshiar, M. H., Sholeh, M., Beig, M., Azizian, K., and Kouhsari, E. (2024). Global trends of antimicrobial resistance rates in Neisseria gonorrhoeae: a systematic review and meta-analysis. Front. Pharmacol. 15:1284665. doi: 10.3389/fphar.2024.1284665
Horn, D. L., Roberts, E. A., Shen, J., Chan, J. D., Bulger, E. M., Weiss, N. S., et al. (2021). Outcomes of β-hemolytic streptococcal necrotizing skin and soft-tissue infections and the impact of clindamycin resistance. Clin. Infect. Dis. 73, e4592–e4598. doi: 10.1093/cid/ciaa976
Houri, H., Kazemian, H., Ebrahim-Saraie, H. S., Taji, A., Tayebi, Z., and Heidari, H. (2017). Linezolid activity against clinical Gram-positive cocci with advanced antimicrobial drug resistance in Iran. J. Glob. Antimicrob. Resist. 10, 200–203. doi: 10.1016/j.jgar.2017.06.002
Hsu, J.-F., Chen, Y.-N., Chu, S.-M., Lee, W.-J., Huang, H.-R., Chiang, M.-C., et al. (2023a). Clonal complex 12 serotype Ib Streptococcus agalactiae strain causing complicated sepsis in neonates: clinical features and genetic characteristics. Microbiol. Spectr. 11:e0377822. doi: 10.1128/spectrum.03778-22
Hsu, J.-F., Lu, J.-J., Chu, S.-M., Lee, W.-J., Huang, H.-R., Chiang, M.-C., et al. (2023b). The clinical and genetic characteristics of Streptococcus agalactiae meningitis in neonates. Int. J. Mol. Sci. 24:15387. doi: 10.3390/ijms242015387
Hsu, J. F., Tsai, M.-H., Lin, L.-C., Chu, S. M., Lai, M. Y., Huang, H. R., et al. (2021). Genomic characterization of serotype III/ST-17 group B Streptococcus strains with antimicrobial resistance using whole genome sequencing. Biomedicines 9:1477. doi: 10.3390/biomedicines9101477
Hsueh, P. R. (2015). In vitro activities of tedizolid and linezolid against Gram-positive cocci associated with acute bacterial skin and skin structure infections and pneumonia. Int. J. Antimicrob. Agents 45:S70. doi: 10.1128/AAC.00390-15
Husen, O., Kannaiyan Abbai, M., Aliyo, A., Daka, D., Gemechu, T., Tilahun, D., et al. (2023). Prevalence, antimicrobial susceptibility pattern and associated factors of group B Streptococcus among pregnant women attending antenatal Care at Bule Hora University Teaching Hospital, Southern Ethiopia. Infect. Drug Resist. 16, 4421–4433. doi: 10.2147/IDR.S415414
Ikebe, T., Chiba, K., Shima, T., Masuda, C., Okuno, R., Ohya, H., et al. (2015). Evaluation of streptococcal toxic shock-like syndrome caused by group B Streptococcus in adults in Japan between 2009 and 2013. J. Infect. Chemother. 21, 207–211. doi: 10.1016/j.jiac.2014.12.002
Ikebe, T., Okuno, R., Uchitani, Y., Takano, M., Yamaguchi, T., Otsuka, H., et al. (2023). Serotype distribution and antimicrobial resistance of Streptococcus agalactiae isolates in non-pregnant adults with streptococcal toxic shock syndrome in Japan in 2014 to 2021. Microbiol. Spectr. 11:e0498722. doi: 10.1128/spectrum.04987-22
Imperi, M., Gherardi, G., Alfarone, G., and Creti, R. (2024). Group B Streptococcus infections in non-pregnant adults, Italy, 2015–2019. Pathogens 13:807. doi: 10.3390/pathogens13090807
Iweriebor, B. C., Afolabi, K. O., Stofile, P. Z., and Obi, L. C. (2023). Group B Streptococcus agalactiae resistant to recommended intrapartum antibiotic prophylaxis isolated from the rectovaginal area of 35–37 weeks pregnant women. Malays. J. Microbiol. 19. doi: 10.21161/mjm.230225
Jalalifar, S., Havaei, S. A., Motallebirad, T., Moghim, S., Fazeli, H., and Esfahani, B. N. (2019). Determination of surface proteins profile, capsular genotyping, and antibiotic susceptibility patterns of group B Streptococcus isolated from urinary tract infection of Iranian patients. BMC. Res. Notes 12:437. doi: 10.1186/s13104-019-4428-4
Jamrozy, D., Bijlsma, M. W., de Goffau, M. C., van de Beek, D., Kuijpers, T. W., Parkhill, J., et al. (2020). Increasing incidence of group B Streptococcus neonatal infections in the Netherlands is associated with clonal expansion of CC17 and CC23. Sci. Rep. 10:9539. doi: 10.1038/s41598-020-66214-3
Ji, W., Zhang, L., Guo, Z., Xie, S., Yang, W., Chen, J., et al. (2017). Colonization prevalence and antibiotic susceptibility of group B Streptococcus in pregnant women over a 6-year period in Dongguan, China. PLoS One 12:e0183083. doi: 10.1371/journal.pone.0183083
Jiang, H., Chen, M., Li, T., Liu, H., Gong, Y., and Li, M. (2016). Molecular characterization of Streptococcus agalactiae causing community-and hospital-acquired infections in Shanghai, China. Front. Microbiol. 7:1308. doi: 10.3389/fmicb.2016.01308
Jiang, H., Su, M., Kui, L., Huang, H., Qiu, L., Li, L., et al. (2017). Prevalence and antibiotic resistance profiles of cerebrospinal fluid pathogens in children with acute bacterial meningitis in Yunnan province, China, 2012–2015. PLoS One 12:e0180161. doi: 10.1371/journal.pone.0180161
Jiao, J., Wu, W., Shen, F., Liu, Z., Zhou, H., Fan, G., et al. (2022). Clinical profile and risk factors of group B streptococcal colonization in mothers from the Eastern District of China. J. Trop. Med. 2022:5236430. doi: 10.1155/2022/5236430
Jisuvei, S. C., Osoti, A., and Njeri, M. A. (2020). Prevalence, antimicrobial susceptibility patterns, serotypes and risk factors for group B Streptococcus rectovaginal isolates among pregnant women at Kenyatta National Hospital, Kenya; a cross-sectional study. BMC Infect. Dis. 20:302. doi: 10.1186/s12879-020-05035-1
Jones, S., Newton, P., Payne, M., and Furfaro, L. (2022). Epidemiology, antimicrobial resistance, and virulence determinants of group B Streptococcus in an Australian setting. Front. Microbiol. 13:839079. doi: 10.3389/fmicb.2022.839079
Kaminska, D., Ratajczak, M., Szumała-Kąkol, A., Dlugaszewska, J., Nowak-Malczewska, D. M., and Gajecka, M. (2020). Increasing resistance and changes in distribution of serotypes of Streptococcus agalactiae in Poland. Pathogens 9:526. doi: 10.3390/pathogens9070526
Kang, H. M., Lee, H. J., Lee, H., Jo, D. S., Lee, H. S., Kim, T. S., et al. (2017). Genotype characterization of group B Streptococcus isolated from infants with invasive diseases in South Korea. Pediatr. Infect. Dis. J. 36, e242–e247. doi: 10.1097/INF.0000000000001531
Kao, Y., Tsai, M.-H., Lai, M.-Y., Chu, S.-M., Huang, H.-R., Chiang, M.-C., et al. (2019). Emerging serotype III sequence type 17 group B Streptococcus invasive infection in infants: the clinical characteristics and impacts on outcomes. BMC Infect. Dis. 19:538. doi: 10.1186/s12879-019-4177-y
Kardos, S., Tóthpál, A., Laub, K., Kristóf, K., Ostorházi, E., Rozgonyi, F., et al. (2019). High prevalence of group B Streptococcus ST17 hypervirulent clone among non-pregnant patients from a Hungarian venereology clinic. BMC Infect. Dis. 19:1009. doi: 10.1186/s12879-019-4626-7
Karim, G. F., SS, A. L.-S., Atya, Q. M., and Abass, K. S. (2019). Aerobic and anaerobic bacteria in tonsils of different ages with recurrent tonsillitis. Indian J. Public Health Res. Dev. 10, 132–136.
Karlowsky, J. A., Biedenbach, D. J., Bouchillon, S. K., Hackel, M., Iaconis, J. P., and Sahm, D. F. (2016). In vitro activity of ceftaroline against bacterial pathogens isolated from patients with skin and soft tissue and respiratory tract infections in African and middle eastern countries: AWARE global surveillance program 2012–2014. Diagn. Microbiol. Infect. Dis. 86, 194–199. doi: 10.1016/j.diagmicrobio.2016.07.012
Karlowsky, J. A., Nichol, K., and Zhanel, G. G. (2015). Telavancin: mechanisms of action, in vitro activity, and mechanisms of resistance. Clin. Infect. Dis. 61, S58–S68. doi: 10.1093/cid/civ534
Karlowsky, J., Walkty, A., Baxter, M., Adam, H., and Zhanel, G. (2014). In vitro activity of oritavancin against Gram-positive pathogens isolated in Canadian hospital laboratories from 2011 to 2013. Diagn. Microbiol. Infect. Dis. 80, 311–315. doi: 10.1016/j.diagmicrobio.2014.09.003
Karlowsky, J. A., Walkty, A. J., Baxter, M. R., Arhin, F. F., Moeck, G., Adam, H. J., et al. (2017). In vitro activity of oritavancin against Gram-positive pathogens isolated in Canadian hospital laboratories from 2011 to 2015. Diagn. Microbiol. Infect. Dis. 87, 349–356. doi: 10.1016/j.diagmicrobio.2017.01.006
Kasem, S., Elhadidi, A., Omar, N., Dawoud, T., Sa'da, O. A., Rahmani, A., et al. (2024). Microbiological characteristics and resistance patterns in a neonatal intensive care unit: a retrospective surveillance Study. Cureus 16:e56027. doi: 10.7759/cureus.56027
Kawaguchiya, M., Urushibara, N., Aung, M. S., Shimada, S., Nakamura, M., Ito, M., et al. (2022). Molecular characterization and antimicrobial resistance of Streptococcus agalactiae isolated from pregnant women in Japan, 2017–2021. IJID Reg. 4, 143–145. doi: 10.1016/j.ijregi.2022.07.002
Kekic, D., Gajic, I., Opavski, N., Kojic, M., Vukotic, G., Smitran, A., et al. (2021). Trends in molecular characteristics and antimicrobial resistance of group B streptococci: a multicenter study in Serbia, 2015–2020. Sci. Rep. 11:540. doi: 10.1038/s41598-020-79354-3
Kernéis, S., Plainvert, C., Barnier, J.-P., Tazi, A., Dmytruk, N., Gislain, B., et al. (2017). Clinical and microbiological features associated with group B Streptococcus bone and joint infections, France 2004–2014. Eur. J. Clin. Microbiol. Infect. Dis. 36, 1679–1684. doi: 10.1007/s10096-017-2983-y
Khan, M. A., Faiz, A., and Ashshi, A. M. (2015). Maternal colonization of group B Streptococcus: prevalence, associated factors and antimicrobial resistance. Ann. Saudi Med. 35, 423–427. doi: 10.5144/0256-4947.2015.423
Khan, U. B., Portal, E. A., Sands, K., Lo, S., Chalker, V. J., Jauneikaite, E., et al. (2023). Genomic analysis reveals new integrative conjugal elements and transposons in GBS conferring antimicrobial resistance. Antibiotics 12:544. doi: 10.3390/antibiotics12030544
Khodaei, F., Najafi, M., Hasani, A., Kalantar, E., Sharifi, E., Amini, A., et al. (2018). Pilus-encoding islets in S. agalactiae and its association with antibacterial resistance and serotype distribution. Microb. Pathog. 116, 189–194. doi: 10.1016/j.micpath.2018.01.035
Kitamura, M., Kimura, K., Ido, A., Seki, T., Banno, H., Jin, W., et al. (2019). Relatively high rates of cefotaxime-and ceftriaxone-non-susceptible isolates among group B streptococci with reduced penicillin susceptibility (PRGBS) in Japan. J. Antimicrob. Chemother. 74, 931–934. doi: 10.1093/jac/dky542
Ko, M. H.-J., Chang, H.-Y., Li, S.-T., Jim, W.-T., Chi, H., Hsu, C.-H., et al. (2021). An 18-year retrospective study on the epidemiology of early-onset neonatal sepsis-emergence of uncommon pathogens. Pediatr. Neonatol. 62, 491–498. doi: 10.1016/j.pedneo.2021.02.005
Kuhn, M., Wing, J., Weston, S., Williams, A., Keefer, C., Engelhardt, A., et al. (2020). Package ‘caret’. R J. 223.
Kumalo, A., Gebre, B., Shiferaw, S., Wolde, W., and Shonde, T. (2023). Group B streptococci recto-vaginal colonization, antimicrobial susceptibility pattern, and associated factors among pregnant women at selected health facilities of Wolaita Sodo Town, Southern Ethiopia. Front. Microbiol. 14:1277928. doi: 10.3389/fmicb.2023.1277928
Laczeski, M. E., Novosak, M. G., Zapata, P. D., and Vergara, M. I. (2014). First study of SCPB gene of streptococcus agalactiae in Misiones, Argentina. Biocell 38:132.
Lagunas-Rangel, F. A. (2018). Antimicrobial susceptibility profiles of bacteria causing urinary tract infections in Mexico: single-centre experience with 10 years of results. J. Glob. Antimicrob. Resist. 14, 90–94. doi: 10.1016/j.jgar.2018.03.004
Laitin, S. M. D., Baditoiu, L. M., Laza, R., Stefan, I. M., Besliu, R. S., Susa, S. R., et al. (2024). Unveiling the dynamics of antimicrobial resistance: a year-long surveillance (2023) at the largest infectious disease profile hospital in Western Romania. Antibiotics 13:1130. doi: 10.3390/antibiotics13121130
Lee, H., Kim, E. S., Song, K.-H., Kim, H. B., Park, J. S., and Park, K. U. (2022). Clinical and molecular epidemiology of invasive group B Streptococcus infections in adults in a referral center in Korea. Eur. J. Clin. Microbiol. Infect. Dis. 41, 1407–1413. doi: 10.1007/s10096-022-04505-5
Lee, W.-T., and Lai, M.-C. (2015). High prevalence of Streptococcus agalactiae from vaginas of women in Taiwan and its mechanisms of macrolide and quinolone resistance. J. Microbiol. Immunol. Infect. 48, 510–516. doi: 10.1016/j.jmii.2014.03.002
Leykun, Y., Genet, C., and Mulu, W. (2021). Group B streptococci vaginal-recto colonization, vertical transmission to newborns, antimicrobial susceptibility profile and associated factors in selected health facilities of Bahir Dar city: a cross-sectional study. Infect. Drug Resist. 14, 5457–5472. doi: 10.2147/IDR.S343429
Li, X., Ding, X., Shi, P., Zhu, Y., Huang, Y., Li, Q., et al. (2019). Clinical features and antimicrobial susceptibility profiles of culture-proven neonatal sepsis in a tertiary children’s hospital, 2013 to 2017. Medicine 98:e14686. doi: 10.1097/MD.0000000000014686
Li, X., Du, Z., Tang, Z., Wen, Q., Cheng, Q., and Cui, Y. (2022). Distribution and drug sensitivity of pathogenic bacteria in diabetic foot ulcer patients with necrotizing fasciitis at a diabetic foot center in China. BMC Infect. Dis. 22:396. doi: 10.1186/s12879-022-07382-7
Li, C., Feng, W. Y., Lin, A. W., Zheng, G., Wang, Y. C., Han, Y. J., et al. (2018). Clinical characteristics and etiology of bacterial meningitis in Chinese children> 28 days of age, January 2014–December 2016: a multicenter retrospective study. Int. J. Infect. Dis. 74, 47–53. doi: 10.1016/j.ijid.2018.06.023
Li, X., Gao, W., Jia, Z., Yao, K., Yang, J., Tong, J., et al. (2023). Characterization of group B Streptococcus recovered from pregnant women and newborns attending in a hospital in Beijing, China. Infecti. Drug Resist. 16, 2549–2559. doi: 10.2147/IDR.S395942
Li, J., Ji, W., Gao, K., Zhou, H., Zhang, L., Mu, X., et al. (2019). Molecular characteristics of group B Streptococcus isolates from infants in southern mainland China. BMC Infect. Dis. 19:812. doi: 10.1186/s12879-019-4434-0
Li, Y., Kong, C., and To, W. W. (2019). Pathogens in preterm prelabour rupture of membranes and erythromycin for antibiotic prophylaxis: a retrospective analysis. Hong Kong Med. J. 25, 287–294. doi: 10.12809/hkmj197991
Li, C., Sapugahawatte, D. N., Yang, Y., Wong, K. T., Lo, N. W. S., and Ip, M. (2020). Multidrug-resistant Streptococcus agalactiae strains found in human and fish with high penicillin and cefotaxime non-susceptibilities. Microorganisms 8:1055. doi: 10.3390/microorganisms8071055
Li, G., Wei, Y., Guo, Y., Gong, H., Lian, J., Xu, G., et al. (2022). Omadacycline efficacy against Streptococcus agalactiae isolated in China: correlation between resistance and virulence gene and biofilm formation. Comput. Intell. Neurosci. 2022:7636983. doi: 10.1155/2022/7636983
Lin, C., Chu, S.-M., Wang, H.-C., Yang, P.-H., Huang, H.-R., Chiang, M.-C., et al. (2021). Complicated Streptococcus agalactiae sepsis with/without meningitis in young infants and newborns: the clinical and molecular characteristics and outcomes. Microorganisms 9:2094. doi: 10.3390/microorganisms9102094
Liu, P., Feng, Q., Liang, Y., Wang, X., Xiao, Z., Huang, L., et al. (2022). Maternal group B streptococcal rectovaginal colonization after intrapartum antibiotic prophylaxis. Children 9:1848. doi: 10.3390/children9121848
Liu, Z., Jiang, X., Li, J., Ji, W., Zhou, H., Gong, X., et al. (2023). Molecular characteristics and antibiotic resistance mechanisms of clindamycin-resistant Streptococcus agalactiae isolates in China. Front. Microbiol. 14:1138039. doi: 10.3389/fmicb.2023.1138039
Liu, Y., and Liu, J. (2022). Group B Streptococcus: virulence factors and pathogenic mechanism. Microorganisms 10:2483. doi: 10.3390/microorganisms10122483
Liu, J. F. Z., Yu, Y., Ding, Y., Liu, Z., Zhang, C., He, H., et al. (2021). Pathogens distribution and antimicrobial resistance in bloodstream infections in twenty-five neonatal intensive care units in China, 2017–2019. Antimicrob. Resist. Infect. Control 10:121. doi: 10.1186/s13756-021-00989-6
Lopes, E. F. T., Machado, M. P., Carriço, J., Cristino, J. M., Ramirez, M., and Martins, E. (2017). Increase of macrolide resistance among group B Streptococcus invasive disease in non-pregnant adults in Portugal (2009–2015) was driven by a capsular variant of a single clone. Int. J. Antimicrob. Agents 50:S116. doi: 10.2807/1560-7917.ES.2018.23.21.1700473
Lu, B., Chen, X., Wang, J., Wang, D., Zeng, J., Li, Y., et al. (2016). Molecular characteristics and antimicrobial resistance in invasive and noninvasive group B Streptococcus between 2008 and 2015 in China. Diagn. Microbiol. Infect. Dis. 86, 351–357. doi: 10.1016/j.diagmicrobio.2016.08.023
Lu, B., Wu, J., Chen, X., Gao, C., Yang, J., Li, Y., et al. (2018). Microbiological and clinical characteristics of group B Streptococcus isolates causing materno-neonatal infections: high prevalence of CC17/PI-1 and PI-2b sublineage in neonatal infections. J. Med. Microbiol. 67, 1551–1559. doi: 10.1099/jmm.0.000849
Ma, A., Thompson, L. A., Corsiatto, T., Hurteau, D., and Tyrrell, G. J. (2021). Epidemiological characterization of group B Streptococcus infections in Alberta, Canada: an update from 2014 to 2020. Microbiol. Spectr. 9:e0128321. doi: 10.1128/Spectrum.01283-21
Madrid, L., Maculuve, S. A., Vilajeliu, A., Sáez, E., Massora, S., Cossa, A., et al. (2018). Maternal carriage of group B Streptococcus and Escherichia coli in a district hospital in Mozambique. Pediatr. Infect. Dis. J. 37, 1145–1153. doi: 10.1097/INF.0000000000001979
Majigo, M. M. J., Luoga, A., Juma, J., and Joachim, A. (2022). Spectrum and antimicrobial susceptibility patterns of pathogen causing neonatal sepsis at regional referral hospital, Dar es Salam, Tanzania. Tanzan. J. Health Res. 23:167.
Majigo, M., Makupa, J., Mwazyunga, Z., Luoga, A., Kisinga, J., Mwamkoa, B., et al. (2023). Bacterial aetiology of neonatal sepsis and antimicrobial resistance pattern at the regional referral hospital, Dar es Salam, Tanzania; a call to strengthening antibiotic stewardship program. Antibiotics 12:767. doi: 10.3390/antibiotics12040767
Malek-Jafarian, M., Hosseini, F., and Ahmadi, A.-R. (2015). Pattern of infection and antibiotic activity among Streptococcus agalactiae isolates from adults in Mashhad, Iran. Rep. Biochem. Mol. Biol. 3, 89–93.
Malița, M. A., Manolescu, L. S. C., Pîrvu, C. F., Costea, R. C., Marcov, E. C., Burlibasa, M., et al. (2023). Cumulative antibiogram: a rapid method to hinder transmission of resistant bacteria to oral cavity of newborn babies. Antibiotics 12:80. doi: 10.3390/antibiotics12010080
Martins, E. R., Pedroso-Roussado, C., Melo-Cristino, J., and Ramirez, M.The Portuguese Group for the Study of Streptococcal Infections (2017). Streptococcus agalactiae causing neonatal infections in Portugal (2005–2015): diversification and emergence of a CC17/PI-2b multidrug resistant sublineage. Front. Microbiol. 8:499. doi: 10.3389/fmicb.2017.00499
Matani, C., Trezzi, M., Matteini, A., Catalani, C., Messeri, D., and Catalani, C. (2016). Streptococcus agalactiae: prevalence of antimicrobial resistance in vaginal and rectal swabs in Italian pregnant women. Inf. Med. 24, 217–221.
Mathur, P., Bhardwaj, N., Mathur, K., Behera, B., Gupta, G., Kapil, A., et al. (2014). Clinical and molecular epidemiology of beta-hemolytic streptococcal infections in India. J. Infect. Dev. Countries 8, 297–303. doi: 10.3855/jidc.3216
Mendes, R. E., Farrell, D. J., Sader, H. S., Flamm, R. K., and Jones, R. N. (2015a). Activity of oritavancin against Gram-positive clinical isolates responsible for documented skin and soft-tissue infections in European and US hospitals (2010–13). J. Antimicrob. Chemother. 70, 498–504. doi: 10.1093/jac/dku421
Mendes, R. E., Farrell, D. J., Sader, H. S., Streit, J. M., and Jones, R. N. (2015b). Update of the telavancin activity in vitro tested against a worldwide collection of Gram-positive clinical isolates (2013), when applying the revised susceptibility testing method. Diagn. Microbiol. Infect. Dis. 81, 275–279. doi: 10.1016/j.diagmicrobio.2014.12.011
Merker, M., Tueffers, L., Vallier, M., Groth, E. E., Sonnenkalb, L., Unterweger, D., et al. (2020). Evolutionary approaches to combat antibiotic resistance: opportunities and challenges for precision medicine. Front. Immunol. 11:1938. doi: 10.3389/fimmu.2020.01938
Metcalf, B., Chochua, S., Gertz, R. Jr., Hawkins, P., Ricaldi, J., Li, Z., et al. (2017). Short-read whole genome sequencing for determination of antimicrobial resistance mechanisms and capsular serotypes of current invasive Streptococcus agalactiae recovered in the USA. Clin. Microbiol. Infect. 23, 574.e7–574.e14. doi: 10.1016/j.cmi.2017.02.021
Miloshevski, D. S. V., and Miloshevska, I. (2015). Vaginal colonization with group B Streptococcus (Streptococcus agalactiae). Acta Microbiol. Hellenica 60:172.
Minotti, C., Di Caprio, A., Facchini, L., Bedetti, L., Miselli, F., Rossi, C., et al. (2023). Antimicrobial resistance pattern and empirical antibiotic treatments in neonatal sepsis: a retrospective, single-center, 12-year study. Antibiotics 12:1488. doi: 10.3390/antibiotics12101488
Mišić, M., Arsović, A., Čukić, J., Rosić, M., Tošić-Pajić, J., Manojlović, N., et al. (2018). Učestalost rezistencije na makrolide i linkozamide kod ambulantnih i bolničkih izolata stafilokoka i streptokoka u jugoistočnoj Srbiji. Srp. Arh. Celok. Lek. 146, 384–390.
Mohamed, A. A. (2023). Antimicrobial resistance rates in Gram-positive uropathogens in Duhok city, Kurdistan region of Iraq. medRxiv. Available online at: https://doi.org/10.1101/2023.02.26.23286459. [Epub ahead of prepeint]
Mohamed, A. M., Khan, M. A., Faiz, A., Ahmad, J., Khidir, E. B., Basalamah, M. A., et al. (2020). Group B Streptococcus colonization, antibiotic susceptibility, and serotype distribution among Saudi pregnant women. Infect. Chemother. 52, 70–81. doi: 10.3947/ic.2020.52.1.70
Mohamed, N., Valdez, R. R., Fandiño, C., Baudrit, M., Falci, D. R., and Murillo, J. D. C. (2024). In vitro activity of ceftaroline against bacterial isolates causing skin and soft tissue and respiratory tract infections collected in Latin American countries, ATLAS program 2016–2020. J. Glob. Antimicrob. Resist. 36, 4–12. doi: 10.1016/j.jgar.2023.11.006
Moltó-García, B., del Carmen, L.-M. M., Cuadros-Moronta, E., Rodríguez-Granger, J., Sampedro-Martínez, A., Rosa-Fraile, M., et al. (2016). Molecular characterization and antimicrobial susceptibility of hemolytic Streptococcus agalactiae from post-menopausal women. Maturitas 85, 5–10. doi: 10.1016/j.maturitas.2015.11.007
Morfin-Otero, R., Noriega, E. R., and Dowzicky, M. J. (2015). Antimicrobial susceptibility trends among Gram-positive and-negative clinical isolates collected between 2005 and 2012 in Mexico: results from the tigecycline evaluation and surveillance trial. Ann. Clin. Microbiol. Antimicrob. 14:53. doi: 10.1186/s12941-015-0116-y
Moroi, H., Kimura, K., Kotani, T., Tsuda, H., Banno, H., Jin, W., et al. (2019). Isolation of group B Streptococcus with reduced β-lactam susceptibility from pregnant women. Emerg. Microbes Infect. 8, 2–7. doi: 10.1080/22221751.2018.1557987
Morozumi, M., Wajima, T., Kuwata, Y., Chiba, N., Sunaoshi, K., Sugita, K., et al. (2014). Associations between capsular serotype, multilocus sequence type, and macrolide resistance in Streptococcus agalactiae isolates from Japanese infants with invasive infections. Epidemiol. Infect. 142, 812–819. doi: 10.1017/S0950268813001647
Motallebirad, T., Fazeli, H., Azadi, D., Shokri, D., Moghim, S., and Esfahani, B. N. (2021a). Determination of capsular serotypes, antibiotic susceptibility pattern, and molecular mechanism of erythromycin resistance among clinical isolates of group B Streptococcus in Isfahan, Iran. Adv. Biomed. Res. 10:27. doi: 10.4103/abr.abr_269_20
Motallebirad, T., Fazeli, H., Ghahiri, A., Shokri, D., Jalalifar, S., Moghim, S., et al. (2021b). Prevalence, population structure, distribution of serotypes, pilus islands and resistance genes among erythromycin-resistant colonizing and invasive Streptococcus agalactiae isolates recovered from pregnant and non-pregnant women in Isfahan, Iran. BMC Microbiol. 21:139. doi: 10.1186/s12866-021-02186-2
Mousavi, S. M., Nasaj, M., Hosseini, S. M., and Arabestani, M. R. (2016). Survey of strain distribution and antibiotic resistance pattern of group B streptococci (Streptococcus agalactiae) isolated from clinical specimens. GMS Hyg. Infect. Control :Doc18:11. doi: 10.3205/dgkh000278
Mubanga, P., Steinberg, W. J., and Van Rooyen, F. C. (2015). Antimicrobial susceptibility profile of uropathogens in Maluti Adventist Hospital patients, 2011. Afr. J. Prim. Health Care Fam. Med. 7, 1–5. doi: 10.4102/phcfm.v7i1.800
Mudzana, R., Mavenyengwa, R. T., and Gudza-Mugabe, M. (2021). Analysis of virulence factors and antibiotic resistance genes in group B Streptococcus from clinical samples. BMC Infect. Dis. 21:125. doi: 10.1186/s12879-021-05820-6
Mudzikati, L., and Dramowski, A. (2015). Neonatal septicaemia: prevalence and antimicrobial susceptibility patterns of common pathogens at Princess Marina Hospital, Botswana. South. Afr. J. Infect. Dis. 30, 108–113. doi: 10.1080/23120053.2015.1074443
Mukesi, M., Iweriebor, B. C., Obi, L. C., Nwodo, U. U., Moyo, S. R., and Okoh, A. I. (2019). The activity of commercial antimicrobials, and essential oils and ethanolic extracts of Olea europaea on Streptococcus agalactiae isolated from pregnant women. BMC Complement. Altern. Med. 19:34. doi: 10.1186/s12906-019-2445-4
Mulu, W., Yimer, M., Zenebe, Y., and Abera, B. (2015). Common causes of vaginal infections and antibiotic susceptibility of aerobic bacterial isolates in women of reproductive age attending at Felegehiwot Referral Hospital, Ethiopia: a cross sectional study. BMC Womens Health 15:42. doi: 10.1186/s12905-015-0197-y
Murray, C. J. L., Ikuta, K. S., Sharara, F., Swetschinski, L., Robles Aguilar, G., Gray, A., et al. (2022). Global burden of bacterial antimicrobial resistance in 2019: a systematic analysis. Lancet 399, 629–655. doi: 10.1016/S0140-6736(21)02724-0
Muteeb, G., Rehman, M. T., Shahwan, M., and Aatif, M. (2023). Origin of antibiotics and antibiotic resistance, and their impacts on drug development: a narrative review. Pharmaceuticals 16:1615. doi: 10.3390/ph16111615
Mwambia, M. T. (2021). “Group B Streptococcus prevalence, antibiotic susceptibility and risk factors for colonization among antenatal women at Mbagathi Hospital, Nairobi City County, Kenya” in Thesis (Nairobi: Kenyatta University).
Mwei, M. K., Mchome, B., John, B., and Maro, E. (2018). Asymptomatic bacteriuria among pregnant women attending antenatal care at Kilimanjaro Christian Medical Centre in Northern Tanzania. Tanzan. J. Health Res. 20:1763931. doi: 10.4314/thrb.v20i4.8
Nabavinia, M., Khalili, M. B., Sadeh, M., Eslami, G., Vakili, M., Azartoos, N., et al. (2020). Distribution of pilus island and antibiotic resistance genes in streptococcus agalactiae obtained from vagina of pregnant women in Yazd, Iran. Iran. J. Microbiol. 12, 411–416. doi: 10.18502/ijm.v12i5.4601
Nadeau, H. C., and Edwards, R. K. (2019). Prophylaxis against early-onset group B Streptococcus infections in pregnant women who are allergic to penicillin. Clin. Obstet. Gynecol. 62, 771–780. doi: 10.1097/GRF.0000000000000455
Nagano, N., Koide, S., Hayashi, W., Taniguchi, Y., Tanaka, H., Maeyama, Y., et al. (2019). Population-level transition of capsular polysaccharide types among sequence type 1 group B Streptococcus isolates with reduced penicillin susceptibility during a long-term hospital epidemic. Int. J. Antimicrob. Agents 53, 203–210. doi: 10.1016/j.ijantimicag.2018.11.003
Newland, R., Chan, C., Chapuis, P., Keshava, A., Rickard, M., Stewart, P., et al. (2020). Relative effects of direct spread, lymph node metastasis and venous invasion in relation to blood borne distant metastasis present at the time of resection of colorectal cancer. Pathology 52, 649–656. doi: 10.1016/j.pathol.2020.06.007
Ngom, N. S., Gassama, O., Dieng, A., Diakhaby, E. B., Ndiaye, S. M. L., Tine, A., et al. (2023). Vaginal carriage of group B Streptococcus (GBS) in pregnant women, antibiotic sensitivity and associated risk factors in Dakar, Senegal. Microbiol. Insights 16:11786361231174419. doi: 10.1177/11786361231174419
Ngonzi, J., Bebell, L. M., Bazira, J., Fajardo, Y., Nyehangane, D., Boum, Y., et al. (2018). Risk factors for vaginal colonization and relationship between bacterial vaginal colonization and in-hospital outcomes in women with obstructed labor in a Ugandan regional referral hospital. Int. J. Microbiol. 2018, 1–7. doi: 10.1155/2018/6579139
Njoku, C., Emechebe, C., and Agbakwuru, A. (2017). Prevalence and determinants of anogenital colonization by group B Streptococcus infection among HIV positive and negative women in Calabar, Nigeria. Int J Women’s Health Reprod. Sci. 6, 11–17. doi: 10.15296/ijwhr.2018.04
Nkembe, N. M., Kamga, H. G., Baiye, W. A., Chafa, A. B., and Njotang, P. N. (2018). Streptococcus agalactiae prevalence and antimicrobial susceptibility pattern in vaginal and anorectal swabs of pregnant women at a tertiary hospital in Cameroon. BMC. Res. Notes 11:480. doi: 10.1186/s13104-018-3589-x
Novosak, M., Bobadilla, F., Delgado, O., Vergara, M., and Laczeski, M. (2020). Phenotypic and genotypic characterization of resistance to macrolides and lincosamides in Streptococcus agalactiae isolated from pregnant women in Misiones, Argentina. Microb. Drug Resist. 26, 1472–1481. doi: 10.1089/mdr.2019.0328
Numanović, F., Smajlović, J., Gegić, M., Delibegović, Z., Bektaš, S., Halilović, E., et al. (2017). Presence and resistance of Streptococcus agalactiae in vaginal specimens of pregnant and adult non-pregnant women and association with other aerobic bacteria. Med. Glas. 14, 98–105. doi: 10.17392/876-16
Ojo, O. O., Awonuga, D., Dedeke, I. O. F., Nwadike, V. U., Adenaya, O. R., and Odelola, O. I. (2019). Prevalence of group B Streptococcus colonisation and antimicrobial susceptibility pattern among pregnant women attending a tertiary health Facility in Ogun State, Southwest Nigeria. J. West Afr. Coll. Surg. 9, 8–14. doi: 10.4103/jwas.jwas_26_21
Oliveira, L. M. A., Simões, L. C., Costa, N. S., Zadoks, R. N., and Pinto, T. C. A. (2022). The landscape of antimicrobial resistance in the neonatal and multi-host pathogen group B Streptococcus: review from a one health perspective. Front. Microbiol. 13:943413. doi: 10.3389/fmicb.2022.943413
Palacios-Saucedo, G. D. C., Rivera-Morales, L. G., Vázquez-Guillén, J. M., Caballero-Trejo, A., Mellado-García, M. C., Flores-Flores, A. S., et al. (2022). Genomic analysis of virulence factors and antimicrobial resistance of group B Streptococcus isolated from pregnant women in northeastern Mexico. PLoS One 17:e0264273. doi: 10.1371/journal.pone.0264273
Panahi, S., Khalili, M. B., Sadeh, M., and Vakili, M. (2023). Determination of pilus-islands profile and antibiotic susceptibility of Streptococcus agalactiae isolated from urine of pregnant women. Iran. J. Microbiol. 15, 219–224. doi: 10.18502/ijm.v15i2.12473
Perim, M. C., da Costa Borges, J., Celeste, S. R. C., de Freitas Orsolin, E., Mendes, R. R., Mendes, G. O., et al. (2015). Aerobic bacterial profile and antibiotic resistance in patients with diabetic foot infections. Rev. Soc. Bras. Med. Trop. 48, 546–554. doi: 10.1590/0037-8682-0146-2015
Perme, T., Golparian, D., Bombek Ihan, M., Rojnik, A., Lučovnik, M., Kornhauser Cerar, L., et al. (2020). Genomic and phenotypic characterisation of invasive neonatal and colonising group B Streptococcus isolates from Slovenia, 2001–2018. BMC Infect. Dis. 20:958. doi: 10.1186/s12879-020-05599-y
Pfaller, M. A., Flamm, R. K., Jones, R. N., Farrell, D. J., and Mendes, R. E. (2016). Activities of tedizolid and linezolid determined by the reference broth microdilution method against 3,032 Gram-positive bacterial isolates collected in Asia-Pacific, Eastern Europe, and Latin American countries in 2014. Antimicrob. Agents Chemother. 60, 5393–5399. doi: 10.1128/AAC.00881-16
Piccinelli, G., Biscaro, V., Gargiulo, F., Caruso, A., and De Francesco, M. A. (2015). Characterization and antibiotic susceptibility of Streptococcus agalactiae isolates causing urinary tract infections. Infect. Genet. Evol. 34, 1–6. doi: 10.1016/j.meegid.2015.07.001
Piérard, D., and Stone, G. G. (2021). In vitro activity of ceftaroline and comparators against bacterial isolates collected globally from patients with skin infections. J. Glob. Antimicrob. Resist. 26, 4–10. doi: 10.1016/j.jgar.2021.04.020
Pimentel, B., Martins, C., Mendonça, J., Miranda, P., Sanches, G., Mattos-Guaraldi, A., et al. (2016). Streptococcus agalactiae infection in cancer patients: a five-year study. Eur. J. Clin. Microbiol. Infect. Dis. 35, 927–933. doi: 10.1007/s10096-016-2617-9
Proudmore, K. E., Gunathilake, M., Crawford, L. C., Freeman, K., Menouhos, D., and Baird, R. W. (2023). Group B streptococcus in the Northern Territory in 2023: clindamycin down but not out. Commun. Dis. Intell. 47:47. doi: 10.33321/cdi.2023.47.52
Qadi, M., AbuTaha, A., Al-Shehab, R., Sulaiman, S., Hamayel, A., Hussein, A., et al. (2021). Prevalence and risk factors of group B Streptococcus colonization in pregnant women: a pilot study in Palestine. Can. J. Infect. Dis. Med. Microbiol. 2021:8686550. doi: 10.1155/2021/8686550
Qiu, Y., Yang, J., Chen, Y., Yang, J., Zhu, Q., Zhu, C., et al. (2021). Microbiological profiles and antimicrobial resistance patterns of pediatric bloodstream pathogens in China, 2016–2018. Eur. J. Clin. Microbiol. Infect. Dis. 40, 739–749. doi: 10.1007/s10096-020-04069-2
Rasamiravaka, T., Batavisoa, E., Ranaivosoa, M. K., and Rasamindrakotroka, A. (2016). Profile and antimicrobial resistance to newly available drugs of urinary tract pathogens among Malagasy pregnant women. Trop. Biomed. 33, 135–140
Renteria, M., Biedenbach, D., Bouchillon, S., Hoban, D., Raghubir, N., Sajben, P., et al. (2014). In vitro activity of tigecycline against isolates collected from complicated skin and skin structure infections and intra-abdominal infections in Africa and Middle East countries: TEST 2007–2012. Diagn. Microbiol. Infect. Dis. 79, 54–59. doi: 10.1016/j.diagmicrobio.2014.01.017
Rostami, S., Moeineddini, L., Ghandehari, F., Khorasani, M. R., Shoaei, P., and Ebrahimi, N. (2021). Macrolide-resistance, capsular genotyping and associated factors of group B streptococci colonized pregnant women in Isfahan, Iran. Iran. J. Microbiol. 13, 183–189. doi: 10.18502/ijm.v13i2.5979
Saad, E. J., Baenas, D. F., Boisseau, C. S., García, M. J., Núñez, S. A., Sanchez, P. E., et al. (2018). Bacteriemia por Streptococcus agalactiae en pacientes adultos y mujeres no embarazadas de dos hospitales universitarios. Rev. Argent. Microbiol. 50, 280–284. doi: 10.1016/j.ram.2017.08.002
Sabroske, E. M., Iglesias, M. A. S., Rench, M., Moore, T., Harvey, H., Edwards, M., et al. (2023). Evolving antibiotic resistance in group B streptococci causing invasive infant disease: 1970–2021. Pediatr. Res. 93, 2067–2071. doi: 10.1038/s41390-022-02375-3
Safari, D., Gultom, S. M., Tafroji, W., Azzahidah, A., Soesanti, F., Khoeri, M. M., et al. (2021). Prevalence, serotype and antibiotic susceptibility of group B Streptococcus isolated from pregnant women in Jakarta, Indonesia. PLoS One 16:e0252328. doi: 10.1371/journal.pone.0252328
Saffar, H., Abdollahi, A., Habibi, S., and Baseri, Z. (2016). Frequency of inducible clindamycin resistance among Gram-positive cocci in a tertiary hospital, Tehran, Iran. Iran. J. Microbiol. 8, 243–248.
Sahraee, S., Milani, F., Roushan, Z. A., Ch, M. H., Rostami, S., Shoja, S., et al. (2019). The prevalence of rectovaginal colonization and antibiotic susceptibility pattern of Streptococcus agalactiae in pregnant women in Al-Zahra Hospital, Rasht, Iran. Infect. Dis. Clin. Pract. 27, 143–147. doi: 10.1097/IPC.0000000000000713
Said, M., Dangor, Y., Mbelle, N., Madhi, S. A., Kwatra, G., and Ismail, F. (2019). Antimicrobial susceptibility and serotype distribution of Streptococcus agalactiae rectovaginal colonising isolates from pregnant women at a tertiary hospital in Pretoria, South Africa: an observational descriptive study. S. Afr. Med. J. 110, 869–871. doi: 10.7196/SAMJ.2020.v110i9.14524
Santana, F. A. F., de Oliveira, T. V. L., de Souza Filho, M. B., da Silva, L. S. C., de Brito, B. B., de Melo, F. F., et al. (2020). Streptococcus agalactiae: identification methods, antimicrobial susceptibility, and resistance genes in pregnant women. World J. Clin. Cases 8, 3988–3998. doi: 10.12998/wjcc.v8.i18.3988
Sapugahawatte, D. N., Li, C., Liyanapathirana, V., Kandauda, C., Gihan, C., Zhu, C., et al. (2022). Colonization of group B Streptococcus in pregnant women and their neonates from a Sri Lankan hospital. Pathogens 11:386. doi: 10.3390/pathogens11040386
Schuab, R. B., Arêas, G. P., Souza, V. C., and Barros, R. R. (2015). Molecular epidemiology of Streptococcus agalactiae recovered from significant bacteriuria. Infect. Dis. 47, 637–642. doi: 10.3109/23744235.2015.1040446
Shabayek, S., and Abdalla, S. (2014). Macrolide-and tetracycline-resistance determinants of colonizing group B Streptococcus in women in Egypt. J. Med. Microbiol. 63, 1324–1327. doi: 10.1099/jmm.0.077057-0
Shadbad, M. A., Kafil, H. S., Rezaee, M. A., Farzami, M. R., Dehkharghani, A. D., Sadeghi, J., et al. (2020). Streptococcus agalactiae clinical isolates in Northwest Iran: antibiotic susceptibility, molecular typing, and biofilm formation. GMS Hyg. Infect. Control 15:Doc23. doi: 10.3205/dgkh000358
Shen, H., Zhu, C., Liu, X., Ma, D., Song, C., Zhou, L., et al. (2019). The etiology of acute meningitis and encephalitis syndromes in a sentinel pediatric hospital, Shenzhen, China. BMC Infect. Dis. 19:560. doi: 10.1186/s12879-019-4162-5
Shipitsyna, E., Shalepo, K., Zatsiorskaya, S., Krysanova, A., Razinkova, M., Grigoriev, A., et al. (2020). Significant shifts in the distribution of vaccine capsular polysaccharide types and rates of antimicrobial resistance of perinatal group B streptococci within the last decade in St. Petersburg, Russia. Eur. J. Clin. Microbiol. Infect. Dis. 39, 1487–1493. doi: 10.1007/s10096-020-03864-1
Shrestha, K., Sah, A. K., Singh, N., Parajuli, P., and Adhikari, R. (2020). Molecular characterization of Streptococcus agalactiae isolates from pregnant women in Kathmandu City. J. Trop. Med. 2020:4046703. doi: 10.1155/2020/4046703
Sigaúque, B., Kobayashi, M., Vubil, D., Nhacolo, A., Chaúque, A., Moaine, B., et al. (2018). Invasive bacterial disease trends and characterization of group B streptococcal isolates among young infants in southern Mozambique, 2001–2015. PLoS One 13:e0191193. doi: 10.1371/journal.pone.0191193
Simoni, S., Vincenzi, C., Brenciani, A., Morroni, G., Bagnarelli, P., Giovanetti, E., et al. (2018). Molecular characterization of Italian isolates of fluoroquinolone-resistant Streptococcus agalactiae and relationships with chloramphenicol resistance. Microb. Drug Resist. 24, 225–231. doi: 10.1089/mdr.2017.0139
Slotved, H.-C., Møller, J. K., Khalil, M. R., and Nielsen, S. Y. (2021). The serotype distribution of Streptococcus agalactiae (GBS) carriage isolates among pregnant women having risk factors for early-onset GBS disease: a comparative study with GBS causing invasive infections during the same period in Denmark. BMC Infect. Dis. 21:1129. doi: 10.1186/s12879-021-06820-2
Soares, G. C. T., Alviano, D. S., da Silva Santos, G., Alviano, C. S., Mattos-Guaraldi, A. L., and Nagao, P. E. (2013). Prevalence of group B Streptococcus serotypes III and V in pregnant women of Rio de Janeiro, Brazil. Braz. J. Microbiol. 44, 869–872. doi: 10.1590/S1517-83822013000300032
Solensky, R., Earl, H. S., and Gruchalla, R. S. (2000). Clinical approach to penicillin-allergic patients: a survey. Ann. Allergy Asthma Immunol. 84, 329–333. doi: 10.1016/S1081-1206(10)62782-2
Sterne, J. A., and Egger, M. (2005). “Regression methods to detect publication and other bias in meta-analysis” in Publication bias in meta-analysis: prevention, assessment and adjustments (Chichester: Wiley), 99–110.
Stewart, A. G., Burnard, D., Sowden, D., and McMillan, D. (2020). Whole genome sequencing for antimicrobial resistance mechanisms, virulence factors and clonality in invasive Streptococcus agalactiae blood culture isolates recovered in Australia. Pathology 52, 694–699. doi: 10.1016/j.pathol.2020.06.006
Størdal, E. H., Solevåg, A. L., Bjørnholt, J. V., Rønnestad, A., and Stensvold, H. J. (2022). Sepsis treatment options identified by 10-year study of microbial isolates and antibiotic susceptibility in a level-four neonatal intensive care unit. Acta Paediatr. 111, 519–526. doi: 10.1111/apa.16189
Suhaimi, M. E., Desa, M. N., Eskandarian, N., Pillay, S. G., Ismail, Z., Neela, V. K., et al. (2017). Characterization of a group B Streptococcus infection based on the demographics, serotypes, antimicrobial susceptibility and genotypes of selected isolates from sterile and non-sterile isolation sites in three major hospitals in Malaysia. J. Infect. Public Health 10, 14–21. doi: 10.1016/j.jiph.2016.01.009
Sulung, F., Nik Zuraina, N. M. N., Singh, K. K. B., Hussin, A., and Hassan, S. A. (2023). Serotypes, virulence and antimicrobial susceptibility of group B streptococci isolated from infections in non-pregnant adults in Malaysia. J. Taibah Univ. Sci. 17:2241706. doi: 10.1080/16583655.2023.2241706
Swann, O., Everett, D. B., Furyk, J. S., Harrison, E. M., Msukwa, M. T., Heyderman, R. S., et al. (2014). Bacterial meningitis in Malawian infants <2 months of age: etiology and susceptibility to World Health Organization first-line antibiotics. Pediatr. Infect. Dis. J. 33, 560–565. doi: 10.1097/INF.0000000000000210
Tan, J., Wang, Y., Gong, X., Li, J., Zhong, W., Shan, L., et al. (2022). Antibiotic resistance in neonates in China 2012–2019: a multicenter study. J. Microbiol. Immunol. Infect. 55, 454–462. doi: 10.1016/j.jmii.2021.05.004
Tang, Y., Yu, F., Hu, Z., Peng, L., and Jiang, Y. (2020). Characterization of aerobic vaginitis in late pregnancy in a Chinese population: a STROBE-compliant study. Medicine 99:e20732. doi: 10.1097/MD.0000000000020732
Teatero, S., Athey, T. B., Van Caeseele, P., Horsman, G., Alexander, D. C., Melano, R. G., et al. (2015a). Emergence of serotype IV group B Streptococcus adult invasive disease in Manitoba and Saskatchewan, Canada, is driven by clonal sequence type 459 strains. J. Clin. Microbiol. 53, 2919–2926. doi: 10.1128/JCM.01128-15
Teatero, S., Ferrieri, P., Martin, I., Demczuk, W., McGeer, A., and Fittipaldi, N. (2017). Serotype distribution, population structure, and antimicrobial resistance of group B Streptococcus strains recovered from colonized pregnant women. J. Clin. Microbiol. 55, 412–422. doi: 10.1128/JCM.01615-16
Teatero, S., McGeer, A., Li, A., Gomes, J., Seah, C., Demczuk, W., et al. (2015b). Population structure and antimicrobial resistance of invasive serotype IV group B Streptococcus, Toronto, Ontario, Canada. Emerg. Infect. Dis. 21, 585–591. doi: 10.3201/eid2014.140759
Terreni, M., Taccani, M., and Pregnolato, M. (2021). New antibiotics for multidrug-resistant bacterial strains: latest research developments and future perspectives. Molecules 26:2671. doi: 10.3390/molecules26092671
Tesfaye, A., Melese, A., and Derbie, A. (2022). Antimicrobial resistance profile and associated factors of group B streptococci colonization among pregnant women attending antenatal clinics in Jigjiga, Southeast Ethiopia. Int. J. Microbiol. 2022, 1–8. doi: 10.1155/2022/9910842
Tsai, M.-H., Hsu, J.-F., Lai, M.-Y., Lin, L.-C., Chu, S.-M., Huang, H.-R., et al. (2019). Molecular characteristics and antimicrobial resistance of group B Streptococcus strains causing invasive disease in neonates and adults. Front. Microbiol. 10:264. doi: 10.3389/fmicb.2019.00264
Tulyaprawat, O., Pharkjaksu, S., Shrestha, R. K., and Ngamskulrungroj, P. (2021). Emergence of multi-drug resistance and its association with uncommon serotypes of Streptococcus agalactiae isolated from non-neonatal patients in Thailand. Front. Microbiol. 12:719353. doi: 10.3389/fmicb.2021.719353
Van Du, V., Dung, P. T., Toan, N. L., Van Mao, C., Bac, N. T., Van Tong, H., et al. (2021). Antimicrobial resistance in colonizing group B Streptococcus among pregnant women from a hospital in Vietnam. Sci. Rep. 11:20845. doi: 10.1038/s41598-021-00468-3
Venkatnarayan, K., Bej, P., and Thapar, R. (2014). Neonatal Sepsis: a profile of a changing Spectrum. J. Nepal Paediatr. Soc. 34, 207–214. doi: 10.3126/jnps.v34i3.11236
Verma, S., Kumari, M., Pathak, A., Yadav, V., Johri, A. K., and Yadav, P. (2023). Antibiotic resistance, biofilm formation, and virulence genes of Streptococcus agalactiae serotypes of Indian origin. BMC Microbiol. 23:176. doi: 10.1186/s12866-023-02877-y
Viechtbauer, W. (2010). Conducting meta-analyses in R with the metafor package. J. Stat. Softw. 36, 1–48. doi: 10.18637/jss.v036.i03
Viechtbauer, W., and Cheung, M. W. (2010). Outlier and influence diagnostics for meta-analysis. Res. Synth. Methods 1, 112–125. doi: 10.1002/jrsm.11
Vuillemin, X., Hays, C., Plainvert, C., Dmytruk, N., Louis, M., Touak, G., et al. (2021). Invasive group B Streptococcus infections in non-pregnant adults: a retrospective study, France, 2007–2019. Clin. Microbiol. Infect. 27:129.e1. doi: 10.1016/j.cmi.2020.09.037
Wang, X., Cao, X., Li, S., Ou, Q., Lin, D., Yao, Z., et al. (2018). Phenotypic and molecular characterization of Streptococcus agalactiae colonized in Chinese pregnant women: predominance of ST19/III and ST17/III. Res. Microbiol. 169, 101–107. doi: 10.1016/j.resmic.2017.12.004
Wang, Y.-H., Chen, C.-L., Hou, J.-N., Wang, Y.-R., Lin, T.-Y., Wang, M.-H., et al. (2015). Serotype distribution and resistance genes associated with macrolide and fluoroquinolone resistance in Streptococcus agalactiae isolates from a hospital in southern Taiwan. Biomed. J. 38:215. doi: 10.4103/2319-4170.138306
Wang, Y.-H., Chen, H.-M., Yang, Y.-H., Yang, T.-H., Teng, C.-H., Chen, C.-L., et al. (2014). Clinical and microbiological characteristics of recurrent group B streptococcal infection among non-pregnant adults. Int. J. Infect. Dis. 26, 140–145. doi: 10.1016/j.ijid.2014.05.026
Wang, S., Li, L., Wu, B., and Wu, W. (2018). Serotype, genotype, and clinical manifestations of group B Streptococcus (GBS) isolated from neonates in China. Iran. J. Pediatr. 28:e14580. doi: 10.5812/ijp.14580
Wang, P., Ma, Z., Tong, J., Zhao, R., Shi, W., Yu, S., et al. (2015a). Serotype distribution, antimicrobial resistance, and molecular characterization of invasive group B Streptococcus isolates recovered from Chinese neonates. Int. J. Infect. Dis. 37, 115–118. doi: 10.1016/j.ijid.2015.06.019
Wang, P., Tong, J. J., Ma, X. H., Song, F. L., Fan, L., Guo, C. M., et al. (2015b). Serotypes, antibiotic susceptibilities, and multi-locus sequence type profiles of Streptococcus agalactiae isolates circulating in Beijing, China. PLoS One 10:e0120035. doi: 10.1371/journal.pone.0120035
Warrier, L. M., Joy, S., and Bashir, R. A. (2022). Group B streptococcal colonization among pregnant women and neonates in a tertiary care hospital in South India. Indian J. Pediatr. 89, 1187–1194. doi: 10.1007/s12098-022-04120-4
Wataradee, S., Samngamnim, S., Boonserm, T., and Ajariyakhajorn, K. (2023). Genotypic and antimicrobial susceptibility of Streptococcus agalactiae causing bovine mastitis in the central region of Thailand. Front. Vet. Sci. 10:1250436. doi: 10.3389/fvets.2023.1250436
Wilkie, G. L., Prabhu, M., Ona, S., Easter, S. R., Tuomala, R. E., Riley, L. E., et al. (2019). Microbiology and antibiotic resistance in peripartum bacteremia. Obstet. Gynecol. 133, 269–275. doi: 10.1097/AOG.0000000000003055
Williams, A. N., Croxen, M. A., Demczuk, W. H., Martin, I., and Tyrrell, G. J. (2023). Genomic characterization of emerging invasive Streptococcus agalactiae serotype VIII in Alberta, Canada. Eur. J. Clin. Microbiol. Infect. Dis. 42, 747–757. doi: 10.1007/s10096-023-04606-9
Woldu, Z. L., Teklehaimanot, T. G., Waji, S. T., and Gebremariam, M. Y. (2014). The prevalence of group B streptococus recto-vaginal colonization and antimicrobial susceptibility pattern in pregnant mothers at two hospitals of Addis Ababa, Ethiopia. Reprod. Health 11:80. doi: 10.1186/1742-4755-11-80
Wu, B., Su, J., Li, L., Wu, W., Wu, J., Lu, Y., et al. (2019). Phenotypic and genetic differences among group B Streptococcus recovered from neonates and pregnant women in Shenzhen, China: 8-year study. BMC Microbiol. 19:185. doi: 10.1186/s12866-019-1551-2
Yan, Y., Hu, H., Lu, T., Fan, H., Hu, Y., Li, G., et al. (2016). Investigation of serotype distribution and resistance genes profile in group B Streptococcus isolated from pregnant women: a Chinese multicenter cohort study. APMIS 124, 794–799. doi: 10.1111/apm.12570
Yang, Y., Xie, S., He, F., Xu, Y., Wang, Z., Ihsan, A., et al. (2024). Recent development and fighting strategies for lincosamide antibiotic resistance. Clin. Microbiol. Rev. 37:e0016123. doi: 10.1128/cmr.00161-23
Yayan, J., Ghebremedhin, B., and Rasche, K. (2015). No resistance to penicillin, cefuroxime, cefotaxime, or vancomycin in Pneumococcal pneumonia. Int. J. Med. Sci. 12, 980–986. doi: 10.7150/ijms.13203
Yong, T. B., Hashim, R., Noor, A. M., Hamzah, S. H., and Ahmad, N. (2015). Identification of Brucella spp. isolated from human brucellosis in Malaysia using high-resolution melt (HRM) analysis. Diagn. Microbiol. Infect. Dis. 81, 227–233. doi: 10.1016/j.diagmicrobio.2014.12.012
Yoon, I. A., Jo, D. S., Cho, E. Y., Choi, E. H., Lee, H. J., and Lee, H. (2015). Clinical significance of serotype V among infants with invasive group B streptococcal infections in South Korea. Int. J. Infect. Dis. 38, 136–140. doi: 10.1016/j.ijid.2015.05.017
Yu, Y., Huang, Q., and Liu, A. (2021). Analysis of pathogens, drug resistance, sensitive antibiotic treatment and risk factors of early-onset sepsis in very low birth weight infants. Am. J. Transl. Res. 13, 12939–12948
Zakerifar, M., Goli, H. R., Kaboosi, H., Rahmani, Z., and Peyravii Ghadikolaii, F. (2023). Antibiotic resistance genes and molecular typing of Streptococcus agalactiae isolated from pregnant women. BMC Pregnancy Childbirth 23:43. doi: 10.1186/s12884-023-05380-4
Zeng, S., Tang, X., Zhao, W., Qiu, H., Wang, H., and Feng, Z. (2016). Clinical analysis of cases of neonatal Streptococcus agalactiae sepsis. Genet. Mol. Res. 15:15027962. doi: 10.4238/gmr.15027962
Zhang, L., Kang, W.-J., Zhu, L., Xu, L.-J., Guo, C., Zhang, X.-H., et al. (2021). Emergence of invasive serotype Ib sequence type 10 group B Streptococcus disease in Chinese infants is driven by a tetracycline-sensitive clone. Front. Cell. Infect. Microbiol. 11:642455. doi: 10.3389/fcimb.2021.642455
Zhang, X., Li, Y., Tao, Y., Ding, Y., Shao, X., and Li, W. (2022). Epidemiology and drug resistance of neonatal bloodstream infection pathogens in East China children’s medical center from 2016 to 2020. Front. Microbiol. 13:820577. doi: 10.3389/fmicb.2022.820577
Zhang, X., Tan, L., Ouyang, P., Ma, H., Peng, J., Shi, T., et al. (2023). Analysis of distribution and antibiotic resistance of Gram-positive bacteria isolated from a tertiary-care hospital in southern China: an 8-year retrospective study. Front. Microbiol. 14:1220363. doi: 10.3389/fmicb.2023.1220363
Zhang, J., Zhao, R., Dong, Y., and Zheng, Y. (2015). Invasive group B streptococcal infection in infants in Shenzhen, China. Int. J. Clin. Exp. Med. 8, 2939–2943.
Zhou, Y., Wang, L.-Q., Yan, Q., Lee, C.-C., Hsu, M.-H., Liao, W.-T., et al. (2022). Genomic analysis of group B Streptococcus from neonatal sepsis reveals clonal CC17 expansion and virulence-and resistance-associated traits after intrapartum antibiotic prophylaxis. Clin. Infect. Dis. 75, 2153–2160. doi: 10.1093/cid/ciac331
Keywords: antibiotic resistance, group B Streptococcus, meta-analysis, antibiotic susceptibility, Joanna Briggs Institute tool
Citation: Hsu C-Y, Moradkasani S, Suliman M, Uthirapathy S, Zwamel AH, Hjazi A, Vashishth R and Beig M (2025) Global patterns of antibiotic resistance in group B Streptococcus: a systematic review and meta-analysis. Front. Microbiol. 16:1541524. doi: 10.3389/fmicb.2025.1541524
Edited by:
Piotr Majewski, Medical University of Bialystok, PolandReviewed by:
Prabin Dawadi, University of Mississippi, United StatesDalia Hamza, Cairo University, Egypt
Copyright © 2025 Hsu, Moradkasani, Suliman, Uthirapathy, Zwamel, Hjazi, Vashishth and Beig. This is an open-access article distributed under the terms of the Creative Commons Attribution License (CC BY). The use, distribution or reproduction in other forums is permitted, provided the original author(s) and the copyright owner(s) are credited and that the original publication in this journal is cited, in accordance with accepted academic practice. No use, distribution or reproduction is permitted which does not comply with these terms.
*Correspondence: Masoumeh Beig, YmVpZ21hc291bWVoQGdtYWlsLmNvbQ==